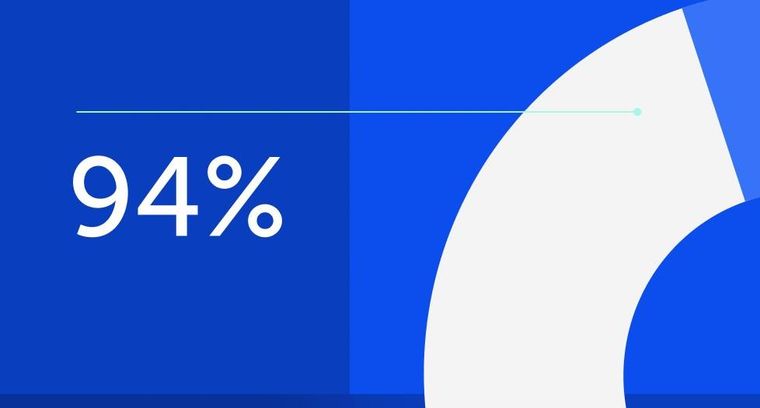
94% of researchers rate our articles as excellent or good
Learn more about the work of our research integrity team to safeguard the quality of each article we publish.
Find out more
DATA REPORT article
Front. Mar. Sci., 16 August 2022
Sec. Marine Pollution
Volume 9 - 2022 | https://doi.org/10.3389/fmars.2022.990351
This article is part of the Research TopicCoastal and Marine Environmental Quality AssessmentsView all 28 articles
Rapid urban and industrial expansion in recent years has significantly impacted coastal ecosystems across the world, including estuaries and bays (Jha et al., 2015; Dheenan et al., 2016). Their watersheds’ high human population density adds to the toxins in the environment. The influence of plastic on coastal ecosystems and the organism has been documented (Janardhanam et al., 2022). Plastic has become the most frequent component of marine pollution and it has been widely spread in the different trophic levels of our ecosystem. Anthropogenic activity generates the majority of the plastics that after aging, weathering, and breaking become the principal sources of microplastics (Cole et al., 2011). Microplastic is often characterized as fibre particles with a size < 5mm and are difficult to get eliminated by a presently practiced sewage treatment protocol because of their tiny size (Hamidian et al., 2021), allowing them to seep into inland rivers and ultimately into marine habitats (Wang et al., 2021). A lot of research on the presence of microplastic in the environment and their consequences on marine species, in terms of physical harm, behavioral changes, tissue lesions, oxidative stress, and gene damage, has been conducted in recent years (Huang et al., 2020; Huang et al., 2021).
Several studies have documented that aquatic ecosystems across the world have been polluted with microplastic (Klein et al., 2015; Zhu et al., 2018; Hariharan et al., 2021; Janardhanam et al., 2022). Microplastics persistent at the water surface for several years, where it is being consumed and accumulated by a variety of species, causing biomagnification in the food chain (Li et al., 2016; Jabeen et al., 2017; Jin et al., 2018; Li et al., 2019). Further, studies have also reported that microplastic in the coastal environment constitutes a significant danger to the food web (Wright et al., 2013, Avio et al., 2015) and in terms of their presence in biological systems. Studies have demonstrated that 9.2 to 92.3% of fish had one or more microplastic within them (Davison and Asch, 2011; Neves et al., 2015; Jabeen et al., 2017).
Microplastics have also been seen in significant quantities around industrial cities along the intertidal zone. In addition, the microplastic also contains a large number of environmental pollutants that adhere to the surface which leads to hazardous effects on organisms (Chen et al., 2017, Guilhermino et al., 2018, Oliveira et al., 2018). It has been well documented that fish that consume microplastic exhibit physical changes as well as decreased development rates, immune defence mechanisms, antioxidant enzyme production, and even reproduction (Sussarellu et al., 2016; Rodriguez-Seijo et al., 2017; Jin et al., 2018; Pannetier et al., 2020). However, the toxicological consequences of weathered polyethylene (wPE) microplastic on aquatic life are difficult to comprehend owing to their size, shape, and manufacturing ingredients (Hariharan et al., 2021).
The glutathione S-transferases (GSTs, EC 2.5.1.18) comprise a supergene family of phase II detoxification enzymes that shield cells against the toxicity of many environmental toxins by enhancing reduced glutathione’s (GSH) nucleophilic assault (Hayes et al., 2005). GSTs have been used as indicators of exposure to environmental contaminants in the majority of aquatic research (Schlenk et al., 2008). Differences in GST activity and expression, whether inherent or induced, may influence aquatic organisms against deleterious effects caused by environmental toxicants since GSTs are crucial for the detoxification of contaminants (Coles and Ketterer, 1990).
To characterize microplastic toxicity and link it to the organismal level of response, the molecular interactions between the microplastic and the biological target must be determined. Hariharan et al. (2021) demonstrated that exposure to weathered polyethylene (wPE) microplastics alters the antioxidant content in the green mussels (Perna viridis). However, to the best of our knowledge, there are no reports on the effect of wPE microplastics on biotransformation enzymes, especially GST in fish. Hence, in this study, we have attempted to understand the effect of wPE microplastics on the liver and gut GST activity in zebrafish.
Three-month-old zebrafish Danio albolineatus, (240 numbers) were obtained from Kolathur fish farm, Tamil Nadu, India. The mean weight and length of fish were ranged between 1.5 - 2.1 g and 3.5- 4.8 cm respectively. The fish were acclimatized to laboratory conditions at a temperature of 27°C ± 1°C, pH 7.2 ± 0.2, and dissolved oxygen content of 5.0 ppm for 7 days prior to the experiment in 100-L glass tanks containing water treated using sand filtration. Fish were kept in a natural daylight cycle and fed with commercial flakes at 1% of body weight daily during the acclimation period.
Preparation of wPE microplastics was done following the methodology of Hariharan et al. (2021). Briefly, fishing ropes that had weathered were gathered from beaches close to Chennai’s shore. Sand and dust particles on the surface layers of the materials were removed by washing them with UV-treated distilled water. The characterization of the worn ropes was done using Fourier Transform Infrared Spectroscopy-FTIR (PerkinElmer Spectrum Version 10.5.4). Characterized wPE debris was frozen at -80°C in Falcon tubes and then manually pulverized into tiny grains using a ball mill-sediment grinder. The wPE microplastic for experiments was then prepared by sieving the fine grains progressively through 32 µm test sieves and such particles were used for the wPE microplastic exposure. The exposure concentrations used for the current study comprised of two different sizes of particles; 1) lesser than 32 µm and 2) between 32 – 34 µm at the relative proportions of 30% and 70%, respectively. With repeated measurement, the dissolved number of wPE particles and the size ratio of the particles to the corresponding concentration per microgram of microplastics were determined. In sterile tubes, the wPE microplastics were carefully mixed with 1 ml milli-Q water to verify the number of particles (free from contaminations). The tubes were stirred briskly to encourage uniform mixing of the particles in the water, and then they were immediately transferred to a Sedgewick-Rafter counting slide and enumerated under a microscope (a Weswox) for counting. To standardize the number of particles in the prepared concentration, the tube was checked for particles that had adhered to their surfaces.
Before microplastic exposure, fish were divided into a batch of 40 fish (20 males and 20 females) and were acclimated in 50 L glass tanks for 1 week. The study consisted of two groups: 1) control and 2) microplastic exposed. For each group three tanks were maintained. The present study used 1 μg L-1 of wPE containing 1204 ± 117 microplastic/m3 and this concentration was used as the sublethal concentration (Hariharan et al., 2021). To prevent the stratification of microplastic particles at the bottom/surface of the experimental chamber, the glass tank was provided with gentle aeration. The exposure lasted for 60 days, and samples were taken every 20 days. Fish behavior was regularly observed, and dead fish, if any, were removed right away from the test chambers. Additionally, utilizing a multi-parameter water quality probe, the physicochemical parameters including water temperature, pH, and dissolved oxygen were recorded twice a day (Hydrolab Quanta Multi-Probe Meter).
At the end of each exposure period, about twelve fish (6 male and 6 female) from each tank were dissected to isolate the internal organs such as the gut and liver for biochemical and mRNA expression study. Three fish were pooled together to ensure the required amount of sample for both enzyme activity and gene expression. Totally 36 fish were used for each time point for the study. The dissected organs were frozen in liquid nitrogen and maintained at −80°C until further analyses. Gut and liver samples were homogenized in ice-cold buffer (0.1 M Tris-HCl, 0.1 mM-EDTA, 0.1% Triton X-100 (v/v), pH 7.8). The homogenates were centrifuged, and the supernatants were collected. The supernatants were used for enzyme and protein analysis. The quantity of protein was measured by the Bradford (1976) method using bovine serum albumin as a standard. GST activity was determined using the 1-Chloro-2,4-dinitrobenzene (CDNB) substrate after conjugating the acceptor substrate with glutathione, as reported by Habig et al. (1974).
Following the manufacturer’s instructions, total RNA was extracted from samples of gut and liver tissues of fish that had been homogenized in TRIzol reagent. A NanoDrop reader was used to evaluate the amount and quality of the RNA samples (NanoDrop Technologies, Synergy). RNA samples with an OD 260/280 nm value between 1.8 and 2.0 were used for the cDNA conversion. Reverse transcription was performed on total RNA (1 μg/μL) in accordance with the manufacturer’s instructions using a high-capacity cDNA reverse transcription kit. Utilizing Light Cycler 480, SYBR Premix Ex Taq TM II, and gene-specific primer pairs, quantitative real-time PCR was carried out using generated cDNAs as templates (Roche). The samples underwent denaturation (10 min at 95°C), amplification (40 cycles for 15 s at 95°C and 1 min at 60°C), quantification using a melting curve program (60 - 99°C) with a temperature rate of 0.1°C/s, and routine monitoring of fluorescence). At 60°C, the predesigned primers for RT-PCR were annealed. Before the primary reaction, all chosen primers were evaluated for efficacy and appropriate concentration. Each PCR reaction was carried out in triplicate, the housekeeping gene beta-actin was employed, and the variations in the abundances of each target gene’s transcripts were represented as their ratio to the expression of the reference gene of Danio rerio are given in the Supplementary Table S1. Based on the 2-ΔΔCT relative responsive methods as described by Livak and Schmittgen (2001) were used to examine the relative fold change in gene expression.
The statistical analysis was carried out using SPSS software (version 20). The data were subjected to normality and homogeneity before a one-way analysis of variance (ANOVA) to test differences between the groups and the exposure periods. Multiple comparison post hoc tests were used to determine the statistical difference between different exposure periods and the p-value of less than 0.05 was considered significant.
The GST mRNA expression patterns in the gut and liver of zebrafish were evaluated using qPCR (Figures 1, 2). As shown in Figure 1(A), an upregulation of GST gene expression in the gut of zebrafish was observed at 40 and 60 d and it was induced up to 10-fold and 9-fold, respectively, in wPE microplastic exposed male zebrafish when compared with the respective control group. Fish exposed to wPE microplastic for 20 d showed an increase in GST gene expression but such an increase was not significantly different from the respective control group (Figure 1A). Similarly female fish exposed to wPE microplastic showed an upregulation of gene expression in the gut and such upregulation was up to 2-, 7- and 5-folds for 20, 40, and 60 d, respectively. All such inductions in GST in the female fishes were statistically significant (p<0.05) (Figure 1B).
Figure 1 Showing the level of glutathione-S-transferase mRNA expression level in fish exposed to weathered polyethylene microplastics (A) Gut Male, (B) Gut Female, (C) Liver Male, (D) Liver Female. The line in each box represent median and whiskers represents the upper and lower 95% confidence intervals of the mean of six determinations using samples from different preparations. One-way analysis of variance (ANOVA) followed by Tukey’s post hoc test was used. The significant (P < 0.05) differences between control and exposure groups were indicated by the alphabets; same alphabet shows no significant difference with respective control group. Different alphabet shows the significant difference with control respective group.
Figure 2 Showing the level of glutathione-S-transferase enzyme level in fish exposed to weathered polyethylene microplastics (A) Gut Male, (B) Gut Female, (C) Liver Male, (D) Liver Female. The line in each box represent median and whiskers represents the upper and lower 95% confidence intervals of the mean of six determinations using samples from different preparations. One-way analysis of variance (ANOVA) followed by Tukey’s post hoc test was used. The significant (P < 0.05) differences between control and exposure groups were indicated by the alphabets; same alphabet shows no significant difference with respective control group. Different alphabet shows the significant difference with control respective group.
Fish exposed to wPE microplastic showed upregulated GST gene expression in the liver of both male and female fish (Figures 1C, D). However female fishes showed the highest GST gene expression when compared to males with respective exposure periods. GST gene expression in male fish was induced by up to 4 and 10-folds after 40 and 60 d of wPE exposure, respectively (Figure 1C). Similarly, the female showed an upregulation of GST expression by up to 6 and 2-folds after 40 and 60 d of wPE exposure, respectively (Figure 1D). In both genders, after 20 days of exposure to wPE the liver GST gene expression was not statistically significant in the respective control group (Figure 1D).
The GST enzyme activity was considerably elevated in the gut and liver of fish exposed to wPE microplastic after respective exposure periods (Figures 2A–D). However, this induction level of the GST enzyme was observed as the exposure period of fish to wPE microplastic increased. The enzyme activity was higher in females exposed to wPE microplastics than in males. In both sexes, the liver has the highest GST activity of both the organs tested. In both male and female fish, the highest GST activity in both the organs was observed after 60 d exposure to wPE microplastics (Figures 2A–D).
Similar increases in GST enzyme activity due to wPE microplastic have been previously reported in mussels (Hariharan et al., 2021). Further Umamaheswari et al. (2021) reported that the microplastic exposure upregulated the GST enzyme both at gene and protein levels in zebrafish. Fish living in increasingly polluted habitats exhibit a predisposition to adjust their GST activity due to the need for detoxification responses, which aids the fish in surviving in a highly contaminated environment (Chatterjee and Bhattacharya, 1984; Gopalakrishnan et al., 2011). The most crucial elements of the xenobiotic detoxification enzymes, GSTs are expressed in a variety of ways. Different basic structures, enzyme characteristics, and physiological roles may be found in members of the GST superfamily (Josephy, 2010). The distribution of each GST class in tissues may also differ and many studies have examined the pathogenic and physiological activities of GSTs as well as their tissue distribution (Ren et al., 2009; Kim et al., 2017). GSTs thus are crucial enzymes for phase II xenobiotic metabolism, and the stimulation of this enzyme would be a sign of host response to pollutants.
Although there was an increase in enzyme activity in the initial exposure period (after 20 d), the gene expression was not apparent. The induction of GST enzyme activity corresponds to GST gene expression at the latter stages of exposure to wPE microplastic. The significant increase in GST activity further supports the theory that this could be a response directly linked to microplastic exposure. Since it is well known that a higher GST activity can signal a greater capacity for detoxification (Bocedi et al., 2019) it can be inferred from our findings that the increased GST gene expression and activity could be part of antioxidant and biotransformation responses of the liver brought on by microplastics. As an antioxidant and second-phase detoxification enzyme, GST is crucial in the detoxification of a variety of electrophilic chemicals into hydrophilic molecules (Lushchak, 2012). Our findings demonstrated that exposure to wPE microplastics upregulated GST expression and activity levels in both the tissues of the fish and also in both genders. The current ecotoxicological risk assessment on zebra fish is a preliminary investigation that will be used to determine the initial health risks to MPs, which will then help to assess the health of the local aquatic organisms, which in turn will help in coastal conservation and fisheries management (Murugan et al., 2005; Kumar et al., 2009; Janardhanam et al., 2022) by developing a biochemical indicator tool for the coastal management.
The results of the current investigation demonstrated that zebrafish glutathione-S-transferase enzymes were modulated as a result of exposure to wPE microplastics. This study also found that exposure to wPE microplastic altered the levels of gene expression for the biotransformation enzymes, suggesting that these enzymes might serve as useful biochemical indicators for the assessment of microplastic exposure in coastal and marine ecosystems. More research is needed to understand the exact role and mechanism of action of GST enzymes in microplastic metabolism and animal survival in coastal environments.
The original contributions presented in the study are included in the article/Supplementary Material. Further inquiries can be directed to the corresponding authors.
Ethical review and approval was not required for the animal study because Commercially cultured and model fish.
PP, MJ, PK performed experiment and laboratory analysis. TH: conceptualization, experimental design, data validation. TR: manuscript corrections and critical suggestions. GKS: statistical analysis of data and manuscript correction. TH and ES: manuscript preparation, reviewing the manuscript and project administration. All authors contributed to the article and approved the submitted version.
The authors thank Head, Research department of Zoology and also the Principal Pachaiyappa’s College for Men, Chennai- 600 030 and Head Department of Biotechnology, University of Madras, Guindy Campus, Chennai-600 025 India. Staff from National Centre For Sustainable Coastal Management, Chennai-600 025 also acknowledged for the suggestions and help through the study.
Author GS was employed by eProov Assessment Solution Pvt., Limited.
The remaining authors declare that the research was conducted in the absence of any commercial or financial relationships that could be construed as a potential conflict of interest.
All claims expressed in this article are solely those of the authors and do not necessarily represent those of their affiliated organizations, or those of the publisher, the editors and the reviewers. Any product that may be evaluated in this article, or claim that may be made by its manufacturer, is not guaranteed or endorsed by the publisher.
The Supplementary Material for this article can be found online at: https://www.frontiersin.org/articles/10.3389/fmars.2022.990351/full#supplementary-material.
Avio CG, Gorbi S, Regoli F. (2015). Experimental development of a new protocol for extraction and characterization of microplastics in fish tissues: First observations in commercial species from Adriatic Sea. Mar. Environ. Res. 111, 18–26. doi: 10.1016/j.marenvres.2015.06.014.
Bocedi A., Noce A., Marrone G., Noce G., Cattani G., Gambardella G., et al. (2019). Glutathione transferase P1-1 an enzyme useful in biomedicine and as biomarker in clinical practice and in environmental pollution. Nutrients 11 (8), 1741. doi: 10.3390/nu11081741
Bradford M. M. (1976). A rapid and sensitive method for the quantification of microgram quantities of protein utilizing the principle of protein–dye binding. Anal. Biochem. 72, 248–254. doi: 10.1006/abio.1976.9999
Chatterjee S., Bhattacharya S.. (1984). Detoxication of industrial pollutants by the glutathione glutathione-S-transferase system in the liver of Anabas testudineus (Bloch). Toxicol. Lett. 22 (2), 187–198. doi: 10.1016/0378-4274(84)90065-1
Chen Q., Gundlach M., Yang S., Jiang J., Velki M., Yin D., et al. (2017). Quantitative investigation of the mechanisms of microplastics and nanoplastics towardzebrafish larvae locomotor activity. Sci. Total Environ. 22 (2), 584–585, 1022-1032. doi: 10.1016/j.scitotenv.2017.01.156
Cole M. P., Lindeque P., Halsband C., Galloway T. S. (2011). Microplastics as contaminants in the marine environment: A review. Marine Pollution Bulletin 62 (12), 2588–2597. doi: 10.1016/j.marpolbul.2011.09.025
Cole M., Lindeque P., Fileman E., Halsband C., Goodhead R., Moger J., et al. (2013). Microplastic ingestion by zooplankton, environmental science and technology Environ. Sci. Technol. 47, 12, 6646–6655. doi: 10.1021/es400663f
Coles B., Ketterer B. (1990). The role of glutathione and glutathione transferases in chemical carcinogenesis. Crit. Rev. Biochem. Mol. Biol. 25 (1), 47–70. doi: 10.3109/10409239009090605
Davison P., Asch R.. (2011). Plastic ingestion by mesopelagic fishes in the North Pacific Subtropical Gyre. Mar. Ecol. Prog. Ser 432, 173–180. doi: 10.3354/meps09142
Dheenan P. S., Jha D. K., Das A. K., Vinithkumar N. V., Prashanthi Devi M., Kirubagaran R. (2016). Geographic information systems and multivariate analysis to evaluate fecal bacterial pollution in coastal waters of Andaman, India. Environ. pollut. 214, 45–53. doi: 10.1016/j.envpol.2016.03.065
Guilhermino L, Vieira LR, Ribeiro D, Tavares AS, Cardoso V, Alves A, et al. (2018). Uptake and effects of the antimicrobial florfenicol, microplastics and their mixtureson freshwater exotic invasive bivalve Corbicula fluminea, Sci. Total Environ. 622-623, 1131–1142. doi: 10.1016/j.scitotenv.2017.12.020
Gopalakrishnan S., Nai Z., Thilagam H., Bei C., Ding J., Wang X. H., et al. (2011). Biochemical responses and DNA damage in red sea bream from coastal fujian province, China. Ecotoxicol. Environ. Saf. 74 (6), 1526–1535. doi: 10.1016/j.ecoenv.2011.05.007
Habig W. H., Pabst M. J., Jakoby W. B. (1974). Glutathione s-transferases: the first enzymatic step in mercapturic acid formation. J. Biol. Chem. 249, 7130–7139.
Hamidian A. H., Jafari O. E., Feizi F., Wu C., Zhang Y., Yang M. Y. (2021). A review on the characteristics of microplastics in wastewater treatment plants: A source for toxic chemicals. J. Cleaner. Production. 295, 126480. doi: 10.1016/j.jclepro.2021.126480
Hariharan G., Purvaja R., Anandavelu I., Robin R. S., Ramesh R. (2021). Accumulation and ecotoxicological risk of weathered polyethylene (wPE) microplastics on green mussel (Perna viridis). Ecotoxicol. Environ. Saf. 208, 111765. doi: 10.1016/j.ecoenv.2020.111765
Hayes J. D., Flanagan J. U., Jowsey I. R. (2005). Glutathione transferases. Annu. Rev. Pharmacol. Toxicol. 45:1, 51–88. doi: 10.1146/annurev.pharmtox.45.120403.095857
Huang W., Song B., Liang J., Niu Q., Zeng G., Shen M., et al. (2021). Microplastics and associated contaminants in the aquatic environment: A review on their ecotoxicological effects, trophic transfer, and potential impacts to human health. J. Hazard. Mater. 405, 124187. doi: 10.1016/j.jhazmat.2020.124187
Huang J. N., Wen B., Meng L. J., Li X. X., Wang M. H., Gao J. Z., et al. (2020). Integrated response of growth, antioxidant defense and isotopic composition to microplastics in juvenile guppy (Poecilia reticulata). J. Hazard. Mater. 399, 123044. doi: 10.1016/j.jhazmat.2020.123044
Jabeen K., Su L., Li J., Yang D., Tong C., Mu J., et al. (2017). Microplastics and mesoplastics in fish from coastal and fresh waters of China. Environ. pollut. 221, 141–149. doi: 10.1016/j.envpol.2016.11.055
Janardhanam M., Sivakumar P., Srinivasan G., Sivakumar R., Marcus P. N., Balasubramaniam S., et al. (2022). Microplastics in demersal sharks from the southeast Indian coastal region. Front. Mar. Sci. 9. doi: 10.3389/fmars.2022.914391
Jha D. K., Vinithkumar N. V., Sahu B. K., Dheenan P. S., Das A. K., Begum M., et al. (2015). Multivariate and geo-spatial approach for seawater quality of chidiyatappu bay, south Andaman islands, India. Mar. pollut. Bull. 96(1–2), 463–470. doi: 10.1016/j.marpolbul.2015.05.004
Jin Y., Xia J., Pan Z., Yang J., Wang W., Fu Z. (2018). Polystyrene microplastics induce Microbiota dysbiosis and inflammation in the gut of adult zebrafish. Environ. pollut. 235, 322–329. doi: 10.1016/j.envpol.2017.12.088
Josephy P. D. (2010). Genetic variations in human glutathione transferase enzymes: significance for pharmacology and toxicology. Hum. Genomics Proteomics 2010, 876940. doi: 10.4061/2010/876940
Kim Y., Cha S. J., Choi H. J., Kim K. (2017). Omega class glutathione s-transferase: Antioxidant enzyme in pathogenesis of neurodegenerative diseases. Oxid. Med. Cell Longev. 2017, 5049532. doi: 10.1155/2017/5049532
Klein S., Worch E., Knepper T. P. (2015). Occurrence and spatial distribution of microplastics in river shore sediments of the Rhine-main area in Germany. Environ. Sci. Technol. 49, 6070–6076. doi: 10.1021/acs.est.5b00492
Kumar T. S., Vijayakumaran M., Murugan T. S., Jha D. K., Sreeraj G., Muthukumar S. (2009). Captive breeding and larval development of the scyllarine lobster petrarctusrugosus. New Z. J. Mar. Freshw. Res. 43:1, 101–112. doi: 10.1080/00288330909509985
Li Y., Li M., Li Z., Yang L., Liu X. (2019). Effects of particle size and solution chemistry on triclosan sorption on polystyrene microplastic. Chemosphere 231, 308–314. doi: 10.1016/j.chemosphere.2019.05.116
Li J., Qu X., Su L., Zhang W., Yang D., Kolandhasamy P., et al. (2016). Microplastics in mussels along the coastal waters of China. Environ. pollut. 214, 177–184. doi: 10.1016/j.envpol.2016.04.012
Livak K. J., Schmittgen T. D. (2001). Analysis of relative gene expression data using real-time quantitative PCR and the 2(-Delta Delta C(T)) Method. Method. Methods (San Diego, Calif.), 25(4)402–408 doi: 10.1006/meth.2001.1262
Lushchak V. I. (2012). Glutathione homeostasis and functions: potential targets for medical interventions. J. Amino Acids 2012, 736837. doi: 10.1155/2012/736837
Murugan T. S., Remany M. C., Mary Leema T., Jha D. K., Santhanakumar J., Vijayakumaran M., et al. (2005). Growth, repetitive breeding, and aquaculture potential of the spiny lobster, panulirus ornatus. New Z. J. Mar. Freshw. 39:2, 311–315. doi: 10.1080/00288330.2005.9517311
Neves D., Sobral P., Ferreira J. L., Pereira T.. (2015). Ingestion of microplastics by commercial fish off the Portuguese coast. Mar. Pollut. Bull. 101 (1), 191–126. doi: 10.1016/j.marpolbul.2015.11.008
Oliveira P., Barboza L. G. A., Branco V., Figueiredo N., Carvalho C., Guilhermino L. (2018). Effects of microplastics and mercury in the freshwater bivalve Corbicula fluminea (Mülle): Filtration rate, biochemical biomarkers and mercury bioconcentration ecotoxicol. Environ. Saf. 164, 155–163. doi: 10.1016/j.ecoenv.2018.07.062
Pannetier P., Morin B., Le Bihanic F. L., Dubreil L., Clérandeau C., Chouvellon F., et al. (2020). Environmental samples of microplastics induce significant toxic effects in fish larvae. Environ. Int. 134, 105047. doi: 10.1016/j.envint.2019.105047
Ren H. L., Xu D. D., Gopalakrishnan S., Qiao K., Huang W. B., Wang K. J. (2009). Gene cloning of a sigma class glutathione s-transferase from abalone (Haliotis diversicolor) and expression analysis upon bacterial challenge. Dev. Comp. Immunol. 33 (9), 980–990. doi: 10.1016/j.dci.2009.04.003
Schlenk D., Celander M., Gallagher E., George S., James M., Kullman S., et al. (2008). “Biotransformation in fishes,” in The toxicology of fishes, vol. pp . Eds. Di Giulio R. T., Hinton D. E. (New York: CRC press), 153–234.
Sussarellu R., Suquet M., Thomas Y., Lambert C., Fabioux C., Pernet M. E., et al. (2016). Oyster reproduction is affected by exposure to polystyrene microplastics. Proceedings of the National Academy of Sciences of the UnitedStates of America 113 (9), 2430–2435. doi: 10.1073/pnas.1519019113
Umamaheswari S., Priyadarshinee S., Bhattacharjee M., Kadirvelu K., Ramesh M. (2021). Exposure to polystyrene microplastics induced gene modulated biological responses in zebra fish (Danio rerio). Chemosphere 281, 128592. doi: 10.1016/j.chemosphere
Rodriguez-Seijo A., Lourenço J., Rocha-Santos T., da Costa J., Duarte A. C., Vala H., et al. (2017). Histopathological and molecular effects of microplastics in Eisenia andrei Bouché. Environ. Pollut. (Barking, Essex : 1987), 220 (Pt A), 495–503. doi: 10.1016/j.envpol.2016.09.092
Wang Q., Zhu X., Hou C., Wu Y., Teng J., Zhang C., et al. (2021). Microplastic uptake in commercial fishes from the bohai Sea, China. Chemosphere 263, 127962. doi: 10.1016/j.chemosphere.2020.127962
Wright S. L., Thompson R. C., Galloway T. S. (2013). The physical impacts of microplastics on marine organisms: A review. Environ. pollut. 178, 483–492. doi: 10.1016/j.envpol.2013.02.031
Keywords: Weathered microplastics, Fish, GST, gene expression, enzyme
Citation: Pandi P, Madhuvandhi J, Priya KK, Thiagarajan R, Gopalakrishnan S, Elumalai S and Thilagam H (2022) Weathered polyethylene microplastics exposure leads to modulations in glutathione-S-transferase activity in fish. Front. Mar. Sci. 9:990351. doi: 10.3389/fmars.2022.990351
Received: 09 July 2022; Accepted: 25 July 2022;
Published: 16 August 2022.
Edited by:
Dilip Kumar Jha, National Institute of Ocean Technology, IndiaReviewed by:
Jun Bo, State Oceanic Administration, ChinaCopyright © 2022 Pandi, Madhuvandhi, Priya, Thiagarajan, Gopalakrishnan, Elumalai and Thilagam. This is an open-access article distributed under the terms of the Creative Commons Attribution License (CC BY). The use, distribution or reproduction in other forums is permitted, provided the original author(s) and the copyright owner(s) are credited and that the original publication in this journal is cited, in accordance with accepted academic practice. No use, distribution or reproduction is permitted which does not comply with these terms.
*Correspondence: S. Elumalai, YW5hbmFuZGFsQGdtYWlsLmNvbQ==; H. Thilagam, dGhpbGFnYW1wYWNoYWl5YXBwYXNAZ21haWwuY29t
†These authors have contributed equally to this work
Disclaimer: All claims expressed in this article are solely those of the authors and do not necessarily represent those of their affiliated organizations, or those of the publisher, the editors and the reviewers. Any product that may be evaluated in this article or claim that may be made by its manufacturer is not guaranteed or endorsed by the publisher.
Research integrity at Frontiers
Learn more about the work of our research integrity team to safeguard the quality of each article we publish.