- 1Marine Biology Station Piran, National Institute of Biology, Piran, Slovenia
- 2Department of Life Sciences, University of Trieste, Trieste, Italy
- 3Department of Earth, Environment and Life Sciences, University of Genoa, Genoa, Italy
- 4NBFC, National Biodiversity Future Center, Palermo, Italy
- 5World Wide Fund for Nature (WWF) Marine Protected Area of Miramare, Trieste, Italy
Several anthropogenic factors are responsible for the decline of Cystoseira sensu lato (hereafter Cystoseira) forests along Mediterranean coasts. Some Cystoseira species are already regionally extinct, and their decline has been widely recorded. Sustainable and efficient techniques for the restoration of Cystoseira are needed. In this context, the objectives of this study were i) to analyse the reproductive traits of three populations of Gongolaria barbata from three nearby donor sites in the northern Adriatic Sea, assessing the differences in their reproductive potential and reproductive success; and ii) to evaluate the outplanting success in terms of the effectiveness of G. barbata restoration, in relation to the different donor and receiving sites (Miramare MPA and in front of the Marine Biology Station Piran - MBSP) and implemented methods (ex situ and hybrid method combining a mesocosm cultivation and a suspended culture in the field). After 2 weeks of cultivation in mesocosms, half of the tiles with germlings were transported to the receiving sites and placed on suspended lantern nets (hybrid method), which were later (after 3 months) transferred to the seabed on concrete plates with protective cages. The remaining tiles were placed on the seabed on concrete plates with protective cages after a 4-week culture (ex situ method). At both sites, lantern nets and plates were randomly placed at 3 m depth. Thallus length was measured monthly in each treatment. Seedlings in suspended culture showed lower performance at the Miramare MPA, most likely due to the unfavourable environmental conditions. The satisfactory results obtained at MBSP demonstrate the efficiency of the hybrid method and confirm its potential to reduce the cost and time required for cultivation. Since unpredictable climatic events pose the greatest threat to restoration performance, these challenges must be considered when establishing new restoration practices. Moreover, herbivore regulation is extremely urgent and should be planned and implemented on a larger regional scale.
Introduction
Canopy-forming fucoids are dominant foundation species found on almost all Mediterranean coasts (Bulleri et al., 2012; Gianni et al., 2013; Assis et al., 2020; Bringloe et al., 2020). In particular, Cystoseira sensu lato (Fucales, Phaeophyta) species (hereafter referred to as Cystoseira), recently subdivided into the three genera Cystoseira, Ericaria and Gongolaria (Molinari-Novoa and Guiry, 2020), can thrive from intertidal to circalittoral rocky bottoms, forming dense forests that are among the most productive assemblages in the Mediterranean coastal zone, with different species replacing each other along a bathymetric gradient (Boudouresque and Lück, 1972; Giaccone, 1973; Ballesteros, 1989; Rull and Gómez Garreta, 1989; Otero-Schmitt and Pérez-Cirera, 1996; Ballesteros et al., 1998; Pizzuto, 1999; Ballesteros et al., 2009).
Important ecosystem services (De La Fuente et al., 2019a) provided by these brown algal forests include high primary production (Ballesteros et al., 2009; Mačić and Svirčev, 2014), rich understory communities of algae and invertebrates (Pitacco et al., 2014; Bianchelli et al., 2016; Mancuso et al., 2021), outstanding fish densities and diversity (Orlando-Bonaca and Lipej, 2005; Cheminée et al., 2013), and a long term carbon sink (Peleg et al., 2020). Other benefits of Cystoseira to humans include the production of several bioactive metabolites (Bruno de Sousa et al., 2017a), with antioxidant, anti-inflammatory, antifungal, antiviral and antibacterial effects (Mhadhebi et al., 2011; Vizetto-Duarte et al., 2016; Bruno de Sousa et al., 2017b; De La Fuente et al., 2020).
As a result of multiple anthropogenic impacts (e.g., coastal urbanisation, eutrophication, sediment loading and overgrazing), Cystoseira stands have gradually disappeared in many Mediterranean coastal areas, often being replaced by filamentous or ephemeral taxa (e.g., Mangialajo et al., 2008; Falace et al., 2010; Vergés et al., 2014; Thibaut et al., 2015; Rindi et al., 2017; Rindi et al., 2018; Catra et al., 2019; Mariani et al., 2019; Bernal-Ibáñez et al., 2021; Orlando-Bonaca et al., 2021a; Orfanidis et al., 2021). In addition, human changes in the marine environment also affect connectivity, leading to habitat fragmentation and loss, and genetic disjunction even at small spatial scales (Alberto et al., 2010).
Furthermore, an important feature of Cystoseira settlements is that their zygotes sink rapidly (Clayton, 1990), so that they fall and stick to the substrate near the parent algae. Due to their low dispersal, the colonisation of new or damaged areas is therefore difficult, so habitat fragmentation has a major impact on these species.
The first strategy to address the decline of Cystoseira and their habitat was their protection through international agreements (e.g., Bern Convention, Barcelona Convention, Directive 92/43/EEC, European Red List of Habitats). Nevertheless, there is little evidence of natural recovery of degraded Cystoseira forests (but see Munda, 2000; Perkol-Finkel and Airoldi, 2010; Iveša et al., 2016; Medrano et al., 2020a).
Restoration of affected areas, but only those where disturbance is no longer present or has been mitigated, is so important as it can greatly accelerate habitat recovery.
Restoration is increasingly recognized as an appropriate strategy to actively trigger the recovery of degraded coastal habitats (Abelson et al., 2020), as proposed in the recently proclaimed UN Decade of Ecosystem Restoration (2021−2030; Waltham et al., 2020). However, implementing successful restoration efforts requires detailed knowledge of the current and past distribution of lost habitats and species, the pressures that led to their decline, and accurate characterization of donor populations (Gann et al., 2019). In recent years, several restoration attempts have been made in the Mediterranean region through different projects to address the loss of Cystoseira (Falace et al., 2018; Verdura et al., 2018; De La Fuente et al., 2019b; Medrano et al., 2020b; Orlando-Bonaca et al., 2021b; Savonitto et al., 2021).
Restoration of brown algal forests can be achieved through: transplantation of adult thalli (Falace et al., 2006; Susini et al., 2007), deployment of bags with fertile receptacles in situ, attached to a hoe and fixed to the seafloor at selected restoration sites (Verdura et al., 2018; Medrano et al., 2020b), and the outplanting of juveniles grown ex situ under laboratory conditions (Falace et al., 2006; Sales et al., 2011; Falace et al., 2018; Verdura et al., 2018; De La Fuente et al., 2019b; Savonitto et al., 2021; Orlando-Bonaca et al., 2021b; Lardi et al., 2022). The latter two techniques are recommended for the recovery of endangered species to avoid depleting donor populations (De La Fuente et al., 2019b).
A sharp decline of Fucales has also been reported in the northern Adriatic basin (Falace et al., 2010; Orlando-Bonaca and Rotter, 2018; Orlando-Bonaca et al., 2021a), leading to displacement by turf-forming taxa in shallow waters. The occurrence of these low-lying algae is likely related to human-induced hydromorphological changes to the shoreline and high sediment resuspension rates (Falace et al., 2010; Orlando-Bonaca and Rotter, 2018) rather than nutrient enrichment, as the northern Adriatic is considered oligotrophic (Mozetič et al., 2012).
Moreover, negative impacts from native herbivorous fish have also been documented: for Sargassum vulgare C. Agardh (Orlando-Bonaca and Mavrič, 2014) and recently for the transplanted Gongolaria barbata (Stackhouse) Kuntze (Orlando-Bonaca et al., 2021b; Savonitto et al., 2021). Currently, G. barbata and Cystoseira compressa (Esper) Gerloff and Nizamuddin are quite common in the Gulf of Trieste only on the Slovenian coast, while they have almost disappeared on the Italian coast. Other species from this group are already rare in Slovenian waters and extinct in Italian waters of the Gulf of Trieste.
Based on previous results of G. barbata restoration in the northern Adriatic (Orlando-Bonaca et al., 2021b; Savonitto et al., 2021), the present study aimed to evaluate the performance of different donor populations in close proximity to each other in the restoration of two receiving sites. To successfully reduce cultivation time (to avoid lengthy maintenance and minimise costs), we compared the performance of the ex situ method (already consolidated at the same sites in the ROC-POP LIFE and J1-1702 projects) with a hybrid method combining cultivation in mesocosm and suspended culture in the field.
In this context, the objectives of this study were (1) to analyse the reproductive traits of three populations of G. barbata from three nearby donor sites (i.e., Izola, Strunjan, Piran) in order to assess the differences in their reproductive potential and reproductive success, and (2) to evaluate the restoration success in relation to the different donor and receiving sites (Miramare MPA vs. Marine Biology Station Piran) and the methods used. The added value of our work is that by combining two methods, for the first time different donor and receiving sites were compared in terms of restoration success of G. barbata.
Materials and methods
Study area
The study area is located in the Gulf of Trieste (Figure 1), a shallow, semi-enclosed bay in the northernmost part of the Adriatic and Mediterranean Seas. The gulf stretches from Cape Savudrija (Croatia) to Grado (Italy) and includes the entire Slovenian coast, with an average depth of about 21 m. The area is known for the lowest winter temperatures (mostly below 10°C) in the Mediterranean and the prevailing winds, which blow mainly from the northeast in an offshore direction (Boicourt et al., 2021). Maximum summer seawater temperatures reach 28°C (data from VIDA oceanographic buoy, https://www.nib.si/mbp/en/). The average salinity is about 37 and is mainly influenced by the freshwater inflow from the Soča (Isonzo) River, while the water circulation is mainly counterclockwise in the lower layer and clockwise in the surface layer (Stravisi, 1983).
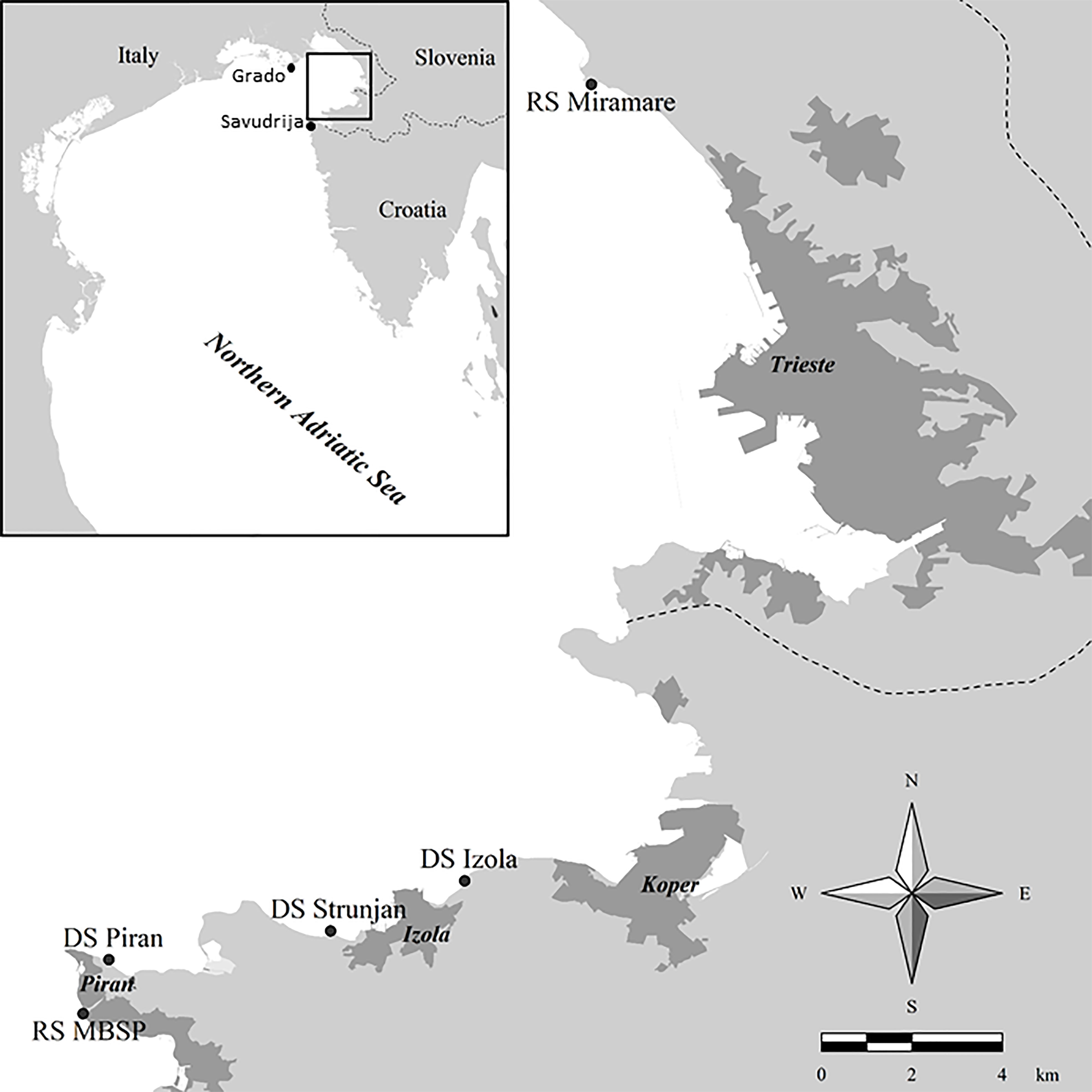
Figure 1 Map of the study area showing donor (= DS) and receiving sites (=RS) for Gongolaria barbata.
The rationale of the experiment, aimed at testing the effect of three donor and two receiving sites, and cultivation methods (hybrid vs. ex situ) on the restoration efficiency of G. barbata, is shown in Figure 2.
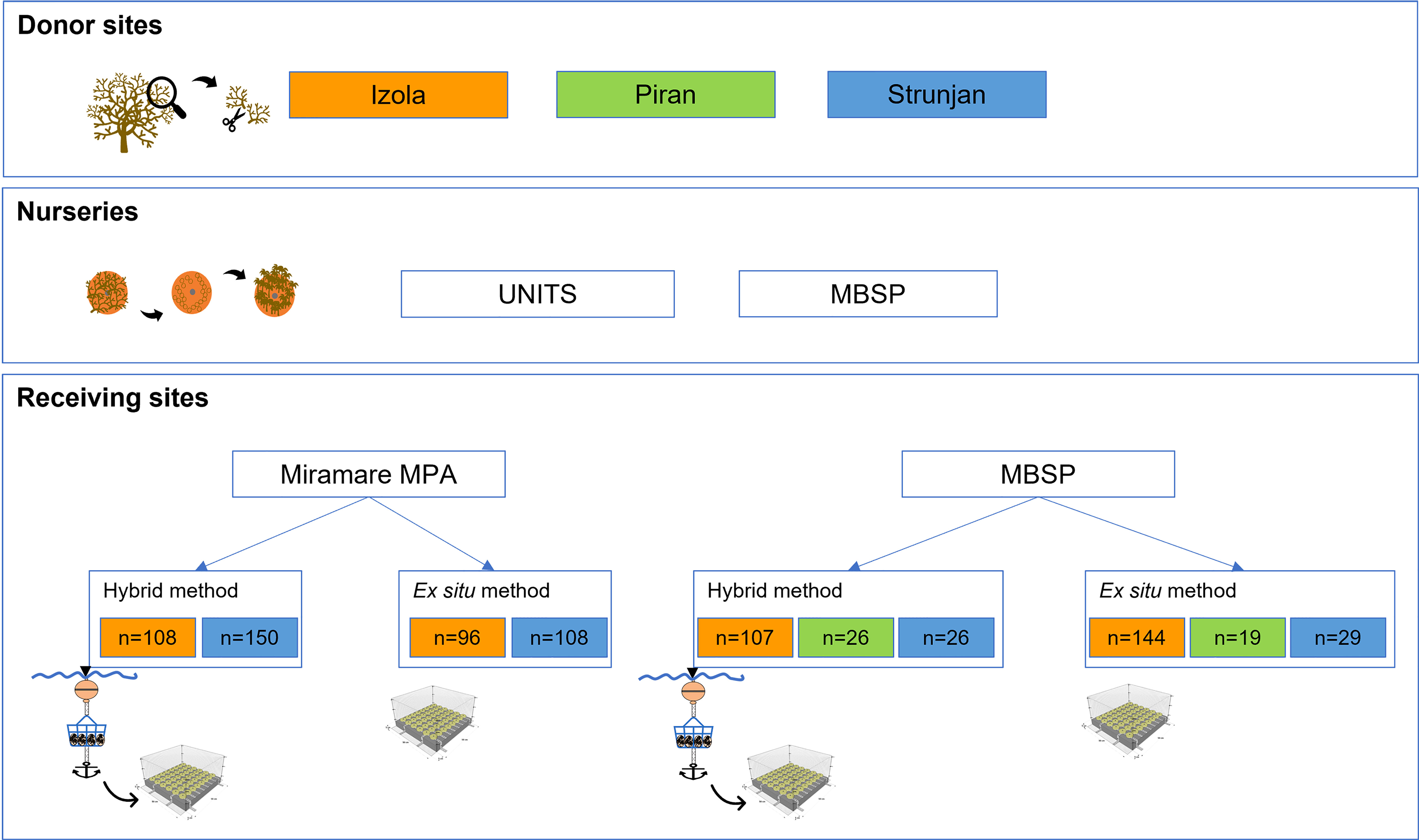
Figure 2 Experiment on G. barbata restoration in the northern Adriatic Sea. UNITS, University of Trieste (Italy), MBSP, Marine Biology Station Piran (Slovenia). It shows: i) the three donor sites where fertile receptacles were collected; ii) the two nursery facilities where germlings were cultivated on clay tiles starting from the fertile receptacles; iii) the two receiving sites where the tiles coming from different donor populations were outplanted using either the hybrid or ex situ method.
Fertile apices of G. barbata were collected in Slovenia from three donor populations located in:
- Izola (45.543567, 13.676371), which is characterised by a healthy and dense populations of G. barbata and C. compressa;
- Strunjan Natural Park (45.53379, 13.638281), where G. barbata forms healthy stands in association with C. compressa and Ericaria crinita (Duby) Molinari and Guiry;
- Piran (45.5284, 13.5754), where belts of G. barbata are present in association with C. compressa and E. crinita.
The distances along the coastline between the donor sites (at an isobath depth of 2 m) are: Izola-Strunjan = 4.621 km, and Strunjan-Piran = 6.873 km. All donor sites have a shallow, rocky seabed and are moderately exposed to wave action. Due to overgrazing by native herbivorous species such as Sarpa salpa (L., 1758) at the Piran sampling site in March 2021, the number of apices collected in this area was lower than at the other two donor sites (authors’ pers. obs.).
The germlings were cultivated in the two nursery facilities at the University of Trieste (UNITS) and at the Marine Biology Station Piran (MBSP).
The cultured germlings were outplanted in:
- the ‘no-take’ zone of the Miramare MPA in Italy (45.701802, 13.714223), where G. barbata once occurred (Bussani and Vuković, 1992) and was recently reintroduced as part of the ROC-POP LIFE project (Savonitto et al., 2021). The MPA is located in a highly urbanized coastal system and is affected by herbivorous fish grazing (Savonitto et al., 2021; Bevilacqua et al., 2022);
- the coastal area off the Marine Biology Station Piran (hereafter MBSP) in Slovenia (45.5177, 13.5680), where less than a decade ago there was a dense brown algal forest consisting mainly of G. barbata and C. compressa. This habitat was completely destroyed in 2016 due to invasive encroachment for beach construction, when no precautions were taken to limit environmental damage. This site is also affected by fish grazing pressure (Orlando-Bonaca et al., 2021b).
Culture in mesocosms
Cultures of G. barbata were carried out following the protocol of Falace et al. (2018) further improved in De La Fuente et al. (2019b).
In early April 2021, apices with mature receptacles were collected simultaneously at 1-2 m depth at the three donor sites. They were then transported to the nursery facilities within a few hours under cool (4°C) and dark conditions. At the laboratory, the receptacles were cleaned with filtered seawater to remove epibionts, and stored at 4°C for 24 h to promote gamete release.
In parallel, approximately 100 randomly selected apices from each donor site were stored at -4°C for further analyses (i.e., morphometric measurements).
In environmentally controlled rooms, approximately 50 mg FW fertile apices were placed on each rough clay tile (4.5 cm diameter with a central hole of 0.6 cm diameter), in aquaria filled with filtered seawater (0.22 μm filter membrane) enriched with Von Stosch solution and germanium dioxide (for details, see Falace et al., 2018; De La Fuente et al., 2019b), and oxygenated with air pumps and bubblers.
At UNITS, 10 aquaria with 50-55 tiles each were used. A total of 204 tiles were seeded with Izola apices and 303 with Strunjan apices. At MBSP, 6 aquaria were used, each containing 50-55 tiles. In total, 251 tiles were seeded with apices from Izola and 55 with apices from Piran. The tiles were labelled according to the donor populations. The differences in culturing efforts and, thus, the unbalanced experimental design depend on the availability of fertile material, which is related to the size of the populations themselves (i.e., Strunjan is larger in terms of population size, while the Piran population was severely damaged by fish grazing during the fertile season).
Temperature was set at 15°C, light intensity at 125 μmol m-2 s-1, and photoperiod at 15:9 h light:dark to simulate conditions during the reproductive period of G. barbata at donor sites. Air temperature was automatically set by the room controller and water temperature was measured daily with a thermometer in each aquarium to ensure it was constant.
Apices placed on the tiles for seeding were removed after twenty-four hours (T0). To avoid nutrient limitation, the culture medium was renewed every 3-5 days. Both the aquaria and the tiles within each aquarium were randomly repositioned at each periodic change of culture medium to provide random culture conditions to all the tiles.
Cultures lasted two weeks for tiles destined for the hybrid method and 4 weeks for tiles destined for the ex situ method.
Hybrid method
To test whether it is possible to shorten the cultivation time in mesocosm, an in situ suspended culture was established after a shorter cultivation time (i.e., 2 weeks).
Therefore, after 2 weeks of culture in the mesocosms (on April 23rd, T2), about half of the tiles were randomly selected and transported to the receiving sites, where they were fixed to plastic lantern nets (55 cm diameter) suspended at 2 m depth, as described in Savonitto et al. (2021); 108 tiles with germlings from Izola and 150 from Strunjan were placed in the Miramare MPA, while 107 from Izola, 26 from Strunjan and 26 from Piran were placed in front of MBSP.
After 3 months in the lantern nets (July), the tiles were placed on the rocky bottom using outplanting modules. The modules consisted of 50x50 cm concrete plates (each capable of holding up to 48-54 tiles) covered with iron anti-grazing cages (mesh size 1x1 cm2) and secured with metal wedges, as described in Orlando-Bonaca et al. (2021b). Tiles were randomly positioned on the modules, interspersing tiles hosting juveniles from the different donor populations.
In the Miramare MPA, the tiles without juveniles were excluded, so that finally 69 tiles from Strunjan and 7 tiles from Izola were placed on two plates. In front of the MBSP, all tiles were laid on three plates on the seabed near the lantern nets.
Ex situ method
After 4 weeks of cultivation in the mesocosms (on May 7th, T4), the tiles destined for the ex situ method were transported to the receiving sites and attached with screws to preassembled outplanting modules (see above). As with the hybrid method, tiles from the different donor sites were marked and randomly placed on the plates (4 plates at Miramare MPA and 4 in front of MBSP). The plates for the two methods were placed very close to each other on the seafloor.
Specifically, 96 tiles with juveniles from Izola and 108 from Strunjan were located in the Miramare MPA, while 144 from Izola, 19 from Strunjan and 29 from Piran were placed in front of the MBSP.
Tiles were monitored in the Miramare MPA and MBSP by SCUBA-divers, and iron cages were cleaned regularly with a wire brush to remove epibionts and sediment, at least twice a month at Miramare, an MPA with dedicated staff, whereas in front of the MBSP they were cleaned only once a month during routine monitoring. The difference in frequency of cleaning was due to the different degree of protection and monitoring to which the two receiving sites are subject.
Collected data
During mesocosm culture, the following data were collected and processed(Table 1):
- Morphometric measurements of receptacles (i.e., length and width in mm): 100 receptacles from each donor site (Izola, Strunjan, Piran) were randomly collected and photographed under a stereomicroscope (Leica MZ 6, Leica Microsystems, Wetzlar, Germany) with a Nikon Coolpix 4500 camera (Nikon Corporation, Tokyo, Japan) (at T0);
- Reproductive traits: 20 tiles per donor site (Izola and Strunjan) were randomly selected at UNITS (at T0) to determine the:
(i) reproductive potential as RP = N. of conceptacles per receptacle of all the receptacles on each tile. The number of conceptacles was determined by counting the total number of ostioles protruding from the outer surface of the receptacle using a stereomicroscope (Supplement 1);
(ii) reproductive success (RS = N. of zygotes per tile x N. of conceptacles per tile-1) by photographic sampling with a Nikon D300 camera (Nikon Corporation, Tokyo, Japan).
- Germling density (i.e., number of germlings per tile): at weeks 1 (T1), 2 (T2), 3 (T3, only at MBSP), and 4 (T4) after fertilisation, germling density was estimated on pictures (photographic sampling with a Nikon D300 camera) randomly selecting 42 tiles from Izola (henceforth as juvIZ) and 76 from Strunjan (juvST) at UNITS, while 30 from Izola (juvIZ) and 10 from Piran (juvPI) at MBSP. No data are available at T4 for juvPI cultured at MBSP.
- Germling length and width: at T4, 40 germlings per donor site (only the donor populations from Izola and Strunjan were considered: juvIZ and juvST at UNITS, juvIZ at MBSP) were randomly selected and photographed directly on the tiles under a stereomicroscope to measure their length and width. They were randomly selected each time, thus not repeated measures. No data are available at T4 for juvPI cultured at MBSP.
- Light intensity (Lux) and seawater temperature (°C) were measured from the time of outplanting on the seabed (T4) using a HOBO Pendant Data Logger (UA-OOx) at the MBSP receiving site.
In the laboratory, receptacles’ length and width, number of zygotes per tile at T0, number of germlings per tile at T1, T2, T3 and T4, and length and width of germlings at T4 were determined by analysing the photos with ImageJ software (Schneider et al., 2012).
In the field, thalli lengths were measured by SCUBA divers at Miramare MPA and MBSP on 20 randomly selected tiles per method and donor site monthly from May to November (7 times). In November, the percentage survival rate (i.e., the % of tiles that had juveniles on them) was determined by SCUBA divers. It is noteworthy that length data are not available for the hybrid method juveniles outplanted in the Miramare MPA from August onwards, as the mortality rate was 100%.
For the assessment of reproductive traits, germling density and germling morphometry in the laboratory and thallus length in the field, the tiles have been considered as replicates, even acknowledging an issue of spatial pseudoreplication since some of the tiles belong to the same aquarium/outplanting module. Logistical constraints did not allow us to have all completely independent replicates but care in interspersion and randomisation allowed us to reduce the risk of an aquarium/module effect. This aspect has been considered in results interpretation, keeping in mind the risk of inflated Type I errors in case of simple pseudoreplication (Millar and Anderson, 2004).
Statistical analysis
One-way ANOVA design was applied to test for possible differences between donor sites (Izola, Piran and Strunjan) in receptacle morphometry (length and width, n=100), reproductive potential (RP, n=20), reproductive success (RS, n=20) and germling morphometry (length and width, n=40) after 4 weeks of culture. Verification of the assumptions (normality with the Kolmogorov-Smirnov test and homoscedasticity with the Bartlett’s test) was performed prior to conducting the analyses. If the assumptions were not fulfilled, square root transformation of the response variable was applied and assumptions re-tested. If still not compliant with ANOVA assumptions, the non-parametric equivalent of ANOVA, the Kruskal-Wallis test, was performed.
Differences in germling density on the tiles according to donor populations (juvIZ, juvPI and juvST) were assessed applying a linear mixed model, after checking for normality of the response variables, using the variable “Donor population” as fixed, while “Time” as random.
As for the field data, two separate analyses were performed on the response variable thallus length measured at the last monitoring time (November 2021, n=20). Potential differences linked to the donor population and the outplanting method has been investigated through a two-way ANOVA design, testing the effect of the fixed orthogonal factors “Donor population” (3 levels: juvIZ, juvPI, juvST), “Outplanting method” (2 levels: ex situ, hybrid) and their interaction. This test has been performed only on MBSP data, because this was the receiving site hosting all donor populations and where it was possible to carry out both methods till the end of the experimentation. A second two way ANOVA design has been applied only considering the ex situ method data for testing the effect of the fixed orthogonal factors “Donor population” (2 levels: juvIZ, juvST), “Receiving site” (2 levels: Miramare MPA, MBSP) and their interaction. Normality and homoscedasticity assumptions were tested by Kolmogorov-Smirnov test and Bartlett’s test respectively, and a square root transformation was required.
For all analyses, post-hoc comparisons on significant terms were performed by Tukey test and significant differences are shown on the corresponding plots and table (for field data). All statistical analyses and plots were performed with “stats” and “car” packages of the free software RStudio (RStudio Team, 2021).
Results
- Culture in mesocosm
Morphometry of the receptacles
Significant differences were found in the length and width of the receptacles between the three donor sites, with the receptacles in Izola being longer and larger (length: 6.02 ± 0.26 SE mm; width: 0.96 ± 0.02 SE mm) than those from Piran (length: 4.66 ± 0.16 SE mm; width: 0.78 ± 0.02 SE mm; p-value < 0.001) and Strunjan (length: 4.70 ± 0.18 SE mm; width: 0.83 ± 0.02 SE mm; p-value < 0.001). No significant differences were found between Piran and Strunjan (Figure 3, Table 2).
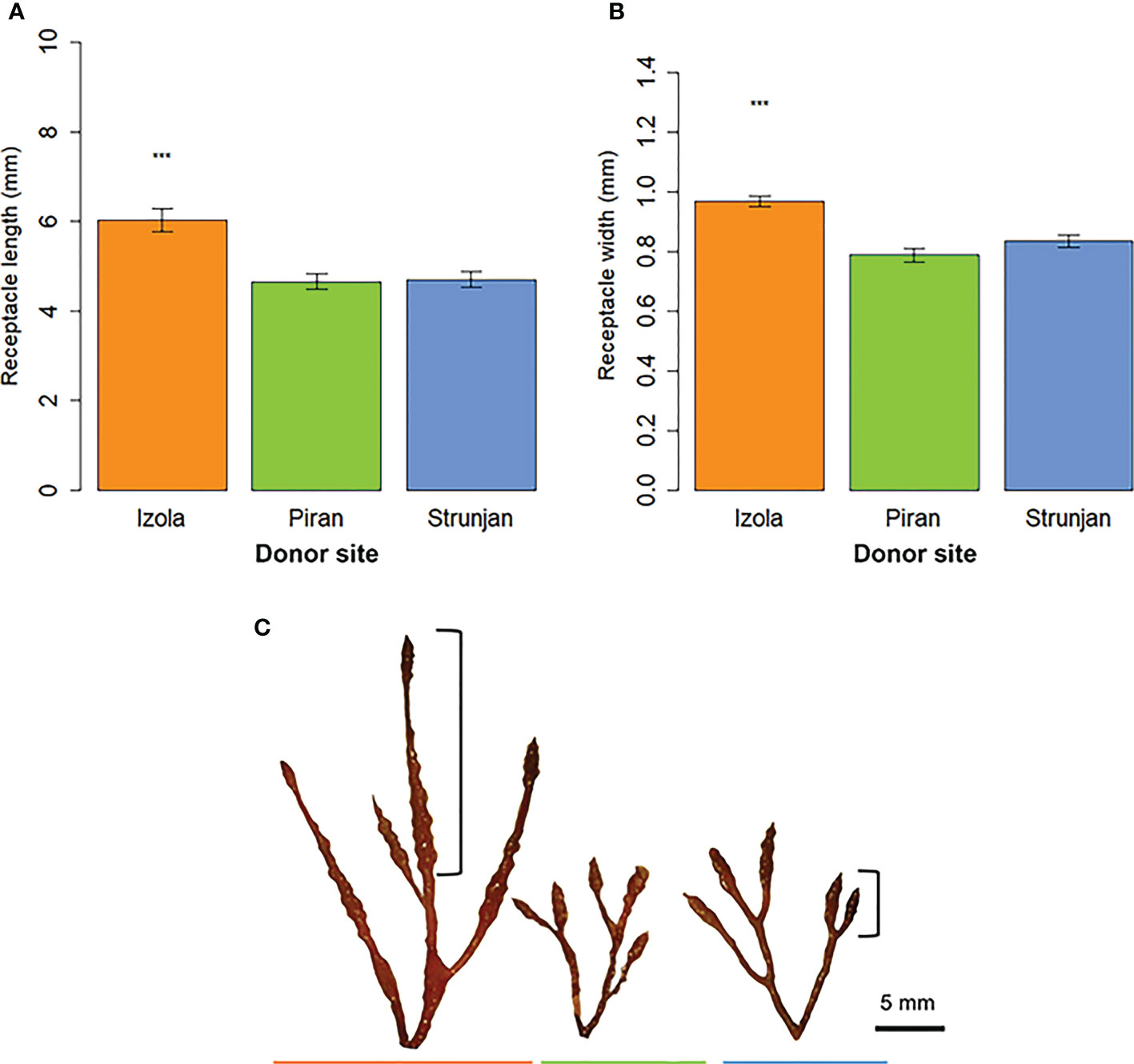
Figure 3 G barbata receptacles’ (A) length and (B) width in the three donor sites (mean ± SE); (C) fertile apices of G barbata populations from Izola (orange), Piran (green) and Strunjan (blue) sampling sites. Significance levels: ‘***’ p-value < 0.001.
The fertile apical fronds from Piran and Strunjan generally had short, simple and cylindrical receptacles that were sparsely mucronate and had few or no aerocysts (Figure 3C). In contrast, fronds from Izola had long fusiform or mucronate receptacles, predominantly prominent conceptacles, and there were numerous single or concatenated aerocysts (Figure 3C; Table 3).
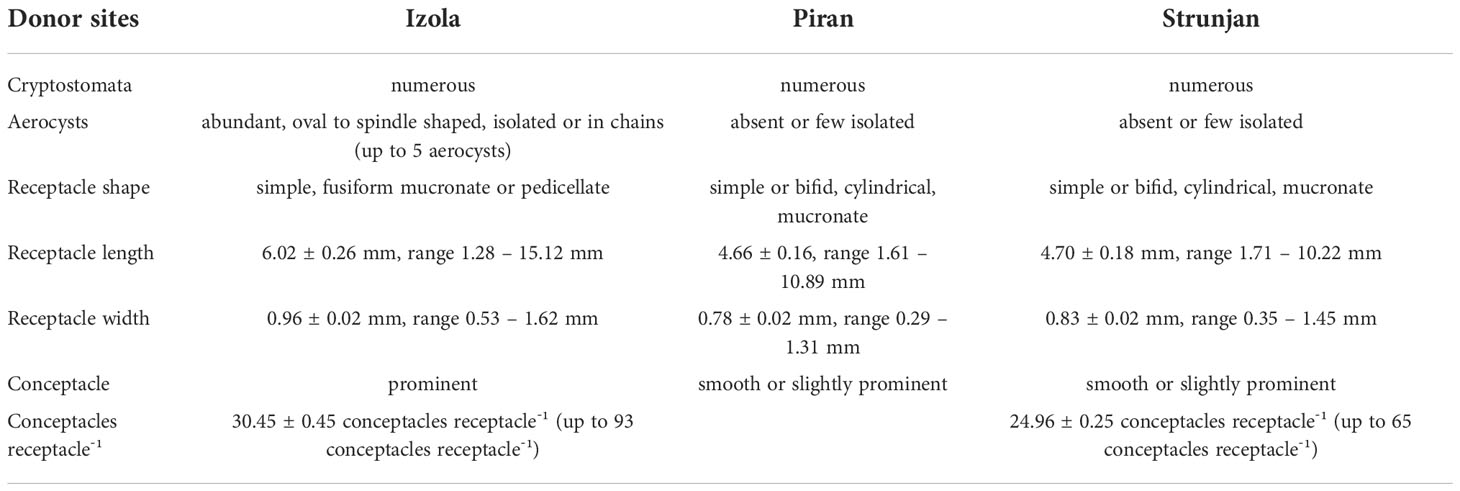
Table 3 Comparison of Gongolaria barbata populations sampled at Izola, Strunjan and Piran donor sites, in terms of receptacles’ features (numeric values are expressed as mean ± SE).
Reproductive traits
The Izola population receptacles had significantly higher reproductive potential (30.5 ± 0.4 SE conceptacles receptacle-1) compared to Strunjan (25.0 ± 0.3 SE conceptacles receptacle-1; p-value < 0.001; Figure 4A, Table 2). Conversely, the reproductive success of Izola receptacles (0.4 ± 0.1 SE zygotes conceptacle-1; p-value < 0.01) was significantly lower than those from Strunjan (0.8 ± 0.1 SE zygotes conceptacle-1; Figure 4B, Table 2).
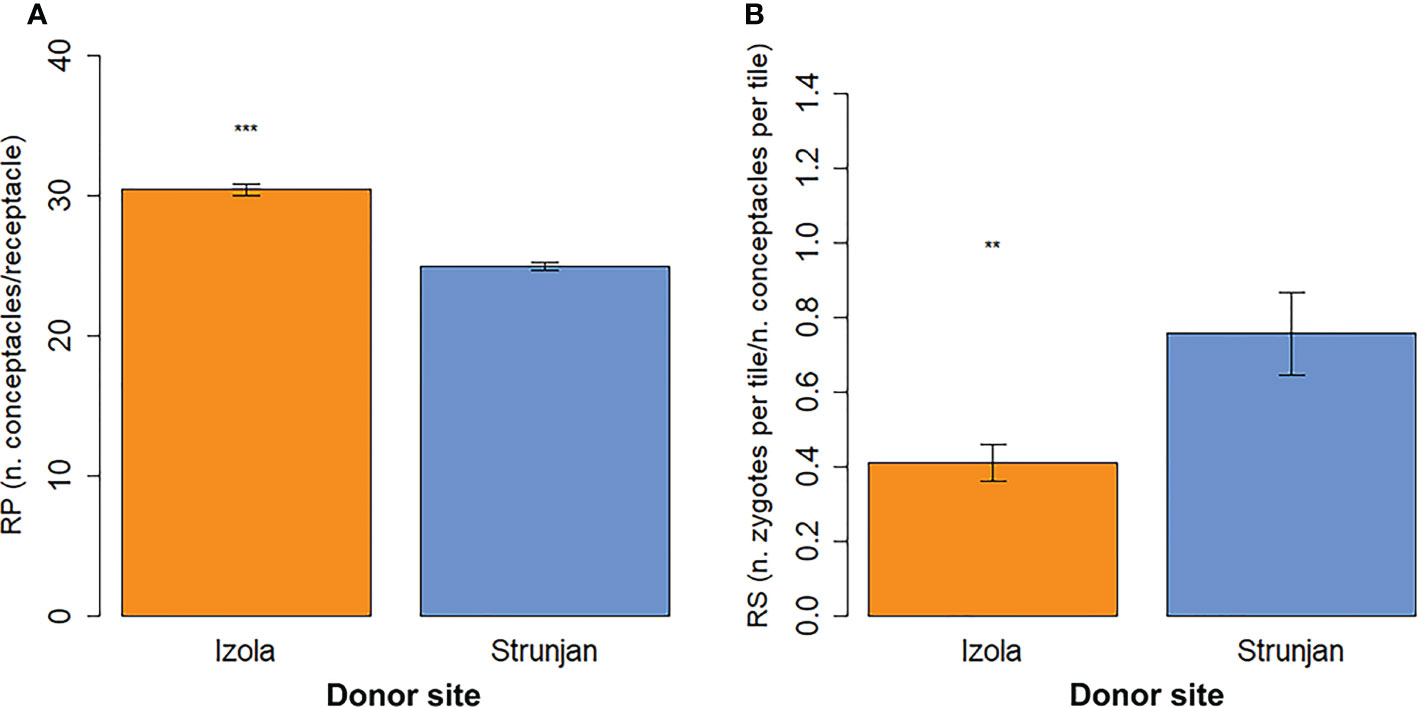
Figure 4 Reproductive potential (A) and reproductive success (B) of the receptacles from Izola and Strunjan donor sites (mean ± SE). Significance levels: ‘***’ p-value < 0.001, ‘**’ p-value < 0.01.
Germling density
Germling density gradually decreased from the first to the fourth week in culture (Figure 5). After 4 weeks, it ranged from 132 ± 15 SE germlings per tile (juvIZ) to 73 ± 8 SE germlings per tile (juvST) (Figure 5). JuvST had significantly different germling density (p-value < 0.001, Table 2), and showed higher values at earlier sampling times (T1-T2), than juvIZ and juvPI, which were again significantly different (p-value = 0.02, Table 2).
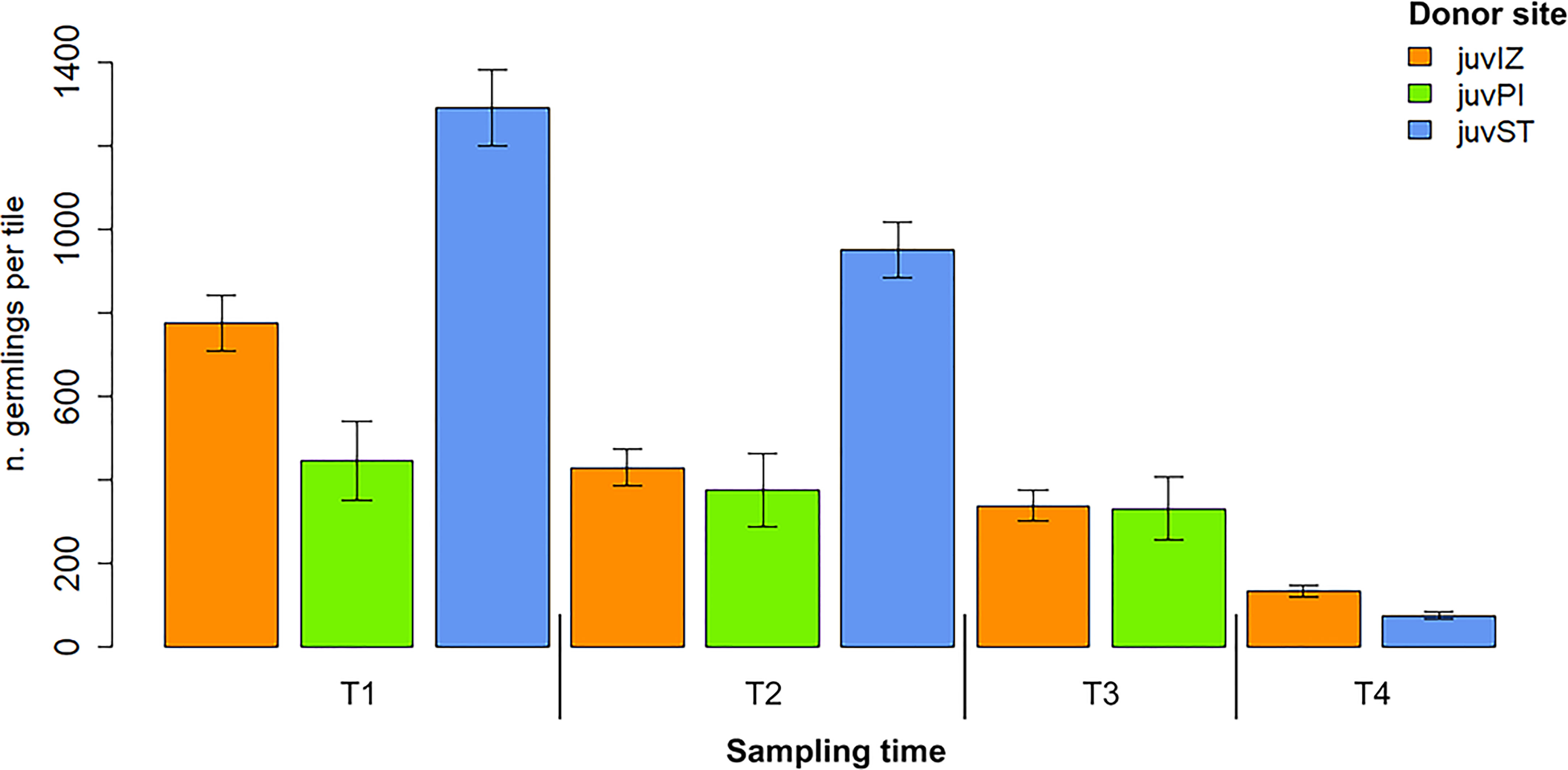
Figure 5 Germlings density (mean ± SE) on tiles from apices collected in Izola (juvIZ), Piran (juvPI) and Strunjan juvST (data for juvIZ are pooled for UNITS and MBSP). No data are available for juvST cultivated at UNITS at T3, and for juvPI cultivated at MBSP at T4.
Morphometry of germlings after 4 weeks
After 4 weeks in culture, significant differences in length and width were observed between juvIZ (length: 1.15 ± 0.07 SE mm; width: 0.29 ± 0.01 SE mm) and juvST (length: 0.65 ± 0.03 SE mm; width: 0.17 ± 0.01 SE mm), with juvIZ having higher values in both morphometric parameters (p-value < 0.001, Figures 6A, B; Table 2).
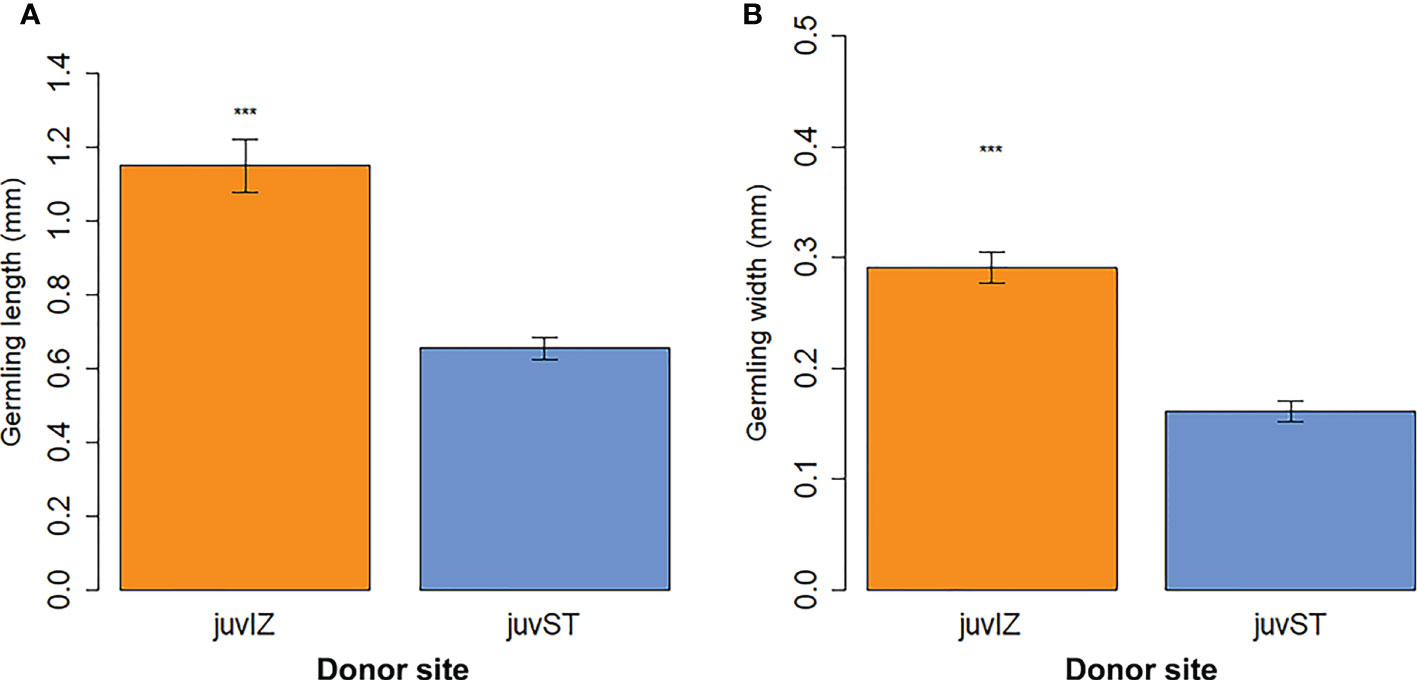
Figure 6 Germling size (mean ± SE) after 4 weeks of culture (data for juvIZ are pooled for UNITS and MBSP): (A) length (mm) and (B) width (mm). No data available for juvPI cultivated at MBSP. Significance levels: ‘***’ p-value < 0.001.
For these last parameters (i.e., reproductive traits, germling density and morphometry), also considering the pseudoreplication issue, the low p-values of statistical tests (always lower than 0.01) allowed us to consider our results reliable.
- Field data
The two-way ANOVA on MBSP data showed a significant effect of the interactions “Donor population” x “Outplanting Method” (p-value =0.0001, Table 4).
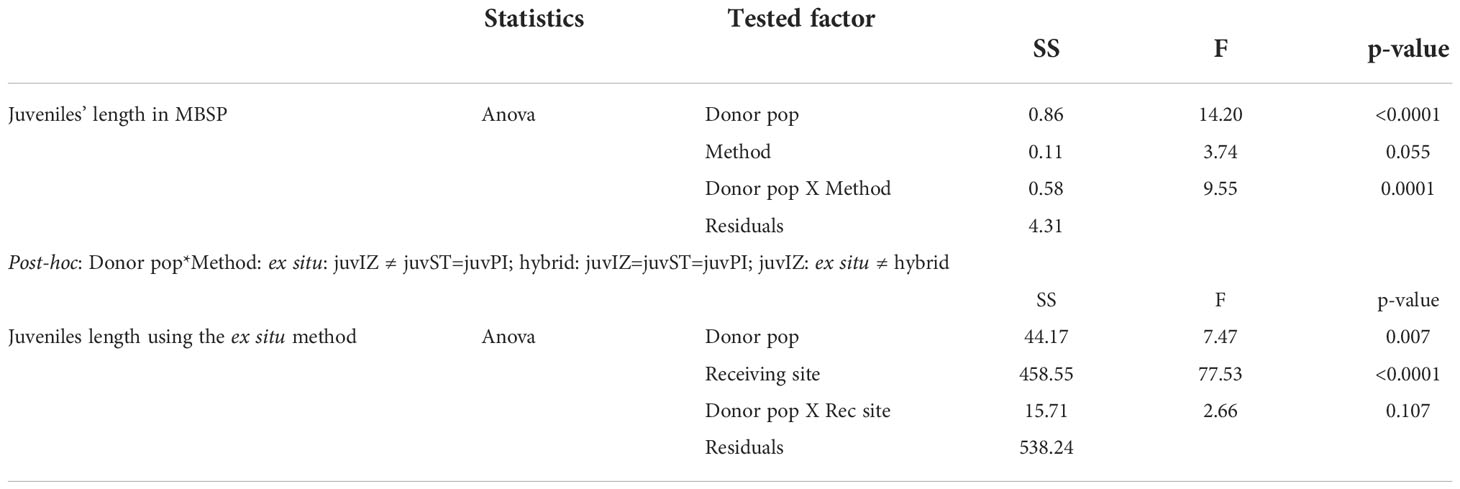
Table 4 Results of the statistical analyses on field data. In the footnotes, results of post-hoc comparisons are reported.
The three populations showed no differences in performance when the hybrid method was applied, while juvIZ performed differently from juvPI and juvST in the ex situ method (p-value <0.001). JuvIZ performed better if outplanted through the ex situ method compared to hybrid (p-value <0.001), while no differences were observed for juvPI and juvST (Table 4, Figure 7A).
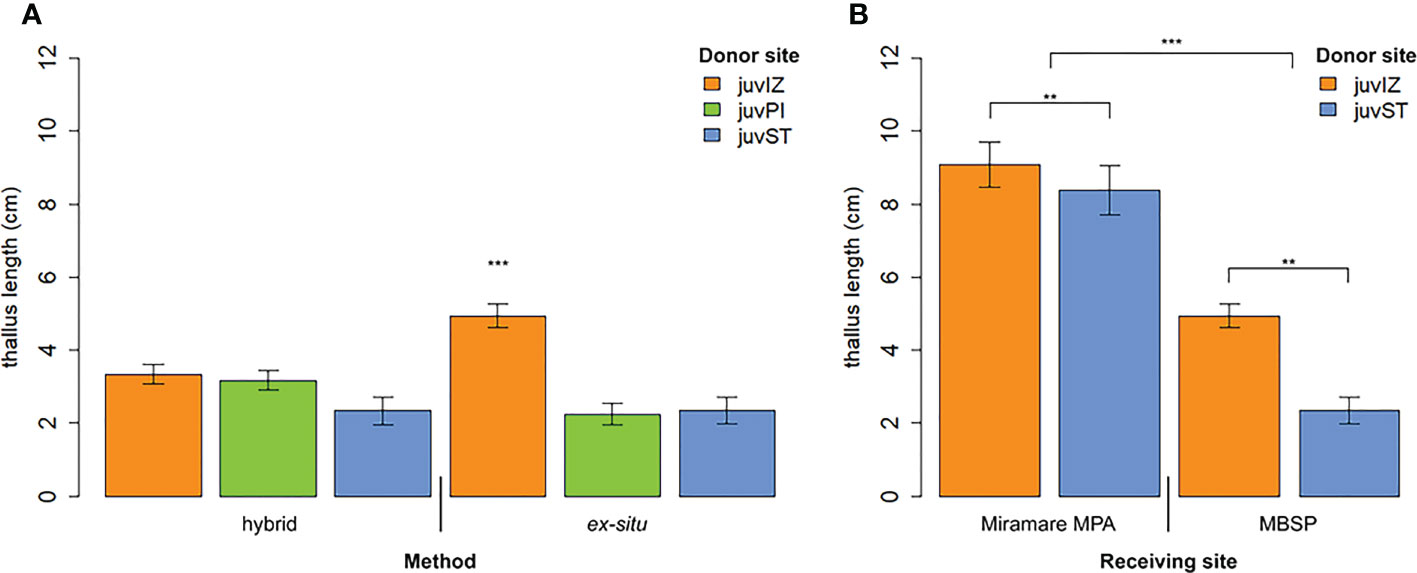
Figure 7 Thallus length (mean ± SE) after 7 months in the field (November 2021) (A) comparing juvIZ, juvST and juvPI and the two outplanting methods at the receiving site in front of the MBSP; (B) comparing juvIZ and juvST in the two receiving sites (Miramare and MBSP) for the ex situ method. Significance levels: ‘***’ p-value < 0.001, ‘**’ p-value < 0.01.
Significant differences were observed in the ex situ method between the two receiving sites: thalli of both donor populations outplanted in Miramare MPA showed significantly higher performances compared to the ones in MBSP (p-value <0.0001, Figure 7B, Table 4). In both receiving sites, juvIZ performed significantly better than juvST (p-value=0.007, Figure 7B, Table 4). At 7 months (November 2021), the average length of thalli outplanted by the ex situ method at Miramare MPA ranged from 8.4 ± 0.7 SE (juvST) to 9.1 ± 0.6 SE cm (juvIZ), while at MBSP it ranged from 2.3 ± 0.4 SE (juvST) to 4.9 ± 0.3 SE cm (juvIZ).
It was not possible to compare the two methods in the Miramare MPA receiving site at 7 months because after only 3 months in the field (July 2021), a lower length (0.3 ± 0.1 SE – juvIZ; 0.5 ± 0.1 SE cm – juvST) was observed and in August (i.e., after 1 month on the sea bottom) juvenile mortality was 100%. For this reason, monitoring of the hybrid method was interrupted at this site.
The extremely low p-values of the statistical tests (always lower than 0.001) allowed us to consider our results reliable even acknowledging that some of the replicates should be considered pseudoreplicated in space.
In front of the MBSP, the percentage of tiles with germlings (% survival) ranged from 58% (juvST), 70% (juvIZ) and 90% (juvPI) for the ex situ method, while for the hybrid method it was 41% for juvST, 83% for juvIZ and 100% for juvPI (Figure 8A). At Miramare MPA, the percentage of tiles with germlings in November 2021 ranged from 44% (juvST) to 78% (juvIZ) for the ex situ, while it was 0% for the hybrid method for both juvIZ and juvST (Figure 8B).
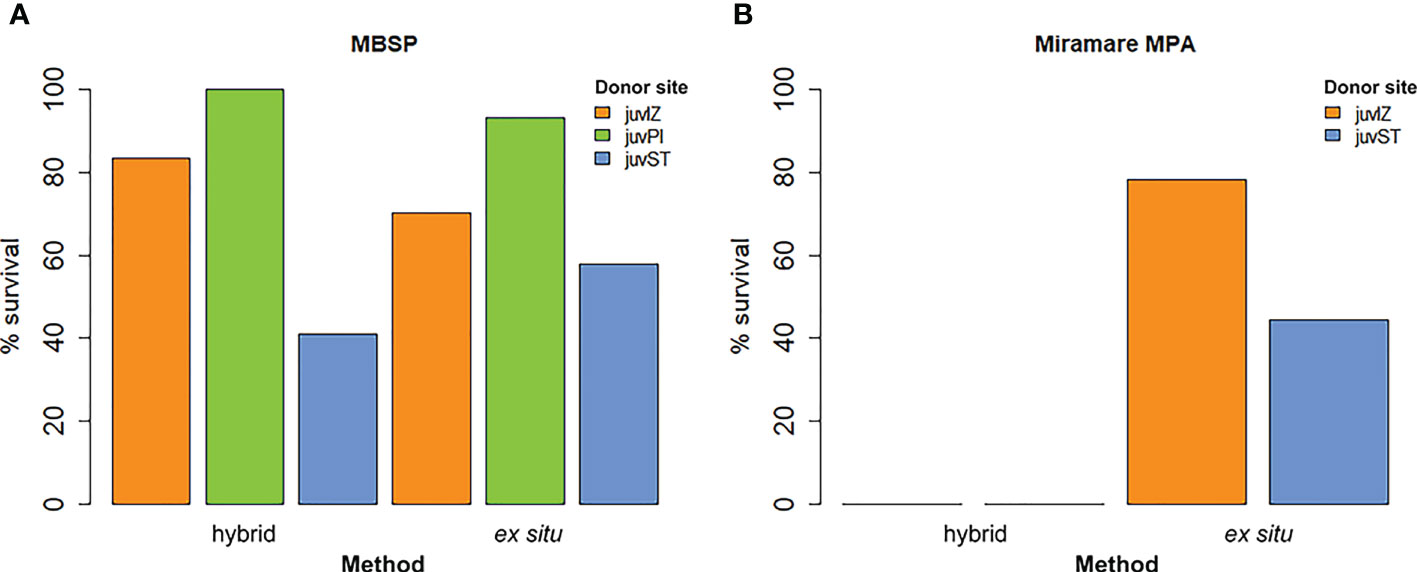
Figure 8 Percentage survival data in the hybrid and ex situ method in November 2021 in the receiving sites (A) in front of the MBSP and (B) in Miramare MPA.
Discussion
For restoration, it is important to gain a deeper knowledge of the phenology of the species to be restored. In the last forty years, many studies have investigated the phenology of Cystoseira spp., highlighting that they usually undergo both morphological and reproductive changes during the year (e.g., Gómez-Garreta et al., 1982; Hoffmann et al., 1992; Alongi et al., 1999; Marzocchi et al., 2003; Falace et al., 2005; Falace and Bressan, 2006; Medrano et al., 2020a). Nevertheless, few studies focused on phenotypic variation among populations of fucoid species and also reported significant differences in reproductive traits (De Paula and De Oliveira, 1982; Silva et al., 2004; Sadogurska et al., 2021).
Within its geographical range, G. barbata shows considerable ecological plasticity and outstanding morphological variability due to environmental factors (Ercegović, 1952; Falace and Bressan, 2006). In the present study, we found relevant differences in the shape and size of apical fronds and the number of aerocysts between populations of G. barbata from Izola and those from Strunjan and Piran (Tables 2 and 3): long spindle-shaped receptacles, bearing aerocysts and always ending with a mucron for the population from Izola (Falace et al., 2005; Falace and Bressan, 2006), while the populations from Strunjan and Piran had short cylindrical receptacles bearing almost no aerocysts and not always mucronated (Orlando-Bonaca et al., 2021b).
In the Black Sea, Sadogurska et al. (2021) reported that this variability might depend on the hydrodynamic regime and season and observed two main morphologies. At sheltered sites, G. barbata had long, sickle-shaped receptacles, sometimes with protruding conceptacles, and numerous concatenated aerocysts. In contrast, on exposed shores, G. barbata had small, oval or spindle-shaped receptacles with smooth surfaces and few aerocysts. Nevertheless, molecular analyses revealed minor differences between the Black Sea samples of G. barbata, representing smaller infraspecific variations. Sadogurska et al. (2021) therefore concluded that the morphological variability of G. barbata in the Black Sea is not due to infraspecific taxa, but to considerable ecological plasticity and seasonal variation. Consequently, the populations sampled for the present study could represent two different ecotypes.
Although the donor sites on the Slovenian coast are close to each other (see Materials and methods), they have different environmental characteristics. The Izola donor site is located at the edge of Koper Bay, which is characterised by high sedimentation and suspension rates reflected in increased water turbidity (Ogorelec et al., 1991). The area is exposed to large freshwater inputs from the Rižana and Badaševica rivers (Cozzi et al., 2012) and is located close to the port of Koper. In addition, some unidentified sources of pollution (probably municipal sewage) affect the ecological status of macroalgae in this area (Orlando-Bonaca and Rotter, 2018; Orlando-Bonaca et al., 2021c). In contrast, Strunjan and Piran are exposed to lower anthropogenic pressures (Orlando-Bonaca et al., 2015), as they are located on a coastline that is still in a pristine state. Moreover, these two sites are located outside the bays of Koper and Piran, which are known to have the highest sedimentation and suspension rates in Slovenian marine waters (Ogorelec et al., 1991). Consequently, the three donor populations might be adapted to different sedimentation rates, salinity, and turbidity, affecting the length of receptacles and the presence of aerocysts. In environments with high water turbidity, the development of aerocysts stimulates the algae to stretch upwards and in this way improve their access to light (Lüning, 1991). The elongated shape of the receptacles could be another adaptation to increase surface area and better capture light to improve photosynthesis. Therefore, the Izola population could be a morphotype adapted to higher sedimentation.
The fertile apices of the Izola population not only had longer and larger receptacles (Figure 3), but also a higher number of conceptacles per receptacle (RP, Figure 4A; Table 2). However, the number of zygotes per tile relative to the number of conceptacles per tile (RS) was higher in the G. barbata population from Strunjan (Figure 4B; Table 2). Furthermore, the density of juvIZ and juvPI was lower than that of juvST after one (T1) and two weeks of culture (T2) (Figure 5). Nevertheless, the progressive decrease in seedling density over time (T4), which can be explained at least in part by the process of “self-thinning” of a growing plant population (Ang and De Wreede, 1992; Steen and Scrosati, 2004), was more pronounced in juvST. Not only the density but also the dimensions of juvST were smaller than those of juvIZ at T4 (Figure 6). This result indicates that the population from Strunjan, although more successful in the first two weeks of culture, was less successful than the others after three to four weeks.
The success of outplanting juveniles in the field was highly variable and depended on both the location of the donor/receiving sites and the outplanting method. As with the ex situ method, the greatest differences in Slovenia (MBSP) were due to the different donor sites. This is because although all experimental tiles were exposed to the same environmental conditions (Supplement 2), juvIZ thalli grew significantly better than juvST and juvPI (Figure 7A). This was probably due to pre-adaptation to the high sediment resuspension rate characteristic of this receiving site. The MBSP receiving site is located at the edge of Piran Bay, which has a similar water turbidity, suspension and sedimentation rate as the Izola donor site at the edge of Koper Bay (Ogorelec et al., 1991). In addition, the tiles were placed near an underwater pipe, to prevent the protective cages from being damaged by fishermen’s trawls, as the site is not a protected area. However, during monitoring, it was found that the proximity of the pipe reduces hydrodynamics and exposes juvenile thalli to higher sediment resuspension during growth. This may have favoured the growth of juvIZ, which are adapted to similar environmental conditions, and disadvantaged juvST and juvPI. Indeed, sedimentation may be one of the most important factors affecting the survival and development of early life stages of macroalgae (Vadas et al., 1992; Schiel and Foster, 2006; Irving et al., 2009). In addition, the lower frequency of cage cleaning in the MBSP site and the resulting lower light experienced by the juveniles under the cages may have contributed to their slower growth.
For the hybrid method, the main differences were due to the receiving site: the method performed much better at MBSP than at Miramare (Figure 8). As this method performed better at Miramare in 2019 (Savonitto et al., 2021), these poorer results could be related to a series of unfavourable environmental conditions that occurred in the Italian part of the Gulf of Trieste in spring and early summer 2021. An impressive bloom of the jellyfish Rhizostoma pulmo, followed by a massive bloom of Noctiluca scintillans, which was much more pronounced in the Italian waters than in the Slovenian ones (ARPA FVG, 2021), characterised the area during the laying of the tiles on the lantern nets and in the following crucial weeks (Supplement 3). In addition, the tiles on the lantern nets were colonised by mussel and oyster recruits from June onwards, and this trend intensified in July (i.e., at the time of outplanting on the sea bottom), favoured by a mussel farm near the MPA that probably served as a larval source. Thus, the molluscs probably displaced the G. barbata seedlings as already observed in Cystoseira populations (Gros, 1978; Benedetti-Cecchi et al., 1996; Thibaut et al., 2005; Mačić et al., 2010; Perkol-Finkel and Airoldi, 2010). Nevertheless, the satisfactory results of the hybrid method at MBSP support the pilot application reported by Savonitto et al. (2021). At MBSP, the lantern net allowed us to successfully reduce the culture time required for seedlings to reach a “refuge size,” and it also seemed to limit the presence of mesograzers, which typically occur on vegetated rocky bottoms. In addition, this intermediate step of keeping seedlings in suspended culture in the sea prior to final outplanting on the seafloor reduced the risk of bacterial or microalgal outbreaks, which are common with prolonged culture in mesocosms (Orlando-Bonaca et al., 2021b; Lardi et al., 2022). Nevertheless, it should be further tested in Slovenian coastal waters to improve the outcomes in terms of thalli growth.
Shortened times in mesocosms correspond to reduced maintenance and overall costs, which is necessary for scaling up efforts beyond a purely experimental scale, as is now required by international policies and standards (Gann et al., 2019).
Controlling grazers with protective cages improved overall outplanting performance, as it has already been shown that the loss of a protective cage for only one week resulted in significant overgrazing of juveniles by herbivorous fish (Orlando-Bonaca et al., 2021b). S. salpa, which is very abundant in the Miramare MPA and also along the Slovenian coast, is most likely the main predator of G. barbata recruits in the Gulf of Trieste, as schools were regularly observed near the experimental plots during monitoring by divers and with the camera also in 2021 (authors’ pers. obs.). Conversely, the influence of grazing by sea urchins and mesograzers (e.g., molluscs, crustaceans) was likely negligible, as our underwater observations showed. In any case, thalli cannot be kept in a cage indefinitely. In spring 2022, the cage that had protected thalli outplanted in spring 2020 (Orlando-Bonaca et al., 2021b) was removed, and after several months without protection, thalli were bitten at the tips but were at least 30 cm long overall (unpublished data). In contrast, the sharp decline of macroalgae in the Miramare MPA has not only affected Cystoseira, as the system has “tipped” into an alternative state dominated by turf algae and other grazing-resistant species (e.g., Dictyota spp., crustose calcareous Rhodophytes; Falace et al., 2010; Bevilacqua et al., 2022). Reversing this state would require enormous effort and the constant maintenance of grazing protection, with great uncertainty about the long-term viability of transplanted stands.
Our results suggest that the characteristics of the donor site may confer higher fitness to the cultured seedlings when they are in the field, as shown by the higher growth of juvIZ under sediment resuspension in front of the MBSP.
Despite its late start compared to restoration on land, marine restoration holds enormous potential as an important tool for developing strategies to ensure that the oceans continue to provide the goods and services on which the well-being of life on Earth depends (Danovaro et al., 2021). The results of this study suggest that outplanting of infralittoral canopy-forming species such as G. barbata in the northern Adriatic should be further tested and improved, at least on the Slovenian coasts of the Gulf of Trieste. Although the cultivation of Cystoseira is already consolidated, albeit with room for improvement (Falace et al., 2018; Verdura et al., 2018; De La Fuente et al., 2019b; Savonitto et al., 2019; Savonitto et al., 2021; Orlando-Bonaca et al., 2021b; Lardi et al., 2022), positive results from the laboratory phase do not guarantee success in outplanting at sea. Successful reestablishment of brown algal forests, even in MPAs, is influenced by factors other than nutrient enrichment and other pollutants, some of which cannot be controlled [i.e., climate change-related trends and events (Grbec et al., 2018), complex and unpredictable environmental dynamics such as jellyfish or N. scintillans blooms (ARPA FVG, 2021)] and others that are more measurable/controllable, such as overgrazing by herbivorous fishes (Gianni et al., 2017). Since environmental conditions influenced by both natural and anthropogenic forces appear to play a critical role in restoration effectiveness, monitoring these variables in donor and receiving sites prior to implementing restoration activities can help determine the best location for restoration and/or predict restoration success. As protection is easier and cheaper than restoration, herbivore regulation (to reduce herbivore density) is extremely urgent and should be planned and implemented on a larger regional scale to save not only brown algal forests, but also other endangered infralittoral vegetation and the habitat/biota they sustain.
Data availability statement
The raw data supporting the conclusions of this article will be made available by the authors, without undue reservation.
Author contributions
MO-B: Conceptualization, Investigation, Data curation, Methodology, Writing - original draft, Writing - review & editing. GS: Conceptualization, Investigation, Data curation, Methodology, Formal analysis, Writing - original draft, Writing - review & editing. VA: Data curation, Methodology, Formal analysis, Writing - original draft, Writing - review & editing. DT: Conceptualization, Investigation, Writing - review & editing. VP: Conceptualization, Investigation, Writing - review & editing. MŠ: Investigation, Writing - review & editing. TM: Investigation, Writing - review & editing. PS: Investigation, Writing - review & editing. AL: Investigation, Writing - review & editing. SC: Conceptualization, Investigation, Writing - review & editing. MC: Writing - review & editing. SK: Investigation, Writing - review & editing. ED: Investigation, Writing - review & editing. MS: Investigation, Writing - review & editing. AF: Conceptualization, Investigation, Data curation, Methodology, Writing - original draft, Writing - review & editing. All authors contributed to the article and approved the submitted version.
Funding
The Slovenian authors acknowledge the project (Factors affecting Adriatic brown algal forests and solutions for habitat restoration, J1-1702) was financially supported by the Slovenian Research Agency. The activities performed by the Italian authors were financed by the LIFE financial instrument of the European Community, project ROC-POP-LIFE (LIFE16 NAT/IT/000816).
Acknowledgments
The Slovenian authors would like to thank Lovrenc Lipej, Leon Lojze Zamuda, Borut Mavrič, Ana Fortič and Tristan Bartole for their help during the fieldwork. All authors thank the reviewers and the editor for their careful review of the manuscript and their constructive comments and suggestions.
Conflict of interest
The authors declare that the research was conducted in the absence of any commercial or financial relationships that could be construed as a potential conflict of interest.
Publisher’s note
All claims expressed in this article are solely those of the authors and do not necessarily represent those of their affiliated organizations, or those of the publisher, the editors and the reviewers. Any product that may be evaluated in this article, or claim that may be made by its manufacturer, is not guaranteed or endorsed by the publisher.
Supplementary material
The Supplementary Material for this article can be found online at: https://www.frontiersin.org/articles/10.3389/fmars.2022.988584/full#supplementary-material
Supplement 1 | (A) Receptacles of Gongolaria barbata with several conceptacles, (B) ostioles, (C) the circles indicate the ostioles. The reproductive potential was assessed by counting the number of ostioles per receptacle, as each ostiole is considered as a proxy of a conceptacle; (D) the ostiole allows eggs and/or zygotes to emerge from the conceptacle.
Supplement 2 | Sea temperature and light intensity measured on experimental plates in front of the MBSP from May to December 2021. Trends in sea temperature and light intensity did not deviate significantly, apart from one event. On 18 and 19 July, isolated thunderstorms with showers and north-easterly winds were recorded, leading to a decrease in water temperature.
Supplement 3 | Unfavourable environmental dynamics that characterized the Italian part of the Gulf of Trieste (northern Adriatic) in spring 2021. (A) Rhizostoma pulmo on a lantern net in the Miramare MPA (credits: Marco Segarich); (B) Jellyfish bloom in Trieste, Italy (credits: Repubblica; https://www.repubblica.it/green-and-blue/2022/04/26/news/bloom_meduse_trieste_riscaldamento_mare_pesca_intensiva-346958254/); Noctiluca scintillans bloom (C) next to Miramare MPA (credits: Area Marina Protetta Miramare) and d) inside the MPA, on the experimental plots (credits: Legambiente FVG; https://www.legambientefvg.it/component/content/article/2-uncategorised/2411-i-complessi-equilibri-degli-ecosistemi-marini-che-succede-nel-nostro-golfo?Itemid=337).
References
Abelson A., Reed D. C., Edgar G. J., Smith C. S., Kendrick G. A., Orth R. J., et al. (2020). Challenges for restoration of coastal marine ecosystems in the anthropocene. Front. Mar. Sci. 7, 544105. doi: 10.3389/fmars.2020.544105
Alberto F., Raimondi P., Reed D. (2010). Habitat continuity and geographic distance predict population genetic differentiationin giant kelp. Ecology 91, 49–56. doi: 10.1890/09-0050.1
Alongi G., Catra M., Cormaci M. (1999). Fenologia morfologica e riproduttiva di Cystoseira elegans sauvageau (Cystoseiraceae, phaeophyta). Bollett. Accad. Gioenia Sci. Nat. Catania 31, 189–200.
Ang P. O. Jr., De Wreede R. E. (1992). Density-dependence in a population of Fucus distichus. Mar. Ecol. Prog. Ser. 90 (2), 169–181. doi: 10.3354/meps090169
ARPA FVG (2021) Fioritura di "Noctiluca scintillans" e le acque del golfo si colorano di rosso. Available at: https://www.arpa.fvg.it/temi/temi/acqua/news/fioritura-di-noctiluca-scintillans-e-le-acque-del-golfo-si-colorano-di-rosso/ (Accessed 13 June 2022).
Assis J., Fragkopoulou E., Neiva J., Abecasis D., Faugeron S., Serrão E. A. (2020). A fine-tuned global distribution dataset of marine forests. Sci. Data 7, 1–9. doi: 10.1038/s41597-020-0459-x
Ballesteros E. (1989). Production of seaweeds in northwestern Mediterranean marine communities: its relation with environmental factors. Sci. Mar. 53 (2–3), 357–364. Available at: https://digital.csic.es/handle/10261/28562.
Ballesteros E., Garrabou J., Hereu B., Zabala M., Cebrian E., Sala E. (2009). Deep-water stands of Cystoseira zosteroides c. agardh (Fucales, ochrophyta) in the northwestern Mediterranean: insights into assemblage structure and population dynamics. Estuar. Coast. Shelf Sci. 82, 477–484. doi: 10.1016/j.ecss.2009.02.013
Ballesteros E., Sala E., Garrabou J., Zabala M. (1998). Community structure and frond size distribution of a deep water stand of Cystoseira spinosa (Phaeophyta) in the northwestern Mediterranean. Eur. J. Phycol. 33 (2), 121–128. doi: 10.1080/09670269810001736613
Benedetti-Cecchi L., Nuti S., Cinelli F. (1996). Analysis of spatial and temporal variability in interactions among algae, limpets and mussels in low-shore habitats on the west coast of Italy. Mar. Ecol. Prog. Ser. 144, 87–96. doi: 10.3354/meps144087
Bernal-Ibáñez A., Gestoso I., Wirtz P., Kaufmann M., Serrão E. A., Canning-Clode J., et al. (2021). The collapse of marine forests: drastic reduction in populations of the family sargassaceae in Madeira island (NE Atlantic). Reg. Environ. Change 21, 71. doi: 10.1007/s10113-021-01801-2
Bevilacqua S., Vellani V., Fabbrizio P., Falace A., Ciriaco S., Segarich M., et al. (2022). Multidecadal monitoring highlighted long-term stability of protected assemblages within a Mediterranean marine reserve. Estuar. Coast. Shelf Sci. 274, 107946. doi: 10.1016/j.ecss.2022.107946
Bianchelli S., Buschi E., Danovaro R., Pusceddu A. (2016). Biodiversity loss and turnover in alternative states in the Mediterranean Sea: a case study on meiofauna. Sci. Rep. 6, 34544. doi: 10.1038/srep34544
Boicourt W. C., Ličer M., Li M., Vodopivec M., Malačič V. (2021). “Sea State: recent progress in the context of climate change,” in Coastal ecosystems in transition: a comparative analysis of the northern Adriatic and Chesapeake bay. geophysical monograph series. Eds. Malone T., Malej A., Faganeli J. (Hoboken, NJ: American Geophysical Union: Wiley), 21–48. doi: 10.1002/9781119543626.ch3
Boudouresque C. F., Lück H. B. (1972). Recherches de bionomie structurale au niveau d'un peuplement benthique sciaphile. J. Exp. Mar. Biol. Ecol. 8 (2), 133–144. doi: 10.1016/0022-0981(72)90016-0
Bringloe T. T., Starko S., Wade R. M., Vieira C., Kawai H., De Clerck O., et al. (2020). Phylogeny and evolution of the brown algae. Crit. Rev. Plant Sci. 39 (4), 281–321. doi: 10.1080/07352689.2020.1787679
Bruno de Sousa C., Gangadhar K. N., Macridachis J., Pavão M., Morais T. R., Campino L., et al. (2017a). Cystoseira algae (Fucaceae): update on their chemical entities and biological activities. Tetrahedron: Asymmetry 28, 1486–1505. doi: 10.1016/j.tetasy.2017.10.014
Bruno de Sousa C., Gangadhar K. N., Morais T. R., Conserva G. A., Vizetto-Duarte C., Pereira H., et al. (2017b). Antileishmanial activity of meroditerpenoids from the macroalgae Cystoseira baccata. Exp. Parasitol. 174, 1–9. doi: 10.1016/j.exppara.2017.01.002
Bulleri F., Benedetti-Cecchi L., Cusson M., Maggi E., Arenas F., Aspden R., et al. (2012). Temporal stability of European rocky shore assemblages: variation across a latitudinal gradient and the role of habitat-formers. Oikos 121, 1801–1809. doi: 10.1111/j.1600-0706.2011.19967.x
Catra M., Alongi G., Leonardi R., Negri M., Sanfilippo R., Sciuto F., et al. (2019). Degradation of a photophilic algal community and its associated fauna from eastern Sicily (Mediterranean Sea). Mediterr. Mar. Sci. 20 (1), 74–89. doi: 10.12681/mms.17765
Cheminée A., Sala E., Pastor J., Bodilis P., Thiriet P., Mangialajo L., et al. (2013). Nursery value of Cystoseira forests for Mediterranean rocky reef fishes. J. Exp. Mar. Biol. Ecol. 442, 70–79. doi: 10.1016/j.jembe.2013.02.003
Clayton M. N. (1990). The adaptive significance of life history characters in selected orders of marine brown macroalgae. Aust. J. Ecol. 15, 439–452. doi: 10.1111/j.1442-9993.1990.tb01469.x
Cozzi S., Falconi C., Comici C., Čermelj B., Kovač N., Turk V., et al. (2012). Recent evolution of river discharges in the gulf of Trieste and their potential response to climate changes and anthropogenic pressure. Estuar. Coast. Shelf Sci. 115, 14–24. doi: 10.1016/j.ecss.2012.03.005
Danovaro R., Aronson J., Cimino R., Gambi C., Snelgrove P. V. R., Van Dover C. (2021). Marine ecosystem restoration in a changing ocean. Restor. Ecol. 29 (S2), e13432. doi: 10.1111/rec.13432
De La Fuente G., Asnaghi V., Chiantore M., Thrush S., Povero P., Vassallo P., et al. (2019a). The effect of Cystoseira canopy on the value of midlittoral habitats in NW Mediterranean, an emergy assessment. Ecol. Modell. 404, 1–11. doi: 10.1016/j.ecolmodel.2019.04.005
De La Fuente G., Chiantore M., Asnaghi V., Kaleb S., Falace A. (2019b). First ex situ outplanting of the habitat-forming seaweed Cystoseira amentacea var. stricta from a restoration perspective. PeerJ 7, e7290. doi: 10.7717/peerj.7290
De La Fuente G., Fontana M., Asnaghi V., Chiantore M., Mirata S., Salis A., et al. (2020). The remarkable antioxidant and anti-inflammatory potential of the extracts of the brown alga Cystoseira amentacea var. stricta. Mar. Drugs 19 (1), 2. doi: 10.3390/md19010002
De Paula E. J., De Oliveira E. C. (1982). Wave exposure and ecotypical differentiation in Sargassum cymosum (Phaeophyta – fucales). Phycologia 21 (2), 145–153. doi: 10.2216/i0031-8884-21-2-145.1
Ercegović A. (1952). “Jadranske cistozire,” in Sur les cystoseira adriatiques (Split: Institut za Oceanografiju i Ribarstvo), 212.
Falace A., Alongi G., Cormaci M., Furnari G., Curiel D., Cecere E., et al. (2010). Changes in the benthic algae along the Adriatic Sea in the last three decades. Chem. Ecol. 26 (S1), 77–90. doi: 10.1080/02757541003689837
Falace A., Bressan G. (2006). Seasonal variations of Cystoseira barbata (Stackhouse) c. agardh frond architecture. Hydrobiologia 555 (1), 193–206. doi: 10.1007/s10750-005-1116-2
Falace A., Kaleb S., de la Fuente G., Asnaghi V., Chiantore M. (2018). Ex situ cultivation protocol for Cystoseira amentacea var. stricta (Fucales, phaeophyceae) from a restoration perspective. PloS One 13 (2), e0193011. doi: 10.1371/journal.pone.0193011
Falace A., Zanelli E., Bressan G. (2005). Morphological and reproductive phenology of Cystoseira compressa (Esper) gerloff & nizamuddin (Fucales, fucophyceae) in the gulf of Trieste (North Adriatic Sea). Annales Ser. Hist. Nat. 15 (1), 71–78. Available at: http://www.dlib.si/details/URN:NBN:SI:doc-QTHLRV24.
Falace A., Zanelli E., Bressan G. (2006). Algal transplantation as a potential tool for artificial reef management and environmental mitigation. Bull. Mar. Sci. 8, 161–166. Available at: https://www.webofscience.com/wos/WOSCC/full-record/000235074300014WOS:000235074300014
Gann G. D., McDonald T., Walder B., Aronson J., Nelson C. R., Jonson J., et al. (2019). International principles and standards for the practice of ecological restoration. Restor. Ecol. 27, S1–S46. doi: 10.1111/rec.13035
Giaccone G. (1973). Écologie et chorologie des Cystoseira de méditerranée. Rapp. Commun. Int. Mer Médit. 22, 49–50.
Gianni F., Bartolini F., Airoldi L., Ballesteros E., Francour P., Guidetti P., et al. (2013). Conservation and restoration of marine forests in the Mediterranean Sea and the potential role of marine protected areas. Adv. Oceanogr. Limnol. 4 (2), 83–101. doi: 10.1080/19475721.2013.845604
Gianni F., Bartolini F., Pey A., Laurent N., Martins G. M., Airoldi L., et al. (2017). Threats to large brown algal forests in temperate seas: The overlooked role of native herbivorous fish. Sci. Rep. 7, 6012. doi: 10.1038/s41598-017-06394-7
Gómez-Garreta M., Ribera Siguán M. A., Seoane Camba J. A. (1982). Estudio fenológico de varias especies del género Cystoseira en mallorca. Collect. Bot. 13 (2), 841–855.
Grbec B., Matić F., Beg Paklar G., Morović M., Popović R., Vilibić I. (2018). Long-term trends, variability and extremes of in situ sea surface temperature measured along the Eastern Adriatic coast and its relationship to hemispheric processes. Pure Appl. Geophys. 175, 4031–4046. doi: 10.1007/s00024-018-1793-1
Gros C. (1978). “Le genre cystoseira sur la côte des albères,” in Répartition - Écologie - morphogenèse (Université Pierre et Marie Curie, Paris: PhD Thesis).
Hoffmann L., Renard R., Demoulin V. (1992). Phenology, growth and biomass of Cystoseira balearica in calvi (Corsica). Mar. Ecol. Progr. Ser. 80 (2), 249–254. doi: 10.3354/meps080249
Irving A. D., Balata D., Colosio F., Ferrando G. A., Airoldi L. (2009). Light, sediment, temperature, and the early life-history of the habitat-forming alga Cystoseira barbata. Mar. Biol. 156 (6), 1223–1231. doi: 10.1007/s00227-009-1164-7
Iveša L., Djakovac T., Devescovi M. (2016). Long-term fluctuations in Cystoseira populations along the west istrian coast (Croatia) related to eutrophication patterns in the northern Adriatic Sea. Mar. pollut. Bull. 106 (1–2), 162–173. doi: 10.1016/j.marpolbul.2016.03.010
Lardi P. I., Varkitzi I., Tsiamis K., Orfanidis S., Koutsoubas D., Falace A., et al. (2022). Early development of Gongolaria montagnei (Fucales, phaeophyta) germlings under laboratory conditions, with a view to enhancing restoration potential in the Eastern Mediterranean. Bot. Mar. 65 (4), 279–287. doi: 10.1515/bot-2021-0105
Lüning K. (1991). Seaweeds: Their environment, biogeography, and ecophysiology (Hoboken, NJ: Wiley Interscience publication. John Wiley & Son, Inc.) 527.
Mačić V., Antolic B., Thibaut T., Svirčev Z. (2010). Distribution of the most common Cystoseira c. agardh species (Heterokontophyta, fucales) on the coast of Montenegro (south-east Adriatic sea). Fresenius Environ. Bull. 19 (6), 1191–1198. Available at: https://hal.archives-ouvertes.fr/hal-00927240.
Mačić V., Svirčev Z. (2014). Macroepiphytes on Cystoseira species (Phaeophyceae) on the coast of Montenegro. Fresenius Environ. Bull. 23 (1), 29–34. Available at: https://www.scopus.com/record/display.uri?eid=2-s2.0-84896913751&origin=inward&txGid=75e02f797b95afbd8db2004f018f608d.
Mancuso F. P., Milazzo M., Chemello R. (2021). Decreasing in patch-size of Cystoseira forests reduces the diversity of their associated molluscan assemblage in Mediterranean rocky reefs. Estuar. Coast. Shelf Sci. 250, 107163. doi: 10.1016/j.ecss.2020.107163
Mangialajo L., Chiantore M., Cattaneo-Vietti R. (2008). Loss of fucoid algae along a gradient of urbanisation, and structure of benthic assemblages. Mar. Ecol. Progr. Ser. 358, 63–74. doi: 10.3354/meps07400
Mariani S., Cefalì M. E., Chappuis E., Terradas M., Pinedo S., Torras X., et al. (2019). Past and present of fucales from shallow and sheltered shores in Catalonia. Reg. Stud. Mar. Sci. 32, 100824. doi: 10.1016/j.rsma.2019.100824
Marzocchi M., Curiel D., Dri C., Scattolin M. (2003). Fenologia morfologica e riproduttiva di Cystoseira barbata (Stackhouse) c. agardh var. barbata (Fucales, fucophyceae) nella laguna di venezia (Nord adriatico). Lav. Soc Ven. Sc. Nat. 28, 21–23.
Medrano A., Hereu B., Cleminson M., Pagès-Escolà M., la Rovira G., Solà J., et al. (2020a). From marine deserts to algal beds: Treptacantha elegans revegetation to reverse stable degraded ecosystems inside and outside a no-take marine reserve. Rest. Ecol. 28 (3), 632–644. doi: 10.1111/rec.13123
Medrano A., Hereu B., Mariani S., Neiva J., Paulino C., Rovira G., et al. (2020b). Ecological traits, genetic diversity and regional distribution of the macroalga Treptacantha elegans along the Catalan coast (NW Mediterranean Sea). Sci. Rep. 10 (1), 1–10. doi: 10.1038/s41598-020-76066-6
Mhadhebi L., Laroche-Clary A., Robert J., Bouraoui A. (2011). Anti-inflammatory, antiproliferative and antioxidant activities of organic extracts from the Mediterranean seaweed, Cystoseira crinita. Afr. J. Biotechnol. 10, 16682–16690. doi: 10.5897/AJB11.218
Millar R. B., Anderson M. J. (2004). Remedies for pseudoreplication. Fish. Res. 70 (2-3), 397–407. doi: 10.1016/j.fishres.2004.08.016
Molinari-Novoa E., Guiry M. (2020). Reinstatement of the genera Gongolaria boehmer and Ericaria stackhouse (Sargassaceae. phaeophyceae). Not. Algarum 172, 1–10.
Mozetič P., Francé J., Kogovšek T., Talaber I., Malej A. (2012). Plankton trends and community changes in a coastal sea (northern adriatic): bottom-up vs. top-down control in relation to environmental drivers. Estuar. Coast. Shelf Sci. 115, 138–148. doi: 10.1016/j.ecss.2012.02.009
Munda I. M. (2000). Long-term marine floristic changes around rovinj (Istrian coast, north Adriatic) estimated on the basis of historical data from Paul kuckuck's field diaries from the end of the 19th century. Nova Hedwigia 71 (1–2), 1–36. doi: 10.1127/nova/71/2000/1
Ogorelec B., Mišič M., Faganeli J. (1991). Marine geology of the gulf of Trieste (northern adriatic): Sedimentological aspects. Mar. Geol. 99, 79–92. doi: 10.1016/0025-3227(91)90084-H
Orfanidis S., Rindi F., Cebrian E., Fraschetti S., Nasto I., Taskin E., et al. (2021). Effects of natural and anthropogenic stressors on fucalean brown seaweeds across different spatial scales in the Mediterranean Sea. Front. Mar. Sci. 8. doi: 10.3389/fmars.2021.658417
Orlando-Bonaca M., Francé J., Mavrič B., Grego M., Lipej L., Flander-Putrle V., et al. (2015). A new index (MediSkew) for the assessment of the Cymodocea nodosa (Ucria) ascherson meadow's status. Mar. Environ. Res. 110, 132–141. doi: 10.1016/j.marenvres.2015.08.009
Orlando-Bonaca M., Lipej L. (2005). Factors affecting habitat occupancy of fish assemblage in the gulf of Trieste (Northern Adriatic Sea). Mar. Ecol. 26 (1), 42–53. doi: 10.1111/j.1439-0485.2005.00037.x
Orlando-Bonaca M., Mavrič B. (2014). Recurrence of Sargassum vulgare c. agardh in Slovenian coastal waters (Adriatic sea). annales. Ser. Hist. Nat. 24 (2), 109–114. Available at: https://www.dlib.si/details/URN:NBN:SI:doc-WAXUZLDP.
Orlando-Bonaca M., Pitacco V., Bajt O., Falnoga I., Jagodic Hudobivnik M., Mazej D., et al. (2021c). Spatial and temporal distribution of trace elements in Padina pavonica from the northern Adriatic Sea. Mar. pollut. Bull. 172, 112874. doi: 10.1016/j.marpolbul.2021.112874
Orlando-Bonaca M., Pitacco V., Lipej L. (2021a). Loss of canopy-forming algal richness and coverage in the northern Adriatic Sea. Ecol. Indic. 125, 107501. doi: 10.1016/j.ecolind.2021.107501
Orlando-Bonaca M., Pitacco V., Slavinec P., Šiško M., Makovec T., Falace A. (2021b). First restoration experiment for Gongolaria barbata in Slovenian coastal waters. what can go wrong? Plants 10 (2), 239. doi: 10.3390/plants10020239
Orlando-Bonaca M., Rotter A. (2018). Any signs of replacement of canopy-forming algae by turf-forming algae in the northern Adriatic Sea? Ecol. Indic. 87, 272–284. doi: 10.1016/j.ecolind.2017.12.059
Otero-Schmitt J., Pérez-Cirera J. L. (1996). Epiphytism on Cystoseira (Fucales, phaeophyta) from the Atlantic coast of northwest Spain. Bot. Mar. 39(1–6), 445–465. doi: 10.1515/botm.1996.39.1-6.445
Peleg O., Guy-Haim T., Yeruham E., Silverman J., Rilov G. (2020). Tropicalisation may invert trophic state and carbon budget of shallow temperate rocky reefs. J. Ecol. 108 (3), 884–854. doi: 10.1111/1365-2745.13329
Perkol-Finkel S., Airoldi L. (2010). Loss and recovery potential of marine habitats: an experimental study of factors maintaining resilience in subtidal algal forests at the Adriatic sea. PloS One 5 (5), e10791. doi: 10.1371/journal.pone.0010791
Pitacco V., Orlando-Bonaca M., Mavrič B., Popovič A., Lipej L. (2014). Mollusc fauna associated with the Cystoseira algal associations in the gulf of Trieste (northern Adriatic Sea). Medit. Mar. Sci. 15 (2), 225–238. doi: 10.12681/mms.466
Pizzuto F. (1999). On the structure, typology and periodism of a Cystoseira brachycarpa j. agardh emend. giaccone community and of a Cystoseira crinita duby community from the eastern coast of Sicily (Mediterranean Sea). Plant Biosyst. 133 (1), 15–35. doi: 10.1080/11263509909381529
Rindi L., Dal Bello M., Benedetti-Cecchi L. (2018). Experimental evidence of spatial signatures of approaching regime shifts in macroalgal canopies. Ecology 99, 1709–1715. doi: 10.1002/ecy.2391
Rindi L., Dal Bello M., Dai L., Gore J., Benedetti-Cecchi L. (2017). Direct observation of increasing recovery length before collapse of a marine benthic ecosystem. Nat. Ecol. Evol. 1 (6), 1–7. doi: 10.1038/s41559-017-0153
RStudio Team (2021). RStudio: Integrated development environment for r (Boston, MA: RStudio, PBC). Available at: https://www.rstudio.com/.
Rull J. L., Gómez Garreta A. (1989). Distribución de las algas epífitas sobre los ejemplares de Cystoseira mediterranea sauv. An. Jardín Bot. Madrid 46(1), 99–106. Available at: https://dialnet.unirioja.es/servlet/articulo?codigo=2955200.
Sadogurska S. S., Neiva J., Falace A., Serrão E. A., Israel A. (2021). The genus Cystoseira s.l. (Ochrophyta, fucales, sargassaceae) in the black Sea: morphological variability and molecular taxonomy of Gongolaria barbata and endemic Ericaria crinita f. bosphorica comb. nov. Phytotaxa 480 (1), 1–21. doi: 10.11646/phytotaxa.480.1.1
Sales M., Cebrian E., Tomas F., Ballesteros E. (2011). Pollution impacts and recovery potential in three species of the genus Cystoseira (Fucales, heterokontophyta). Estuar. Coast. Shelf Sci. 92 (3), 347–357. doi: 10.1016/j.ecss.2011.01.008
Savonitto G., Alongi G., Falace A. (2019). Reproductive phenology, zygote embryology and germling development of the threatened Carpodesmia barbatula (= Cystoseira barbatula) (Fucales, phaeophyta) towards its possible restoration. Webbia 74 (2), 317–323. doi: 10.1080/00837792.2019.1692594
Savonitto G., de la Fuente G., Tordoni E., Ciriaco S., Srijemsi M., Bacaro G., et al. (2021). Addressing reproductive stochasticity and grazing impacts in the restoration of a canopy-forming brown alga by implementing mitigation solutions. Aquat. Conserv.: Mar. Freshw. Ecosyst. 31 (7), 1611–1623. doi: 10.1002/aqc.3555
Schiel D. R., Foster M. S. (2006). The population biology of large brown seaweeds: Ecological consequences of multiphase life histories in dynamic coastal environments. Annu. Rev. Ecol. Evol. Syst. 37 (1), 343–372. doi: 10.1146/annurev.ecolsys.37.091305.110251
Schneider C. A., Rasband W. S., Eliceiri K. W. (2012). NIH Image to ImageJ: 25 years of image analysis. Nat. Methods 9 (7), 671–675. doi: 10.1038/nmeth.2089
Silva P. C., Pedroche F. F., Chacana M. E., Aguilar-Rosas R., Aguilar-Rosas L. E., Raum J. (2004). Geographic correlation of morphological and molecular variation in Silvetia compressa (Fucaceae, fucales, phaeophyceae). Phycologia 43 (2), 204–214. doi: 10.2216/i0031-8884-43-2-204.1
Steen H., Scrosati R. (2004). Intraspecific competition in Fucus serratus and F. evanescens (Phaeophyceae: Fucales) germlings: Effects of settlement density, nutrient concentration, and temperature. Mar. Biol. 144 (1), 61–70. doi: 10.1007/s00227-003-1175-8
Stravisi F. (1983). The vertical structure annual cycle of the mass field parameters in the gulf of Trieste. Bollet. Ocean. Teor. Appl. 1, 239–250. Available at: https://hdl.handle.net/11368/1701345.
Susini M. L., Mangialajo. L., Thibaut. T., Meinesz. A. (2007). Development of a transplantation technique of Cystoseira amentacea var. stricta and Cystoseira compressa. Hydrobiologia 580 (1), 241–244. doi: 10.1007/s10750-006-0449-9
Thibaut T., Blanfuné A., Boudouresque C. F., Verlaque M. (2015). Decline and local extinction of fucales in French Riviera: the harbinger of future extinctions? Medit. Mar. Sci. 16 (1), 206–224. doi: 10.12681/mms.1032
Thibaut T., Pinedo S., Torras X., Ballesteros E. (2005). Long-term decline of the populations of fucales (Cystoseira spp. and Sargassum spp.) in the albères coast (France, north-western Mediterranean). Mar. pollut. Bull. 50 (12), 1472–1489. doi: 10.1016/j.marpolbul.2005.06.014
Vadas R. L., S.R., Johnson S., Norton T. A. (1992). Recruitment and mortality of early post-settlement stages of benthic algae. Br. phycol. Bull. 27 (3), 331–351. doi: 10.1080/00071619200650291
Verdura J., Sales M., Ballesteros E., Cefalì M. E., Cebrian E. (2018). Restoration of a canopy-forming alga based on recruitment enhancement: methods and long-term success assessment. Front. Plant Sci. 9. doi: 10.3389/fpls.2018.01832
Vergés A., Tomas F., Cebrian E., Ballesteros E., Kizilkaya Z., Verg A., et al. (2014). Tropical rabbit fish and the deforestation of a warming temperate sea. J. Ecol. 102, 1518–1527. doi: 10.1111/1365-2745.12324
Vizetto-Duarte C., Custódio L., Acosta G., Lago J. H. G., Morais T. R., Bruno de Sousa. C., et al. (2016). Can macroalgae provide promising anti-tumoral compounds? a closer look at Cystoseira tamariscifolia as a source for antioxidant and anti-hepatocarcinoma compounds. PeerJ 4, e1704. doi: 10.7717/peerj.1704
Keywords: Gongolaria barbata, canopy-forming algae, restoration, ex situ, cultivation, Mediterranean
Citation: Orlando-Bonaca M, Savonitto G, Asnaghi V, Trkov D, Pitacco V, Šiško M, Makovec T, Slavinec P, Lokovšek A, Ciriaco S, Chiantore M, Kaleb S, Descourvières EP, Srijemsi M and Falace A (2022) Where and how - new insight for brown algal forest restoration in the Adriatic. Front. Mar. Sci. 9:988584. doi: 10.3389/fmars.2022.988584
Received: 07 July 2022; Accepted: 10 November 2022;
Published: 28 November 2022.
Edited by:
Elisabetta Manea, National Research Council (CNR), ItalyReviewed by:
Eva Cacabelos, University of the Azores, PortugalAntonella Petrocelli, National Research Council (CNR), Italy
Copyright © 2022 Orlando-Bonaca, Savonitto, Asnaghi, Trkov, Pitacco, Šiško, Makovec, Slavinec, Lokovšek, Ciriaco, Chiantore, Kaleb, Descourvières, Srijemsi and Falace. This is an open-access article distributed under the terms of the Creative Commons Attribution License (CC BY). The use, distribution or reproduction in other forums is permitted, provided the original author(s) and the copyright owner(s) are credited and that the original publication in this journal is cited, in accordance with accepted academic practice. No use, distribution or reproduction is permitted which does not comply with these terms.
*Correspondence: Martina Orlando-Bonaca, Martina.Orlando@nib.si