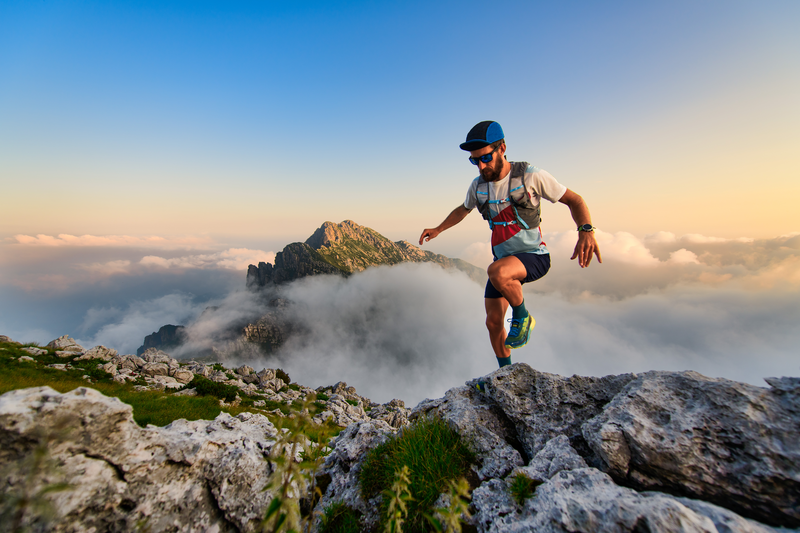
94% of researchers rate our articles as excellent or good
Learn more about the work of our research integrity team to safeguard the quality of each article we publish.
Find out more
ORIGINAL RESEARCH article
Front. Mar. Sci. , 26 September 2022
Sec. Coastal Ocean Processes
Volume 9 - 2022 | https://doi.org/10.3389/fmars.2022.989841
This article is part of the Research Topic Marine and Coastal Environments under Extreme Stress View all 9 articles
The Persian Gulf is a thermally extreme environment in which reef corals have adapted to survive through temperature ranges that would be lethal to corals from other regions. Despite offering a unique opportunity to better understand how corals from other regions may adapt in the future, through a changing climate, much of the Gulf coral and fish communities remain to be described. In the southwestern Gulf nation of Qatar few reef sites have been described to date. We here characterize reef communities from 16 sites around the Qatar Peninsula, encompassing depths from 3 to 25m. We found the healthiest coral reef communities to be in deeper offshore reefs, with high coral and fish species richness and high coral abundance, likely a result of their occurrence below summer thermocline depths and distance from urban pressures. In contrast, we found shallow reefs, both nearshore and offshore, to have low species richness and abundance relative to deeper reefs, presumably due to impacts from recurrent bleaching events and development pressures over recent decades. The results of this work underscore the Qatar Peninsula as being at the biogeographic epicenter of the wider Gulf. However, further temperature increases may push both fishes and corals over their physiological limits. Management efforts at both the regional and global level are needed to reduce thermal stressors and preserve the rich reef ecosystems found in the waters surrounding Qatar.
Coral reefs around the world are at threat from climate change (Morrison et al., 2019; Cornwall et al., 2021; Obura et al., 2022). Global warming and temperature anomalies have already resulted in the degradation of up to 50% of the world’s coral reefs with 14% loss just in the last decade (IPBES, 2019; Souter et al., 2021). This has caused a loss of 50% of ecosystem services provided by coral reefs since 1950, including a decline in 60% of coral reef associated fishes catch per unit effort (Eddy et al., 2021). In some regions of the world, coral reefs may be better prepared than others to these changing conditions, having already experienced extreme environmental conditions over the past centuries (Burt et al., 2020).
The Persian Gulf, also referred to as Arabian Gulf (thereafter termed “the Gulf”), is one of the most thermally extreme marine environments in the world that contain reef coral communities (Coles, 2003; Vaughan et al., 2019). Summer seawater temperatures often reach 36°C in shallow waters with these warm temperature sustained down to ~15 meters depth, and winter temperatures sit around 20°C, although they have occasionally been recorded to drop down to 12°C (Coles, 2003; Rakib et al., 2021). Salinity in the Gulf is also higher than in other coral reef ecosystems, with values of 44 psu on average, and living corals observed in regions of the Gulf where salinity was measured at 50 psu (Sheppard, 1988; Coles, 2003). Although coral diversity in the Gulf is lower than elsewhere as a result of the environmental stress, the Gulf harbors a number of coral species that have adapted to an environment where corals from other regions of the world would not survive (Coles, 2003). Similarly, the fishes of the Gulf have developed the ability to persist in environmental conditions that would be lethal to fishes in other parts of the world (Feary et al., 2010; D’Agostino et al., 2020), with winter temperatures well below the optimal range for coral reef fishes in the Indo-Pacific, and summer temperatures reaching values that would cause respiratory failure in fishes from other regions (Nilsson et al., 2009; Gardiner et al., 2010).
In the southwestern Gulf, the coastline of the Qatar Peninsula was historically bordered by thriving coral assemblages, extending over 200 km of coastline and heavily dominated by Acropora table corals (Shinn, 1973; Shinn, 1976). But the combination of a cold-water bleaching event, recurrent warm bleaching events, dredging, industrial development, increased sedimentation from extensive coastal development, and pollution from the industrial sector, resulted in the functional extinction of coastal coral communities between the 1960s and the late 2000s (Maghsoudlou et al., 2008; Burt et al., 2016). In deep offshore sites, corals were typically less impacted over time but the global 1998 bleaching event resulted in widespread mortality even at these deeper sites (Rezai et al., 2004; Riegl and Purkis, 2009). One recent study examined the coral communities of Qatar, at one deep (~18m) offshore site and two shallow (~3m) inshore sites (Burt et al., 2016). The authors found that the deeper site had high coral cover and coral richness but that it remained impaired compared with earlier observations. In contrast, one inshore site was dominated by extensive dead coral rubble amongst which a small number of corals had survived, indicating the site was once populated by a healthy coral community. The other inshore site was dominated by bare rock and sand, with a low coral cover despite carrying a species richness of eleven coral species (Burt et al., 2016).
Currently, the coral reefs of the Gulf and their associated fishes are at risk (Sheppard et al., 2010; Sheppard, 2016). The region is exposed to repetitive temperature anomalies in the warmest months of the year, exacerbating the already extreme environment (Riegl and Purkis, 2015; Burt et al., 2019), and the Gulf is warming at a rate more than double the global average (Lachkar et al., 2021). With the increase in bleaching events, less time is available for recovery, and only some of the most resistant coral species may be selected over time (Burt et al., 2011a; Riegl et al., 2018). In Qatar, the effects of bleaching events are currently hard to quantify given the limited availability of baseline quantitative surveys. In one recent study that surveyed coral communities in Qatar, the surveys were isolated to just three known reefs (Burt et al., 2016). Additionally, there are no quantitative surveys of coral reef fishes in Qatar published to date. We here present a comprehensive spatial analysis of coral reef communities in Qatar. We investigated 16 sites around the Qatar Peninsula, including shallow inshore sites (3-6 m), and deeper sites (up to 25m). We studied both reef coral and fishes communities, and investigated patterns of species richness, abundance, composition, and communities.
Benthic surveys were conducted at sixteen coral reef sites throughout Qatar’s territorial waters and fish surveys were conducted at nine of these sites (Figure 1). Shallow coral sites (3-6 m depth) mostly include some three-dimensional reef structure, while deeper sites have a flatter topography with non-accreting coral communities lying directly on limestone domes amongst the surrounding dominant sedimentary sea bed (Sheppard et al., 2010). Each site had minimal depth variability. At each site, six parallel 30m-long transects were laid along the seafloor at a 5-10 m distance from each other, serving as reference for both fish and benthic surveys. All surveys were conducted on SCUBA. At sites where both fish and corals were surveyed (see Table 1 for details), fish surveys were conducted as the transect tape was being laid out and were followed by the benthic surveys. Due to safety issues related to depth and currents only five transects for fishes were able to be completed at two sites (Mushroom Garden and Fasht East Halul).
Figure 1 Map of the 16 coral reefs at which benthic composition was assessed. In blue: deep coral reef (18-25m). In teal: medium-depth coral reef (10-16m). In yellow: shallow coral reef (3-6m). In orange: sites where fish surveys were conducted. The map was produced in R (R Core Team, 2019), using the packages ggplot2 (Wickham, 2016), rnaturalearth (South, 2017), and sf (Pebesma, 2018).
Table 1 List of sites surveyed in Qatar, and which survey type (fishes and/or benthic) was conducted at each site.
Coral reef fishes were surveyed at nine surveys sites. At each site, fishes were visually censused with all fishes observed within 30 m x 1 m belt transects identified to species and enumerated. This approach has been widely used elsewhere in the Gulf in the past (Burt et al., 2011b; Burt et al., 2013b; Vaughan et al., 2021), and the narrow transect width reflects the relatively high local turbidity that can affect accuracy on wider transects (Sale and Sharp, 1983). As reef fishes communities in the Gulf are known to be seasonally dynamic, surveys were performed during the summer/autumn season when reef fishes communities exhibit peak annual species diversity and abundance (McCain et al., 1984; Coles and Tarr, 1990; Burt et al., 2009; Vaughan et al., 2021), with fish surveys conducted at four sites in October 2016 (Bulhambar, Fasht East Halul, Sheraoh Island and Fasht al Udayd) and the remaining sites (Um al Arshan, Mushroom Garden, Ras Dhow, Fasht al Hurabi, and Al Ashat) surveyed in August 2017.
Each fish species was classified into one of four trophic guilds: planktivores, herbivores (including grazing and browsing species that incidentally consume small invertebrates, such as parrotfishes), pelagic carnivores (targeting fishes, cephalopods and other large animals), and benthic carnivores (targeting benthic invertebrates). Classification was based on the major dietary component described in Fishbase (www.fishbase.org), Randall (Randall, 1995) or from local dietary studies where available (Shraim et al., 2017), with the closest congener used where data were unavailable for a particular species.
At each site, an underwater camera mounted on a frame captured a 0.25 m2 photoquadrat image every 3m along each transect, for a total of 11 photoquadrats per transect, or 66 photoquadrats per site. This approach has been successfully used to characterize benthic community structure in several coral assemblages within the Gulf (e.g., Burt et al., 2013a; Bento et al., 2016; Burt et al., 2016; Burt et al., 2019). The photoquadrat surveys were performed in 2016–2017, with the exception of the deep site North-3, which was surveyed in 2015. All sites initially surveyed were revisited in 2017, during which no change in benthic community were observed.
The photoquadrat images were analyzed using CoralNet (https://coralnet.ucsd.edu), an online platform for analyzing coral reef images (Beijbom et al., 2012; Beijbom et al., 2015; Williams et al., 2019; Chen et al., 2021). Each image was automatically assigned 50 random points by CoralNet. All points were then manually annotated by an expert coral scientist. Scleractinian corals were identified to the species level; non-scleractinian-coral invertebrates were identified as anemone, ascidian, bryozoan, bivalve, sea urchin, hydrozoan, octocorallia, sponge, zoanthid, or other invertebrate; algae were categorized as crustose coralline algae (CCA), freshly algae or turf algae; and substrate categories included dead coral, rock pavement, broken coral rubble, sand, shell hash/gravel, and shadow. Once all the points were annotated, the percent cover of each benthic category was calculated within each quadrat and the data was exported into Microsoft Excel for further analyses.
All fishes abundance data were converted to density 100 m-2 prior to analyses, and density and richness data were log(n+1) transformed prior to statistical analyses. Benthic cover data were converted to percentages. Nested Analyses of Variance (ANOVAs) were used to test for differences in fish species richness, fish density, and coral cover. Normality of residuals was verified with a Shapiro‒Wilk normality test. Homoscedasticity and independence were verified visually by plotting the model residuals against the fitted model. The fish analyses were based on two depths (deep & medium versus shallow) and coral analyses were based on three depths (deep, medium, and shallow). For each nested ANOVA, sites were nested within depth. Where significant differences occurred, post-hoc tests were used to identify significantly different groups using unequal-N Tukey’s for fishes, and least square means pairwise comparisons with Tukey adjustment for multiple comparisons for corals. Differences in coral richness among depths were analyzed with a Kruskal-Wallis test with a Dunn’s multiple comparison posthoc test.
Non-metric multidimensional scaling (nMDS) ordination plots based on Bray-Curtis distance matrices were used to graphically illustrate differences in community structure in fishes and corals, among sites and depths. Vector plots were used to illustrate the strength and direction of any species having strong correlation with either ordination axis (Pearson’s r >0.5). A similarity permutation (SIMPER) analysis was used to identify the fish species driving differences between deep/medium-depth and shallow reef sites and to determine the strength of their contribution to these differences. In order to reduce the influence of outliers, species occurring in less than 5% of samples (13 species) were removed prior to multivariate analyses as recommended by McCune and Grace (McCune and Grace, 2002). An analysis of similarity (ANOSIM) was used to verify that coral community patterns were significantly different among depth categories. Pairwise ANOSIM tests with Bonferroni correction were then conducted to identify which groups were different. The species driving the differences were identified using a species indicator analysis. All fishes analyses were conducted in Primer, and all coral analyses were conducted in R (R Core Team, 2019) with the packages car (Fox and Weisberg, 2019), FSA (Ogle et al., 2019), ggplot2 (Wickham, 2016), indicspecies (Cáceres and Legendre, 2009), lsmeans (Lenth, 2016), multcomp (Hothorn et al., 2008), multcompView (Graves et al., 2015), nlme (Pinheiro et al., 2019), rcompanion (Mangiafico, 2019), and vegan (Oksanen et al., 2015).
In total, 46 species of fishes from 23 families were observed on the coral reefs in this study (Supplementary Material; Table S1), and species richness varied among sites (Figure 2A). The total species richness observed at each site ranged from 3 to 25 species, with the highest total richness tending to occur at the deep offshore sites (Um al Arshan, Mushroom Garden, and Fasht East Halul) where total richness averaged 21.7 ± 1.8 species site-1, double the species richness observed at shallow sites (mean: 10.8 ± 2.6 species site-1 from Fasht al Hurabi to Al Ashat). In the medium-depth sites, Bulhambar had a richness comparable to the deep northern sites (23 species) while Ras Dhow had richness comparable to the shallow, southern sites (12 species).
Figure 2 (A, B) Total species richness per site in (A) fishes and (B) scleractinian corals. The color coding refers to the depth of the sites (see Figure 1 for details). (C) Mean density of fishes (100 m-2, ± SE) per site. Different letters indicate a significant difference (ANOVA post-hoc: Tukey’s unequal-N test, α = 0.05). (D) Percent cover (± SE) of scleractinian corals per site. Different letters indicate a significant difference (ANOVA post-hoc: comparison of least square means with Tukey adjustments, α = 0.05) (A-D) Sites with similar colorations are located at similar depths. (E) Breakdown of differences in the density of the five most abundant families of fishes (per 100 m2, ± SE). The remaining 17 “other families” each contributed < 5% to total abundance. (F) Breakdown of differences in the percent cover of the seven most abundant families of scleractinian corals. (G) Breakdown of major trophic groups of fishes at each site (100 m-2, ± SE). (H) Breakdown of major benthic categories at each site. All sites are organized from left to right from deeper to shallower. See Table 1 for the list of site names that match the site codes used here.
A nested ANOVA of the mean species richness observed within transect at each site reflected these observations, showing significantly higher species richness in the deep offshore and medium-depth midshore sites compared with the shallow sites (F(1,41) = 90.9, p< 0.001) along with differences among sites within depths (F(7,41) = 74.7, p< 0.001). With the exception of one species (the redtoothed triggerfish, Odonus niger) all species observed in Qatar have been reported elsewhere in the Gulf in earlier records (see Supplementary Material; Table S1). O. niger is a common species on coral reefs in the Gulf of Oman and throughout the western Indian Ocean (Randall, 1995), but its occurrence in Qatar represents the first record that we are aware of for this species occurring within the Gulf.
Overall the mean density of individual reef fishes was 169.7 ± 17.9 individuals 100 m-2 across Qatar, but there was broad variation in abundance among sites (Figure 2C). In contrast with the species richness patterns, the mean density of fishes at the deep offshore reefs and medium-depth midshore reefs (136.3 ± 6.6 ind. 100 m-2) was significantly lower than at the shallow reef sites, where there were > 50% more fish (214.7 ± 4.4 ind. 100 m-2; Nested ANOVA F(1,41) = 30.2, p< 0.001). However, there were also significant differences among sites when controlling for depth (Figure 2C; nested ANOVA: sites(depth) F(7,41) = 39.7, p< 0.001), with the high density of fish at Fasht al Udayd (450 ± 33 individuals 100 m-2) being significantly more abundant than at all sites except Fasht East Halul (Figure 2C). Excluding this site, the average density of fishes in deep and shallow sites were virtually identical (deep/medium-depth mean: 136.4 ± 16.2 vs shallow mean: 136.3 ± 20.7 individuals 100 m-2).
There was broad variation in the major families characterizing reef fish communities at the deep and shallow sites (Figure 2E). At the deeper offshore and midshore sites, the major contributors to abundance were Pomacentridae (mean: 24.3% of total fish abundance) and Labridae (19%), with the largest contribution (42.7%) coming from “other families”, reflecting the significantly higher species diversity at these sites (Figure 2A). In contrast, at shallow sites Pomacentridae were rare (2.9%) and Labridae entirely absent (0%), with nearly three-quarters of the total fish abundance instead represented by Lutjanidae (50.2%) and Nemipteridae (22% of total abundance).
Of the 46 species observed in this study, 54% (25 species) were classified as benthic carnivores, 17% (8) as pelagic carnivores, 9% (4) as planktivores and the remaining 20% (9) as herbivores. Because abundance varied among species, the relative contribution of trophic groups to overall abundance differed slightly from the taxonomic breakdown, with benthic carnivores contributing 56% of overall abundance, 34% as pelagic carnivores, 8% as planktivores, and only 2.1% of abundance made up of herbivores. However, the relative contribution of trophic groups varied significantly between depths and sites within depths (Figure 2G; multivariate nested ANOVA: depth F(4) = 45.6, sites(depth) F(28) = 14.5, p< 0.001 each). Benthic carnivores made up the largest component of the fish communities at seven of the nine reef sites (Figure 2G), and while there was significant variation among sites within depths (nested ANOVA F(7,41) = 20.7, p< 0.001), they did not differ in abundance between deeper and shallow reefs (F(1,41) = 4.0, p > 0.05). A similar pattern was observed with the rarest group, herbivores, which did not differ in abundance between deeper and shallow reefs, although there was variation among sites within depths (F(1,41) = 2.7, p > 0.05 and F(7,41) = 17.2, p< 0.001, respectively). In contrast, both pelagic carnivores and planktivores did vary between depths (F(7,41) = 119.4, p< 0.001 and F(7,41) = 24.6, p< 0.001, respectively). Pelagic carnivores made up nearly half (49%) of the total fish abundance at shallow sites, and were the most abundant member of the fish community at two of these shallow sites, while at deep reefs they made up just 11% of total abundance, on average. The inverse pattern was observed for planktivores, which were relatively common on deep reefs (20% of abundance, on average), but were entirely absent from all shallow reefs (Figure 2G).
In total, 34 scleractinian coral species were identified in transects in this study (Supplementary Table S2). Total richness at each site varied between 3 and 24 species, with the highest richness in the deeper sites (Figure 2B). Overall, the deep sites had a richness of 21 ± 1.0, the medium-depth sites had a richness of 15.3 ± 0.7, and the shallow sites had a richness of 5.0 ± 0.8. A nested ANOVA of the mean species richness observed within transect at each site supported the pattern, showing significant differences among sites within depths (F(15,77) = 165.8, p< 0.0001), with a Krukal-Wallis test revealing that species richness was significantly different among each of the three depth ranges surveyed ( = 68.7, p = 1 × 10-15). Mean species richness was the highest at deep offshore sites, was intermediate in medium-depth sites, and was the lowest at shallow sites.
Coral cover varied from 3% to 39% (Figure 2D). Except for North 3 which only had 5% coral cover, all deep sites had a higher percent cover (27-39%) of reef-building corals than the medium depth (3-17%) and shallow sites (7-20% coral cover). A nested ANOVA of the mean coral cover observed within each quadrat at each site showed significant differences among sites within depths (F(15,1026) = 145.32, p< 0.0001), with a Krukal-Wallis test revealing that coral cover was significantly different among each of the three depth ranges surveyed ( = 229.3, p< 2 × 10-16). Mean coral cover was the highest at deep offshore sites and was the lowest at medium-depth sites.
Coral composition was variable across sites and depths (Figure 2F) but one family, the Merulinidae, was dominant in nearly all sites, representing 63.5% of all scleractinian coral cover. The second most common family was the Poritidae, also present at all sites, representing 17.5% of total coral cover. Agariciidae (2.8%) and Dendrophylliidae (2.6%) were only present in deep sites. Psammocoridae 6.0%) were found in deep and medium-depth sites but only in one of the five shallow sites. Acroporidae (2.1%) were patchy and only found in 5 sites (Binzayan, Fasht East Halul, Um al Schaer, Halul and Sheraoh Island) but were not restricted to a single depth range. The Incertae sedis family (comprising Leptastrea and Pleasiastrea) were absent from three shallow sites and one medium-depth site. All other families represented less than 5% of the total coral cover and were grouped as “other”.
Live scleractinian corals represented 15.8% of all substrate, throughout sites and depths (Figure 2H). The most common living category overall was algae (35.2%), which were present at all sites. The algae category included mostly turf algae but also some filamentous algae. Crustose coralline algae (CCA, 1.9%) were mostly found at shallow and medium-depth sites. Octocorals (0.2%) were only found in a handful of locations. The site Mushroom Garden hosted a soft coral (octocoral) community covering 2% of the benthos and composed solely of the coral Junceella juncea. Sponges (0.7%) were found at all sites but in low abundance. All other categories were non-living, represented mostly by shellhash (20.2%) and sand (15.5%).
Multivariate analyses were used to explore patterns in the overall fish community and to identify particular species driving divergence between deep and shallow sites. An ordination of the overall fish assemblage showed that fish communities clustered into two distinct groups representing fish communities at the deep and medium depth offshore sites and those of the shallow sites (Figure 3A), and these groups differed significantly (nested PERMANOVA Pseudo-F(1,49)=6.1, p = 0.01). Transects from deeper sites were relatively tightly grouped and overlapping for most sites, indicating a high degree of similarity in community structure. The only site which deviated from this main cluster was Ras Dhow, with the broad spread for this site reflecting the greater variability of fish among transects in this low abundance site (see above), although it still largely overlapped with the deep site communities. The fish communities at shallow sites grouped together, although transects were more tightly clustered within sites rather than broadly overlapping, indicating that communities were relatively more site-specific. Variation in communities along the first axis was primarily driven by differences in the relative abundance of the shrimp goby Cryptocentrus lutheri. Differences between deep and shallow reef fish communities were primarily aligned on the second axis. Lutjanus ehrenbergii and Pomacanthus maculosus were highly associated with shallow sites, while six species were strongly associated with deep sites (five of which were absent from shallow sites, Cephalopholis hemistiktos excluded).
Figure 3 Non-metric multidimensional scaling plots illustrate community composition and clustering in (A) fishes and (B, C) corals in Qatar. (A) Each point represents the fish community in one transect with dark blue symbols indicating deep sites and yellow symbols representing shallow sites. Vectors are used to indicate the strength and direction of species strongly correlated with either axis (only species with r > 0.6 are included); the outer circle represents a scale for a correlation of r = 1). (B) Each point represents the coral community in one transect (C) Ordination plot of the scleractinian coral communities.
A SIMPER analysis was performed to determine which species were driving differences between deeper and shallow sites, and their relative contribution. Overall, there was 85.2% dissimilarity between communities in deep/medium depth and shallow sites. The ten most important species identified as driving differences between the deeper and shallow reef fish communities are listed in Table 2, which together contributed nearly 60% of the total dissimilarity between these groups. The three most important species driving differences between depths were primarily associated with shallow sites, and these three species together contributed a quarter of all dissimilarity between shallow and deep reef fish communities (L. ehrenbergii, Scolopsis ghanam and P. maculosus). Only one additional important driver species, Acanthopagrus bifasciatus, was more common to shallow sites, and this contributed an additional 4.5% to dissimilarity. The remaining six of the top ten driver species identified by SIMPER were more abundant in deep sites, and together these species contributed an additional 29% of the total variation between deep and shallow sites. Five of these six species were entirely absent from shallow sites (Table 2), with the one exception, Cephalopholis hemistiktos, being an order of magnitude less abundant at the shallow sites.
Table 2 The ten most important species driving differences between deep and shallow reef fish communities as identified from SIMPER analysis, ranked by importance.
As for fishes, multivariate analyses were used to explore patterns in the coral community. An NMDS ordination plot of the coral assemblages showed that corals clustered into three distinct groups, representing coral communities from deeper offshore sites, from medium-depth midshore sites, and shallow inshore sites (Figure 3B). These groups were significantly different from each other (ANOSIM, R = 0.56, p< 0.0001; pairwise comparisons, R(shallow-medium) = 0.72, R(shallow-deep) = 0.77, R(medium-deep) = 0.19, adj. p< 0.0003). The first NMDS axis separated shallow site communities from the deep and medium-depth site communities, and the second NMDS axis separated the deep communities from the medium-depth communities. Variation in communities along the first axis was primarily driven by differences in the relative abundance of Cyphastrea microphthalma, Cyphastrea serailia, and Porites harrisoni, which characterized the shallower sites, and Dipsastraea pallida, which characterized the deeper sites (Figure 3C). The separation between medium-depth and deep sites along the second NMDS axis, was primarily driven by differences in the abundance of Porites harrisoni and Acropora downingi, which characterized the medium-depth sites, and Turbinaria reniformis, T. peltata, Goniopora spp., and Dipsastraea favus, which characterized the deep sites. An indicator species analysis (De Cáceres et al., 2010) revealed that 21 species were significantly associated with one or two depth groups (Table 3). The three most important species were Dipsastraea favus (deep indicator species), Psammocora stellata (deep indicator species), and Cyphastrea microphthalma (shallow indicator). Medium-depth sites only had one significant indicator species, Psammocora profundacella.
Table 3 List of significant indicator coral species, associated with one or two depth groups. r is a correlation statistic.
Coral reefs are the most diverse and productive ecosystems in the Gulf (Sheppard and Sheppard, 1991). While coral assemblages have been described in most countries around the Gulf, this is the first study to date that has extensively and quantitatively described coral assemblages and coral reef fishes in Qatar. We found the healthiest coral reef communities to be in the deeper offshore reefs, with high coral and fish species richness and high coral abundance.
Forty-six species of reef fishes from 23 families were observed in this study. Although this is only a minor fraction of the 302 reef-associated species occurring across the Gulf (Grandcourt, 2012), it is comparable to the diversity observed in neighboring nations during short-term surveys performed at spatial scales similar to those conducted here (Saudi Arabia: 52 species (McCain et al., 1984), Bahrain: 55 species (Smith and Saleh, 1987), Gulf coast of UAE: 29 species (Burt et al., 2011b). This observed species richness is likely still under-representative of the total diversity of reef fishes on Qatar reefs, as surveys were conducted only once per site and on six or fewer transects. Indeed, another 19 fish species have been reported to occur in Qatar, in a subsample of six families: surgeonfishes, parrotfishes, butterflyfishes, snappers, sea breams, and groupers (Bouwmeester et al., 2020). For example, in the western Saudi Arabian Gulf, more comprehensive surveys conducted over two years brought the estimated richness from short-term surveys from 52 species (McCain et al., 1984) to a total of 101 species (Coles and Tarr, 1990). It is, therefore, recommended that a broad scale, long-term monitoring program be established in order to enhance the biodiversity estimates for fishes associating with coral reefs in Qatar.
Our results showed that nearly twice as many species occurred at deep, offshore reefs compared with shallow reefs, with differences in composition underpinning broadly divergent multivariate community structure between depths. This echoes the results of other studies in the Gulf which have found that species richness increases with depth (Smith and Saleh, 1987; Coles and Tarr, 1990). Shallow reefs in the Gulf experience more variable and extreme environmental conditions than the deep, offshore reefs (Sheppard et al., 1992), and the extreme conditions on shallow reefs may represent a direct physiological barrier that is beyond the survival capacity for some fishes due to their elevated respiratory demand (Shraim et al., 2017; Brandl et al., 2020). The reduced richness at the shallow sites may also be an indirect reflection of differences in the coral community. It is well recognized that the best developed and most diverse coral communities in the Gulf occur offshore as a result of the less extreme thermal conditions (Sheppard et al., 1992), and the greater three-dimensional complexity provided by corals at these sites may also play a role in supporting the higher richness of fish species at these sites (Luckhurst and Luckhurst, 1978; Darling et al., 2017).
The differences in fish species richness across depths were echoed in the trophic structure of these communities. Similar to earlier studies in the southern Gulf (e.g. Feary et al., 2010), we found that benthic carnivores were the most common trophic group on Qatar’s reefs. This group showed no variation across depths, suggesting that the availability of food items was relatively consistent across sites. Planktivores were significantly more abundant at deep sites, while pelagic carnivores were more common to shallow sites. The variation in these trophic groups across depths is less likely to be based on food availability than to the particular species associating with these groups. The planktivores more common to the deep sites were almost entirely made up by two damselfishes species (Chromis xanthopterygia and Neopomacentrus cyanomos), which did not occur on shallow reefs here. These species are also extremely rare on shallow reefs in the southern Gulf coast of the UAE (Feary et al., 2010; Burt et al., 2011b) but are known to occur in high abundance at shallow depths on the more environmentally benign reefs of Saudi Arabia and Kuwait where they are often among the most common species in transects (Downing, 1985; Krupp and Almarri, 1996). This suggests that their distribution on reefs in Qatar is likely structured more by environmental conditions than food availability, with these fish unable to cope with the extreme temperatures of the shallow sites in the southern basin of the Gulf. In contrast, pelagic predators were significantly more common to shallow sites. Here, the vast majority of individuals making up this trophic group was the snapper Lutjanus ehrenbergii, a species that is among the most common fishes in shallow reef environments throughout the southern basin of the Gulf as well as in southern Iran (Rezai and Savari, 2004; Feary et al., 2010; Burt et al., 2011b; Vaughan et al., 2021). This species was entirely absent from most deep reefs in Qatar, and was only consistently observed in transects at a single deep site (Fasht east of Halul). It is unclear why this species is found at such low abundance on the deep sites in Qatar. Damselfishes and other small-bodied fishes that could serve as prey items are considerably more common at deep sites than shallow sites, suggesting that food availability is unlikely to be limiting L. ehrenbergii distribution on these sites. This species has been suggested to aggregate on nearshore reefs during the summer before migrating to deeper environments over the winter (Vaughan et al., 2021), and it is possible that the timing of our surveys in summer and early autumn captured this species during a period of unusually high seasonal abundance on shallow reefs. Further research on the temporal shifts in community structure is warranted.
Coral reef fishes are generally very dependent on the health of their coral reef habitats, relying on them for shelter, food, and nurseries (Bell and Galzin, 1984; Friedlander and Parrish, 1998). In Qatar reefs, we found 34 coral species from 13 families throughout the photoquadrat surveys, out of the 66 coral species reported for the entire Gulf (DiBattista et al., 2016). Additional species (i.e. Turbinaria cf. patula, Goniopora spp., Alveopora tizardi, Montipora aequituberculata, Cycloseris costulata, C. fragilis, Hydnophora pilosa) were observed on the reefs surveyed but they were outside the transects (Bouwmeester et al., 2020), and all located in the deeper offshore reefs. Additional surveys in these deeper reefs will likely provide quantitative information on the abundance and distribution of these less common species.
In shallow sites, coral cover was generally low and was replaced with abundant algal cover. Algae compete directly with corals for light and space (McCook et al., 2001) and in mesotrophic waters such as the Gulf, algae easily outcompete corals if not regulated by herbivore species (Ledlie et al., 2007; Cheal et al., 2010). We found that herbivorous fishes were mostly absent from these reefs, a surprising rarity that has also been observed on other reefs in the southern Gulf (e.g. Feary et al., 2010; Vaughan et al., 2021) most notably due to a near absence of functionally important parrotfishes (Hoey et al., 2016; Hoey et al., 2018). Without herbivores preventing overgrowth of corals by algae and creating available substrate for coral larvae to settle on and develop, the long-term sustainability of reef corals may be compromised (Hughes et al., 2007; Green and Bellwood, 2009). With low coral cover and richness, shallow sites generally contained few fishes, although in one site (Fasht al Udayd) fish abundance was higher than elsewhere, with at least twice as many fishes than any other surveyed site. The site had low coral richness and abundance; however, it contained a three-dimensional skeletal reef structure that provided an intricate habitat for fishes (pers. obs.). This structure was no longer formed by living corals and consisted in consolidated dead coral skeleton covered in algae. The site may have once been a thriving coral reef but for now, it serves as a habitat for fishes and other marine life. If the reef architecture was to erode and fall apart, the site would likely no longer be able to continue to support the current fish communities. Indeed, after major coral mortality events, fish communities may remain minimally affected for as long as the reef architecture remains but eventually they would decline as well, alongside the eroding and flattening of the reef substrate (Garpe et al., 2006; Graham et al., 2009).
Deep coral reefs hosted much higher coral cover and richness than shallower sites. While several shallow sites were known prior to this study (i.e. Fuwayrit, Fasht al Hurabi, Al Ashat, Sheraoh Island) (Foster et al., 2013; Burt et al., 2016; Kardousha et al., 2016; Warren et al., 2016), few deep sites had been documented, other than Halul and Um al Arshan. This study characterized an additional nine sites to the deep and medium-depth sites that were previously undescribed. The discovery of such a diverse and abundant ecosystem is of high value at a time when shallow reefs in Qatar are heavily suffering from global and local anthropogenic impacts (Sheppard et al., 2010; Riegl et al., 2013; Sheppard, 2016). Indeed, shallow Qatari reefs are heavily exposed to coastal constructions (Richer, 2008) and the variability of sea surface temperatures is extremely high between the summer and the winter (Coles, 2003; Rakib et al., 2021). Although seawater temperatures are known to be naturally hot in the summer, summer marine heat waves are increasingly frequent (Riegl et al., 2018; Burt et al., 2019), creating a slow but steady decline of the Gulf’s coral communities, resulting in the loss of 40% of coral cover across the region since the late 1990s (Burt et al., 2021). While the shallow reefs of Qatar are declining, deeper reefs may temporarily provide a more stable environment where Gulf corals may be able to survive. Their distance from the shore and land-associated anthropogenic stresses is a clear advantage, and the depth currently offers cooler waters in the warmer months of the years. Indeed, while the sea surface temperatures can exceed 36°C, at the offshore locations 35°C water temperatures occur from several meters below the surface down to a thermocline at ~16m depth, below which temperatures stabilized at ~32°C (pers. obs.), matching previously reported summer temperature profiles in the Gulf (Rakib et al., 2021), with deep-water coral assemblages occurring below this thermocline depth, providing a vertical refuge from heat stress. However, should Gulf seawater temperatures continue to increase over time, then the thermocline may be pushed further down, thus exposing the remaining deep coral reef ecosystems to extreme thermal conditions similar to those found in shallow inshore sites.
The results of this work reveal Qatar to be in a central position to the ecological dynamics of the Gulf and present an important starting point for quantitative coral reef ecology work both in Qatar and in the remaining Gulf. The data collected during this comprehensive baseline assessment serve as an important foundation for the growing ecosystem-based management approaches being adopted in Qatar (Burt et al., 2017; Fanning et al., 2021), allowing development of a spatially and temporally integrated long-term monitoring program. We show that the deep, offshore seamounts in Qatar represent a unique blend of the diverse, planktivore-dominated reef fish communities of the northern Gulf, while the fish assemblages on shallow reefs are more representative of those across the environmentally extreme southern basin of the Gulf. The unique position of Qatar at the interface between these two divergent environments allows the persistence of quite distinct reef fish and coral communities across a relatively narrow geographic area, enhancing the overall biodiversity of the nation. While these observations are positive and shed further light on the importance of coral reef ecosystems in Qatar, the long-term persistence of these ecosystems and their associated fish communities are in question. Widespread coastal development has resulted in the loss and degradation of many nearshore reefs in Qatar and recent increases in sea surface temperatures have caused recurrent bleaching events that have eliminated much of the remaining shallow coral reefs around the peninsula (Burt et al., 2016; Burt et al., 2017). While the deep reefs have thus far escaped the worst of these impacts, there are indications that changes are underway in these systems as well. Acropora table corals that had reportedly been dominant members of the coral communities at offshore, deep reefs in Qatar, are now exceedingly rare (Foster et al., 2013; Burt et al., 2016; Riegl et al., 2018). Given that such reef-building corals provide important three-dimensional complexity known to be important in supporting fishes (Graham and Nash, 2013), it is likely that the loss of this formerly-dominant species is indirectly impacting the reef-associated fish assemblage. Given the projected increases in sea surface temperatures in the Gulf in the coming decades (Sheppard, 2003), further losses of coral cover and shifts in community structure may result in additional indirect effects on reef fishes. In addition, given that many reef fish and corals are living near their thermal limits in the Gulf (Coles and Tarr, 1990; Price et al., 1993; Feary et al., 2012), it is also likely that further temperature increases may push many of these organisms beyond their physiological capacity and cause demographic changes that would translate to the population and community levels (Pörtner and Farrell, 2008), particularly in extreme shallow reef environments. Development of management efforts aimed at reducing stressors that may exacerbate these climate-related changes are warranted.
The original contributions presented in the study are included in the article/Supplementary Material. Further inquiries can be directed to the corresponding author.
RBH, PR and JAB conceptualized and designed the study. All authors contributed to field work and data collection. JB and JAB analyzed and interpreted the data, and prepared a first draft of the manuscript. All authors reviewed and approved the final version of the manuscript.
This publication was made possible by the NPRP award [NPRP8-952-1-186] from the Qatar National Research Fund (a member of The Qatar Foundation) through the National Priority Research Program. The statements made herein are solely the responsibility of the authors. JAB was supported by funding from grants CG007 and CG009 by Tamkeen, and their support is greatly appreciated. Open access funding is provided by the Qatar National Library to RBH under Fund OAF-7342-QNL.
The authors declare that the research was conducted in the absence of any commercial or financial relationships that could be construed as a potential conflict of interest.
All claims expressed in this article are solely those of the authors and do not necessarily represent those of their affiliated organizations, or those of the publisher, the editors and the reviewers. Any product that may be evaluated in this article, or claim that may be made by its manufacturer, is not guaranteed or endorsed by the publisher.
The Supplementary Material for this article can be found online at: https://www.frontiersin.org/articles/10.3389/fmars.2022.989841/full#supplementary-material
Beijbom O., Edmunds P. J., Kline D. I., Mitchell B. G., Kriegman D. (2012). “Automated annotation of coral reef survey images,” in Proceedings of the 2012 IEEE Conference on Computer Vision and Pattern Recognition (CVPR) (Providence, RI). 1170–1177. doi: 10.1109/CVPR.2012.6247798
Beijbom O., Edmunds P. J., Roelfsema C., Smith J., Kline D. I., Neal B. P., et al. (2015). Towards automated annotation of benthic survey images: variability of human experts and operational modes of automation. PloS One 10 (7), e0130312. doi: 10.1371/journal.pone.0130312
Bell J., Galzin R. (1984). Influence of live coral cover on coral-reef fish communities. Mar. Ecol. Prog. Ser. 15 (3), 265–274. doi: 10.3354/meps015265
Bento R., Hoey A. S., Bauman A. G., Feary D. A., Burt J. A. (2016). The implications of recurrent disturbances within the world’s hottest coral reef. Mar. Pollut. Bull. 105 (2), 466–472. doi: 10.1016/j.marpolbul.2015.10.006
Bouwmeester J., Riera R., Range P., Ben-Hamadou R., Samimi-Namin K., Burt J. (2020). “Coral and reef fish communities in the thermally extreme Persian/Arabian Gulf: insights into potential climate change effects,” in Perspectives on the marine animal forests of the world (Cham, Switzerland: Springer), 63–86.
Brandl S. J., Johansen J. L., Casey J. M., Tornabene L., Morais R. A., Burt J. A. (2020). Extreme environmental conditions reduce coral reef fish biodiversity and productivity. Nat. Commun. 11 (1), 3832. doi: 10.1038/s41467-020-17731-2
Burt J., Al-Harthi S., Al-Cibahy A. (2011a). Long-term impacts of coral bleaching events on the world’s warmest reefs. Mar. Environ. Res. 72 (4), 225–229. doi: 10.1016/j.marenvres.2011.08.005
Burt J. A., Al-Khalifa K., Khalaf E., AlShuwaikh B., Abdulwahab A. (2013a). The continuing decline of coral reefs in Bahrain. Mar. Pollut. Bull. 72 (2), 357–363. doi: 10.1016/j.marpolbul.2012.08.022
Burt J., Bartholomew A., Usseglio P., Bauman A., Sale P. (2009). Are artificial reefs surrogates of natural habitats for corals and fish in Dubai, united Arab Emirates? Coral. Reefs. 28 (3), 663–675. doi: 10.1007/s00338-009-0500-1
Burt J. A., Ben-Hamadou R., Abdel-Moati M. A. R., Fanning L., Kaitibie S., Al-Jamali F., et al. (2017). Improving management of future coastal development in Qatar through ecosystem-based management approaches. Ocean. Coast. Manage. 148, 171–181. doi: 10.1016/j.ocecoaman.2017.08.006
Burt J., Camp E., Enochs I., Johansen J., Morgan K., Riegl B., et al. (2020). Insights from extreme coral reefs in a changing world. Coral. Reefs. 39 (3), 495–507. doi: 10.1007/s00338-020-01966-y
Burt J. A., Feary D. A., Bauman A. G., Usseglio P., Cavalcante G. H., Sale P. F. (2011b). Biogeographic patterns of reef fish community structure in the northeastern Arabian peninsula. ICES. J. Mar. Sci. 68 (9), 1875–1883. doi: 10.1093/icesjms/fsr129
Burt J. A., Feary D. A., Cavalcante G., Bauman A. G., Usseglio P. (2013b). Urban breakwaters as reef fish habitat in the Persian Gulf. Mar. Pollut. Bull. 72 (2), 342–350. doi: 10.1016/j.marpolbul.2012.10.019
Burt J. A., Paparella F., Al-Mansoori N., Al-Mansoori A., Al-Jailani H. (2019). Causes and consequences of the 2017 coral bleaching event in the southern Persian/Arabian Gulf. Coral. Reefs. 38 (4), 567–589. doi: 10.1007/s00338-019-01767-y
Burt J., Range P., Claereboudt M., Al-Mealla R., Salimi P., Salimi M., et al. (2021). “Chapter 4. status and trends of coral reefs of the ROPME Sea area,” in Status of coral reefs of the world: 2020. Eds. Souter D., Planes S., Wicquart J., Logan M., Obura D., Staub F. (Lausanne, Switzerland: Global Coral Reef Monitoring Network & United Nations Environment Programme), 1–13.
Burt J. A., Smith E. G., Warren C., Dupont J. (2016). An assessment of Qatar’s coral communities in a regional context. Mar. Pollut. Bull. 105 (2), 473–479. doi: 10.1016/j.marpolbul.2015.09.025
De Cáceres M., Legendre P. (2009). Associations between species and groups of sites: indices and statistical inference. Ecology 90 (12), 3566–3574. doi: 10.1111/j.1600-0706.2010.18334.x
Cheal A., MacNeil M. A., Cripps E., Emslie M., Jonker M., Schaffelke B., et al. (2010). Coral–macroalgal phase shifts or reef resilience: links with diversity and functional roles of herbivorous fishes on the Great Barrier Reef. Coral. Reefs. 29 (4), 1005–1015. doi: 10.1007/s00338-010-0661-y
Chen Q., Beijbom O., Chan S., Bouwmeester J., Kriegman D. (2021). “A new deep learning engine for CoralNet,” in Proceedings of the 2021 IEEE/CVF International Conference on Computer Vision (ICCV) Workshops. 3693–3702. doi: 10.1109/ICCVW54120.52021.00412
Coles S. L. (2003). Coral species diversity and environmental factors in the Arabian Gulf and the Gulf of Oman: a comparison to the Indo-Pacific region. Atoll. Res. Bull. 507, 1–19. doi: 10.5479/si.00775630.507.1
Coles S. L., Tarr B. A. (1990). Reef fish assemblages in the western Arabian Gulf: a geographically isolated population in an extreme environment. Bull. Mar. Sci. 47 (3), 696–720.
Cornwall C. E., Comeau S., Kornder N. A., Perry C. T., van Hooidonk R., DeCarlo T. M., et al. (2021). Global declines in coral reef calcium carbonate production under ocean acidification and warming. Proc. Natl. Acad. Sci. 118 (21), e2015265118. doi: 10.1073/pnas.2015265118
D’Agostino D., Burt J. A., Reader T., Vaughan G. O., Chapman B. B., Santinelli V., et al. (2020). The influence of thermal extremes on coral reef fish behaviour in the Arabian/Persian Gulf. Coral. Reefs. 39 (3), 733–744. doi: 10.1007/s00338-019-01847-z
Darling E. S., Graham N. A., Januchowski-Hartley F. A., Nash K. L., Pratchett M. S., Wilson S. K. (2017). Relationships between structural complexity, coral traits, and reef fish assemblages. Coral. Reefs. 36 (2), 561–575. doi: 10.1007/s00338-017-1539-z
De Cáceres M., Legendre P., Moretti M. (2010). Improving indicator species analysis by combining groups of sites. Oikos 119 (10), 1674–1684. doi: 10.1111/j.1600-0706.2010.18334.x
DiBattista J. D., Roberts M. B., Bouwmeester J., Bowen B. W., Coker D. J., Lozano-Cortés D. F., et al. (2016). A review of contemporary patterns of endemism for shallow water reef fauna in the Red Sea. J. Biogeogr. 43 (3), 423–439. doi: 10.1111/jbi.12649
Downing N. (1985). “Coral reef communities in an extreme environment: the northwestern Arabian Gulf,” in Proceedings of the 5th International Coral Reef Congress. 343–348.
Eddy T. D., Lam V. W., Reygondeau G., Cisneros-Montemayor A. M., Greer K., Palomares M. L. D., et al. (2021). Global decline in capacity of coral reefs to provide ecosystem services. One Earth 4 (9), 1278–1285. doi: 10.1016/j.oneear.2021.08.016
Fanning L. M., Al-Naimi M. N., Range P., Ali A.-S. M., Bouwmeester J., Al-Jamali F., et al. (2021). Applying the ecosystem services - EBM framework to sustainably manage Qatar’s coral reefs and seagrass beds. Ocean. Coast. Manage. 205, 105566. doi: 10.1016/j.ocecoaman.2021.105566
Feary D., Burt J., Bauman A., Usseglio P., Sale P., Cavalcante G. (2010). Fish communities on the world’s warmest reefs: what can they tell us about the effects of climate change in the future? J. Fish. Biol. 77 (8), 1931–1947. doi: 10.1111/j.1095-8649.2010.02777.x
Feary D. A., Burt J. A., Cavalcante G. H., Bauman A. G. (2012). ““Extreme physical factors and the structure of Gulf fish and reef communities,”,” in Coral reefs of the Gulf (Dordrecht: Springer), 163–170.
Foster K. A., Foster G., Al-Harthi S. (2013). Coral assemblages in the southeastern Arabian Gulf (Qatar and Abu Dhabi, UAE): various stages of acropora recovery a decade after recurrent elevated temperature anomalies. Open J. Mar. Sci. 3 (02), 28. doi: 10.4236/ojms.2013.32A004
Fox J., Weisberg S. (2019). An {R} companion to applied regression, third edition (Sage, Thousand Oaks, CA).
Friedlander A. M., Parrish J. D. (1998). Habitat characteristics affecting fish assemblages on a Hawaiian coral reef. J. Exp. Mar. Biol. Ecol. 224 (1), 1–30. doi: 10.1016/S0022-0981(97)00164-0
Gardiner N. M., Munday P. L., Nilsson G. E. (2010). Counter-gradient variation in respiratory performance of coral reef fishes at elevated temperatures. PloS One 5 (10), e13299. doi: 10.1371/journal.pone.0013299
Garpe K. C., Yahya S. A., Lindahl U., Öhman M. C. (2006). Long-term effects of the 1998 coral bleaching event on reef fish assemblages. Mar. Ecol. Prog. Ser. 315, 237–247. doi: 10.3354/meps315237
Graham N. A., Nash K. L. (2013). The importance of structural complexity in coral reef ecosystems. Coral. Reefs. 32 (2), 315–326. doi: 10.1007/s00338-012-0984-y
Graham N., Wilson S., Pratchett M. S., Polunin N. V., Spalding M. (2009). Coral mortality versus structural collapse as drivers of corallivorous butterflyfish decline. Biodivers. Conserv. 18 (12), 3325–3336. doi: 10.1007/s10531-009-9633-3
Grandcourt E. (2012). “Reef fish and fisheries in the Gulf,” in Coral reefs of the Gulf. Eds. Riegl B., Purkis J. S. (Dordrecht: Springer), 127–161.
Graves S., Piepho H.-P., Selzer L. (2015) multcompView: Visualizations of paired comparisons. R package version 0.1-7. Available at: https://CRAN.R-project.org/package=multcompView.
Green A. L., Bellwood D. R. (2009). Monitoring functional groups of herbivorous reef fishes as indicators of coral reef resilience: a practical guide for coral reef managers in the Asia Pacific region (Gland, Switzerland: IUCN).
Hoey A. S., Berumen M. L., Bonaldo R. M., Burt J. A., Feary D. A., Ferreira C. E., et al. (2018). “The ecology of parrotfishes in marginal reef systems,” in Biology of parrotfishes. Eds. Hoey A. S., Bonaldo R. M. (Boca Raton, FL, USA: CRC Press), 276–301.
Hoey A. S., Feary D. A., Burt J. A., Vaughan G., Pratchett M. S., Berumen M. L. (2016). Regional variation in the structure and function of parrotfishes on Arabian reefs. Mar. Pollut. Bull. 105 (2), 524–531. doi: 10.1016/j.marpolbul.2015.11.035
Hothorn T., Bretz F., Westfall P. (2008). Simultaneous inference in general parametric models. Biom. J. 50 (3), 346–363. doi: 10.1002/bimj.200810425
Hughes T. P., Rodrigues M. J., Bellwood D. R., Ceccarelli D., Hoegh-Guldberg O., McCook L., et al. (2007). Phase shifts, herbivory, and the resilience of coral reefs to climate change. Curr. Biol. 17 (4), 360–365. doi: 10.1016/j.cub.2006.12.049
IPBES (2019). “Summary for policymakers of the global assessment report on biodiversity and ecosystem services of the intergovernmental science-policy platform on biodiversity and ecosystem services”. Eds. Díaz S., Settele J., Brondízio E. S., Ngo H. T., Guèze M., Agard J., Arneth A., Balvanera P., Brauman K. A., Butchart S. H. M., Chan K. M. A., Garibaldi L. A., Ichii K., Liu J., Subramanian S. M., Midgley G. F., Miloslavich P., Molnár Z., Obura D., Pfaff A., Polasky S., Purvis A., Razzaque J., Reyers B., Roy Chowdhury R., Shin Y. J., Visseren-Hamakers I. J., Willis K. J., Zayas C. N. (Bonn, Germany: IPBES Secretariat).
Kardousha M., Al-Muftah A., Al-Khayat J. (2016). Exploring Sheraoh island at south-Eastern Qatar: First distributional records of some inland and offshore biota with annotated checklist. J. Mar. Sci. Res. Dev. 6 (191), 2. doi: 10.4172/2155-9910.1000191
Krupp F., Almarri M. A. (1996). “Fishes and fish assemblages of the Jubail marine wildlife sanctuary” in A marine wilslife sanctuary for the Arabian Gulf. environmental research and conservation following the 1991 Gulf war oil spill. Eds. Krupp F., Abuzinada A. H., Nader L. A. (Frankfurt a.M: NCWCD, Riyadh and Senckenberg Research Institute), 339–350.
Lachkar Z., Mehari M., Al Azhar M., Lévy M., Smith S. (2021). Fast local warming is the main driver of recent deoxygenation in the northern Arabian Sea. Biogeosciences 18 (20), 5831–5849. doi: 10.5194/bg-18-5831-2021
Ledlie M. H., Graham N. A. J., Bythell J. C., Wilson S. K., Jennings S., Polunin N. V. C., et al. (2007). Phase shifts and the role of herbivory in the resilience of coral reefs. Coral. Reefs. 26 (3), 641–653. doi: 10.1007/s00338-007-0230-1
Lenth R. V. (2016). Least-squares means: The R package lsmeans. J. Stat. Software 69 (1), 1–33. doi: 10.18637/jss.v069.i01
Luckhurst B., Luckhurst K. (1978). Analysis of the influence of substrate variables on coral reef fish communities. Mar. Biol. 49 (4), 317–323. doi: 10.1007/BF00455026
Maghsoudlou A., Araghi P. E., Wilson S., Taylor O., Medio D. (2008). “Status of coral reefs in the ROPME sea area (The Persian Gulf, Gulf of Oman, and Arabian Sea),” in Status of coral reefs of the world: 2008 (Townsville, Australia: Global Coral Reef Monitoring Network and Reef and Rainforest Research Center).
Mangiafico S. (2019) Rcompanion: Functions to support extension education program evaluation. R package version 2.3.7. Available at: https://CRAN.R-project.org/package=rcompanion.
McCain J., Tarr A., Carpenter K., Coles S. (1984). “Marine ecology of Saudi Arabia: a survey of coral reefs and reef fishes in the northern area, Arabian Gulf, Saudi Arabia,” in Fauna of Saudi Arabia, 102–126.
McCook L., Jompa J., Diaz-Pulido G. (2001). Competition between corals and algae on coral reefs: a review of evidence and mechanisms. Coral. Reefs. 19 (4), 400–417. doi: 10.1007/s003380000129
McCune B., Grace J. (2002). “Nonmetric-multidimensional scaling,” in Analysis of ecological communities (Gleneden Beach, OR, USA: MjM Software Design), 125–142.
Morrison T. H., Hughes T. P., Adger W. N., Brown K., Barnett J., Lemos M. C. (2019). Save reefs to rescue all ecosystems. Nature 573, 333–336. doi: 10.1038/d41586-019-02737-8
Nilsson G. E., Crawley N., Lunde I. G., Munday P. L. (2009). Elevated temperature reduces the respiratory scope of coral reef fishes. Glob. Change Biol. 15 (6), 1405–1412. doi: 10.1111/j.1365-2486.2008.01767.x
Obura D., Gudka M., Samoilys M., Osuka K., Mbugua J., Keith D. A., et al. (2022). Vulnerability to collapse of coral reef ecosystems in the Western Indian ocean. Nat. Sustain. 5 (2), 104–113. doi: 10.1038/s41893-021-00817-0
Ogle D., Wheeler P., Dinno A. (2019) FSA: Fisheries stock analysis. R package version 0.8. 25. Available at: https://github.com/droglenc/FSA.
Oksanen J., Blanchet F. G., Kindt R., Legendre P., Minchin P. R., O’Hara R., et al. (2015). Package ‘vegan’. community ecology package, version, 2.2-1. Available online at: https://CRAN.R-project.org/package=vegan
Pebesma E. (2018). Simple features for R: standardized support for spatial vector data. R. J. 10 (1), 439–446. doi: 10.32614/RJ-2018-009
Pinheiro J., Bates D., DebRoy S., Sarkar D., Team R. C. (2019) Nlme: Linear and nonlinear mixed effects models. R package version 3.1-140. Available at: https://CRAN.R-project.org/package=nlme.
Pörtner H. O., Farrell A. P. (2008). Physiology and climate change. Science 322 (5902), 690–692. doi: 10.1126/science.1163156
Price A., Sheppard C., Roberts C. (1993). The Gulf: its biological setting. Mar. Pollut. Bull. 27, 9–15. doi: 10.1016/0025-326X(93)90004-4
Rakib F., Al-Ansari E. M. A. S., Husrevoglu Y. S., Yigiterhan O., Al-Maslamani I., Aboobacker V. M., et al. (2021). Observed variability in physical and biogeochemical parameters in the central Arabian Gulf. Oceanologia 63 (2), 227–237. doi: 10.1016/j.oceano.2020.12.003
R Core Team (2019). “R: A language and environment for statistical computing” (Vienna, Austria: R Foundation for Statistical Computing). Available at: https://www.R-project.org.
Rezai H., Savari A. (2004). Observation on reef fishes in the coastal waters off some Iranian islands in the Persian Gulf. Zool. Middle. East. 31 (1), 67–76. doi: 10.1080/09397140.2004.10638024
Rezai H., Wilson S., Claereboudt M., Riegl B. (2004). “Coral reef status in the ROPME sea area: Arabian/Persian Gulf, Gulf of Oman and Arabian Sea,“ in. of Coral Reefs of the World. Ed Wilkinson C. (AIMS, Townsville, QLD), 1, 155–170.
Richer R. (2008). “Conservation in Qatar: impacts of increasing industrialization” (Georgetown University School of Foreign Service in Qatar).
Riegl B., Johnston M., Purkis S., Howells E., Burt J., Steiner S. C., et al. (2018). Population collapse dynamics in Acropora downingi, an Arabian/Persian Gulf ecosystem-engineering coral, linked to rising temperature. Glob. Change Biol. 24 (6), 2447–2462. doi: 10.1111/gcb.14114
Riegl B. M., Purkis S. J. (2009). Model of coral population response to accelerated bleaching and mass mortality in a changing climate. Ecol. Modell. 220 (2), 192–208. doi: 10.1016/j.ecolmodel.2008.09.022
Riegl B., Purkis S. (2015). Coral population dynamics across consecutive mass mortality events. Glob. Change Biol. 21 (11), 3995–4005. doi: 10.1111/gcb.13014
Riegl B., Purkis S. J., Al-Cibahy A. S. (2013). “Climate change disrupts long-term community stability and adaptation in Persian/Arabian Gulf.
Sale P., Sharp B. (1983). Correction for bias in visual transect censuses of coral reef fishes. Coral. Reefs. 2 (1), 37–42. doi: 10.1007/BF00304730
Sheppard C. (1988). “Similar trends, different causes: Responses of corals to stressed environments in Arabian seas,” in Proc. 6th Int. Coral Reef Symp. (Townsville, QLD) 3, 297–302.
Sheppard C. R. (2003). Predicted recurrences of mass coral mortality in the Indian ocean. Nature 425 (6955), 294–297.
Sheppard C. (2016). Coral reefs in the Gulf are mostly dead now, but can we do anything about it? Mar. Pollut. Bull. 105 (2), 593–598. doi: 10.1016/j.marpolbul.2015.09.031
Sheppard C., Al-Husiani M., Al-Jamali F., Al-Yamani F., Baldwin R., Bishop J., et al. (2010). The Gulf: A young sea in decline. Mar. pollut. Bull. 60 (1), 13–38. doi: 10.1016/j.marpolbul.2009.10.017
Sheppard C., Price A., Roberts C. (1992). Marine ecology of the Arabian region: patterns and processes in extreme tropical environments (Toronto, Canada: Academic Press), 359 p.
Sheppard C. R. C., Sheppard A. L. S. (1991). “Corals and coral communities of Arabia, volume 12,” in Fauna of Saudi Arabia, 3–170.
Shinn E. A. (1973). “Recent intertidal and nearshore carbonate sedimentation around rock highs, e quatar, Persian Gulf,” in The Persian Gulf. Ed. Purser B. H. (Berlin, Heidelberg: Springer).
Shinn E. A. (1976). Coral reef recovery in Florida and the Persian Gulf. Environ. Geol. 1 (4), 241. doi: 10.1007/bf02407510
Shraim R., Dieng M. M., Vinu M., Vaughan G., McParland D., Idaghdour Y., et al. (2017). Environmental extremes are associated with dietary patterns in Arabian Gulf reef fishes. Front. Mar. Sci. 4, 285. doi: 10.3389/fmars.2017.00285
Smith G. B., Saleh M. A. (1987). Abundance and bathymetric distribution of Bahrain (Arabian Gulf) reef ichthyofaunas. Estuar. Coast. Shelf. Sci. 24 (3), 425–431. doi: 10.1016/0272-7714(87)90061-8
Souter D., Serge P., Wicquart J., Logan M., Obura D., Staub F. (2021). Status of coral reefs of the world: 2020 executive summary, (Townsville, Australia: Coral Reef Initiative, Global Coral Reef Monitoring Network).
South A. (2017) Rnaturalearth: World map data from natural earth. R package version 0.1.0. Available at: https://CRAN.R-project.org/package=rnaturalearth.
Vaughan G., Al-Mansoori N., Burt J. (2019). “The Arabian Gulf,” in World seas: An environmental evaluation, second edition. Ed. Sheppard. C. (Amsterdam, NL: Elsevier Science), 1–23.
Vaughan G. O., Shiels H. A., Burt J. A. (2021). Seasonal variation in reef fish assemblages in the environmentally extreme southern Persian/Arabian Gulf. Coral. Reefs. 40 (2), 405–416. doi: 10.1007/s00338-020-02041-2
Warren C., Dupont J., Abdel-Moati M., Hobeichi S., Palandro D., Purkis S. (2016). Remote sensing of Qatar nearshore habitats with perspectives for coastal management. Mar. Pollut. Bull. 105 (2), 641–653. doi: 10.1016/j.marpolbul.2015.11.036
Keywords: Scleractinia, climate change, thermal adaptation, coral communities, fish communities, Persian Gulf, Arabian Gulf
Citation: Bouwmeester J, Ben-Hamadou R, Range P, Al Jamali F and Burt JA (2022) Spatial patterns of reef fishes and corals in the thermally extreme waters of Qatar. Front. Mar. Sci. 9:989841. doi: 10.3389/fmars.2022.989841
Received: 08 July 2022; Accepted: 06 September 2022;
Published: 26 September 2022.
Edited by:
Michelle Jillian Devlin, Fisheries and Aquaculture Science (CEFAS), United KingdomReviewed by:
Parviz Tavakoli-Kolour, University of the Ryukyus, JapanCopyright © 2022 Bouwmeester, Ben-Hamadou, Range, Al Jamali and Burt. This is an open-access article distributed under the terms of the Creative Commons Attribution License (CC BY). The use, distribution or reproduction in other forums is permitted, provided the original author(s) and the copyright owner(s) are credited and that the original publication in this journal is cited, in accordance with accepted academic practice. No use, distribution or reproduction is permitted which does not comply with these terms.
*Correspondence: Radhouane Ben-Hamadou, YmVuaGFtYWRvdUBxdS5lZHUucWE=
Disclaimer: All claims expressed in this article are solely those of the authors and do not necessarily represent those of their affiliated organizations, or those of the publisher, the editors and the reviewers. Any product that may be evaluated in this article or claim that may be made by its manufacturer is not guaranteed or endorsed by the publisher.
Research integrity at Frontiers
Learn more about the work of our research integrity team to safeguard the quality of each article we publish.