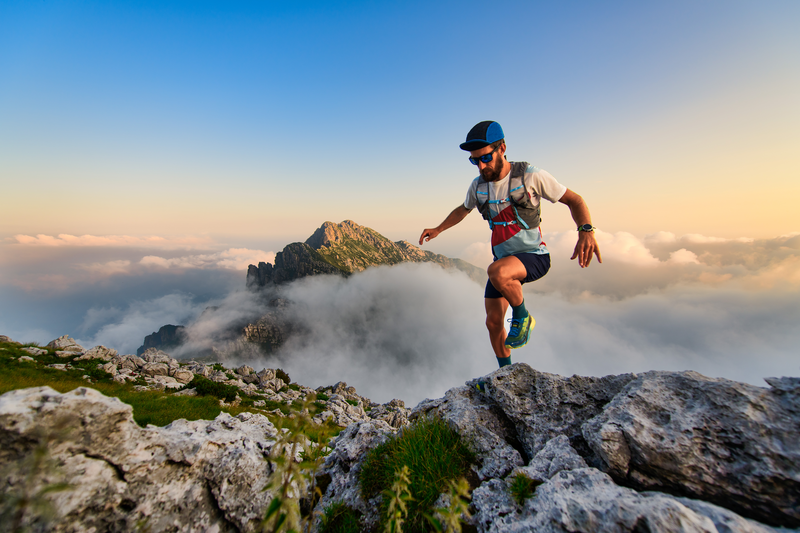
95% of researchers rate our articles as excellent or good
Learn more about the work of our research integrity team to safeguard the quality of each article we publish.
Find out more
ORIGINAL RESEARCH article
Front. Mar. Sci. , 29 September 2022
Sec. Marine Ecosystem Ecology
Volume 9 - 2022 | https://doi.org/10.3389/fmars.2022.993202
This article is part of the Research Topic Degradation, Ecological Restoration and Adaptive Management of Estuarine Wetlands under Intensifying Global Changes View all 20 articles
Coastal wetlands serve as sources and sinks of carbon, nitrogen, phosphorus, and sulfur, and their ecological stoichiometry intuitively indicates the biogeochemical cycle process of the region. This study investigated the changing trend of the contents of soil organic carbon (i.e., SOC), total nitrogen (i.e., TN), total phosphorus (i.e., TP), and total sulfur (i.e., TS) and their ecological stoichiometric ratios in 2019-2021 in the Yellow River Delta (including north and south banks) under the Internationally Important Wetland Biodiversity Conservation Project by conducting field surveys and experiments. The results showed that SOC, TN, and TP showed the highest content in the North Unrestoration, while the TS content appeared highest in the North Restoration. In addition, ecological restoration improved the biogenic element stability in both banks and improved the stability of ecological stoichiometry on the north bank while decreasing the ecological stoichiometry stability on the south bank. Notably, the changing trend of soil C/S in the North Unrestoration exhibited a considerably different profile similarity from the North Restoration, which indicates that the ecological restoration changed the wetland ecosystem from the perspective of soil C/S. Furthermore, the high content of soil biogenic elements SOC and high ratio of soil ecological stoichiometry C/N and C/S in the Yellow River Delta induce that they are more sensitive to environmental change. Over the three years, the contributions of soil moisture content, soil salinity, and pH to soil biogenic element contents and their stoichiometric ratios were 32.20%, 49.30%, and 18.50% on the north bank, respectively, and 85.70%, 8.50%, and 5.80% on the south bank, respectively. This study implies that ecological restoration generally has a positive effect on the soil biogenic element contents and their ecological stoichiometry in the Yellow River Delta and provides a reference for delta restoration.
Coastal wetlands are one of the most valuable, fragile, and highly productive ecosystems and play an important role in adjusting the global biogeochemical cycles of biogenic elements (Bai et al., 2012; Lu et al., 2018; Du et al., 2022). However, coastal wetlands have been significantly affected by climate change and intensive anthropogenic activities in recent years and have attracted extensive attention globally (Syvitski et al., 2009; Giosan et al., 2014; Nicholls, 2020). Therefore, many ecological restoration efforts have been conducted all over the world, and their effects are considerable (Gao et al., 2010; Couvillion et al., 2013; Xu et al., 2020; Liu et al., 2021). As one of the youngest coastal wetlands, the Yellow River Delta has faced significant dangers (e.g., wetland salinization, wetland degradation and shrinkage, biodiversity decrease) from climate change and human activities in recent years (Lu et al., 2018). More than ten big ecological restoration projects have been performed in the Yellow River Delta since 2020, including Suaeda salsa restoration, Spartina alterniflora removal, hydrological connectivity enhancement, etc. (Liu et al., 2018; Zhao et al., 2020; Jiang et al., 2021; Ning et al., 2021). Given the critical role of the Yellow River Delta, understanding the effects of ecological restoration on the soil biogenic elements and ecological stoichiometry becomes an imperative task.
In general, the number of ecological restorations steadily increased from 1992 to 2014, and the total cost of the projects continued to increase in China (Liang et al., 2018; Li et al., 2019b). Notably, the outcome of ecological restorations is usually unclear, and the effectiveness of the ecological restoration measures is opaque (Li et al., 2019b). Soil organic carbon storage could be decreased by 108-250 million metric tons under a “future-without-action” in Louisiana’s wetland (Couvillion et al., 2013). In addition, 35%-75% of the soil organic carbon could be offset if the projects (397 different projects) had been completed (Couvillion et al., 2013). The phosphorus storage capacity increased with increasing time since wetland restoration (freshwater replenishment) in the Yellow River Delta (Xu et al., 2020). However, the risk of losing phosphorus from the sediments decreased with increasing time (Xu et al., 2020). The implementation of wetland ecological restoration (removal of reeds at harvest) in 2005 in the Yellow River Delta decreased the soil pH, electric conductivity, soil organic matter, total nitrogen, and total sulfur while increasing the total phosphorous and potassium (Gao et al., 2010).
The stoichiometric approach is a useful tool for examining the fluxes of multiple elements and their ratios to deeply understand ecosystem processes (Ptacnik et al., 2005). For instance, soil C/N is a typical symbol of soil quality (Elser et al., 2003). The soil C/P and C/S are sensitive indicators for the mineralization ability of phosphorus and sulfur (Du et al., 2022). In addition, the soil N/P is usually used as an indicator of biogenic element restriction type (Zhang et al., 2012b; Cao et al., 2015; Du et al., 2022). Coastal ecological restoration, as a combined natural and anthropogenic activity, alters the soil ecological stoichiometry (Zeng et al., 2017; Guo et al., 2018). The plant recovery project in Fujian decreased soil C/N (Guo et al., 2018). In addition, the soli C/N decreased, while the soil C/P and soil N/P increased after the Caragana korshinskii recovery project on the Loess Plateau of China (Zeng et al., 2017). The “Redfield-like” ratios of C/N/P stoichiometric ratios did not exist in the wetland soil of the Yellow River Delta (Qu et al., 2014). To date, the majority of the existing studies have focused on changes in coastal soil biogenic elements and ecological stoichiometry for different soil profiles or restored sites (Lu et al., 2018; Zhang et al., 2021b; Du et al., 2022), while the effects of ecological restoration projects need to be clarified, especially for a specific project.
In this study, we examined the effects of a special ecological restoration project (The Internationally Important Wetland Biodiversity Conservation Project, hereafter IIWBCP) on the soil organic carbon (hereafter, SOC), total nitrogen (hereafter, TN), total phosphorus (hereafter, TP), total sulfur (hereafter, TS), carbon-nitrogen ratio (hereafter, C/N), carbon-phosphorus ratio (hereafter, C/P), carbon-sulfur ratio (hereafter, C/S), and nitrogen-phosphorus ratio (hereafter, N/P) in 2019-2021 on the north and south banks of the Yellow River Delta. Notably, we chose the unrestored sites in both banks (i.e., the north and south banks of the Yellow River Delta) to exclude the effects of other factors and better compare the effects of ecological restoration. We addressed the following questions: (1) does ecological restoration improve conditions of soil biogenic elements and ecological stoichiometry, and (2) what is the possible potential mechanism of ecological restoration? This study provides a scientific basis for determining the effects of coastal ecological restoration on biogenic elements and ecological stoichiometry.
The study area is located in the Nature Reserve of the Yellow River Delta (37°35′N~38°12′N, 118°33′E~119°20′E), which is located in northeastern Shandong Province and is surrounded by Bohai Bay and Laizhou Bay (Zhao et al., 2020). In addition, it is one of the most complete, extensive, and youngest coastal wetland ecosystems in the warm temperate zone (Bai et al., 2012). The rainy season of the Yellow River Delta is from June to August, and this region has a warm-temperate and continental monsoon climate (Qu et al., 2014; Lu et al., 2018). The annual average temperature in the Yellow River Delta is 12.1°C, and the average annual rainfall and evaporation are 551.6 mm and 1962 mm, respectively (Lu et al., 2018). Due to the negative impacts of the intensive human activities (e.g., reclamation and construction of coastal defence) and local environmental changes (e.g., drought and land use change), the loss and degradation of coastal wetlands in the Yellow River Delta has become a major issue (Wang et al., 2012). Therefore, the vulnerable ecological environments of the Yellow River Delta and their restorations have attracted extensive attention (Bai et al., 2012; Zhang et al., 2021a).
The soil biogenic elements SOC, TN, TP, and TS in the coastal wetlands of the Yellow River Delta mainly concentrates in the topsoil, and the values are approximately 1.10-22.80 g/kg (Xu et al., 2020), 0.08-1.64 g/kg (Jia et al., 2015; Xu et al., 2020), 0.46-0.65 g/kg (Xu et al., 2020; Du et al., 2022) and 0.11-0.54 g/kg (Lu et al., 2018), respectively. The biological stoichiometry of C/N, C/P, C/S, and N/P in the Yellow River Delta are 10-15, 2-10, 5-25, and 0-0.80, respectively (Du et al., 2022). In addition, the Yellow River Delta has the lowest formation time compared to other wetland ecosystems in China’s warm and humid zone (Jiang et al., 2021) and its soil N/P is much lower compared with the Sanjiang Plain, whose N/P ranges about 5.04-21.43 (Prusty et al., 2009; Zhang et al., 2012b; Cao et al., 2015). SOC is significantly negatively correlated with soil pH and positively correlated with water content in the Yellow River Delta (Zhao et al., 2020).
The IIWBCP in the Yellow River Delta was primarily designed to protect the biodiversity at the Dawenliu and Huanghekou Management Stations by adding freshwater, improving bird habitat, enhancing vegetation diversity and building sluices. The ecological restoration on the north bank mainly aims to replenish freshwater, while that on the south bank mainly aims to promote vegetation growth. This project was implemented on the north and south banks of the Yellow River Delta in 2019. In order to solve the sediment deposition issue in the wetland of the project area, the fresh water from the Yellow River enters the wetland after settling sand. The control gate joined the little portions of the wetland and allowed water to flow into the deep ditch and diversion canal in the wetland to modify the water level. The entire water system cycle is completed when the water passes through the drainage gate and onto the floodplain downstream. After the implementation of the ecological restoration for three years, the ecological conditions were significantly changed, especially the soil biogenic elements and their stoichiometry. To explore the effect of the ecological restoration on both banks of the Yellow River Delta, our study set 6 unrestored sites and 17 restored sites on the north bank, and 6 unrestored sites and 30 restored sites on the south bank (Figure 1). Notably, the unrestored sites and the restored sites are both located in interaction area of freshwater and saline water, and the unrestored sites have relatively low impact from anthropogenic activities.
Figure 1 Location map on the north and south banks of the Yellow River Delta. The purple points represent the unrestored sites and the restored sites.
The topsoil (0-10 cm) of 59 sample points was collected during 6 periods (2019/7, 2019/11, 2020/5, 2020/7, 2021/4, and 2021/6). We collected 3 soil samples with a volume of 100 cm3 at 1 site, and then we mixed 3 samples into 1; thus, 354 soil samples were collected in total. First, the plant residues, stones, and other debris in the soil samples were removed in the laboratory (Du et al., 2022). Then, we weighed the wet soil weight M1 and located the soil samples in a 60° oven to dry to constant weight and weighed M2. Then, we calculated the soil moisture content (hereafter, SMC) as (M1-M2)/M1. Then, one part of the soil samples were ground through sieving using a 20-mesh sieve (0.85 mm) for the soil salinity (hereafter, SS) and pH measurements (Jia et al., 2015). Another part of the soil samples was continually ground through sieving using a 100-mesh sieve (0.149 mm) for the contents of soil biogenic element (Du et al., 2022). SS and pH were measured using a portable salinity meter (JENCO 3010 M) and a pH meter (HANNA HI 8424), respectively, in the supernatant of soil–water 1:5 (mass: volume) (Tandon, 2005). The TN and TS contents of the soil samples were determined using an elemental analyzer (Vario EL III). TP was determined using inductively coupled plasma (ICP), and SOC was determined using the potassium dichromate volumetric method (Walkley and Black, 1934).
To explore the significant difference between different periods of the biogenic elements and biological stoichiometry in the north and south banks of the Yellow River Delta, a significance test that is a posterior analysis was performed. Specifically, we adopted the nonparametric Kruskal–Wallis test for a nonnormally distributed variable (McKight and Najab, 2010) and one-way ANOVA with LSD (Line Segment Detector) post hoc multiple test for normally distributed variables (Stahle and Wold, 1989). Notably, the variables were modified to obtain a normal distribution by using square root (sqrt), logarithmic (ln), or inverse transformations (1/). In addition, the T level of statistical significance was set to P (probability) <0.05, and the statistical analyses were performed using SPSS 20.0 statistical software (IBM, Armonk, NY, USA).
To identify correlations among environmental conditions, biogenic elements, and ecological stoichiometry in the soil samples, Redundancy Analysis (hereafter, RDA) was conducted using the Canoco 5.0 software package (Microcomputer Power, Ithaca, USA) (Van Den Wollenberg, 1977; Lu et al., 2018). We adopted regular indicators (SS, SMC and pH) as the environmental factors (Jia et al., 2015). For the RDA, the variables are represented by arrows, and a longer arrow indicates that the corresponding parameter is more important. In addition, a small angle between two arrows indicates that the correlation between the two corresponding variables is strong (Bonari et al., 2017; Chen et al., 2019; Jarideh et al., 2021).
To compare the similarity of the mean profile and changing trends of grouped samples under different conditions on the north and south banks of the Yellow River Delta, we adopted profile analysis. The profile analysis method is a multivariate analysis of variance (MANOVA) with repeated measures (Crowder and Hand, 2017). Specifically, profile similarity is used to determine whether there is any interaction between the analysis groups. Profile coincidence is used to see if there is a substantial difference between the groups. Horizontal profile is used to see if there is a substantial difference between certain parameters (Crowder and Hand, 2017).
The average contents of soil SOC in North Unrestoration, North Restoration, South Unrestoration, and South Restoration were 5.19, 4.99, 4.09, and 4.34 g/kg, respectively (Figures 2A–D). The soil SOC contents of 2020/5, 2021/4, and 2021/6 in North Unrestoration were significantly higher than those in 2019/11 and 2020/7 (P<0.05). Significant differences in soil SOC in South Unrestoration were observed in 2019/11 and 2021/4 and in 2019/11 and 2021/6 (P<0.05). The North Unrestoration exhibited the highest soil TN content (0.40 g/kg), followed by the North Restoration (0.39 g/kg) and South Unrestoration (0.34 g/kg), while the lowest soil TN content (0.33 g/kg) was observed in the South Restoration (Figures 2E–H). 2020/7 and 2021/6, 2019/7, and 2020/7 showed a significant difference in North Restoration and South Unrestoration, respectively (P<0.05).
Figure 2 The soil SOC content of (A) the North Unrestoration, (B) the North Restoration, (C) the South Unrestoration, and (D) the South Restoration. The soil TN content of (E) the North Unrestoration, (F) the North Restoration, (G) the South Unrestoration, and (H) the South Restoration. The soil TP content of (I) the North Unrestoration, (J) the North Restoration, (K) the South Unrestoration, and (L) the South Restoration. The soil TS content of (M) North Unrestoration, (N) North Restoration, (O) South Unrestoration, and (P) South Restoration. Data means ± SE. Differences in SOC-South Unrestoration, TN-South Restoration, TS-South Restoration and TP-North Unrestoration were tested by the nonparametric Kruskal–Wallis test, and the rest were all tested by one-way ANOVA with LSD post hoc multiple tests. Bars with different letters are significantly different (P<0.05). SOC represents soil organic carbon, TN represents total nitrogen, TP represents total phosphorus, TS represents total sulfur, and the following figure captions are the same.
The average contents of soil TP in North Unrestoration, North Restoration, South Unrestoration, and South Restoration were 0.64, 0.59, 0.63, and 0.60 g/kg, respectively (Figures 2I–L). The soil TP contents of 2021/6 and 2020/7 were significantly different in the North Restoration. Furthermore, the soil TP contents of 2019/11, 2020/5, and 2020/7 were significantly different from that of 2021/6 in the South Unrestoration (P<0.05). The South Restoration exhibited the highest TS contents (0.42 g/kg), followed by the North Restoration (0.41 g/kg) and South Unrestoration (0.39 g/kg), while the lowest soil TS contents (0.30 g/kg) was observed in the North Unrestoration (Figures 2M–P). The soil TS contents of 2019/7 and 2020/7, 2021/4 in the North Restoration, 2020/5 and 2019/7 in the South Unrestoration, and 2021/6, 2020/5, 2020/7, and 2021/4 in the South Restoration were significantly different (P<0.05). In addition, the soil SOC, TN, and TP showed the highest contents in the North Unrestoration, while the TS contents appeared highest in the North Restoration (Figure 2).
The highest average values of soil C/N, C/P, C/S, and N/P (i.e., 21.77, 8.25, 32.83, and 0.76, respectively) appeared in the South Restoration, North Unrestoration, North Unrestoration, and North Restoration, respectively (Figures 3A–P). Soil C/N in South Restoration was significantly different for 2020/5 and 2021/4 (Figure 3D), and C/P in South Unrestoration for 2019/11 was significantly different from that of 2019/7 and 2021/6 (Figure 3G). In addition, the soil C/S of 2020/5 and 2019/7 in the North Restoration and that of 2020/5, 2020/7, and 2019/7 in the South Unrestoration were significantly different (Figures 3J, K). The light fluctuation of soil N/P in the Yellow River Delta was observed (Figures 3M–P).
Figure 3 The C/N content of (A) the North Unrestoration, (B) the North Restoration, (C) the South Unrestoration, and (D) the South Restoration. The C/P content of (E) the North Unrestoration, (F) the North Restoration, (G) the South Unrestoration, and (H) the South Restoration. The C/S content of (I) the North Unrestoration, (J) North Restoration, (K) South Unrestoration, and (L) South Restoration. The N/P content of (M) the North Unrestoration, (N) the North Restoration, (O) the South Unrestoration, and (P) the South Restoration. Data means ± SE. Differences for the C/N-North Unrestoration, C/N-North Restoration, C/N-South Unrestoration, C/N-South Restoration, C/P-South Restoration, and C/S-South Restoration were tested by the nonparametric Kruskal–Wallis test, and the rest were all tested by one-way ANOVA with LSD post hoc multiple tests. Bars with different letters are significantly different (P<0.05). C/N represents the ratio between soil organic carbon and total nitrogen, C/P represents the ratio between soil organic carbon and total phosphorus, C/S represents the ratio between soil organic carbon and total sulfur, N/P represents the ratio between total nitrogen and total phosphorus, and the following figure captions are the same.
Generally, the soil SOC was an order of magnitude larger than TN, TP, and TS (Figures 4A–D). In addition, the average soil ecological stoichiometry showed C/S (30.70)> C/N (18.29) > C/P (7.51) > N/P (0.62) in the Yellow River Delta (Figures 4E–H). As Figures 4A–D show, the soil SOC, TN, TP, and TS of North Unrestoration and South Unrestoration fluctuated more than those of North Restoration and South Restoration, respectively. However, the soil C/N, C/P, C/S, and N/P ratios of North Unrestoration and South Restoration fluctuated more than those of North Restoration and South Unrestoration, respectively (Figures 4E–H).
Figure 4 The comparison between the North Unrestoration, North Restoration, South Unrestoration and South Restoration of (A) SOC, (B) TN, (C) TP, (D) TS, (E) C/N, (F) C/P, (G) C/S, (H) N/P.
As Figure 5 shows, the changing characteristics of soil SOC, TN, TP, and TS in the North Unrestoration and North Restoration and South Unrestoration and South Restoration were profile similarity, profile coincidence, and trend horizontality, which means that the unrestored and restored sites were not significantly different. We further conclude that the ecosystem remained natural after ecological restoration was performed for 3 years. Notably, the changing trends of soil SOC in the North Unrestoration and North Restoration were not strongly coincident (Figure 5A), indicating that the ecological restoration had great effects on the soil SOC content.
Figure 5 The profile analysis of (A) SOC on the north bank, (B) SOC on the south bank, (C) TN on the north bank, (D) TN on the south bank, (E) TP on the north bank, (F) TP on the south bank, (G) TS on the north bank and (H) TS on the south bank. The significance level (P) was set as 0.05.
The changing characteristics of soil C/P and N/P in the North Unrestoration and North Restoration and South Unrestoration and South Restoration were profile similarity, profile coincidence, and trend horizontality (Figures 6C, D, G, H). Soil C/N in the North Unrestoration and Restoration, and soil C/S in the South Unrestoration and South Restoration were both profile similarity, profile coincidence, and trend horizontality (Figures 6A, F). Notably, the changing trend of soil C/N in the South Unrestoration and South Restoration was not trend horizontality considering all the factors (Figure 6B). However, the changing trend of soil C/S in the North Unrestoration exhibited a considerably different profile similarity from the North Restoration, indicating that the three-year restoration effort altered the wetland environment in terms of soil C/S (Figure 6E). Furthermore, the North Unrestoration of soil C/S was much greater than that of the North Restorations. In general, the soil C/N and C/S ratios were more susceptible to environmental change.
Figure 6 The profile analysis of (A) C/N in the north bank, (B) C/N in the south bank, (C) C/P in the north bank, (D) C/P in the south bank, (E) C/S in the north bank, (F) C/S in the south bank, (G) N/P in the north bank and (H) N/P in the south bank. The significance level (P) was set as 0.05.
The basic characteristics of the first two axes of RDA sorting are listed in Table 1. In addition, the order of importance and significance of test findings of the impacts of environmental variables on soil biogenic elements and ecological stoichiometry were determined using the Monte Carlo replacement test of environmental factors and are shown in Table 2.
On the north bank in 2019, the importance order of the environmental factors for the soil biogenic element contents and ecological stoichiometry: SMC (51.10%) >SS (26.60%) > pH (22.40%) (Table 2). Soil TP was positively correlated with SS but negatively correlated with SMC, while pH was positively correlated with C/N but negatively correlated with C/S. In addition, soil SMC was positively correlated with soil SOC, TN, TS, C/P, and N/P (Figure 7A). On the north bank in 2020, the importance order of the environmental factors for the soil biogenic element contents and ecological stoichiometry: pH (61.90%) > SMC (34.90%) >SS (3.20%) (Table 2). Further analysis showed that both soil SMC and pH were positively correlated with soil TS, TN, and N/P (Figure 7B). On the north bank in 2021, the importance order of the environmental factors for the soil biogenic element contents and ecological stoichiometry: SS (68.90%) > SMC (20.70%) > pH (10.40%) (Table 2). Soil SMC and pH were positively correlated with soil SOC, TN, TS, C/P, and N/P and negatively correlated with soil C/S. Soil SS was positively correlated with soil TP and C/S and negatively correlated with soil SOC, TN, TS, C/P, and N/P. Soil pH was positively correlated with soil C/N and negatively correlated with soil TP and C/S (Figure 7C). Overall, the importance order of the environmental factors for the soil biogenic element contents and ecological stoichiometry: SS (49.30%) > SMC (32.20%) > pH (18.50%) (Table 2) on the north bank over three years from 2019 to 2021 (Table 2). Soil SMC was positively correlated with soil SOC, TN, TP, C/N, C/S, C/P, N/P, and weakly correlated with soil TS. Soil SS was negatively correlated with all soil biogenic element contents and their stoichiometric ratios except for soil C/S (Figure 7D). Furthermore, pH was negatively correlated with all soil biogenic element contents and their stoichiometric ratios.
Figure 7 RDA of physicochemical properties and soil biogenic element contents and their stoichiometric ratios for the north bank. (A) 2019; (B) 2020; (C) 2021; (D) 2019-2021. Purple four-pointed stars represent unrestored sites, and green circles represent restored sites.
On the south bank in 2019, soil SMC, SS, and pH contributed 12.00%, 42.70%, and 45.20% to the soil biogenic element contents and stoichiometric ratios, respectively (Table 2). Soil pH was positively correlated with soil TN, SOC, and N/P. Soil pH showed a strong positive correlation with soil TN, SOC, N/P, and a negative correlation with soil TP (Figure 8A). On the south bank of 2020, the contribution rates of soil SMC, SS, and pH to the soil biogenic element contents and stoichiometric ratio were 45.50%, 38.40%, and 16.10%, respectively. Among them, soil SMC (P=0.026) and SS (P=0.004) were significant environmental variables (Table 2). Soil SMC was positively correlated with soil TP, SOC, C/N, C/S, and C/P and negatively correlated with soil TS, TN, and N/P. Soil SS was positively correlated with soil TS and N/P. Soil pH was negatively correlated with all soil biogenic element concentrations and stoichiometric ratios (Figure 8B). On the south bank of 2021, the contribution rates of soil SMC, SS, and pH were 21.90%, 17.50%, and 60.60%, respectively, (Table 2). Soil SMC was positively correlated with soil TP, SOC, C/P, C/S, and C/N and negatively correlated with soil TN and N/P. There was a negative correlation between soil SS and soil TS. In addition, there was a positive correlation between soil pH and TN, TP, and a negative correlation with soil TS, SOC, and TN (Figure 8C). For the south bank in 2019-2021, the contributions of soil SMC, SS, and pH to the soil biogenic element contents and their stoichiometric ratios were 85.70%, 8.50%, and 5.80%, respectively, with SMC being significant (P=0.014) (Table 2). The environmental factors were ranked in order of importance: SMC> SS> pH. Soil SMC was positively correlated with soil SOC, TP, C/P, C/S, and C/N and negatively correlated with soil TN, TS, and N/P (Figure 8D). Soil SS was positively correlated with soil TN, TS, and N/P and negatively correlated with the rest of the soil biogenic element contents and their stoichiometric ratios.
Figure 8 RDA of soil physical and chemical properties and soil biogenic element contents in the south bank. (A) 2019; (B) 2020; (C) 2021; (D) 2019-2021. The purple quadrangular stars represent the unrestored sites, and the green circles represent the restored sites.
The biogenic elements in coastal wetlands had different levels of significance under different temporal and spatial conditions due to the easily variable local water salt conditions, vegetation distribution varying with season and microbial activities (Zhou et al., 2007; Cao et al., 2015; Marty et al., 2017; Zhang et al., 2021b). The soil SOC in the Yellow River Delta was much greater than soil TN, TP, and TS, which was likely due to the new-born wetlands (Chen et al., 2008). The biogenic elements of the Yellow River Delta were mainly from the Bohai Sea, which had a high organic carbon content (Wang et al., 2018a). In this study, we found that the ecological restoration in both banks promoted the stability of the biogenic elements, which was probably due to environmental homogenization by replenishing freshwater and growing plants in the North Restoration and South Restoration (Yang et al., 2020; Cai et al., 2021). Additionally, due to the short observation period, ecological restoration did not alter the trends of soil SOC, TN, TP, and TS in the Yellow River Delta. The biogenic components, in particular the soil SOC content, may change significantly over time. Because soil SOC was the primary component in the Yellow River Delta and thus soil SOC was more sensitive, the soil SOC of restored sites actually differed from the unrestored sites only slightly at this time (Figure 5A).
The implementation of ecological restoration affected the environmental variables significantly (Figures 7, 8), thereby, the biogenic element and ecological stoichiometry were changed. In this study, we found that the contribution of soil SS and SMC to the soil biogenic elements and their stoichiometric ratio were higher than that of soil pH, and SS and high SMC were favorably correlated with most biogenic element contents and their stoichiometric ratios on the north bank. The implementation of ecological restoration increased the soil SMC, decreased the soil SS, and increased the soil biogenic element contents and their stoichiometry (Cui et al., 2021; Ma et al., 2021; Zhang et al., 2022). However, soil pH was negatively correlated with all soil biogenic element contents and their stoichiometric ratios because low pH soil was beneficial to nitrogen accumulation (Tang et al., 2014). After the implementation of the restoration project, the retention capacity of N in the wetland soil of the Yellow River Delta was limited by the mass fraction of soil SOC, and the increase in the C/N mass ratio in a certain range promoted the mineralization of organic N, resulting in soil N loss (Wild et al., 2019).
The ecological stoichiometry was determined by the contents of biogenic elements (Du et al., 2022). The content of soil TS is lower than the TN and TP in the Yellow River Delta (Lu et al., 2018; Du et al., 2022). Analogously, soil C/S was larger than C/N and C/P (Lu et al., 2018). In addition, the soil N/P was much lower in the Yellow River Delta (Lu et al., 2018; Du et al., 2022). Furthermore, we found that the effect of ecological restoration on ecological stoichiometry was distinct on the north and south banks (Figures 5E–H). Specifically, ecological restoration on the north bank increased the stability of the ecological stoichiometry, while ecological restoration on the south bank decreased the stability of the ecological stoichiometry. This was probably because ecological restoration on the north bank focuses on water replenishment, while that on the south bank focuses on vegetation growth. On the one hand, the water replenishment results in environmental homogenization, thus the stability is increased. On the other hand, vegetation was more easily influenced by climate change (e.g., the growing season and its subphases (i.e., the growing and senescent phases) (Ding et al., 2020). Generally, ecological stoichiometry was more sensitive than biogenic elements because that the ecological stoichiometry is calculated by two element content (Sardans et al., 2021; Du et al., 2022). For ecological stoichiometry in the Yellow River Delta, the soil C/N and C/S were more sensitive to environmental change due to their high ratio (Lu et al., 2018; Du et al., 2022).
The south bank’s ecological restoration relied on vegetation development, and marsh soil with vegetation and well-developed roots can efficiently hold more nitrogen (Liang et al., 2019). The findings of RDA for the south bank suggested that pH was the most important physical and chemical factor influencing the N concentration. Soil organic matter was the most direct source of nitrogen, and soil with lower pH was helpful for nitrogen buildup (Tang et al., 2014). Soil SMC was favorably associated with soil SOC, TP, C/P, C/S, and C/N and negatively associated with soil TN, TS, and N/P. There was a substantial positive relationship between soil SS and TN, TS, and N/P and a significant inverse relationship between soil SS and the concentrations of other biogenic element contents and their stoichiometric ratios. Previous research also found that soil TP in the Suaeda salsa wetland in the Yellow River Delta intertidal zone was positively correlated with soil SMC and SS, with obvious seasonal differences, but the correlation between soil TP, SOC and pH was not strong. In addition, the source of soil TS in the wetland soil on both banks was mainly TS in seawater, which could explain the reason why soil TN and TS were closely related to soil SS to some extent (Wei et al., 2021).
The result of the biogenic elements and ecological stoichiometry in this study was consistent with a previous study in the Yellow River Delta (Lu et al., 2018), especially the soil SOC (0.47%), TN (0.04%), TP (0.06%), and TS (0.04%) contents in the soil. In addition, the C/N ratio was a sensitive indicator of soil quality and a symbol of soil nitrogen mineralization capacity (Marty et al., 2017). In addition, C/N was inversely proportional to the decomposition rate of organic matter, which means that soil with a lower C/N value had a faster mineralization rate (Zhu et al., 2013). In this study, the range of C/N was 10-50 (Figure 6E), which was consistent with Du et al. (2022). The result indicated that the soil organic matter in the Yellow River Delta has completely humified, and SOC mineralization has begun to be limited (Du et al., 2022). Notably, the soil C/N value found in the Yellow River Delta is greater than that observed in some Chinese wetlands (e.g. Sanjing Plain, Halahai wetlands, Changyi wetland, Jinjiang wetland) (Bai et al., 2010; Zhang et al., 2012b; Wang et al., 2014; Cao et al., 2015; Zeng et al., 2017; Guo et al., 2018).
Soil C/P was regarded as a marker for the ability of soil P mineralization Schadt and Classen (2007) and an index of the latent capacity of P to be absorbed from the environment by microbial mineralization (Wang et al., 2018b). Furthermore, the soil C/S ratio was an indicator of the possible effects of microbial biomass on the availability of soil S (He et al., 1997). In this study, the ranges of soil C/P and C/S were 3-15 and 0-100, respectively. This result means that the mineralization of P and S was the main process, and the available phosphorus and sulfur would increase due to the relatively lower C/P and C/S (Heinze et al., 2010; Lu et al., 2018). In addition, the soil C/P and C/S ratios were close to those of other Chinese wetlands (Zhang et al., 2012a; Zhang et al., 2021b). The soil N/P ratio indicated the status of soil N saturation and was used to determine the thresholds for soil quality limitation (Zhao et al., 2015). Notably, the average soil N/P of this research (i.e., 0.62) was lower than that of other wetlands (>1.2) (Prusty et al., 2009; Cao et al., 2015). Actually, the sedimentation environment, hydrodynamics, grain size, vegetation biomass, and other factors control the element contents and biological stoichiometry in the world (Hu et al., 2016; Li et al., 2019a).
Ecological restoration altered the water table and wet-dry conditions, and thus the mineralization rates of soil SOC, TN, TP, and TS were affected (Comín, 2010; Lamers et al., 2015). Therefore, the biogenic elements and ecological stoichiometry have changed in the Yellow River Delta under ecological restoration. After studying the specific project in the Yellow River Delta, three key points were identified which could potentially imply for conducting ecological restoration. First, we clarify the importance of soil biogeochemical cycles, namely, that soil biogenic elements and ecological stoichiometry play many roles in vegetation and microorganisms (Hu et al., 2016). The biogenic element contents and their ratio are essential evaluation indicators for ecological restoration (Wortley et al., 2013). Therefore, we propose that the success of the ecological restoration will be enhanced by emphasizing continuous observation after project implementation (Li et al., 2019b). Second, the main components of soil biogenic elements and ecological stoichiometry should receive sufficient attention during the implementation of ecological restorations, because they are much more sensitive to environmental change (Du et al., 2022). Third, the choice of unrestored sites, which need to well reflect the natural conditions, cannot be ignored (Durbecq et al., 2020). Notably, profile analysis can be adopted to distinguish the effects of ecological restoration.
In this study, The soil biogenic element content (SOC, TN, TP, TS) and ecological stoichiometry (C/N, C/P, C/S, N/P) in the Yellow River Delta under ecological restoration (IIWBCP) were investigated. The results showed that ecological restoration improved the biogenic element stability in both banks of the Yellow River Delta due to the environmental homogenization. However, the restoration increased the ecological stoichiometry’s stability in the north bank while decreasing the ecological stoichiometry’s stability in the south bank because of the different restoration focuses. The changing trend of soil C/S in the North Unrestoration showed a significant difference from the North Restoration, implying that ecological restoration alters the wetland ecosystem from a soil C/S perspective. Notably, the high content of soil biogenic elements SOC and high ratio of soil ecological stoichiometry C/N and C/S in the Yellow River Delta induce that they were more sensitive to environmental change. Furthermore, the main environmental variables in the north and south banks from 2019 to 2021 were soil SS and SMC, respectively. The ecological restoration have changed the environmental condition, thus the biogenic element contents and ecological stoichiometry were changed to some extent. Further and more detailed investigations of biogenic elements and ecological stoichiometry are needed to demonstrate the influences of ecological restoration and provide a reference for future restoration in local sites and global wetlands.
The original contributions presented in the study are included in the article/Supplementary Material. Further inquiries can be directed to the corresponding authors.
DL and YL contributed to the writing of the manuscript. DL and YX involved in the field work and data collection. DL, BC and ZN contributed to concept of study. BC, ZN, SZ, ZB, SF and CC provided important guidance on methods and writing. All authors contributed critically to drafts and gave approval for publication. All authors contributed to the article and approved the submitted version.
This work was supported by Key Project of National Natural Science Foundation of China (No. U2243208), Key Project of the National Natural Science Foundation of China (No. U1901212), the National Postdoctoral Program for Innovative Talents (No. BX20220040) and the General Program of China Postdoctoral Science Foundation (No. 2022M710413).
The authors would like to thank Bo Pang, Fang Gao, Hanxu Zhang, and Xinyu Li for their assistances in the field work, Jin Li, Xuejuan Chen, and Wenxin Yang for their support in the laboratory work, and Qing Wang and Tian Xie for their guidance on the analysis.
The authors declare that the research was conducted in the absence of any commercial or financial relationships that could be construed as a potential conflict of interest.
All claims expressed in this article are solely those of the authors and do not necessarily represent those of their affiliated organizations, or those of the publisher, the editors and the reviewers. Any product that may be evaluated in this article, or claim that may be made by its manufacturer, is not guaranteed or endorsed by the publisher.
Bai J., Ouyang H., Xiao R., Gao J., Gao H., Cui B., et al. (2010). Spatial variability of soil carbon, nitrogen, and phosphorus content and storage in an alpine wetland in the qinghai - Tibet plateau, China. Soil Res. 48 (8), 730–736. doi: 10.1071/sr09171
Bai J. H., Wang J. J., Yan D. H., Gao H. F., Xiao R., Shao H. B., et al. (2012). Spatial and temporal distributions of soil organic carbon and total nitrogen in two marsh wetlands with different flooding frequencies of the yellow river delta, China. Clean.: Soil. Air. Water. 40 (10), 1137–1144. doi: 10.1002/clen.201200059
Bonari G., Migliorini M., Landi M., Protano G., Fanciulli P. P., Angiolini C. (2017). Concordance between plant species, oribatid mites and soil in a Mediterranean stone pine forest. Arthropod-Plant. Interact. 11 (1), 61–69. doi: 10.1007/s11829-016-9466-4
Cai Y., Liang J., Zhang P., Wang Q., Wu Y., Ding Y., et al. (2021). Review on strategies of close-to-natural wetland restoration and a brief case plan for a typical wetland in northern China. Chemosphere. 285, 131534. doi: 10.1016/j.chemosphere.2021.131534
Cao L., Song J. M., Li X. G., Yuan H. M., Li N., Duan L. Q., et al. (2015). Geochemical characteristics of soil c, n, p, and their stoichiometrical significance in the coastal wetlands of laizhou bay, bohai Sea. Clean.: Soil. Air. Water. 43 (2), 260–270. doi: 10.1002/clen.201300752
Chen H. W., Lin H. C., Chuang Y. H., Sun C. T., Chen W. Y., Kao C. Y. (2019). Effects of environmental factors on benthic species in a coastal wetland by redundancy analysis. Ocean. Coast. Manage. 169, 37–49. doi: 10.1016/j.ocecoaman.2018.12.003
Chen W., Shi Y., Tian S., Wang W. (2008). Study on distribution characteristics of soil nitrogen and phosphorus in new-born wetland of yellow river estuary. J. Soil Water Conserv. 22 (01), 69–73. doi: 10.13870/j.cnki.stbcxb.2008.01.031
Comín F. A. (2010). Ecological restoration: A global challenge (Cambridge, United Kingdom: Cambridge University Press).
Couvillion B. R., Steyer G. D., Wang H. Q., Beck H. J., Rybczyk J. M. (2013). Forecasting the effects of coastal protection and restoration projects on wetland morphology in coastal Louisiana under multiple environmental uncertainty scenarios. J. Coast. Res. 67 (sp1), 29–50. doi: 10.2112/Si_67_3
Crowder M. J., Hand D. J. (2017). Analysis of repeated measures. routledge. (New York) Available at: https://www.taylorfrancis.com/books/mono/10.1201/9781315137421/analysis-repeated-measures-martin-crowder-david-hand.
Cui H., Bai J., Du S., Wang J., Keculah G. N., Wang W., et al. (2021). Interactive effects of groundwater level and salinity on soil respiration in coastal wetlands of a Chinese delta. Environ. pollut. 286, 117400. doi: 10.1016/j.envpol.2021.117400
Ding Y. X., Li Z., Peng S. Z. (2020). Global analysis of time-lag and -accumulation effects of climate on vegetation growth. Int. J. Appl. Earth Obs. Geoinf. 92, 102179. doi: 10.1016/j.jag.2020.102179
Du S., Bai J., Zhao Q., Wang C., Guan Y., Jia J., et al. (2022). Deposition flux, stocks of c, n, p, s, and their ecological stoichiometry in coastal wetlands with three plant covers. Front. Ecol. Evol. 10. doi: 10.3389/fevo.2022.840784
Durbecq A., Jaunatre R., Buisson E., Cluchier A., Bischoff A. (2020). Identifying reference communities in ecological restoration: The use of environmental conditions driving vegetation composition. Ecol. Restor. 28 (6), 1445–1453. doi: 10.1111/rec.13232
Elser J. J., Acharya K., Kyle M., Cotner J., Makino W., Markow T., et al. (2003). Growth rate–stoichiometry couplings in diverse biota. Ecol. Lett. 6 (10), 936–943. doi: 10.1046/j.1461-0248.2003.00518.x
Gao H. F., Bai J. H., Wang Q. G., Huang L. B., Xiao R. (2010). Profile distribution of soil nutrients in unrestored and restored wetlands of the yellow river delta, China. Int. Conf. Ecol. Inf. Ecosystem. Conserv. (Iseis. 2010). 2, 1652–1661. doi: 10.1016/j.proenv.2010.10.176
Giosan L., Syvitski J., Constantinescu S., Day J. (2014). Climate change: Protect the world's deltas. Nature. 516 (7529), 31–33. doi: 10.1038/516031a
Guo P. Y., Sun Y. S., Su H. T., Wang M. X., Zhang Y. X. (2018). Spatial and temporal trends in total organic carbon (TOC), black carbon (BC), and toted nitrogen (TN) and their relationships under different planting patterns in a restored coastal mangrove wetland: Case study in fujian, China. Chem. Speciat. Bioavailab. 30 (1), 47–56. doi: 10.1080/09542299.2018.1484673
Heinze S., Raupp J., Joergensen R. G. (2010). Effects of fertilizer and spatial heterogeneity in soil pH on microbial biomass indices in a long-term field trial of organic agriculture. Plant Soil. 328 (1-2), 203–215. doi: 10.1007/s11104-009-0102-2
He Z. L., Wu J., ODonnell A. G., Syers J. K. (1997). Seasonal responses in microbial biomass carbon, phosphorus and sulphur in soils under pasture. Biol. Fertil. Soils. 24 (4), 421–428. doi: 10.1007/s003740050267
Hu Y. F., Peng J. J., Yuan S., Shu X. Y., Jiang S. L., Pu Q., et al. (2016). Influence of ecological restoration on vegetation and soil microbiological properties in alpine-cold semi-humid desertified land. Ecol. Eng. 94, 88–94. doi: 10.1016/j.ecoleng.2016.05.061
Jarideh S., Alvaninezhad S., Gholami P., Mirzaei M. R., Armin M. (2021). Soil properties and soil organic carbon stock changes resulted from deforestation in a semi-arid region of zagros forests, Iran. Arid. Ecosyst. 11 (1), 18–26. doi: 10.1134/S2079096121010078
Jia J., Junhong B., Zhaoqin G., Xiaojun W., Guangliang Z., Zhijian X., et al. (2015). Carbon and nitrogen contents and storages in the soils of intertidal salt marshes in the yellow river delta. Wetl. Sci. 13 (06), 714–721. doi: 10.13248/j.cnki.wetlandsci.2015.06.010
Jiang Y., Wang Y., Zhou D., Ke Y., Bai J., Li W., et al. (2021). The impact assessment of hydro-biological connectivity changes on the estuary wetland through the ecological restoration project in the yellow river delta, China. Sci. Total. Environ. 758, 143706. doi: 10.1016/j.scitotenv.2020.143706
Lamers L. P., Vile M. A., Grootjans A. P., Acreman M. C., van Diggelen R., Evans M. G., et al. (2015). Ecological restoration of rich fens in Europe and north America: From trial and error to an evidence-based approach. Biol. Rev. Camb. Philos. Soc 90 (1), 182–203. doi: 10.1111/brv.12102
Liang K., Fan Y., Meki K., Meng D., Yan Q., Zheng H., et al. (2019). The seasonal dynamics of nitrogen and rhizosphere effects in the typical saline-alkali vegetation communities of the yellow river estuary wetland. Environ. Chem. 38 (10), 327–2335. doi: 10.7524/j.issn.0254-6108.2018120301
Liang S., Jiang W., Sun J. (2018). “The comprehensive renovation and restoration of coastal zone in China,” in The 28th international ocean and polar engineering conference (Sapporo, Japan: OnePetro).
Li P., Ke Y., Bai J., Zhang S., Chen M., Zhou D. (2019a). Spatiotemporal dynamics of suspended particulate matter in the yellow river estuary, China during the past two decades based on time-series landsat and sentinel-2 data. Mar. pollut. Bull. 149, 110518. doi: 10.1016/j.marpolbul.2019.110518
Liu Z., Fagherazzi S., Cui B. (2021). Success of coastal wetlands restoration is driven by sediment availability. Commun. Earth Environ. 2 (1), 44. doi: 10.1038/s43247-021-00117-7
Liu S., Hou X., Yang M., Cheng F., Coxixo A., Wu X., et al. (2018). Factors driving the relationships between vegetation and soil properties in the yellow river delta, China. Catena. 165, 279–285. doi: 10.1016/j.catena.2018.02.004
Li S., Xie T., Pennings S. C., Wang Y., Craft C., Hu M. (2019b). A comparison of coastal habitat restoration projects in China and the united states. Sci. Rep. 9 (1), 14388. doi: 10.1038/s41598-019-50930-6
Lu Q. Q., Bai J. H., Zhang G. L., Zhao Q. Q., Wu J. J. (2018). Spatial and seasonal distribution of carbon, nitrogen, phosphorus, and sulfur and their ecological stoichiometry in wetland soils along a water and salt gradient in the yellow river delta, China. Phys. Chem. Earth. 104, 9–17. doi: 10.1016/j.pce.2018.04.001
Marty C., Houle D., Gagnon C., Courchesne F. (2017). The relationships of soil total nitrogen concentrations, pools and C:N ratios with climate, vegetation types and nitrate deposition in temperate and boreal forests of eastern Canada. Catena. 152, 163–172. doi: 10.1016/j.catena.2017.01.014
Ma S., Tao B. X., Han G. X., Wang X. J., Li P., Chu X. J. (2021). Effects of spring rainfall distribution on soil respiration in a coastal wetland of the yellow river delta. Chin. J. Ecol. 40 (7), 10. doi: 10.13292/j.1000-4890.202107.007
McKight P. E., Najab J. (2010). Kruskal-Wallis test. Corsini. Encyclopedia. Psychol. doi: 10.1002/9780470479216
Nicholls R. (2020). Delta challenges and trade-offs from the Holocene to the anthropocene BT-deltas in the anthropocene. Eds. Nicholls R. J., Adger W. N., Hutton C. W., Hanson ,. S. E. (Cham, Germany: Cham).
Ning Z., Chen C., Xie T., Zhu Z., Wang Q., Cui B., et al. (2021). Can the native faunal communities be restored from removal of invasive plants in coastal ecosystems? A global meta-analysis. Global Change Biol. 27 (19), 4644–4656. doi: 10.1111/gcb.15765
Prusty B. A. K., Chandra R., Azeez P. A. (2009). Distribution of carbon, nitrogen, phosphorus, and sulfur in the soil in a multiple habitat system in India. Soil Res. 47 (2), 177–189. doi: 10.1071/Sr08087
Ptacnik R., Jenerette G. D., Verschoor A. M., Huberty A. F., Solimini A. G., Brookes J. D. (2005). Applications of ecological stoichiometry for sustainable acquisition of ecosystem services. Oikos. 109 (1), 52–62. doi: 10.1111/j.0030-1299.2005.14051.x
Qu F. Z., Yu J. B., Du S. Y., Li Y. Z., Lv X. F., Ning K., et al. (2014). Influences of anthropogenic cultivation on c, n and p stoichiometry of reed-dominated coastal wetlands in the yellow river delta. Geoderma. 235, 227–232. doi: 10.1016/j.geoderma.2014.07.009
Sardans J., Janssens I. A., Ciais P., Obersteiner M., Penuelas J. (2021). Recent advances and future research in ecological stoichiometry. Perspect. Plant Ecol. Evol. Syst. 50, 125611. doi: 10.1016/j.ppees.2021.125611
Schadt C. W., Classen A.T. (2007). Soil microbiology, ecology and biochemistry. Soil Sci. Soc. Am. 71(4), 1420. doi: 10.2136/sssaj2007.0017br
Stahle L., Wold S. (1989). Analysis of variance (Anova). Chemometr Intell. Lab. Syst. 6 (4), 259–272. doi: 10.1016/0169-7439(89)80095-4
Syvitski J. P. M., Kettner A. J., Overeem I., Hutton E. W. H., Hannon M. T., Brakenridge G. R., et al. (2009). Sinking deltas due to human activities. Nat. Geosci. 2 (10), 681–686. doi: 10.1038/Ngeo629
Tandon H. L. S. (2005). Methods of analysis of soils, plants, waters, fertilisers & organic manures. Fertiliser Development and Consultation Organisation. (Delhi, India)
Tang F. K., Zhou J. X., Cui M., Liu Y. G., Lei R. G. (2014). Effects of different returning farmland to forestlands on accumulation of soil organic carbon and nitrogen in typical karst area of southwestern China. J. Beijing Forestry. Univ. 36 (2), 44–50. doi: 10.13332/j.cnki.jbfu.2014.02.012
Van Den Wollenberg A. L. (1977). Redundancy analysis an alternative for canonical correlation analysis. Psychometrika. 42 (2), 207–219. doi: 10.1007/BF02294050
Walkley A., Black I. A. (1934). An examination of the degtjareff method for determining soil organic matter, and a proposed modification of the chromic acid titration method. Soil Sci. 37 (1), 29–38. doi: 10.1097/00010694-193401000-00003
Wang J., Bai J., Zhao Q., Lu Q., Jia J., Wen X. (2014). Profile characteristics of carbon, nitrogen and phosphorus in soils of phragmites australis marshes in halahai wetlands. Wetl. Sci. 12 (6), 7. doi: 10.13248/j.cnki.wetlandsci.2014.06.002
Wang C. Y., Lv Y. C., Li Y. W. (2018a). Riverine input of organic carbon and nitrogen in water-sediment system from the yellow river estuary reach to the coastal zone of bohai Sea, China. Cont. Shelf. Res. 157, 1–9. doi: 10.1016/j.csr.2018.02.004
Wang M., Qi S., Zhang X. (2012). Wetland loss and degradation in the yellow river delta, Shandong province of China. Environ. Earth Sci. 67 (1), 185–188. doi: 10.1007/s12665-011-1491-0
Wang L. J., Wang P., Sheng M. Y., Tian J. (2018b). Ecological stoichiometry and environmental influencing factors of soil nutrients in the karst rocky desertification ecosystem, southwest China. Glob. Ecol. Conserv. 16,e00449. doi: 10.1016/j.gecco.2018.e00449
Wei Z., Bai J., Zhang L., Zhang G., Wang X., Wang W., et al. (2021). Dynamics of soil biogenic element in phragmites australis wetlands in intertidal zone in the yellow river estuary. J. Beijing Normal. Univ. (Natural. Science). 57 (1), 43–50. doi: 10.12202/j.0476-0301.2020425
Wild B., Li J., Pihlblad J., Bengtson P., Rutting T. (2019). Decoupling of priming and microbial n mining during a short-term soil incubation. Soil Biol. Biochem. 129, 71–79. doi: 10.1016/j.soilbio.2018.11.014
Wortley L., Hero J. M., Howes M. (2013). Evaluating ecological restoration success: a review of the literature. Restor. Ecol. 21 (5), 537–543. doi: 10.1111/rec.12028
Xu G., Song J., Zhang Y., Lv Y., Han G. (2020). Enhancement of phosphorus storage capacity of sediments by coastal wetland restoration, yellow river delta, China. Mar. pollut. Bull. 150, 110666. doi: 10.1016/j.marpolbul.2019.110666
Yang Y. L., Mou X. J., Wen B. L., Liu X. T. (2020). Soil carbon, nitrogen and phosphorus concentrations and stoichiometries scross a chronosequence of restored inland soda saline- alkali wetlands, Western songnen plain, northeast China. Chin. Geogr. Sci. 30 (5), 934–946. doi: 10.1007/s11769-020-1155-7
Zeng Q., Lal R., Chen Y., An S. (2017). Soil, leaf and root ecological stoichiometry of caragana korshinskii on the loess plateau of China in relation to plantation age. PloS One. 12 (1), e0168890. doi: 10.1371/journal.pone.0168890
Zhang Z. M., Cui B. S., Fan X. Y., Zhang K. J., Zhao H., Zhang H. G. (2012a). Wetland network design for mitigation of saltwater intrusion by replenishing freshwater in an estuary. Clean.: Soil. Air. Water. 40 (10), 1036–1046. doi: 10.1002/clen.201100735
Zhang H. H., Kariman K., Zhu L., Liu Y., Chen J. J., Zhu L., et al. (2021b). Spatial distribution of carbon, nitrogen and sulfur in surface soil across the pearl river delta area, south China. Geoderma. Reg. 25, e00390. doi: 10.1016/j.geodrs.2021.e00390
Zhang L., Lan S., Zhao L., Yi H., Han G. (2022). Temporal variation of water-use efficiency and water sources of phragmites australis in the coastal wetland: Based on stable isotopic composition (δ13C and δ18O). Ecol. Indic. 139, 108957. doi: 10.1016/j.ecolind.2022.108957
Zhang Z. S., Lu X. G., Song X. L., Guo Y., Xue Z. S. (2012b). Soil c, n and p stoichiometry of deyeuxia angustifolia and carex lasiocarpa wetlands in sanjiang plain, northeast China. J. Soils. Sediments. 12 (9), 1309–1315. doi: 10.1007/s11368-012-0551-8
Zhang H., Sun Y., Zhang W., Song Z., Ding Z., Zhang X. (2021a). Comprehensive evaluation of the eco-environmental vulnerability in the yellow river delta wetland. Ecol. Indic. 125, 107514. doi: 10.1016/j.ecolind.2021.107514
Zhao Q. Q., Bai J. H., Wang X., Zhang W., Huang Y. J., Wang L. L., et al. (2020). Soil organic carbon content and stock in wetlands with different hydrologic conditions in the yellow river delta, China. Ecohydrol. Hydrobiol. 20 (4), 537–547. doi: 10.1016/j.ecohyd.2019.10.008
Zhao F., Sun J., Ren C., Kang D., Deng J., Han X., et al. (2015). Land use change influences soil c, n, and p stoichiometry under 'Grain-to-Green program' in China. Sci. Rep. 5, 10195. doi: 10.1038/srep10195
Zhou J. L., Wu Y., Kang Q. S., Zhang J. (2007). Spatial variations of carbon, nitrogen, phosphorous and sulfur in the salt marsh sediments of the Yangtze estuary in China. Estuar. Coast. Shelf. Sci. 71 (1-2), 47–59. doi: 10.1016/j.ecss.2006.08.012
Keywords: wetland topsoil, water and salt conditions, biogenic elements, ecological stoichiometry, coastal wetlands
Citation: Li D, Li Y, Xie Y, Cui B, Ning Z, Zhang S, Bi Z, Fu S and Che C (2022) Effects of ecological restoration on soil biogenic elements and their ecological stoichiometry in the Yellow River Delta, China. Front. Mar. Sci. 9:993202. doi: 10.3389/fmars.2022.993202
Received: 13 July 2022; Accepted: 13 September 2022;
Published: 29 September 2022.
Edited by:
Laibin Huang, University of California, Davis, United StatesCopyright © 2022 Li, Li, Xie, Cui, Ning, Zhang, Bi, Fu and Che. This is an open-access article distributed under the terms of the Creative Commons Attribution License (CC BY). The use, distribution or reproduction in other forums is permitted, provided the original author(s) and the copyright owner(s) are credited and that the original publication in this journal is cited, in accordance with accepted academic practice. No use, distribution or reproduction is permitted which does not comply with these terms.
*Correspondence: Baoshan Cui, Y3VpYnNAYm51LmVkdS5jbg==; Zhonghua Ning, am9laHVhLm5pbmdAYm51LmVkdS5jbg==
Disclaimer: All claims expressed in this article are solely those of the authors and do not necessarily represent those of their affiliated organizations, or those of the publisher, the editors and the reviewers. Any product that may be evaluated in this article or claim that may be made by its manufacturer is not guaranteed or endorsed by the publisher.
Research integrity at Frontiers
Learn more about the work of our research integrity team to safeguard the quality of each article we publish.