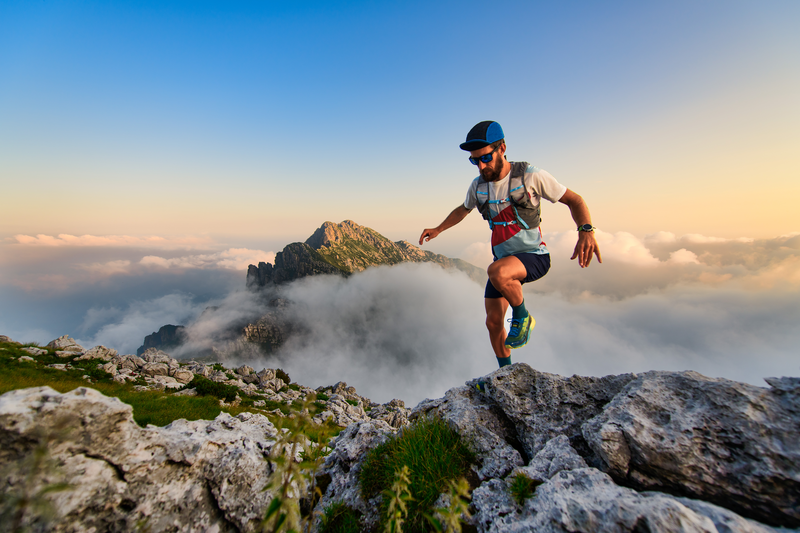
94% of researchers rate our articles as excellent or good
Learn more about the work of our research integrity team to safeguard the quality of each article we publish.
Find out more
ORIGINAL RESEARCH article
Front. Mar. Sci. , 07 November 2022
Sec. Marine Fisheries, Aquaculture and Living Resources
Volume 9 - 2022 | https://doi.org/10.3389/fmars.2022.992715
This article is part of the Research Topic Advances and Perspectives in Integrated Multi-Trophic Aquaculture View all 6 articles
Aquaponics has the potential to produce sustainable and accessible quality food through the integration of hydroponics and aquaculture. Plants take up dissolved nutrients in fish wastewater, allowing water reuse for fish. However, the simultaneous presence of fish and plants in the same water loop has made phytosanitary treatments of diseases such as powdery mildew problematic due to risks of toxicity for fish and beneficial bacteria, limiting its commercialization. Entomopathogenic and mycoparasitic fungi have been identified as safe biological control agents for a broad range of pests. This study aimed to investigate the efficacy of entomopathogenic fungi, Lecanicillium attenuatum (LLA), Isaria fumosorosea (IFR), and mycoparasitic fungus Trichoderma virens (TVI) against Podosphaera xanthii. Also, we investigated the possible harmful effects of the three fungal biocontrol agents in aquaponics by inoculating them in aquaponics water and monitoring their survival and growth. The findings showed that the three biocontrol agents significantly suppressed the powdery mildew at 107 CFU/ml concentration. Under greenhouse conditions (65-73% relative humidity (RH)), a significant disease reduction percentage of 85% was recorded in L. attenuatum-pretreated leaves. IFR-treated leaves had the least AUDPC (area under disease progress curve) of ~434.2 and disease severity of 32% under 65-73% RH. In addition, L. attenuatum spores were the most persistent on the leaves, the spores population increased to 9.54 × 103 CFUmm-2 from the initial 7.3 CFUmm-2 under 65-73%. In contrast, in hydroponics water, the LLA, IFR, and TVI spores significantly reduced by more than 99% after 96 hrs. Initial spore concentrations of LLA of 107 CFU/ml spores were reduced to 4 x 103 CFU after 96 hrs. Though the results from this study were intended for aquaponics systems, relevance of the results to other cultivation systems are discussed.
Graphical Abstract The general flow of the study is shown starting with the set-up of the leaves in growth chambers, treatment with entomopathogenic fungi and inoculation of P. xanthii. In the middle of the flow is a comparisons of untreated leaves (A) with LLA-treated leaves (B) after 20 days. The graph below the leaves shows the disease severities under different relative humidities. On the right are time-based pictures showing the survival of the entomopathogenic fungi in aquaponics water at 0hr and after 96hr.
The continuous growth of the global population demands increased food production, depleting natural resources such as land, water, and nutrients. Hence, there is an urgent need to adopt sustainable food production systems that ensure a reliable and healthy food supply. Aquaponics is a food production method that uses wastewater from fish culture to cultivate plants in hydroponics; in a coupled or decoupled system (Monsees et al., 2017; Lennard and Goddek, 2019). The wastewater from the fish culture unit, carrying dissolved fish wastes, is constantly reclaimed between the recirculating aquaculture unit (RAS) and the hydroponics unit (coupled) or fed periodically to the hydroponics unit in a separate compartment (decoupled). The dissolved fish wastes are converted into plant essential nutrients by beneficial bacteria (Rakocy, 2012). The high water reuse capacity (up to 90%) and the conversion of dissolved fish wastes into plant essential nutrients (among other benefits) make aquaponics approaches a potentially sustainable food production system (Goddek et al., 2019).
Despite these benefits, the simultaneous presence of fish, plant, and beneficial bacteria in the same water loop (especially in coupled aquaponics), has made pest and disease management a pressing challenge in aquaponics, limiting its commercialization (Stouvenakers et al., 2020). Therefore, there is a need to establish control methods with little or no negative influence on plants, fish, and beneficial bacteria. In previous studies focused on identifying an integrated pest and disease management suitable for aquaponics (Folorunso et al., 2021), it was found that existing commercial biological controls are natural remedies for aquaponics pests and could pose little or no harm to non-target organisms. On the other hand, pathogen management still relies on chemical controls, which can pose high toxicity risk to fish and beneficial bacteria. Moreover, plant-pathogen proliferation is more problematic because of the conducive aquaponics/hydroponics ambient environment for fungi (Stouvenakers et al., 2019).
Powdery mildew, caused by Podosphaera xanthii, is one of the most severe plant diseases in greenhouse hydroponics/aquaponics cucumbers and other crops (Schuerger and Hammer, 2003; Savvas et al., 2009; Pollastro et al., 2022). Chemical fungicides have primarily been most widely used control mechanisms in stand-alone hydroponics or field agriculture. But, as stated, spray drifts of fungicides in hydroponics units can cause harmful effects to fish and beneficial bacteria in coupled aquaponics where the water is recircled back to the fish and the biofilter or limit the water reuse capacity of decoupled aquaponics systems (Folorunso et al., 2021; Rašković et al., 2021). Thus, consensus efforts are currently being channeled towards developing control approaches with little or no effects on non-target organisms. Though biological control agents (BCAs) have been identified as sustainable alternatives (Rivas-García et al., 2020), there are currently no BCAs certified for use in multitrophic or integrated systems such as aquaponics.
Additionally, despite the successes reported in many field trials, inconsistencies and mismatches in the transition of results from laboratory trials to the field have made the adoption of many BCAs less attractive (Sawant et al., 2017; Ni and Punja, 2021). Fungal biological control agents are comparatively more tolerant to varying environmental conditions than other microbial biocontrol; hence, they are considered as better alternatives against powdery mildew pathogens (Tesfagiorgis et al., 2014; Gafni et al., 2015). Ampelomyces quisqualis, Trichoderma afroharzianum, T. asperellum, T. aspelloides, T. harzianum, T. viride, and Clonostachys rosea f. catenulata (= Gliocladium catenulatum) have been tested against powdery mildew under laboratory, field, and greenhouse conditions (Gafni et al., 2015; Hafez et al., 2018; Ni and Punja, 2021). However, desired efficacy levels are not achieved when these species are often applied alone (without fungicides) (Sawant et al., 2017; Sarhan et al., 2020; Ni and Punja, 2021). Insufficient growth of the BCAs and varying environmental conditions were identified by Carbó et al. (2020) and Giotis et al. (2012) as limiting factors. Therefore, currently, there are interests in fungal biocontrol agents that are solely efficient and adaptable to varying environmental conditions. Some entomopathogenic and mycoparasitic fungi are more persistent and adaptable to varying environmental conditions (Rivas et al., 2014; Xie et al., 2015; Carbó et al., 2020). Lecanicillium and Isaria species are among the most commonly used entomopathogenic fungi against greenhouse pests.
Lecanicillium species are entomopathogenic fungi that have been commercialized as biopesticides for whitefly control and have a dual role against aphids and pathogens (e.g., Mycotal® and Vertalec®). However, there are conflicting reports on their efficacy towards control of powdery mildew pathogens. Kim et al. (2007); Kim et al. (2008) found no significant differences in the activity of L. muscarium and L. longisporium against cucumber powdery mildew due to varying environmental factors. L. attenuatum have been identified as a highly persistent entomopathogenic fungus with a rapid germination rate (Wang et al., 2017). It acts as mycoparasites, producing compounds such as chitinase, allowing penetration into pests and other fungi (Askary et al., 1998; Kim et al., 2007). Studies such as Askary et al. (1998); Kim et al. (2008), and Goettel et al. (2008), among others, have identified its potential against powdery mildew. There has been a limited number of studies (Drummond et al., 1987) focusing on the efficacy of L. lecanii in optimal and suboptimal conditions.
Isaria fumosorosea is another entomopathogenic fungus with high efficacies against pests such as whiteflies, aphids, thrips, citrus psyllid, and spider mites (Zimmermann, 2008; Avery et al., 2011). Though I. fumosorosea strains can be isolated from powdery mildew-infested plants (Kavkova and Curn, 2005), only one study has investigated its potential against powdery mildew pathogens. Kavkova and Curn (2005) found that cucumber plants pretreated with I. fumosorosea in high relative humidity conditions were not different in their susceptibility to powdery mildew than untreated controls. Thus, knowledge gaps in their use as a biological control against powdery mildew partially anchor the basis for conducting this study.
Trichoderma spp. are mycoparasitic fungi considered the most versatile biocontrol agents due to the secretion of bioreactive compounds that hinder the growth of fungal pathogens. Several Trichoderma species have been tested against powdery mildew with certain levels of success (Chet and Inbar, 1994; Elad, 2000; Woo et al., 2014). However, their efficacy in controlling powdery mildew declines as the disease spreads (Elad, 2000; Liu et al., 2020). Susceptibilities to temperature, relative humidity, and UV irradiation are the major ecological factors determining the effectiveness of entomopathogenic and mycoparasitic fungi (Henis and Chet, 1975; Devi et al., 2005; Abbaszadeh et al., 2011). However, in many instances, relative humidity often exacerbates effects due to its influence on the intensity of temperature and UV irradiation (Abbaszadeh et al., 2011). T. virens is a soil-based species that is highly useful for controlling Pythium ultimum and Rhizoctonia spp. in field and greenhouse crops (Rubin, 2010). It has a considerable high tolerance to varying environmental conditions (Anand et al., 2006). Yet, there is no study on their efficacy in controlling cucumber powdery mildew. The existing knowledge gap on the optimal physiological conditions and efficiency has limited their usage and commercialization. Additionally, since it has been reported that foliar applications in aquaponics drift to aquaponics water (Rašković et al., 2021), it is imperative to investigate the natural capability of the potential fungal biocontrol agents to survive or reproduce in aquaponics. This is because their survival in aquaponics water may be harmful to the fish and beneficial bacteria in coupled aquaponics, where there is a simultaneous presence of fish and nitrifying bacteria in the same loop as plants.
Thus, the present study sought to investigate the efficacy of Lecanicillium attenuatum (LLA), Isaria fumosorosea (IFR), and mycoparasitic fungus Trichoderma virens (TVI) against cucumber powdery mildew under different relative humidity conditions. This was achieved by assessing; (a.) the disease progress and severity, (b.) the disease reduction capacity of the BCAs, (c.) the growth and persistence of the BCAs, and (d.) their survival in the aquaponics medium.
The pathogen of cucumber powdery mildew, Podosphaera xanthii, identified through a diagnostic guide (Kristkova et al., 2009), was collected from the greenhouse of the Faculty of Fisheries and Protection of Waters (FFPW) in the Czech Republic, where the natural infestation was recorded in August 2020. The pathogen was cultured on leaves of healthy cucumber plants (variety Superstar F1, Semo a.s., Czech Republic) and grown for two weeks in an MLR-352 growth chamber (25 ± 1°C; 16h of light, 60-65% RH (Akribis, UK). Fresh cultures of the pathogen were made every two weeks simply by tapping the old infected leaves with new potted cucumber leaves.
Lecanicillium attenuatum, strain CCM 9195 (a new strain, sequence of strain CCM 9195 have been submitted to the NCBI database, barcoding database website https://www.ncbi.nlm.nih.gov, accession No. of the strain is OP503941), was isolated in 2008 from bark beetle adults in NP Sumava, Czech Republic. The strain was identified as L. attenuatum based on microscopic observation and cytochemical analysis using Deng et al. (2010), and Simmons (2007) approaches. The strains of I. fumosorosea-based product, PFR-97 20% WDG, and T. virens-based product, SoilGard®, used in this study were procured from Certis USA LLC., USA. Bioproduct PFR-97 20% WDG, was based on the blastospores of the naturally occurring strain Apopka 97 of the fungus, Isaria fumosorosea, while bioproduct SoilGard® was based on the blastospores of the naturally occurring strain GL-21 of the fungus, Trichoderma virens. Both strains were re-isolated from the bioproducts, and pure cultures were used for the experiments.
All three strains were cultivated in potato dextrose agar (PDA, Difco Laboratories, Sparks, MD, USA) at 25 ± 1°C. Conidial suspensions of each strain were prepared by scraping off conidia (using an inoculating loop) into sterile 0.05% Tween 80 solution. Suspensions were then filtered through a cheesecloth to remove mycelia debris, and the conidia concentration was determined by a Neubauer hemocytometer (Bright-Line™, Sigma-Aldrich, Germany). The suspensions were subsequently adjusted to 1.0x107 conidia/ml.
Experiments were conducted under three relative humidity conditions, ≥ 95%, 65-73%, and ≤40% RH. These relative humidity conditions were regulated in experimental growth chambers (Figure 1A). Each growth chamber contained a smaller grow box (110 × 85× 30 mm) enclosed in a chamber box (145 × 120 × 65 mm). The leaf was placed on the water-filled smaller grow box so that the leaf’s petiole was embedded in the water through a hole driven on the lid. This was then placed in the bigger grow box (chamber box). Relative humidity, ≥ 95%, was regulated using Kim et al. (2007) approach. A soaked paper towel was placed at the bottom of the bigger grow box before the chamber was covered with the lid (Figure 1Aa). Without the soaked paper towels, the relative humidity ranged between 65-73% (Figure 1Ab). When the growth chambers were opened to the ambient temperature of the laboratory (21-24 0C) and not laid with the soaked paper towel, the relative humidity was ≤40%. The relative humidity in each growth chamber was measured using an electronic thermo-hygrometer (TFA Dostmann GmbH & Co.KG, Germany). Each treatment had six replicates (Fungi isolates and relative humidity conditions).
Figure 1 (A) shows the regulating features of the three relative humidity conditions of the growth chambers, ≥ 95% RH (a), 65-73% RH (b), and ≥ 40% RH. (B) shows the flow of activities, treatment setup (1), suspension preparation (2), pretreatment of leaves with isolates (3), and the inoculation of the P. xanthii (4).
To assess the efficacy of the fungal biocontrol agents, detached cucumber leaves were pretreated with the prepared suspension of the fungal isolates (Figures 1B1, 1B2). The pretreatment was carried out by dipping the detached leaves into a suspension of 1.0 x 107 spores/ml for 30 seconds (Figure 1B3). Then, the leaves were left to dry freely on a tabletop for 30 minutes before being placed in the growth chamber. The control leaves were dipped in 0.05% aqueous Tween 80 solution. After 48 hours, powdery mildew pathogen, P. xanthii isolates were inoculated on the treated leaves by scrapping off the conidia from the highly infected leaves with a loop and touching the healthy leaves at 3 points (Figure 1B4). The growth chamber units within treatments were kept 50-60 cm from each other, and the treatments were kept two meters apart to reduce inter-plot interference.
The domination and inhibition capacity of the BCAs was assessed by estimating the area of the leaves covered by the P. xanthii. This was done by estimating the percentage of powdery mildew coverage on the leaves. Disease severity was scored using a 12-grade scale described by Horsfall and Barratt (1945) with minor modifications: 0 = 0%, 1 = 0–3%, 2 = 3–6%, 3 = 6–12%, 4 = 12–25%, 5 = 25–50%, 6 = 50–75%, 7 = 75–87%, 8 = 87–94%, 9 = 94–97%, 10 = 97–100%, 11 = 100% disease. A mean disease severity (DS) was calculated for each treatment by adding the products of the number of infected leaves and their corresponding ratings, thereby dividing the sum by the product of maximum rating value (12), the number of leaves in that entire observation and 100. The formula was expressed as follows;
where Ngrp is the number of leaves in a group, R is the rating value, and Nobsis the number of leaves in the observation. Disease severity assessment was further complemented by evaluating the final disease levels (FDL), the percentage of the leaf area covered by powdery mildew on day 20 after inoculating the BCAs.
To assess the efficacy of the BCAs and the percentage reduction of the disease, the area under the disease progress curve (AUDPC) was calculated using the percentage of the leaf covered by powdery mildew during the experiment. It summarizes the disease intensity over a certain period. A mean AUDPC value was calculated for each treatment at each corresponding relative humidity by adding up the average percentage of the disease (percentage of leaves covered by powdery mildew) in previous and current situations. It is then multiplied by the time (days) in-between differences. The formula was expressed as follows;
where Yi is the percentage of the leaves covered by powdery mildew (Yi/100) at the ith observation and Xi is the day of the ith observation, while n indicates the total number of observations [modified from Shaner and Finney (1977)].
To evaluate the persistence of the BCAs on leaves, three leaves were randomly selected from each treatment on the first day and after 20 days of inoculation. Leaf discs (25 mm in diameter) were collected from each leaf and suspended in a 100 ml 0.05% Tween 80 solution in Erlenmeyer flasks. The flasks were subjected to an orbital shaker (150 rpm) for 30 minutes. The resulting leachate solutions were diluted to obtain a countable number of colony-forming units (CFU). Next, aliquots were spread on plates containing PDA (39 g/l PDA) with 0.25 g of antibiotic chloramphenicol (Sigma-Aldrich, Germany). 0.5 ml was inoculated from each dilution on the growing medium (PDA) surface and cultured at 25°C and 18 h/6 h lighting. The number of CFU of T. virens was counted after two days (48 hrs), while the CFU of I. fumosorosea and L. attenuatum were counted after five days (120 hrs).
In order to investigate the possible effects of the fungal isolates on fish and microbial communities in a typical aquaponics system, it is essential to know if the fungal isolates have the natural capability to germinate in the aquaponics water. Hence, we inoculated them into the aquaponics water and observed their spores germination and sporulation over a stipulated period. This was carried out in two phases; a germination test to observe the spores structures under the microscope at different timepoints and a further quantification of the colonies at the timepoints.
Twelve 200 ml of water were collected in a 250 ml conical flask from the mineralization tank of the FFPW tilapia-based aquaponics system. The water samples were subsequently aerated on a rotary shaker (Sheller, Korea) at 200 rpm. After 48 hours, prepared suspension of the isolates containing 1.0x107 conidia/ml was inoculated into the aerated mineralized water. For comparison, the isolates were inoculated into 200 ml 0.05% tween 80 solutions as control. For the germination test, the RAS-inoculated fungal isolates were cultured in a PDA just after the inoculation in the water. The mycellium and possible sporulation patterns were observed under the microscope after 24 hours. Then, for quantification, in a separate assay, they were cultivated in PDA by adding 0.5ml of the solution to PDA in separate Petri dishes, 90 by 15 mm. The isolate cultivations were carried out at 0, 1, 6, 12, 24, 48, and 96hr from when the water samples were collected. The water samples were left in a shaker for the entirety of the experiment. They were cultivated for 24 hours under room temperature and >70% relative humidity. The CFUs of the isolates were counted after 24 to 120 hrs of cultivation. Prior to counting, the structures of the isolates were first observed under a light microscope to compare their physiology with the control inoculated in tween solution.
The experimental efficacy design was in a randomized complete block design, with each treatment having six replicates. Data were collected from each replicate on days 10, 15, and 20. To assess the impact of the relative humidity on the disease severity and AUDPC, the data were subjected to a two-way analysis of variance (ANOVA) using R statistical software (Wickham, 2016). The comparisons between treatments were performed using Fisher’s protected Least Significant Difference (LSD). We set the statistical significance at the conventional p< 0.05 level. The break-in y-scale bars followed the approach of Xu et al. (2021). To assess the performance of each treatment to the control, we calculated the disease percentage reduction (DPR) for each treatment using the formula below:
Xc is the % of leaf area covered by powdery mildew (for control), and Xt is the corresponding value of the treatments.
On day 20 of inoculation of P. xanthii, leaves in the control group (under ≤40% RH) were covered by the pathogen CFUs. This was used as a sign to mark the end of the experiment. The efficacy of microbiological agents on the progress of the disease considering the assessment of AUDPC was statistically significant (P<0.05) under ≥95% relative humidity (≥95% RH) and 65-73% relative humidity (65-73% RH) (Figure 2). The AUDPC value of the LLA-treated leaves in ≥95% was the lowest (319.6) over the given study period (20 days). The AUDPC of the untreated cucumber leaves (control) grown under ≥ 95% relative humidity was significantly higher (930) than the AUDPC of IFR (529.6) and TVI (606.7) (Figure 2). Similarly, under the 65-73% RH condition, the AUDPC values of the leaves treated with BCAs, LLA (486.7), IFR (434.2), and TVI (545.8), were significantly different from the control. Nevertheless, at ≤ 40% RH, the AUDPC of IFR and TVI treated cucumber leaves values were high, and both were significantly different from the control and LLA.
Figure 2 The disease progress of powdery mildew on cucumber leaves treated with microbiological agents, Lecanicillium attenuatum (LLA), Trichoderma virens (TVI), and Isaria fumosorosea (IFR), and control (Cont). The detached cucumber leaves were grown for 20 days under different relative humidity conditions, ≥95% RH, 65-73% RH, and ≤40% RH. The boxes are the mean ± SE (n = 6). The different letters indicate that values are significantly different at P< 0.05 according to the Least Significance Difference test.
The result indicated that all BCAs tested reduced disease severity (DS %) as compared to control untreated leaves. Considering the analysis for disease severity, at a ≥95% relative humidity condition, both LLA and IFR-treated leaves showed significantly less severity than the control and TVI-treated cucumber leaves. Under 65-73% relative humidity conditions, leaves treated with IFR had low severity rates (32.8%) (Figure 3). Under ≤40% relative humidity condition, there was no significant difference between the disease severity of the control and BCAs (Figure 3).
Figure 3 The disease severity (DS) curves for powdery mildew development on cucumber plants when treated with Lecanicillium attenuatum (LLA), Trichoderma virens (TVI), and Isaria fumosorosea (IFR), and untreated control (Cont). The data represent the disease severity (%) for each week’s treatment under the designed relative humidity conditions, ≥95% RH, 65-73% RH, and ≤40% RH. All the leaves in the treatments were pretreated 72 hours before inoculating P. xanthii. The vertical bars are the mean ± SE (n = 6). The letters indicate that values are significantly different at P< 0.05 according to the Least Significance Difference test.
After 20 days of inoculation with P. xanthii, the final disease level, measured by the percentage of the leaf area covered by powdery mildew, was recorded. The leaves treated with LLA had a significant 11% and 9% of the leaf area covered by powdery mildew under 65-73% RH and ≥95% RH, respectively (Table 2, Figure 4). Under ≥95% RH condition, IFR and TVI were statistically significant from the control with FDL of 18.5% and 25.8%, respectively (Table 1). The FDL leaves treated with the BCAs under 65-73RH significantly differed from the control. It stagnated between 58% and 60% under 65-73 and ≥95% RH, respectively (Table 1). Similarly, the final disease of the cucumber leaves grown under a relative humidity condition of ≤40% showed no significant difference between the treatments and the control.
Figure 4 Efficacy of microbiological agents, L. attenuatum on cucumber leaves artificially infected with powdery mildew. (A) leaves treated with 0.05% tween 80 solution (control) after 20 days of the treatment, and (B) leaves treated with L. attenuatum after 20 days. Both treatments were applied 48 hours before the inoculation of P. xanthii. Other pictorial presentation showing a comparisons of TVI and IFR-treated leaves can be found in the Figure 1 of the supplementary material.
Table 1 The final disease level (FDL) of the cucumber leaves treated with microbiological control agents, Lecanicillium attenuatum (LLA), Isaria fumosorosea (IFR), and Trichoderma virens (TVI), and the untreated control (control) on the 20th day.
The potency of the microbiological agents at disease reduction varied with relative humidity conditions. All the microbial agents were most efficient at controlling the powdery mildew under the ≥95% and 65-73 relative humidity conditions. LLA was the most efficient, with a disease percentage reduction of 85% under 65-73% RH conditions (Figure 5). The disease reduction of IFR and TVI under the same condition was 68.6% and 56%, respectively (Figure 5). In contrast, all the BCAs were largely ineffective under ≤40% RH conditions.
Figure 5 The efficacy of the BCAs: Lecanicillium attenuatum (LLA), Isaria fumosorosea (IFR), and Trichoderma virens (TVI) measured by disease reduction percentage (DPR) of powdery mildew pathogen, Podosphaera xanthii, on cucumber leave grown for 20 days under different relative humidity conditions. The vertical bars are the mean ± SE (n = 6). The different letters indicate that values are significantly different at P< 0.05 according to the Least Significance Difference test.
Nonetheless, there was no significant difference between the mean FDL of the BCAs. In contrast, at low humidity (≤40%), only TVI-treated leaves showed a disease severity (85%) significantly different from the control. The mean FDL of LLA-treated and IFR-treated leaves were insignificant to the control.
A significant reduction in fungal populations were observed in the low relative humidity condition (≤40% RH). TVI significantly reduced from 1.0 × 102 CFU per mm2 of disc area on the first day to 4.97 on day 20. Similarly, the 7.9 × 10 and 7.3 × 10 CFU of I. fumosorosea and T. virens per mm2 of disc area before the inoculation of P. xanthii spores (respectively), significantly reduced to 3.42 × 10 and 1.17× 10 at day 20 respectively (Table 2). In contrast, all the fungal isolates significantly increased under the higher relative humidity conditions, 65-73% and ≥95%. L. attenuatum spores significantly increased to 9.54 × 103 CFUmm-2 in 65-73% RH and 5.51 × 103 CFUmm-2 in ≥95% RH growth chamber. On the other hand, I. fumosorosea spores significantly increased from 7.9 × 10 before the inoculation of P. xanthii spores to 1.60 × 104 CFUmm-2 in 65-73% RH and 5.78 × 103 CFUmm-2 in ≥95% RH growth chamber (Table 2).
Table 2 The mean CFU of fungi isolates found per unit area (mm2) of leaf discs of cucumber leaves treated with biological agents, Lecanicillium attenuatum (LLA), Trichoderma virens (TVI), and Isaria fumosorosea (IFR).
L. attenuatum germinated the most after 24 hrs with a fungal load of about 106 (p<0.05). The fungal load of the LLA, IFR, and TVI significantly reduced from 0 hr to more than 99% after 96 hrs. L. attenuatum fungal load reduced to about 4 x 103 CFU after 96 hrs (Figure 6). On the other hand, IFR fungal load sharply reduced from 4.5 x 105 in 0 hr to 1.8 x 103 in 96 hrs. Similarly, the variation in the structures of the microbes over the 96 hours showed that the organisms could not sporulate in this medium (Figure 7).
Figure 6 The comparisons of colony-forming units of the BCAs, L. attenuatum (LLA), I. Fumosorosea (IFR), and T. virens (TVI), inoculated into aquaponics water in decoupled aquaponics and 0.05% tween 80 solution. The CFUs per culture were counted after 24 to120 hrs of cultivation. Capital letters indicate a significant difference between 1 and 96 hrs, while the small letters indicate significant differences within the time points (1 and 96 hrs). The vertical bars are the mean ± SE (n = 3). The different letters indicate that values are significantly different at P< 0.05 according to the Least Significance Difference test.
Figure 7 The structures of the fungal biocontrol agents, Isaria fumosorosea (IFR), Lecanicillium attenuatum (LLA), and Trichoderma virens (TVI) under an electron microscope, 96 hrs after inoculation in the aquaponics water.
Several studies have identified biological controls as possible alternatives to fungicides against pathogens in aquaponics. Nonetheless, there is currently no biological control treatment approved for use against powdery mildew in aquaponics systems. In addition, there is still a paucity of information on favorable environmental conditions that optimize the efficacy of the BCAs in field and greenhouse crops. Therefore, we investigated the effectiveness of two naturally occurring entomopathogenic fungi and mycoparasitic fungus against P. xanthii under different relative humidity conditions. The efficacy of the BCAs varied depending on the relative humidity of the growth chambers.
All the BCAs investigated in this study showed significant effects against the powdery mildew pathogen, P. xanthii., L. attenuatum was the most efficient, reducing the disease severity by 85% and having a final disease level of 11%. Furthermore, we found that at a relative humidity condition of 65-73%, all the three BCAs showed a strong efficacy against the powdery mildew. Though the result from this study was aimed at providing in-depth information on adopting these fungal biocontrol agents for aquaponics, the results from our study are however, relevant for all cultivation systems. Our findings are consistent with the greenhouse results of Kim et al. (2007). Only a few studies have investigated the potential of L. attenuatum against powdery mildew, but there are results from related species, L. lecanii. Romero et al. (2007) investigated the efficacy of Ampelomyces quisqualis, L. lecanii, and Bacillus substilis against melon powdery mildew under two relative humidity conditions, 75–80% and 90–95%. After three weeks, the authors recorded 61% and 81% disease reduction in leaves treated with L. lecanii under 75-85% and 90–95% RH, respectively. In contrast, Kim et al. (2008) reported no significant differences in the activity of two isolates of L. lecanii against cucumber powdery mildew under RH conditions ≥95%. The discrepancy in the results could be associated with the biological and physical states of the microbiological control agents. Also, it could be related to differences in the strains of L. attenuatum. On the other hand, the focus of this study did not cover the mechanism of action of the fungi isolates against P. xanthii. The effectiveness of L. lecanii against several plant diseases is associated with its antagonistic, parasitic, and disease resistance-inducing characteristics (Goettel et al., 2008).
Isaria fumosorosea has severally been developed as mycopesticides for a broad range of pests, including whiteflies (Faria & Wraight, 2007), mites and ticks (Pena et al., 1996), termites (Yanagawa et al., 2008), thrips (Panyasiri et al., 2007) and aphids (Yeo et al., 2003). However, aside from the study by Kavkova and Curn (2005), which investigated the development and survival of Sphaerotheca fuliginea after treatment with I. fumosorosea, none of the existing studies have investigated its potential as a biocontrol agent against powdery mildew pathogen. Our study found that I. fumosorosea significantly reduced the disease intensity and spread under 65-73% and ≥95% RH conditions. Similarly, the disease severity of leaves treated with IFR under 65-73% RH condition was slightly less than disease severity under ≥95% RH. This could be partly associated with the preference of the powdery mildew spores to the drier condition in the 65-73RH growth chamber. Therefore, favoring an increased mycoparasitism and sporulation of I. fumosorosea. Here, the present study did not investigate the mechanisms of the fungus against the pathogen. But, other studies have identified mycotoxins such as Beauvericin, pyridine-2,6-dicarboxylic acid, and dipicolinic acid found in Isaria fumosorosea (Zimmermann, 2008).
Our study found that TVI was the least efficient BCAs under 65-73% and ≥95% relative humidity conditions. However, its final disease levels significantly differed from the controls under the three relative humidity conditions. Currently, no study has delved into the efficacy of the T. virens against P. xanthii. In contrast, similar results were reported with other species such as Trichoderma harzianum, T. album, T. viride, and T. hamatum (Mmbaga et al., 2008; Elsisi, 2019). The relatively low-efficiency level of Trichoderma in the soilless medium could be associated with its preference for soil medium. Even though a comparatively higher population of TVI spores was found on the leaf hours after inoculation, the population of TVI spores was the least under the three relative humidity conditions on day 20. This could be associated with the fact that T. virens are natural soil-based microbes (Rubin, 2010). Thus, its low survival on a non-soil growing medium may make the beneficial microbial biocontrol agent less suitable for non-soil systems such as aquaponics. Like L. attenuatum and I. fumosorosea, few studies have delved into the mode of action of T.virens.
We also demonstrated that the population of the BCA spores differs at different relative humidities. The population of L. attenuatum and I. fumosorosea spores were significantly higher under 65-73% RH than in ≥95% RH conditions. This may be associated with the fact that P. xanthii has an affinity for drier conditions, and spores of L. attenuatum does not germinate in the absence of P. xanthii (Miller et al., 2004; Rennberger et al., 2018). But, the persistence of the spores under optimal relative humidity conditions (≥95%), where there are few powdery spores, shows that L. attenuatum and I. fumosorosea spores sporulate survive on the leaves’ wax. However, these growth stages cannot protect the plants against further infestation of P. xanthii (Miller et al., 2004). As a consequence, a repeated application would be necessary to replace the spores and protect the plant. Miller et al. (2004) reported that spores of L. lecanii applied did not germinate except in the presence of powdery mildew (or some other fungus). In addition, the applied spores were found in ungerminated form, except when they were within immediate (B/100 mm) proximity to S. macularis f. sp. fragariae spores or colonies. Despite this, there are arguments that the spores population of heterotrophic microorganisms such as these fungi can be manipulated in aquaponics systems (Stouvenakers et al., 2019).
Despite the observed growth of the BCAs in the aquaponics water, the chains of their mycelia were found to ‘shrink’ with time. All the fungal loads of the BCAs inoculated in the aquaponics water were reduced by over 99% after 96 hrs of inoculation. This indicates that after 6-7 days of application, there may not be any traces of the BCAs in a typical coupled aquaponics system In addition, a 10% runoff of spraying solution ending up in hydroponics/aquaponics suggested by Folorunso et al. (2021), would arithmetically mean that a less significant population of the fungi would be left after 96 hrs. Though, no study has evaluated the surviving abilities of these BCAs in aquaponics, their sharp loss over the short timeframe found in our study could be associated with certain factors including; unfavourable water quality parameters (e.g. temperature, dissolve oxygen, pH); biotic factors (such as antagonistic aquatic microbes) or water chemical parameters (e.g. C:N ratio, biochemical oxygen demand, chemical oxygen demand) (Stouvenakers et al., 2020; Mann and Davis, 2021; Sharma et al., 2021). This partly explains why entomopathogenic fungi have not been reported as an integral part of the microbial communities in aquaponics. (Eck et al., 2019; Schmautz et al., 2022). In addition, the inability of these BCAs to survive over time in a typical aquaponics system could also be associated with the absence of organic carbon sources, essential for entomopathogenic cell divisions and further survival (Stouvenakers et al., 2019). Therefore, if these BCAs drift off to an aquaponic nutrient medium during spraying, their survival in such medium may depend (over time) on the availability of sufficient carbon. In another study (Leonard et al., 2002), the survival of BCAs in water was reported to vary due to the absence or presence of an inadvertent supply of carbon sources.
Stouvenakers et al. (2019) reviewed the possibility of using indigenous heterotrophic microorganisms in aquaponics as plant protection against pathogens. The authors suggested that the multiplication of heterotrophic microbes in aquaponics can be harnessed by adding organic compounds such as humic substances. Though, unregulated multiplication of the heterotrophic microorganisms in coupled aquaponics systems can establish a competitive environment with the nitrifying bacteria (Leonard et al., 2002; Stouvenakers et al., 2019). Thus, this can alter the optimization of the biofilter. Therefore, if a disease reduction level achieved with the first dose application is still below the economic threshold, a second application should be withheld and applied later to avoid possible effects on the natural microbial load of the aquaponics systems. It is noteworthy to state that the significant drop in the fungi population does not significantly translate to biological significant, as none of the fungi used in this study at these concentrations has been associated with any human health defects.
Aside from Sirakov et al. (2016) study, which investigated the efficacy of indigenous bacterial isolates of aquaponics against Pythium ultimum in vitro, there are currently no research studies on the use of BCAs adoptable for aquaponics. The present study showed a remarkable result in controlling and suppressing P. xanthii in cucumber leaves pretreated with entomopathogenic and mycoparasitic fungi. Findings show that at a 107 CFUml-1 concentration, L. attenuatum was the most efficient under 65-73% relative humidity, a practically sustainable condition in the greenhouse. The overall AUDPC of the treated leaves over the given period (20 days) and disease severity showed that the leaves treated with L. attenuatum, I. fumosorosea, and T. virens were significantly the least infected by P. xanthii. So, L. attenuatum, I. fumosorosea, and T. virens efficiently controlled powdery mildew under high relative humidity conditions. An accidental drift or runoff of the fungal isolates into the aquaponics system during foliar application may not generate a significant problem, as their survival in aquaponics was non-significant. Their low survival in water could make them a sustainable and selective option for controlling fish pathogens at higher concentrations and stipulated timeframe. Hence, future research should focus on the dual role of the fungal isolates against both plant and fish pathogens in aquaponics.
The raw data supporting the conclusions of this article will be made available by the authors, without undue reservation.
EAF, AB, RG, MK, and JM contributed to the conception and design of the study. EAF wrote the first draft of the manuscript and performed the statistical analysis. EAF, AB, RG, MK, and JM contributed to the data interpretation and revision of the manuscript. EAF, AB, JM, MK, and RG wrote sections of the manuscript. All authors contributed to manuscript revision, and approved the submitted version.
The study was financed by the Grant Agency of the University of South Bohemia (GAJU), No. 053/2021/Z, Ministry of Education, Youth and Sports of the Czech Republic (MSMT) under project CENAKVA (LM2018099), and the Ministry of Agriculture of the Czech Republic, National Agency for agricultural Research (NAZV), No. QK 22010255.
The technical assistance of the laboratory member, Mrs. Olga Divisova, is gratefully appreciated. The authors greatly appreciate the help of the reviewers in improving this article.
Author MK was employed by Dairy Research Institute, Ltd.
The remaining authors declare that the research was conducted in the absence of any commercial or financial relationships that could be construed as a potential conflict of interest.
All claims expressed in this article are solely those of the authors and do not necessarily represent those of their affiliated organizations, or those of the publisher, the editors and the reviewers. Any product that may be evaluated in this article, or claim that may be made by its manufacturer, is not guaranteed or endorsed by the publisher.
The Supplementary Material for this article can be found online at: https://www.frontiersin.org/articles/10.3389/fmars.2022.992715/full#supplementary-material
Abbaszadeh G., Dhillon M., Srivastava C., Gautam R. D. (2011). Biopesticides international. Biopesticides International 7, 1–14.
Anand P., Isar J., Saran S., Saxena R. K. (2006). Bioaccumulation of copper by Trichoderma viride. Biores. Technol. 97, 1018–1025. doi: 10.1016/j.biortech.2005.04.046
Askary H., Carriere Y., Belanger R. R., Brodeur J. (1998). Pathogenicity of the fungus Verticillium lecanii to aphids and powdery mildew. Biocontrol Sci. Technol. 8, 23–32. doi: 10.1080/09583159830405
Avery P. B., Wekesa V. W., Hunter W. B., Hall D. G., McKenzie C. L., Osborne L. S., et al. (2011). Effects of the fungus isaria fumosorosea (Hypocreales: Cordycipitaceae) on reduced feeding and mortality of the Asian citrus psyllid, diaphorina citri (Hemiptera: Psyllidae). Biocontrol Sci. Technol. 21, 1065–1078. doi: 10.1080/09583157.2011.596927
Carbó A., Torres R., Usall J., Ballesta J., Teixidó N. (2020). Biocontrol potential of Ampelomyces quisqualis strain CPA-9 against powdery mildew: Conidia production in liquid medium and efficacy on zucchini leaves. Sci. Hortic. 267, 109337. doi: 10.1016/j.scienta.2020.109337
Chet I., Inbar J. (1994). Biological control of fungal pathogens. Appl. Biochem. Biotechnol. 48, 37–43. doi: 10.1007/BF02825358
Deng J. X., Kim C. S., Oh E. S., Yu S. H. (2010). First report of foliar blight on dendropanax morbifera caused by Alternaria panax. Mycobiology 38, 316–320. doi: 10.4489/MYCO.2010.38.4.316
Devi K. U., Sridevi V., Ch. M., Padmavathi J. (2005). Effect of high temperature and water stress on in vitro germination and growth in isolates of the entomopathogenic fungus beauveria bassiana (Bals.) vuillemin. J. Invertebr. Pathol. 88, 181–189. doi: 10.1016/j.jip.2005.02.001
Drummond J., Heale J. B., Gillespie A. T. (1987). Germination and effect of reduced humidity on expression of pathogenicity in Verticillium lecanii against the glasshouse whitefly Trialeurodes vaporariorum. Ann. Appl. Biol. 111, 193–201. doi: 10.1111/j.1744-7348.1987.tb01446.x
Eck M., Sare A. R., Massart S., Schmautz Z., Junge R., Smits T. H. M., et al. (2019). Exploring bacterial communities in aquaponic systems. Water 11, 260. doi: 10.3390/w11020260
Elad Y. (2000). Biological control of foliar pathogens by means of Trichoderma harzianum and potential modes of action. Crop Prot. 19, 709–714. doi: 10.1016/S0261-2194(00)00094-6
Elsisi A. A. (2019). Evaluation of biological control agents for managing squash powdery mildew under greenhouse conditions. Egyptian J. Biol. Pest Control 29, 89. doi: 10.1186/s41938-019-0194-9
Faria M. R., Wraight S. P. (2007). Mycoinsecticides and mycoacaricides: A comprehensive list with worldwide coverage and international classification of formulation types. Biol. Control 43, 237–256. doi: 10.1016/j.biocontrol.2007.08.001
Folorunso E. A., Roy K., Gebauer R., Bohatá A., Mraz J. (2021). Integrated pest and disease management in aquaponics: A metadata-based review. Rev. Aquacult. 13, 971–995. doi: 10.1111/raq.12508
Gafni A., Calderon C. E., Harris R., Buxdorf K., Dafa-Berger A., Zeilinger-Reichert E., et al. (2015). Biological control of the cucurbit powdery mildew pathogen Podosphaera xanthii by means of the epiphytic fungus Pseudozyma aphidis and parasitism as a mode of action. Front. Plant Sci. 6. doi: 10.3389/fpls.2015.00132
Giotis C., Markellou E., Theodoropoulou A., Kostoulas G., Wilcockson S., Leifert C. (2012). The effects of different biological control agents (BCAs) and plant defence elicitors on cucumber powdery mildew (Podosphaera xanthii). Organic Agric. 2, 89–101. doi: 10.1007/s13165-012-0031-x
Goddek S., Joyce A., Wuertz S., Körner O., Bläser I., Reuter M., et al. (2019). “Decoupled aquaponics systems,” in Aquaponics food production systems (Cham, Switzerland: Springer International Publishing), 201–229.
Goettel M. S., Koike M., Kim J. J., Aiuchi D., Shinya R., Brodeur J. (2008). Potential of lecanicillium spp. for management of insects, nematodes and plant diseases. J. Invertebr. Pathol. 98, 256–261. doi: 10.1016/j.jip.2008.01.009
Hafez Y. M., El-Nagar A. S., Elzaawely A. A., Kamel S., Maswada H. F. (2018). Biological control of Podosphaera xanthii the causal agent of squash powdery mildew disease by upregulation of defense-related enzymes. Egyptian J. Biol. Pest Control 28, 57. doi: 10.1186/s41938-018-0058-8
Henis Y., Chet I. (1975). “Microbiological control of plant pathogens,” in Advances in applied microbiology, vol. 19 . Ed. Perlman D. (Cambridge, Massachusetts: Academic Press) 85–111. doi: 10.1016/S0065-2164(08)70426-X
Horsfall J. G., Barratt R. W. (1945). An improved grading system for measuring plant disease. Phytopathology 35, 655.
Kavkova M., Curn V. (2005). Paecilomyces fumosoroseus (Deuteromycotina: Hyphomycetes) as a potential mycoparasite on Sphaerotheca fuliginea (Ascomycotina: Erysiphales). Mycopathologia 159, 53–63. doi: 10.1007/s11046-003-0787-3
Kim J. J., Goettel M. S., Gillespie D. R. (2007). Potential of Lecanicillium species for dual microbial control of aphids and the cucumber powdery mildew fungus, sphaerotheca fuliginea. Biol. Control 40, 327–332. doi: 10.1016/j.biocontrol.2006.12.002
Kim J. J., Goettel M. S., Gillespie D. R. (2008). Evaluation of lecanicillium longisporum, vertalec® for simultaneous suppression of cotton aphid, Aphis gossypii, and cucumber powdery mildew, Sphaerotheca fuliginea, on potted cucumbers. Biol. Control 45, 404–409. doi: 10.1016/j.biocontrol.2008.02.003
Kristkova E., Lebeda A., Sedlakova B. (2009). Species spectra, distribution and host range of cucurbit powdery mildews in the Czech republic, and in some other European and middle Eastern countries. Phytoparasitica 37, 337–350. doi: 10.1007/s12600-009-0045-4
Lennard W., Goddek S. (2019). “Aquaponics: The basics,” in Aquaponics food production systems: Combined aquaculture and hydroponic production technologies for the future. Eds. Goddek S., Joyce A., Kotzen B., Burnell G. M. (Cham, Switzerland: Springer International Publishing) 113–143. doi: 10.1007/978-3-030-15943-6_5
Leonard N., Guiraud J. P., Gasset E., Cailleres J. P., Blancheton J. P. (2002). Bacteria and nutrients–nitrogen and carbon–in a recirculating system for sea bass production. Aquacult. Eng. 26, 111–127. doi: 10.1016/S0144-8609(02)00008-0
Liu B., Ji S., Zhang H., Wang Y., Liu Z. (2020). Isolation of Trichoderma in the rhizosphere soil of syringa oblata from Harbin and their biocontrol and growth promotion function. Microbiol. Res. 235, 126445. doi: 10.1016/j.micres.2020.126445
Mann A. J., Davis T. S. (2021). Entomopathogenic fungi to control bark beetles: A review of ecological recommendations. Pest Manage. Sci. 77, 3841–3846. doi: 10.1002/ps.6364
Miller T. C., Gubler W. D., Laemmlen F. F., Geng S., Rizzo D. M. (2004). Potential for using Lecanicillium lecanii for suppression of strawberry powdery mildew. Biocontrol Sci. Technol. 14, 215–220. doi: 10.1080/09583150310001639204
Mmbaga M. T., Sauvé R. J., Mrema F. A. (2008). Identification of microorganisms for biological control of powdery mildew in Cornus florida. Biol. Control 44, 67–72. doi: 10.1016/j.biocontrol.2007.10.018
Monsees H., Kloas W., Wuertz S. (2017). Decoupled systems on trial: Eliminating bottlenecks to improve aquaponic processes. PloS One 12, 1–18. doi: 10.1371/journal.pone.0183056
Ni L., Punja Z. K. (2021). Management of powdery mildew on greenhouse cucumber (Cucumis sativus l.) plants using biological and chemical approaches. Can. J. Plant Pathol. 43, 35–42. doi: 10.1080/07060661.2020.1746694
Panyasiri C., Attathom T., Poehling H.-M. (2007). Pathogenicity of entomopathogenic fungi-potential candidates to control insect pests on tomato under protected cultivation in Thailand. J. Plant Dis. Prot. 114, 278–287. doi: 10.1007/BF03356230
Pena J. E., Osborne L. S., Duncan R. E. (1996). Potential of fungi as biocontrol agents of Polyphagotarsonemus latus (Acari: Tarsonemidae). Entomophaga 41, 27. doi: 10.1007/BF02893289
Pollastro S., Laguardia C., Dongiovanni C., Rotondo P. R., De Miccolis Angelini R. M., Raguseo C., et al. (2022). Mating type and fungicide resistance in populations of podosphaera xanthii in south Italy. Plant Pathol. 71, 1369–1380. doi: 10.1111/ppa.13560
Rakocy J. E. (2012). “Aquaponics-integrating fish and plant culture,” in Aquaculture production systems. Ed. Tidwell J. H. (Hoboken, NJ: Wiley-Blackwell) 344–386. doi: 10.1002/9781118250105.ch14
Rašković B., Dvořák P., Mráz J. (2021). Effects of biodegradable insecticides on biofilter bacteria: Implications for aquaponics. Turkish J. Fish. Aquat. Sci. 21, 169–177. doi: 10.4194/1303-2712-v21_4_02
Rennberger G., Gerard P., Keinath A. P. (2018). Occurrence of Foliar Pathogens of Watermelon on Commercial Farms in South Carolina Estimated with Stratified Cluster Sampling. Plant Disease 102 (11), 2285–2295. doi: 10.1094/PDIS-03-18-0468-RE
Rivas-García T., González-Estrada R. R., Chiquito-Contreras R. G., Reyes-Pérez J. J., González-Salas U., Hernández-Montiel L. G., et al. (2020). Biocontrol of phytopathogens under aquaponics systems. Water 12, 1–15. 2061 doi: 10.3390/w12072061
Rivas F., Nuñez P., Jackson T., Altier N. (2014). Effect of temperature and water activity on mycelia radial growth, conidial production and germination of lecanicillium spp. isolates and their virulence against Trialeurodes vaporariorum on tomato plants. BioControl. 59, 99–109. doi: 10.1007/s10526-013-9542-y
Romero D., Vicente A. D., Zeriouh H., Cazorla F. M., Fernández-Ortuño D., Torés J. A., et al. (2007). Evaluation of biological control agents for managing cucurbit powdery mildew on greenhouse-grown melon. Plant Pathol. 56, 976–986. doi: 10.1111/j.1365-3059.2007.01684.x
Rubin A. L. (2010). “Microbial pest control agents: Use patterns, registration requirements, and mammalian toxicity,” in Hayes’ handbook of pesticide toxicology, 3rd ed. Ed. Krieger R. (New York: Academic Press) 441–461 doi: 10.1016/B978-0-12-374367-1.00013-6
Sarhan E. A. D., Abd-Elsyed M. H. F., Ebrahiem A. M. Y. (2020). Biological control of cucumber powdery mildew (Podosphaera xanthii) (Castagne) under greenhouse conditions. Egyptian J. Biol. Pest Control 30, 65. doi: 10.1186/s41938-020-00267-4
Savvas D., Giotis D., Chatzieustratiou E., Bakea M., Patakioutas G. (2009). Silicon supply in soilless cultivations of zucchini alleviates stress induced by salinity and powdery mildew infections. Environ. Exp. Bot. 65, 11–17. doi: 10.1016/j.envexpbot.2008.07.004
Sawant I. S., Wadkar P. N., Ghule S. B., Rajguru Y. R., Salunkhe V. P., Sawant S. D. (2017). Enhanced biological control of powdery mildew in vineyards by integrating a strain of trichoderma afroharzianum with sulphur. Biol. Control 114, 133–143. doi: 10.1016/j.biocontrol.2017.08.011
Schmautz Z., Walser J.-C., Espinal C. A., Gartmann F., Scott B., Pothier J. F., et al. (2022). Microbial diversity across compartments in an aquaponic system and its connection to the nitrogen cycle. Sci. Total Environ. 852, 158426. doi: 10.1016/j.scitotenv.2022.158426
Schuerger A. C., Hammer W. (2003). Suppression of powdery mildew on greenhouse-grown cucumber by addition of silicon to hydroponic nutrient solution is inhibited at high temperature. Plant Dis. 87, 177–185. doi: 10.1094/PDIS.2003.87.2.177
Shaner G., Finney R. E. (1977). The effect of nitrogen fertilization on the expression of slow-mildewing resistance in Knox wheat. Phytopathology 77, 1051. doi: 10.1094/Phyto-67-1051
Sharma L., Oliveira I., Gonçalves F., Raimundo F., Singh R. K., Torres L., et al. (2021). Effect of soil chemical properties on the occurrence and distribution of entomopathogenic fungi in Portuguese grapevine fields. Pathogens 10, 137. doi: 10.3390/pathogens10020137
Simmons E. G. (2007). Alternaria: an identification manual. Utrecht: CBS Fungal Biodivers. Centre p, 38.
Sirakov I., Lutz M., Graber A., Mathis A., Staykov Y., Smits T. H. M., et al. (2016). Potential for combined biocontrol activity against fungal fish and plant pathogens by bacterial isolates from a model aquaponic system. Water 8, 518. doi: 10.3390/w8110518
Stouvenakers G., Dapprich P., Massart S., Jijakli M. H. (2019). “Plant pathogens and control strategies in aquaponics,” in Aquaponics food production systems: Combined aquaculture and hydroponic production technologies for the future. Eds. Goddek S., Joyce A., Kotzen B., Burnell G. M. (Cham, Switzerland: Springer International Publishing), 353–378. doi: 10.1007/978-3-030-15943-6_14
Stouvenakers G., Massart S., Depireux P., Jijakli M. H. (2020). Microbial origin of aquaponic water suppressiveness against pythium aphanidermatum lettuce root rot disease. Microorganisms 8, 1683. doi: 10.3390/microorganisms8111683
Tesfagiorgis H. B., Laing M. D., Annegarn H. J. (2014). Evaluation of biocontrol agents and potassium silicate for the management of powdery mildew of zucchini. Biol. Control 73, 8–15. doi: 10.1016/j.biocontrol.2014.03.010
Wang D., Deng J., Pei Y., Li T., Jin Z., Liang L., et al. (2017). Identification and virulence characterization of entomopathogenic fungus Lecanicillium attenuatum against the pea aphid Acyrthosiphon pisum (Hemiptera: Aphididae). Appl. Entomol. Zool. 52, 511–518. doi: 10.1007/s13355-017-0503-2
Wickham H. (2016). “Data analysis,” in Ggplot2: Elegant graphics for data analysis. Ed. Wickham H. (Cham, Switzerland: Springer International Publishing) 189–201. doi: 10.1007/978-3-319-24277-4_9
Woo S. L., Ruocco M., Vinale F., Nigro M., Marra R., Lombardi N., et al. (2014). Trichoderma-based products and their widespread use in agriculture. Open Mycol. J. 8, 71–126. doi: 10.2174/1874437001408010071
Xie M., Zhang Y.-J., Peng D.-L., Zhou J., Zhang X.-L., Zhang Z.-R., et al. (2015). Persistence and viability of Lecanicillium lecanii in Chinese agricultural soil. PloS One 10, e0138337. doi: 10.1371/journal.pone.0138337
Xu S., Chen M., Feng T., Zhan L., Zhou L., Yu G. (2021). Use ggbreak to effectively utilize plotting space to deal with Large datasets and outliers. Front. Genet. 12. doi: 10.3389/fgene.2021.774846
Yanagawa A., Yokohari F., Shimizu S. (2008). Defense mechanism of the termite, Coptotermes formosanus shiraki, to entomopathogenic fungi. J. Invertebr. Pathol. 97, 165–170. doi: 10.1016/j.jip.2007.09.005
Yeo H., Pell J. K., Alderson P. G., Clark S. J., Pye B. J. (2003). Laboratory evaluation of temperature effects on the germination and growth of entomopathogenic fungi and on their pathogenicity to two aphid species. Pest Manage. Sci. 59, 156–165. doi: 10.1002/ps.622
Keywords: aquaponics, Lecanicillium attenuatum, Isaria fumosorosea, Trichoderma virens, powdery mildew, biological control, Aquaponics, hydroponics
Citation: Folorunso EA, Bohatá A, Kavkova M, Gebauer R and Mraz J (2022) Potential use of entomopathogenic and mycoparasitic fungi against powdery mildew in aquaponics. Front. Mar. Sci. 9:992715. doi: 10.3389/fmars.2022.992715
Received: 12 July 2022; Accepted: 13 October 2022;
Published: 07 November 2022.
Edited by:
Luis R. Martinez-Cordova, University of Sonora, MexicoReviewed by:
Nemat O. Keyhani, University of Florida, United StatesCopyright © 2022 Folorunso, Bohatá, Kavkova, Gebauer and Mraz. This is an open-access article distributed under the terms of the Creative Commons Attribution License (CC BY). The use, distribution or reproduction in other forums is permitted, provided the original author(s) and the copyright owner(s) are credited and that the original publication in this journal is cited, in accordance with accepted academic practice. No use, distribution or reproduction is permitted which does not comply with these terms.
*Correspondence: Jan Mraz, am1yYXpAZnJvdi5qY3UuY3o=
†ORCID ID: Ewumi Azeez Folorunso, orcid.org/0000-0003-2664-6195
Andrea Bohatá, orcid.org/0000-0002-3646-5366
Miloslava Kavkova, orcid.org/0000-0001-7004-8315
Radek Gebauer, orcid.org/0000-0003-0894-0577
Jan Mraz, orcid.org/0000-0003-3545-1269
Disclaimer: All claims expressed in this article are solely those of the authors and do not necessarily represent those of their affiliated organizations, or those of the publisher, the editors and the reviewers. Any product that may be evaluated in this article or claim that may be made by its manufacturer is not guaranteed or endorsed by the publisher.
Research integrity at Frontiers
Learn more about the work of our research integrity team to safeguard the quality of each article we publish.