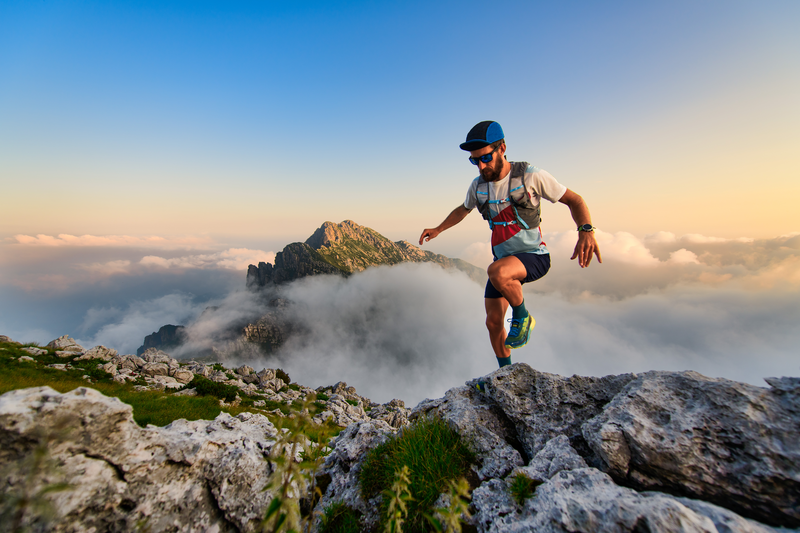
95% of researchers rate our articles as excellent or good
Learn more about the work of our research integrity team to safeguard the quality of each article we publish.
Find out more
ORIGINAL RESEARCH article
Front. Mar. Sci. , 28 October 2022
Sec. Marine Megafauna
Volume 9 - 2022 | https://doi.org/10.3389/fmars.2022.989025
Satellite telemetry tags, used to monitor the migratory behavior of cetaceans, have the potential to be a vehicle for infection due to their invasive nature. Antibiotic coatings have been previously employed to reduce the chances of infection via the formation of a stable biofilm on the surface of the tags. However, increased use of antibiotics has the potential to lead to the development of antibiotic-resistant pathogens. To prevent the formation of antibiotic-resistant pathogens, a polydopamine surface coating that, when exposed to oxygen, releases low doses (~40-100µM) of hydrogen peroxide over a prolonged period (>24 hours) can be used to replace current antibiotic coatings used in the field. These pDA coatings can reduce bacterial adhesion from model bacteria from the two most common genotypes found on the skin of cetaceans (Psychrobacter and Tenacibaculum). The adhesion of Psychrobacter bacteria was reduced by 80% (p<0.01) while Tenacibaculum was reduced by 70% (p<0.001). When the bacteria were dosed with a non-lethal quantity of hydrogen peroxide (200µM) prior to being exposed to pDA surface coatings, there was no decrease in the efficacy of the coatings. This indicates a resistance to hydrogen peroxide will not be formed quickly. Overall, the polydopamine surface coatings were able to reduce the adhesion of model bacteria strains on the surface of medical grade stainless steel, which could increase the functional tag service life while reducing the chances of infection.
Monitoring behavioral patterns of marine mammals is a vital component in the ongoing study of general ocean health and the ecology of individual populations. Currently, satellite telemetry tags and biopsy samples are used to monitor migration patterns and animal health status respectively. Telemetry tags and biopsy tips are, most commonly, made from medical grade stainless steel (316L) (Lagerquist et al., 2008; Balmer et al., 2014) or titanium (Andrews et al., 2008). Biopsy tips are used to gather tissue samples which are analyzed to determine the animals’ health status and tissue composition. These results can shed light on the current pollutants in the ocean as most marine mammals store pollutants in their blubber tissue. Biopsy samples are commonly used for sexing but are also used to run genetic analysis to determine potential population interbreeding through DNA sequencing. Satellite telemetry tags have been used to monitor migration and diving behavior of large marine mammals. Cetacean migration behavior has been monitored using transdermal tags which penetrate through the skin implant into the underlying blubber tissue. While these are useful tools to track movement, they can act as a vehicle for infection. Both these devices, telemetry tags and biopsy tips, penetrate through exterior dermal layer and into the underlying blubber tissue (Andrews et al., 2019). Biopsy tips are less likely to cause infection as they are not meant to remain implanted. However, telemetry tags are used to monitor the migration and behavior patterns of large cetaceans for prolonged periods of time (>2 weeks) which can allow time for bacteria present in the microbiome to attach and proliferate on the tag surface.
The microbiome located on the surface of the skin in cetaceans is complex and vital to individual health and well-being (Nelson et al., 2015; Ross et al., 2019). Cetacean microbiomes change year-round and vary geographically (e.g., they differ in feeding and breeding habitats of migratory whales) (Bierlich et al., 2018). However, there are two main genera of bacteria that make up a majority of the microbiome on cetaceans year-round: Psychrobacter and Tenacibaculum (Apprill et al., 2014; Bierlich et al., 2018). Studies of the impact the microbiome has on the general mammalian health have found that these bacteria, when on the surface of the skin are harmless or beneficial to the individual (Nelson et al., 2015). However, if the bacteria were to get into the underlying tissue, the potential for infection would increase and could lead to a rise in morbidity and mortality rates. Thus, objects like satellite telemetry tags that penetrate the skin over extended periods provide a vehicle for bacteria to attach and proliferate, potentially leading to biofilm formation and subsequent infection.
To prevent biofilm formation and subsequent tissue infection, antibiotic-based surface coatings can be used in certain invasive instruments (Mate et al., 2007; IWC, 2020).The primary ways that they prevent bacterial infections include permeabilizing the cell wall as well as DNA, disrupting protein synthesis, or disrupting cell wall synthesis. These coatings are primarily comprised of the antibiotic’s gentamicin or vancomycin. Gentamicin is used against gram-negative bacteria and is used to prevent protein synthesis within the bacteria cells, ultimately, causing cell death (Hahn and Sarre, 1969). Vancomycin can be used on gram-positive bacteria cells to disrupt cell wall synthesis, causing unwanted pores and subsequent cell death (Watanakunakorn, 1984). These antibiotics, while effective, are subject to resistance formed within a variety of bacterial species (Shakil et al., 2008). The most common bacterial species to form a resistance to both Vancomycin and Gentamicin is Staphylococcus (Livermore, 2000) and can be attributed to overuse. Therefore, there has been growing interest in developing non-antibiotic-based antimicrobial solutions. Recently, the scientific community has discouraged the use of antibiotics in cetaceans in favor of sterilization of instruments or, if necessary, implementation of a non-antibiotic-based antimicrobial system. Some of these antimicrobial solutions include the use of reactive oxygen species (ROS) (Fang, 2011; Vatansever et al., 2013) and other antimicrobial agents such as silver ions (Ag+) (Pal et al., 2007; Jung et al., 2008). These methods interrupt bacterial growth through permeabilizing the cell wall and damaging the cell’s DNA (Linley et al., 2012). ROS can be found in the natural wound environment and not only reduces the chances of infection by creating a concentration gradient (Yoo and Huttenlocher, 2009; Zhu et al., 2017), but also acts as a mediator in the wound healing cascade (Ojha et al., 2008; Li et al., 2016). The chances of a resistance being formed from the use of ROS are low when compared to the chance with use of antibiotics due to the necessity of these molecules in cellular signaling pathways. However, due to the inherent instability of many ROS, it is difficult to recreate the natural release profile and subsequent gradient in vitro (Madanská et al., 2004).
Polydopamine (pDA), a well-known marine adhesive derived from mussel foot proteins, has previously been used as an antimicrobial agent to prevent bacterial and viral proliferation (Su et al., 2016; Mude et al., 2021). The antimicrobial capability as well as the adhesive character of pDA is derived from the abundance of catechol present on the monomer structure. When catechol is oxidized or forms a bond to a surface – either organic or inorganic – a hydroxy radical (OH˙) will form and rapidly oxidize into the ROS hydrogen peroxide (H2O2) (Liu et al., 2014) (See Figure 1). The ability for pDA surface coatings to generate H2O2 is now being explored as a tool to address wound healing and infection more directly. To improve adhesive and cohesive character of pDA surface coatings, studies have explored how to deposit thicker coatings onto various substrates by altering coating conditions such as pH and coating time (CT) (Zangmeister et al., 2013; Terrill, 2015). It is thought that thicker pDA coatings will increase the quantity of cohesive bonds and strengthen the adhesive character of the coating however, it is unknown how the thickness will impact the release behavior. It has been found that the generation from catechol containing microgels and pDA surface coatings on metallic substrates takes place over a prolonged period (>24 hours).
Figure 1 Schematic representation of deposition of pDA coatings onto the surface of a satellite telemetry tag. (A) An uncoated tag is placed into a dopamine-HCl solution for 24 hours and (B) a conformational pDA coating, which appears brown in color, is formed. (C) When exposed to oxygen, the catechol on unpolymerized dopamine molecules will oxidize and form the reactive oxygen species hydrogen peroxide.
As infection is a concern for the metallic satellite telemetry tags used in cetacean conservation practices, the ability to reduce bacterial adhesion to a metallic substrate without a pronounced chance of resistance being formed is a growing area of interest. This concern has led to the preventative use of antibiotic coatings on the surface of implantable devices (e.g., Mate et al., 2007). However, with increasing use of antibiotics, there comes an increasing concern regarding antibiotic resistant pathogens within cetacean populations (IWC, 2020). Currently, there are no documented accounts of antibiotic resistant pathogens developing as a result of antibiotic use on the surface of satellite telemetry tags, however, the potential has driven an interest in non-antibiotic-based approaches to prevent infection. Polydopamine surface coatings can act in place of antibiotic coatings to prevent bacterial adhesion and colonization while minimizing the chances of resistance being formed. In this work, we first address the ability for pDA surface coatings to act as a vehicle for local controlled delivery of H2O2 to prevent the adhesion of prevalent bacterial types associated with infection, some terrestrial and some aquatic, to the surface of medical grade stainless steel. Secondly, we evaluate the potential for a resistance to form in response to a previous non-lethal exposure to H2O2 from an external source. As H2O2 is known to be present in the wound environment, bacteria may be exposed to H2O2 multiple times and subsequently form a resistance to the ROS. Ultimately, the goal of this work is to develop a non-antibiotic-based pDA surface coating which can be applied to invasive instruments commonly used to monitor cetacean populations, such as consolidated satellite telemetry tags (Figure 1).
316L stainless steel coupons (10mm x 20mm rectangular samples) with varying surface roughness [Standard roughness average (Ra) = 0.635µm, Brushed Ra = 0.813µm, Mirrored Ra = 0.15µm] were used and coated using previously established methods (Tyo et al., 2019). The varying surface finishes of the coupons were chosen due to patterning and polishing techniques used on satellite telemetry tags to determine the depth of penetration and general finishing techniques used to sharpen the tip. Briefly, coupons were exposed to a 2mg/mL dopamine-HCl (Sigma-Aldrich, St. Louis MO) solution in a Tris-HCl buffer (Sigma-Aldrich, St. Louis MO) of pH 7.4 at room temperature (21°C) or 8.5 at 35°C for 24 hours. After coating, coupons were removed and gently shaken in PBS (Sigma-Aldrich, St. Louis MO) (pH 7.4) to remove any non-adherent pDA from the surface. Coupons that were not used immediately after coating were set to air dry in a nitrogen-rich environment until use. Coatings created using a pH 7.4 solution at room temperature have previously demonstrated a release profile that resulted in a solution with a concentration of H2O2 of approximately 40µM after 24 hours. These coatings are referred to as our Low Release coatings. The coatings created using a pH 8.5 solution at body temperature had twice the release as the Low Release coatings (~80µM) and are referred to as our High Release coatings.
As the release of H2O2 from the surface coatings is dependent on the polymerization of pDA, the degree of polymerization of the pDA coatings was evaluated. Coupons with a standard surface finish were coated using the previously described protocol and the quantity of hydroxyl (OH) groups was evaluated using X-ray photon spectroscopy (XPS). C1s and O1s photoelectrons were evaluated at a random location on each coating and analyzed using CasaXPS® software using a 70% Gaussian, 30% Lorenztian peak fit. The quantity of each bond type was determined using the intensity of the peaks. A value of 533eV was used to determine OH groups from the O1 peaks while oxidized catechol were determined to be at a binding energy of 531.5-532eV within the O1 peaks. To compare the release from the coatings with the degree of polymerization, a ferrous oxidative (FOX) assay (Sigma-Aldrich, St. Louis MO) was performed on coupons coated at the same time as those used for XPS analysis. A FOX assay is used to measure the quantity of H2O2 in solution through a colorimetric change. This colorimetric change occurs via the initial oxidation of Fe2+ to Fe3+ after which, xylenol orange will be oxidized and a complex which has an absorption around 560nm will be produced (Wolff, 1994). This product is then measured using a plate reader and used as an indicator of H2O2 content.
The addition of pDA to a surface has been well established to increase surface hydrophilicity. Thus, the impact on surface hydrophilicity with the addition of our pDA surface coatings was evaluated. First, medical-grade stainless steel coupons of varying surface finishes were coated with our Low Release and High Release pDA coatings. Next, the coatings were allowed to air dry, and the contact angle was evaluated using a goniometer (Ossila Contact Angle Goniometer). A drop of diH2O (5µL) was placed onto the surface from a height of 10mm. The drop was allowed to equilibrate, and an image was taken; the contact angle was then recorded using both sides of the drop. Measurements from 0-90° delineate wetting, hydrophilic surfaces, while measurements greater than 90° distinguish a non-wetting hydrophobic surface.
The thickness of the pDA coatings was evaluated previously with XPS analysis (see Tyo et al., 2019 for those results). In this study, AFM was attempted to evaluate for thickness of the coatings; however, it was found that dip coating to prevent coating deposition on half the stainless-steel coupon resulted in uneven coating thickness. The results from the preliminary AFM experiments can be seen in Supplemental Figures 1, 2.
The dosing concentration of H2O2 required to induce a bacteriostatic response in Escherichia coli (E. coli) (ATCC BAA-2471), Staphylococcus epidermidis (S. epi) (ATCC 12228), and Tenacibaculum skagerrakense (T. skag) (ATCC BAA-459) was determined prior to the evaluation of bacterial adhesion. The dose required to induce bacteriostatic behavior for 12 hours was determined by incubating cell suspensions (1.3x105 CFU/cm2) in their respective growth media determined by the manufacturer with different initial doses of H2O2 over at least 24 hours. E. coli and S. epi were incubated at 37°C in a tryptic soy broth (TSB) solution while T. skag was incubated at room temperature (21°C) in a marine broth (ATCC, Manassas VA). The turbidity of the solutions was evaluated at using absorbance readings of optical density (OD) at 600nm (OD600). The absorbance readings were taken using UV-Vis turbidity measurements to determine cell concentration. These concentrations were compared to controls to determine the length of bacteriostatic effects in response to the various doses. The threshold for bacteriostatic behavior was determined to be a turbidity measurement below 0.2.
Stainless steel coupons, with varying surface roughness, were coated using the Low Release and High Release pDA surface coatings and were incubated in bacterial cell suspensions (105 CFU/mL) for one-third of the bacterial growth period. The four bacterium types used were Psychrobacter cryohalolentis (P. cryo) (ATCC BAA-1226), T. skag, S. epi, and E. coli. Coupons, coated and uncoated, were incubated in P. cryo at room temperature (21°C) for 24 hours, T. skag at room temperature for 20 hours, S. epi at 37°C for 24 hours, and E. coli at 37°C for 6 hours. P. cryo and T. skag were used as a model for the bacteria present in the microbiome on healthy whale skin. Both belong to the two most abundant genera found on the surface of healthy whale skin. S. epi was used to evaluate the reaction of a gram-positive bacteria to pDA surface coatings while E. coli was used to evaluate the response of more substrate sensitive pathogens.
After incubating samples in the bacterial suspensions, coupons were removed and rinsed in diH2O to remove any non-adherent bacteria. Bacteria were then fixed to the surface using a 4% glutaraldehyde solution. Once removed, coupons were serially dehydrated in ethanol before imaging using a field-emission scanning electron microscope (FESEM). Bacteria counts were conducted, and the coated coupons were compared to the uncoated coupons.
There is a concern for a resistance, or tolerance, to develop after exposure to a non-lethal bolus dose of a ROS. To evaluate this potential, bacterial suspensions (105 CFU/mL) were exposed to a 200µM dose of H2O2 for 24 hours. After the exposure time, the bacteria were seeded on an agar plate and were grown until individual bacterial colonies were observed: E. coli and S. epi were grown for 24 hours, P. cryo for 72 hours, and T. skag for 60 hours. These colonies were then used to create new bacterial suspensions (105 CFU/mL) and adhesion was evaluated on coated and uncoated standard finished stainless steel coupons as described in section 2.4.
All tests were performed in triplicate unless otherwise specified and statistical analyses were run using a one-way analysis of variance (ANOVA) with Tukey’s HSD analysis and Student’s t-test for comparing means of multiple groups and two groups, respectively, with a p-value less than or equal to 0.05 considered significant (JMP Pro 15 and MS Excel).
After the pDA coatings were synthesized, they were characterized using a broad spectrum XPS scan (Figure 2). The O1 peaks were used to determine the relative quantity of OH side groups which corresponds to the degree of polymerization (Zangmeister et al., 2013). Coatings that had a higher quantity of OH groups indicated a lower degree of polymerization while those with lower quantities of OH groups indicated a higher level of polymerization. It was observed that the pDA coatings that release a larger quantity of H2O2 (High Release) had a lower degree of polymerization when compared to the pDA coatings that released a smaller quantity of H2O2 (Low Release) (XPS intensity measurements: 60202 vs 33698).
Figure 2 Quantity of C-O bonds on the surface of High Release and Low Release pDA coupons with their corresponding H2O2 release. Coupons were coated using the High Release (pH 8.5 at 35°C) and Low Release (pH 7.4 at 21°C) coating conditions and were subsequently analyzed for release and quantity of C-O bonds. (A-C) XPS results of O1s. There was a higher concentration of C-O bonds on the high coated coupons when compared to the low coated coupons. (D) Release evaluated from coupons. The higher concentration of C-O bonds corresponds with a higher concentration of H2O2 in solution after 24 hours. A * indicates a significant difference (p<0.05).
Without the addition of pDA surface coatings, the surface of medical-grade stainless steel was evaluated to be hydrophobic (θ = 103°). When the Low Release and High Release pDA surface coatings were added to the surface of standard stainless steel, the contact angle became significantly more hydrophilic (θ = 30° and 25°, respectively) (Figure 3). On both the brushed and mirrored surface, when the pDA coatings were applied, the surfaces became hydrophilic and complete wetting was observed.
Figure 3 Contact angle using diH2O on standard and brushed SS with and without pDA surface coatings. (A) When a drop of diH2O was places on the surface of an uncoated piece of SS with a standard surface finish the angle made between the drop and surface was approximately 103°. When the pDA coating was applied, the surface became significantly more hydrophilic (θ < 30°, p<0.05). There was no significant difference in hydrophilicity between the pDA coatings. (B) There was no significant difference in contact angle with underlying substrate roughness changed for the uncoated SS surfaces. However, when the pDA surface coatings were applied to either a brushed or mirrored surface there was significantly more spreading (p<0.05). The contact angle was not able to be measured as complete spreading was observed on these surfaces.
Turbidity measurements were taken at various timepoints up to 48 hours after being exposed to a single bolus dose of H2O2. The quantity of H2O2 required to induce a bacteriostatic response for up to 12 hours was determined and used to evaluate how sensitive each bacteria type is to H2O2. Table 1 summarizes the doses required to induce bacteriostatic conditions in the four species of bacteria evaluated. S. epi was the most sensitive bacteria, responding with a dose as low as 50µM. E. coli and P. cryo displayed a bacteriostatic response with the addition of a 100µM dose. T. skag was the most robust bacteria, requiring a dose of at least 150µM to display bacteriostatic behavior. The growth curves generated from these experiments can be found in Supplemental Figures 3-5.
Table 1 The minimum quantity of H2O2 required to induce a bacteriostatic response for 12 hours in the bacterium types tested for adhesion to 316L SS coupons.
Previously published results found that the Low Release pDA coatings significantly reduced the adhesion of E. coli on the standard surface finish (70% reduction) while the adhesion was increased on the brushed surface with the addition of the Low Release pDA coating (25% increase) (Figure 4). There was no statistical difference in adhesion on the mirrored surface with the addition of the Low Release pDA coating. However, when E. coli were exposed to the High Release pDA coating, there was a significant reduction in adhesion observed – at least 60% from each respective uncoated surface control – for all surface finishes (p<0.05) (Figure 4). There was a baseline increase in adhesion observed when the surface roughness was increased (roughly a 3-fold increase) (Figure 4), however, this result is consistent with other findings and is a well-known response of E. coli (Ortega et al., 2010). The impact of surface finish was observed on uncoated surfaces as well as Low Release and High Release pDA surface coatings (Supplemental Figure 6).
Figure 4 Adhesion of E. coli to the surface of 316L SS coupons. Coupons were coated using both the Low Release (pH 7.4, 21°C) and High Release (pH 8.5, 35°C) coating conditions. The coupons were then incubated for 6 hours in a solution of E. coli and the adhesion was evaluated. The adhesion of E. coli on Low Release coatings was significantly reduced on the standard surface finish. The adhesion was significantly inhibited by the High Release surface coatings on all surface finishes. An asterisk indicates a significant difference from the respective uncoated surface (* p<0.05** p<0.01). An analysis of any significant differences between surfaces can be found in Supplemental Figure 6.
The adhesion of S. epi bacteria to the surface of uncoated and Low Release pDA coatings was not statistically different on the standard and brushed surface finishes. On the mirrored surface finish, adhesion was significantly reduced (60% reduction when compared to an uncoated mirrored surface) (p<0.05) (Figure 5). The adhesion between all surface finishes was not statistically different without the pDA coating, however, when pDA surface coatings were added there was a significant reduction in adhesion observed on the mirrored surface finish when compared to the standard and brushed surface finishes (Supplemental Figure 7). The High Release pDA surface coating on the standard and brushed surface finishes did not reduce bacterial adhesion, instead, S. epi adhesion was increased (p<0.05) (Figure 5). The increase with the addition of the High Release pDA compared to the uncoated surface was not observed for the mirrored surface finish, rather, a decrease was still observed when compared to the uncoated surface (25% reduction) (p<0.05) (Figure 5).
Figure 5 Adhesion of S. epi to the surface of 316 SS coupons. Coupons were coated using both the Low Release (pH 7.4, 21°C) and High Release (pH 8.5, 35°C) coating conditions. The coupons were then incubated for 24 hours in a solution of S. epi and the adhesion was evaluated. The adhesion of S. epi on Low Release coatings was not significantly reduced on standard and brushed surfaces but was significantly inhibited on the mirrored surface. Bacterial adhesion was significantly increased on all High Release coatings when compared to the low coated coupons, however on the mirrored surface the high coated pDA coupons still exhibited significantly less adhesion when compared to the corresponding uncoated coupons. (* p<0.05** p<0.01*** p<0.001). An analysis of any significant differences between surfaces can be found in Supplemental Figure 7.
Low Release pDA coatings did not reduce the adhesion of P. cryo on standard and mirrored surface finishes but did significantly reduce the adhesion on a brushed surface (34% reduction) (p<0.05) (Figure 6). The High Release pDA coatings inhibited bacterial adhesion on all surface finishes when compared to the respective uncoated surface (>30% reduction on all surfaces) (Figure 6). Bacterial adhesion was reduced by 80% on the standard surface finish, 66% on the brushed surface finish, and 30% on the mirrored surface with the addition of the High Release pDA surface coatings (Figure 6). Of the tested surface finishes, the mirrored surface finish was the least effective at reducing bacterial adhesion when the High Release pDA coating was added (Supplemental Figure 8).
Figure 6 Adhesion of P. cryo to the surface of 316 SS coupons. Coupons were coated using both the Low Release (pH 7.4, 21°C) and High Release (pH 8.5, 35°C) coating conditions. The coupons were then incubated for 24 hours in a solution of P. cryo, and the adhesion was evaluated. The adhesion of P. cryo on Low Release coatings was not significantly reduced for the standard and mirrored surface types but was significantly reduced on the brushed surface. The adhesion was significantly inhibited on all High Release coatings. A * indicates a significant difference from the respective uncoated surface (* p<0.05** p<0.01). An analysis of any significant differences between surfaces can be found in Supplemental Figure 8.
Stainless steel coupons coated with Low Release and High Release pDA coatings were both observed to significantly decrease the overall bacterial adhesion of T. Skag bacteria (Figure 7). When the underlying surface finish was changed from the standard surface finish, there was an increase in adhesion for brushed and mirrored surfaces with Low Release pDA coatings (Supplemental Figure 9). With the High Release pDA coating, there was a significant reduction on the mirrored surfaces (approximately a 40% reduction) when compared to both the standard and brushed surface finishes (Supplemental Figure 9). However, these adhered bacteria exhibit different morphology between the stagnant growth phase (round/globular) and exponential growth (rod like/spindly) (Supplemental Figure 10) (Frette et al., 2004). When comparing the morphology of the adherent bacteria, it was found that the High Release pDA surface coating both decreased the total adherent bacteria (Figure 7) and increased the proportion of adherent bacteria that were in the stagnant growth phase. All bacteria on the surface of uncoated stainless steel – regardless of surface finish – were in the exponential growth phase.
Figure 7 Adhesion of T. skag to the surface of 316 SS coupons. Coupons were coated using both the Low Release (pH 7.4, 21°C) and High Release (pH 8.5, 35°C) coating conditions. The coupons were then incubated for 20 hours in a solution of T. skag and the adhesion was evaluated. It was found that the addition of pDA surface coatings significantly reduced bacterial adhesion when compared to the uncoated surface. There was a significant difference between the low coated and high coated pDA coatings on the standard and mirrored surface, however, there was no difference in bacterial adhesion on the brushed surface between the coatings. A * indicates a significant difference from the respective uncoated surface (* p<0.05** p<0.01*** p<0.01). A # indicates an individual significant difference (# p<0.05## p<0.01). An analysis of any significant differences between surfaces can be found in Supplemental Figure 8.
After exposure to a non-lethal dose of H2O2, there was no significant difference observed between the adhesion of E. coli on the surface of High Release pDA coatings when compared to E. coli that were not previously exposed to a high dose of H2O2 (Figure 8A). However, there was still a significant decrease in the adhesion of E. coli to the surface of the standard surface finish stainless steel from the uncoated surface to the coated surfaces regardless of previous exposure to H2O2.
Figure 8 The effect of pre-treating various bacterium types with 200µM of hydrogen peroxide prior to evaluating adhesion on pDA coated surfaces. Stainless steel coupons with a standard surface finish with and without the High Release pDA coating were exposed to bacteria that either were never exposed to a non-lethal dose of hydrogen peroxide or were pre-treated with a bolus dose of 200µM for 24 hours before being propagated. On all bacteria tested, no resistance was seen (i.e., there was no increase in adhesion on the pDA coated coupons when pre-treated bacteria was compared to the non-treated bacteria). (A), E. coli saw no significant decrease when compared to the non-treated pDA coated coupons, but the adhesion was significantly inhibited compared to the uncoated surface. (B) S. epi saw an increase in adhesion from the non-treated bacteria with the addition of the pDA coating however, pre-treated bacteria were less likely to adhere to the pDA coating. (C) P. cryo bacteria saw an additional decrease in bacterial adhesion on pDA coated coupons when compared to both the uncoated and coated surface. (D) T. skag saw similar results to that of E. coli where there was no resistance seen after treatment but also no additional susceptibility to the pDA coating. A * indicates a significant difference from the uncoated control (*p<0.05**p<0.01***p<0.001), while a ### indicates an individual significant difference (p<0.001).
As previously described, on a standard surface finish (control) there was a significant increase in the quantity of S. epi adhered to the stainless steel with the addition of a High Release pDA coating (Figure 5). However, after S. epi was exposed to a non-lethal dose of H2O2 for 24 hours prior to being exposed to a High Release pDA coating, a significant reduction in adhesion was observed when compared to both the uncoated surface (44% reduction) and the previously unexposed bacteria on a High Release pDA coating (30% reduction) (Figure 8B).
After P. cryo was exposed to a non-lethal dose of H2O2 and then exposed to a High Release pDA coated surface, there was a significant reduction in adhesion when compared to both the uncoated surface (90% reduction) and the High Release pDA coating (17% reduction) with previously unexposed bacteria (p<0.01) (Figure 8C). After T. skag was exposed to the non-lethal dose of H2O2 and then exposed to a High Release pDA coating, there was a significant reduction in adhesion when compared to the uncoated surface (40% reduction, p<0.05) but was not significantly different from the High Release pDA coating with previously unexposed bacteria (Figure 8D).
Due to the prevalent concern with the use of antibiotics to prevent biofilm formation on the surface of satellite telemetry tags, alternative antimicrobial solutions which reduce the chances of resistance being formed has become necessary. One such antimicrobial that can prevent bacterial growth without a resistance formation is H2O2. The pDA surface coatings that were used in this work were previously established to be conformational and stable in solution on 316L stainless steel coupons with various surface finishes and produce low doses of H2O2 over a prolonged period of time (Tyo et al., 2019). This work furthered the characterization of the pDA coatings and found the difference in release quantities between the Low Release coating condition (pH 7.4 solution at 21°C) and the High Release coating condition (pH 8.5 solution at 35°C) to be related to the quantity of OH groups (Figure 2) which has been attributed to the degree of polymerization. Due to this correlation, we can conclude the degree of polymerization directly affects the concentration of OH groups which subsequently effects the release profile. The two release profiles evaluated in this study for concentration of OH groups were then used to evaluate bacterial adhesion. The ability to tailor the release of H2O2 based on the coating conditions places our pDA surface coatings in a unique position. These coatings could be tailored to prevent the adhesion of a specific bacterium based on its unique susceptibility to the ROS H2O2.
Prior to testing bacterial adhesion with pDA surface coatings, bacteria were evaluated for their bacteriostatic threshold. It was found that all bacterium types tested remained in a bacteriostatic condition for at least 12 hours when exposed to a dose of H2O2 greater than 150µM. A summary of the minimum exogenous dose required to induce bacteriostatic conditions for 12 hours as well as the reduction in adhesion when exposed to the High Release coating can be found in Table 2. As all the bacterium types tested showed a threshold at which their growth was inhibited, this makes H2O2 a potentially viable antimicrobial to use as a mechanism to reduce or prevent bacterial adhesion.
Table 2 Summary of bacterial responses to one-time bolus doses of H2O2 and High Release pDA surface coatings.
Bacteria exposed to medical grade (316L) stainless steel were observed to have little problem adhering to the surface while those exposed to pDA coated stainless steel did not form the same stable adhesive bonds – with one notable exception: S. epi (Figure 9). The Low Release pDA surface coating proved to be effective at preventing bacterial adhesion to stainless steel with a standard surface finish on half the tested strains (E. coli and T. skag) while the High Release coating was able to reduce adhesion on all gram-negative bacterial strains for the three tested surface finishes. The Low Release pDA coating only reduced bacterial adhesion with E. coli on a standard surface finish (Figure 4) and was able to consistently reduce adhesion, regardless of surface finish, with T. skag (Figure 7). The two aquatic bacterial strains exhibited a sensitivity to the High Release pDA surface coating regardless of surface finish. These gram-negative bacteria strains are more likely to be susceptible to ROS as they do not inherently possess a mechanism to degrade H2O2 into its byproducts. Staphylococcus spp have the less common ability to degrade H2O2 into its byproducts – water and oxygen – and use them as a source of nutrients to increase growth (Ryan and Kleinberg, 1995; Painter et al., 2015). We see this behavior when S. epi was exposed to our High Release pDA coatings regardless of surface finish. Once the effectiveness of our High Release pDA surface coatings to reduce bacterial adhesion was established, the potential for a resistance to be formed or for efficacy against bacterial adhesion to decline after prior exposure needed to be established. Most works examining resistance use bolus doses of H2O2 which induce a 90% reduction in viability. Interestingly, S. epi seemed to become more susceptible to pDA coatings after prior exposure to H2O2 (200µM) (Figure 8) while the other bacteria species tested showed no significant change in adhesion between pre-treated and untreated bacteria. It has been observed that extracellular DNA contributes to an increase in bacterial adhesion and subsequent biofilm formation (Pakkulnan et al., 2019). As H2O2 can damage DNA, both intracellular and extracellular, it is possible that any extracellular DNA deposited on the surface of the stainless steel which would have acted to improve bacterial adhesion is unable to due to the presence of the pDA surface coatings. These coatings have demonstrated they can reduce bacterial adhesion to the surface of 316L stainless steel which indicates that they could be beneficial if applied in the field. As the largest chance for infection comes prior to and during implantation of the satellite telemetry tags, the oxidation and subsequent release of H2O2 from the pDA coatings could act to reduce the chance for infection during the tagging process.
Figure 9 Summary of bacterial adhesion on 316L stainless steel in response to High Release pDA surface coating. Bacteria exposed to a High Release pDA surface coating for one-third of their full growth cycle were not able to adhere to the surface of medical grade stainless steel as effectively as they could on an uncoated surface. The only exception, from the bacteria tested, was S. epi which adhered more effectively to a High Release pDA coated surface. Bacteria can be seen on FESEM images and are highlighted in red.
The addition of pDA surface coatings also significantly increased the surface hydrophilicity (Figure 3) which could potentially lead to a reduction in bacterial adhesion without the additional hydrogen peroxide generation. However, each bacteria species will have different responses to surface features such as hydrophobicity which can be seen in the responses from each bacterium to both Low Release and High Release pDA coatings. S. epi and P. cryo showed no significant reduction in bacterial adhesion when the Low Release coating was added, despite there being a significant increase in surface hydrophilicity. However, when exposed to the High Release pDA coating, there was a significant reduction in bacterial adhesion for P. cryo bacteria (80% ± 6.6%, p<0.01). There was no significant difference found in surface hydrophilicity between the pDA coatings which indicates that the reduction in adhesion was most likely due to the H2O2 generated by the High Release coating.
Currently, pDA coatings are primarily used to improve surface adhesivity, either by priming the surface to better bind to a secondary substrate or acting synergistically with a secondary adhesive (Beckford and Zou, 2014; He et al., 2014; Tran et al., 2018), or are used detect the presence of various molecules (Ma et al., 2013; Lin et al., 2014; Xu et al., 2019). However, this work indicates pDA surface coatings can be an effective way to reduce bacterial adhesion and subsequently reduce the chance of biofilm formation and infection on medical grade stainless steel. As infection is a concern with the use of implantable devices, not just for cetaceans but for all marine animals, these coatings could be applied to a range of tools used in marine conservation. Due to the sensitive nature of pDA assembly and polymerization, we cannot say with certainty that the coatings, when applied to another metallic substrate (e.g., titanium) will exhibit the same release behavior. However, the same optimization and characterization techniques our group has used to develop these pDA coatings can be used to create coatings that would work on virtually any surface.
Stainless steel coated with pDA has a demonstrated ability to reduce bacterial adhesion of E. coli, T. skag, and P. cryo regardless of the underlying surface roughness. The coating, which has a tailorable release profile through the alteration of coating conditions, has the potential to reduce bacterial adhesion and therefore prevent biofilm formation which could lead to subsequent infection. In addition to being able to prevent bacterial adhesion, the quantities of H2O2 released are within the therapeutic range and have the potential to aid in wound healing mechanisms. Future work will evaluate the ability for the pDA coatings to act as a wound healing aid via direct cellular contact or through the prolonged release of micro-doses of H2O2.
The raw data supporting the conclusions of this article will be made available by the authors, without undue reservation.
AS, BL, AZ, and RR designed and directed the project. AS and JW designed and completed all the bacterial experiments. AS designed and performed the characterization experiments. MH performed and analyzed FOX assays. AS, CD, and LL formatted and analyzed the data. AS drafted the manuscript. All authors contributed to the final version of the manuscript. AZ and RR supervised the project and oversaw the overall direction of the project. All authors contributed to the article and approved the submitted version.
This work was funded by the following grants: ONR (N00014-13-1-0653) and NOAA awarded to Cascadia Research Collective and to Marine Ecology and Telemetry Research.
The authors would like to acknowledge the Michigan Technological University machine shop for their contributions machining the stainless steel for testing.
The authors declare that the research was conducted in the absence of any commercial or financial relationships that could be construed as a potential conflict of interest.
All claims expressed in this article are solely those of the authors and do not necessarily represent those of their affiliated organizations, or those of the publisher, the editors and the reviewers. Any product that may be evaluated in this article, or claim that may be made by its manufacturer, is not guaranteed or endorsed by the publisher.
The Supplementary Material for this article can be found online at: https://www.frontiersin.org/articles/10.3389/fmars.2022.989025/full#supplementary-material
Andrews R. D., Pitman R. L., Ballance L. T.. (2008). Satellite tracking reveals distinct movement patterns for Type B and Type C killer whales in the southern Ross Sea, Antarctica. Polar Biol. 31 (12), 1461–1468.
Andrews R. D., Baird R. W., Calambokidis J., Goertz C. E. C., Gulland F. M. D., Heide-Jorgensen M. P., et al. (2019). Best practice guidelines for cetacean tagging. IWC J. Cetacean Res. Manage. 20 (1), 27–66. doi: 10.47536/jcrm.v20i1.237
Apprill A., Robbins J., Eren A. M., Pack A. A., Reveillaud J., Mattila D., et al. (2014). Humpback whale populations share a core skin bacterial community: Towards a health index for marine mammals? PloS One 9 (3), e90785. doi: 10.1371/journal.pone.0090785
Balmer B. C., Wells R. S., Howle L. E., Barleycorn A. A., McLellan W. A., Ann Pabst D., et al. (2014). Advances in cetacean telemetry: A review of single-pin transmitter attachment techniques on small cetaceans and development of a new satellite-linked transmitter design. Mar. Mammal Sci. 30 (2), 656–673. doi: 10.1111/mms.12072
Beckford S., Zou M. (2014). Wear Resistant PTFE thin film enabled by a polydopamine adhesive layer. Appl. Surf Sci. 292, 350–356. doi: 10.1016/j.apsusc.2013.11.143
Bierlich K. C., Miller C., DeForce E., Friedlaender A. S., Johnston D. W., Apprill A. (2018). Temporal and regional variability in the skin microbiome of humpback whales along the Western Antarctic peninsula. Appl. Environ. Microbiol. 84 (5), e02574-17. doi: 10.1128/AEM.02574-17
Fang F. C. (2011). Antimicrobial actions of reactive oxygen species. mBio 2 (5), e00141-11. doi: 10.1128/mBio.00141-11
Frette L., Jorgensen N. O. G., Irming H., Kroer N. (2004). Tenacibaculum skagerrakense sp. nov., a marine bacterium isolated from the pelagic zone in skagerrak, Denmark. Int. J. Syst. Evol. Microbiol. 54 (Pt 2), 519–524. doi: 10.1099/ijs.0.02398-0
Hahn F. E., Sarre S. G. (1969). Mechanism of action of gentamicin. J. Infect. Dis. 119 (4), 364–369. doi: 10.1093/infdis/119.4-5.364
He S., Zhou P., Wang L., Xiong X., Zhang Y., Deng Y., et al. (2014). Antibiotic-decorated titanium with enhanced antibacterial activity through adhesive polydopamine for dental/bone implant. J. R Soc. Interface 11 (95), 20140169. doi: 10.1098/rsif.2014.0169
IWC (2020). Report of the joint US office of naval research, international whaling commission and US national oceanic and atmospheric administration workshop on cetacean tag development, tag follow-up and tagging best practices. (International Whaling Commission, Impington, UK)
Jung W. K., Koo H. C., Kim K. W., Shin S., Kim S. H., Park Y. H. (2008). Antibacterial activity and mechanism of action of the silver ion in staphylococcus aureus and escherichia coli. Appl. Environ. Microbiol. 74 (7), 2171–2178. doi: 10.1128/AEM.02001-07
Lagerquist B. A., Mate B. R., Ortega-Ortiz J. G., Winsor M., Urbán-Ramirez J. (2008). Migratory movements and surfacing rates of humpback whales (Megaptera novaeangliae) satellite tagged at Socorro island, Mexico. Mar. Mammal Sci. 24 (4), 815–830. doi: 10.1111/j.1748-7692.2008.00217.x
Li R., Liu X., Qiu W., Zhang M. (2016). In vivo monitoring of H2O2 with polydopamine and Prussian blue-coated microelectrode. Anal. Chem. 88 (15), 7769–7776. doi: 10.1021/acs.analchem.6b01765
Lin L.-S., Cong Z.-X., Cao J.-B., Ke K.-M., Peng Q.-L., Gao J., et al. (2014). Multifunctional Fe3O4@Polydopamine core–shell nanocomposites for intracellular mRNA detection and imaging-guided photothermal therapy. ACS Nano 8 (4), 3876–3883. doi: 10.1021/nn500722y
Linley E., Denyer S. P., McDonnell G., Simons C., Maillard J. Y. (2012). Use of hydrogen peroxide as a biocide: new consideration of its mechanisms of biocidal action. J. Antimicrob. Chemother. 67 (7), 1589–1596. doi: 10.1093/jac/dks129
Liu Y., Ai K., Lu L. (2014). Polydopamine and its derivative materials: Synthesis and promising applications in energy, environmental, and biomedical fields. Chem. Rev. 114 (9), 58. doi: 10.1021/cr400407a
Livermore D. M. (2000). Antibiotic resistance in staphylococci. Int. J. Antimicrob Agents 16, 3–10. doi: 10.1016/S0924-8579(00)00299-5
Madanská J., Vitková Z., Capková Z. (2004). Examination of the stability of hydrogen peroxide solutions. Ceska Slov Farm 53 (5), 261–263.
Mate B., Mesecar R., Lagerquist B. (2007). The evolution of satellite-monitored radio tags for large whales: One laboratory’s experience. Deep Sea Res. Part II: Topic Stud. Oceanogr 54 (3), 224–247. doi: 10.1016/j.dsr2.2006.11.021
Ma Y.-R., Zhang ,. X.-L., Zeng T., Cao D., Zhou Z., Li ,. W.-H., et al. (2013). Polydopamine-coated magnetic nanoparticles for enrichment and direct detection of small molecule pollutants coupled with MALDI-TOF-MS. ACS Appl. Mater Interfaces 5 (3), 1024–1030. doi: 10.1021/am3027025
Mude H., Maroju P. A., Balapure A., Ganesan R., Ray Dutta J. (2021). Quaternized polydopamine coatings for anchoring molecularly dispersed broad-spectrum antimicrobial silver salts. ACS Appl. Bio Mater 4 (12), 8396–8406. doi: 10.1021/acsabm.1c00952
Nelson T. M., Apprill A., Mann J., Rogers T. L., Brown M. V. (2015). The marine mammal microbiome: current knowledge and future directions. Microbiol. Aust. 36 (1), 8–13. doi: 10.1071/MA15004
Ojha N., Roy S., He G., Biswas S., Velayutham M., Khanna S., et al. (2008). Assessment of wound-site redox environment and the significance of Rac2 in cutaneous healing. Free Radical Biol. Med. 44 (4), 682–691. doi: 10.1016/j.freeradbiomed.2007.10.056
Ortega M. P., Hagiwara T., Watanabe H., Sakiyama T. (2010). Adhesion behavior and removability of escherichia coli on stainless steel surface. Food Control 21 (4), 573–578. doi: 10.1016/j.foodcont.2009.08.010
Painter K. L., Strange E., Parkhill J., Bamford K. B., Armstrong-James D., Edwards A. M. (2015). Staphylococcus aureus adapts to oxidative stress by producing H2O2-resistant small-colony variants via the SOS response. Infect Immun. 83 (5), 1830–1844. doi: 10.1128/IAI.03016-14
Pakkulnan R., Anutrakunchai C., Kanthawong S., Taweechaisupapong S., Chareonsudjai P., Chareonsudjai S. (2019). Extracellular DNA facilitates bacterial adhesion during burkholderia pseudomallei biofilm formation. PloS One 14 (3), e0213288. doi: 10.1371/journal.pone.0213288
Pal S., Tak Y. K., Song J. M. (2007). Does the antibacterial activity of silver nanoparticles depend on the shape of the nanoparticle? a study of the gram-negative bacterium escherichia coli. Appl. Environ. Microbiol. 73 (6), 1712–1720. doi: 10.1128/AEM.02218-06
Ross A. A., Rodrigues Hoffmann A., Neufeld J. D. (2019). The skin microbiome of vertebrates. Microbiome 7 (1), 79. doi: 10.1186/s40168-019-0694-6
Ryan C. S., Kleinberg I. (1995). Bacteria in human mouths involved in the production and utilization of hydrogen peroxide. Arch. Oral. Biol. 40 (8), 753–763. doi: 10.1016/0003-9969(95)00029-O
Shakil S., Khan R., Zarrilli R., Khan A. U. (2008). Aminoglycosides versus bacteria – a description of the action, resistance mechanism, and nosocomial battleground. J. Biomed. Sci. 15 (1), 5–14. doi: 10.1007/s11373-007-9194-y
Su L., Yu Y., Zhao Y., Liang F., Zhang X. (2016). Strong antibacterial polydopamine coatings prepared by a shaking-assisted method. Sci. Rep. 6, 24420. doi: 10.1038/srep24420
Tran N. T., Flanagan D. P., Orlicki J. A., Lenhart J. L., Proctor K. L., Knorr D. B. (2018). Polydopamine and polydopamine–silane hybrid surface treatments in structural adhesive applications. Langmuir 34 (4), 1274–1286. doi: 10.1021/acs.langmuir.7b03178
Tyo A., Welch S., Hennenfent M., Kord Fooroshani P., Lee B. P., Rajachar R. (2019). Development and characterization of an antimicrobial polydopamine coating for conservation of humpback whales. Front. Chem. 7 (618). doi: 10.3389/fchem.2019.00618
Vatansever F., de Melo W. C., Avci P., Vecchio D., Sadasivam M., Gupta A., et al. (2013). Antimicrobial strategies centered around reactive oxygen species–bactericidal antibiotics, photodynamic therapy, and beyond. FEMS Microbiol. Rev. 37 (6), 955–989. doi: 10.1111/1574-6976.12026
Watanakunakorn C. (1984). Mode of action and in-vitro activity of vancomycin. J. Antimicrob. Chemother. 14 Suppl D, 7–18. doi: 10.1093/jac/14.suppl_d.7
Wolff S. P. (1994). Ferrous ion oxidation in presence of ferric ion indicator xylenol orange for measurement of hydroperoxides. Meth. Enzymol. 233, 182–189. doi: 10.1016/S0076-6879(94)33021-2
Xu S., Zhang G., Fang B., Xiong Q., Duan H., Lai W. (2019). Lateral flow immunoassay based on polydopamine-coated gold nanoparticles for the sensitive detection of zearalenone in maize. ACS Appl. Mater Interfaces 11 (34), 31283–31290. doi: 10.1021/acsami.9b08789
Yoo S. K., Huttenlocher A. (2009). Innate immunity: wounds burst H2O2 signals to leukocytes. Curr. Biol. 19 (14), R553–R555. doi: 10.1016/j.cub.2009.06.025
Zangmeister R. A., Morris T. A., Tarlov M. J. (2013). Characterization of polydopamine thin films deposited at short times by autoxidation of dopamine. Langmuir 29 (27), 8619–8628. doi: 10.1021/la400587j
Keywords: polydopamine (coating), cetacean marine mammal, satellite telemetry, biofilm prevention, antimicrobial
Citation: Smies A, Wales J, Hennenfent M, Lyons L, Dunn C, Robbins J, Lee BP, Zerbini A and Rajachar RM (2022) Non-antibiotic antimicrobial polydopamine surface coating to prevent stable biofilm formation on satellite telemetry tags used in cetacean conservation applications. Front. Mar. Sci. 9:989025. doi: 10.3389/fmars.2022.989025
Received: 11 July 2022; Accepted: 14 October 2022;
Published: 28 October 2022.
Edited by:
Peter H. Dutton, Southwest Fisheries Science Center (NOAA), United StatesReviewed by:
Michele Thums, Australian Institute of Marine Science (AIMS), AustraliaCopyright © 2022 Smies, Wales, Hennenfent, Lyons, Dunn, Robbins, Lee, Zerbini and Rajachar. This is an open-access article distributed under the terms of the Creative Commons Attribution License (CC BY). The use, distribution or reproduction in other forums is permitted, provided the original author(s) and the copyright owner(s) are credited and that the original publication in this journal is cited, in accordance with accepted academic practice. No use, distribution or reproduction is permitted which does not comply with these terms.
*Correspondence: Rupak M. Rajachar, cnVwYWtyQG10dS5lZHU=
Disclaimer: All claims expressed in this article are solely those of the authors and do not necessarily represent those of their affiliated organizations, or those of the publisher, the editors and the reviewers. Any product that may be evaluated in this article or claim that may be made by its manufacturer is not guaranteed or endorsed by the publisher.
Research integrity at Frontiers
Learn more about the work of our research integrity team to safeguard the quality of each article we publish.