- 1U.S. Geological Survey St. Petersburg Coastal and Marine Science Center, St. Petersburg, FL, United States
- 2Department of Geosciences, Florida Atlantic University, Boca Raton, FL, United States
- 3Department of Earth and Planetary Sciences, Rutgers University, Piscataway, NJ, United States
- 4Marine and Coastal Programs, Dial Cordy & Associates, Inc., Miami, FL, United States
The Holocene reefs off southeast Florida provide unique insights into the biogeographical and ecological response of western Atlantic coral reefs to past climate change that can be used to evaluate future climate impacts. However, previous studies have focused on millennial-scale change during the stable mid-Holocene, making it difficult to make inferences about the impact of shorter-term variability that is relevant to modern climate warming. Using uranium-series dating of newly discovered subfossil coral rubble deposits, we establish a new high-resolution record of coral community development off southeast Florida during a period of variable climate in the late Holocene. Our results indicate that coral communities dominated by reef-building Acropora palmata and Orbicella spp. persisted in the nearshore environments off southeast Florida ~75 km north of their primary historical ranges between ~3500 and 1800 years before present. This timing coincides with regional warming at the northern extent of the Atlantic Warm Pool, suggesting a likely link between regional oceanographic climate and the expansion of cold-sensitive reef-building coral communities to the high-latitude reefs off southeast Florida. These findings not only extend the record of coral-reef development in southeast Florida into the late Holocene, but they also have important implications for future range expansions of reef-building coral communities in response to modern climate change.
Introduction
Since the late 1970s, researchers have documented a continuous decline in live coral cover throughout the tropical western Atlantic (Gardner et al., 2003; Bruno and Selig, 2007; Jackson et al., 2014). Climate-driven coral bleaching events and regional coral disease outbreaks have been implicated as the major causes of this decline, and on many reefs, have resulted in the near extirpation of several ecologically critical coral taxa including the once dominant reef-builders, Acropora palmata and Orbicella spp. (Aronson and Precht, 2001; Bruno et al., 2007; Edmunds, 2015; Randall and van Woesik, 2015). The loss of these taxa has had a dramatic effect on the health and function of coral-reef ecosystems throughout the region (Kuffner and Toth, 2016), prompting aggressive management actions such as establishment of marine protected areas and coral restoration programs (Mellin et al., 2016; Bruno et al., 2019). However, without substantial global efforts to mitigate climate change, projected increases in the frequency and intensity of heat-associated stress events over the next century are expected to severely limit the efficacy of most local conservation efforts (Aronson and Precht, 2006; Kennedy et al., 2013; Hoegh-Guldberg et al., 2018; Bruno et al., 2019; Goreau and Hayes, 2021).
Despite diminishing coral populations in the tropics, there is growing evidence that Acropora and Orbicella spp. are currently expanding their ranges northward into the cooler, subtropical waters off southeast Florida and in the northwestern Gulf of Mexico in an apparent response to rising ocean temperatures (Precht and Aronson, 2004; Precht et al., 2014). Similar warming-induced range expansions of tropical corals have also been documented on subtropical and temperate reefs in Japan (Yamano et al., 2011), the Mediterranean Sea (Serrano et al., 2013), and southeastern Australia (Baird et al., 2012; Tuckett et al., 2017), suggesting that higher latitude reef environments across the globe may provide crucial refuge to thermally sensitive coral species in a future warming world (Precht and Aronson, 2004; Beger et al., 2014; Makino et al., 2014). However, because these trends were only recently documented, there is still considerable uncertainty regarding whether coral populations can persist in high latitude environments under varying future climate-change scenarios (Yara et al., 2012; Muir et al., 2015; Hoegh-Guldberg et al., 2017).
The fossil record offers a unique opportunity to examine the biogeographical response of coral-reef communities to climate change over temporal scales longer than modern ecological studies (Precht and Aronson, 2006; Precht and Miller, 2007; Greenstein and Pandolfi, 2008; Pandolfi, 2011). For instance, geological investigations of relict reefs off the coast of southeast Florida have revealed that well developed A. palmata-dominated reefs were flourishing well north of their historical ranges during the Holocene Thermal Maximum (HTM) [~10500 to 5400 years before present (B.P.)] (Lighty et al., 1978; Precht and Aronson, 2004; Precht et al., 2014). Warmer climate conditions at this time coupled with the northward transport of coral larvae via the warm-water Florida Current likely permitted a more northerly distribution of cold-sensitive A. palmata, leading to the development of an expansive barrier reef complex along the shallow continental shelf (Lighty et al., 1978; Precht and Aronson, 2004; Banks et al., 2007; Stathakopoulos and Riegl, 2015; Toth et al., 2021). Significant reef development continued off southeast Florida until the late Holocene when changing climate conditions eventually resulted in the southward contraction of A. palmata to its historical limit at Fowey Rocks Reef off Miami, Florida (Toth et al., 2021).
The fossil reefs off southeast Florida provide a long-term context that, when compared with modern coral-reef communities, can help inform future conservation responses to climate-associated reef degradation (Precht and Miller, 2007). To date, however, most published studies from the region have relied on a limited number of submerged outcrops, narrow-diameter reef cores, and surface sampling with coarse millennial-scale resolutions (e.g., Lighty et al., 1978; Banks et al., 2007; Stathakopoulos and Riegl, 2015; Toth et al., 2021) making it difficult to extrapolate the higher frequency variability relevant to modern climate warming. Furthermore, existing records from southeast Florida are limited to the relatively stable climate of the HTM, which may be a poor analogue for more dynamic climate-change scenarios (Toth et al., 2021). To address this issue, we present a new high-resolution record of A. palmata- and Orbicella spp.-coral community development on the southeast Florida reef tract (SFRT) during the more variable climate of the late Holocene. Our record is based on high-precision Uranium-Thorium (U-Th) dating of paleo-reef deposits recently discovered off the coast of northern Broward County, Florida. Our results not only extend the timeline of high-latitude reef development off southeast Florida into the late Holocene, but they also provide critical insights into the potential range expansion of tropical western Atlantic reef-building coral taxa in response to modern climate warming.
Materials and Methods
Regional setting
The Florida reef tract is a semi-continuous complex of coral-reef habitats extending ~580 km along Florida’s Atlantic coastline from Dry Tortugas National Park to offshore northern Palm Beach County (Figure 1A). It can be divided into two primary biogeographical subregions based on their characteristic geomorphology and accretion histories: the southeast Florida reef tract (SFRT) and the Florida Keys reef tract (FKRT) (Lidz et al., 2003; Banks et al., 2007). Although both subregions are connected by the northward flowing Florida Current (Frys et al., 2020), modern stony coral assemblages exhibit considerable latitudinal variability with decreasing diversity and abundance north of their shared boundary at Fowey Rocks reef in Biscayne National Park (Blair and Flynn, 1989; Precht and Miller, 2007; Burman et al., 2012). Historically, this reef was the northernmost extent of A. palmata growth in the western Atlantic, marking a distinct transition to cooler, more turbid environmental conditions along the SFRT to the north (Vaughan, 1914; Smith, 1943; Jaap, 1984). Although various stress-tolerant, eurytopic species of stony coral are still present throughout the SFRT, modern benthic communities are now dominated by non-accreting organisms such as macroalgae, sponges, and soft corals (Raymond, 1972; Goldberg, 1973; Moyer et al., 2003; Walker, 2012a).
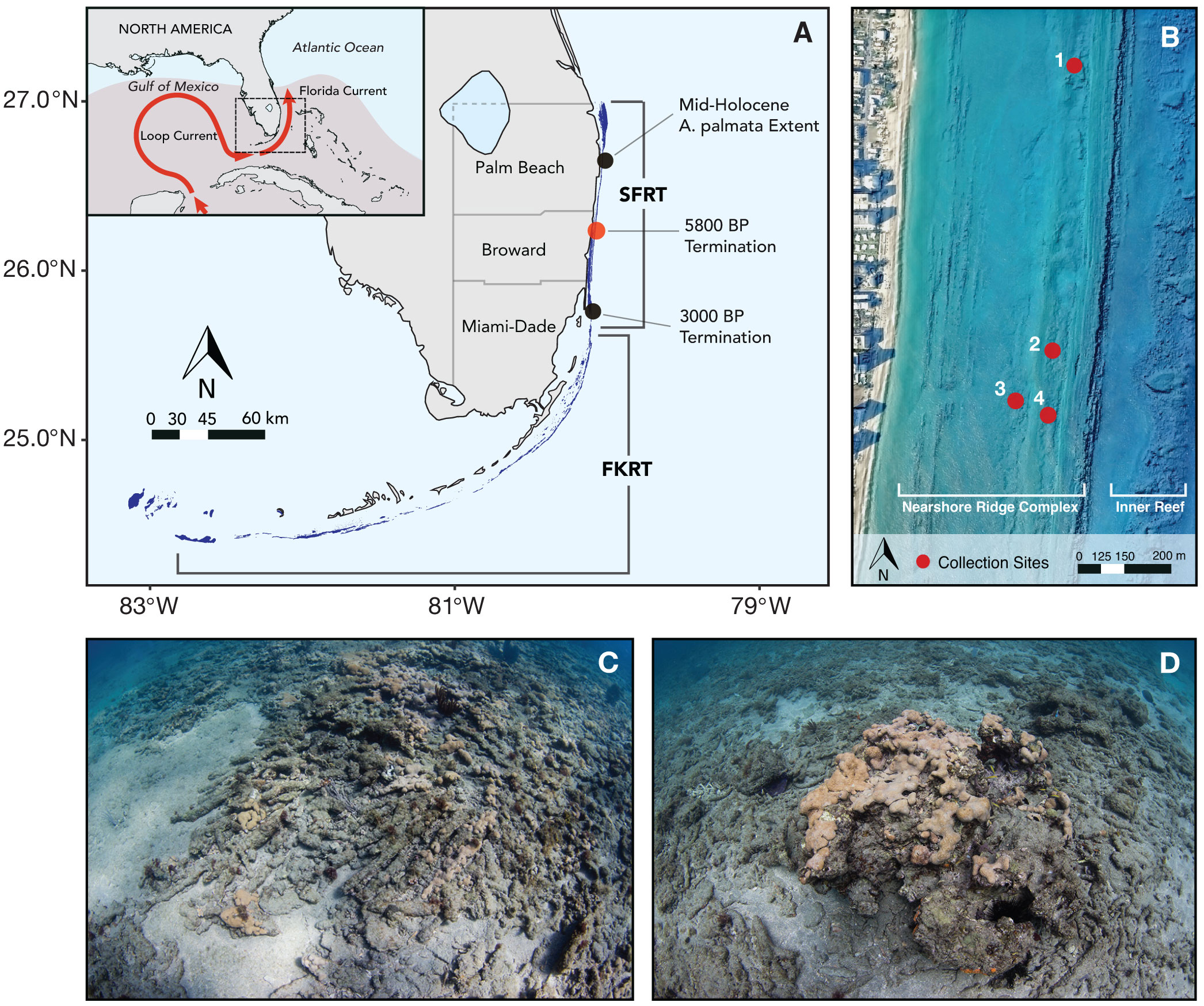
Figure 1 Description of the study area in relation to the broader biogeographical setting of the Florida reef tract. (A) Location of study area (red dot) on Southeast Florida reef tract (SFRT) extent, the full extent of which is shown by the dark blue polygon. Inset map shows main currents affecting the region. Estimated maximum Atlantic Warm Pool extent in fall (defined as sea surface temperatures (SSTs) warmer than 28.5° [Wang et al., 2008]) is shaded in red. (B) Map of study area with sampling sites marked in red. Map was made by overlaying 1-m resolution lidar bathymetry and 1-m resolution aerial imagery. Bathymetric map was generated with digital elevation models acquired by U.S. Army Corp of Engineers in 2017. Aerial imagery was collected by PhotoScience, Inc. on March 8, 2013. (C) Interlocked sub-fossil Acropora palmata coral branches overlying antecedent coquinoid limestone surface. (D) Sub-fossil Orbicella spp. coral colony (~1.5 m diameter) surrounded by unconsolidated Acropora-dominated coral rubble and smaller branching coral rubble. Photos by A.B.M.
Despite the lack of significant reef-building coral communities on the SFRT at present, previous geological investigations have revealed an underlying structure composed almost entirely of Holocene-aged A. palmata coral framework (Lighty et al., 1978; Precht and Aronson, 2004; Banks et al., 2007; Stathakopoulos and Riegl, 2015; Toth et al., 2021). Extensive mapping and coring studies delineated these structures into two discrete, progressively deeper relict reef terraces known as the inner reef (~8 m below mean sea level [bMSL]) and outer reef (~16 m bMSL) (after Moyer et al., 2003, based on Lighty et al., 1978). Radiometric dating indicates that reef development on the outer reef reached its northernmost extent off northern Palm Beach County during the early to mid-Holocene but then started contracting southward at ~7800 years B.P. when climate conditions began changing throughout the subtropical western Atlantic. As the outer reef contracted southward, it also backstepped to the inner reef on the central and southern portions of the reef tract as sea level rose. However, by 3000 years B.P., coral-reef development throughout the SFRT had essentially shut down, leaving behind relict reef structures with minimal stony coral cover (Precht et al., 2000; Toth et al., 2021).
Inshore of the inner reef off Broward and Miami-Dade counties there also exists a series of shore-parallel hardbottom ridges called the “nearshore ridge complex (NRC)” (3–6 m bMSL). Unlike the offshore relict reefs, which are composed almost entirely of mid-Holocene A. palmata framework, the NRC is characterized by submerged beach ridges composed of Pleistocene-aged coquinoid limestone known as the Anastasia Formation (Raymond, 1972; Banks et al., 2007). The NRC has been the site of recent range expansions of A. cervicornis, which were first documented in the late 1990s (Vargas-Ángel et al., 2003). Occasional colonies of A. palmata and O. annularis have also been identified throughout the NRC as far north as Broward County (Precht and Aronson, 2004, Burman et al., 2012), but they are relatively rare compared to the expansive A. cervicornis thickets in the area (Burman et al., 2012; Walker et al., 2012b; D’Antonio et al., 2016).
Sample collection and U-Th dating
Subfossil coral rubble deposits were sampled along the northern extent of the nearshore ridge complex (NRC) ~600 meters off the coast of northern Broward County in southeast Florida (26°13’N, 80°5’W) (Figure 1B). Initial research dives revealed the presence of extensive subfossil coral rubble deposits scattered across the surface of exposed coquina ridges, forming a relatively thin veneer (< 1.2 m) of landward-fining coral rubble interspersed with partially buried subfossil massive corals (Figures 1C, D). Based on these dives, four collection sites representing the full spatial extent of the subfossil coral deposits were selected. Within each site, coral fragments were collected haphazardly along two 50 x 5 m belt transects oriented parallel to the shoreline and from single 50-cm diameter pits (depths between 30 and 60 cm) excavated at a random point along each transect. For massive corals too large to collect by hand, 5-cm wide sections were removed from the outer surface of the colony using a hammer and chisel. After collection, each sample was identified to the lowest taxonomic level possible and prepared for U-Th dating (See Supplementary material for details). Although the majority of samples encountered in this study were identifiable to the species level, several Orbicella spp. colonies were indistinguishable between Orbicella faveolata and O. annularis (sensu stricto) due to significant bioerosion. However, we note that the majority of Orbicella colonies encountered in our study were identified as O. annularis (sensu stricto) based on the presence of irregular lobes or columnar morphology.
To constrain the timing of coral community development, a total of 40 subfossil A. palmata and Orbicella spp. coral samples collected across all four sites were selected for U-Th dating. High-precision U-Th dating offers an opportunity to derive centennial- or even decadal-scale reconstructions from Holocene and historic coral-reef death assemblages (Clark et al., 2017; Leonard et al., 2020; Hammerman et al., 2022). In this study, U-Th ages were determined using a ThermoScientific NEPTUNE PLUS Multi-collector Inductively Coupled Plasma Mass Spectrometer (MC-ICP-MS) at the Department of Earth and Planetary Sciences, Rutgers University (see Supplementary material for details). To construct a relative chronology of coral community development, the probability distribution of the U-Th ages was calculated using a non-parametric Kernel Density Estimation (KDE) approach in R (IsoplotR 3.3 package, Vermeesch, 2018). In this analysis, peaks in the KDE distribution reflect temporally clustered ages of broken and transported A. palmata branches as well as displaced or in situ subfossil Orbicella spp. colonies and are therefore interpreted as estimated peaks in net coral community growth and detrital skeletal accumulation (see Supplementary material for details). The resulting age distribution was then compared with long-term regional paleoclimate records in order to identify potential controls on coral community development.
Results
The U-Th ages of 40 subfossil A. palmata and Orbicella spp. coral samples collected across all four sites ranged between 4276 ± 24 (2σ) and 975 ± 6 (2σ) years B.P (Table S1). Kernel density estimation revealed a bimodal age distribution with 82.5% of all ages falling within two primary temporal clusters centered at 3036 ± 379 and 2322 ± 238 years B.P. (Figure 2) Although the proportion of A. palmata and Orbicella spp. sample ages varied between each growth cluster, there was no significant difference in ages between taxa (ANOVA, F1,38 = 2.675, p = 0.11), indicating minimal taphonomic bias due to time averaging (see Supplementary material for details). Lastly, consecutive coral ages were separated by an average of ~85 years, indicating that centennial-scale patterns of coral community development were captured by our sampling strategy.
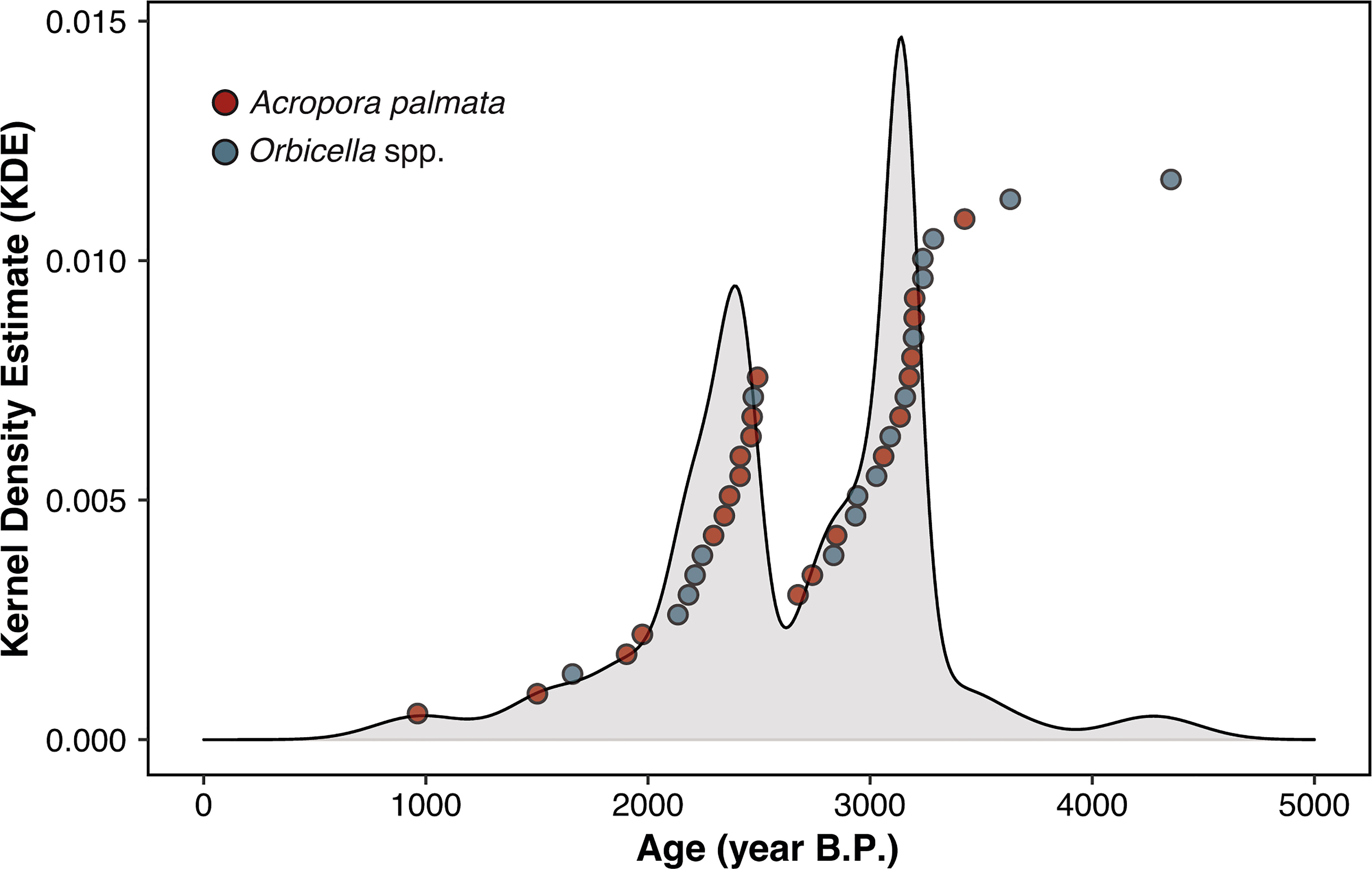
Figure 2 Kernel Density Estimate distribution curve produced from the U-Th age data [in years before present (BP)] obtained from subfossil Acropora palmata and Orbicella spp. coral skeletons (see Supplementary material for more details). Individual U-Th ages are shown as points.
Discussion
Our results provide new evidence that coral communities dominated by A. palmata and Orbicella spp. began colonizing the NRC off southeast Florida as early as 1500 years after the shutdown of reef accretion on the adjacent inner reef tract (5800 years B.P., Stathakopoulos and Riegl, 2015) and at least 1800 years after the NRC was flooded by rising sea level (based on Khan et al., 2017). Although several mechanisms have been proposed to explain the regional decline of reef development, extensive radiometric dating of relict reef framework indicates that accretion likely terminated at the end of the HTM when changing climate conditions forced the latitudinal limit of cold-sensitive A. palmata corals southward (Precht and Aronson, 2004; Precht et al., 2014; Toth et al., 2021). The specific climate mechanism responsible for conditions inhospitable to reef growth has been attributed to an orbitally forced deepening of the polar jet stream over the North American continent and an associated increase in the frequency of winter cold fronts reaching southeast Florida (Toth et al., 2021). Both A. palmata and Orbicella corals are highly sensitive to cold-water temperatures, and as a result, periodically undergo widespread mass mortality in the subtropical western Atlantic following severe cold fronts (Hudson et al., 1976; Roberts et al., 1982; Lirman et al., 2011; Jones et al., 2020). Therefore, increase in cold-front frequency would have likely limited their ability to build reef frameworks on the SFRT after the HTM (Toth et al., 2021). Our findings, however, suggest that despite changing winter climate conditions, nearshore reef temperatures off southeast Florida were periodically favorable for A. palmata and Orbicella spp. coral growth until at least 1800 years B.P.
Although millennial-scale climate change in the North Atlantic region during the late Holocene was dominated by externally forced cooling (Wanner et al., 2008; Marcott et al., 2013), paleoclimate records from the subtropical western Atlantic indicate higher frequency sea-surface temperature (SST) variability over shorter centennial timescales. In particular, SST reconstructions from the northern Gulf of Mexico and Florida Straits reveal a period of sustained SST warming between ~3500 and 2000 years B.P. (Figures 3A, B; Poore et al., 2004; Lund and Curry, 2004; Thirumalai et al., 2018; Thirumalai et al., 2021). Both sites are located at the northern extent of the Atlantic Warm Pool (AWP) (Wang and Enfield, 2001), a feature that forms annually when warm Caribbean water masses are transported around the Florida Peninsula and into the subtropical western Atlantic via the Loop and Florida currents (Lee et al., 1995; Lund and Curry, 2004; Figure 1A). Because of this specific circulation pattern, ocean temperatures in southeast Florida are strongly influenced by the extent of the AWP (Domingues et al., 2018), which has been shown to fluctuate over centennial timescales with the North Atlantic Oscillation (NAO) and Atlantic Multidecadal Variability (AMV) (Richey et al., 2007; Wang et al., 2008). Centennial-scale warming at the northern extent of the AWP corresponds to the timing of late Holocene range expansions on the NRC centered at ~3000 and 2300 years B.P., (Figures 3A, B, D), suggesting a potential coupling between the northern extent of coral community growth in southeast Florida and regional oceanographic variability.
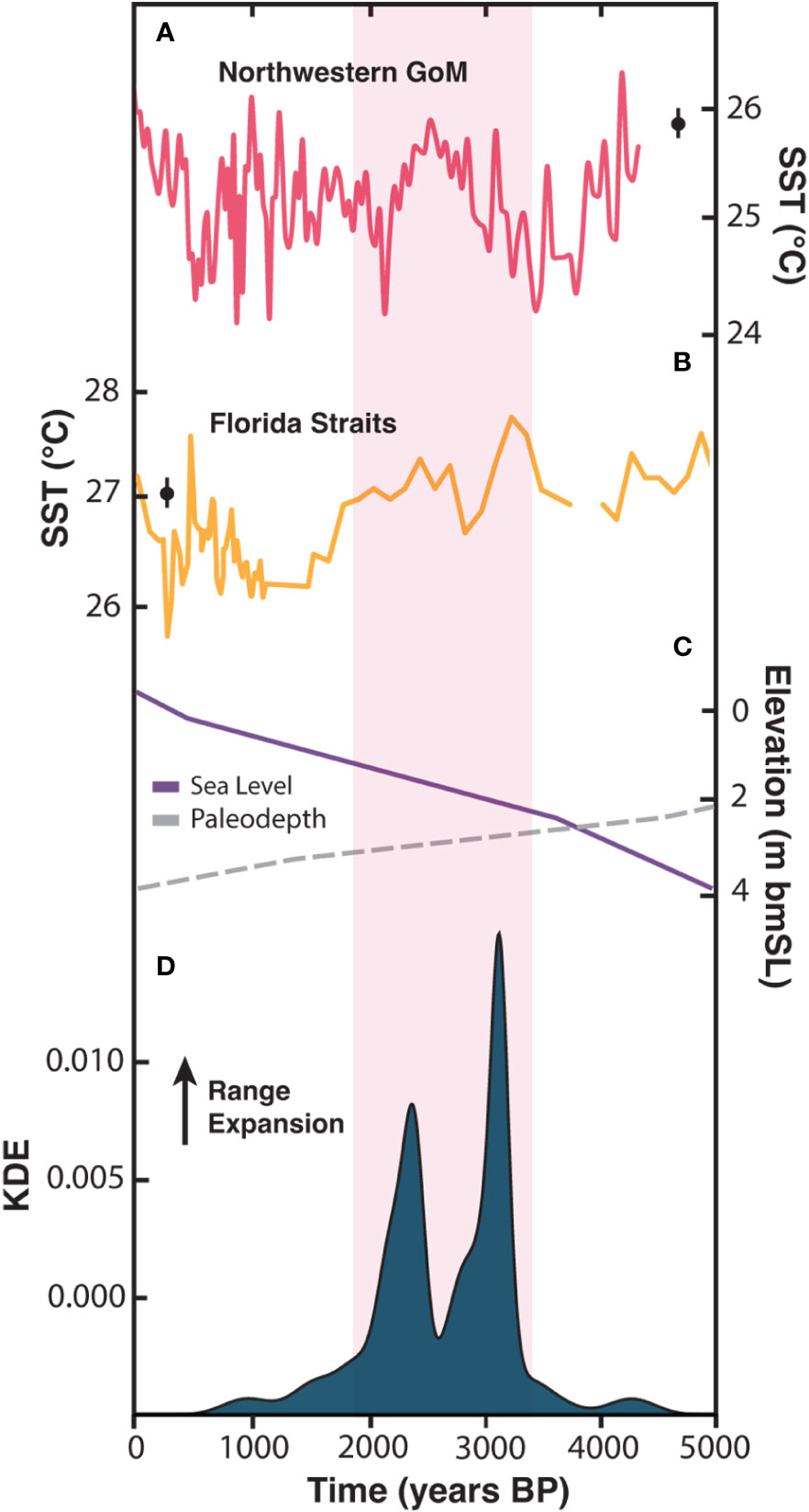
Figure 3 Comparison between late Holocene reef growth on the nearshore ridge complex (NRC) off southeast Florida, sea level, and sea-surface temperature (SST) variability in the subtropical western Atlantic. SST reconstructions from (A) the Garrison Basin in northwestern Gulf of Mexico (GoM) [Thirumalai et al., 2021] and (B) the Florida Straits (SST composite created from records near the Dry Tortugas [Lund and Curry, 2004] by Thirumalai et al., 2021). Modern observations of mean-annual SST at each location are indicated by black dot with error bars (error bars indicate standard deviation over 1970—2010 CE; SST was taken from HadISST, Rayner et al., 2003). (C) Estimated late Holocene relative sea level from Khan et al., 2017 and calculated paleo-water depths (see Supplementary material). (D) Kernel Density estimate (KDE) distribution of the 40 U-Th ages obtained from Acropora palmata and Orbicella spp. samples at study site. Pink shaded bar shows relative warming in both records and overlap with reef growth.
Because of its proximity to the continental shelf, the Florida Current exerts a significant moderating effect on ocean temperatures off southeast Florida, especially after the passage of strong winter cold fronts. For example, in 2010, Florida’s reefs experienced one of the most severe cold events on record, with water temperatures throughout the Florida Keys plummeting below the lower thermal limits of most coral species (<16°C) for several consecutive weeks (Lirman et al., 2011; Kemp et al., 2016). However, the nearshore and offshore reefs in southeast Florida never experienced lethal temperatures below 16°C (SECREMP, 2011) despite colder air temperatures than in the Florida Keys. As a result, cold-water bleaching and mortality were not reported north of Miami, including on the shallowest segments of the NRC where interaction with inlet outflow results in the greatest heat flux (Lirman et al., 2011). We hypothesize that centennial-scale expansions of the AWP and associated warming of the Florida Current may have enhanced this buffering effect off southeast Florida during the late Holocene, allowing for the expansion of cold-sensitive A. palmata and Orbicella coral communities despite increased regional cold-front frequency and intensity.
Our U-Th dating results show that by ~1800 years B.P. A. palmata- and Orbicella-dominated reef communities were no longer present on the NRC off northern Broward County with the exception, perhaps, during a brief period at 975 years B.P. (indicated by a single A. palmata age). The regional extirpation of these corals corresponds to a long-term shift towards cooler SSTs throughout the North Atlantic (Wanner et al., 2008), including at the northern AWP sites in the Gulf of Mexico and Florida Straits (Figures 3A, B; Poore et al., 2004; Lund and Curry, 2004; Thirumalai et al., 2018; Thirumalai et al., 2021). Significantly cooler temperatures relative to 3500–2000 years B.P. may have diminished the warm water buffering effect of the Florida Current, thus limiting the ability of A. palmata and Orbicella spp. corals to persist in southeast Florida for the last ~1800 years. We hypothesize that high multidecadal SST variability during the past 1800 years likely limited the establishment and subsequent growth of A. palmata and Orbicella spp. communities on the NRC despite recurrent warm intervals. Not surprisingly, A. palmata and Orbicella spp. corals were absent on the NRC during the Little Ice Age (1450 to 1850 CE) when SSTs in the northern AWP region were punctuated by cooling as much as 2°C compared to instrumental records spanning between 1970 and 2010 CE (Richey et al., 2007; Thirumalai et al., 2018).
Sea level also plays a prominent role in the structural development of coral reefs by controlling the accommodation space provided as it rises (Neumann and Macintyre, 1985; Buddemeier and Hopley, 1988; Hubbard, 1988; Macintyre, 2007). When sea-level rise is in equilibrium with carbonate production, reef accretion is generally vertical; however, when it is higher or lower relative to carbonate production, vertical accretion is inhibited (Neumann and Macintyre, 1985). Based on reconstructed sea-level estimates for the western Atlantic region (Khan et al., 2017), paleodepths on the NRC off northern Broward County averaged ~1.9 m MSL between ~3300 and 1800 years B.P. (Figure 3C). Sea level increased just ~0.9 m at an average rate of only 0.7 m per 1000 years during this period, and vertical reef accretion was, therefore, negligible. This contrasts with the offshore mid-Holocene reefs that exhibited average accretion rates ranging between 2.53 and 5.34 m per thousand years when sea-level rise was considerably faster (Lighty et al., 1978; Stathakopoulos and Riegl, 2015). Slower sea-level rise, shallow water depths, and the resulting lack of vertical accommodation space on the NRC likely explains the limited vertical reef accretion on the NRC during the late Holocene. Sea level, however, cannot explain the termination of A. palmata and Orbicella spp. coral communities on the NRC because it continued to rise – albeit slowly – throughout the late Holocene (Khan et al., 2017).
Although regional climate was likely the primary control on centennial-scale expansions of A. palmata and Orbicella spp. communities off southeast Florida during the late Holocene, several other factors have been shown to limit reef growth over broad spatial and temporal scales. For instance, high nutrient runoff and turbidity during climatic wet periods can inhibit reef growth by lowering coral calcification rates and enhancing the severity of disease outbreaks (Hallock and Schlager, 1986; van Woesik and Done, 1997; Bruno et al., 2003). However, hydroperiod reconstructions from the southeastern Everglades indicate a shift from wet to dry conditions at ~2800 years B.P., which does not correlate with the timing of coral community development or termination at our study site (Glaser et al., 2013). Increased rates of siliciclastic sedimentation have also been implicated as a limiting factor for high-latitude coral community development in southeast Florida (Smith et al., 1950), but there is no apparent mechanism or available record that would suggest appreciable localized changes in sedimentation over the <2000-year period represented by this study. Cold-water upwelling has also been shown to inhibit modern coral community development in southeast Florida; however, significant cold-water excursions (below 20°C) associated with upwelling are limited to the northernmost extent of the reef tract beyond 26.7°N (Walker et al., 2012b; Jones et al., 2020). Additional detailed proxy records are needed to fully resolve these factors, but we conclude that climate was likely the primary factor modulating coral community development off southeast Florida during the late Holocene.
Although our findings indicate persistence of reef-building coral assemblages in southeast Florida during late Holocene warm periods, there is still considerable uncertainty regarding whether the current rate of climate warming will result in a similar response. As climate change continues to accelerate, there is increasing evidence that extreme temperature events are also becoming more frequent, severe, and widespread (Stott, 2016), posing a substantial threat to subtropical reef communities existing near their thermal and environmental thresholds (Beger et al., 2014). Benthic temperature monitoring across the SFRT indicates that maximum summer temperatures are already regularly exceeding the bleaching threshold previously established for stony coral communities in the western Atlantic (Jones et al., 2020; Manzello et al., 2007). As a result, coral bleaching and disease outbreaks are increasing in frequency and severity throughout the region, with some of the most dramatic declines recently observed during the 2015−16 El Nino Southern Oscillation (Walton et al., 2018; Jones et al., 2020). Since then, coral losses have continued largely due to an associated outbreak of stony coral tissue loss disease, which has recently decimated coral populations throughout the western Atlantic (Precht et al., 2016; Walton et al., 2018; Alvarez-Filip et al., 2019). Although rising ocean temperatures may have facilitated long-term range expansions of cold-sensitive coral taxa during late-Holocene warm periods, it is uncertain whether the current warming rate will support a similar response given the suite of other disturbances that coral reefs are now facing.
Conclusions
Our findings provide new evidence for an expanded period of high-latitude Acropora- and Orbicella-dominated coral community development on the NRC off northern Broward County ~1500 years after the shutdown of reef accretion on the adjacent offshore barrier reef. Despite post-HTM cooling in the North Atlantic and an atmospheric circulation pattern conducive to higher cold-front frequency in southeast Florida, A. palmata and Orbicella spp. coral communities persisted in the shallow waters off southeast Florida for over 2000 years. The timing of this expansion coincides with shorter term centennial-scale warming relative to present at the northern extent of the AWP. We suggest that shorter-term warming and expansion of the AWP likely facilitated the expansion of cold-sensitive coral communities on the NRC by modulating local SSTs via the Florida Current. This warm water buffering effect is currently observed on the modern reef tract, however, would have greatly diminished after ~2000 years B.P. when SSTs in the AWP became highly variable with frequent cold-water excursions. As a result, A. palmata and Orbicella spp. coral community development terminated on the NRC off northern Broward County after ~1800 years B.P. and has been absent ever since.
Given the high temporal resolution of our study and the higher climate variability at the time, we suggest that the expansion and persistence of A. palmata and Orbicella spp. coral communities off southeast Florida during the late Holocene may provide a more comparable analogue for current and future trends compared with records from the mid-Holocene. Projected climate change is not only expected to result in continued ocean warming throughout the North Atlantic, but also amplify winter extremes over the North American continent (Kodra et al., 2011; Cohen et al., 2021). Our results suggest that contemporaneous warming in the northern AWP region may facilitate continued expansion and persistence of cold-sensitive coral communities on the nearshore reefs off southeast Florida despite worsening winter climate conditions like during the late Holocene; however, this will depend on the rate of modern warming and the impacts of recent disease outbreaks and other anthropogenic disturbances.
Data availability statement
The original contributions presented in the study are included in the article/Supplementary Material. Further inquiries can be directed to the corresponding author.
Author contributions
AM designed the study, analyzed the data, and wrote the manuscript. RM conducted radiometric dating. AM and AO completed the fieldwork. All authors contributed to study design and edited/approved the final manuscript. All authors contributed to the article and approved the submitted version.
Funding
Funding for the study was provided in part by a “Protect our Reefs” research grant awarded by Mote Marine Laboratory to AEO [POR-2014-3] and a graduate research grant awarded to ABM by the American Academy of Underwater Sciences (AAUS). This research was also supported by the USGS Climate Research and Development Program and Coastal/Marine Hazards and Resources Program. Any use of trade, firm, or product names is for descriptive purposes only and does not imply endorsement by the U.S. Government.
Acknowledgments
We thank Claudio Zuccarelli, Caitlin Hanley, Rob Carroll, Jacob Russell, and Scott Hurwood for their support in the field and Thomas Beasley at FIU for his assistance with SEM. All samples were collected under a Florida Fish and Wildlife Conservation Commission (FWC) Special Activity License (SAL-18-1659A-SRP) and with permission from Broward County Environmental Protection & Growth Management.
Conflict of interest
WP was employed by Dial Cordy & Associates, Inc., an environmental consulting firm. His participation in the research was independent and unrelated to his work at Dial Cordy & Associates, Inc.
The remaining authors declare that the research was conducted in the absence of any commercial or financial relationships that could be construed as a potential conflict of interest.
Publisher’s note
All claims expressed in this article are solely those of the authors and do not necessarily represent those of their affiliated organizations, or those of the publisher, the editors and the reviewers. Any product that may be evaluated in this article, or claim that may be made by its manufacturer, is not guaranteed or endorsed by the publisher.
Supplementary material
The Supplementary Material for this article can be found online at: https://www.frontiersin.org/articles/10.3389/fmars.2022.995256/full#supplementary-material
References
Alvarez-Filip L., Estrada-Saldívar N., Pérez-Cervantes E., Molina-Hernández A., González-Barrios F. J. (2019). A rapid spread of the stony coral tissue loss disease outbreak in the Mexican Caribbean. PeerJ 7, e8069. doi: 10.7717/peerj.8069
Aronson R. B., Precht W. F. (2001). 9. Evolutionary Paleoecology of Caribbean Coral Reefs. In Evolutionary paleoecology (pp. 171–234). Columbia University Press.
Aronson R. B., Precht W. F. (2006). Conservation, precaution, and Caribbean reefs. Coral reefs 25 (3), 441–450. doi: 10.1007/s00338-006-0122-9
Baird A. H., Sommer B., Madin J. S. (2012). Pole-ward range expansion of Acropora spp. along the east coast of Australia. Coral Reefs 31 (4), 1063–1063. doi: 10.1007/s00338-012-0928-6
Banks K. W., Riegl B. M., Shinn E. A., Piller W. E., Dodge. R. E. (2007). Geomorphology of the southeast Florida continental reef tract (Miami-Dade, broward, and palm beach counties, USA. Coral Reefs 26, 617–633. doi: 10.1007/s00338-007-0231-0
Beger M., Sommer B., Harrison P. L., Smith S. D., Pandolfi J. M. (2014). Conserving potential coral reef refuges at high latitudes. Diversity Distribut. 20 (3), 245–257. doi: 10.1111/ddi.12140
Blair S. M., Flynn B. S. (1989). Biological monitoring of hard bottom reef communities off Dade County Florida: community description. In Land M., Jaap W. (eds), Proc Am Ac Underw Sci (9th Ann Sci Diving Symp, Woods Hole, MA). 9–24.
Bruno J. F., Côté I. M., Toth L. T. (2019). Climate change, coral loss, and the curious case of the parrotfish paradigm: why don't marine protected areas improve reef resilience? Annu. Rev. Mar. Sci. 11, 307–334. doi: 10.1146/annurev-marine-010318-095300
Bruno J. F., Petes L. E., Drew Harvell C., Hettinger A. (2003). Nutrient enrichment can increase the severity of coral diseases. Ecol. Lett. 6 (12), 1056–1061. doi: 10.1046/j.1461-0248.2003.00544.x
Bruno J. F., Selig E. R. (2007). Regional decline of coral cover in the indo-pacific: timing, extent, and subregional comparisons. PloS One 2 (8), e711. doi: 10.1371/journal.pone.0000711
Bruno J. F., Selig E. R., Casey K. S., Page C. A., Willis B. L., Harvell C. D., et al. (2007). Thermal stress and coral cover as drivers of coral disease outbreaks. PloS Biol. 5 (6), e124. doi: 10.1371/journal.pbio.0050124
Buddemeier R. W., Hopley D. (1988). Turn-ons and turn-offs: cause and mechanisms of the initiation and termination of coral reef growth. Proc. 6th Int. Coral Reef Symposium (Australia) 1, 253–261.
Burman S. G., Aronson R. B., van Woesik R. (2012). Biotic homogenization of coral assemblages along the Florida reef tract. Mar. Ecol. Prog. Ser. 467, 89–96. doi: 10.3354/meps09950
Clark T. R., Roff G., Zhao J. X., Feng Y. X., Done T. J., McCook L. J., et al. (2017). U-Th Dating reveals regional-scale decline of branching A. palmata corals on the great barrier reef over the past century. Proc. Natl. Acad. Sci. 114 (39), 10350–10355. doi: 10.1073/pnas.1705351114
Cohen J., Agel L., Barlow M., Garfinkel C. I., White I. (2021). Linking Arctic variability and change with extreme winter weather in the united states. Science 373 (6559), 1116–1121. doi: 10.1126/science.abi9167
D’Antonio N. L., Gilliam D. S., Walker B. K. (2016). Investigating the spatial distribution and effects of nearshore topography on Acropora cervicornis abundance in southeast Florida. PeerJ 4, e2473. doi: 10.7717/peerj.2473
Domingues R., Goni G., Baringer M., Volkov D. (2018). What caused the accelerated sea level changes along the US East coast during 2010–2015? Geophys. Res. Lett. 45 (24), 13–367. doi: 10.1029/2018GL081183
Edmunds P. J. (2015). A quarter-century demographic analysis of the Caribbean coral, orbicella annularis, and projections of population size over the next century. Limnol. Oceanog. 60 (3), 840–855. doi: 10.1002/lno.10075
Frys C., Saint-Amand A., Le Hénaff M., Figueiredo J., Kuba A., Walker B., et al. (2020). Fine-scale coral connectivity pathways in the Florida reef tract: implications for conservation and restoration. Front. Mar. Sci. 7, 312. doi: 10.3389/fmars.2020.00312
Gardner T. A., Côté I. M., Gill J. A., Grant A., Watkinson A. R. (2003). Long-term region-wide declines in Caribbean corals. science 301 (5635), 958–960. doi: 10.1126/science.1086050
Glaser P. H., Hansen B. C., Donovan J. J., Givnish T. J., Stricker C. A., Volin J. C. (2013). Holocene Dynamics of the Florida Everglades with respect to climate, dustfall, and tropical storms. Proc. Natl. Acad. Sci. 110 (43), 17211–17216. doi: 10.1073/pnas.1222239110
Goldberg W. M. (1973). The ecology of the coral-octocoral communities off the southeast Florida coast: geomorphology, species composition, and zonation. Bull. Mar. Sci. 23 (3), 465–488.
Goreau T. J., Hayes R. L. (2021). Global warming triggers coral reef bleaching tipping point. Ambio, 50 (6), 1137–1140. doi: 10.1007/s13280-021-01512-2
Greenstein B. J., Pandolfi J. M. (2008). Escaping the heat: range shifts of reef coral taxa in coastal Western Australia. Global Change Biol. 14 (3), 513–528. doi: 10.1111/j.1365-2486.2007.01506.x
Hallock P., Schlager W. (1986). Nutrient excess and the demise of coral reefs and carbonate platforms. Palaios 1 (4), 389–398. doi: 10.2307/3514476
Hammerman N. M., Roff G., Lybolt T., Eyal G., Pandolfi J. M. (2022). Unraveling moreton bay reef history: An urban high-latitude setting for coral development. Front. Ecol. Evol. 10, 884850. doi: 10.3389/fevo.2022.884850
Hoegh-Guldberg O., Jacob D., Bindi M., Brown S., Camilloni I., Diedhiou A., et al. (2018). Impacts of 1.5°C Global Warming on Natural and Human Systems. In: Global Warming of 1.5°C. An IPCC Special Report on the impacts of global warming of 1.5°C above pre-industrial levels and related global greenhouse gas emission pathways, in the context of strengthening the global response to the threat of climate change, sustainable development, and efforts to eradicate poverty [Masson-Delmotte V., Zhai P., Pörtner H.-O., Roberts D., Skea J., Shukla P.R., et al (eds.)]. In Press.
Hoegh-Guldberg O., Poloczanska E. S., Skirving W., Dove S. (2017). Coral reef ecosystems under climate change and ocean acidification. Front. Mar. Sci. 4, 158. doi: 10.3389/fmars.2017.00158
Hubbard D. K. (1988). Controls of modern and fossil reef development: common ground for biological and geological research. In Proc. 6th Int. Coral Reef Symp, Townsville, Australia, (Vol. 1, pp. 243–252).
Hudson J. H., Shinn E. A., Halley R. B., Lidz B. (1976). Sclerochronology: a tool for interpreting past environments. Geology 4 (6), 361–364. doi: 10.1130/0091-7613(1976)4<361:SATFIP>2.0.CO;2
Jaap W. C. (1984). Ecology of the south Florida coral reefs: a community profile (No. FWS/OBS-82/08; MMS-84-0038) (St. Petersburg (USA: Florida Dept. of Natural Resources). Marine Research Lab.
Jackson J., Donovan M., Cramer K., Lam V. (2014). Status and trends of Caribbean coral reefs (Switzerland: Gland: Global Coral Reef Monitoring Network, IUCN, Gland), 1970–2012.
Jones N. P., Figueiredo J., Gilliam D. S. (2020). Thermal stress-related spatiotemporal variations in high-latitude coral reef benthic communities. Coral Reefs 39 (6), 1661–1673. doi: 10.1007/s00338-020-01994-8
Kemp D. W., Colella M. A., Bartlett L. A., Ruzicka R. R., Porter J. W., Fitt W. K. (2016). Life after cold death: reef coral and coral reef responses to the 2010 cold water anomaly in the Florida keys. Ecosphere 7 (6), 1–17. doi: 10.1002/ecs2.1373
Kennedy E. V., Perry C. T., Halloran P. R., Iglesias-Prieto R., Schönberg C. H., Wisshak M., et al. (2013). Avoiding coral reef functional collapse requires local and global action. Curr. Biol. 23 (10), 912–918. doi: 10.1016/j.cub.2013.04.020
Khan N. S., Ashe E., Horton B. P., Dutton A., Kopp R. E., Brocard G., et al. (2017). Drivers of Holocene sea-level change in the Caribbean. Quatern. Sci. Rev. 155, 13–36. doi: 10.1016/j.quascirev.2016.08.032
Kodra E., Steinhaeuser K., Ganguly A. R. (2011). Persisting cold extremes under 21st-century warming scenarios. Geophys. Res. Lett. 38 (8), 1–5. doi: 10.1029/2011GL047103
Kuffner I. B., Toth L. T. (2016). A geological perspective on the degradation and conservation of western Atlantic coral reefs. Conserv. Biol. 30 (4), 706–715. doi: 10.1111/cobi.12725
Lee T. N., Leaman K., Williams E., Berger T., Atkinson L. (1995). Florida Current meanders and gyre formation in the southern straits of Florida. J. Geophys. Res.: Oceans 100 (C5), 8607–8620. doi: 10.1029/94JC02795
Leonard N. D., Lepore M. L., Zhao J. X., Rodriguez-Ramirez A., Butler I., Clark T. R., et al. (2020). AU-Th dating approach to understanding past coral reef dynamics and geomorphological constraints on future reef growth potential; mazie bay, southern great barrier reef. Paleoceanog. Paleoclimatol. 35 (2), e2019PA003768. doi: 10.1029/2019PA003768
Lidz B. H., Reich C. D., Shinn E. A. (2003). Regional quaternary submarine geomorphology in the Florida keys. GSA Bull. 115 (7), 845–866. doi: 10.1130/0016-7606(2003)115<0845:RQSGIT>2.0.CO;2
Lighty R. G., Macintyre I. G., Stuckenrath. R. (1978). Submerged early Holocene barrier reef south-east Florida shelf. Nature 275, 59–60. doi: 10.1038/276059a0
Lirman D., Schopmeyer S., Manzello D., Gramer L. J., Precht W. F., Muller-Karger F., et al. (2011). Severe 2010 cold-water event caused unprecedented mortality to corals of the Florida reef tract and reversed previous survivorship patterns. PloS One 6 (8), e23047. doi: 10.1371/journal.pone.0023047
Lund D. C., Curry W. B. (2004). Late Holocene variability in Florida current surface density: Patterns and possible causes. Paleoceanography 19 (4), 1–17. doi: 10.1029/2004PA001008
Macintyre I. G. (2007). “Demise, regeneration, and survival of some western Atlantic reefs during the Holocene transgression,” in Geological approaches to coral reef ecology (New York, NY: Springer), 181–200.
Makino A., Yamano H., Beger M., Klein C. J., Yara Y., Possingham H. P. (2014). Spatio-temporal marine conservation planning to support high-latitude coral range expansion under climate change. Diversity Distributions 20 (8), 859–871. doi: 10.1111/ddi.12184
Manzello D. P., Berkelmans R., Hendee J. C. (2007). Coral bleaching indices and thresholds for the Florida reef tract, Bahamas, and st. Croix, US virgin islands. Mar. pollut. Bull. 54 (12), 1923–1931. doi: 10.1016/j.marpolbul.2007.08.009
Marcott S. A., Shakun J. D., Clark P. U., Mix. A. C. (2013). A reconstruction of regional and global temperature for the past 11,300 years. Science 339, 1198–1201. doi: 10.1126/science.1228026
Mellin C., Aaron MacNeil M., Cheal A. J., Emslie M. J., Julian Caley M. (2016). Marine protected areas increase resilience among coral reef communities. Ecol. Lett. 19 (6), 629–637. doi: 10.1111/ele.12598
Moyer R. P., Riegl B., Banks K., Dodge R. E. (2003). Spatial patterns and ecology of benthic communities on a high-latitude south Florida (Broward county, USA) reef system. Coral Reefs 22 (4), 447–464. doi: 10.1007/s00338-003-0334-1
Muir P. R., Wallace C. C., Done T., Aguirre J. D. (2015). Limited scope for latitudinal extension of reef corals. Science 348 (6239), 1135–1138. doi: 10.1126/science.1259911
Neumann A. C., Macintyre I. (1985). Reef response to sea level rise: keep-up, catch-up or give-up. Proc. 5th Int. Coral Reef Congress 3, 105–110.
Pandolfi J. M. (2011). “The paleoecology of coral reefs,” in Coral reefs: an ecosystem in transition (Dordrecht: Springer), 13–24.
Poore R. Z., Quinn T. M., Verardo S. (2004). Century-scale movement of the Atlantic intertropical convergence zone linked to solar variability. Geophys. Res. Lett. 31 (12), 1–4. doi: 10.1029/2004GL019940
Precht W. F., Aronson R. B. (2004). Climate flickers and range shifts of reef corals. Front. Ecol. Environ. 2 (6), 307–314. doi: 10.1890/1540-9295(2004)002[0307:CFARSO]2.0.CO;2
Precht W. F., Aronson R. B. (2006). Death and resurrection of Caribbean coral reefs: a palaeoecological perspective. Conserv. Biol. Series-Cambridge 13, 40. doi: 10.1017/CBO9780511804472.004
Precht W. F., Deslarzes K. J., Hickerson E. L., Schmahl G. P., Nuttall M. F., Aronson R. B. (2014). Back to the future: the history of acroporid corals at the flower garden banks, gulf of Mexico, USA. Mar. Geol. 349, 152–161. doi: 10.1016/j.margeo.2013.12.012
Precht W. F., Gintert B. E., Robbart M. L., Fura R., Van Woesik R. (2016). Unprecedented disease-related coral mortality in southeastern Florida. Sci. Rep. 6 (1), 1–11. doi: 10.1038/srep31374
Precht W. F., Miller S. L. (2007). “Ecological shifts along the Florida reef tract: the past as a key to the future,” in Geological approaches to coral reef ecology. Ed. Aronson R. B. (New York, NY: Springer), 237–312.
Precht W. F., Macintyre I. G., Banks K., Fisher L. E. (2000). Backstepping of Holocene reefs along Florida's east coastin Proceedings of the 9th International Coral Reef Symposium, Bali, Indonesia. 23–27pp.
Randall C. J., van Woesik R. (2015). Contemporary white-band disease in Caribbean corals driven by climate change. Nat. Climate Change 5 (4), 375–379. doi: 10.1038/nclimate2530
Raymond W. F. (1972). A geologic investigation of the offshore sands and reefs of broward county, Florida (Florida State University, Tallahassee, Florida).
Rayner N. A. A., Parker D. E., Horton E. B., Folland C. K., Alexander L. V., Rowell D. P., et al. (2003). Global analyses of sea surface temperature, sea ice, and night marine air temperature since the late nineteenth century. Journal of Geophysical Research: Atmospheres, 108(D14). doi: 10.1029/2002JD002670
Richey J. N., Poore R. Z., Flower B. P., Quinn T. M. (2007). 1400 years multiproxy record of climate variability from the northern gulf of Mexico. Geology 35 (5), 423–426. doi: 10.1130/G23507A.1
Roberts H. H., Rouse L. J., Walker N. D., Hudson J. H. (1982). Cold-water stress in Florida bay and northern bahamas; a product of winter cold-air outbreaks. J. Sediment. Res. 52 (1), 145–155. doi: 10.1306/212F7EFA-2B24-11D7-8648000102C1865D
Serrano E., Coma R., Ribes M., Weitzmann B., Garcia M., Ballesteros E. (2013). Rapid northward spread of a zooxanthellate coral enhanced by artificial structures and sea warming in the western Mediterranean. PloS One 8 (1), e52739. doi: 10.1371/journal.pone.0052739
Smith F. W. (1943). LITTORAL FAUNA OF THE MIAMI AREA. I THE MADREPORAPIA. In Proceedings of the Florida Academy of Sciences. 6 (1), 41–48.
Smith F. W., Williams R. H., Davis C. C. (1950). An ecological survey of the subtropical inshore waters adjacent to Miami. Ecology 119-146, 1943. doi: 10.2307/1931366
Stathakopoulos A., Riegl B. M. (2015). Accretion history of mid-Holocene reefs from the southeast Florida continental reef tract, USA. Coral Reefs 34, 173–187. doi: 10.1007/s00338-014-1233-3
Stott P. (2016). How climate change affects extreme weather events. Science 352 (6293), 1517–1518. doi: 10.1126/science.aaf7271
Thirumalai K., Quinn T. M., Okumura Y., Richey J. N., Partin J. W., Poore R. Z., et al. (2018). Pronounced centennial-scale Atlantic ocean climate variability correlated with Western hemisphere hydroclimate. Nat. Commun. 9 (1), 1–11. doi: 10.1038/s41467-018-02846-4
Thirumalai K., Richey J. N., Quinn T. M. (2021). Holocene Evolution of Sea-surface temperature and salinity in the gulf of Mexico. Paleoceanog. Paleoclimatol. 36 (8), e2021PA004221. doi: 10.1029/2021PA004221
Toth L. T., Precht W. F., Modys A. B., Stathakopoulos A., Robbart M. L., Hudson J. H., et al. (2021). Climate and the latitudinal limits of subtropical reef development. Sci. Rep. 11 (1), 1–15. doi: 10.1038/s41598-021-87883-8
Tuckett C. A., De Bettignies T., Fromont J., Wernberg T. (2017). Expansion of corals on temperate reefs: direct and indirect effects of marine heatwaves. Coral Reefs 36 (3), 947–956. doi: 10.1007/s00338-017-1586-5
van Woesik R., Done T. J. (1997). Coral communities and reef growth in the southern great barrier reef. Coral Reefs 16 (2), 103–115. doi: 10.1007/s003380050064
Vargas-Ángel B., Thomas J. D., Hoke S. M. (2003). High-latitude Acropora cervicornis thickets off fort Lauderdale, Florida, USA. Coral Reefs 22 (4), 465–473. doi: 10.1007/s00338-003-0336-z
Vaughan T. W. (1914). Investigations of the geology and geologic processes of the reef tracts and adjacent areas in the Bahamas and Florida. Carnegie Inst Wash Yb 12, 183.
Vermeesch P. (2018). IsoplotR: A free and open toolbox for geochronology. Geosci. Front. 9 (5), 1479–1493. doi: 10.1016/j.gsf.2018.04.001
Walker B. K. (2012a). Spatial analyses of benthic habitats to define coral reef ecosystem regions and potential biogeographic boundaries along a latitudinal gradient. PloS One 7 (1), e30466. doi: 10.1371/journal.pone.0030466
Walker B. K., Larson E. A., Moulding A. L., Gilliam D. S. (2012b). Small-scale mapping of indeterminate arborescent acroporid coral (Acropora cervicornis) patches. Coral Reefs 31 (3), 885–894. doi: 10.1007/s00338-012-0910-3
Walton C. J., Hayes N. K., Gilliam D. S. (2018). Impacts of a regional, multi-year, multi-species coral disease outbreak in southeast Florida. Front. Mar. Sci. 5, 323. doi: 10.3389/fmars.2018.00323
Wang C., Enfield D. B. (2001). The tropical Western hemisphere warm pool. Geophys. Res. Lett. 28 (8), 1635–1638. doi: 10.1029/2000GL011763
Wang C., Lee S. K., Enfield D. B. (2008). Climate response to anomalously large and small Atlantic warm pools during the summer. J. Climate 21 (11), 2437–2450. doi: 10.1175/2007JCLI2029.1
Wanner H., Beer J., Bütikofer J., Crowley T. J., Cubasch U., Flückiger J., et al. (2008). Mid-to late Holocene climate change: an overview. Quatern. Sci. Rev. 27 (19-20), 1791–1828. doi: 10.1016/J.QUASCIREV.2008.06.013
Yamano H., Sugihara K., Nomura K. (2011). Rapid poleward range expansion of tropical reef corals in response to rising sea surface temperatures. Geophys. Res. Lett. 38 (4), 1–6. doi: 10.1029/2010GL046474
Keywords: coral reefs, climate change, coral range expansion, late Holocene, Florida, southeast Florida reef tract
Citation: Modys AB, Oleinik A, Mortlock RA, Toth LT and Precht WF (2022) Climate-modulated range expansion of reef-building coral communities off southeast Florida during the late Holocene. Front. Mar. Sci. 9:995256. doi: 10.3389/fmars.2022.995256
Received: 15 July 2022; Accepted: 04 November 2022;
Published: 06 December 2022.
Edited by:
Hajime Kayanne, The University of Tokyo, JapanReviewed by:
Chuki Hongo, Wakayama Prefectural Nanki Kumano Geopark Center, JapanClark Sherman, University of Puerto Rico at Mayagüez, Puerto Rico
Copyright © 2022 Modys, Oleinik, Mortlock, Toth and Precht. This is an open-access article distributed under the terms of the Creative Commons Attribution License (CC BY). The use, distribution or reproduction in other forums is permitted, provided the original author(s) and the copyright owner(s) are credited and that the original publication in this journal is cited, in accordance with accepted academic practice. No use, distribution or reproduction is permitted which does not comply with these terms.
*Correspondence: Alexander B. Modys, YW1vZHlzQGdtYWlsLmNvbQ==