- 1Fishery College, Guangdong Ocean University, Zhanjiang, China
- 2Southern Marine Science and Engineering Guangdong Laboratory (Zhanjiang), Zhanjiang, China
- 3Guangdong Provincial Key Laboratory of Pathogenic Biology and Epidemiology for Aquatic Economic Animals, Zhanjiang, China
- 4Guangdong Evergreen Feed Industry Co. Ltd, Zhanjiang, China
- 5Hainan Blue Grain Technology Co., Ltd., Sanya, China
An estimation of the utilization of endogenous nutrients from the yolk sac that occurs during embryonic and early larval development can be a valuable approach to studying the nutritional requirements of fish larvae. In this study, fertilized eggs, embryos (before the membrane, 24 hours after fertilization), newly-hatched larvae (0-d-old), and open-mouthed larvae (3-day-old, unfed rotifers) samples of cobia (Rachycentron canadum) were collected to determine the amino acid and fatty acid profiles. Crude protein composition varies significantly for cobia during the different stages of development. The total amino acids, except for lysine(Lys), histidine (His), methionine(Met), serine(Ser), and glycine(Gly), had no significant change in the early development stage (P > 0.05); the content of other six essential amino acids (EAA) and six non-essential amino acids (NEAA) was consistent with the changing trend of total essential amino acid. From hatching newly hatched larvae to open-mouthed larvae, except for Leu, Phe and Ser, decreased significantly (P<0.05). From the utilization rate of FAA, the utilization rate of Lys and Leu was the highest (60.26%) and (52.21%) in the embryonic and endogenous nutritional stages, respectively. The water content of the open-mouthed larvae of cobia was significantly higher than that of the fertilized eggs, embryos, and newly hatched larvae (P < 0.05). Three kinds of saturated fatty acids (SFA), five kinds of monounsaturated fatty acids (MUFA), and eight kinds of polyunsaturated fatty acids (PUFA) were detected in the dry samples of each developmental stage. Based on these results, juvenile cobia can thrive with a wide range of crude protein concentrations. Further refinement of commercial cobia production feeds would have beneficial consequences for economic and environmental concerns due to the positive impacts of optimizing the protein component as well as the ability to maintain the rapid growth rates. The results show that the larval development of cobia needs to consume large amounts of n3PUFA (especially DHA and EPA) to improve the larvae’s survival rate. Research methods will always have limitations when evaluating the relationship between early life nutrition interventions and well-being outcomes later in life. This study provides essential information regarding cobia larvae’s fatty acid requirements.
Introduction
The nutritional physiology of fish embryos and larvae is one of the frontier fields of fish nutrition research. The nutrition required for the development stage of fish embryos and early larvae mainly comes from the endogenous substances stored in the yolk. Its main components (protein and amino acids, fats and fatty acids, carbohydrates, etc.) and proportion are the material basis for the development of fish embryos and early larvae, which played an important role in the development and survival rate of larvae and juveniles in the subsequent mixed nutrition stage and exogenous nutrition stage (Hastey et al., 2010; Tong et al., 2017). Studies have shown that the consumption sequence of endogenous nutritional components was helpful in determining the nutritional demand of open larvae, which directly affected the metabolic capacity of larvae development (Lubzens et al., 2010; Tong et al., 2017). After the yolk of larvae was exhausted, they entered the exogenous nutrition stage, and the demand for amino acids and fatty acids during the development of larvae and juveniles was also different (Mansour et al., 2011; Jia et al., 2014).
As the main nutrients during the embryonic and post-embryonic development of fish (especially during endogenous nutrition), lipids were not only involved in regulating physiological activities and constructing tissues and organs but also the main sources of energy (Xu et al., 2013). Therefore, the composition and content of lipids and fatty acids play a crucial role in the growth and survival of fish in the early stage of development (Jia et al., 2014), especially the n-3 series of highly unsaturated fatty acids were essential fatty acids for post-embryonic development of marine fish, such as docosahexaenoic acid (DHA) and eicosapentaenoic acid (EPA) (Wang et al., 2006). At the same time, the composition and content of fatty acids varied with fish species (Shi et al., 2010; Huang et al., 2013). Rainuzzo et al. (1997) believed that for larvae starting exogenous nutrition, the ideal fatty acid composition in the diet should be similar to that of fertilized eggs or yolk-sac larvae. Evaluating the utilization of endogenous yolk-sac nutrients during embryonic and early larval development was an effective method of studying the nutritional requirements of larvae (Lanes et al., 2012; Huang et al., 2020). Therefore, the study on the changes in lipid and fatty acid characteristics during the embryonic stage of marine fish and the development of yolk-sac larvae is helpful in understanding the requirements characteristics of dietary fatty acids in the early larvae of marine fish.
Cobia (Rachycentron canadum) is a great potential species for aquaculture. It plays an important role in China’s southern coastal marine cage culture industry (Huang et al., 2020; Amenyogbe et al., 2021; Huang et al., 2021; Zhang et al., 2021; Amenyogbe et al., 2022; Huang et al., 2022). Although the artificial breeding technology of cobia has continued to improve, in the actual process of cobia fry cultivation, the survival rate of cobia larvae was lower during the open-mouth larvae stage due to the short transition time from endogenous nutrition to exogenous nutrition. In spite of great commercial interest, there is limited information about the biology, nutritional requirements, or techniques for intensive rearing of larvae of this species. Cobia’s nutritional requirements and metabolic characteristics during early development had not been reported. Also, limited information is currently available about the amino acid (AA) and fatty acid (FA) composition of eggs and larvae of cobia. A comprehensive understanding of the metabolic changes of AA and FA during the initial development of this species, as well as its nutritional needs, would be a priceless resource. Therefore, this study adopted biochemical methods to detect and analyze the composition and content of amino acids and fatty acids in the early developmental stages of cobia and compared the actual utilization of amino acids and fatty acids in the embryonic and endogenous nutritional stages of larvae. The purpose is to comprehensively grasp the demand characteristics of amino acids and fatty acids in the early stage of cobia, reveal the causes of high mortality in the open-mouth larvae stage of cobia, and provide a scientific basis for the breeding technology of cobia fry.
Materials and methods
Experimental materials
The fertilized eggs, embryos, and larvae of experimental cobia were taken from the Sanya breeding base of Hainan Blue Grain Technology Co., Ltd. in April 2019 in China. Embryos and larvae were obtained by artificial breeding. On March 1, 2019, self-raised 3-year-old adult cobia fish were selected from seawater cages(4m×4m×8m) as parent fishes (6 males, weighing 9kg-12kg; 6 females, weighing 10-14kg). The parental cultivation and breeding management were carried out in the same size cages. Refer to the literature for specific methods (Huang et al., 2019; Kuang et al., 2021). The chilled squid was used for the breeding of breeding parents and for intensive breeding. At 6 pm on April 11, the broodstock was anesthetized with MS-222 (100mg/L) and then were induced to spawn by injecting LRH-A3 and HCG oxytocin. The female fish was injected with LRH-A (8 μg/kg) and HCG (1 200 IU/kg), and the male fish was injected with 1/2 of the female fish. The oxytocin effect time was 12 h. At 6:00 am on April 12, the fertilized eggs were obtained by pulling the net in the cage with an 80-mesh sieve. Then, it was transferred to a circular incubator tank (100 L), and after removing the unfertilized eggs, high-quality fertilized eggs were obtained (the fertilization rate reached 98%). Then, took about 100,000 fertilized eggs and transferred them to two circular incubator tanks (100 L) for hatching, with a density of about 500 ind/L. Placed a heating rod in the incubator tank to keep the water temperature as stable as the possible and controlled temperature at (28 ± 1) °C. Each incubation tank was aerated continuously, the water was changed twice a day, and 1/2 of the water in the incubation tank was changed daily. Sampling: the samples were collected according to different developmental stages, including fertilized eggs, embryonic stage (before membrane emergence, 24 h after fertilization), newly-hatched larvae (0-day-old), and open-mouthed larvae (3-day-old, without fed rotifers). The developmental stages of sampling are shown in Figure 1. Four samples at the early development stage were sampled randomly. Each group of samples was divided into three parallels. Each parallel sample was sampled with a fresh weight of 8 g. The obtained samples were spread on an 80-mesh sieve, and the surface moisture was absorbed by qualitative filter paper and then stored in a 5 ml sealed centrifuge tube. The prepared samples were frozen in the refrigerator at -20°C for 24 h, then transferred and stored at -80°C (Faulk and Holt, 2008). During the measurement, the samples were vacuum freeze-dried to constant weight, milled, and mixed to be tested.
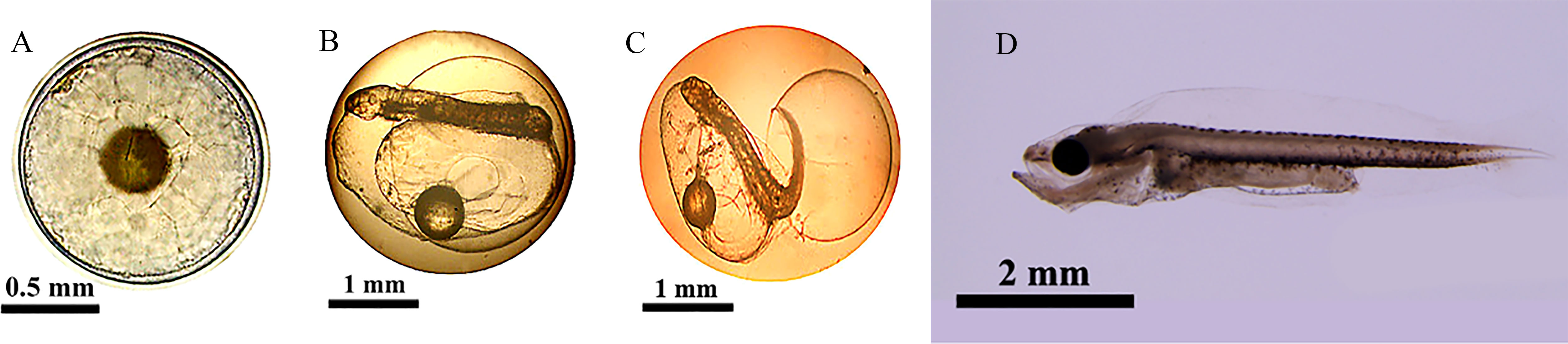
Figure 1 Different developmental stages of cobia sampled in this experiment (Kuang et al., 2021) (A) fertilized eggs, (B) embryonic stage, (C) newly-hatched larvae stage, (D) open-mouthed larvae stage. Fertilized eggs: The fertilized egg was round, colorless, and translucent, with one oil ball, small periegg space, egg diameter of 1.2 mm, and oil ball diameter of 0.3mm. The fertilized egg floated on the water surface. It presented the state that the animal pole faced down and the plant pole faced up due to a large oil ball at the plant pole. Embryonic stage: 24 hours after fertilization, the embryo body coiled in the egg membrane and circled around the yolk, and the caudal fin differentiation was apparent. The heart beats continuously, with a frequency of about 185 times/min, and blood flow can be observed. The tail twisted violently, causing the embryo body to rotate frequently in the egg membrane. At 25 h and 30 min after fertilization, the egg membrane near the head gradually bulged with the strengthening of the swing of the tail of the embryo. Newly-hatched larvae stage: The total length was 2.0 mm, about 26 hours after fertilization; under the violent swing of the tail, the larvae were basically separated from the egg membrane, and the process of membrane emergence lasted for about 30 minutes. Open-mouthed larvae stage: The total length was 4.4mm, the length diameter of the yolk-sac was 0.3mm, and the diameter of the oil ball was 0.2mm. The intestinal peristalsis was intensified, the mouth cleft was frequently opened and closed, the eye sac was black, the pectoral fin was enlarged, which can be freely condensed and opened, and the back of the anal fin membrane had a brown pigment plexus.
Experimental method
The moisture in the samples was determined by the freeze-drying method, and total lipids were extracted by the chloroform-methanol method.
About 30 mg of the ground sample was weighed and placed in a hydrolysis tube, 6 mol/L hydrochloric acids were added, and the tube was sealed and hydrolyzed at 110°C for 24h. After cooling, the hydrolyzate was transferred to a 25 mL volumetric flask and filtered after constant volume. Draw 1mL of the filtrate, place it in a vacuum desiccator to dry, dissolve the residue in 2mL of deionized water and evaporated to dryness, repeated 1-2 times, and finally add pH 2.2 buffer to dissolve. Measured with Hitachi 835-50 automatic analyzer. The specific determination referred to the standard GB/T 18654.11-2008. Tryptophan was not detected because it was destroyed during acid hydrolysis in the determination process.
Free amino acid analysis was carried out by DABS-Cl Pre-column Derivatization-high performance liquid chromatography. The specific operation steps were as follows: weighed 10.0-20.0mg protein equivalent sample into a 25mL colorimetric tube, added water to the volume, and then extracted by ultrasonic. Aspirated 3.0mL of constant volume solution and added 3.0mL of 60g/L sulfosalicylic acid, centrifuged for 15min, aspirated 2.0mL of supernatant, added 1.0mol/L sodium hydroxide solution to adjust the pH to neutral and added water to constant volume to 5.0mL. Then, 30μL of 0.1mol/L sodium bicarbonate solution and 60μL of Sigma DABS-Cl derivatization solution were added in sequence, and the mixture was thoroughly mixed in an EOFO-944621 vortex mixer and then derivatized in a 70°C water bath, and then filtered through a 0.45μm filter membrane. Waters E2695 high-performance liquid chromatograph with UV detector was used for detection. Chromatographic conditions: the liquid chromatography column was MGC18, the mobile phase was 12mol/L citric acid solution and 30%A+70% acetonitrile (containing 4% N, N-dimethylformamide), and the flow rate was 1.4mL/min, the column temperature was 35°C, and the wavelength was 436 nm.
The samples for fatty acid determination were extracted by the chloroform-methanol method in fatty acid analysis (Folch et al., 1957), and the fatty acids were analyzed by gas chromatography (Zhao et al., 2017; Li et al., 2018). The operation steps were as follows: weighed 0.1g of the sample into a 50mL digestion tube, added 2mL of chromatographically pure n-hexane, 3mL of formyl chloride, in turn, methylate in water bathed at 70°C for 2h, and then added 5mL of 6% K2CO3 and 2mL of n-hexane. Then, it was thoroughly shaken and centrifuged, and the supernatant was taken for gas chromatography analysis. The flame ionization detector (FID) of Shimadzu GC-2010 high-performance gas chromatograph was used for detection. Chromatographic conditions: the capillary column was Supelco SP-2560, the size was 100m×0.25mm× 0.20μm, the temperature of the injection port and the detector was 260°C, the carrier gas was high-purity N2, the column flow rate was 1.8mL/min, and the pre-column pressure 357.4 kPa, the column temperature heating program was the initial temperature of 140°C and constant temperature for 5min, increased to 240°C at 4°C/min and constant temperature for 10 min, and the split injection was 1.0 μL, and the split ratio was 90:1. Fatty acids were identified through the mixed standard comparison of 37 fatty acid methyl esters in Supelco, and the normalization of chromatographic peak area counted the relative percentage of fatty acids.
Data processing and analysis
Data were presented as mean ± standard deviation (x ± SD). The experimental data were analyzed by one-way ANOVA with SPSS 19.0 software. If the statistical data were significantly different, Duncan’s method was used for multiple comparison tests, and the significance level was set at 0.05. Homogeneity of variance tests was performed before data analysis.
Amino acid utilization in the embryonic stage (%):
Amino acid utilization in the endogenous nutritional stage of larvae (%):
In the formula, Wu、We、Wn, and Wb were the amino acid content of fertilized eggs, the embryonic stage, newly-hatched larvae stage, and open-mouthed larvae, respectively (mg/g DW)
The actual content of each fatty acid in unit dry matter (mg/g DW):
Fatty acid utilization in the embryonic stage (mg/g DW):
Fatty acid utilization in the embryonic stage (%):
Fatty acid utilization in the endogenous nutritional stage of larvae (mg/g DW):
Fatty acid utilization in the endogenous nutritional stage of larvae (%):
Where Tl was the content of total fat in unit dry matter (mg/g DW), and Cl was the percentage content (%) of each fatty acid, DW was dry weight. Mu、Me、, Mn and Mb were the actual content of fatty acids (mg/g DW) in fertilized eggs, embryos, newly- hatched larvae, and open-mouthed larvae, respectively.
Result
Amino acid composition and changes
The amino acid contents of fertilized eggs, the embryonic stage, the newly-hatched larvae, and open-mouthed larvae were measured in Table 1, including 9 essential amino acids (EAA) and 8 kinds of non-essential amino acids (NEAA). Among the EAA, except for Lys, His, and Met, which had no significant changes in the early developmental stage (P>0.05), the content change trend of the other six EAA was consistent with the changing trend of total essential amino acids, which the content decreased significantly from fertilized eggs to the open-mouthed larvae stage (P < 0.05). The content of Leu and Lys in essential amino acids was the highest at each developmental stage. Among the NEAA, except for Ser and Gly, which had no significant changes in the early developmental stage (P>0.05), the contents of other NEAA also decreased significantly (P<0.05) from fertilized eggs to the open-mouthed larvae stage. The contents of EAA and NEAA in fertilized eggs were 248.72mg/g dry weight and 232.91mg/g dry weight, respectively. The contents of EAA and NEAA in the open-mouthed larvae stage were 195.33mg/g dry weight and 193.44mg/g dry weight, respectively. In terms of utilization of EAA, the utilization rate of Ile was the highest (18.12%) in the embryonic stage, followed by Phe (13.52%) and Leu (9.85%). In the endogenous nutritional stage of larvae, the utilization rate of Phe was the highest (25.45%), followed by Thr (15.31%) and Arg (13.59%) (Figure 2A).
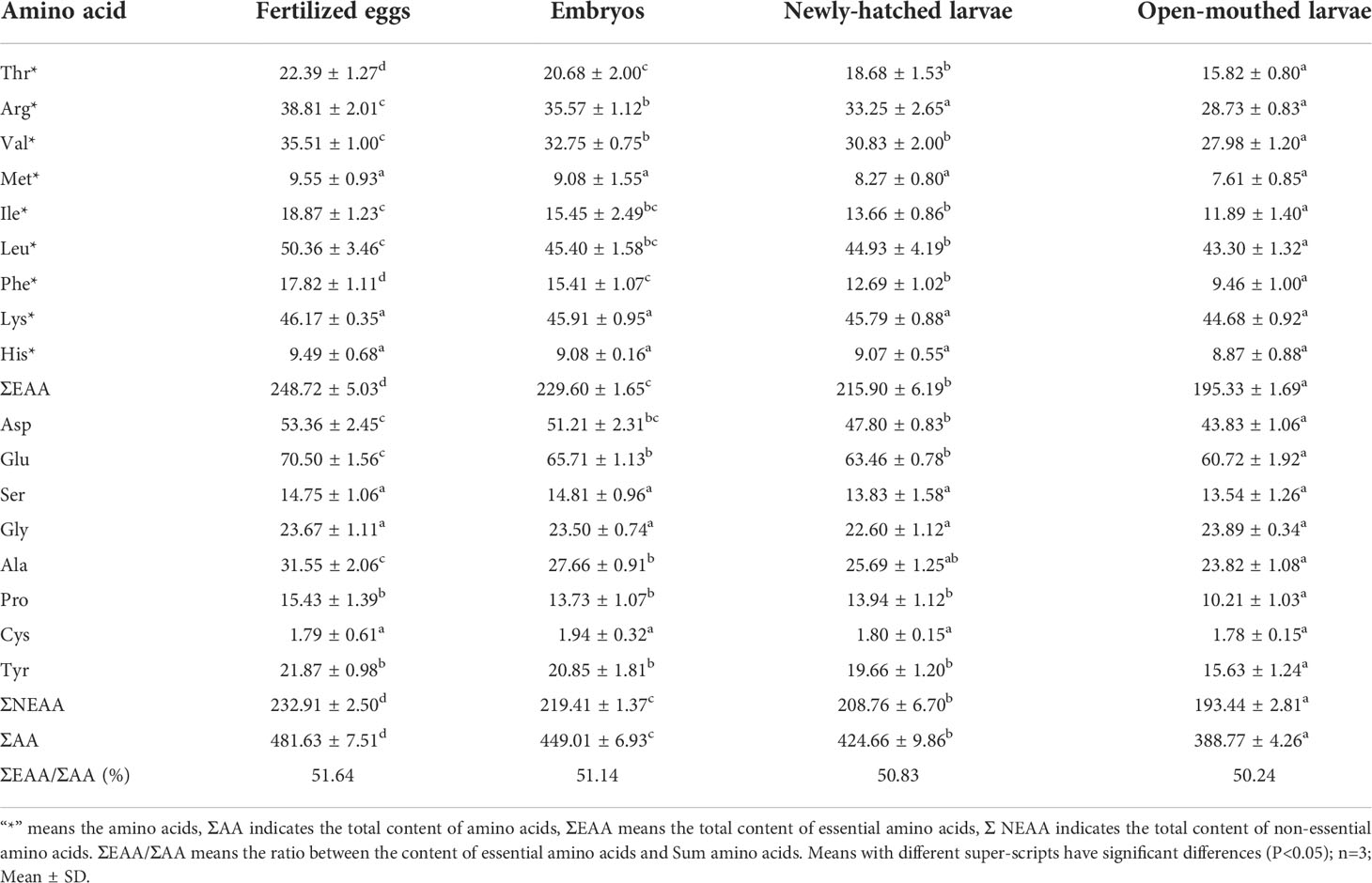
Table 1 Changes in the total content of amino acid for cobia in early development stages (mg/g dry weight).
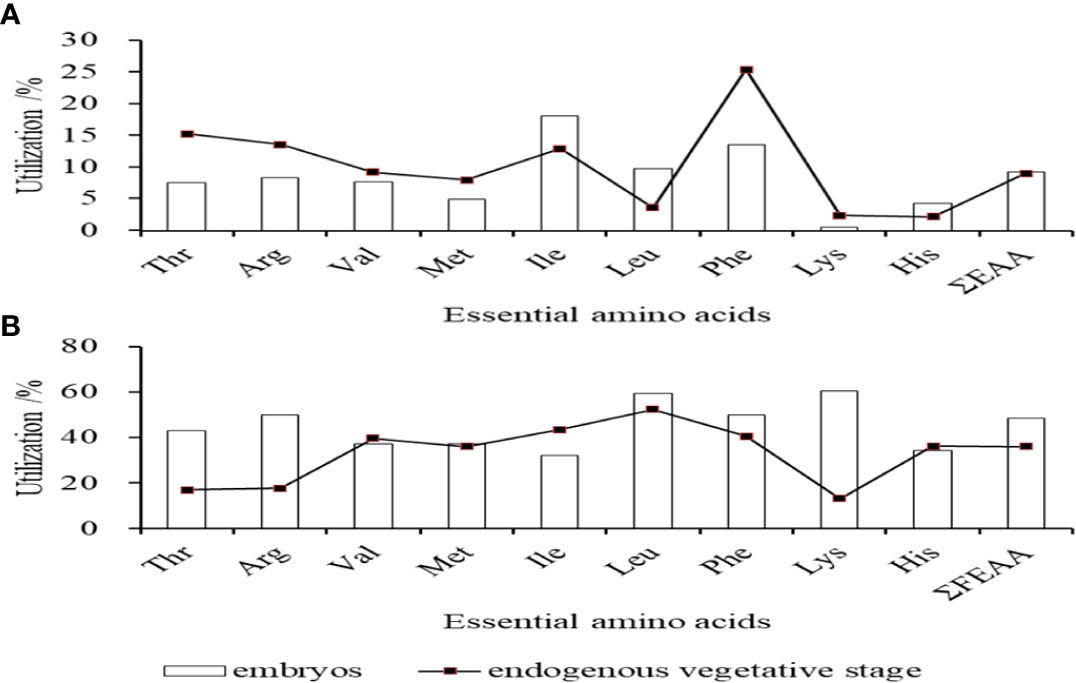
Figure 2 Utilization of essential amino acids in embryos and endogenous vegetative stages of cobia (A) in total amino acids; (B) in free amino acids.
The contents of free amino acids (FAA) at each stage, from fertilized egg development to open-mouthed larvae, were listed in Table 2. It can be seen that the change in FAA content in each developmental stage was much greater than that of total amino acid content. From fertilized eggs to open-mouthed larvae, the content of free essential amino acids (FEAA) decreased more than free non-essential amino acids (FNEAA), from 91.45 mg/g to 10.18 mg/g and 54.73 mg/g to 7.27 mg/g, respectively. The content of each FAA was lower in the embryonic, newly-hatched larvae stage and before feeding larvae than in fertilized eggs, and there was a significant difference between them (P<0.05). After hatching (newly-hatched larvae stage to open-mouthed larvae stage), except for Leu, Phe and Ser decreased significantly (P<0.05), and the content of other FAA decreased, but there was no significant difference (P > 0.05). From the utilization rate of FAA, the utilization rate of Lys was the highest (60.26%) in the embryonic stage, followed by Leu (59.30%) and Arg (50.00%). In the endogenous nutritional stage of larvae, the utilization rate of Leu was the highest (52.21%), followed by Phe (40.41%) and Val (39.63%) (Figure 2B).
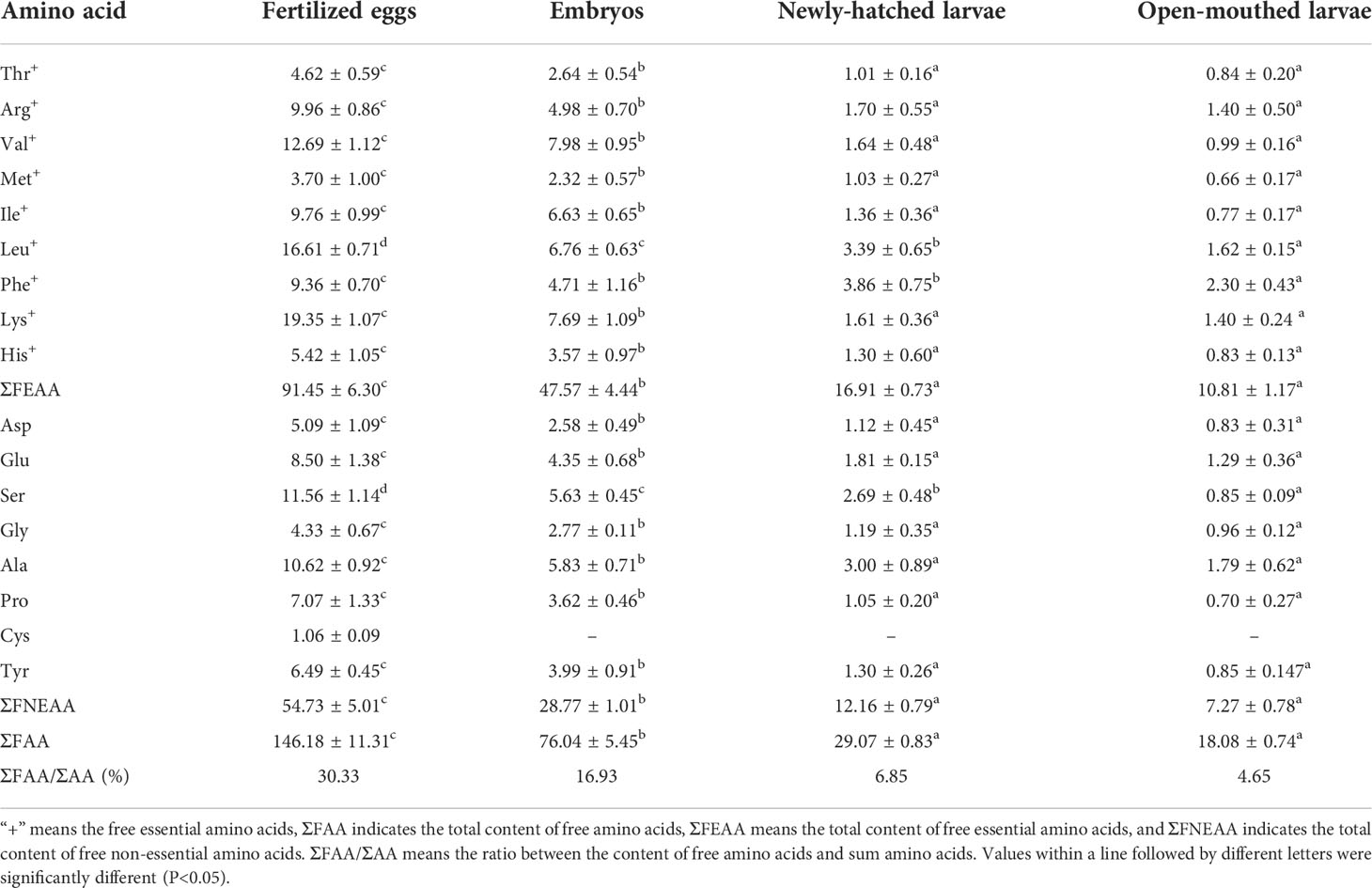
Table 2 Changes in the total content of free amino acid for cobia in early development stages (mg/g dry weight).
Moisture and total lipid content
The water content of open-mouthed larvae of cobia was significantly higher than that of fertilized eggs, embryos, and newly-hatched larvae (P<0.05), but there was no significant difference between the fertilized eggs, embryos, and newly-hatched larvae (P>0.05). Moisture content increased with individual development (Table 3). The total lipid content of fertilized eggs was 224.16mg/g (dry weight), which was significantly higher than that of the embryos stage (200.59 mg/g) (P<0.05), but both were significantly lower than those of newly-hatched larvae (301.36mg/g) (P<0.05). After hatching, with individual development, the total lipid content of fry decreased significantly from 301.36mg/g in newly-hatched larvae to 55.87mg/g in open-mouthed larvae (P<0.05) (Table 3).
Fatty acid composition and changes
A total of 16 kinds of fatty acids with a carbon chain length of C14 ~ C22 were detected in the dry samples in the early development stages of cobia, including 3 kinds of saturated fatty acids (SFA), 5 kinds of monounsaturated fatty acids (MUFA) and 8 kinds of polyunsaturated fatty acids (PUFA) (Table 4).
The percentage content of C18:1n9c in the total fatty acids in the early developmental stages of cobia was the highest (21.97%-23.91%), and the content was stable without significant difference (P>0.05) (Table 4). The percentage of C16:0 was abundant in each developmental stage and showed a significant downward trend with individual development (P<0.05), and its value decreased from 21.78% to 15.82%. The percentage of C18:0 had no significant difference among different developmental stages (P>0.05), and its value ranged from 7.94% to 9.25%. The percentage of C16:1 showed a slight downward trend with individual development (P>0.05), and its value decreased from 6.61% in fertilized eggs to 6.02% in the open-mouthed larvae stage.
During the early development of cobia, the percentage of C22:6n3 (DHA) increased significantly with individual development (P<0.05), and the value increased from 17.92% to 24.64% (Table 4). The percentage of C20:5n3 (EPA) in newly-hatched larvae and open-mouthed larvae was significantly higher than that in fertilized egg stage (P<0.05). With individual development, the change of DHA+EPA was similar to that of DHA. The percentage of DHA+EPA increased significantly from fertilized eggs and embryos to newly-hatched larvae (P<0.05), and the value increased from 21.39% to 29.27%; There was no significant difference in DHA+EPA content with newly-hatched larvae (P>0.05) (Table 4).
From fertilized eggs to open-mouthed larvae, the proportion of SFA in fatty acid composition showed a significant downward trend with individual development (P<0.05), and its value decreased from 32.71% to 24.66%. There was no significant difference in the proportion of MUFA among different developmental stages (P>0.05), and its value ranged from 29.54% to 32.24%. On the contrary, the proportion of PUFA increased significantly with individual development (P<0.05), and its value increased from 35.06% to 45.80% (Table 4).
Changes in the actual content of major fatty acids
Among the main fatty acids in fertilized eggs and embryos of cobia, the actual contents of the top three were C16:0, C18:1n9c, and C18:0. From fertilized eggs to newly-hatched larvae, the actual content of C18:3n3 decreased slightly (P>0.05), and the actual content of other single fatty acids decreased significantly (P<0.05). From newly-hatched larvae to open-mouthed larvae, the actual contents of C16:0, C22:5n3 (DPA), and C22:6n3(DHA) decreased significantly with individual development (P<0.05), and the actual contents of other individual amino acids decreased slightly (P>0.05), which also led to a significant decrease in the actual content of SFA and PUFA with larvae development (P<0.05) (Table 5).
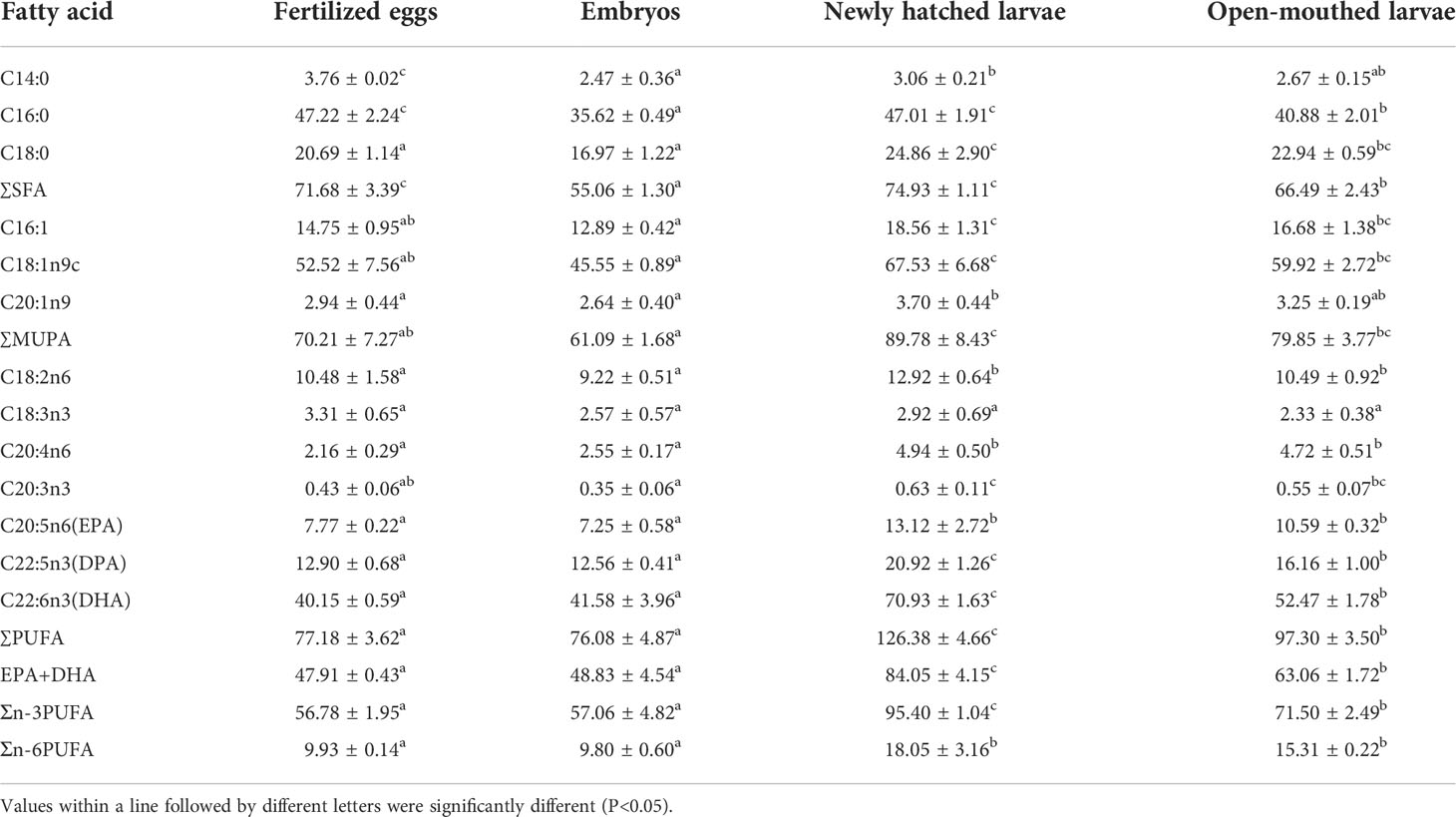
Table 5 The actual content of the main fatty acid of cobia in different development stages n=3, mg/g dry weight.
Utilization of main fatty acids in the embryo and endogenous nutritional stage
The highest actual utilization of single fatty acid in embryos stage was C16:0 (11.60 mg/g), followed by C18:1n9c (6.97 mg/g) and C18:0 (3.72 mg/g) (Figure 3A). In terms of utilization rate, C14:0 was the highest (34.45%), followed by C16:0 (24.56%), C18:3n3 (22.28%) and C18:0 (17.98%). The actual utilization of C20:4n-6 (ARA) and C22:6n-3 (DHA) in embryos was very low, and the data monitoring results showed negative values (Figure 1). In terms of SFA, MUFA, and PUFA, the highest utilization rate in embryos was SFA (16.62 mg/g), and the highest utilization rate was also SFA (23.19%).
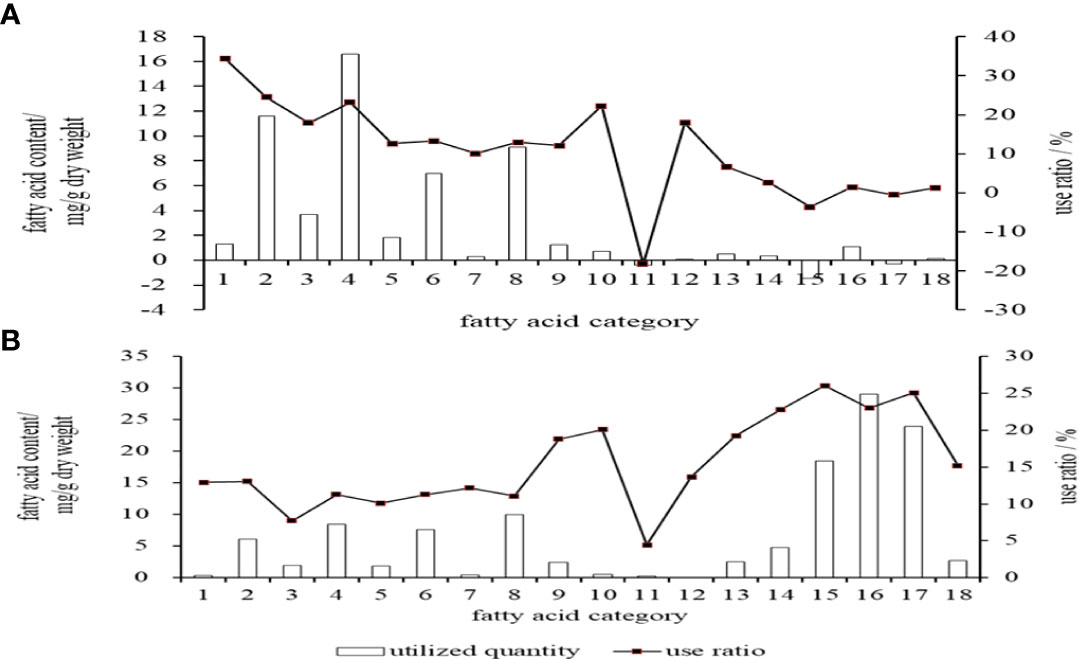
Figure 3 Utilization degree of the main fatty acids of cobia. (A: in embryos stage; B: in endogenous vegetative larvae stage). 1.C14:0, 2.C16:0, 3.C18:0, 4. ∑SFA 5.C16:1, 6.C18:1n9c, 7.C20:1n9, 8.∑MUPA, 9.C18:2n6, 10.C18:3n3, 11.C20:4n6, 12.C20:3n3, 13.C20:5n-6(EPA), 14.C22:5n3 (DPA), 15.C22:6n3(DHA), 16.∑PUFA,17. ∑n-3PUFA, 18. ∑n-6PUFA.
In the endogenous nutrition larvae stage, the highest actual utilization of single fatty acid was C22:6n3 (DHA) (18.46mg/g), followed by C16:0 (6.14mg/g) and DPA (4.77mg/g) (Figure 3B). In terms of utilization rate, DHA was the highest (26.03%), followed by C22:5n-3(DPA) (22.78%) and C20:5n-6(EPA) (19.26%). In terms of SFA, MUFA, and PUFA, PUFA had the highest utilization and utilization rate, which were 29.08 mg/g and 23.01%, respectively (Figure 3B).
Discussion
Composition and variation of total amino acids and free amino acids in early developmental stages of cobia
The yolk is the source of all the nutrients required for cell differentiation, organ development, and growth during embryogenesis (Tocher, 2010; Costa et al., 2018). Therefore, fish eggs should contain all the essential nutrients required for embryonic development and larval growth (Anggo et al., 2015; Costa et al., 2018). Amino acids play an important role in the normal life metabolism of fish in the early developmental stages. This study measured the composition and variation of total amino acids and free amino acids in fertilized eggs, embryonic stage, newly-hatched larvae stage, and open-mouthed larvae stage. The contents of total amino acids, EAA, and NEAA decreased from fertilized eggs to the open-mouthed larvae stage. Similar results had been reported in Marine Crab, Charybdis japonica(Crustacea: Decapoda) (Xu et al., 2013), Maccullochella macquariensis (Gunasekera et al., 2001), Cynoglossus semilaevis (Chang et al., 2007) and Sebastes schlegelii (Han et al., 2019). The contents of Leu and Lys in the EAA remained the highest from fertilized eggs to open-mouthed larvae stage in the early development, which may be that they can be decomposed into acetyl-CoA and acetoacetate in the biological metabolism, and they were both intermediate products of lipid and carbohydrate metabolism, and then entered the tricarboxylic acid cycle to release energy. This played an important role in the formation of embryonic tissues, organs formation, and metabolism (Tong et al., 2017). Among the NEAA, Glu had the highest content, which may play an important role in the metabolism and conversion of sugar-lipid-protein. Glutamate was catalyzed by enzymes to generate α-ketoglutarate, which entered the tricarboxylic acid cycle and then formed oxaloacetate under the action of aspartate aminotransferase, and formed glucose through gluconeogenesis, which stored energy to promote embryonic development (Han et al., 2019).
In this experiment, the amino acids of embryos and larvae in cobia were derived from the hydrolysis of nutrients in the yolk sac, and the total amino acids of open-mouthed larvae were significantly lower than those of embryonic and newly-hatched larvae. Studies have shown that the lack or deficiency of any EAA can lead to slow growth of fish or reduced feed conversion rate (Han et al., 2019). In this study, Phe, Ile, Thr, and Arg decreased rapidly, and the utilization of EAA was much higher than that of NEAA. Previous studies have shown that the addition of EAA to the open-mouth larvae diet can promote survival rates and growth rates, such as C. japonica (Xu et al., 2013) and red sea bream (Pagrus major) (Pez-Alvarado and Kanazawa, 1995). The content of FAA in the fertilized eggs of cobia was very high, which was 30.33% of the total amino acid content. This was consistent with the determination results of FAA contents in other marine fish floating eggs, which was 20%-50% of the total amino acid content (Yu et al., 2014; Rayner et al., 2017).
On the contrary, the content of FAA in the sinking eggs of marine fish was only 2%-3% of the total amino acid content, which was less than 5% in freshwater fish eggs (Gunasekera et al., 1999). Hence, the difference in FAA profile can be attributed to the different food environments (Rayner et al., 2017). It is therefore encouraged to design a diet that optimizes the FAA profile in the live feed. The content of Val, Leu, Ile, Ser, and Ala in the fertilized eggs of cobia was very rich, which was entirely consistent with the main FAA in the floating eggs of some marine fish. The commonality was the result of hydrolysis of yolk protein during oocyte development.
In this experiment, the total amount of FAA decreased significantly from fertilized eggs to open-mouthed larvae. In other studies of marine larvae, there were found that the total amount of FAA pools continued to decline during the development of fertilized eggs to open-mouth larvae, and there would be differences among different species. The reduction of content occurred in each FAA, and there was no selectivity for the remaining essential free amino acids (FEAA). This was similar to the results of Pelates sexlineatus (Brown et al., 2005) and Cynoglossus semilaevis (Chang et al., 2007).
In the early stage of larval ontogeny, FAA had two main functions: that is participating in the synthesis of fish protein and being decomposed and utilized as energy. The consumption of FEAA in fertilized eggs of cobia may be related to the synthesis of new proteins. Studies have shown that one of the functions of FAA in the development of marine fish was as a matrix for energy metabolism (Li et al., 2009; Finn and Fyhn, 2010; Tocher, 2010). Furthermore, the pattern of oxidative catabolic substrates varies according to whether the fertilized eggs of the fish had oil globules. The importance of the FAA pool of species with oil globules in the development of marine fish was lower than that of species without oil globules (Buentello et al., 2011). 70% of the energy of fish eggs without oil balls came from the catabolism of amino acids, and 30% of the energy came from the catabolism of triglycerides. For fertilized eggs with oil globules, 50% of the energy came from the catabolism of amino acids (mainly FAA); 50% of the energy came from the catabolism of neutral lipids, such as wax esters and triglycerides (Rønnestad et al., 1999; Baras et al., 2018). The fertilized eggs of cobia had oil globules, and its energy metabolism mode belonged to the latter.
After the larvae hatch from the embryo, the yolk sac was completely consumed and enters the exogenous nutrition stage. The time when larvae began to take in exogenous nutrients was the most critical period. It must start to actively look for and ingest food and rely on its intestinal digestion and absorption to accumulate exogenous nutrients for its own growth and energy consumption. The main sources of exogenous nutrients were protein, small peptides, and FAA, but their relative utilization may be related to species, developmental stage of larvae, feeding rate, and the proportion of these components in food (Infante and Cahu, 2001; Rannestad et al., 2003). Larvae can absorb proteins through pinocytosis and intracellular digestion of mucosal cells. However, the digestive system of larvae still has limited ability to digest proteins (Rannestad et al., 2003). Small peptides may originate from the autolysis of live bait, and its absorption efficiency in the intestine was higher than that of proteins. Zambonino Infante et al. (1997) found that replacing 20% of protein with dipeptide and tripeptide in micro-pellet feed could improve the development and survival rate of Dicentrarchus labrax larvae. The absorption efficiency of FAA was higher than that of proteins and peptides. After marine larvae and juveniles ingested biological diets (rotifers, Artemia larvae, etc.), FAA can be rapidly released and absorbed by larvae and juveniles, as reported in Senegalese sole (Rannestad et al., 2003). Rønnestad et al. (1999) suggested that there should be a considerable proportion of amino acids in early larval diets. As a measure of the nutritional quality of larvae after hatching (Oberg and Fuiman, 2015; Costa et al., 2018) and to estimate the requirements for early stages of development (Saavedra et al., 2015), amino acid profiles of eggs and larvae after hatching can be used. Identifying potential AA deficiency in diets is possible by analyzing AA profiles from sampled eggs and larvae and correcting the AA profiles towards a diet that is more balanced in AA (Saavedra et al., 2015). Studying amino acid requirements in fish larvae has been successfully conducted using this approach (Oberg and Fuiman, 2015; Saavedra et al., 2015; Costa et al., 2018). On the basis of data from this study, further research can be conducted to analyze the composition of different sources of live food offered to cobia larvae to meet their nutritional requirements.
Fatty acid composition and its changes in the early developmental stage of cobia
Fatty was considered to be an important metabolic energy source during embryonic development. It can be seen that the utilization of SFA (31.48%) in cobia during embryonic development was greater than that of MUFA (22.12%) and PUFA (12.76%). From fertilized egg development to the open-mouthed larvae stage, C16:0 and C18:1n9c were mainly consumed as energy sources. There was no significant difference in the contents of C18:2n6 and C18:3n3 among between fertilized eggs, newly-hatched larvae, and open-mouthed larvae, but there was a particular downward trend. This may indicate that they were not utilized for energy or carbon chain elongation or desaturation to synthesize long-chain, highly unsaturated fatty acids (HUFA). For C20 polyunsaturated fatty acids, there seem to be two models for the developmental process: one was the n-3 series of fatty acids, such as C20:5n3, whose content was significantly reduced, may be used as an energy source or as a precursor of C22:5n3, and then further desaturated to synthesize DHA, which was very important in fish development. This was because marine fish do not have the ability to convert C:18 PUFAs to C:20 and C:22 long-chain HUFAs. Second, the content of C20:4n6 (ARA) remained unchanged during development. This was also reported by Cejas et al. (2004) in the study of the fertilized eggs and larvae of Diplodus sargus. Although n-3 polyunsaturated fatty acids account for a considerable proportion of lipids in the cell membranes of marine fish, the synthesis of prostaglandins in marine fish mainly comes from ARA rather than EPA (Chang et al., 2007; Siriwardhana et al., 2012).
As a way to assess the quality of spawn and larvae, fatty acid profiles of eggs and larvae are commonly used (Tocher, 2010). Additionally, it serves as a measure of nutritional needs, particularly for marine organisms, including marine fish (Garrido et al., 2012). In this study, the sustained accumulation of ARA in the early development stage may indicate that cobia had a specific need for ARA during open-mouthed feeding. This seems to be consistent with the findings that ARA and DHA were preferentially retained in the phospholipid fraction of Gadus morhua, Coryphaena hippurus, Florida pompano (Trachinotus carolinus), and Common snook (Centropomus undecimalis) larvae during starvation, and that the content of these fatty acids was essential for their survival (Hauville et al., 2016). DHA was necessary for the development of the brain and optic nerve of marine fish (Mourente et al., 1991; Garg et al., 2017). Due to the lack of DHA, vision and nerves may be damaged, resulting in abnormal organism behavior (Forsyth et al., 2017; Garg et al., 2017). There was no significant difference in DHA content from fertilized eggs to newly-hatched larvae, but it decreased significantly when developed to open-mouthed larvae, which was also reflected in EPA. This indicated that there was little biotransformation or synthesis of DHA at the stage of yolk-sac absorption and that these two PUFAs may be selected for metabolism. Therefore, supplementation of DHA at the open-mouthed cobia larvae was necessary to obtain their good growth performance due to the apparent consumption of EPA and DHA during the development of larvae in cobia.
After the cobia larvae were hatched, lipids from the oil globules of fertilized eggs were the main energy substances from newly-hatched larvae and open-mouthed larvae. The endogenous nutrients in the yolk sac were gradually consumed, so the lipids content of the larvae decreased. When the fish body consumes the original amount stored in the body and fails to absorb nutrients from the outside in time, the larvae can only use their energy storage substances to provide energy to maintain life, so the fish body fatty was further reduced rapidly. In this study, among the three types of fatty acids in the open-mouthed larvae of cobia, the content of PUFA was higher than that of SFA and MUFA, which was consistent with the research results of most marine fish such as Melanogrammus aeglefinus (Plante et al., 2007). The actual content of the main fatty acids in the fertilized eggs of cobia ranked in the top four as C18:1n9c, C16:0, C22:6n3 (DHA), and C18:0, which was similar to the composition of the main fatty acids in the fertilized eggs of many marine fish (Ohkubo and Matsubara, 2002), indicating that these fatty acids were particularly important for the early development of cobia.
Characteristics of fatty acid digestion in cobia embryos and endogenous nutrition stages of larvae
In the early embryonic development stage of fish, SFA and MUFA were often used first as important energy sources, while n3PUFA will be properly preserved. The sequence of fatty acid utilization was SFA, MUFA, n6PUFA, and n3PUFA, which had been confirmed in many fish species, such as Oplegnathus fasciatus (Xu et al., 2013), Larimichthy crocea (Wang et al., 2006), Solea senegalensis (Mourente and Vazquez, 1996), Maccullochella macquarensis (Gunasekera et al., 2001). In this study, the utilization of fatty acids in the embryonic stage of cobia completely conformed to this order. However, the order of fatty acids utilization in the endogenous nutrition stage of larvae was exactly reversed. The actual utilization rate of fatty acids in the embryonic stage was SFA (23.19%), MUFA (12.98%), n6PUFA (1.28%), and n3PUFA (-0.50%), and the actual utilization of single fatty acid was higher for C14:0 (34.45%), C16:0 (24.56%), C20:3n3 (17.98%) and C18:0 (17.98%). It can be seen that the actual utilization of n3PUFA in cobia embryos was very low; n3PUFA was preferentially preserved, especially DHA, which measured data showed a negative value. This means that DHA was completely preserved during the embryonic stage of cobia, which was very similar to Solea senegalensis (Mourente and Vazquez, 1996) and Takifugu flavidus (Shi et al., 2010). After hatching, in the endogenous nutrient stage, the actual utilization of n3PUFA by larvae increased rapidly. The order of actual utilization of fatty acids in the open-mouthed larvae stage was just opposite to that in the embryonic stage, which was n3PUFA (25.06%), n6PUFA (15.20%), SFA (11.27%), and MUFA (11.06%). The higher utilization of single fatty acid were C22:6n-3(DHA)(26.03%), C22:5n3(DPA)(22.78%) and C20:5n3(EPA) (19.26%).In addition, the actual utilization of C22:6n3(DHA) by larvae was the highest (18.46mg/g). A large amount of n3PUFA (especially DHA) was utilized and consumed in open-mouthed larvae, which also illustrated the importance of n3PUFA (such as DHA) for developing open-mouthed larvae in cobia. This phenomenon that n3PUFAs was consumed in large amount in the open-mouthed larvae stage was similar to the research of Inimicus japonicus (Huang et al., 2013) and Oplegnathus fasciatus (Xu et al., 2013). However, the study on Pelteobagrus fulvidraco found that its 7-day-old larvae preferentially retained a large amount of DHA under starvation conditions, and the percentage of DHA was still higher than in the previous period (Lu et al., 2008). This may be due to the fact that marine fish require more DHA in the early development stage than that of freshwater fish.
The Causes of low survival rate in the early stage of cobia
Studies have confirmed that highly unsaturated fatty acids (DHA, EPA, and ARA) were essential fatty acids for the growth and development of marine fish larvae (Han et al., 2019). It was found that the ability to synthesize essential fatty acids in marine fish was much lower than that of freshwater fish (Gregory et al., 2010). In the endogenous nutritional stage of larvae in cobia, there was neither exogenous nutrition nor the ability to grow DHA and EPA from short-chain fatty acids (e.g., C18:2n6 and C18:3n3) under the dehydrogenation of fatty acid desaturase and the elongation of fatty acid elongase (Zheng et al., 2009). DHA and EPA are also essential fatty acids for human and animal growth and development (Izquierdo, 1996; Gunasekera et al., 2001). The larval stage was a period of rapid development of brain nerves and optic nerves, which required a large amount of DHA and other vital nutrients to meet the needed of brain nerve and optic nerve development (Lu et al., 2008). At the open-mouthed larvae stage of cobia, n3 PUFA was selectively utilized in a large amount, and a large amount of n3 PUFA needed to be supplemented from exogenous food. The content of n3 PUFA in biological bait directly affected the growth and survival of marine fish larvae (Izquierdo, 1996). However, if the open-mouthed larvae were fed with a diet containing little n3PUFA, such as Artemia larvae without n3PUFA strengthened, which will lead to a further lack of n3PUFA in the cobia larvae. In addition, too low DHA will cause a decrease in stress ability and even death of marine fish larvae (Liu and Chen, 2002), which may be one of the reasons for the low survival rate in the open-mouthed larvae stage of cobia fry cultivation. Therefore, baits rich in EPA and DHA should be fed in time at open-mouthed larvae of cobia, such as rotifers and Artemia larvae strengthened with chlorella. Studies have found that adding appropriate ARA to the pellet feed of large yellow croaker (Pseudosciaena crocea) juveniles can significantly improve the ability of juveniles to resist external stress (Xie, 2011). Therefore, the domestication of the pelleted diet should be completed as soon as possible from the post-larval stage to the juvenile stage of cobia, and nutrients rich in ARA should be supplemented in the pelleted feed in time so as to reduce the occurrence of death peaks caused by nutritional deficiencies in the juvenile stage.
Conclusion
Research methods will always have limitations when evaluating the relationship between early life nutrition interventions and well-being outcomes later in life. During hatching eggs, the embryonic stage, newly hatched larvae, and larvae with open mouths, changes in amino acids and fats were examined in cobia, a marinated fish. It was found that protein composition varies significantly during ontogeny for cobia during the different stages of development. Also, some AAs experienced substantial changes. In addition, the lipid content of cobia decreased during the development phase, suggesting that they were used as a primary energy source. As a result of the findings, n3PUFA (especially DHA and EPA) are critical for cobia larval growth. Enhancement of bait enriched with DHA and EPA was suggested for cobia larvae breeding to improve survival rates. Although cobia culture is not as popular as other species, it may still serve as a foundation for developing a well-balanced diet for early-stage larval fishes. These studies provide fundamental information regarding cobia larvae’s fatty acid requirements. The results of the current research provide vital information on the Changes in amino acid and fatty acid composition during early development in cobia (Rachycentron canadum) that will enable scientists and to lager extend farmers to meritoriously evaluate the nutrient requirement of fish in order to improve the survival rate of larvae of cultured species in aquaculture.
Data availability statement
The data supporting the results of this study are obtainable from the corresponding authors upon reasonable demand.
Ethics statement
The use of all animals in this project was conducted under the Animal Welfare Act, the PHS Animal Welfare Policy, the NIH Guide for Care and Use of Laboratory Animals, and the policies and procedures of the People’s Republic of China, Guangdong province, and Guangdong Ocean University. The study was conducted in compliance with the regulations for administering laboratory animals in Guangdong province, China, and in compliance with the Guangdong Ocean University Research Council’s guidelines for the care and use of laboratory animals (approval number: GDOU-LAE-2020-013).
Author contributions
J-SH was involved in project administration, data collection, formal analysis, processing, and writing – of the original draft. R-XL and Y-MC were involved in data collection. J-DZ: supervision and involvement in resources. EA was involved in writing – original draft and writing – reviewing and editing. GC: conceptualized, involved in methodology, acquired funding, supervision, and involved in resources. All authors contributed to the article and approved the submitted version.
Funding
This work was supported by grants from the China Agriculture Research System of MOF and MARA (CARS-47), Southern Marine Science and Engineering Guangdong Laboratory (Zhanjiang) (ZJW-2019-06).
Acknowledgments
Our acknowledgment goes to all the founders of this study.
Conflict of interest
R-TX and Y-MC were employed by Guangdong Evergreen Feed Industry Co. Ltd, Zhanjiang, 524003, China and Hainan Blue Grain Technology Co., Ltd. Sanya, 572000, China, respectively.
The remaining authors declare that the research was conducted in the absence of any commercial or financial relationships that could be construed as a potential conflict of interest.
Publisher’s note
All claims expressed in this article are solely those of the authors and do not necessarily represent those of their affiliated organizations, or those of the publisher, the editors and the reviewers. Any product that may be evaluated in this article, or claim that may be made by its manufacturer, is not guaranteed or endorsed by the publisher.
References
Amenyogbe E., Huang J.-S., Chen G and Wang W.-Z. (2021). Probiotic potential of indigenous (Bacillus sp. RCS1, Pantoea agglomerans RCS2, and Bacillus cereus strain RCS3) isolated from cobia fish (Rachycentron canadum) and their antagonistic effects on the growth of pathogenic Vibrio alginolyticus, vibrio harveyi, streptococcus iniae, and Streptococcus agalactiae. Front. Mar. Sci. 8. doi: 10.3389/fmars.2021.672213
Amenyogbe E., Yang E.-J., Xie R.-T., Huang J.-S., Chen G. (2022). Influences of indigenous isolates Pantoea agglomerans RCS2 on growth, proximate analysis, haematological parameters, digestive enzyme activities, serum biochemical parameters, antioxidants activities, intestinal morphology, disease resistance, and molecular immune response in juvenile's cobia fish (Rachycentron canadum). Aquaculture 737942, 0044–8486. doi: 10.1016/j.aquaculture.2022.737942
Anggo A. D., Ma’ruf W. F., Swastawati F., Rianingsih L. (2015). Changes of amino and fatty acids in anchovy (Stolephorus sp) fermented fish paste with different fermentation periods. Proc. Environ. Sci. 23, 58–63. doi: 10.1016/j.proenv.2015.01.009
Baras E., Arifin O. Z., Slembrouck J., Subagja J., Kristanto A. H., Legendre M. (2018). Oil globule size in fish eggs: A matter of biome and reproductive strategy. Fish. Fish. 19 (6), 996–1002. doi: 10.1111/faf.12307
Brown M. R., Battaglene S. C., Morehead D. T., Brock M. (2005). Ontogenetic changes in amino acid and vitamins during early larval stages of striped trumpeter (Latris lineata). Aquaculture 248 (1-4), 263–274. doi: 10.1016/j.aquaculture.2005.04.018
Buentello J. A., Pohlenz C., Margulies D., Scholey V. P., Wexler J. B., Tovar-Ramírez D., et al. (2011). A preliminary study of digestive enzyme activities and amino acid composition of early juvenile yellowfin tuna (Thunnus albacares). Aquaculture 312 (1–4), 205–211. doi: 10.1016/j.aquaculture.2010.12.027
Cejas J. R., Almansa E., Jérez S., Bolaños A., Felipe B., Lorenzo A. (2004). Changes in lipid class and fatty acid composition during development in white seabream (Diplodus sargus) eggs and larvae. Comp. Biochem. Physiol. Part B: Biochem. Mol. Biol. 139 (2), 209–216. doi: 10.1016/j.cbpc.2004.07.010
Chang Q., Liang M. Q., Chen S. Q., Zhang H. H., Wang J. L., Zhang X. M. (2007). Changes in amino acid and fatty acid composition during development in tongue sole (Cynoglossus semilavis) eggs and larvae. Acta Hydrobiologica Sin. 31 (6), 767–773. doi: 10.3321/j.issn:1000-3207.2007.06.001
Costa D. C., Takata R., De S. e Silva W., Bessonart M., Gadea J. L., Magnone L., et al. (2018). Description of amino acid and fatty acid content during initial development of lophiosilurus alexandri (Siluriformes: Pseudopimelodidae), a carnivorous freshwater catfish. Neotropical Ichthyology 16 (2), e180014. doi: 10.1590/1982-0224-20180014
Faulk C. K., Holt G. J. (2008). Biochemical composition and quality of captive-spawned cobia Rachycentron canadum eggs. Aquaculture 279 (1-4), 70–76. doi: 10.1016/j.aquaculture.2008.03
Finn R. N., Fyhn H. J. (2010). Requirement for amino acids in ontogeny of fish. Aquaculture Research 41(5), 684–716. doi: 10.1111/j.1365-2109.2009.02220.x
Folch J., Lees M., Stanley G. S. (1957). A simple method for the isolation and purification of total lipides from animal tissues. J. Biol. Chem. 226 (1), 497–509. doi: 10.1016/S0021-9258(18)64849-5
Forsyth S., Gautier S., Salem N. (2017). The importance of dietary DHA and ARA in early life: a public health perspective. Proc. Nutr. Soc. 76 (04), 568–573. doi: 10.1017/s0029665117000313
Garg P., Pejaver R. K., Sukhija M., Ahuja A. (2017). Role of DHA, ARA, & phospholipids in brain development: An Indian perspective. Clin. Epidemiol. Global Health 5 (4), 155–162. doi: 10.1016/j.cegh.2017.09.003
Garrido S., Saiz E., Peters J., Ré P., Alvarez P., Cotano U., et al. (2012). Effect of food type and concentration on growth and fatty acid composition of early larvae of the anchovy (Engraulis encrasicolus) reared under laboratory conditions. J. Exp. Mar. Biol. Ecol. 434-435 (1), 16–24. doi: 10.1016/j.jembe.2012.07.021
Gregory M. K., See V. H. L., Gibson R. A., Schuller K. A. (2010). Cloning and functional characterisation of a fatty acyl elongase from southern bluefin tuna (Thunnus maccoyii). Comp. Biochem. Physiol. Part B: Biochem. Mol. Biol. 155 (2), 178–185. doi: 10.1016/j.cbpb.2009.11.002
Gunasekera R. M., De Silva S. S., Ingram B. A. (1999). The amino acid profiles in developing eggs and larvae of the freshwater percichthyid fishes, trout cod, Maccullochella macquariensis and Murray cod, Maccullochella peelii peelii. Aquat. Living Resour. 12 (4), 255–261. doi: 10.1016/S0990-7440(00)86636-X
Gunasekera R. M., De Silva S. S., Ingram B. A. (2001). Chemical changes in fed and starved larval trout cod, Maccullochella macquarensis during early development. Fish. Physiol. Biochem. 25 (4), 255–268. doi: 10.1023/A:1023247718139
Han H.-Z., Wang T.-T., Zhang M.-L., Wang F., Liu Y., Sun N., et al. (2019). Analysis on the composition of amino acid and fatty acid and their changes during early growth stage of Sebastes schlegelii. Acta Hydrobiologica Sin. 43 (3), 526–536. doi: 10.7541/2019.064
Hastey R. P., Phelps R. P., Davis D. A., Cummins K. A. (2010). Changes in free amino acid profile of red snapper Lutjanus campechanus, eggs, and developing larvae. Fish. Physiol. Biochem. 36, 473–481. doi: 10.1007/s10695-009-9317-7
Hauville M. R., Main K. L., Migaud H., Gordon Bell J. (2016). Fatty acid utilization during the early larval stages of Florida pompano (Trachinotus carolinus) and common snook (Centropomus undecimalis). Aquacult. Res. 47 (5), 1443–1458. doi: 10.1111/are.12602
Huang J.-S., Amenyogbe E., Chen G., Wang W.-Z. (2020). Biochemical composition and activities of digestive and antioxidant enzymes during the egg and yolk-sac larval development of the cobia (Rachycentron canadum). Aquacult. Res. 00, 1–14. doi: 10.1111/are.15017
Huang J.-S., Amenyogbe E., Yang L.-T., Wang Z.-L., Chen G., Wang W.-Z., et al. (2022). Cloning and expression analysis of hif-1α and downstream genes during hypoxic stress in cobia (Rachycentron canadum). Aquacult. Int. 30, 803–824. doi: 10.1007/s10499-021-00820-4
Huang X. X., Feng L. F., Wen W., Chen Q. K., Wei L. K. (2013). The changes in lipid and fatty acid profiles of devil stinger Inimicus japonicas during the development of embryo and yolksac larvae. J. Fish. China 37 (4), 526–535. doi: 10.3724/SP.J.1231.2013.38335
Huang J.-S., Li H.-J., Guo Z.-X., Zhang J.-D., Wang W.-Z., Wang Z.-L., et al. (2021). Identification and expression analysis of cobia (Rachycentron canadum) liver-related miRNAs under hypoxia stress. Fish. Physiol. Biochem. 47 (6), 1951–1967. doi: 10.1007/s10695-021-01017-5
Huang J.-S., Lu Z., Zhang J.-D., Chen G., Pan C.-H., Deng W.-X. (2019). Analysis of amino acid composition of different tissues collected from cultured cobia (Rachycentron canadum) broodstock. Mar. Sci. 43 (4), 61–69. doi: CNKI:SUN:HYKX.0.2019-04-009
Infante J. Z., Cahu C. (2001). Ontogeny of the gastrointestinal tract of marine fish larvae. Comp. Biochem. Physiol. Part C: Toxicol. Pharmacol. 130 (4), 477–487. doi: 10.1016/S1095-6433(00)80321-9
Izquierdo M. (1996). Essential fatty acid requirements of cultured marine fish larvae. Aquacult. Nutr. 2 (4), 183–191. doi: 10.1111/j.1365-2095.1996.tb00058.x
Jia Y. D., Meng Z., Liu X. F., Lei J. L. (2014). Biochemical composition and quality of turbot (Scophthalmus maximus) eggs throughout the reproductive season. Fish. Physiol. Biochem. 40, 1093–1104. doi: 10.1007/s10695-014-9908-9
Kuang J., Chen G., Ma Q., Huang J.-S., Zhang J.-D., Shi G., et al. (2021). Embryonic development and morphological characteristics of larvae and juveniles of cobia (Rachycentron canadum). J. Fish. China 45 (11), 1814–1824. doi: 10.11964/jfc.20200812389
Lanes C. F. C., Bizuayehu T. T., Bolla S., Martins C., de Oliveira Fernandes J. M., Bianchini A., et al. (2012). Biochemical composition and performance of Atlantic cod (Gadus morhua l.) eggs and larvae obtained from farmed and wild broodstocks. Aquaculture 324–325, 267–262. doi: 10.1016/j.aquaculture.2011.10.036
Li Z.H., Xie S., Wang J.X., Sales J., Li P., Chen D.Q. (2009). Effect of intermittent starvation on growth and some antioxidant indexes of Macrobrachium nipponense (De Haan). Aquaculture Research 40, 526–532. doi: 10.1111/J.1365-2109.2008.02123.X
Li J., Li X., Ji R., Xu W., Mai K.-S., Ai Q.-H. (2018). The effects of linseed oil and soybean oil on fatty acid composition and Δ6Fad gene expression in liver and muscle of large yellow croaker (Larimichthys crocea). Acta Hydrobiologica Sin. 42 (2), 232–239. doi: 10.7541/2018.029
Liu J. K., Chen X. L. (2002). A survey of research on n-3 HUFA nutrition for marine fish larvae. J. Ocean Univ. Qingdao 32 (6), 897–902. doi: 10.1007/s11769-002-0026-8
Lubzens E., Young G., Bobe J., Cerda J. (2010). Oogenesis in teleosts: How fish eggs are formed. Gen. Comp. Endocrinol. 165, 367–389. doi: 10.1016/j.ygcen.2009.05.022
Lu S. F., Zhao N., Liu H. B., He R. G. (2008). Changes in fatty acid composition during development in pelteobagrus fulvidraco fertilized eggs and larvae. J. Fish. China 32 (5), 711–716. doi: 10.3724/sp.j.00001
Mansour N., Lahnsteiner F., McNiven M. A., Richardson G. F., Pelletier C. S. (2011). Relationship between fertility and fatty acid profile of sperm and eggs in Arctic char, Salvelinus alpinus. Aquaculture 318, 371–378. doi: 10.1016/j.aquaculture.2011.05.023
Mourente G., Tocher D. R., Sargent J. R. (1991). Specific accumulation of docosahexaenoic acid (22:6n- 3) in brain lipids during development of juvenile turbot Scophthalmus maximus l. Lipids 26 (11), 871–877. doi: 10.1007/BF02535970
Mourente G., Vazquez R. (1996). Changes in the content of total lipid, lipid classes and their fatty acids of developing eggs and unfed larvae of the Senegal sole, Solea senegalensis kaup. Fish. Physiol. Biochem. 15 (3), 221–235. doi: 10.1007/BF01875573
Oberg E. W., Fuiman L. A. (2015). Linking fatty acids in the diet and tissues to quality of larval southern flounder (Paralichthys lethostigma). J. Exp. Mar. Biol. Ecol. 467 (1), 7–15. doi: 10.1016/j.jembe.2015.02.021
Ohkubo N., Matsubara T. (2002). Sequential utilization of free amino acids, yolk proteins and lipids in developing eggs and yolk-sac larvae of barfin flounder Verasper moseri. Mar. Biol. 140 (1), 187–196. doi: 10.1007/s002270100647
Pez-Alvarado L. J., Kanazawa A. (1995). Optimum levels of crystalline amino acids in diets for larval red sea bream (Pagrus major); proceedings of the ICES marine science symposia (F. Copenhagen, Denmark: International Council for the Exploration of the Sea). 1991.
Plante S., Pernet F., Haché R., Ritchie R., Ji B., McIntosh D. (2007). Ontogenetic variations in lipid class and fatty acid composition of haddock larvae Melanogrammus aeglefinus in relation to changes in diet and microbial environment. Aquaculture 263 (1-4), 107–121. doi: 10.1016/j.aquaculture.2006.09
Rainuzzo J. R., Reitan K. I., Olsen Y. (1997). The significance of lipids at early stages of marine fish: a review. Aquaculture 155 (1-4), 103–115. doi: 10.1016/S0044-8486(97)00121-X
Rannestad I., Tonheim S., Fyhn H., Rojas-Garc C. R., Kamisakaa Y., Kovenc W., et al. (2003). The supply of amino acids during early feeding stages of marine fish larvae: a review of recent findings. Aquaculture 227 (1-4), 147–164. doi: 10.1016/s0044-8486(03)00500-3.
Rayner T. A., Hwang J.-S., Hansen B. W. (2017). Anticipating the free amino acid concentrations in newly hatched pelagic fish larvae based on recently fertilized eggs and temperature. J. Plankton Res. 39 (6), 1012–1019. doi: 10.1093/plankt/fbx058
Rønnestad I., Thorsen A., Finn R. N. (1999). Fish larval nutrition: a review of recent advances in the role of amino acids. Aquaculture 177, 201–216.
Saavedra M., Candeias-Mendes A., Castanho S., Teixeira B., Mendes R., Pousão-Ferreira P. (2015). Amino acid profiles of meagre (Argyrosomus regius) larvae: Towards the formulation of an amino acid balanced diet. Aquaculture 448 (1), 315–320. doi: 10.1016/j.aquaculture.2015.06.003
Shi Y. H., Zhang G. Y., Liu J. Z., Zhu Y. Z., Zang W. L., Wang C. H. (2010). Growth, development and behavior ecology of tawny puffer (Takifugu flavidus) larvae and juveniles. J. Fish. China 34 (10), 1509–1517. doi: 10.3724/SP.J.1231.2010.07004
Siriwardhana N., Kalupahana N. S., Moustaid-Moussa N. (2012). Health benefits of n-3 polyunsaturated fatty acids. Adv. Food Nutr. Res. 65, 211–222. doi: 10.1016/b978-0-12-416003-3.00013-5
Tocher D. R. (2010). Fatty acid requirements in ontogeny of marine and freshwater fish. Aquacult. Res. 41 (5), 717–732. doi: 10.1111/j.1365-2109.2008.02150.x
Tong X., Yang X., Bao C., Wang J., Tang X., Jiang D., et al. (2017). Changes of biochemical compositions during development of eggs and yolk-sac larvae of turbot Scophthalmus maximus. Aquaculture 473, 317–326. doi: 10.1016/j.aquaculture.2017.02.024
Wang D. L., Xu S. L., Yan X. J., Wang Y. (2006). Fatty acid composition and their changes in larvae and juveniles of Pseudosciaena crocea. J. Fish. China 30 (2), 241–245. doi: 10.3321/j.issn:1000-0615.2006.02.017
Xie F. J. (2011). Study on nutritional physiology of amino acid and fatty acid for large yellow croaker (Pseudosciaena crocea) larvae [D] (Qingdao: Ocean University of China). Thesis for Doctor of Science.
Yu H., Li R., Liu S., Xing R., Chen X., Li P. (2014). Amino acid composition and nutritional quality of gonad from jellyfish Rhopilema esculentum. Biomed. Prev. Nutr. 4 (3), 399–402. doi: 10.1016/j.bionut.2014.04.007
Zambonino Infante J. L., Cahu C. L., Peres A. (1997). Partial substitution of di-and tripeptides for native proteins in sea bass diet improves Dicentrarchus labrax larval development. J. Nutr. 127 (4), 608–614. doi: 10.1046/j.1365-277X.1997.00046.x
Zhang J.-D., Amenyogbe E., Yang E.-J., Wang Z.-L., Chen G., Huang J.-S. (2021). Feeding habits and growth characteristics of cobia (Rachycentron canadum) larval and juvenile stages. Aquaculture 736612, 0044–8486. doi: 10.1016/j.aquaculture.2021.736612
Zhao S., Wu W., Zhu L., Dai X.-F., Zhu L.-Y., Lu Y.-X., et al. (2017). Effects of substituting palm oil for fish oil and soy oil in feed on the growth performance and muscular fatty acid composition of Pelteobagrus vachelli. Acta Hydrobiologica Sin. 41 (5), 1000–1009. doi: 10.7541/2017.125
Zheng X., Ding Z., Xu Y., Monroig O., Morais S., Tocher D. R. (2009). Physiological roles of fatty acyl desaturases and elongases in marine fish: Characterisation of cDNAs of fatty acyl Δ6 desaturase and elovl5 elongase of cobia (Rachycentron canadum). Aquaculture 290 (1-2), 122–131. doi: 10.1016/j.aquaculture.2009.02
Keywords: Rachycentron canadum, fertilized eggs, embryos, larvae, amino acids, fatty acids
Citation: Huang J-s, Li R-x, Xie R-t, Chen Y-m, Zhang J-d, Amenyogbe E and Chen G (2022) Changes in amino acid and fatty acid composition during early development in cobia (Rachycentron canadum). Front. Mar. Sci. 9:995616. doi: 10.3389/fmars.2022.995616
Received: 16 July 2022; Accepted: 30 August 2022;
Published: 16 September 2022.
Edited by:
Alaa El-Din Hamid Sayed, Assiut University, EgyptReviewed by:
Dian-Chang Zhang, South China Sea Fisheries Research Institute (CAFS), ChinaD. K. Meena, Central Inland Fisheries Research Institute (ICAR), India
Copyright © 2022 Huang, Li, Xie, Chen, Zhang, Amenyogbe and Chen. This is an open-access article distributed under the terms of the Creative Commons Attribution License (CC BY). The use, distribution or reproduction in other forums is permitted, provided the original author(s) and the copyright owner(s) are credited and that the original publication in this journal is cited, in accordance with accepted academic practice. No use, distribution or reproduction is permitted which does not comply with these terms.
*Correspondence: Eric Amenyogbe, YW1lbnlvZ2JlZXJpY0B5YWhvby5jb20=; Gang Chen, Y2hlbmdAZ2RvdS5lZHUuY24=