- 1Laboratory of Environmental Geochemistry and Marine Pollution, Center of Human and Natural Sciences, Department of Oceanography, Federal University of Espírito Santo, Vitória, Espírito Santo, Brazil
- 2Laboratory of Marine Biogeochemistry, Center of Bioscience, Department of Oceanography and Limnology, Federal University of Rio Grande do Norte, Natal, Rio Grande do Norte, Brazil
- 3Laboratory of Ecology and Biodiversity of Aquatic Microproducers, Center of Human and Natural Sciences, Department of Oceanography, Federal University of Espírito Santo, Vitória, Espírito Santo, Brazil
- 4Laboratory of Chemistry, Institute of Exact Sciences, Department of Chemistry, Federal University of Minas Gerais, Belo Horizonte, Minas Gerais, Brazil
- 5Environmental Research Center, FAESA University Center, Vitória, ES, Brazil
- 6Laboratory of Applied Limnology, Federal University of São João del Rei, São João de Rei, Minas Gerais, Brazil
- 7Department of Civil Engineering, Technical University of Denmark, Lyngby, Denmark
- 8Laboratory of Limnology and Environmental Planning, Center of Human and Natural Sciences, Department of Oceanography and Ecology, Federal University of Espírito Santo, Vitória, Espírito Santo, Brazil
Recently, many dam rupture disasters related to the mining industry have caused significant disruption to ecosystems globally. Contaminants, including nutrients in excess, can be transported from the river watersheds to the ocean in great quantities. In 2015, millions of m3 of iron ore tailings were released in the Doce River in southeastern Brazil, leading to several damaging effects to the river and marine ecosystem. So far, few studies have integrated the impact of these tailings on the river and the sea simultaneously. This research aims to provide a perspective on the macronutrients and iron concentrations and balance in the Doce River and the adjacent continental shelf, the related abiotic factors and the phytoplankton response under a new steady state of iron enrichment environment. The iron ore tailings increased the concentrations of nutrients in the river and on the continental shelf, especially for dissolved silica and iron. There was also a long-term indirect phosphorus enrichment associated to adsorption/desorption onto Fe-oxides and a nitrogen increase possibly due to N-fixing enhanced by iron. N fixation/remineralization predominates in the river watershed, while in the sea, the hydrodynamics provides nutrients supplied by the river and recycling from sediment resuspension. Nutrient ratios show DIP limitation in the river, while the complex marine environment brings both limitations on DIN, DIP, and DSi, as well as a stoichiometric equilibrium to the dataset. The phytoplankton response to the abiotic factors was more representative in the river watershed than on the continental shelf. This work emphasizes the importance of reporting local data for understanding global land-ocean nutrient fluxes and their potential influence on primary productivity and trophic chain balance.
1 Introduction
Coastal waters influenced by relevant fluvial discharge receive significant amounts of weathered and anthropogenic materials providing essential compounds for ecosystem balance. Once in the ocean, the particles, molecules, compounds, and ions are continuously transformed by biogeochemical interactions and physical dynamics. During this process, internal transformations such as abiotic and biotic recycling and remineralization of carbon (C), nitrogen (N), phosphorous (P), silicate (Si), and iron (Fe) in coastal oceans occur and may be altered by human activities leading to nutrient enrichment from developed watersheds (Howarth, 2000; Marone et al., 2005; Paerl, 2009).
Nutrients become limiting when available in concentrations below those required for phytoplankton growth. They are needed in specific stoichiometric proportions for organic matter production, in a ratio traditionally called the Redfield ratio of N:P:Si (16:1:16). Silica (Si) is specific for some microalgae, such as diatoms, for which this element is the main component of the frustules (Conley et al., 1989). Primary production can be limited when one of these nutrients occurs below the expected reference value. Likewise, limitation occurs due to disproportionate distribution when there is an excess of one in relation to the other (Garnier et al., 2010). The organic and inorganic forms of N are often more soluble and, therefore, more available than P, enhancing the limitation of this nutrient (Paerl, 2009). Furthermore, nitrogen can be fixed directly from the atmosphere by N-fixing species, while phosphorus has exclusively geological sources. In most oceans, it is common for nitrogen to limit primary production (Tyrrell, 2001; Voss et al., 2013), although, in some environments, phosphorus is often limiting (Krom et al., 1991).
In addition to the macronutrients described above, iron (Fe) is the main micronutrient that controls the primary productivity and balance of the marine ecosystem. Fe is considered a co-limiting factor for the uptake of macronutrients (nitrogen, phosphorus, and silicate) in oligotrophic regions (Morel et al., 1991). Nitrogen and phosphorus are major constituents of cellular biomass, and iron is needed for electron transfer in proteins and enzymes, including those essential for photosynthesis. As mentioned by Bristow et al. (2017), the Redfield ratio is complemented by iron in a proportion of 0.0075 parts, which means N:P:Si:Fe corresponds to 16:1:16:0.0075.
River flow is the primary hydrological input determining the nutrients delivered from the continent to the coastal sea (Meybeck, 1994). Environmental changes in the natural circulation of nutrients and iron and their possible implications for the functioning of coastal ecosystems must be investigated within a temporal and spatial context, considering flux rates between continental and adjacent coastal systems. Current industrial, urban, and rural activities add excessive nutrients, metals, and other materials to aquatic systems (Howarth, 2000). As physical and biogeochemical processes determine the availability of nutrients in hydrological systems, multidisciplinary approaches are crucial to understanding these land-ocean fluxes in coastal regions heavily impacted by anthropogenic activities.
In this sense, the Doce River (Southern Brazil) stands as an important fluvial system both in its variable streamflow, varying from low (under 459 m3/s) to high flows (above 646 m3/s) (Oliveira and da Silva Quaresma, 2017) and from the anthropogenic impacts along its watershed (Santolin et al., 2015). Besides commonplace contamination, the Doce River had become a crucial system of study after the global most extensive environmental disaster in the mining industry in November 2015, when millions of m3 of iron ore tailings were spilled into its watershed after the Fundão dam rupture (Southern Brazil) (Segura et al., 2016). This study is part of large-scale environmental monitoring carried out in the affected area (river and ocean), with monthly data collection from October 2018 to September 2021. The understanding of nutrient dynamics in the coastal waters impacted by iron ore spills is important for supporting knowledge on primary productivity (Costa et al., 2021), considering that some of those, such as silicate and iron, are among the main constituents of tailings (Grilo et al., 2018; Orlando et al., 2020). This can disturb the aquatic biota, leading to an unbalanced food chain and loss of ecosystem health, making this system an in-situ laboratory for monitoring the effects of human activities on the land-ocean interface. The goal of this research is to understand what influences the macronutrients and dissolved iron inputs through the Doce River to the continental shelf during wet and dry seasons, and the impact on primary production, thorough investigation of a) macronutrients (N, Si, and P) and dissolved iron ratios, and C and N isotopes in the lower Doce River and its adjacent continental shelf; b) the phytoplankton responses to this nutrient ratio; c) the interactions among abiotic variables with biotic variables throughout the river and the continental shelf after the dam rupture.
2 Materials and methods
2.1 Study area
The mouth of the Doce River is a wave-dominated delta system, where sea-level changes have created a submerged delta over the continental shelf (Rossetti et al., 2015). Its large sediment volume strongly influences the adjacent continental shelf with materials transported from its watershed (Albino and Suguio, 2010; Bastos et al., 2015; Quaresma et al., 2015; Santolin et al., 2015). The Doce River watershed comprises an interstate area of approximately 83,400 km2 (86% in Minas Gerais state and 14% in Espírito Santo state) in southeast Brazil, as shown in Figure 1. The upper watershed section is part of the “Iron Quadrangle” Region, one of the largest and most recognized mineral deposits in the world (Bundschuh et al., 2012), where the Fundão dam containing iron ore tailings collapsed in 2015, dumping 3.5 x 107 of m3 of ore tailing into the study area (ANA - Agência Nacional de Águas e Saneamento Básico, 2015; Segura et al., 2016; Cagnin et al., 2017).
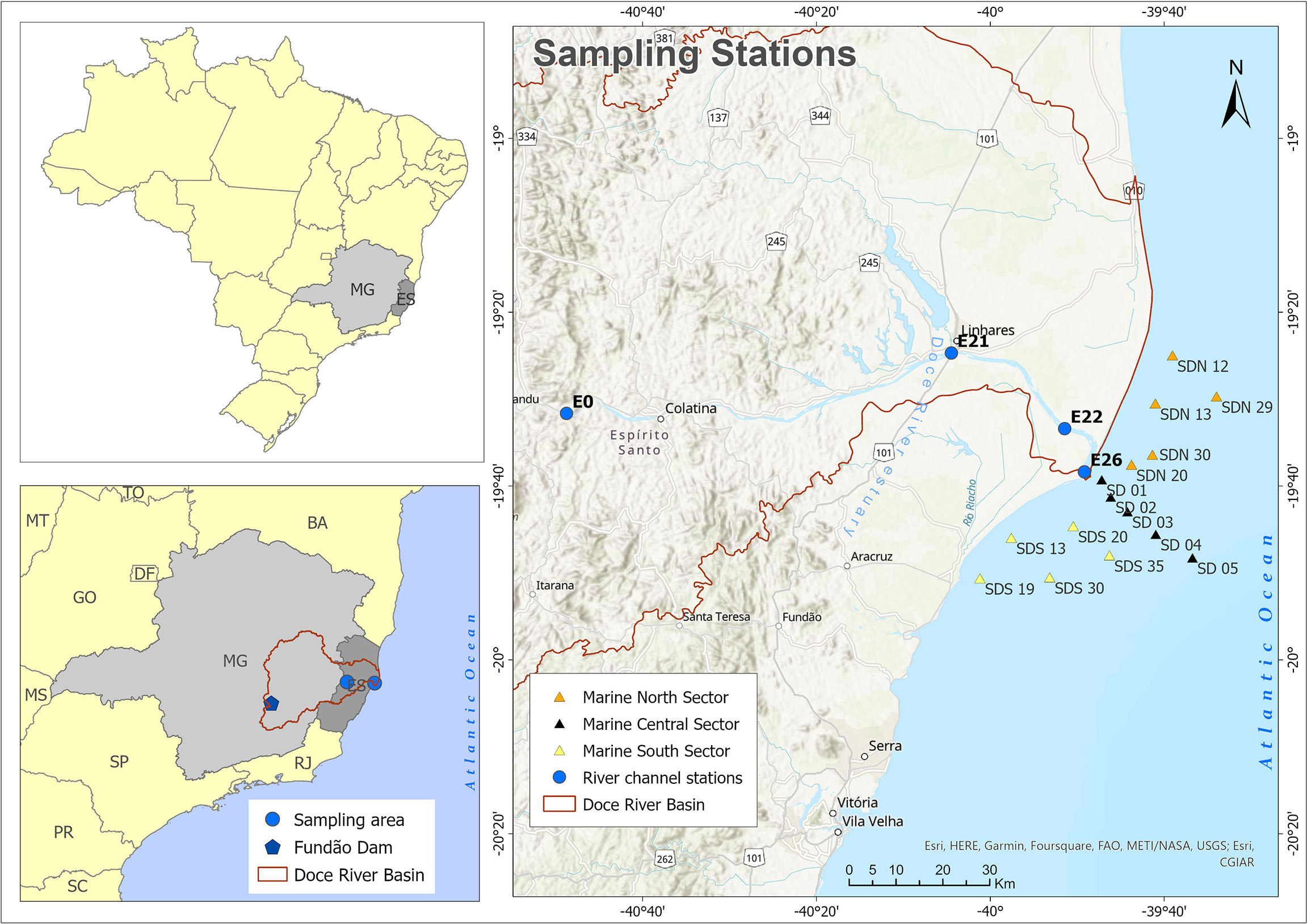
Figure 1 Study area on the southeast coast of Brazil. Light grey is the Minas Gerais State, where the Fundão iron ore tailing dam collapsed (blue star); Dark grey is the Espírito Santo State, where the coastal sampling area is located. Doce River watershed boundary is delimited by the brown line. The blue dots are the sampling area and sampling stations in the Doce River channel; the orange triangles are the marine north sector (MN), the black triangles are the marine central sector (MC) and the yellow triangles are the marine south sector (MS).
According to Oliveira and da Silva Quaresma (2017), the Doce River streamflow and suspended sediment load characteristics can be divided into two contrasting seasons. The dry season is from April to September, characterized by lower median streamflow (368 m3/s). The wet season occurs between October and March and is characterized by higher median streamflow (965 m3/s) with increased sediment loads and high-water discharge. During flooding conditions (more frequent in December and January), the river discharge is higher than 2.300 m3/s (Oliveira and da Silva Quaresma, 2017). The collected data in this study were categorized monthly according to these flow regimes (wet and dry seasons/periods, Table 1).
The most frequent winds on the continental shelf blow from the N, NE, and E, especially during the wet season (October to March). The passage of frontal systems shifts the wind direction to S-SE quadrants during the fall and winter (April to September - dry season) (Cavalcanti et al., 2009). Waves with 0.5 to 1.5 m heights coming from the NE-ENE wind quadrant occur more frequently offshore. SE waves related to frontal systems (up to 2.0 m) are less common and occur mainly during the dry season. Waves from the NE ranging between 1.0 and 1.5 m are frequent during the wet season in the onshore region (Albino et al., 2016).
The study area comprises four sampling stations downstream of the river channel (lower Doce River) and fifteen stations on the continental shelf adjacent to the river mouth (Espírito Santo state, Southeast Brazil) (Figure 1). The sampling scheme was defined in the Aquatic Biodiversity Monitoring Program (PMBA) by the Brazilian environmental and legal agencies.
The fluvial sampling network embraces four sampling sites stretching along 130 km of the lower section of the Doce River. Station E0 is located 12.1 km downstream of the last reservoir in the Doce River channel and 85.2 km upstream of station E21, which is 30.2 km farther upstream from station E22, while station E26 lies 13.3 km downstream. These sampling stations are influenced by a mosaic of land use and land cover (LULC) in the Doce River watershed. The LULC were determined according to Souza et al. (2020) by using Landsat 7 ETM+ cloud-free mosaics processed with a random forest classifier available in Google Earth Engine for LULC classification (available in mapbiomas.org), using ArGIS 10.1. The Doce River watershed primarily comprises seminatural systems with 64.95% (56,045 km2) of the drainage area, where pasture is the main use (36,348 km2). Natural system composes 34.19% (29,492.52 km2) with forest formation as the main land cover (25,483.44 km2). Forest cover is highly fragmented, with 308,861 patches with a mean area of 0.08 ± 5.25 km2. Urban-industrial systems encompass 0.86% (742.48 km2) with 2,111 urban spots, with an average size 0.26 ± 1,62 km2. In addition, the estimated population density according to Comintê da Bacia Hidrogréfica do Rio Doce (CBH-DOCE) (2010) is 3.4 million inhabitants throughout the watershed, of which about 0.5 million are in the areas closest to the sampling stations. Half of this population have poor and incomplete sewage treatment systems (Comintê da Bacia Hidrogréfica do Rio Doce (CBH-DOCE), 2010; ANA - Agência Nacional de Águas e Saneamento Básico, 2019).
The continental shelf stations were defined based on previous studies that identify terrigenous deposits close to the Doce River mouth (Bastos et al., 2015; Quaresma et al., 2020). The continental shelf was aggregated here into three sectors with five sampling stations each: North Marine Sector (MN), Central Marine Sector (MC), and South Marine Sector (MS). Both sets of sampling sites (river channel and adjacent continental shelf) have been affected by tailing deposition after the Fundão Mine dam rupture.
2.2 Data collection and analysis
The monthly field sampling began in October 2018 and lasted until September 2021. However, the coronavirus pandemic generated a data gap from April 2020 to November 2020 and March 2021. The sampling will be treated here by acronyms on a chronological scale, from S1 to S27, where S means field sampling with an increasing number referring to each month (Table 1). In total, fifteen sampling months correspond to the wet season and twelve to the dry season.
Horizontal and vertical distributions of chemicals were carried out for river channel (surface) and continental shelf (surface and bottom) waters. River channel samples were collected by a reversible Niskin bottle (2.8 L) that was used in a horizontal position in a subsurface layer (15 cm) due to the turbulence effect of the deep river current. Seawater samples were collected at the surface (50 cm below the waterline) and at the bottom (approximately 1.0 m above the seafloor) using a 10 L Van Dorn sampler (Aratu®, Brazil). The water depths regarding sampling sites were around 2.5 m for the river channel (depending on river flow) and from 10 to 45 m on the continental shelf. For total N and P measurements in the river channel and continental shelf, water samples were collected unfiltered into acid-washed 125 ml LDPE bottles (Nalgon®). Dissolved nutrients and iron were filtered on board through 0.45 µm pore size filters (Millipore cellulose acetate membrane filters; 47 mm in diameter, ®Merck KGaA, Germany) and stored in LDPE bottles. The water aliquots for macronutrient analyses were kept frozen. Samples for iron analyses were acidified with Suprapur 65% (®Merck) until pH< 2.0 and held at< 4°C until the laboratory chemical quantifications.
2.2.1 Macronutrients analysis
Total and dissolved macronutrients were analysed by colorimetry on a Segmented Flow Analysis - Continuous Flow Analysis (SEAL AutoAnalyzer3 High Resolution - AA3HR). The analytical circuit for the automatic determination consists of individual channels for each nutrient where each manifold has its own set up of reagent injection for proper time reaction. All dissolved nutrients were obtained according to the method of Grasshoff et al. (1999). Nitrite and nitrate were determined by diazotization at 543 nm λmax, with reduction in a Cd-Cu column (recovery > 95%). The phosphate (as ) was determined by the phosphomolybdic method (810 nm λmax); the ammoniacal nitrogen ( and , here designed as Amon-N) was determined by the blue-green coloured complex formed by salicylate and measured at 600 nm λmax; and silicate (as H3SiO4) was determined by the silicomolybdic method at 885 nm λmax. The detection limits were 0.005 μM for nitrite, 0.002 μM for nitrate and ammonia, 0.003 μM for soluble silicates, and 0.008 µM for ortho-phosphate. Detection limits were calculated using the quotient of the standard deviation of 10 blanks by calibration curve inclination and multiplied by 3.
The total nitrogen (TN) and phosphorus (TP) were analysed after digestion to acidic conditions by a strong oxidant, the Persulfate of Potassium, where organic nitrogen compounds are oxidized to nitrate and organic phosphorus to orthophosphate (Valderrama, 1981). For oxidation to occur, an alkaline intermediate (sodium hydroxide, Sigma-Aldrich®) is required for the nitrogen components and an acidic (boric acid, Sigma-Aldrich®) for the phosphoric components. Digestion was carried out at 120-130°C in an autoclave for 2 hours. After digestion, the colorimetric method described above determined the phosphorus and nitrogen. N and P readings were performed in the AA3HR autoanalyzer.
Dissolved inorganic nitrogen (DIN) was considered as the sum of dissolved nitrogenous compounds such as Amon-N, , and . concentrations correspond to dissolved inorganic phosphorus (DIP) and silicate to dissolved inorganic silicon (DSi). The organic nitrogen (Norg) and organic phosphorus (Porg) were calculated by the difference between the total N and P and its dissolved inorganic fraction, considering that potassium persulfate digestion converts the dissolved and particulate organic N and P. Percentages were calculated for each fraction (organic and inorganic) within TP, TN, DIP, and DIN.
2.2.2 DFe analysis
For dissolved iron (DFe< 0.45 µm cut-off size fraction) analyses, the Fe was pre-concentrated by passing the sample through a cationic resin column (Chelex® 100 sodium form; ©Merck KGaA, Darmstadt, Hesse, Germany). For this procedure, seawater sample aliquots were neutralized to a final pH of around 7.0 using 1 M NaOH. The DFe were eluted from the resin with Suprapur® 10% solution and quantified by Inductively Coupled Plasma – Mass Spectroscopy (7500cx ICP-MS, Agilent Technologies), according to EPA method 6020A (U.S. EPA, 1998). The method’s accuracy was checked by analysing fortified samples (spiked with 10 µg L-1), showing a mean recovery of 95% and a detection limit of 0.012 µg/L for DFe.
2.2.3 Dissolved carbon analysis
Dissolved Organic Carbon (DOC) content was obtained from filtered (Whatman GF© 0.47 µm) water samples through direct injection in the Total Organic Carbon Analyzer (TOC-L/CPN-SHIMADZU). The total dissolved carbon of the samples was detected as carbon dioxide in a non-dispersive infrared (NDIR) detector after high-temperature catalytic oxidation (HTCO). Dissolved inorganic carbon was detected after acidification with phosphoric acid and estimated from the difference between the two measurements.
2.2.4 Isotopic analysis
For the analysis of isotopes in the suspended particulate matter (SPM), 2 L of water was filtered onboard through 0.45 µm borosilicate membranes. Filters containing recovered SPM for isotope analysis were immediately frozen at -20°C. These filters were cut into 9 mm diameter circles and decarbonated in a steaming HCl atmosphere inside a desiccator for 16 hours. The samples remained at 60°C in a drying oven for 8 hours, and the filters containing about 5 mg of particulate material were encapsulated in tin capsules for subsequent injection into the EA-EMRI. The determinations of δ13C of total organic carbon (TOC) and δ15N of total nitrogen (N) were performed using a Flash EA elemental analyser coupled to the Delta V Plus isotopic ratio mass spectrometer from Thermo. Two pulses of reference gas (N2) with an intensity of 7000 mV were introduced at the beginning of each run, and two pulses of reference gas (CO2) with an intensity of 14000 mV at the end of the run. The reference gases were previously calibrated from the average value obtained after ten determinations of the IAEA USGS40 certified standard. The results of δ15N and δ13C are expressed in parts per thousand (‰), calculated according to the value of the second pulse of their respective reference gas. Values are reported as the average of two injections. The detection and quantification limits of the δ13C and δ15N analyses are related to the amplitude of the analyte’s signal, estimated at ≥ 500 mV (DL). To ensure the analytical quality of the results, the IAEA USGS40 certified standard was injected every six injections (or three samples), with a deviation of less than ± 0.5 ‰ being accepted. Due to analytical limitations, isotopic analysis was performed only in the first twelve campaigns in the river channel.
2.2.5 Chlorophyll a and phytoplankton analysis
Chlorophyll a (Chl-a in µg.L-1) was determined after sample filtration (250 – 1 L) on fiberglass filters (47 mm with a nominal pore size of 0.7 µm; Whatman GF/F). Pigment extraction was made with 90% acetone overnight; chlorophyll a and phaeopigments were determined by fluorometry (Turner Designs TD700, with 460 and 670 nm for excitation and emission, respectively) before and after acidification (HCl) (American Public Health Association - APHA, 2005). Phytoplankton abundance was determined using Uthermöl sedimentation chambers, and individuals were counted under an inverted microscope (Utermöhl, 1958). It counted at least 100 individuals of the most abundant taxa, ensuring the confidence intervals of ± 20% in a significance level of 95% (Lund et al., 1958). Total phytoplankton density was considered the sum of each taxon’s abundance, and diatom density was the sum of the taxon belonging to Bacillariophyta.
2.3 Statistical analysis and data treatment
A descriptive approach of the data from TN, TP, DIN, DIP, DSi, Porg, Norg, and DFe was conducted for river surface and marine surface and bottom waters. The ratios of DIN : DIP and DIN : DSi were also statistically evaluated in the dry and wet seasons. The Redundancy Analysis (RDA) was carried out separately for river channel and continental shelf samples (grouping surface - S and bottom - B samples), and for the wet and dry seasons to test the response of the biotic variables (chlorophyll-a [Chl-a], total phytoplankton density [Total density], diatom density [Diatom]) to abiotic variables (DIN, DIP, DSi, Porg, Norg and DFe, dissolved carbon [DOC], particulate suspended material [SPM]). These analyses were performed using R (version 3.1.3; R Core Team, 2020) and the “vegan” package (Oksanen et al., 2020). We also separately conducted a spatial analysis of TP, DIP, TN, NO2-, NO3-, Amon-N, DSi, and DFe between river stations and marine sectors (Kruskal-Wallis and Dunn’s post hoc test, p< 0.05). A temporal analysis was performed using the Mann-Witney test (p< 0.05) to evaluate the statistical difference between these macro and micronutrients in the wet and dry seasons.
Environmental limitation regarding dissolved iron levels (DFe) was evaluated from the Fe tracer (Fe*), which corresponds to the biological demand of DFe needed for orthophosphate assimilation by the phytoplankton (Parekh et al., 2005; Zhu et al., 2017). Equation 1 includes the ratio of 7.5 mmol DFe: 1 mol DIP (Bristow et al., 2017) for determining DFe bioavailability or limitation as:
where,
[DFe] is the concentration of dissolved iron in mmol.L-1;
RFe,P corresponds to the ratio of DFe bioutilization for orthophosphate assimilation (7.5 mmol);
[DIP] is the concentration of dissolved inorganic orthophosphate in mmol.L-1.
Positive values of Fe* indicate that DFe is bioavailable in the environment, while negative values show DFe is limited for biological demand.
3 Results
3.1 Land and ocean nutrients concentration
According to the concentrations of nutrients in the surface waters, TP was approximately five-fold higher in the river channel (1.71 ± 2.14 μmol/L) than on the continental shelf (0.38 ± 0.25 μmol/L) in the wet season, with no significant differences on the continental shelf surface and bottom waters during both dry (0.5 ± 0.3 μmol/L vs. 0.56 ± 0.47 μmol/L) and wet (0.38 ± 0.25 μmol/L vs. 0.41 ± 0.24 μmol/L) seasons (Mann-Witney, p > 0.05). (Table 2). TP was slightly more concentrated in the river when the flow was lower. In the surface water, the DIP levels in both regions did not show significant differences in the dry and wet seasons and were about two-fold higher in the river channel than on the continental shelf. Within the continental shelf, there were no statistically significant differences (Mann-Witney, p > 0.05) between the surface and bottom waters.
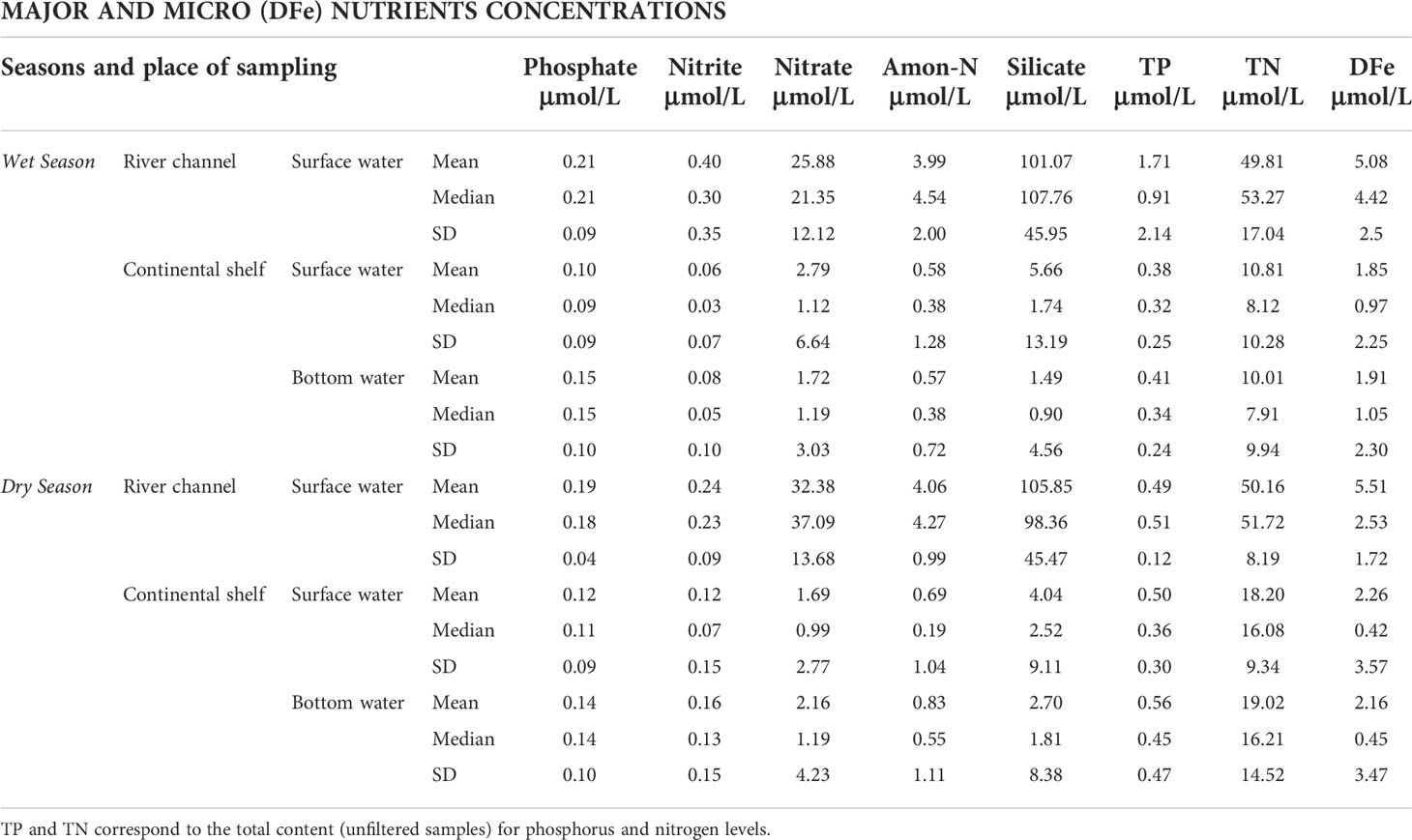
Table 2 Dissolved major (phosphate, nitrite, nitrate, ammoniacal nitrogen – Amon-N - and silicate) and micronutrient (dissolved iron - DFe) mean, median and standard deviation (SD) values in the Doce river channel and the continental shelf adjacent to the Doce River mouth.
TN mean concentrations in the surface waters were approximately five-fold and three-folds higher in the river channel than on the continental shelf during wet and dry seasons, respectively (Table 2). No significant difference was observed within the continental channel between the surface and bottom waters (Mann-Witney, p > 0.05). An approximately seven-fold or two-fold higher surface water content was recorded in the river channel compared to the continental shelf during dry and wet seasons, respectively, with no statistically significant difference between surface and bottom waters during dry and wet seasons on the continental shelf (Mann-Witney, p > 0.05). was about 9 and 19 times more concentrated in the river surface waters than the continental shelf in wet and dry seasons, respectively, with a higher river mean in the dry season (32.38 ± 13.68 μmol/L). On the continental shelf, content varied between surface and bottom; it was more concentrated at the surface when the flow was higher (2.79 ± 6.64 μmol/L, wet season) and at the bottom during the dry periods (1.69 ± 2.77 μmol/L) (Mann-Whitney, p< 0.05). Amon-N river channel surface water content was about 7 (wet season) and 6 times higher (dry season) compared to the continental shelf, with no significant differences between the seasons in both continental shelf waters.
DSi was 18 folds (wet season) and 26 folds (dry season) more concentrated in the river channel than on the continental shelf (Table 2). The mean concentration was similar between the dry and wet seasons in the river channel. On the continental shelf, DSi concentrations were higher in marine surface waters (5.66 ± 13.19 μmol/L, wet season) in both wet and dry periods and were almost 2-folds more concentrated in bottom waters in the dry season than in the wet season. DFe surface water concentration was about 2.7-fold higher in the wet season and 2.4-fold higher in the dry season between the river channel and continental shelf. There was no significant difference between surface and bottom waters in the continental shelf.
Spatio-temporal analysis was performed between river channel sampling stations and continental shelf sectors (Table 2; Supplementary Material) and between dry and wet seasons (Table 3; Supplementary Material) for TP, DIP, TN, , , Amon-N, DSi, and DFe for all 27 field sampling periods. There was a statistical spatial difference for DIP, and Amon-N between upstream station E0 in relation to the other stations of the lower Doce River channel (Kruskal-Wallis, p< 0.05). Medians were significantly higher at E0 (DIP = 0.26 μmol/L, = 0.42 μmol/L and Amon-N = 5.56 μmol/L). The DSi median was significantly higher at the E26 station, located 1.2 km closer to the river mouth (DSi = 112.7 μmol/L) than other fluvial stations. On the continental shelf, the median of DIP and were significantly higher in the southern sector (DIP = 0.15 μmol/L; = 1.25 μmol/L). The median DFe was significantly higher in the central sector (DFe = 0.87 μmol/L) compared to the northern and southern sectors on the continental shelf. The other nutrients (e.g. TP and TN) did not vary spatially within the sampling stations and sectors.
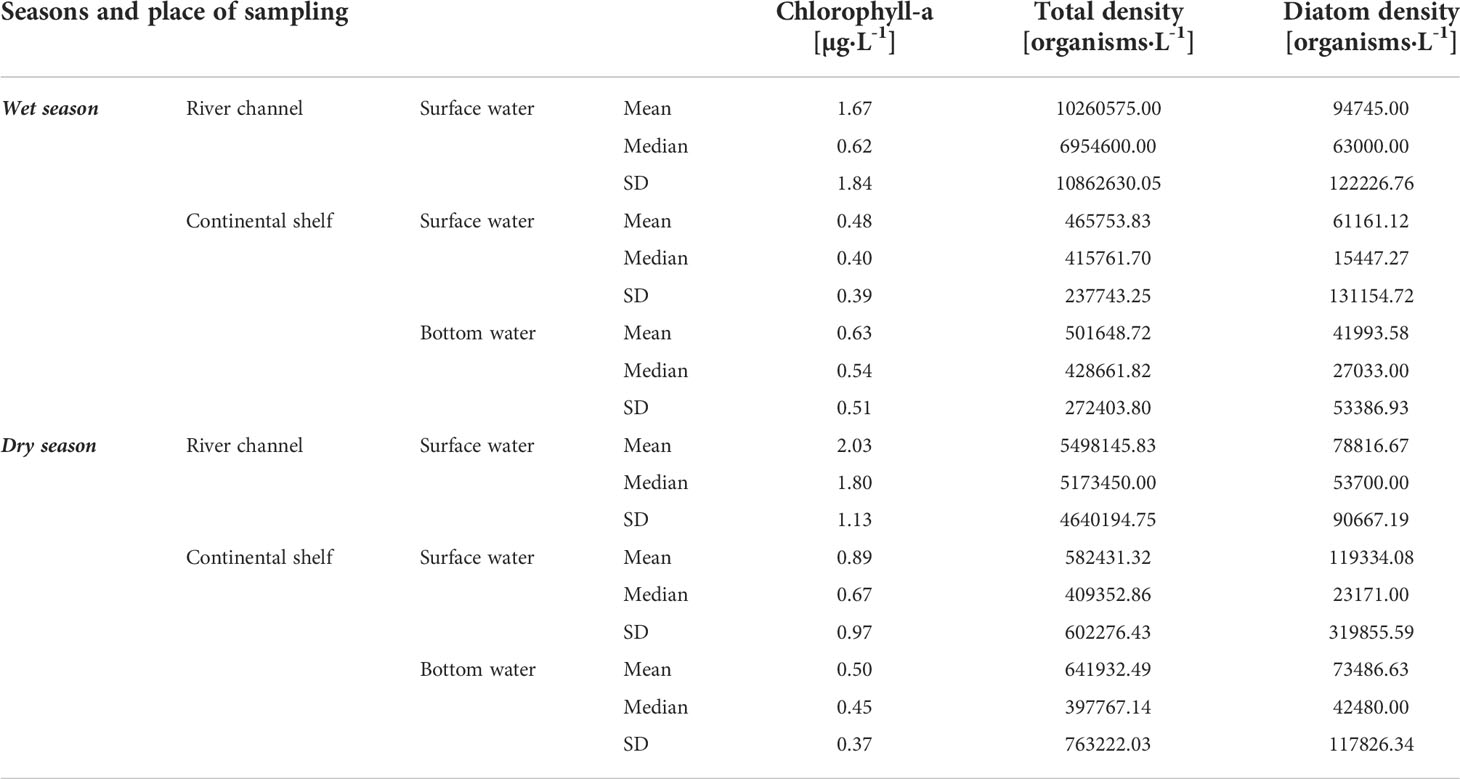
Table 3 Chlorophyll-a, Total phytoplankton density and total diatom density mean, median and standard deviation (SD) values in the Doce River channel and the continental shelf adjacent to the Doce River mouth.
The temporal analysis (Table 3; Supplementary Material) showed a significant difference in the river channel for TP and DFe, with higher medians in the wet months (0.91 μmol/L for TP and 4.42 μmol/L for DFe; Mann-Whitney, p< 0.05); and for with higher medians in the dry season (37.09 μmol/L, Mann-Whitney, p< 0.05). On the continental shelf, the medians were significantly higher (Mann- Whitney, p< 0.05) in the dry season for TP (0.44 μmol/L, TN (16.15 μmol/L), (0.1 μmol/L) Amon-N (0.5 μmol/L) and DSi (2.2 μmol/L). The DFe presented a higher median in the rainy season on the continental shelf (1.01 μmol/L; Mann-Whitney, p< 0.05).
3.2 δ13C and δ15N isotopic composition
Variation of stable isotope values of carbon (δ13C) and nitrogen (δ15N) along all riverine samplings was from -30 to -22 ‰ and from 1 to 16 ‰, respectively (Figure 2). The isotopic data are available just for the twelve first sampling in the river channel. During this year (12 sampling periods, from Oct/2018 to Sep/2019), the organic matter source was variable and corresponded to sewage, C3 plants, and plankton sources. Sewage source occurs more often during the wet season in the river channel.
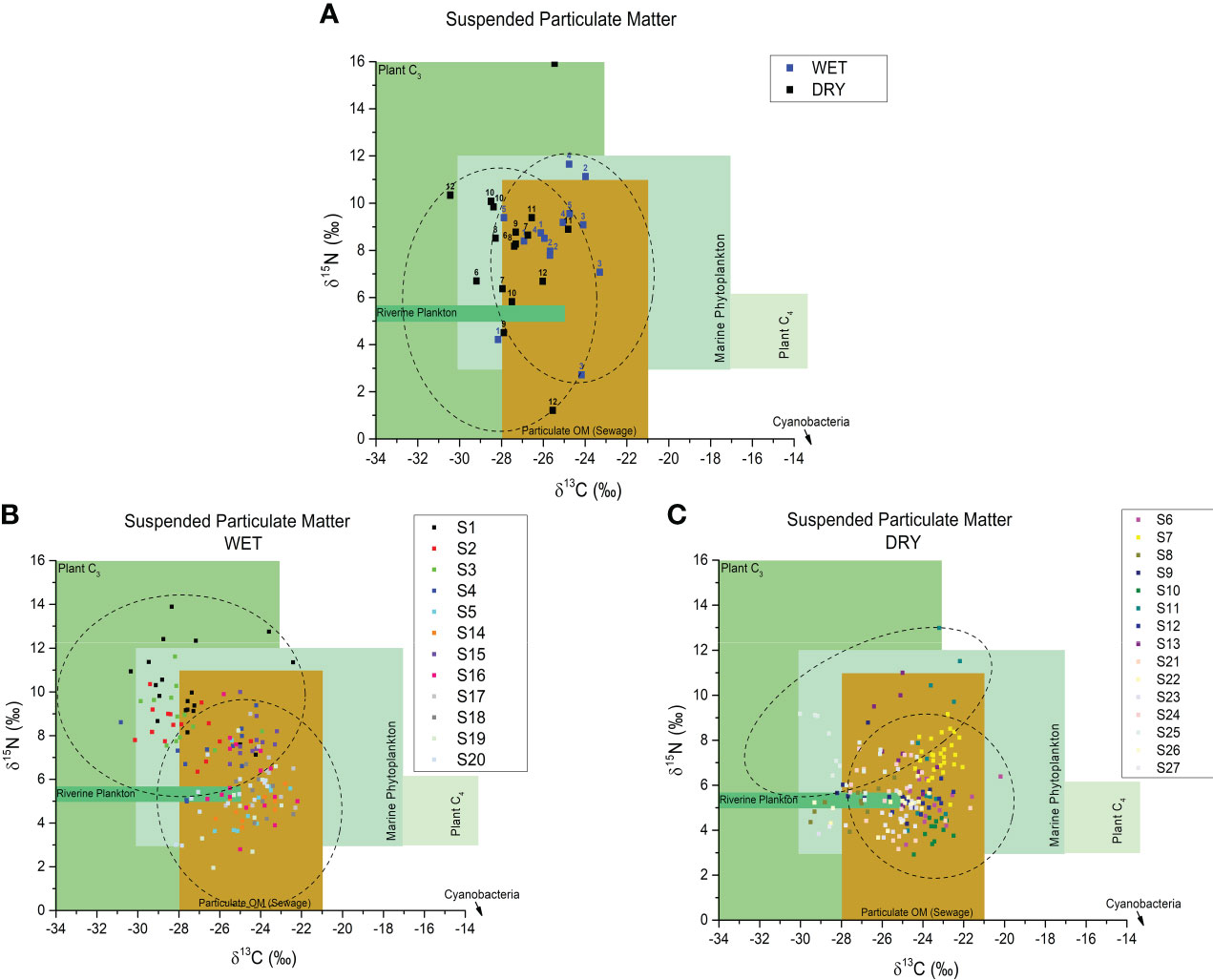
Figure 2 Distribution of isotopic composition of suspended particulate matter (SPM) relative to δ13C and δ15N and organic matter sources according to Maksymowska et al. (2000) classification in the river channel (A) and on the continental shelf (B, C) in both dry and wet seasons.
For the marine sampling during 27 periods, the variation of stable isotope values of carbon (δ13C) and nitrogen (δ15N) was from -31.4 to -20.2 ‰ and from 1.9 to 13.9 ‰, respectively. When comparing the δ13C and δ15N values of this study (Figure 2) with those found in the literature (Maksymowska et al., 2000), it is possible to observe a mixture of sources for this particulate organic material, with terrestrial/fluvial plankton and marine planktonic sources as the main contributors. It is also possible to notice that during the wet period (high flows), there was a more significant variation in the values of these isotopes among the studied sampling periods.
3.3 Chlorophyll-a, total phytoplankton density, and total diatom density
In order to synthesize the results of the biological variables, the averages of chlorophyll-a, total density of phytoplankton (as Total density), and total density of diatoms (as Diatom) were used (Table 3). All biotic data have higher averages in the river channel than on the continental shelf, except for the diatom density, which is about 1.5 higher in continental shelf surface water during the dry season. About 1.5 times more diatoms were recorded in the river channel than the continental shelf surface water during the wet season, while within the continental shelf, surface waters values were 1.5 (wet) and 1.6 (dry) higher than in the bottom waters. Chlorophyll-a, which represents phytoplanktonic biomass, is about 3.5 (wet) and 2.3 (dry)-fold higher in the river channel than on the continental shelf. The total density of phytoplankton is about 20-fold higher in the river compared to the continental shelf in the wet season and 9-fold higher in the river in the dry season. Chlorophyll-a is relatively more concentrated in the bottom marine water than on the surface in the wet season, with the opposite occurring in the dry season.
3.4 Nitrogen and phosphorus fractionation
The total organic content of P (Porg) and N (Norg) and dissolved inorganic fraction (DIP and DIN) and their representativity for TP and TN, respectively, are emphasized in the present study (Table 4). Although the particulate inorganic fractions of phosphorus (PIP) have a significant contribution in the total content, especially from river sources, this fraction was not analysed in this study because it cannot be assimilated by plankton (Cotner and Biddanda, 2002) and remains primarily inert in the sediment (Ruttenberg and Berner, 1993; Delaney, 1998). It should be noted that the values given in % do not represent the gross concentration of nutrients in the two bodies of water, which means that the % may be higher even if the concentration is lower from one environment to another.
The average percentage of DIP to TP ranged from 27.7% to 42.3%, higher in the river channel in the dry season and the bottom continental shelf waters in both wet and dry periods (Table 4). On the other hand, considering the proportionality of Porg in the TP content, the organic fraction was predominant in both environments, ranging between 57.7 to 72.3% of the total phosphorus. Regarding nitrogen content, the river channel was more enriched in DIN than the sea, representing 74.5% to 85.6% of TN (Table 4). On the continental shelf, the concentration of DIN was significantly lower and is about 2-fold higher in the wet season (approximately 32%) than the dry season (approximately 16.2%). Among the inorganic nitrogen species, nitrate is the most representative, corresponding to 83% of the DIN in the river channel and 64% in the marine region. The Norg is lower in the river than in the sea, being higher in the dry season (river = 25.5%; sea = approx. 83%).
3.5 DIP, DIN, and DSi ratios and limitation
Figure 3 shows the dispersion diagram of the Redfield ratio of all sampled waters. The river channel and continental shelf stations were grouped, respectively, and the information was cross-referenced between the DIN : DSi and DIN : DIP ratios for the dry and wet seasons and each seawater layer. Thus, the predominance of phosphate limitation in the Doce River channel was observed for both seasons. For a few samples, silica is also limited in the river. On the continental shelf, there was a limitation in all macronutrients with a relative predominance of limitation in DIN and DIP in surface samples and DSi in bottom samples. DSi was relatively less limiting in surface wet season samples than in dry periods. The distribution of ratios on the continental shelf also showed several samples with values close to the stoichiometric proportion proposed by Redfield.
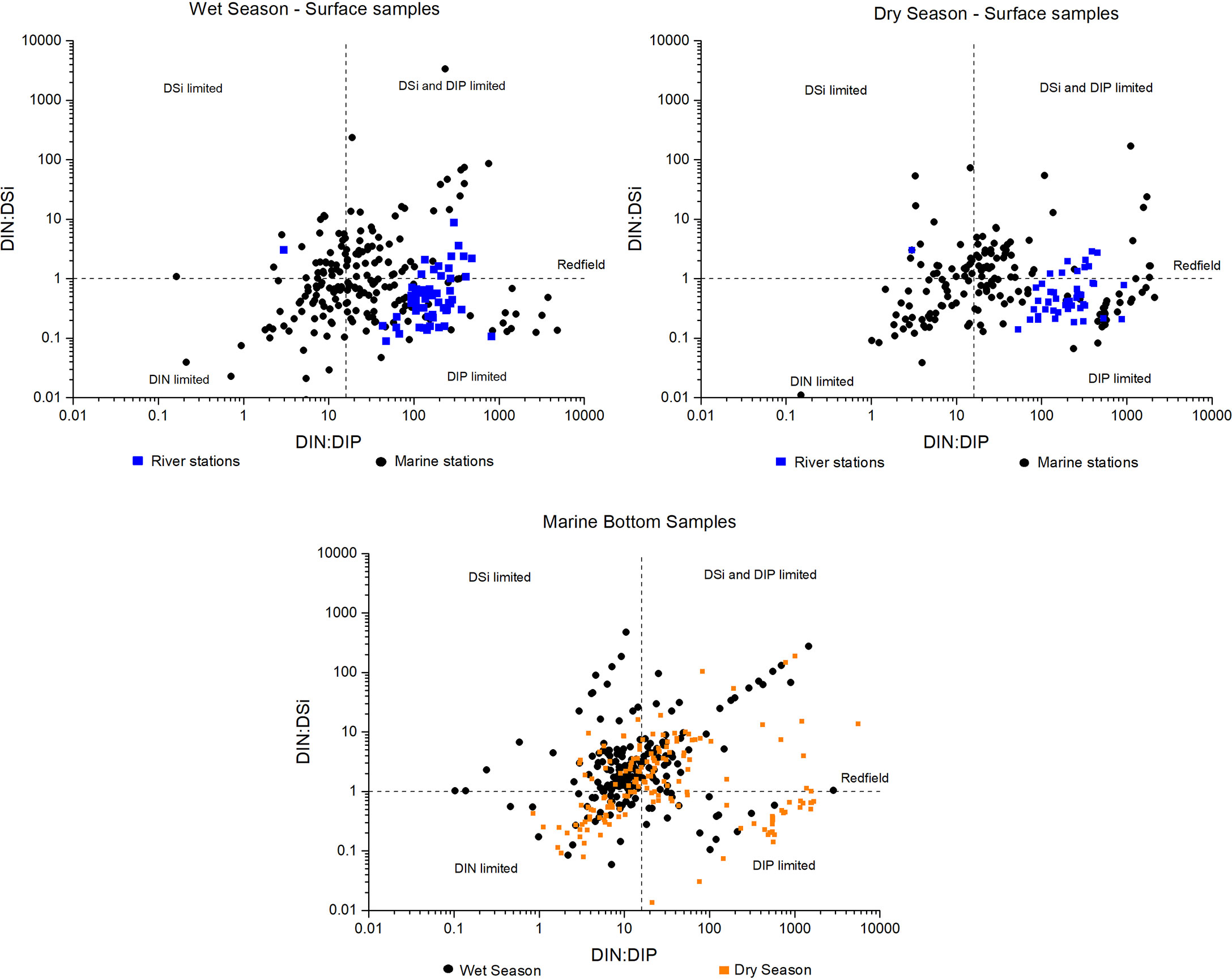
Figure 3 Inorganic macronutrients relationship between DIN, DIP and DSi during wet (high river flows) and dry (low river flows) seasons. The blue squares are all the samples from the Doce River channel along the 4 stations and the black dots and orange squares are all continental shelf surface and bottom water samples, respectively, for the 27 samplings in both environments. The upper left quadrant corresponds to DSi-limited samples, the upper-right quadrant to DSi and DIP-limited samples, the lower-left quadrant corresponds to DIN-limited samples, and the lower-right quadrant to DIP-limited samples.
3.6 Fe limitation
Fe* tracer presented mostly positive values (bioavailable) in the Doce River channel during the wet season (high river flows), whereas limited conditions (negative values of Fe*) were observed in 44% of samples during the dry season (low river flows; Jun/2019 and Jun/2021; Figure 4). On the continental shelf, negative conditions of Fe* were observed in 42% of samples during the wet season and in 51% of samples in the dry season (Table 1; Figure 4). The higher frequency of Fe* bioavailability is during the wet seasons for both river channel and continental shelf, especially for higher river flow conditions (e.g., Jan/2019 and Mar/2020; Figure 4). However, high Fe* bioavailability was not always flow-dependent and positive values of Fe* was remarkable on the continental shelf during the dry season from Apr/2019 to Jun/2019 (Figure 4).
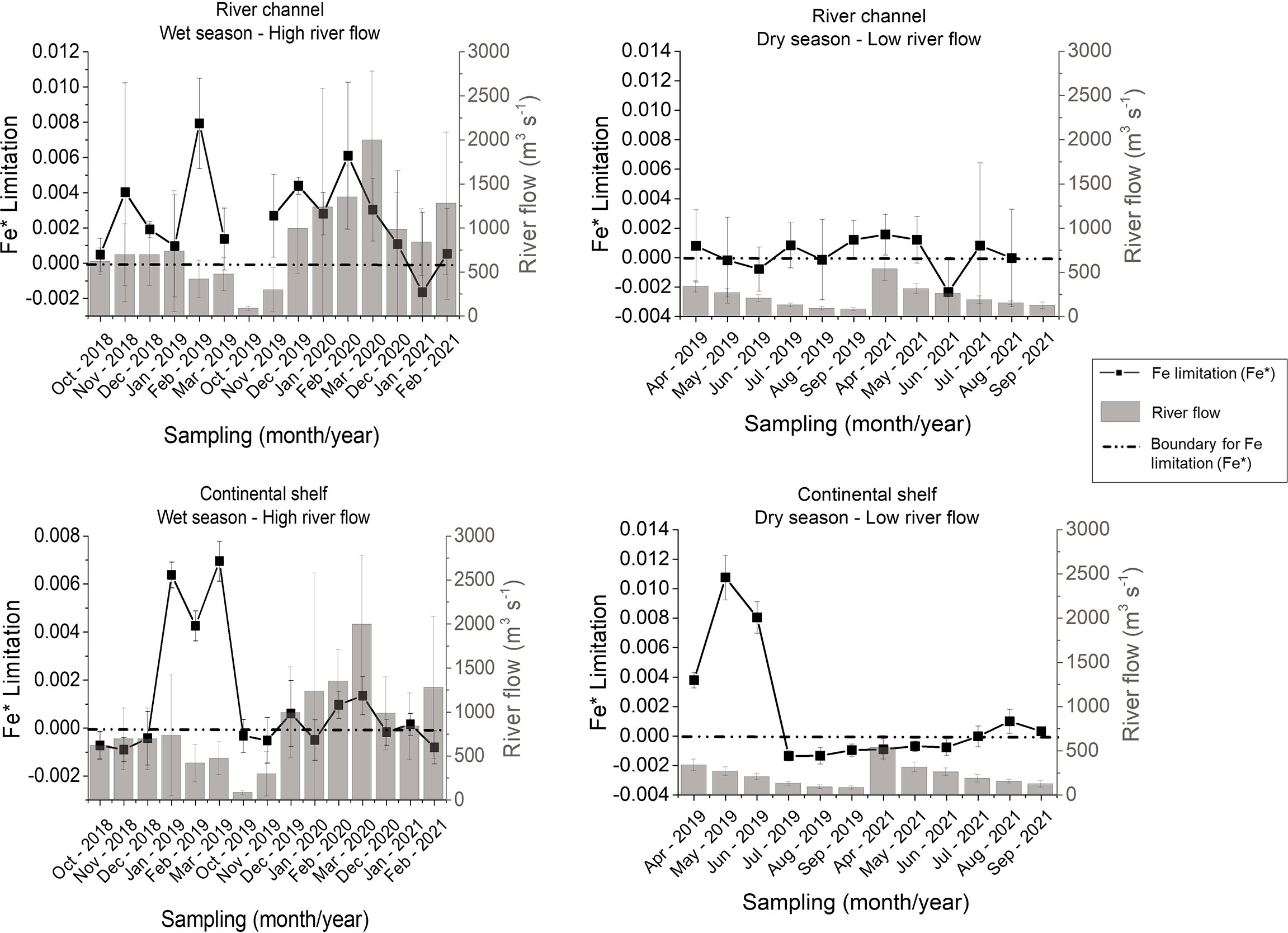
Figure 4 Plots of Fe* (P) in the Doce River channel and on the adjacent continental shelf during wet (high river flows) and dry seasons (low river flows). The black squares represent the Fe* limitation. The grey histogram represents the river flow in m3/s. Above the zero reference line (dotted line) DFe is available and below is limited.
3.7 Redundancy analysis
The Redundancy Analysis (RDA) was applied to evaluate the relationship between abiotic factors, such as Porg, DIP, Norg, , , ammonia nitrogen (Amon-N), DSi, suspended particulate matter (SPM), dissolved organic carbon (DOC) and dissolved iron (DFe); versus biotic factors such as chlorophyll a (Chl-a), total phytoplankton density (Total density) and diatom density (Diatom). The RDA was performed separately for the Doce River channel and the continental shelf for dry and wet seasons. The RDA for both the continental shelf and riverine data sets showed a significant effect of abiotic variables in phytoplankton density and biomass. The correlations between biotic and abiotic variables were higher in the riverine system (r > 0.6, Table 1; Supplementary Material), especially in the dry season. This pattern is similar to the continental shelf, which has lower correlation values between biotic and abiotic variables (r > 0.3, Table 1; supplementary material). In the Doce River, lower values of dissolved nutrients were related to higher values of chlorophyll-a and phytoplankton density during the dry season (Figure 5A). On the continental shelf, during the dry season (Figure 5C), there was no trend towards a relationship between phytoplankton total density and nutrients (dissolved and/or particulate). The chlorophyll-a varies according to the lower nutrient concentration, and the diatom density is related to the SPM in surface and bottom samples (Figure 5E). On the other hand, in the wet season (Figure 5D and 6F), nutrient changes seem to be related to the increase in chlorophyll-a and total density but were not associated with the increase in diatom density.
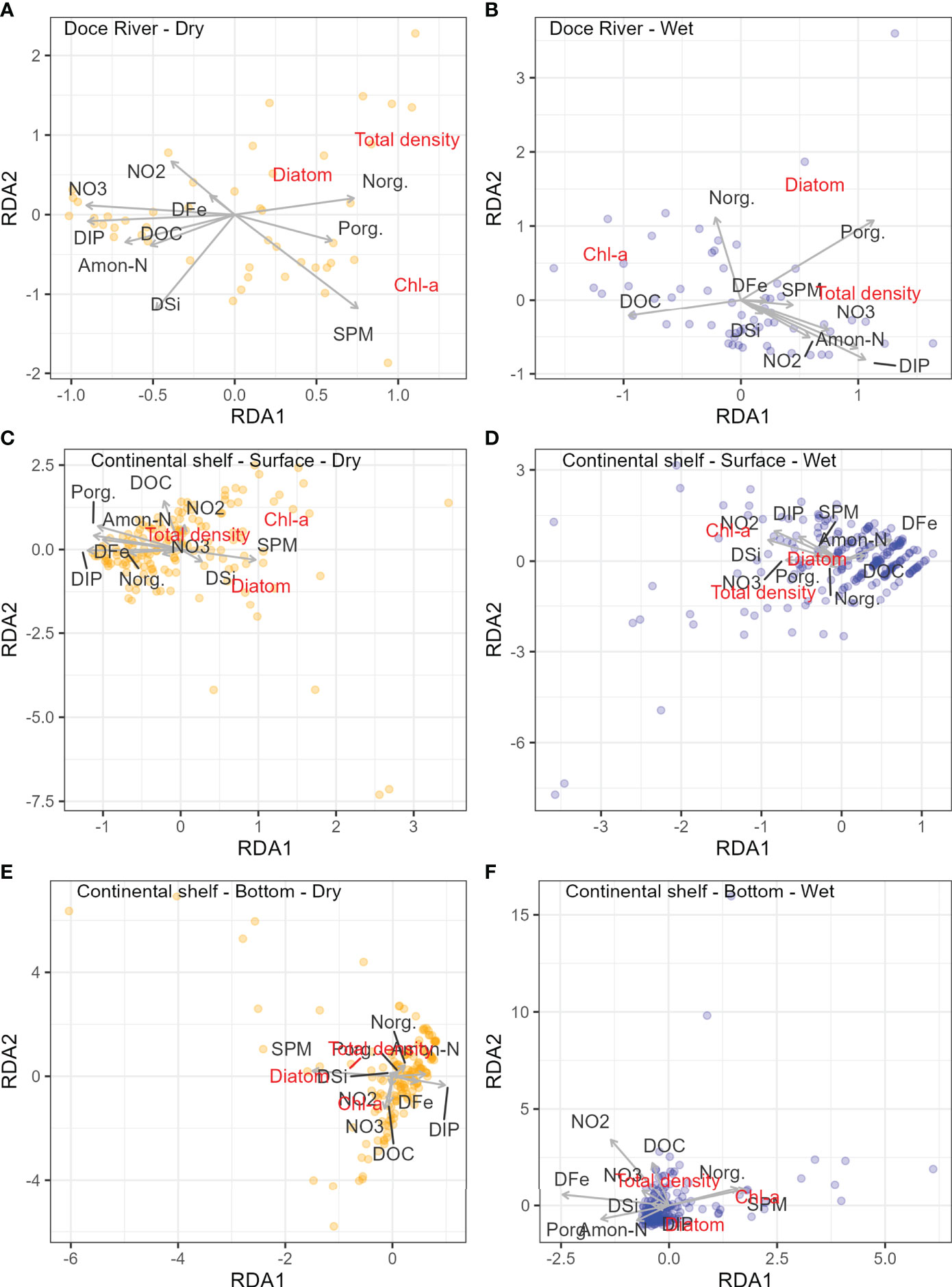
Figure 5 Redundancy analysis (RDA) of dissolved inorganic phosphate (DIP), total organic phosphorus (Porg), nitrite ( ), nitrate ( ), ammoniacal nitrogen (Amon-N), organic nitrogen (Norg), dissolved silicate (DSi), suspended particulate matter (SPM), dissolved organic carbon (COD), as explanatory variables, and chlorophyll a (Chl-a), diatom density (Diatom) and total phytoplankton density (Total density), as the response variable, for the river channel (A: Dry season; B: Wet season) and continental shelf (C: Surface – Dry season; D: Surface – Wet Season; E: Bottom – Dry Season; F: Bottom – Wet Season).
4 Discussion
4.1 Land and ocean nutrients distribution
Rivers are a significant pathway in the global geochemical cycles of elements, but little consideration has been given to the geographic distribution of their loads and contents due to scientists’ focus on global scales. The geographic origin of river load results from the combined influences of lithology, relief, climate, and freshwater; estuarine ecosystems are critical zones of macro and micro nutrient processing along the land-sea continuum (Meybeck, 1994). Expanding human activities increasingly impacts nutrient inputs and limitations for primary production. In addition to the common polluting sources that reach the Doce River watershed, the collapse of the Fundão dam - recognized as the most prominent Brazilian environmental disaster - transformed the hydrogeochemical and biological features of the river watershed and its adjacent continental shelf. Therefore, the results of this research contribute to understanding this chronic impact on the input and distribution of macronutrients and dissolved iron throughout these hydrological systems.
The Doce River channel presented, on average, higher dissolved nutrient concentrations than the continental shelf, both in dry and wet seasons, highlighting the influence of this system on chemical elements transported from the land to the sea (Table 2). Total phosphorus values were often found above the limits allowed by Brazilian legislation in the Doce River watershed (3.12 μmol/L, Brasil et al., 2005) from sampling periods S14 to S17 (Table 1, high river flow), which is attributed to the dumping of wastewater from wastewater treatment plants (WTPs) and to the incomplete sewage treatment systems (Comintê da Bacia Hidrogréfica do Rio Doce (CBH-DOCE), 2010; ANA - Agência Nacional de Águas e Saneamento Básico, 2019). The TP can reach 100 μmol/L in these WTPs (Barroso et al., 1997). The contribution of sewage to the organic nutrient content is confirmed by isotopic analysis in this study (Figure 2), which reflects in the continental shelf. In 2013, only 10% of the domestic and industrial wastewater in the Doce River watershed was treated (IGAM - Instituto Mineiro de Gestão Das Águas, 2010).
The DIP did not vary between seasons on the river and the continental shelf but was relatively higher in bottom marine waters than at the surface, which could be explained by its release from the bio/mobilized sediment, by the remineralization of particulate organic phosphorus (POP) coming from the surface or by the lower assimilation of this nutrient in the deep (Cotner and Biddanda, 2002). It is recognized that the ore tailings altered the long-term phosphorus supply on the estuary and continental shelf due to its adsorption onto iron oxides from tailings and desorption with the increase of salinity (Costa et al., 2021; Queiroz et al., 2021). Although the tailings are not a significant source of phosphorus, the iron oxides carry this nutrient along the watershed and the continental shelf. According to the environmental reports available on the FLACSO website (RRDM, 2021b), the DIP occurs around 25% to 35% above reference values from before the dam collapse (Figueiredo et al., 2016) in the coastal zone reached by tailings, highlighting the influence of this impact on the balance of P between land and ocean.
Nitrogen species were more concentrated in the river than on the continental shelf, and their dynamics varied (Table 2). The data available from previous studies have shown the occurrence of 33% to 55% for , 20% for and 40% to 50% for Amon-N above the values before the ore tailings spill (Figueiredo et al., 2016; RRDM 2021b). However, in this case, the nitrogenous species might be associated with using amine-based flocculants in the dam (Costa et al., 2021) and the Fe enrichment (Table 2 and Figure 4) that enhances primary productivity. DFe is required for vital metabolic processes, such as photosynthesis, respiration, and nitrogen uptake/assimilation (Morel et al., 2003). The continental runoff, weathering, and tailing remobilization influence the riverine availability during wet seasons. Some authors also report a long-term enrichment of in this coastal zone after the dam collapse, as for phosphates (Costa et al., 2021). It seems that the nitrogen oxidized species are controlled by the surficial sedimentary plume from the river, by the uptake and recycling processes, and by the meteoceanographic dispersion (winds and waves) on the continental shelf. The higher Amon-N concentration in the continental shelf in the dry season could induce marine regeneration production with low river flow (Azam et al., 1983).
DSi is more remarkably concentrated in the river than on the continental shelf and higher in surface marine waters than at the bottom. Although this nutrient can come from several other geologic sources along the river watershed (Conley, 2002; Humborg et al., 2006), it works as an indirect tracer of tailings in the coastal zone as it is a major part of its constitution Grilo et al., 2018; (Orlando et al., 2020). In the acute phase of the impact, when the dam failed in 2015, there was a peak concentration of DSi (175.47 μmol/L) and (50.69 μmol/L) in the study coastal region (Costa et al., 2021). Over six years after the dam rupture, DSi still occurs at around 40% to 65% above pre-disaster reference values (RRDM 2021b, Figueiredo et al., 2016; Costa et al., 2021). The DSi continental shelf concentrations were lower than the river, possibly due to plankton uptake and DSi dispersion by meteorological factors. The data in Table 2 indicated that DSi is distributed on the continental shelf by the particulate surface dispersion in the wet season, and it is made available again by the remobilization of the seabed by the waves in the dry season, similarly which has been observed for metal(oid)s in the continental shelf adjacent to the Doce river mouth after the disaster (Longhini et al., 2022). Although the DSi indicate surface dispersion of the river input on the continental shelf, salinity is not significantly affected by freshwater, being always > 30 in shelf waters (Fernandes et al., 2020; Conceicao et al., 2021; Bonecker et al., 2022).The enrichment of DSi in the river channel highlights the influence of the continental nutrient input on the continental shelf. The DSi is crucial for maintaining diatom density and trophic chain balance in aquatic systems (Garnier et al., 2010).
The DFe enrichment at the river and the adjacent continental shelf occurs by the ore tailings Fe oxides. Therefore, this micronutrient has been dumped in the coastal region since the Fundão dam collapsed. In the acute phase of the impact, iron reached concentrations of 35.2 mg Fe/L (or approximately 57 μmol Fe/L) in the estuarine water (Sá et al., 2021). This enrichment can support the primary productivity in the river and sea, enhancing biological N fixing (Berman-Frank et al., 2007).
Regarding the spatial analysis, station E0, further upstream in the lower Rio Doce, had higher DIP, and Amon-N concentrations (Kruskal-Wallis, p< 0.05; Table 2, Supplementary Material). The E0 station is located downstream of the Mascarenhas Hydroelectric Plant, in the municipality of Baixo Guandu, where a large amount of the tailings is retained and is progressively released at times of natural flooding and floodgate openings, affecting the availability of nutrients (RRDM 2021a). The DSi and concentrations were higher at E26, the river station closest to the sea, and this might occur due to the river-ocean interactions and accumulation of fine sediments in the estuary, corresponding to a two-way route in the supply of nutrients between the river and the inner shelf (Table 2; Supplementary Material). Concerning the continental shelf sectors, the increase in the medians of DIP and to the south sector (MS) indicates the recycling of these nutrients (DIP) or the input and transport of elements from the river (such as ) to the south by the predominance of N, NE and E winds in the region (Cavalcanti et al., 2009). The DFe was more concentrated in the central marine sector (MC) due to its location right in front of the river mouth (Kruskal-Wallis, p< 0.05, Table 2, Supplementary Material). The increase of DFe in the Doce River mouth (E26 and MC) points out not only a natural river input of this micronutrient but also a continuous release and remobilization of sediments enriched in iron ore tailings accumulated along the Doce River drainage watershed and the inner continental shelf (Hatje et al., 2017).
The results of the temporal variability of the nutrients highlight the co-occurrence of P and Fe, which were potentiated by the leaching and weathering of the river banks and the continental region of the Fundão dam in rainy periods. The data suggest nutrient recycling during dry periods, especially denitrification and remineralization processes on the continental shelf. The isotopic analysis showed that during the dry period (low flows), relative values of δ13C and δ15N indicating autochthonous sources of organic matter (phytoplankton), possibly due to the decrease in the terrestrial/riverine input with the lower river flow, consequently predominating the regenerate primary production. The DFe presented a higher median in the rainy season on the continental shelf (1.01 μmol/L; Mann-Whitney, p< 0.05), which is possibly related to the land drainage, even though other processes had influenced the DFe availability, as will be discussed in the “DFe Limitation” section. The increase in DSi concentration in the dry period in both environments is particularly interesting. The silicate dynamics indicate the occurrence of fine sediment highly vulnerable to bottom remobilization by currents and waves, making DSi available in the water column in dry months when these meteoceanographic factors act more intensely (Quaresma et al., 2015; Longhini et al., 2022). Due to the greater energy of the river, the tendency is to transport and deposit the fine material on the inner shelf (Quaresma et al., 2020). The ore tailings are composed of very fine and colloidal particles of sediment that are partially stationed on the adjacent continental shelf (Grilo et al., 2018) since a significant layer of tailings was deposited in this environment. This condition reinforces the hypothesis that the fine suspended particulate matter (SPM) generated by hydrodynamics at the river mouth promotes DSi enrichment from the ocean into the river and vice versa, as seen spatially at E26 station.
4.2 Nitrogen and phosphorus fractionation
Phosphorus and nitrogen are essential constituents of organisms, forming the basis of DNA and RNA, cell membranes and energy, amino acids, etc. The organic and inorganic fractions of P and N undergo a continuous transformation in the aquatic system (Paytan and McLaughlin, 2007; Garnier et al., 2010). Once available for plankton assimilation, the fractionation of nutrients is mediated by bacteria through the synthesis/remineralization of the organic matter (Li and Peng, 2002; Brea et al., 2004). This analysis is addressed here in a generic (% average) and descriptive way for both environments (Table 4).
The increase in the superficial organic load of P by the river in the wet season (approx. 70%) is remarkable. This organic load might come from sewage and other biological sources, such as C3 plants and phytoplankton (Figure 2). Porg is also more concentrated on the continental shelf in the dry period and originated mainly from a phytoplankton source (Figure 2). DIP is usually less concentrated in waters because it is rapidly assimilated by phytoplankton and is then converted to organic phosphorus (Paytan and McLaughlin, 2007). The zooplankton ingests the phytoplankton and excretes dissolved inorganic and organic phosphorus (Anderson and Zeutschel, 1970), while phytoplankton cell lysis also makes DIP and Porg available again to the water column (Azam et al., 1983). These biogeochemical cycles are also constantly influenced by bacteria that convert Porg into DIP enabling primary producers´ uptake (Paytan and McLaughlin, 2007).
The DIN increase during dry and wet periods in the river channel was particularly interesting. The values ranged from 74.5 to 85.6%, leaving only a tiny Norg fraction (Table 4). The greater riverine availability of DFe could partly explain this observation, required in high concentrations by nitrogen-fixing species, enhancing this process (Berman-Frank et al., 2007), in agreement with the higher phytoplankton density in the river channel in both dry and wet seasons (Table 3). On the continental shelf, the concentration of DIN is significantly lower and is about 2-fold higher in the wet season (approximately 32%) than the dry season (about 16.5%). This might indicate greater nutrient recycling through assimilation and conversion from DIN to Norg on the continental shelf in the dry season, which is confirmed by the higher phytoplankton biomass (given by chlorophyll a, Table 3) in the dry season. Among the inorganic fraction, is the most representative, corresponding to approximately 82% of the DIN in the river channel and 64% on the continental shelf. This condition is favourable for new primary production (Garnier et al., 2010) and suggests a high rate of nitrification processes. There is no difference between the concentration of DIN and Norg in the marine water layers. This pattern is observed in some of the data suggesting a homogeneity in the fractionation and mixing of nutrients in the water column on the continental shelf.
4.3 Nutrients limitation
Phytoplanktonic stoichiometric ratios can vary on a regional scale of C:N:P through growth rates, taxonomy composition, environmental concentrations of CO2 (g), and availability of nutrients (Arrigo, 2005). The N:P ratios reflect an overall balance in the ocean between the phytoplankton production of particulate organic matter, remineralization, denitrification, and N2 fixation (Takahashi et al., 1985). Silicic acid is also essential in this balance due to diatoms’ requirement for constructing siliceous valves (Epstein, 1999). Along with , these organisms demand approximately a 1:1 Si and N molar ratio (Dugdale et al., 1995; Wu and Who, 2003; Carey et al., 2019).
The vast majority of samples from the Doce River channel are limited in phosphorus in this study. Although a significant anthropogenic source of this nutrient has been seen (e.g., sewage, Figure 2), the simultaneous enrichment of iron can act as a phosphorus scavenger in the lower Doce River due to Fe-P bonding, making it unavailable for assimilation. In addition, the imbalance between DIN and PIN, where nitrogen is more concentrated, causes phosphorus limitation in this environment.
Understanding coupled biogeochemical processing with Si, N, and P has direct implications for freshwater and coastal ecosystems, as the amount of Si to N and P exported by rivers to coastal receiving waters can determine phytoplankton species assemblage and food web structure (Carey et al., 2019). It has been demonstrated for coastal regions that iron limitation can cause diatoms to increase the DIN : DSi uptake ratio, depleting the water of Si, and leading to secondary Si limitation. DFe is sometimes limited (see DFe limitation in Figure 3). Clearly, Si is very important and can potentially influence the N response of the primary producers. Silica limitation in the continental shelf’s bottom waters can occur either because this nutrient was more depleted in this layer or because there was greater consumption by diatoms. Once depleted, diatoms cannot develop and primary production is compromised, giving rise to the proliferation of non-siliceous and potentially toxic microalgae (Cugier et al., 2005). DSi was relatively less limiting in surface wet season samples due to higher river discharge.
The close similarity between nitrogen and phosphorus ratios in plankton and deep-water nutrients proposed by Redfield suggested that life in the ocean adjusts the nutrients according to its requirements. The opposite is assumed, that life has adapted to the oceanic ratios (Voss et al., 2013). The consensus is that nitrogen is limited on short time scales, whereas on geological time scales, phosphorus is the limiting nutrient (Falkowski, 1997, Tyrrell, 2001). Human activities have impacted the mass balance of these nutrients in the environment, as occurred after the Fundão Dam disaster (Costa et al., 2021). Certain nutrients can be limited by the depletion in the supply or by the excess of one over the other, generating an imbalance. Due to the numerous hydrogeochemical processes on the continental shelf, this equilibrium is not always reached, where DIN, DIP, and DSi are limited at different times (Figure 3).
Fe* tracer presented mostly positive values in the Doce River channel during the wet season (high river flows), which indicates high DFe availability for biological productivity; whereas limited conditions for DFe were observed in 44% of samples during the dry season (low river flows; Jun/2019 and Jun/2021; Figure 4). In the continental shelf, adverse conditions for Fe* tracer were observed in 42% of samples during the wet season and in 51% of samples in the dry season (Figure 4); which means that DFe might be a potential limiting factor for primary production during those samplings. Once Fe* tracer is calculated from its stoichiometric ratio against orthophosphate, this limitation can occur either by low DFe levels or its imbalance against DIP concentrations (Zhu et al., 2017). Assuming the threshold of DIP assimilation by the phytoplankton of 0.2 µmol/L (Fisher et al., 1992), DFe concentration needed to sustain the primary production should be higher than 1.5 µmol/L (Zhu et al., 2017). Therefore, DFe concentrations found in the Doce river channel (5.51 ± 1.72 µmol/L in the dry season; 5.08 ± 2.50 µmol/L in the wet season) and on the continental shelf (2.26 ± 3.57 µmol/L in the dry season; 1.85 ± 2.25 µmol/L in the wet season) were high enough and did not explain the DFe limitation by Fe* tracer, suggesting that DIP might be more limiting for primary productivity production than DFe. Although different sources and biogeochemical processes govern the orthophosphate, the iron oxides present in the ore tailings work as carriers of P, adsorbing this nutrient along the watershed and transporting it to the lower Doce River and the continental shelf (Queiroz et al., 2021), where they can be dissociated by the increase in salinity (Sundareshwar and Morris, 1999). Despite this Fe-P, the supply of phosphates is not always guaranteed in the amount required by phytoplankton, making this nutrient often limiting in the river and continental shelf (Figure 3).
The higher frequency of DFe bioavailability in the wet seasons for both river channel and continental shelf can indicate the influence of continental loads and riverine discharge for DFe inputs, especially during flooding conditions (e.g., Jan/2019 and Mar/2020; Figure 4). However, high DFe bioavailability was not always flow-dependent; positive values of Fe* was remarkable on the continental shelf during the dry season (Figure 4). These results indicate that other meteoceanographic processes are acting to increase DFe concentrations on the continental shelf, such as the resuspension of fine particles from the seafloor and sediment-water column exchanges by waves action (Longhini et al., 2022), which tends to be intensified in the dry months (Quaresma et al., 2020). It is also important to mention that the DFe content changed significantly after the iron ore tailings reached the coastal waters, with lower settlement rates (Grilo et al., 2018) and high content of soluble and stable inorganic forms (Longhini et al., 2020).
4.4 Redundancy analysis - biotic and abiotic interactions
Redundancy Analysis (RDA) is an extension of multiple regression and can be used to evaluate the effects of a set of explanatory variables on a set of response variables (Legendre and Legendre, 2012). The correlations between biotic and abiotic variables were higher in the river channel system (Table 1, Supplementary Material), especially in the dry season. This pattern is similar on the continental shelf, but with lower values of correlation between biotic and abiotic variables, suggesting that other factors (e.g., response time between uptake and remineralization) are also important drives to the phytoplankton density and biomass on the continental shelf. All differences suggest that the effects of nutrients on phytoplankton change over time.
In the Doce River, lower values of dissolved nutrients were related to higher values of chlorophyll-a and phytoplankton density during the dry season (Figure 5A). These results suggest a depletion of the dissolved nutrient fraction incorporated into biomass of the larger species, such as diatoms (Table 3), which stock nitrogen and phosphorus in more complex organic molecules (with all these variables located on the right side of the first axis in RDA). During the wet season, higher phytoplankton densities were related to the dissolved fraction of nutrients. However, chlorophyll-a was plotted on the opposite side of the total density, considering the first axis (Figure 5B). These results suggest a dominance of small taxa with rapid absorption and cycling of nutrients (Liao et al., 2019), which results in high abundances without high biomass.
On the continental shelf, during the dry season (Figure 5C), there was no trend towards a relationship between phytoplankton total density and nutrients (dissolved and/or particulate), indicating that other factors could be related to the variation in community abundance. The variation in chlorophyll-a, related to lower nutrient concentrations, may also be related to the higher depth of the mixing zone in this period (Lemos et al., 2018), allowing taxa, such as diatoms, to take advantage of nutrients available from deeper zones (Table 2). Relationships between diatom density and SPM in surface and bottom samples (Figure 5E) may be indicative of surface-bottom interactions. On the other hand, in the wet season (Figure 5D, 6F ), nutrient changes seem to be related to the increase in chlorophyll-a and total density but not associated with the rise in diatom density.
5 Conclusion
Human activities along with the Doce River watershed to the marine continuum impact nutrient inputs and can limit primary production in the coastal zone. The iron ore dumping in the Doce River watershed and its adjacent continental shelf reflected a chronic increase in nutrients that lasted for six years since the Fundão dam rupture. We noticed an increase in dissolved iron and silica, which are the main constituents of the tailings. Indirectly, a long-term enrichment of phosphorus from sewage and other sources occurs due to its adsorption and transport by iron oxides. This Fe-P coupling may also explain the phosphates limitation in the river watershed, making it unavailable to the biota. In the same way, nitrogen enrichment can be indirectly enhanced by the greater fixation stimulated by the iron and directly by the use of amine-based flocculants in the dam.
In terms of processes, N fixation/remineralization predominates in the river channel, while on the continental shelf, the hydrodynamics provide a variable environment, with a more significant influence on the river nutrient supply in the wet season and higher nutrient re-availability from sediment resuspension in the dry season, which can promote, respectively, new and regenerated primary production. The nutrient ratios show that the complex marine environment confers both limitations on DIN, DIP, and DSi, as well as a stoichiometric equilibrium sometimes. Iron is not significantly limited, most of the time available to the biota. The nutrients spatial distribution highlights that, although the river is more concentrated in nutrients and is the primary source of them to the continental shelf, physical interactions (e.g., low flow and tide) can lead to the supply from the inner continental shelf into the estuary.
In the Doce River channel, lower values of dissolved nutrients were related to higher values of chlorophyll-a and phytoplankton density during the dry season, suggesting a depletion of the dissolved nutrient and incorporation into biomass (e.g., diatoms). The chlorophyll-a was opposite the total density in the wet season, suggesting a dominance of small taxa with rapid uptake and cycling of nutrients in the river channel. On the continental shelf, the variation in chlorophyll-a, related to lower nutrient concentrations, may be related to the higher depth of the mixing zone in this period, allowing taxa (e.g., diatoms), to take advantage of nutrients available from deeper zones. In the wet season, nutrient changes seem to be related to the increase in chlorophyll-a and total density but not associated with the rise in diatom density on the continental shelf.
The results of this work show the importance of investigating and reporting local data for understanding global land-ocean nutrient fluxes and their potential influence on primary productivity and trophic chain balance. We suggest that specific studies focus on the phytoplankton community should be conducted for accurate conclusions on the impact of iron ore on primary productivity.
Data availability statement
The datasets presented in this study can be found in online repositories. The names of the repository/repositories and accession number(s) can be found below: https://flacso.org.br/projetos/gerenciador-cif-camaras-tecnicas.
Author contributions
RC: Participated in conceptualization, sampling procedures, data curation, formal analysis, methodology, writing - original draft, writing - review & editing. CL: Participated in conceptualization, data curation, formal analysis, methodology, writing - original draft, writing - review & editing. EC, CS, DM, VP, PB, AD-B, BG, IB, AL and FS: Participated in data curation, writing - review & editing. SZ-A: Participated in data curation, writing - original draft, writing - review & editing. RN and GB: Participated in conceptualization, data curation, writing - review & editing. All authors contributed to the article and approved the submitted version.
Funding
We thank the Renova Foundation and the Rede Rio Doce Mar (Brazil) for funding this study as part of the Biological Diversity Monitoring Program (PMBA) (Technical-Scientific Agreement, DOU number 30/2018, between FEST - Fundação Espírito-Santense de Tecnologia and Renova Foundation).
Acknowledgments
We are grateful to Aroldo Rocha for constructing the study area map and to the researchers and the crew for supporting sample collection during cruises onboard the Deep Sea ship (Dracares Apoio Marítimo e Portuário).
Conflict of interest
The authors declare that the research was conducted in the absence of any commercial or financial relationships that could be construed as a potential conflict of interest.
Publisher’s note
All claims expressed in this article are solely those of the authors and do not necessarily represent those of their affiliated organizations, or those of the publisher, the editors and the reviewers. Any product that may be evaluated in this article, or claim that may be made by its manufacturer, is not guaranteed or endorsed by the publisher.
Supplementary material
The Supplementary Material for this article can be found online at: https://www.frontiersin.org/articles/10.3389/fmars.2022.990809/full#supplementary-material
References
Albino J., Contti Neto N., Oliveira T. C. A. (2016). “The beaches of espírito Santo,” in Brazilian Beach systems. Eds. Short A. D., Klein A. H. F. (Coastal Research Library: Springer), 333–361.
Albino J., Suguio K. (2010). Sedimentation processes and beach morphodynamics active at the doce river mouth, espírito Santo state, Brazil. An. Acad. Bras. Ciênc. 82, 1031–1044. doi: 10.1590/S0001-37652010000400023
American Public Health Association - APHA (2005). Standard methods for the examination of water and wastewater (Washington, DC: American public health association).
ANA - Agência Nacional de Águas e Saneamento Básico (2015) Encarte especial sobre a bacia do Rio doce: rompimento da barragem de mariana. conjuntura dos recursos hídricos no brasil. Available at: https://www.snirh.gov.br/portal/centrais-de-conteudos/conjuntura-dos-recursos-hidricos/encarteriodoce_22_03_2016v2.pdf (Accessed June 27, 2022).
ANA - Agência Nacional de Águas e Saneamento Básico (2019) Atlas de esgoto: Despoluição das bacias hidrográficas. Available at: http://atlasesgotos.ana.gov.br/ (Accessed June 03, 2022).
Anderson G. C., Zeutschel R. P. (1970). Release of dissolved organic matter by marine phytoplankton in coastal and offshore areas of the northeast pacific ocean. Limnol. Oceanogr. 15 (3), 402–407. doi: 10.4319/lo.1970.15.3.0402
Arrigo K. R. (2005). Marine microorganisms and global nutrient cycles. Nature 437, 349–355. doi: 10.1038/nature04159
Azam F., Fenchel T., Field J. G., Gray J. S., Meyer-Reil L. A., Thingstad F. (1983). The ecological role of water column microbes in the sea. Mar. Ecol. Prog. Ser. 10, 257–263. doi: 10.3354/meps010257
Barroso G. F., Dias Júnior C., Güntzel A. (1997). Preliminary assessment eutrophication potential of sewage effluents of four wastewater treatment plant's in espírito Santo state (Brazil). Verh. Internat. Verein. Limnol. 26, 666–670.
Bastos A. C., Quaresma V. S., Marangoni M. B., D'Agostini D. P., Bourguignon S. N., Cetto P. H., et al. (2015). Shelf morphology as an indicator of sedimentary regimes: a synthesis from a mixed siliciclastic and carbonate shelf on the eastern Brazilian margin. J. S. Am. Earth Sci. 63, 125–136. doi: 10.1016/j.jsames.2015.07.003
Berman-Frank I., Quigg A., Finkel Z. V., Irwin A. J., Haramaty L. (2007). Nitrogen-fixation strategies and fe requirements in cyanobacteria. Limnol. Oceanogr. 52, 2260–2269. doi: 10.4319/lo.2007.52.5.2260
Bonecker A. C. T., Menezes B. S., Junior C. D., da Silva C. A., Ancona C. M., de Oliveira Dias C., et al. (2022). An integrated study of the plankton community after four years of fundão dam disaster. Sci. Total. Environ. 806, 150613. doi: 10.1016/j.scitotenv.2021.150613
Brasil, Ministério do Meio Ambiente, Resolução CONAMA (2005) Dispõe sobre a classificação dos corpos de água e diretrizes ambientais para o seu enquadramento, bem como estabelece as condições e padrões de lançamento de efluentes, e dá outras providências. Available at: https://www.icmbio.gov.br/cepsul/images/stories/legislacao/Resolucao/2005/res_conama_357_2005_classificacao_corpos_agua_rtfcda_altrd_res_393_2007_397_2008_410_2009_430_2011.pdf (Accessed May 28, 2022).
Brea S., Alvarez-Salgado X. A., Alvarez M., Pérez F. F., Mémery L., Mercier H., et al. (2004). Nutrient mineralization rates and ratios in the eastern south Atlantic. J. Geophys. Res. 109, C05030, 1–15. doi: 10.1029/2003JC002051
Bristow L. A., Mohr W., Ahmerkamp S., Kuypers M. M. (2017). Nutrients that limit growth in the ocean. Curr. Biol. 27 (11), R474–R478. doi: 10.1016/j.cub.2017.03.030
Bundschuh J., Litter M. I., Parvez F., Román-Ross G., Nicolli H. B., Jean J.-S., et al. (2012). One century of arsenic exposure in Latin America: a review of history and occurrence from 14 countries. Sci. Total. Environ. 429, 2–35. doi: 10.1016/j.scitotenv.2011.06.024
Cagnin R. C., Quaresma V. S., Chaillou G., Franco T., Bastos A. C. (2017). Arsenic enrichment in sediment on the eastern continental shelf of Brazil. Sci. Total. Environ. 607, 304–316. doi: 10.1016/j.scitotenv.2017.06.162
Carey J. C., Jankowski K., Julian P., Sethna L. R., Thomas P. K., Rohweder J. (2019). Exploring silica stoichiometry on a large floodplain riverscape. Front. Ecol. Evol. 7. doi: 10.3389/fevo.2019.00346
Cavalcanti I. F., de A., Ferreira N. J., Dias, da S. M. A. F., Silva M. G. A. J. (2009). Tempo e clima no brasil (São Paulo: Oficina de Textos).
Comintê da Bacia Hidrogréfica do Rio Doce (CBH-DOCE) (2010) PIRH doce volume I (Accessed September 28, 2022).
Conceicao L. R., Demoner L. E., Pereira J. B., Perassoli F., Ghisolfi R. D., Bastos A. C., et al. (2021). Copepod community structure after a mining dam disaster in the southwestern Atlantic ocean. Estuar. Coast. Shelf. Sci. 254, 107325. doi: 10.1016/j.ecss.2021.107325
Conley D. J. (2002). Terrestrial ecosystems and the global biogeochemical silica cycle, glob. Biogeochem. Cycles. 16 (4), 1121. doi: 10.1029/2002GB001894
Conley D. J., Kilham S. S., Theriot E. C. (1989). Differences in silica content between marine and freshwater diatoms. Limnol. Oceanogr. 34, 205–213. doi: 10.4319/lo.1989.34.1.0205
Costa E. S., Cagnin R. C., Silva C. A., Longhini C. M., Sá F., Lima A. T., et al. (2021). Iron ore tailings as a source of nutrients to the coastal zone. Mar. pollut. Bull. 171, 112725. doi: 10.1016/j.marpolbul.2021.112725
Cotner J. B., Biddanda B. A. (2002). Small players, large role: microbial influence on biogeochemical processes in pelagic aquatic ecosystems. Ecosystems 5 (2), 105–121. doi: 10.1007/s10021-001-0059-3
Cugier P., Billen G., Guillaud J. F., Garnier J., Ménesguen A. (2005). Modelling the eutrophication of the seine bight (France) under historical, present and future riverine nutrient loading. J. Hydrol. 304, 381–396. doi: 10.1016/j.jhydrol.2004.07.049
Delaney M. L. (1998). Phosphorus accumulation in marine sediments and the oceanic phosphorus cycle. Glob. Biogeochem. Cycles. 12 (4), 563–572. doi: 10.1029/98GB02263
Dugdale R. C., Wilkerson F. P., Minas H. J. (1995). The role of a silicate pump in driving new production. Dee-Sea. Res. I. 42, 697–719. doi: 10.1016/0967-0637(95)00015-X
Epstein E. (1999). Silicon. Annu. Rev. Plant Physiol. Plant Mol. Biol. 50, 641–664. doi: 10.1146/annurev.arplant.50.1.641
Falkowski P. G. (1997). Evolution of the nitrogen cycle and its influence on the biological sequestration of CO2 in the ocean. Nature 387, 272–275. doi: 10.1038/387272a0
Fernandes L. F. L., Paiva T. R. M., Longhini C. M., Pereira J. B., Ghisolfi R. D., Lázaro G. C. S., et al. (2020). Marine zooplankton dynamics after a major mining dam rupture in the doce river, southeastern Brazil: Rapid response to a changing environment. Sci. Total. Environ. 736, 139621. doi: 10.1016/j.scitotenv.2020.139621
Figueiredo J. A., Costa E., Milán E., Barroso G. F., Neto R. R. (2016). Nutrientes limitantes e as relações orgânicas e inorgânicas entre o fósforo, nitrogênio e silício no sistema pelágico da BES e PNBC. Geochim. Brasiliensis. 30 (2), 95–95. doi: 10.21715/GB2358-2812.2016302095
Fisher T. R., Peelel E. R., Ammerman J. W., Harding L. W. Jr. (1992). Nutrient limitation of phytoplankton in Chesapeake bay. Mar. Ecol. Prog. Ser. 82, 51–63. doi: 10.3354/meps082051
Garnier J., Beusen A., Thieu V., Billen G., Bouwman L. (2010). N: P: Si nutrient export ratios and ecological consequences in coastal seas evaluated by the ICEP approach. Glob. Biogeochem. Cycles. 24, 4. doi: 10.1029/2009GB003583
Grilo C. F., Quaresma V., da S., Amorim G. F. L., Bastos A. C. (2018). Changes in flocculation patterns of cohesive sediments after an iron ore mining dam failure. Mar. Geol. 400, 1–11. doi: 10.1016/j.margeo2018.03.004
Hatje V., Pedreira R. M. A., De Rezende C. E., Schettini C. A. F., De Souza G. C., Marin D. C., et al. (2017). The environmental impacts of one of the largest tailing dam failures worldwide. Sci. Rep. 7, 1–13. doi: 10.1038/s41598-017-11143-x
Howarth R. (2000). Clean coastal waters: understanding and reducing the effects of nutrient pollution (Washington D.C: National Academy Press).
Humborg C., Pastuszak M., Aigars J., Siegmund H., Morth C. M., Ittekkot V. (2006). Decreased silica land-sea fluxes through damming in the Baltic Sea catchment: Significance of particle trapping and hydrological alterations. Biogeochemistry 77, 265–281. doi: 10.1007/s10533-005-1533-3
IGAM - Instituto Mineiro de Gestão Das Águas (2010) Plano integrado de recursos hídricos da bacia hidrográfica do Rio doce (PIRH) e planos de ações para as unidades de planejamento e gestão de recursos hídricos no Âmbito da bacia do Rio doce. Available at: https://www.cbhdoce.org.br/pirh-parh-pap/pirh (Accessed June 03, 2022).
Krom M. D., Brenner S., Kress N., Gordon L. I. (1991). Phosphorus limitation of primary productivity in the eastern Mediterranean Sea. Limnol. Oceanogr. 36, 424–432. doi: 10.4319/lo.1991.36.3.0424
Lemos A. T., Ghisolfi R. D., Mazzini P. L. F. (2018). Annual phytoplankton blooming using satellite-derived chlorophyll-a data around the vitória-trindade chain, southeastern Brazil. Deep-Sea. Res. I.: Oceanogr. Res. Pap. 136, 62–71. doi: 10.1016/j.dsr.2018.04.005
Liao T., Yu H., Dai C., Zhao M. (2019). Impact of cell size effect on nutrient-phytoplankton dynamics. Complexity 2019, 1–23. doi: 10.1155/2019/8205696
Li Y.-H., Peng T.-H. (2002). Latitudinal change of remineralization ratios in the oceans and its implication for nutrient cycles, glob. Biogeochem. Cycles. 16, 1130. doi: 10.1029/2001GB001828
Longhini C. M., Rodrigues S. K., Costa E. S., Silva C. A., Cagnin R. C., Gripp M., et al. (2022). Environmental quality assessment in a marine coastal area impacted by mining tailing using a geochemical multi-index and physical approach. Sci. Total. Environ. 803, 149883. doi: 10.1016/j.scitotenv.2021.149883
Lund J. W. G., Kipling C., Le Cren E. D. (1958). The inverted microscope method of estimating algal numbers and the statistical basis of estimations by counting. Hydrobiologia 11, 143–170. doi: 10.1007/BF00007865
Maksymowska D., Richard P., Piekarek-Jankowska H., Riera P. (2000). Chemical and isotopic composition of the organic matter sources in the gulf of gdansk (Southern Baltic Sea). Estuar. Coast. Shelf. Sci. 51, 5. doi: 10.1006/ecss.2000.0701
Marone E., Machado E. C., Lopes R. M., Silva E. T. D. (2005). Land-ocean fluxes in the paranaguá bay estuarine system, southern Brazil. Braz. J. Oceanogr. 53 (3/4), 169–181. doi: 10.1590/S1679-87592005000200007
Meybeck M. (1994). “Origin and variable composition of present day riverborne material,” in Material fluxes on the surface of the earth. Ed. Council N. R. (Washington, D.C: The National Academies Press), 61–73. doi: 10.17226/1992
Morel F. M., Hudson R. J., Price N. M. (1991). Limitation of productivity by trace metals in the sea. Limnol. Oceanogr. 36, 1742–1755. doi: 10.4319/lo.1991.36.8.1742
Oksanen J., Blanchet F. G., Kindt R., Legendre P., Minchin P. R., O’hara R. B., et al. (2020) Package ‘vegan’. r package version 2.5-7. Available at: http://cran.rproject.org/web/packages/vegan/vegan.pdf.
Oliveira K. S. S., da Silva Quaresma V. (2017). Temporal variability in the suspended sediment load and streamflow of the doce river. J. S. Am. Earth Sci. 78, 101–115. doi: 10.1016/j.jsames.2017.06.009
Orlando M. T. D., Tadeu M., Galvão E. S., Sant’Ana A. C., Rangel C. V. G. T., Pinheiro C. G. O., et al. (2020). Tracing iron ore tailings in the marine environment: An investigation of the fundão dam failure. Chemosphere 257, 127184. doi: 10.1016/j.chemosphere.2020.127184
Paerl H. W. (2009). Controlling eutrophication along the freshwater–marine continuum: Dual nutrient (N and p) reductions are essential. Estuar. Coasts. 32, 593–601. doi: 10.1007/S12237-009-9158-8
Parekh P., Follows M. J., Boyle E. A. (2005). Decoupling of iron and phosphate in the global ocean. Glob. Biogeochem. Cycles. 19, 2. doi: 10.1029/2004GB002280
Paytan A., McLaughlin K. (2007). The oceanic phosphorus cycle. Chem. Rev. 107 (2), 563–576. doi: 10.1021/cr0503613
Quaresma V. S., Bastos A., Leite M. D., Costa A., Cagnin R. C., Grilo C., et al. (2020). The effects of a tailing dam failure on the sedimentation of the eastern Brazilian inner shelf. Cont. Shelf. Res. 205, 104172. doi: 10.1016/j.csr.2020.104172
Quaresma V. S., Catabriga G., Bourguignon S. N., Godinho E., Bastos A. C. (2015). Modern sedimentary processes along the doce river adjacent continental shelf Brazilian. J. Geol. 45, 635–644. doi: 10.1590/2317-488920150030274
Queiroz H. M., Ferreira T., Barcellos D., Nóbrega G. N., Antelo J., Otero X. L., et al. (2021). From sinks to sources: The role of fe oxyhydroxide transformations on phosphorus dynamics in estuarine soils. J. Environ. Manage. 278, 111575. doi: 10.1016/j.jenvman.2020.111575
R Core Team (2020). R: A language and environment for statistical computing (Vienna: R Foundation for Statistical Computing). Available at: https://www.R-project.org/.
Rossetti D. F., Polizel S. P., Cohen M. C. L., Pessenda L. C. R. (2015). Late pleistocene-Holocene evolution of the doce river delta, southeastern Brazil: implications for the understanding of wave-influenced deltas. Mar. Geol. 367, 171–190. doi: 10.1016/j.margeo.2015.05.012
RRDM, Rede Rio Doce Mar (2021a). “Relatório anual do PMBA/Fest-RRDM – ambiente marinho. RT-39B, p. 485,” in Programa de monitoramento da biodiversidade aquática (Fundação Espírito-Santense de Tecnologia), 613. Available at: https://flacso.org.br/projetos/gerenciador-cif-camaras-tecnicas/.
RRDM, Rede Rio Doce Mar (2021b). “Relatório anual do PMBA/Fest-RRDM – ambiente marinho. RT-39D, p. 336,” in Programa de monitoramento da biodiversidade aquática (Fundação Espírito-santense de Tecnologia), 1410. Available at: https://flacso.org.br/projetos/gerenciador-cif-camaras-tecnicas/.
Ruttenberg K. C., Berner R. A. (1993). Authigenic apatite formation and burial in sediments from non-upwelling, continental margin environments. Geochim. Cosmochim. Acta 57 (5), 991–1007. doi: 10.1016/0016-7037(93)90035-U
Sá F., Longhini C. M., Costa E. S., da Silva C. A., Cagnin R. C., de Oliveira Gomes L. E., et al (2021). Time-sequence development of metal (loid) s following the 2015 dam failure in the Doce river estuary, Brazil. Sci. Total Environ. 769. doi: 10.1016/j.scitotenv.2020.144532
Santolin C. V. A., Ciminelli V. S. T., Nascentes C. C., Windmoller C. C. (2015). Distribution and environmental impact evaluation of metals in sediments from the doce river watershed, Brazil. Environ. Earth Sci. 74, 1235–1248. doi: 10.1007/s12665-015-4115-2
Segura F. R., Nunes E. A., Paniz F. P., Paulelli A. C. C., Rodrigues G. B., Braga G. U. B., et al. (2016). Potential risks of the residue from samarco's mine dam burst (Bento rodrigues, Brazil). Environ. pollut. 218, 813–825. doi: 10.1016/j.envpol.2016.08.005
Souza C. M., Shimbo J. Z., Rosa M. R., Parente L. L., Alencar A. A., Rudorff B. F. T., et al. (2020). Reconstructing three decades of land use and land cover changes in Brazilian biomes with landsat archive and earth engine. Int. J. Remote Sens. 12, 2735. doi: 10.3390/rs12172735
Sundareshwar P. V., Morris J. T. (1999). Phosphorus sorption characteristics of intertidal marsh sediments along an estuarine salinity gradient. Limnol. Oceanogr. 44 (7), 1693–1701. doi: 10.4319/lo.1999.44.7.1693
Takahashi T., Broecker W., Langer S. (1985). Redfield ratio based on chemical data from isopycnal surfaces. J. Geophys. Res. 90, 6907–6924. doi: 10.1029/JC090iC04p06907
Tyrrell T. (2001). The relative influences of nitrogen and phosphorus on oceanic primary production. Nature 400, 525–531. doi: 10.1038/22941
Utermöhl H. (1958). Zur vervollkommnung der quantitativen phytoplankton-methodik (SIL Communications). Mitteilungen: Internationale Vereinigung für Theoretische und Angewandte Limnologie. 1–38. doi: 10.1080/05384680.1958.11904091
Valderrama J. C. (1981). The simultaneous analysis of total nitrogen and phosphorus in natural waters. Mar. Chem. 10, 109–122. doi: 10.1016/0304-4203(81)90027-X
Voss M., Bange H. W., Dippner J. W., Middelburg J. J., Montoya J. P., Ward B. (2013). The marine nitrogen cycle: recent discoveries, uncertainties and the potential relevance of climate change. Philos. Trans. R. Soc Lond. B. Biol. Sci. 368 (1621), 20130121. doi: 10.1098/rstb.2013.0121
Wu J.-T., Who T. L. (2003). Silicate as the limiting nutrient for phytoplankton in a subtropical eutrophic estuary of Taiwan. Estuar. Coast. Shelf. Sci. 58 (1), 155–162. doi: 10.1016/S0272-7714(03)00070-2
Keywords: nutrients, iron, phytoplankton, ore tailing, river and ocean contamination
Citation: Cagnin RC, Longhini CM, Costa ES, da Silva CA, Zorzal-Almeida S, Mendes D, Pasa VMD, Barbeira PJS, Delazari-Barroso A, Gücker B, Boëchat IG, Lima AT, Neto RR, Sá F and Barroso GF (2022) Macronutrients and dissolved iron in a land-ocean approach: Influences of contamination by ore tailings in Southeastern Brazil. Front. Mar. Sci. 9:990809. doi: 10.3389/fmars.2022.990809
Received: 10 July 2022; Accepted: 20 October 2022;
Published: 10 November 2022.
Edited by:
Rafik Balti, University of Jendouba, TunisiaReviewed by:
Thato Mtshali, Department of Environment, Forestry and Fisheries (DEFF), South AfricaTeruya Maki, Kindai University, Japan
Stephen James Malcolm, Centre for Environment, Fisheries and Aquaculture Science (CEFAS), United Kingdom
Copyright © 2022 Cagnin, Longhini, Costa, da Silva, Zorzal-Almeida, Mendes, Pasa, Barbeira, Delazari-Barroso, Gücker, Boëchat, Lima, Neto, Sá and Barroso. This is an open-access article distributed under the terms of the Creative Commons Attribution License (CC BY). The use, distribution or reproduction in other forums is permitted, provided the original author(s) and the copyright owner(s) are credited and that the original publication in this journal is cited, in accordance with accepted academic practice. No use, distribution or reproduction is permitted which does not comply with these terms.
*Correspondence: Renata Caiado Cagnin, cmVuYXRhY2FpYWRvQGhvdG1haWwuY29t