- 1Innovation Team for Crustacean Aquaculture and Restocking, Dalian Ocean University, Dalian, China
- 2Sanya Tropical Fisheries Research Institute, Sanya, China
This study investigated the effects of replacing commercial feeds with fresh black soldier fly larvae (BSFL) on the immune enzyme activities, water quality in the culturing environment, and intestinal microbiota of Pacific white shrimp (Litopenaeus vannamei). Five different feeding regimes were designed, in which 0% (control group), 25%, 50%, 75%, and 100% commercial feeds were replaced with equal wet weight of fresh BSFL, respectively. The experiment lasted for 45 days, and the results showed that the serum T-NOS (total nitric oxide synthase), AKP (alkaline phosphatase), and ACP (acid phosphatase) activity were significantly increased in the BSFL25% group. However, the immune enzymes of the hepatopancreas decreased significantly in all the BSFL-containing groups compared to the control group. Total ammonia nitrogen concentrations throughout the experimental phase and nitrate concentrations in the middle and later phases of the experiment were negatively correlated with the replacement rate of fresh BSFL in the diet, whereas pH was positively correlated with the replacement rate of fresh BSFL in the diet. Proteobacteria and Bacteroidetes were dominant phyla in the intestines of shrimps, but the relative abundances of Proteobacteria and Firmicutes decreased, with those of Bacteroidetes and Planctomycetes increased in the BSFL-containing groups. Among them, higher relative abundances of potential probiotics such as Motilimonas, Shimia, Pseudoalteromonas, and Shewanella and lower relative abundance of genus Vibrio were observed in the fresh BSFL-containing groups. Furthermore, shrimps fed with BSFL-containing diets had higher bacterial richness and diversity in the intestines. In conclusion, a proper replacement of commercial feed with fresh BSFL had a positive effects on the immune-related enzyme activities, water quality, and intestinal health in the L. vannamei.
Introduction
Global aquaculture production of shrimp has increased dramatically within the past two decades. The Pacific white shrimp, Litopenaeus vannamei, is the most important farmed crustacean species, contributing 53% of the total farmed crustacean production (Yuan et al., 2021). With the upgrading of consumption, L. vannamei has become an attractive and popular species for aquaculture in the United States, Ecuador, Thailand, and China (Saoud et al., 2003; Mcgraw et al., 2010). Meanwhile, this has also brought challenges to the feed industry, because over 90% of farmed shrimp rely on high-protein diets containing a high percentage of marine fish meal (FM), which is a major ingredient in commercial feeds due to its high digestible protein content, balanced amino acid composition, and high palatability (Cruz-Suárez et al., 2007; Lemos et al., 2009; Council, 2011). However, FM also has a lot of disadvantages: the first of which is that the resource of FM cannot meet the continuously increasing demand of the aquaculture industry, and the contradiction is further aggravated by the natural resource decline and global competition for the FM from livestock and poultry industry (Adelizi et al., 1998; Samira and Mehdi, 2015) . In addition, the processing of FM produces a large number of pollutants containing nitrogen and sulfur compounds, which could be deleterious to human healthe and the environment. (Oyarzun et al., 2019). Finally, FM contains a large amount of phosphorus and nitrogen. It can cause the deterioration of farming water quality and the occurrence of diseases in aquatic animals when the unabsorbed nitrogen and phosphorus are discharged into the farming environment with residual bait and manure (Lai et al., 2009; Marinho-Soriano et al., 2009). Therefore, it has become an important topic for aquaculture industry to find a new protein source. The Food and Agricultural Organization (FAO) has highlighted the potential utility of insects as food and feed sources, which usually possess an outstanding capacity to upgrade low-quality organic material, require minimal water and cultivable land, and emit little greenhouse gases to produce large quantities of high-quality animal protein (Van Huis, 2013). Among them, Locusta migratoria, Tenebrio molitor, and Hermetia illucens are candidates to replace FM in commercial feed (EMEHINAIYE, 2012; Gasco et al., 2016; Bruni et al., 2018).
The black soldier fly (Hermetia illucens) is one of the most promising insect species for commercial exploitation in China, and it could live on animal manure or plant material by converting low-value organic waste into a protein-rich biomass (Diener et al., 2009). This makes it a suitable source of food for aquatic animals (Newton et al., 1977; Bondari and Sheppard, 1981). Recently, black soldier fly larvae (BSFL) has been used as a substitute for FM in several aquatic animal species including Oncorhynchus mykiss (Bruni et al., 2018), Pelteobagrus fulvidraco (Xiao et al., 2018), and Cherax cainii (Foysal et al., 2019). Interestingly, some studies have indicated that BSFL have the effect of enhancing the immunity of aquatic animals, such as C. cainii (Foysal et al., 2019) and Cyprinus carpio var. Jian (Li et al., 2017). In addition, BSFL have also been demonstrated to promote growth and nutrition absorptive capacity in aquatic animals (Li et al., 2017; Xiao et al., 2018).
Although there have been a lot of reports on feeding L. vannamei by BSFL (Cummins et al., 2017), these studies have more emphasis on the evaluation of the effects of replacing FM in commercial feed with BSFL in powder form on aquatic animals. Processing insects into powder form as the raw protein source is an industrial necessity and easy to preserve. However, fresh insects also have their advantages, such as simple feeding, low nutrient losses, and easier nutrition enhancement. A recent study by our team showed no significant negative effects on shrimp growth when up to 50% of the commercial feed was replaced by fresh BSFL (He et al., 2022). Aquaculture outcome is not defined solely by growth performance, but issues concerning aquatic animals’ welfare also need to be taken into accounts, such as the water quality of the aquaculture environment and the immune capacity of different tissues. Commercial feeds have been found to negatively affect water quality parameters including pH, nitrite nitrogen (NO2-N), and total ammonia nitrogen (TAN), which may in turn affect the growth of aquatic animals (Pullin and Lowe-McConnell, 1982). Fresh BSFL instead of commercial feed may be a potential method to reduce water quality degradation in aquaculture environments. This capacity can maintain the health of aquatic animals and control disease outbreaks, which may improve farming profits and reduce the use of antibiotics in aquaculture. In addition, we would like to increase this replacement rate through more comprehensive studies. To the best of our knowledge, no information is available about the effects of feeding BSFL on the intestinal microbiota of L. vannamei. Intestinal microbiota helps the host to digest indigestible feed components such as cellulose, and it also participates in the maturation of intestinal mucosa, immune response, and disease resistance (Maslowski and Mackay, 2011; Llewellyn et al., 2014). Fresh insects contain bioactive compounds that appear to modulate intestinal microbiota and have a positive impact on animal health (Gasco et al., 2018). Therefore, it is necessary to evaluate whether the intestinal microbial changes of shrimp are the factors limiting the replacement rate and finding ways to improve the replacement rate.
In this experiment, the effects of fresh BSFL on hepatopancreas and serum immune enzyme activity, the water quality of the aquaculture environment, and the intestinal microbiota of L. vannamei were assessed to provide more information for farming L. vannamei using fresh BSFL as a protein source and to contribute for sustainable development of aquaculture industry.
Materials and method
Feeding regime
In this experiment, five feeding regimes were designed by replacing different levels (0%, 25%, 50%, 75%, and 100%, respectively) of commercial feeds with fresh BSFL. The commercial feeds (crude protein: 42%; crude lipid: 6%) were used as a control feeding regime. The BSFL were hatched in our laboratory and were fed the bran regularly every day (the bran and water mix by a volume ratio of 3:1). Then, the BSFL were collected and stored at −20°C before they turn into mature insects. The proximate composition of commercial feeds and fresh BSFL was shown in Table 1. Feeding regimes and theoretical proximate composition of ingested diets for each group were listed in Table 2.
Feeding experiment
L. vannamei postlarvae (body length is about 0.4 cm) were purchased from a commercial hatchery (Hainan Province, China) and were reared by our team on a basic non-insect diet. The larval shrimp were temporarily reared for 7 days before the experiment to acclimate to the rearing environment and experimental diets. Then, the experimental shrimp were fasted for 12 h and weighed. A total of 750 shrimp (body length: 3.27 ± 0.23 cm) were randomly distributed into 15 glass fiber tanks filled with 300 L of seawater, at a density of 50 shrimp per tank; the five experimental diets were randomly assigned to triplicate tanks. The shrimp used in the experiment were equivalent in size and weight. Shrimp were fed four times a day (6:00, 12:00, 18:00, and 24:00) during the 45-day feeding. The feeding weight of each group was based on the feeding amount from hand-feeding to visual satisfaction and on the weight of shrimp, whereas the replacement rate of fresh BSFL was controlled by adjusting the feeding times of fresh BSFL. The remaining feeds and feces were removed daily and about 20% of water was exchanged every day. The water temperature, salinity, and dissolved oxygen (DO) were maintained at 28°C ± 3°C, 27 ± 2, and 7.02 ± 0.69 mg/L, respectively.
Sample collection of shrimp
Shrimps were starved for 12 h before sampling. Then, the whole intestines of three shrimps from each tank were aseptically dissected, the intestinal contents were collected and immediately put in liquid nitrogen, and samples were preserved with dry ice and sent to Biomarker Technology Co. Ltd (Beijing, China) for DNA extraction and analyses by high-throughput sequencing. The blood of another five shrimps in each tank was collected and mixed with anticoagulant solution (1:1), and then the separated serum was removed by centrifuging (3000 rpm, 10 min) after keeping for 6 h at 4°C, which were immediately frozen in lipid nitrogen and stored at −80°C for serum immune enzyme activity analysis. In addition, the hepatopancreas of three shrimps from each tank was quickly removed, frozen in liquid nitrogen, then stored at −80°C until the determination of immune enzyme activities.
Assay of immune enzyme activities of serum and hepatopancreas
The hepatopancreas samples were homogenized in 10 volumes (w/v) of ice-cold physiological saline solution and centrifuged at 4000 rpm at 4°C for 20 min, and the supernatant was conserved at 4°C. The activity of alkaline phosphatase (AKP), acid phosphatase (ACP), and total nitric oxide synthase (T-NOS) in the hepatopancreas and serum was analyzed according to the instructions of commercially available kits (Nanjing Jiancheng Biotech Co., Nanjing, China). The protein content of hepatopancreas samples was determined as described by Bradford (1976).
Measurement of chemical water quality parameters
The DO (mg/L), temperature (T, °C), pH, and salinity (S) of the water in the shrimp tanks were measured daily using a portable water quality monitor-P1 (Zhongyi IOT Ltd. China) before feeding the shrimp. For other water quality parameters, 100 mL of water samples was gathered from tanks every 4 or 5 days and was used for further analysis. Subsequently, the water sample was inspected for NO2-N and TAN following Standard Methods (Rice et al., 2012), and the chemical oxygen demand (COD) was measured according to Standard Methods (Eaton et al., 2005).
DNA extraction, PCR amplification of 16S amplicon sequencing, and sequence data processing
The shrimp intestinal microbiota was analyzed by 16S high-throughput sequencing. Total DNA was extracted using MN NucleoSpin 96 Soil Kit. Subsequently, the full-length 16s rRNA gene was amplified using barcoded conserved primers 27F (5′- AGRGTTTGATYNTGGCTCAG-3′) and 1492R (5′-TASGGHTACCTTGTTASGACTT-3′). The PCR amplification was performed in a total volume of 50 μL containing 25 μL of KOD FX Neo Buf (2×), 10 μL of 2 mM dNTP, 1 μL of KOD FX Neo (TOYOBO), 2.5 μL of 10 μM each primer, and 60 ng of DNA template, and finally, ddH2O was added to 50 μL. PCR amplification was performed on the PCR system under the following conditions: (a) 1*(3 min at 95°C), (b) 25*(30 s at 95°C; 30 s at 55°C; 1 min at 72°C), and (c) 10 min at 72°C, 10°C until halted by the user (Yuan et al., 2019).
The PCR products were purified, quantified, and homogenized to form a sequencing library (SMRT Bell), and then the quality inspection was carried out. The product that qualified the library was sequenced on a PacBio Sequel platform at Biomarker Technology Co. Ltd (Beijing, China). Bioinformatics analysis for this research was performed with the help of BMK Cloud (Biomarker Technologies Co., Ltd., Beijing, China). Filtering and demultiplexing of raw reads were generated by sequencing to obtain circular consensus sequencing (CCS) reads by SMRT Link software (version 8.0) (minPasses ≥ 5 and minPredictedAccuracy ≥ 0.9). The CCS sequences were subsequently assigned to corresponding samples according to the barcode by lima (version 1.7.0). The Cutadapt quality control program (version 2.7) was used for quality filtering by identifying forward and reverse primers, and then discarding CCS reads without primers and reads beyond the length range (1,200–1,650 bp). Chimeric sequences were detected and removed using the UCHIME algorithm (V 8.1) to obtain the clean reads.
Measurement of bacterial communities
The resulting datasets were analyzed using USEARCH (V 10.0). The obtained high-quality sequences were clustered as operational taxonomic units (OTUs) based on 97% similarity. and filtered for OTUs with reabundace<0.005%. Taxonomy annotation of OTUs was performed using the SILVA database (release132) by RDF classifier (V 2.2) (confidence threshold was 80%). Selected OTUs were aligned against the core alignment template of the SILVA database using PyNAST (V 1.2.2) to facilitate downstream diversity analysis. The OTU abundance information was normalized by a standard sequence number corresponding to the sample with the fewest sequences, and further analysis of alpha and beta diversity was performed based on the normalized output data. All sequences in this study have been deposited in the Sequence Read Archive (SRA) of the NCBI database under the accession number PRJNA859541. The beta diversity was analyzed by non-metric multidimensional scaling (NMDS) based on the unweighted UniFrac distance matrix to show differences in microbial community structure between each group. The linear discriminant analysis (LDA) effect size (LEfSe) analysis was used to analyze the differences in microbial community abundance between the control group and fresh BSFL-containing groups; the threshold for the LDA score was set at 4. The alpha diversity (Chao1, Ace, Shannon, and Simpson) and beta diversity were calculated and displayed by BMKCloud (www.biocloud.net).
Calculations and statistical analysis
One-way analysis of variance (ANOVA) was used to test the effect of dietary manipulation. Firstly, all the data were tested for normal distribution and homogeneity of variance. Then, the group means could be further compared using the LSD test for multiple comparisons. All statistical analyses were performed using the SPSS 23 software. The level of significant difference was set at P < 0.05 and the results were presented as means ± S.E.M. (standard error of the mean).
Results
The immune enzyme activities after farming experiment in serum and hepatopancreas
The activities of T-NOS, AKP, and ACP in the serum increased first and then decreased with the increase of the replacement rate of fresh BSFL. The activity of T-NOS was higher in the group containing fresh BSFL than that of the control group, and the maximum value was found in the BSFL75% group, with significant differences compared to the control group (P < 0.05). The maximum value of AKP activity was found in the BSFL25%, and when the replacement level was equal to or above 50%, the activity of AKP was significantly decreased compared to the control group (P < 0.05). The maximum activity of ACP was found in the BSFL25%, with significant differences (P < 0.05) compared to the control group and non-significant differences (P>0.05) in the other BSFL-containing replacement groups (Table 3).
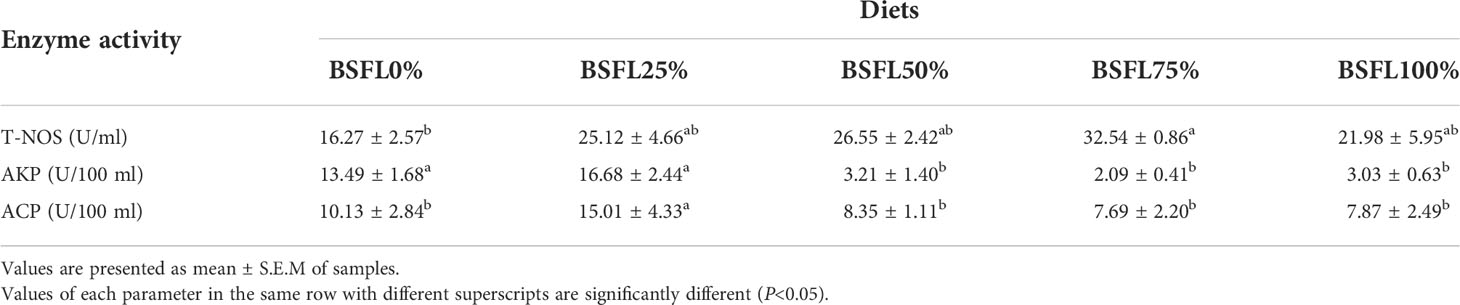
Table 3 The serum immune enzyme activities of L. vannamei fed different experimental diets for 8 weeks.
The activity of T-NOS, AKP, and ACP of shrimp hepatopancreas was negatively related to the proportion of fresh BSFL in the diet, with T-NOS activity that significantly reduced (P < 0.05) when the BSFL replacement level was equal to or above 50%. The hepatopancreas AKP and ACP activity in all BSFL-containing groups was significantly lower (P < 0.05) than that of the control group (Table 4).
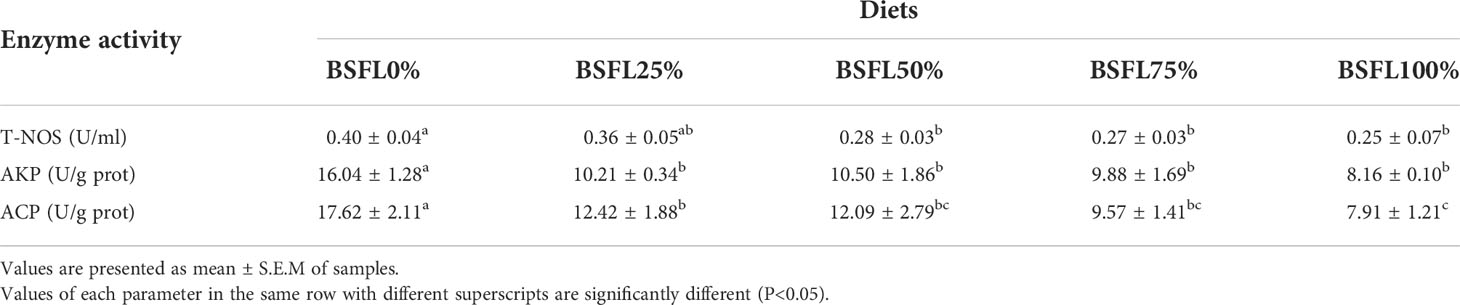
Table 4 The hepatopancreatic immune enzyme activities of L. vannamei fed different experimental diets for 8 weeks.
Chemical water parameters of shrimp tanks
Chemical water parameters were monitored and recorded in Figure 1. The results of water chemical parameters showed considerable variations among the experimental groups throughout the 45 days of the experimental period. The TAN and NO2-N trends of shrimp tanks were negatively related to the dietary fresh BSFL replacement level, whereas the value of NO2-N in the middle and late periods of culture was decreased significantly in BSFL-containing diet groups than that of the control group (P<0.05). The highest concentration of TAN and NO2-N was observed in the BSFL0% group with 0.88 ± 0.30 mg/L and 1.918 ± 0.17 mg/L, respectively, whereas the lowest concentration of TAN and NO2-N was observed in the BSFL100% group with 0.13 ± 0.04 mg/L and 0.02 ± 0.01 mg/L, respectively. The differences in the value of COD of shrimp tanks were insignificant among the treatments (P>0.05). In the middle and late phase of culture, the value of pH of shrimp tanks was positively related to the replacement rate of fresh BSFL in the diet, where the pH value was significantly higher (P<0.05) than that of the control group when the BSFL replacement level was equal to or above 75%.
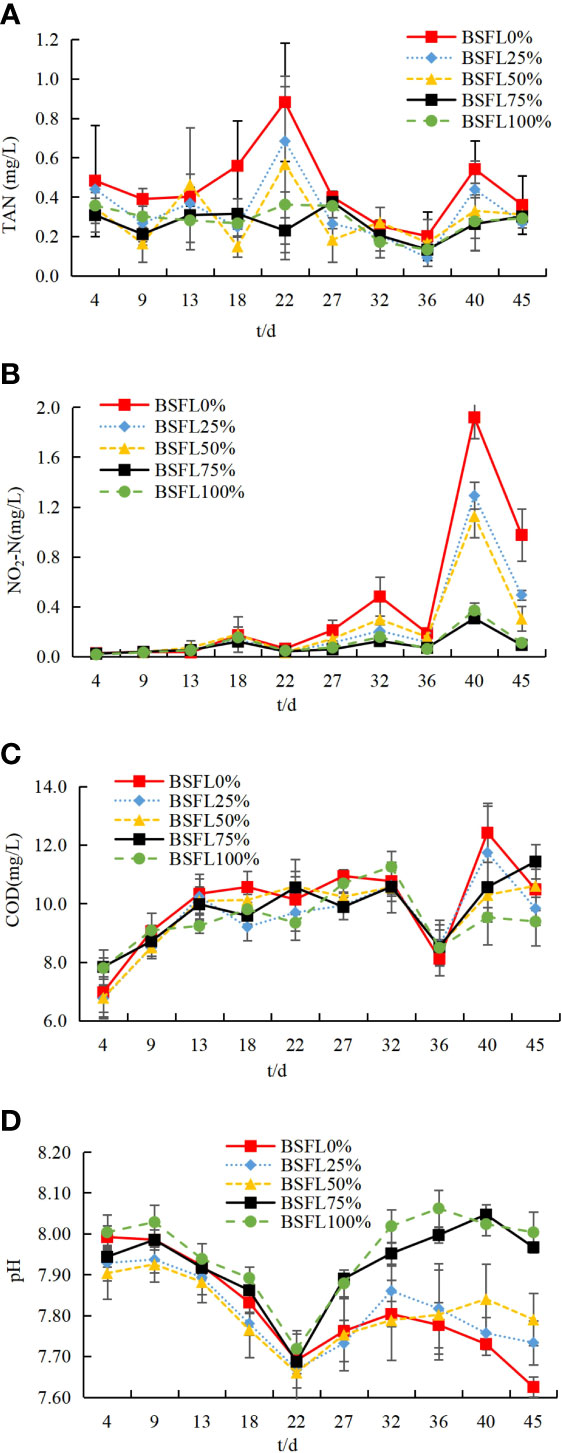
Figure 1 Trends of total ammonia nitrogen (TAN) (A), NO2-N (B) chemical oxygen demand (COD) (C) and pH (D) concentrations (mean±SD per sampling date) in different treatments throughout the experimental period.
PacBio sequencing and microbial complexity in shrimp intestine
A total of 177,569 CCS sequences were obtained after 15 samples were sequenced and identified by Barcode; each sample generated at least 6,081 CCS sequences with an average of 7,838 CCS sequences. The sequences with more than 97% similarity were clustered into OTUs to exploring the bacterial community diversity among all treatments. Both rarefaction analysis and Shannon index curves indicated that all sequenced samples reached the asymptote (Figure 2). The rarefaction analysis indicated that the rarefaction curves reached the asymptote and sufficient sampling depth was achieved for each sample when the number of random sampling sequences of each sample exceeds 5,000 (Figure 2). The bacterial richness and diversity indices were calculated from OTUs to estimate and compare the bacterial diversity in each diet (Table 5). The richness indices of Chao1 and Ace varied from 58 to 246.44 and from 67.42 to 242.65, respectively. The intestinal microbial richness of shrimp was positively correlated with dietary fresh BSFL levels according to the Chao1 and Ace indices, with a significant increase observed in the BSFL75% and BSFL100% groups (P<0.05). Bacterial diversity was estimated by the Shannon and Simpson indices, which varied from 1.15 to 3.61 and from 0.06 to 0.48, respectively. The Simpson and Shannon indices showed a trend of increasing and then decreasing intestinal microbial diversity of shrimp as the dietary fresh BSFL level increased; the maximum was found in the BSFL75% group, with a significant increase when the fresh BSFL level was equal to or above 75% (P<0.05). The Good’s coverage value was > 0.99, indicating that a high degree of sequencing coverage, with all microbiota in each group, was represented by the number of OTUs identified.
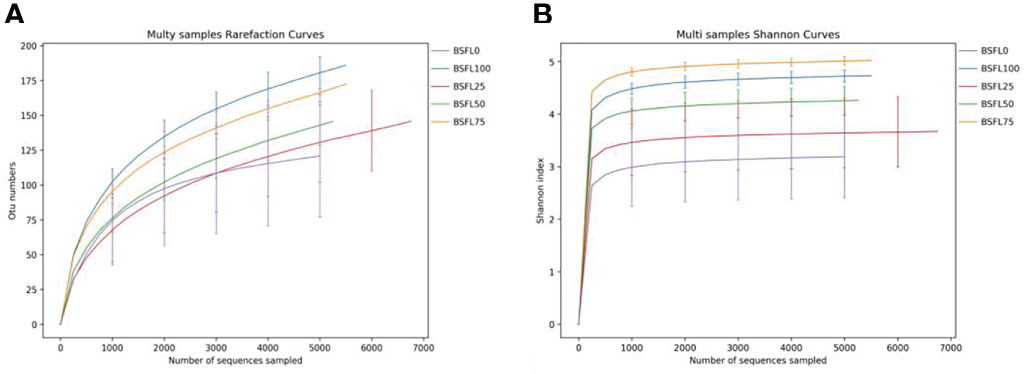
Figure 2 Rarefaction curves (A) and Shannon-Wiener curves (B) of the intestinal bacterial community of L. vannamei fed with diets having different levels of BSFL for 45 days.
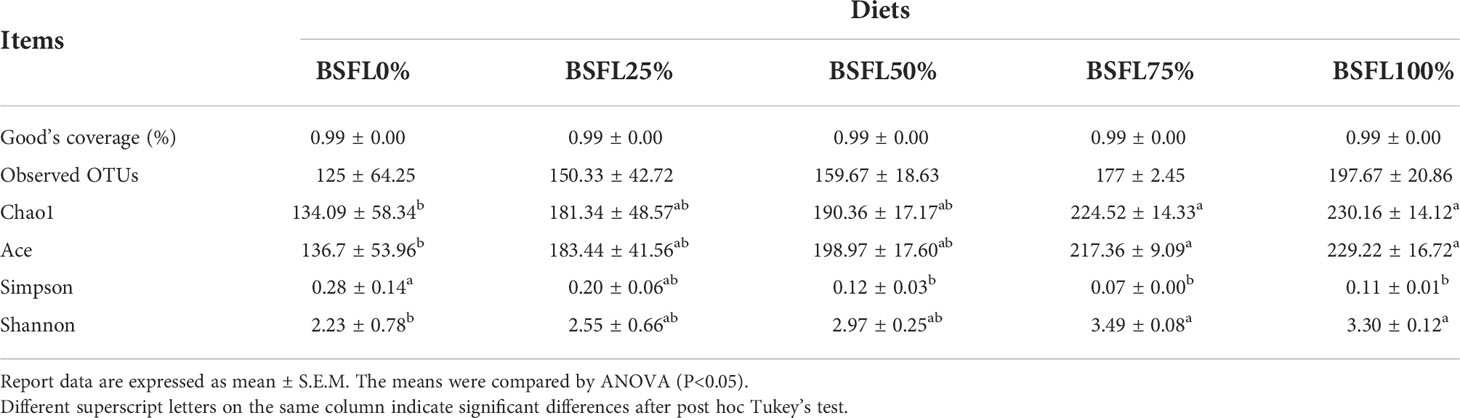
Table 5 Good’s coverage value and alpha diversity indices values of intestinal microbiota of L. vannamei fed with diets having different levels of BSFL for 45 days.
Composition and difference of microbiota among five groups
In shrimp intestine, 87 OTUs (31.07%, 33.4%, 35.95%, 31.52%, and 29.39% in diet BSFL0%, BSFL25%, BSFL50%, BSFL75%, and BSFL100% groups, respectively) were shared among all samples (Figure 3). Significant differences were also observed among bacterial communities (beta diversity) in type (unweighted UniFrac) of taxa. The feeding effect on intestinal microbiota of shrimp was explained as displayed in unweighted NMDS (non-metric multi-dimensional scaling) (Figure 4). The bacterial communities of fresh BSFL-containing diet groups clustered together and separately from the control group were shown by the NMDS plot. It suggested that the composition of the shrimp intestinal community is significantly influenced by feeding on fresh BSFL. At the phylum level, the intestinal microbiota of shrimps was mainly dominated by Proteobacteria, Bacteroidetes, and Planctomycetes regardless of the diet. The relative abundance of Proteobacteria in all fresh BSFL-containing groups was lower than that of the control group, with a significant difference when the fresh BSFL replacement level was equal to or above 50%. The relative abundance of Planctomycetes was higher in all fresh BSFL-containing groups than that of the control group, with significant differences found in the BSFL100% group. The relative abundance of Bacteroidetes was higher in all groups containing fresh BSFL than that of the control group, with significant differences found in the BSFL25% and BSFL75% groups. Only Firmicutes were not the dominant phyla in the intestinal microbiota of shrimp whose diet contained fresh BSFL than that of the control group, whereas Verrucomicrobia and Actinobacteria were found to be dominant phyla in the higher fresh BSFL level groups (BSFL75% and BSFL100%) and control groups (Figure 5; Table 6). At the genus level, the intestinal microbiota of shrimp was mainly dominated by Ruegeria and Vibrio regardless of the diet. The relative abundance of Ruegeria was higher in all groups containing fresh BSFL than that of the control group, with significant differences found in the BSFL100% group. The trend in the relative abundance of Vibrio was the opposite compared to Ruegeria, with significant differences found in the BSFL100% group. Only Fusibacter and Ralstonia were not the dominant genera in the intestinal microbiota of the group containing fresh BSFL than that of the control group, while the intestinal microbiota of the groups containing fresh BSFL was characterized by a high relative abundance of Motilimonas, Shimia, Pseudoalteromonas, and Donghicola. Shewanella was the dominant genus found only in the BSFL50%, BSFL75%, and BSFL100% groups (Figure 6 and Table 7).
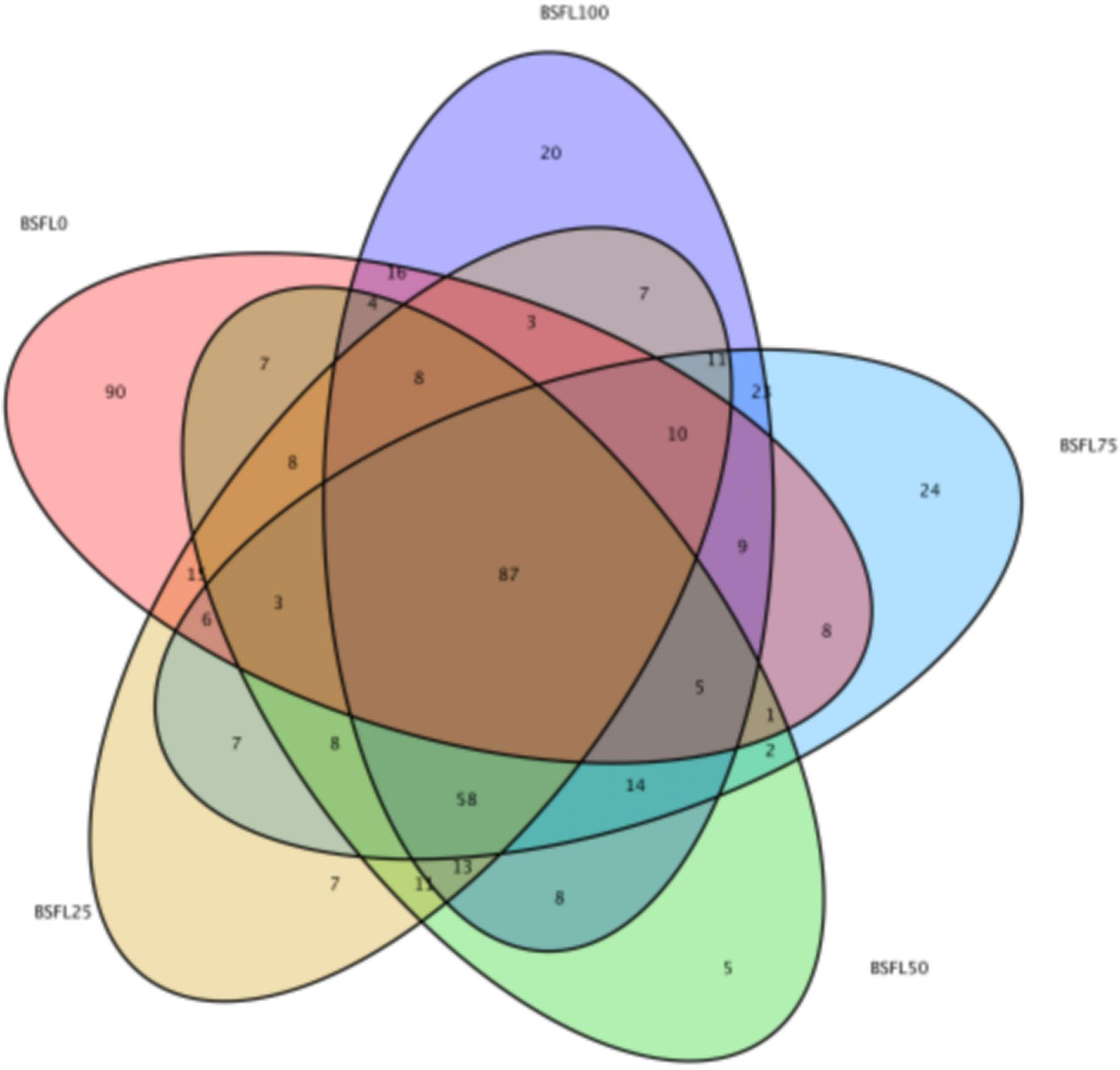
Figure 3 Venn diagram showing the distribution of all OTUs shared by L. vannamei in five groups. The figures in the Venn diagram indicate the number of the sequences that are associated with the OTUs in each group.
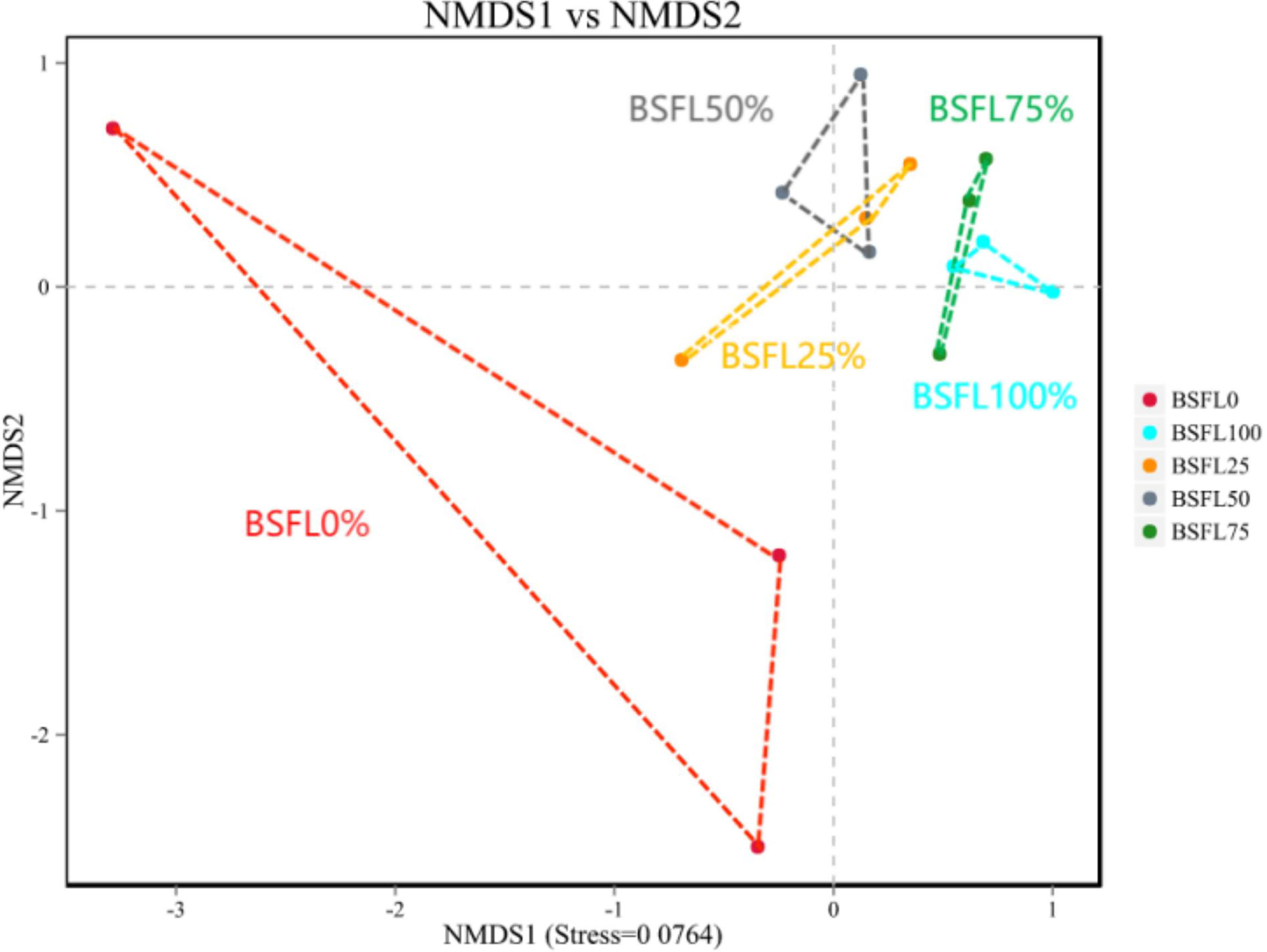
Figure 4 Non-MetricMulti-Dimensional Scaling (NMDS) of unweighted distances of intestinal microbiota associated to different diets.
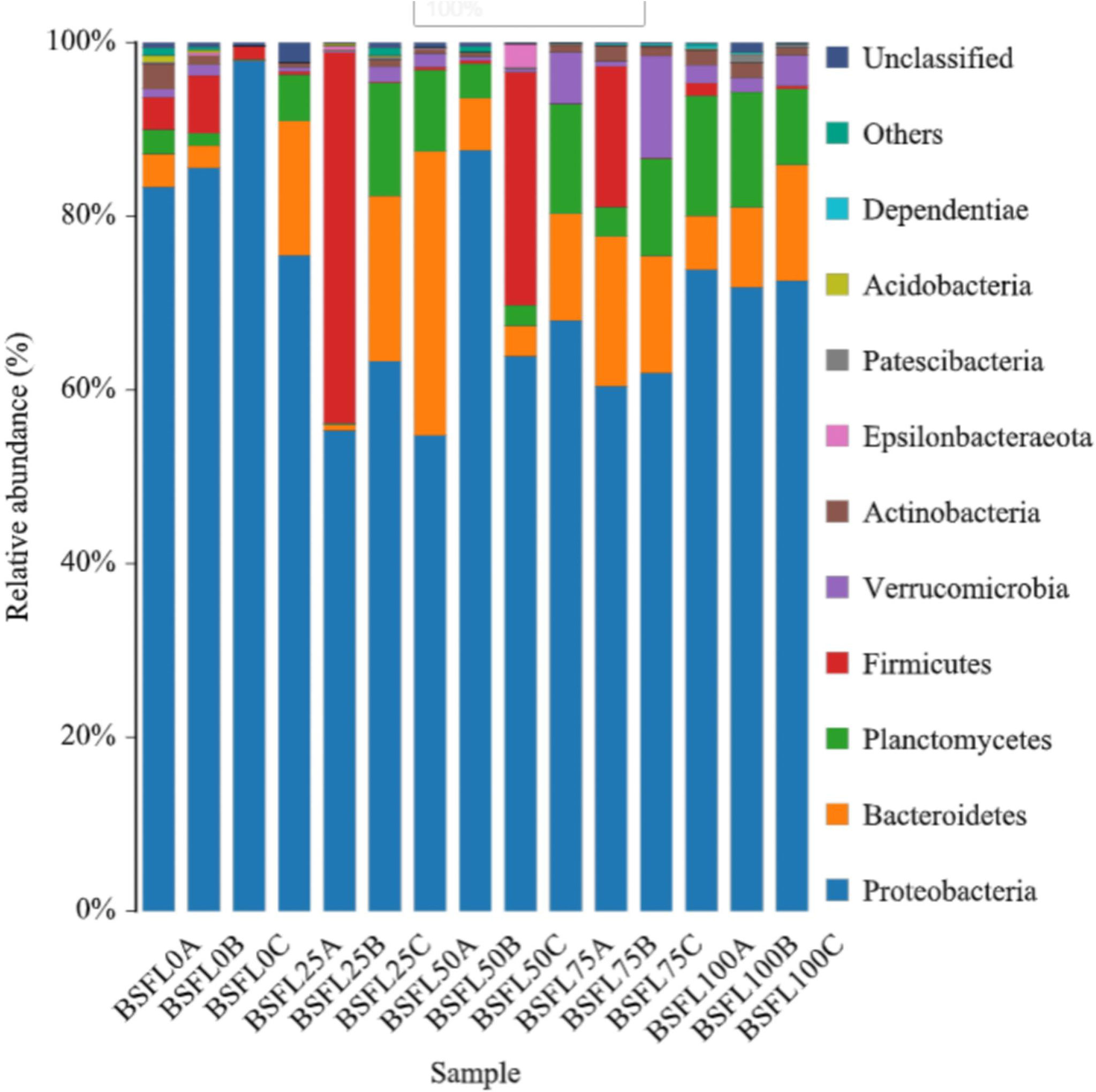
Figure 5 Relative abundances of bacterial phyla in the intestine of L. vannamei fed with diets having different levels of BSFL. The most dominant phyla (> 1% of the total sequences) are shown in the schematic individually, and the relative abundances of main phyla is indicated in Table 6.
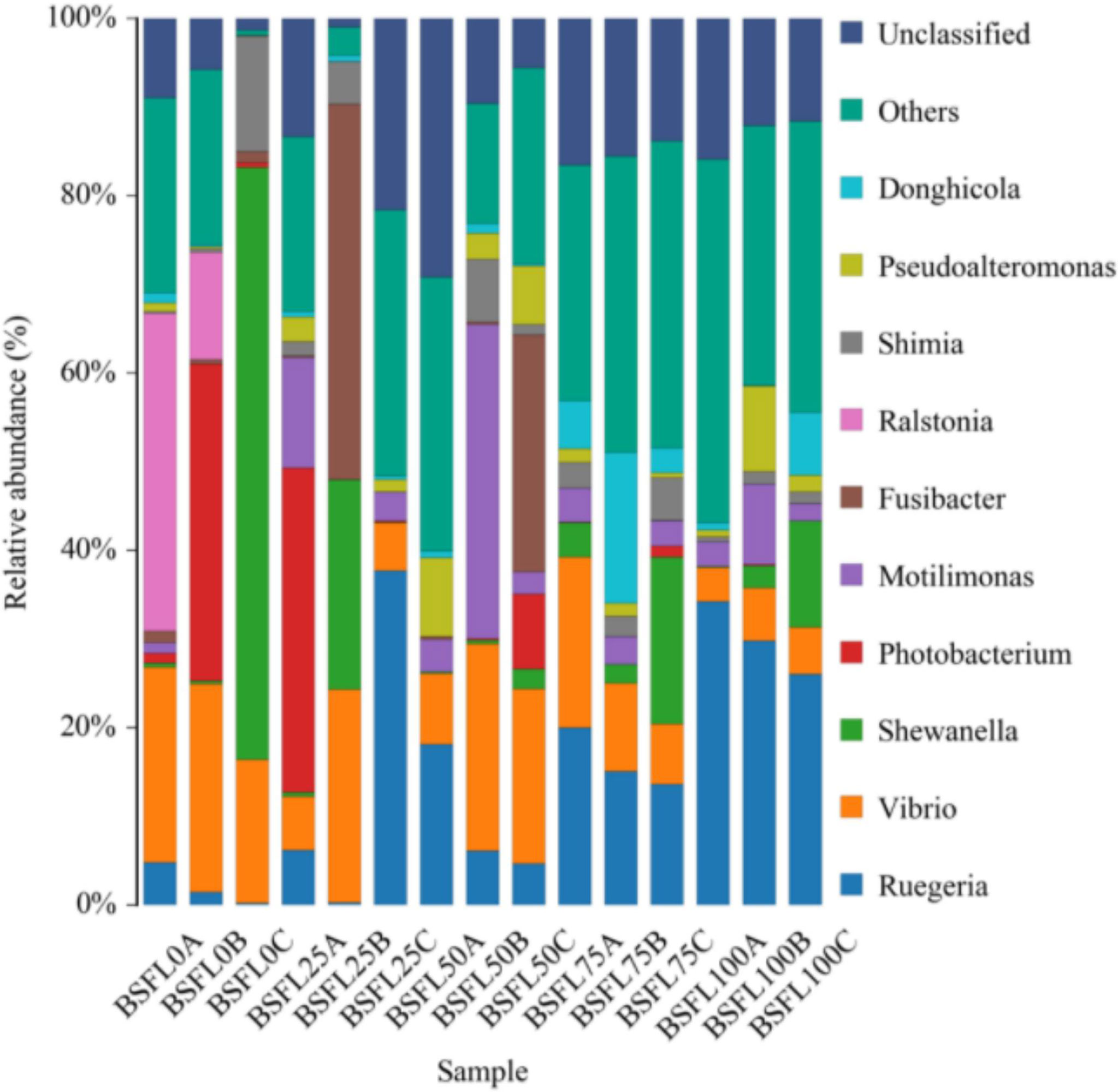
Figure 6 Relative abundances of bacterial genera in the intestine of L. vannamei fed with diets having different levels of BSFL. The most dominant genera (> 1% of the total sequences) are shown in the schematic individually, and the relative abundances of main genera is indicated in Table 7.
The statistical results were further supported by the LEfSe analysis, which showed significant differences in intestinal bacterial abundances between shrimps fed the diets containing fresh BSFL and those fed with commercial feeds (Figure 7). The results showed that the relative abundance of class Gammaproteobacteria and genus Ralstonia belonging to phylum Proteobacteria was significantly higher in the control group. Moreover, the significantly higher relative abundance of family Rubinisphaeraceae (Planctomycetes), order Planctomycetales (Planctomycetes), order Pirellulales (Planctomycetes), family Pirellulaceae (Planctomycetes), class Planctomycetacia (Planctomycetes), genus Motilimonas (Proteobacteria), family Psychromonadaceae (Proteobacteria), genus Ruegeria (Proteobacteria), order Flavobacteriales (Bacteroidetes), family Flavobacteriaceae (Bacteroidetes), class Bacteroidia (Bacteroidetes) was found in the fresh BSFL-containing groups.
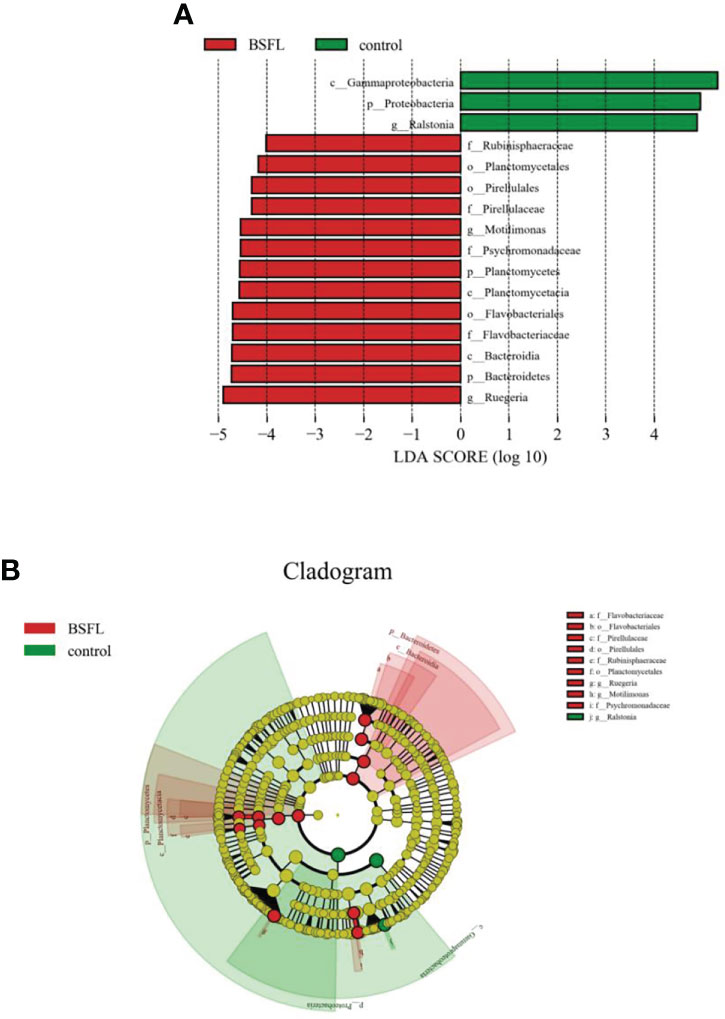
Figure 7 The LDA effects size (LEfSe) analysis of bacterial taxa between the control and fresh BSFL-containing groups. (A) Bar chart displays LDA scores of the control and fresh BSFL-containing groups. The LDA scores represented the differencein relative abundance with exponent fold change of 10 between two treatments. Significant differences are defined as P < 0.05 and LDA score >0.04. (B) Cladogram displays significantly enriched bacterial taxa (from the phylum to the genus level).
Discussion
The effects on immune enzyme activity of shrimp
The proper level of fresh BSFL can increase the immune enzyme activity of shrimp serum but led to a decrease in hepatopancreas immune enzyme activity. Among the non-specific immune indicators of L. vannamei, the activity of AKP, ACP, and T-NOS is closely related to the health status and immune capacity of the organism. Inducible nitric oxide synthase (iNOS) produces large amounts of NO to kill pathogens after being stressed by immune stimulants or various environmental stressors (Chakravortty and Hensel, 2003). In addition, both ACP and AKP are marker enzymes for lysosomal enzymes, which are important for the immune system of crustaceans (Sgeirsson et al., 1995; Xiao et al., 2018). Higher activities of T-NOS, AKP, and ACP were detected in the shrimp serum when 25% commercial feed was replaced by fresh BSFL. This was following the previous study (Xiao et al., 2018), which lysozyme and phagocytic percentages in the serum of P. fulvidraco were increased by the appropriate level of BSFL meal supplemented in the diet. Furthermore, the defatted insect meal (T. Molitor) was added to the diet also increased the phenol oxidase activity of L. vannamei (Rios et al., 2019). We suggest that chitin in BSFL may be responsible for the improved immune enzyme activity of L. vannamei. Many studies have also shown that chitin and its derivatives could enhance the immune response of aquatic animals (Khoushab and Yamabhai, 2010; Ngo and Kim, 2014). However, fresh BSFL also has negative effects: elevated fat level and reduced protein level in shrimp diets with the increase of fresh BSFL replacement level in the diet. Some research indicated that feeding diets with excessive fat levels have a negative impact on the immune system of shrimp (Zhang et al., 2013). In addition, the protein level in the diet is also closely related to the immune capacity of aquatic animals (Council, 2011). Therefore, the replacement rate of fresh BSFL is limited by their nutrition composition.
Interestingly, we may break this limitation by changing the culture substrate of fresh BSFL. The fresh BSFL is like a capsule which nutrition composition is closely related to its culture substrate (Shumo et al., 2019). Meneguz et al. (2018) found that the protein content of BSFL was significantly increased by using agro-industrial by-products instead of organic waste. Fresh BSFL with a lower fat level and higher protein are obtained by adjusting the culture substrate. Furthermore, studies have shown that BSFL are deficient in essential amino acids and unbalanced EAA/NEAA (Cummins et al., 2017), and this disadvantage cannot be improved by changing the culture substrate of the BSFL. The amino acid content of larvae changed in a small range when BSFL were raised on different rearing substrates, especially when compared with the significant difference in amino acid composition of the culture substrates (Spranghers et al., 2017). This also suggests that if fresh BSFL are to be used in shrimp culture, it is better to supplement the diet with additives, such as the addition of free amino acids to improve the amino acid balance of the diet.
Water quality of shrimp tanks
Fresh BSFL can improve water quality and reduce water change, thus decreasing the chance of external disease invasion. Water quality has been observed to be influenced by feeding activities, which may affect the growth performance of aquatic animals. In this study, continuous monitoring of water quality in each group showed that fresh BSFL replacement level was negatively correlated with ammonia nitrogen and nitrate nitrogen and positively correlated with pH. This phenomenon may be explained by the deterioration of water quality due to feed losses (Cha et al., 2008). Previous studies indicated that only 20%–30% of feed nutrients are retained in the shrimp culture and the remaining 70%–80% are accumulated in the aquatic system, which can lead to eutrophication and water quality deterioration (Paez-Osuna, 2001). Furthermore, the residues of fresh BSFL are mainly chitin exoskeleton, which is more difficult to dissolve and easier to be removed by siphonage than the commercial feeds. Excessive pollutants in the culture environments (such as ammonia, nitrite, and COD derived from soluble excretions and organic decomposition) can lead to anoxic conditions which are harmful to benthic biota owing to the oxygen-consuming decomposition (Li et al., 2011; Wang et al., 2016). Stress in shrimp can be caused by the overload of toxic nitrogen metabolites, which can also reduce immune capacity leading to susceptibility to disease (Kautsky et al., 2000). In addition, the ammoniacal nitrogen was converted to microbial biomass by heterotrophic bacteria, along with the production of CO2 (Ebeling et al., 2006), resulting in a decrease in the pH of aquaculture water environments. Previous studies have shown that the decrease in pH induces an increase of the oxygen consumption rate of shrimp (Chen and Lin, 1995). Large water exchange strategies are often adopted to maintain water quality in intensive and semi-intensive shrimp farming when water quality deteriorates, which increases the risk of pathogen invasion and transmission, energy consumption, and labor cost. Therefore, fresh BSFL are suitable for practical application in high-density shrimp culture.
Microbiota analysis of shrimp intestine
Generally, the high intestinal microbial richness and diversity are considered a positive phenomenon because they usually represent the better health status of the host (Sekirov et al., 2010; Apper et al., 2016). In this experiment, the richness and diversity of intestinal microbiota of L. vannamei can be enhanced when commercial feeds were replaced by fresh BSFL. This was in agreement with the findings of most studies, which indicate that the shrimp community is plastic and can be manipulated by the addition of insects to the diets. Specifically, Foysal et al. (2019) found that the diversity and richness of intestinal microbiota in marron (C. cainii) was positively correlated with dietary BSFL meal level. In addition, the richness and diversity of intestinal microbiota in rainbow trout were significantly improved after FM was partially replaced by BSFL meal in the diets (Terova et al., 2019). In addition, the composition of the dominant intestinal microbiota of L. vannamei was also significantly affected by the diet containing fresh BSFL. Previous studies showed that the intestinal microbiota of L. vannamei was dominated by Proteobacteria and Bacteroidetes, which constitute the core flora, regardless of the diet administered (Yuan et al., 2019). However, chronic intestinal microecological disorders or intestinal inflammation was often caused by the excessive relative abundance of Proteobacteria (Mukhopadhya et al., 2012; Shin et al., 2015). In addition, intestinal inflammation is usually accompanied by the increase of Proteobacteria and by the decrease of Bacteroidetes. We suggest that chitin in BSFL may be a key factor in improving the intestinal health of shrimp. It was known that the insects contain a large amount of chitin which is a mucopolysaccharide polymer hardly digested by many aquatic animal species. However, many studies found chitin added to the diet of aquatic animals can improve the diversity of intestinal microbiota and inhibit the growth of pathogens in the intestine (Zhou et al., 2013; Karlsen et al., 2017; Udayangani et al., 2017). In addition, fresh BSFL are also rich in lauric acid, a medium-chain fatty acid that inhibits Gram-positive bacteria and plays a role in regulating the composition of intestinal bacteria (Skřivanová et al., 2005; Skřivanová et al., 2006).
However, feeding fresh BSFL inhibited the relative abundance of the Firmicutes. In this experiment, only the control group was characterized by a high abundance of Firmicutes. This was inconsistent with previous findings in rainbow trout (O. mykiss), a higher relative abundance of Firmicutes was found in the insect-containing groups (Terova et al., 2019). Bruni et al. (2018) found that Firmicutes were only detected in the fecal samples of O. mykiss fed with BSFL. Firmicutes include different lactic acid bacteria, which are generally considered to be beneficial microbes. Firmicutes play an important role in breaking down indigestible carbohydrates such as starches and fibers, thereby contributing to making more efficient use of energy in diets (Smriga et al., 2010; Catalán et al., 2018). Thus, feeding fresh BSFL has a negative effect on the digestibility of shrimp. It was analyzed that the relative abundance of Firmicutes in the intestine of shrimp may be limited by two factors. Firstly, the plant component of the diet was typically associated with higher Firmicutes found in several studies (Heikkinen et al., 2006; Desai et al., 2012). The decrease in the relative abundance of Firmicutes may be caused by a reduction in the plant component of the fresh BSFL feeding regime. Secondly, the composition of the intestinal microbiota of aquatic animals was also modulated by the insect-rearing substrates. The highest inhibitory activities against Gram-positive bacteria were found in larvae reared on chitin, cellulose, bacteria, and plant oil (Vogel et al., 2018). The wheat bran was used as a rearing substrate for BSFL in this study, which may also explain the lower relative abundance of Firmicutes as Gram-positive bacteria in the intestine of shrimp. These results also remind us that additional probiotics belonging to Firmicutes should be added to the diet to improve the digestibility of shrimp when commercial feeds are replaced by fresh BSFL, such as Streptococcus, Lactobacillus, and Carnobacterium.
At the level of genus, fresh BSFL can promote the relative abundance of probiotic bacteria while suppressing the relative abundance of Vibrio. Some unique probiotics were only found in the fresh BSFL-containing groups, such as Motilimonas, Shimia, Pseudoalteromonas, and Shewanella. These bacteria designated as probiotics had been reported to be positively correlated with diets containing insects in general (Terova et al., 2019). Pseudoalteromonas can produce a variety of extracellular active substances with antimicrobial, bacteriolytic, galactose decomposition, cellulose degradation, and other functions, so it has high utilization potential and important ecological implications (Holmström and Kjelleberg, 1999). Shewanella as the dominant bacteria was found in the high fresh BSFL replacement groups, and some studies have shown that Shewanella contributes to the nutrition absorption of rainbow trout (Koziska and Pêkala, 2004). In addition, the prolific growth of Vibrio can lead to the whole microecological balance being destroyed. This ultimately results in the growth and immune capacity being inhibited and even death burst in aquatic animals (Sung et al., 2001). In the present experiment, the fresh BSFL-containing groups had better water quality, which may have suppressed the relative abundance of Vibrio. Beyond that, the proliferation of beneficial bacteria can be stimulated by chitin, which can effectively compete with Vibrio.
In addition, the relative abundances of microbial taxa across the control group and fresh BSFL-containing groups in this trial were contrasted using the LEfSe analysis, which revealed that the significantly enriched probiotic bacteria again validated the positive effect of feeding fresh BSFL on the shrimp intestinal microbiota. Interestingly, the emergence of Planctomycetes as the dominant bacteria in the intestine of shrimp has not been reported in previous studies, but our results showed a significant increase in the relative abundance of Planctomycetes in the fresh BSFL-containing groups. Planctomycetes are widely found in natural systems such as seawater, freshwater, soil, and other artificial systems such as wastewater. They play an important role in farming wastewater nitrogen removal systems by generating nitrogen (N2) from total ammonia nitrogen (NH4+) and nitrite (NO2−N). Thus, Planctomycetes are considered eco-friendly bacteria (Kuypers et al., 2003; Van Teeseling et al., 2015). We suggested that the elevated relative abundance of the Planctomycetes in the shrimp intestine may be related to the change in the aquatic environment. Some studies have shown that the intestinal microbiota of aquatic animals interacts with the microflora in the water environment. In addition, the positive effect of fresh insects on water quality might benefit the enrichment of the Planctomycetes in the shrimp intestine. However, this hypothesis requires further research.
Conclusions
This study demonstrated that replacing commercial feeds by fresh black soldier fly larvae in the diets at a proper rate had positive effects on immune and digestive system in Pacific white shrimp L. vannamei with an improvement on water quality during culturing experiment. The decreased immune enzyme activities in hepatopancreas was found in groups with BSFL replacement rate above 50%, suggesting an optimal proportion of BSFL replacement should be under 50%. In the coming work, the replacement level of commercial feeds with fresh BSFL could be improved by changing the culture substrates for the insects, as well as a dietary supplementation with nutritional ingredients or probiotics, which can contribute to sustainable shrimp farming by reducing FM consumption.
Data availability statement
The datasets presented in this study can be found in online repositories. The names of the repository/repositories and accession number(s) can be found below: https://www.ncbi.nlm.nih.gov/-PRJNA859541.
Author contributions
YH designed and performed the experiments with other teammates with the help of YJ. YH drafted the manuscript. QY and AW took samples. SW and NZ measured the activity of immune enzymes. YC and AW performed the statistical analysis of the original data. SH and YM revised the manuscript. All authors contributed to the article and approved the submitted version.
Acknowledgments
We are sincerely grateful to thank Prof. Jianguang Qin, Prof. Xiaodong Li and Associate Prof. Rantao Zuo for their pieces of advice and comments on this manuscript. This work was financially supported by the National Key R&D Program of China (Grant No. 2020YFD0900202 and 2018YFD0900400), Project of Selecting Best Candidates to Lead Key Researches in Liaoning 2021JH1/10400040 and Grant for marine economic development from Liaoning provincial department of natural resources (202233).
Conflict of interest
The authors declare that the research was conducted in the absence of any commercial or financial relationships that could be construed as a potential conflict of interest.
Publisher’s note
All claims expressed in this article are solely those of the authors and do not necessarily represent those of their affiliated organizations, or those of the publisher, the editors and the reviewers. Any product that may be evaluated in this article, or claim that may be made by its manufacturer, is not guaranteed or endorsed by the publisher.
References
Adelizi P. D., Rosati R. R., Warner K., Wu Y. V., Muench T. R. (1998). Evaluation of fish-meal free diets for rainbow trout, Oncorhynchus mykiss. Aquac. Nutr. 4, 255–262. doi: 10.1046/j.1365-2095.1998.00077.x
Apper E., Weissman D., Respondek F., Guyonvarch A., Baron F., Boisot P., et al. (2016a). Hydrolysed wheat gluten as part of a diet based on animal and plant proteins supports good growth performance of Asian seabass (Lates calcarifer), without impairing intestinal morphology or microbiota. Aquaculture 453, 40–48. doi: 10.1016/j.aquaculture.2015.11.018
Bondari K., Sheppard D. C. (1981). Soldier fly larvae as feed in commercial fish production. Aquaculture 24, 103–109. doi: 10.1016/0044-8486(81)90047-8
Bradford M. M. (1976). A rapid and sensitive method for the quantitation of microgram quantities of protein utilizing the principle of protein-dye binding. Analytical Biochem. 72, 248–254. doi: 10.1016/0003-2697(76)90527-3
Bruni L., Pastorelli R., Viti C., Gasco L., Parisi G. (2018). Characterisation of the intestinal microbial communities of rainbow trout (Oncorhynchus mykiss) fed with Hermetia illucens (black soldier fly) partially defatted larva meal as partial dietary protein source. Aquaculture 487, 56–63. doi: 10.1016/j.aquaculture.2018.01.006
Catalán N., Villasante A., Wacyk J., Ramírez C., Romero J. (2018). Fermented soybean meal increases lactic acid bacteria in gut microbiota of atlantic salmon (Salmo salar). Probiotics Antimicrobial Proteins 10, 1–11. doi: 10.1007/s12602-017-9366-7
Chakravortty D., Hensel M. (2003). Inducible nitric oxide synthase and control of intracellular bacterial pathogens. Microbes Infection 5, 621–627. doi: 10.1016/S1286-4579(03)00096-0
Cha S. H., Lee J. S., Song C. B., Lee K. J., Jeon Y. J. (2008). Effects of chitosan-coated diet on improving water quality and innate immunity in the olive flounder, Paralichthys olivaceus. Aquaculture 278, 110–118. doi: 10.1016/j.aquaculture.2008.01.025
Chen J. C., Lin C. Y. (1995). Responses of oxygen consumption, ammonia-n excretion and urea-n excretion of Penaeus chinensis exposed to ambient ammonia at different salinity and pH levels. Aquaculture 136, 243–255. doi: 10.1016/0044-8486(95)01060-2
Cruz-Suárez L. E., Nieto-López M., Guajardo-Barbosa C., Tapia-Salazar M., Scholz U., Ricque-Marie D, et al. (2007). Replacement of fish meal with poultry by-product meal in practical diets for Litopenaeus vannamei, and digestibility of the tested ingredients and diets. Aquaculture 272, 466–476. doi: 10.1016/j.aquaculture.2007.04.084
Cummins V. C., Rawles S. D., Thompson K. R., Velasquez A., Kobayashi Y., and Hager J, et al. (2017). Evaluation of black soldier fly (Hermetia illucens) larvae meal as partial or total replacement of marine fish meal in practical diets for pacific white shrimp (Litopenaeus vannamei). Aquaculture 473, 337–344. doi: 10.1016/j.aquaculture.2017.02.022
Desai A. R., Links M. G., Collins S. A., Mansfield G. S., Hill J. E. (2012). Effects of plant-based diets on the distal gut microbiome of rainbow trout (Oncorhynchus mykiss). Aquaculture 350-353, 134–142. doi: 10.1016/j.aquaculture.2012.04.005
Diener S., Zurbruegg C., Tockner K. (2009). Conversion of organic material by black soldier fly larvae: Establishing optimal feeding rates. Waste Manag. Res. 27, 603–610. doi: 10.1177/0734242X09103838
Eaton A. D., Clesceri L. S., Rice E. W., Greenberg A. E., Franson M. (2005). Standard methods for the examination of water and waste water. Centennial Edition., APHA, AWWA, WEF, Washington, DC
Ebeling J. M., Timmons M. B., Bisogni J. J. (2006). Engineering analysis of the stoichiometry of photoautotrophic, autotrophic, and heterotrophic removal of ammonia-nitrogen in aquaculture systems. Aquaculture 257, 346–358. doi: 10.1016/j.aquaculture.2006.03.019
Emehinaiye P. A. (2012). Growth performance of oreochromis niloticus fingerlings fed with varing levels of migratory locust (locustamigratoria) meal. Bachelor of Aquaculture and Fisheries Management, Federal University of Agriculture, Abeokuta.
Foysal M. J., Fotedar R., Tay C. Y., Gupta S. K. (2019). Dietary supplementation of black soldier fly (Hermetica illucens) meal modulates gut microbiota, innate immune response and health status of marron (Cherax cainii, Austin 2002) fed poultry-by-product and fishmeal based diets. PeerJ 7, e6891. doi: 10.7717/peerj.6891
Gasco L., Finke M., Huis A. V. (2018). Can diets containing insects promote animal health? J. Insects as Food Feed 4, 1–4. doi: 10.3920/JIFF2018.x001
Gasco L., Henry M., Piccolo G., Marono S., Gai F., Renna M., et al. (2016). Tenebrio molitor meal in diets for European sea bass (Dicentrarchus labrax l.) juveniles: Growth performance, whole body composition and in vivo apparent digestibility. Anim. Feed Sci. Technol. 220, 34–45. doi: 10.1016/j.anifeedsci.2016.07.003
Heikkinen J., Vielma J., Kemil Inen O., Tiirola M., Eskelinen P., Kiuru T., et al. (2006). Effects of soybean meal based diet on growth performance, gut histopathology and intestinal microbiota of juvenile rainbow trout (Oncorhynchus mykiss). Aquaculture 261 (1), 259–268. doi: 10.1016/j.aquaculture.2006.07.012
He Y., Liu X., Zhang N., Wang S., Wang A., Zuo R., et al. (2022). Replacement of commercial feed with fresh black soldier fly (Hermetia illucens) larvae in pacific white shrimp (Litopenaeus vannamei). Aquacult. Nutr. 2022, 9130400. doi: 10.1155/2022/9130400
Holmström C., Kjelleberg S. (1999). Marine pseudoalteromonas species are associated with higher organisms and produce biologically active extracellular agents. FEMS Microbiol. Ecol. 30, 285–293. doi: 10.1111/j.1574-6941.1999.tb00656.x
Karlsen Ø., Amlund H., Berg A., Olsen R. E. (2017). The effect of dietary chitin on growth and nutrient digestibility in farmed Atlantic cod, Atlantic salmon and Atlantic halibut. Aquacult. Res. 48, 123–133. doi: 10.1111/are.12867
Kautsky N., Rönnbäck P., Tedengren M., Troell M. (2000). Ecosystem perspectives on management of disease in shrimp pond farming. Aquaculture 191, 145–161. doi: 10.1016/S0044-8486(00)00424-5
Khoushab F., Yamabhai M. (2010). Chitin research revisited. Mar. Drugs 8, 1988–2012. doi: 10.3390/md8071988
Koziska A., Pêkala A. (2004). First isolation of shewanella putrefaciens from freshwater fish - a potential new pathogen of fish. Bulletin- Eur. Assoc. Fish. Pathol. 24 (4), 189–193.
Kuypers M. M. M., Sliekers A. O., Lavik G., Schmid M., Jørgensen B. B., Kuenen J. G., et al. (2003). Anaerobic ammonium oxidation by anammox bacteria in the black Sea. Nature 422 (6932), 608–611. doi: 10.1038/nature01472
Lai H., Hou J., Su C., Chen C. (2009). Effects of chloramphenicol, florfenicol, and thiamphenicol on growth of algae chlorella pyrenoidosa, isochrysis galbana, and tetraselmis chui. Ecotoxicol. Environ. Saf. 72, 329–334. doi: 10.1016/j.ecoenv.2008.03.005
Lemos D., Lawrence A. L., Iii A. (2009). Prediction of apparent protein digestibility of ingredients and diets by in vitro pH-stat degree of protein hydrolysis with species-specific enzymes for juvenile pacific white shrimp Litopenaeus vannamei. Aquaculture 295, 89–98. doi: 10.1016/j.aquaculture.2009.06.011
Li S., Ji H., Zhang B., Zhou J., Yu H. (2017). Defatted black soldier fly (Hermetia illucens) larvae meal in diets for juvenile Jian carp (Cyprinus carpio var. Jian): Growth performance, antioxidant enzyme activities, digestive enzyme activities, intestine and hepatopancreas histological structure. Aquaculture 477, 62–70. doi: 10.1016/j.aquaculture.2017.04.0157
Li X., Li J., Wang Y., Fu L., Fu Y., Li B., et al. (2011). Aquaculture industry in china: Current state, challenges, and outlook. Rev. Fish. Sci. 19 (3), 187–200. doi: 10.1080/10641262.2011.573597
Llewellyn M. S., Boutin S., Hoseinifar S. H., Derome N. (2014). Teleost microbiomes: The state of the art in their characterization, manipulation and importance in aquaculture and fisheries. Front. Microbiol. 5, 207. doi: 10.3389/fmicb.2014.00207
Marinho-Soriano E., Nunes S. O., Carneiro M., Pereira D. C. (2009). Nutrients' removal from aquaculture wastewater using the macroalgae gracilaria birdiae. Biomass Bioenergy 33, 327–331. doi: 10.1016/j.biombioe.2008.07.002
Maslowski K. M., Mackay C. R. (2011). Diet, gut microbiota and immune responses. Nat. Immunol. 12, 5–9. doi: 10.1038/ni0111-5
Mcgraw W. J., Davis D. A., Oddington D. T., Rouse D. B. (2010). Acclimation of litopenaeus vannamei postlarvae to low salinity: Influence of age, salinity endpoint, and rate of salinity reduction. J. World Aquacult. Soc. 33, 78–84. doi: 10.1111/j.1749-7345.2002.tb00481.x
Meneguz M., Schiavone A., Gai F., Dama A., Lussiana C., Renna M., et al. (2018). Effect of rearing substrate on growth performance, waste reduction efficiency and chemical composition of black soldier fly (Hermetia illucens) larvae. J. Sci. Food Agric. 98, 5776–5784. doi: 10.1002/jsfa.9127
Mukhopadhya I., Hansen R., El-Omar E. M., Hold G. L. (2012). IBD–what role do proteobacteria play? Nat. Rev. Gastroenterol. Hepatol. 9, 219–230. doi: 10.1038/nrgastro.2012.14
Newton G. L., Booram C. V., Barker R. W., Hale O. M. (1977). Dried hermetia illucens larvae meal as a supplement for swine. J. Anim. Sci. 44, 395–400. doi: 10.2527/jas1977.443395x
Ngo D. H., Kim S. K. (2014). Antioxidant effects of chitin, chitosan, and their derivatives. Adv. Food Nutr. Res. 73, 15–31. doi: 10.1016/B978-0-12-800268-1.00002-0
Oyarzun P., Alarcon L., Calabriano G., Bejarano J., Nunez D., et al. (2019). Trickling filter technology for biotreatment of nitrogenous compounds emitted in exhaust gases from fishmeal plants. J. Environ. Manage. 232, 165–170. doi: 10.1046/j.1365-2095.1998.00077.x
Paez-Osuna F. (2001). The environmental impact of shrimp aquaculture: A global perspective. Environ. pollut. 112, 229–231. doi: 10.1016/S0269-7491(00)00111-1
Pullin R. S., Lowe-McConnell R. H. (1982). The biology and culture of tilapias: Proceedings of the international conference on the biology and culture of tilapias, 2-5 September 1980 at the study and conference center of the Rockefeller foundation (Bellagio, Italy: WorldFish).
Rios A., Lefebvre T., Hong D., Henry M., Motte C. (2019). Replacing fish meal with defatted insect meal (Yellow mealworm tenebrio molitor) improves the growth and immunity of pacific white shrimp (Litopenaeus vannamei). Anim. Open Access J. From MDPI 9 (5), 258. doi: 10.3390/ani9050258.
Rice E. W., Baird R. B., Eaton A. D., Clesceri L. S. (2012). Standard methods for the examination of water and wastewater. Washington, DC: American Public Health Association.
Samira H., Mehdi S. M. (2015). The effect of replacing fish meal in the diet with Enzyme-Treated soybean meal (HP310) on growth and body composition of rainbow trout fry. Molecules 20(12). doi: 10.3390/molecules201219751.
Saoud I. P., Davis D. A., Rouse D. B. (2003). Suitability studies of inland well waters for Litopenaeus vannamei culture. Aquaculture 217, 373–383. doi: 10.1016/S0044-8486(02)00418-0
Sekirov I., Russell S. L., Antunes L. C. M., Finlay B. B. (2010). Gut microbiota in health and disease. Physiol. Rev 90 (3), 859–904. doi: 10.1152/physrev.00045.2009
Sgeirsson B., Hartemink R., Chlebowski J. F. (1995). Alkaline phosphatase from Atlantic cod (Gadus morhua). kinetic and structural properties which indicate adaptation to low temperatures. Comp. Biochem. Physiol. Part B Biochem. Mol. Biol. 110, 315–329. doi: 10.1016/0305-0491(94)00171-P
Shin N. R., Whon T. W., Bae J. W. (2015). Proteobacteria: Microbial signature of dysbiosis in gut microbiota. Trends Biotechnol. 33 (9), 496–503. doi: 10.1016/j.tibtech.2015.06.011
Shumo M., Osuga I. M., Khamis F. M., Tanga C. M., Fiaboe K., Subramanian S., et al. (2019). The nutritive value of black soldier fly larvae reared on common organic waste streams in Kenya. Sci. Rep. 9 (1), 10110. doi: 10.1038/s41598-019-46603-z
Skřivanová E., Marounek M., Benda V., Březina P. (2006). Susceptibility of escherichia coli, salmonella sp. and clostridium perfringens to organic acids and monolaurin. Veterinární Medicína 51, 81–88.
Skřivanová E., Marounek M., Dlouha G., Kaňka J. (2005). Susceptibility of clostridium perfringens to C2–C18 fatty acids. Lett. Appl. Microbiol. 41, 77–81. doi: 10.1111/j.1472-765X.2005.01709.x
Smriga S., Sandin S. A., Azam F. (2010). Abundance, diversity, and activity of microbial assemblages associated with coral reef fish guts and feces. FEMS Microbiol. Ecol. 73, 31–42. doi: 10.1111/j.1574-6941.2010.00879.x
Spranghers T., Ottoboni M., Klootwijk C., Ovyn A., Smet S. D. (2017). Nutritional composition of black soldier fly ( Hermetia illucens ) prepupae reared on different organic waste substrates. J. Sci. Food Agric. 97, 2594–2600. doi: 10.1002/jsfa.8081
Sung H. H., Hsu S. F., Chen C. K., Ting Y. Y., Chao W. L. (2001). Relationships between disease outbreak in cultured tiger shrimp (Penaeus monodon) and the composition of vibrio communities in pond water and shrimp hepatopancreas during cultivation. Aquaculture 192, 101–110. doi: 10.1016/S0044-8486(00)00458-0
Terova G., Rimoldi S., Ascione C., Gini E., Ceccotti C., Gasco L., et al. (2019). Rainbow trout (Oncorhynchus mykiss) gut microbiota is modulated by insect meal from hermetia illucens prepupae in the diet. Rev. Fish. Biol. Fish. doi: 10.1007/s11160-019-09558-y
Udayangani R. M. C., Dananjaya S. H. S., Nikapitiya C., Heo G. J., Zoysa M. D. (2017). Metagenomics analysis of gut microbiota and immune modulation in zebrafish ( Danio rerio ) fed chitosan silver nanocomposites. Fish. Shellfish Immunol. 66, 173–184. doi: 10.1016/j.fsi.2017.05.018
Van Huis A. (2013). Potential of insects as food and feed in assuring food security. Annu. Rev. Entomology 58, 563–583. doi: 10.1146/annurev-ento-120811-153704
Van Teeseling M. C., Mesman R. J., Kuru E., Espaillat A., Cava F., Brun Y. V., et al. (2015). Anammox planctomycetes have a peptidoglycan cell wall. Nat. Commun. 6 (1), 1–6. doi: 10.1038/ncomms7878
Vogel H., Müller A., Heckel D., Gutzeit H., Vilcinskas A. (2018). Nutritional immunology: Diversification and diet-dependent expression of antimicrobial peptides in the black soldier fly Hermetia illucens. Dev. Comp. Immunol 78, 141–148. doi: 10.1016/j.dci.2017.09.008
Wang Q., Li Z., Lian Y., Du X., Zhang S., Yuan J., et al. (2016). Farming system transformation yields significant reduction in nutrient loading: Case study of hongze lake, Yangtze river basin, China. Aquaculture 457, 109–117. doi: 10.1016/j.aquaculture.2016.02.025
Xiao X., Jin P., Zheng L., Cai M., Yu Z., Yu J., et al. (2018). Effects of black soldier fly (Hermetia illucens) larvae meal protein as a fishmeal replacement on the growth and immune index of yellow catfish (Pelteobagrus fulvidraco). Aquacult. Res. 49 (4), 1569–1577. doi: 10.1111/are.13611
Yuan Y., Jin M., Luo J., Xiong J., Ward T. L., Ji F., et al. (2019). Effects of different dietary copper sources on the growth and intestinal microbial communities of pacific white shrimp (Litopenaeus vannamei). Aquacult. Nutr. 25(4), 828–840. doi: 10.1111/anu.12901
Yuan J., Zhang X., Li F., Xiang J. (2021). Genome sequencing and assembly strategies and a comparative analysis of the genomic characteristics in penaeid shrimp species. Front. Genet. 12, 658619. doi: 10.3389/fgene.2021.658619
Zhang S. P., Li J. F., Wu X. C., Zhong W. J., Xian J. A., et al. (2013). Effects of different dietary lipid level on the growth, survival and immune-relating genes expression in pacific white shrimp, Litopenaeus vannamei. Fish. Shellfish Immunol. 34 (5), 1131–1138. doi: 10.1016/j.fsi.2013.01.016
Keywords: Pacific white shrimp Litopenaeus vannamei, immune enzyme activity, water quality, intestinal microbiota, black soldier fly Hermetia illucens
Citation: He Y, Zhang N, Wang A, Wang S, Che Y, Huang S, Yi Q, Ma Y and Jiang Y (2022) Positive effects of replacing commercial feeds by fresh black soldier fly (Hermetia illucens) larvae in the diets of Pacific white shrimp (Litopenaeus vannamei): Immune enzyme, water quality, and intestinal microbiota. Front. Mar. Sci. 9:987363. doi: 10.3389/fmars.2022.987363
Received: 07 July 2022; Accepted: 16 August 2022;
Published: 08 September 2022.
Edited by:
Helena Peres, University of Porto, PortugalReviewed by:
Ida Kinasih, Universitas Islam Negeri Sunan Gunung Djati Bandung, IndonesiaFernanda Cornejo, National Autonomous University of Mexico, Mexico
Jia-Song Zhang, South China Sea Fisheries Research Institute (CAFS), China
Chengbo Sun, Guangdong Ocean University, China
Copyright © 2022 He, Zhang, Wang, Wang, Che, Huang, Yi, Ma and Jiang. This is an open-access article distributed under the terms of the Creative Commons Attribution License (CC BY). The use, distribution or reproduction in other forums is permitted, provided the original author(s) and the copyright owner(s) are credited and that the original publication in this journal is cited, in accordance with accepted academic practice. No use, distribution or reproduction is permitted which does not comply with these terms.
*Correspondence: Yusheng Jiang, jys@dlou.edu.cn