- College of Animal Science and Technology, Anhui Agricultural University, Hefei, China
Elucidating the mechanisms of alkaline tolerance in freshwater teleosts will help in the development of commercial saline-alkaline aquaculture. The large-scale loach (Paramisgurnus dabryanus) is a viable species for such aquaculture, but the mechanisms of its tolerance of alkaline water are unclear. Large-scale loach was exposed to 40, 50, and 60 mmol L-1 NaHCO3 for 12, 48, and 96 h to evaluate the transcriptional changes of branchial Rhesus (Rh) glycoproteins, and aquaporins (Aqp)1 and Aqp3. Rhag transcript levels increased with longer exposure times. Rhag expression also rose considerably at higher carbonate alkalinities. Rhbg mRNA levels declined significantly under carbonate alkalinity exposure. A marked up-regulation of Rhcg was observed in the gills of the loach. Exposure to 60 mmol L-1 NaHCO3 also induced a significant up-regulation of aqp1. By contrast, aqp3 expression was significantly lower after 48 h exposure. The current findings reveal that the large-scale loach up-regulates Rhag and Rhcg to enhance ammonia efflux from the gills when exposed to high alkalinity. It is proposed that this species maintains appropriate osmolality when adapting to an alkaline environment by down-regulating aqp3 (to impede urea removal) and up-regulating aqp1 in the gills (to excrete excessive internal water).
Introduction
Saline-alkaline water is widely distributed across the world, with an estimated 45.87 million hectares in China alone (Lin et al., 2013; Song et al., 2021). It was characterized by high salinity, high carbonate alkalinities, and high pH, that pose homeostatic challenges to aquatic species (Geng et al., 2016; KoKou et al., 2019; Pellegrin et al., 2020; Song et al., 2021). Only a few native species, such as Gymnocypris przewalskii (Li et al., 2020) and Leuciscus waleckii (Xu et al., 2013), can inhabit such environments. Selecting or breeding saline-alkaline-resistant fish strains and developing commercial saline-alkaline aquaculture production have become efficient means of sustaining fisheries as the worldwide shortage of freshwater resources worsens. Consequently, there is an urgent need to improve our understanding of the mechanisms by which fish adapt to saline-alkaline waters.
The high pH of saline-alkaline water reduces H+ levels in the body, which limits NH3 efflux from the gills (Bolner and Baldisserotto, 2007). High pH also restricts Na+ influx, resulting in osmolar imbalance in fish (Cui et al., 2020). Thus, effective osmoregulation and NH3 excretion are essential for fish inhabiting saline-alkaline waters. The gills are the dominant site of osmoregulation and excretion of nitrogen waste in teleosts (Evans et al., 2005). Several channel transporters play important roles in small molecule exchange across the gills into the surrounding water. For example, Rhesus (Rh) glycoproteins, including Rh blood group-associated glycoproteins (Rhag), Rh family B glycoprotein (Rhbg), and Rh family C glycoprotein (Rhcg) have been shown to enhance NH3 outflow (Wright and Wood, 2009; Eom et al., 2020). Aquaporins (Aqps) 1 and 3 are modulated by ambient salinity and alkalinity in Oreochromis mossambicus female × O. urolepis hornorum male (Su et al., 2020), suggesting that Aqps contribute to osmoregulation in fish. Consequently, elucidating the alkaline adaptation strategies of freshwater teleosts requires an understanding of the regulatory mechanisms of channel transporters localized in their gills.
The large-scale loach (Paramisgurnus dabryanus) is one of the most economically significant farmed species in East Asia (Liu et al., 2018), with worldwide production reaching 368,406 metric tons in 2020 (valued at 865.527 million US dollars). The large-scale loach is a typical air-breathing species (Zhang et al., 2016) that exhibits excellent resistance to environmental stresses, including ammonia tolerance (Zhang et al., 2017; Zhang et al., 2019; Zhang et al., 2021; Shang et al., 2021), and may be a viable species for saline-alkaline aquaculture. However, the mechanisms of its tolerance of alkaline water remain unclear. The present study aims to examine the regulation of Rh glycoproteins and Aqps-related genes in response to high carbonate alkalinity in order to reveal their significance in the alkaline tolerance of large-scale loach. The findings will inform our understanding of the mechanisms by which freshwater fish become acclimatized to saline-alkaline environments and provide information for the breeding and selection of alkali-tolerant strains.
Materials and methods
Ethics statement
This study was carried out in accordance with the principles of the Basel Declaration and the recommendations of the Guide for the Care and Use of Laboratory Animals published by the Animal Care Committee of Anhui Agricultural University (Hefei, China). The protocol was approved by the Animal Care Committee of Anhui Agricultural University.
Experimental fish
Experimental large-scale loach (20.3 ± 2.8 g, unsexed) were obtained from a fish market in Hefei (China) and acclimated in laboratory cycling tanks containing aerated freshwater (water temperature 23.0 ± 1.0 °C, dissolved oxygen ≥ 5.0 mg L-1) for 7 days. During acclimatization, the fish were fed a commercial meal twice daily (crude protein 35%, crude lipid 7%).
Experimental design
After 24 h fasting, fish were exposed to various concentrations (nominally 40, 50, and 60 mmol L-1; measured values: 39.6 mmol L-1 pH 9.40, 48.0 mmol L-1 pH 9.50, and 59.6 mmol L-1 pH 9.60, respectively) of carbonate alkalinity (as NaHCO3) for 12, 48, and 96 h in a volume of 10 L at 25.0 ± 1.0 °C using a plastic box (diameter 35.5 cm, height 15.5 cm). The carbonate alkalinity levels were designed according to an acute NaHCO3 toxicityin this species reported by Wu et al., 2017. The NaHCO3 solution was completely exchanged every 24 h to maintain the alkaline level. Control fish were collected at the beginning of the experiment (0 h). Three replicate tanks containing four fish were sampled for each exposure period. Fish were not fed during the experimental period.
Sample collection
At the end of each exposure period all the fish were anaesthetized with tricaine methane sulfonate (MS-222, 200 mg L-1), executed by a blow to the head, and the gills removed by dissection. Until RNA isolation, all specimens were stored at -80 °C.
Real-time qPCR
Total RNA was isolated from samples of large-scale loach gills using an Ultrapure RNA Kit (CoWin Biotech, Beijing) following manufacturer’s instructions. The quality and integrity of the isolated RNA were verified by 2% agarose gel electrophoresis and NanoDrop 2000 spectrophotometer (Thermo Fisher Scientific, USA) and stored at -20 °C. RNA (1 μg) from each sample was reverse transcribed to cDNA using MonScript RTIII Super Mix with dsDNase (Monad Biotech, Wuhan) and a thermal cycler (T100, BIO-RAD, USA) following the manufacturer’s protocol.
Gene-specific primers were used to measure relative gene expression. (Table 1). Quantitative real-time PCR (qPCR) was carried out using AceQ qPCR SYBR Green Master Mix (Vazyme Biotech, Nanjing) and a quantitative thermal cycler (CFX Connect 96, BIO-RAD, USA) in a 20 μl reaction volume. Amplification conditions were as follows: pre-denaturation for 5 min at 95°C, followed by 40 cycles of 10 sec at 95°C and 30 sec at 60°C. Relative mRNA levels were calculated using the 2-△△Ct method as described by Livak and Schmittgen (2001), with the housekeeping gene β-actin being used as an internal standard to normalize the results.
Data analysis
Values are expressed as mean ± standard error. The variance homogeneity of the data was examined using Levene’s test. One-way (exposure time, carbonate alkalinity concentration) and two-way (exposure time × carbonate alkalinity concentration) analysis of variance (ANOVA) were used to compare mRNA expression levels. Tukey’s multiple tests were performed when significant differences were found at the 0.05 level. Statistical analyses were performed using SPSS software (SPSS Inc., Chicago, IL, USA).
Results and discussion
Changes in Rh glycoproteins-related gene expression in response to carbonate alkalinity
Significant interactions were observed between carbonate alkalinity concentration and exposure time on Rhag, Rhbg, and Rhcg expression in the gills of large-scale loach after various periods of acute exposure (two-way ANOVA, P < 0.05, Table 2). Rhag transcript levels increased markedly as exposure time increased (P < 0.05, Figure 1A). High carbonate alkalinity also induced a significant increase in Rhag expression during the various periods of NaHCO3 exposure (P < 0.05, Figure 1A). Up-regulation of the Rhag gene was previously reported in the gills of Anabas testudineus exposed to high levels of ammonia (Chen et al., 2017). Rhag in erythrocytes have been shown to enhance NH3 efflux from red cells into plasma (Wright and Wood, 2009). Rhag may be present in pillar cells between the lamellar blood space and the gill epithelium, allowing NH3 to permeate the branchial epithelium (Wright and Wood, 2009). Ammonia excretion was clearly reduced by exposure to high alkalinity (Li et al., 2020). Thus, the observed high level of Rhag transcripts accompanying the blocking of ammonia excretion might be attributed to the development of ammonia tolerance. The various periods of carbonate alkalinity exposure induced significant declines in Rhbg mRNA level (P < 0.05), while Rhbg expression was unaffected by NaHCO3 concentrations (P > 0.05, Figure 1B). Similarly, changes in Rhbg transcription in response to ammonia were not observed in Micropterus salmoides (Egnew et al., 2019), Oncorhynchus mykiss, or Cyprinus carpio (Sinha et al., 2013). The present results suggest that the function of Rhbg in large-scale loach is not primarily relevant to ammonia elimination during alkalinity stress. A marked up-regulation of Rhcg was observed in the fish after extended alkalinity exposure (P < 0.05, Figure 1C), but it was unaffected by alkalinity concentrations (P > 0.05, Table 2). Rhcg facilitates the movement of NH3 across the apical gill membrane and its excretion into surrounding water (Wright and Wood, 2009). Our results suggest that the Rhcg presented on the apical side of the branchial epithelium facilitate NH3 excretion from the gills, in accordance with observations in M. salmoides (Egnew et al., 2019), Dicentrarchus labrax (Shrivastava et al., 2019), and O. mykiss (Eom et al., 2020).
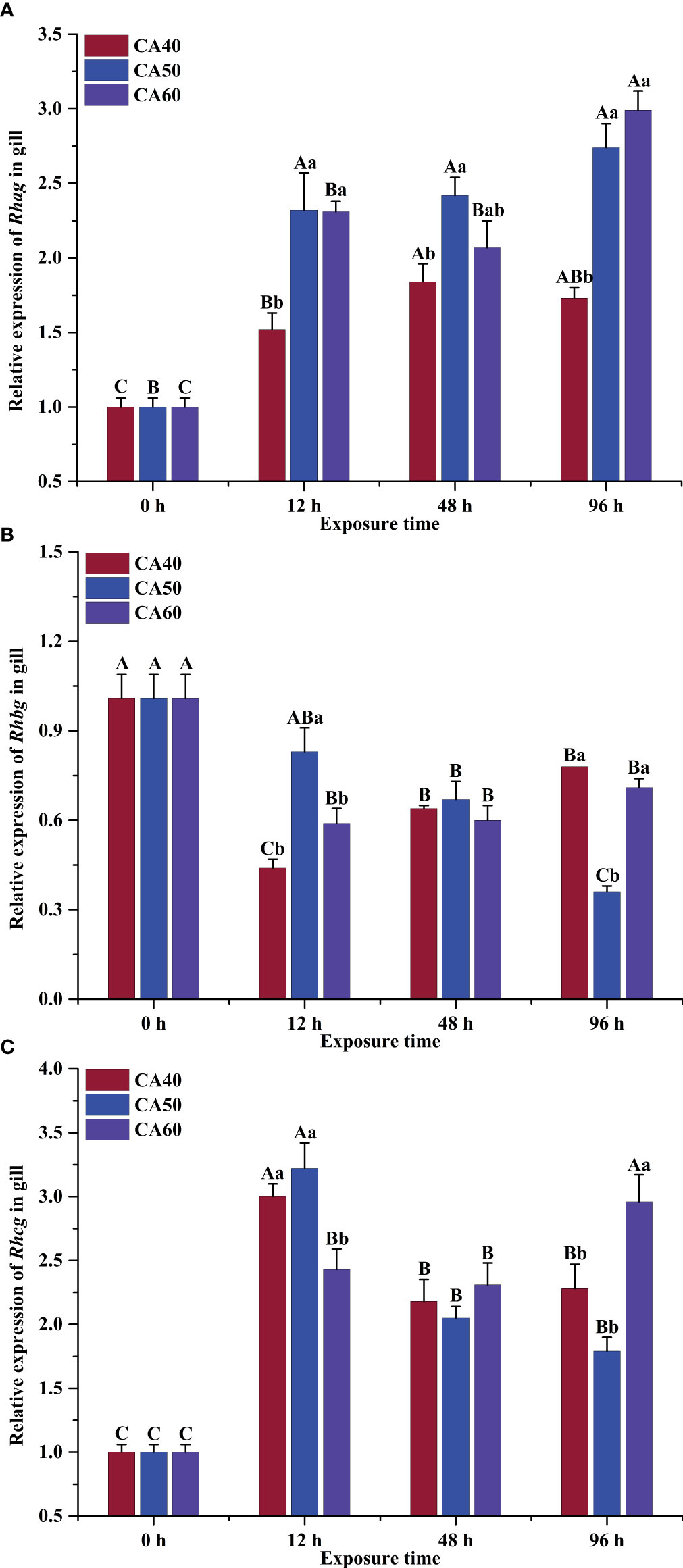
Figure 1 Effects of carbonate alkalinity (CA) on the relative expression of Rhag (A), Rhbg (B) and Rhcg (C) in the gills of large-scale loach. The different capital letters are significant differences among the different exposure times in the same CA concentration. The different lowercase letters are significant differences among the different CA concentrations at the same exposure time. The bars represent the mean ± S.E. (n=3).
Changes in Aqps-related genes expression in response to carbonate alkalinity loading
There was a significant interaction between carbonate alkalinity concentration and exposure time on aqp1 and aqp3 expression in the gills of large-scale loach (two-way ANOVA, P < 0.05, Table 2). Exposure to 60 mmol L-1 NaHCO3 induced a significant up-regulation of aqp1 (P < 0.05), while no effect was observed after the various periods of 40 and 50 mmol L-1 NaHCO3 exposure (P > 0.05, Figure 2A). Moreover, aqp1 mRNA levels increased obviously as carbonate alkalinity concentration increased (Figure 2A). The physiological function of Aqp1 in teleosts is primarily related to passive water exchange (Deane et al., 2011). Elevated ambient alkalinity resulted in the high transcription of aqp1 in the gills of large-scale loach to facilitate excretion of excess internal water and to maintain osmotic balance. Similar results were observed in the gills of hybrid tilapia after 24 h of alkaline treatment (Su et al., 2020). By contrast, aqp3 expression was found to be significantly lower in the gills of large-scale loach after 48 h of 60 mmol L-1 NaHCO3 exposure (P < 0.05), while expression in 40 and 50 mmol L-1 groups were not statistically different from the control (P > 0.05, Figure 2B). In addition to affecting water permeabilization, Aqp3 has been shown to transport urea (Cutler et al., 2007). Decreased mRNA transcription of aqp3 was also reported in O. mossambicus during seawater acclimation (Breves et al., 2016). Therefore, the absence of Aqp3 in the gills of large-scale loach may result in the blocking of urea elimination to maintain high internal osmolality.
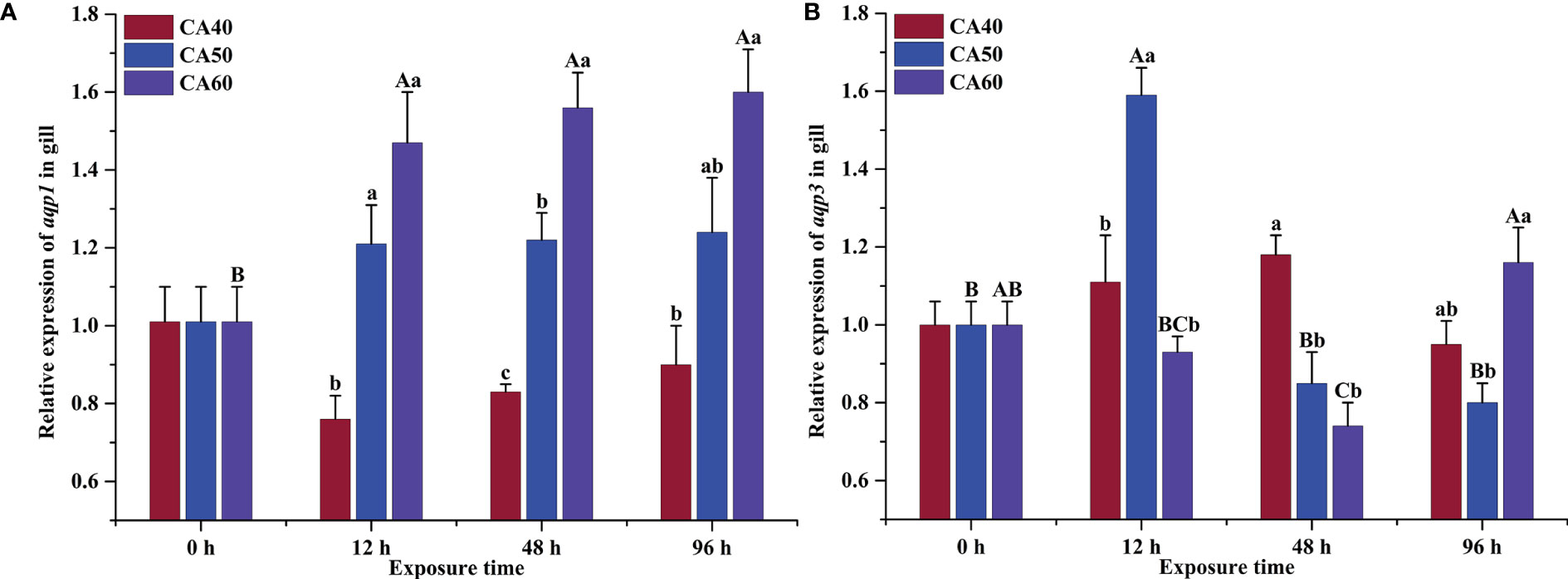
Figure 2 Effects of carbonate alkalinity (CA) on the relative expression of aqp1 (A) and aqp3 (B) in the gills of large-scale loach. The different capital letters are significant differences among the different exposure times in the same CA concentration. The different lowercase letters are significant differences among the different CA concentrations at the same exposure time. The bars represent the mean ± S.E. (n = 3).
In summary, branchial ammonia transport-related and osmoregulation-related gene expression in large-scale loach are significantly affected by carbonate alkalinity. The large-scale loach up-regulate Rhag and Rhcg to facilitate NH3 efflux from the gills during exposure to high alkalinity. Elevated transcription of aqp1 in the gills in order to excrete excess internal water, and down-regulation of aqp3 in order to block urea elimination together maintain appropriate osmolality as an adaptation to alkaline environments.
Data availability statement
The raw data supporting the conclusions of this article will be made available by the authors, without undue reservation.
Ethics statement
The animal study was reviewed and approved by Animal Care Committee of Anhui Agricultural University (Hefei, China).
Author contributions
MH, wrote the paper. M-XW, L-JZ, and DM performed the experiments and analyzed the data. Y-LZ, reviewed and edited the manuscript. All authors contributed to the article and approved the submitted version.
Funding
This work was funded by the National Natural Science Foundation of China (No. 32101248).
Conflict of interest
The authors declare that the research was conducted in the absence of any commercial or financial relationships that could be construed as a potential conflict of interest.
Publisher’s note
All claims expressed in this article are solely those of the authors and do not necessarily represent those of their affiliated organizations, or those of the publisher, the editors and the reviewers. Any product that may be evaluated in this article, or claim that may be made by its manufacturer, is not guaranteed or endorsed by the publisher.
References
Bolner K. S. C., Baldisserotto B. (2007). Water pH and urinary excretion in silver catfish Rhamdia quelen. J. Fish Biol. 70, 50–64. doi: 10.1111/j.1095-8649.2006.01253.x
Breves J. P., Inokuchi M., Yamaguchi Y., Seale A. P., Hunt B. L., Watanabe S., et al. (2016). Hormonal regulation of aquaporin 3: opposing actions of prolactin and cortisol in tilapia gill. J. Endocrinol. 230, 325–337. doi: 10.1530/JOE-16-0162
Chen X. L., Zhang B., Chng Y. R., Ong J. L. Y., Chew S. F., Wong W. P., et al. (2017). Ammonia exposure affects the mRNA and protein expression levels of certain rhesus glycoproteins in the gills of climbing perch. J. Exp. Biol. 220, 2916–2931. doi: 10.1242/jeb.157123
Cui W., Ma A., Huang Z., Wang X., Sun Z., Liu Z., et al. (2020). Transcriptomic analysis reveals putative osmoregulation mechanisms in the kidney of euryhaline turbot Scophthalmus maximus responded to hypo-saline seawater. J. Oceanol. Limnol. 38, 467–479. doi: 10.1007/s00343-019-9056-2
Cutler C. P., Martinez A.-S., Cramb G. (2007). The role of aquaporin 3 in teleost fish. Comp. Biochem. Physiol. A Mol. Integr. Physiol. 148, 82–91. doi: 10.1016/j.cbpa.2006.09.022
Deane E. E., Luk J. C., Woo N. Y. S. (2011). Aquaporin 1a expression in gill, intestine, and kidney of the euryhaline silver sea bream. Front. Physiol. 2. doi: 10.3389/fphys.2011.00039
Egnew N., Renukdas N., Ramena Y., Yadav A. K., Kelly A. M., Lochmann R. T., et al. (2019). Physiological insights into largemouth bass (Micropterus salmoides) survival during long-term exposure to high environmental ammonia. Aquat. Toxicol. 207, 72–82. doi: 10.1016/j.aquatox.2018.11.027
Eom J., Fehsenfeld S., Wood C. M. (2020). Is ammonia excretion affected by gill ventilation in the rainbow trout Oncorhynchus mykiss? Respir. Physiol. Neurobiol. 275, 103385. doi: 10.1016/j.resp.2020.103385
Evans D. H., Piermarini P. M., Choe K. P. (2005). The multifunctional fish gill: Dominant site of gas exchange, osmoregulation, acid-base regulation, and excretion of nitrogenous waste. Physiol. Rev. 85, 97–177. doi: 10.1152/physrev.00050.2003
Geng L., Tong G., Jiang H., Xu W. (2016). Effect of salinity and alkalinity on Luciobarbus capito gill Na+/K+-ATPase enzyme activity, plasma ion concentration, and osmotic pressure. BioMed. Res. Int. 2016, 4605839. doi: 10.1155/2016/4605839
KoKou F., Con P., Barki A., Nitzan T., Slosman T., Mizrahi I., et al. (2019). Short- and long-term low-salinity acclimation effects on the branchial and intestinal gene expression in the European seabass (Dicentrarchus labrax). Comp. Biochem. Physiol. A Mol. Integr. Physiol. 231, 11–18. doi: 10.1016/j.cbpa.2019.01.018
Li H., Lai Q., Yao Z., Liu Y., Gao P., Zhou K., et al. (2020). Ammonia excretion and blood gas variation in naked carp (Gymnocypris przewalskii) exposed to acute hypoxia and high alkalinity. Fish Physiol. Biochem. 46, 1981–1990. doi: 10.1007/s10695-020-00850-4
Lin T., Lai Q., Yao Z., Lu J., Zhou K., Wang H. (2013). Combined effects of carbonate alkalinity and pH on survival, growth and haemocyte parameters of the Venus clam Cyclina sinensis. Fish Shellfish Immunol. 35, 525–531. doi: 10.1016/j.fsi.2013.05.006
Liu J., Wang Q., Yuan J., Zhang T., Ye S., Li W., et al. (2018). “Integrated rice-field aquaculture in China, a long-standing practice, with recent leapfrog developments,” in Aquaculture in China: Success stories and modern trends, vol. 180 . Eds. Gui J.-F., Tang Q., Li Z., Liu J., Silva S. S. D. (Hoboken: John Wiley & Sons Ltd).
Livak K. J., Schmittgen T. D. (2001). Analysis of relative gene expression data using realtime quantitative PCR and the 2-ΔΔCT method. Methods 25, 402–408. doi: 10.1006/meth.2001.1262
Pellegrin L., Nitz L. F., Maltez L. C., Copatti C. E., Garcia L. (2020). Alkaline water improves the growth and antioxidant responses of pacu juveniles (Piaractus mesopotamicus). Aquaculture 519, 734713. doi: 10.1016/j.aquaculture.2019.734713
Shang Z.-H., Huang M., Wu M.-X., Mi D., You K., Zhang Y.-L. (2021). Transcriptomic analyses of the acute aerial and ammonia stress response in the gill and liver of large-scale loach (Paramisgurnus dabryanus). Comp. Biochem. Physiol. C Toxicol. Pharmacol. 250, 109185. doi: 10.1016/j.cbpc.2021.109185
Shrivastava J., Ndugwa M., Caneos W., De Boeck G. (2019). Physiological trade-offs, acid-base balance and ion-osmoregulatory plasticity in European sea bass (Dicentrarchus labrax) juveniles under complex scenarios of salinity variation, ocean acidification and high ammonia challenge. Aquat. Toxicol. 212, 54–69. doi: 10.1016/j.aquatox.2019.04.024
Sinha A. K., Liew H. J., Nawata C. M., Blust R., Wood C. M., De Boeck G. (2013). Modulation of Rh glycoproteins, ammonia excretion and Na+ fluxes in three freshwater teleosts when exposed chronically to high environmental ammonia. J. Exp. Biol. 216, 2917–2930. doi: 10.1242/jeb.084574
Song L., Zhao Y., Song Y., Zhao L., Ma C., Zhao J. (2021). Effects of saline-alkaline water on growth performance, nutritional processing, and immunity in Nile tilapia (Oreochromis niloticus). Aquaculture 544, 737036. doi: 10.1016/j.aquaculture.2021.737036
Su H., Ma D., Zhu H., Liu Z., Gao F. (2020). Transcriptomic response to three osmotic stresses in gills of hybrid tilapia (Oreochromis mossambicus female × O. urolepis hornorum male). BMC Genomics 21, 110. doi: 10.1186/s12864-020-6512-5
Wright P. A., Wood C. M. (2009). A new paradigm for ammonia excretion in aquatic animals: role of rhesus (Rh) glycoproteins. J. Exp. Biol. 212, 2303–2312. doi: 10.1242/jeb.023085
Wu P., Geng L., Jiang H., Tong G., Li C., Xu W. (2017). Tolerance of three cobitidae fish species to high salinity and alkalinity. J. Fishery Sci. China 24 (2), 248–257. doi: 10.3724/SP.J.1118.2017.16151
Xu J., Li Q., Xu L., Wang S., Jiang Y., Zhao Z., et al. (2013). Gene expression changes leading extreme alkaline tolerance in amur ide (Leuciscus waleckii) inhabiting soda lake. BMC Genomics 14, 682. doi: 10.1186/1471-2164-14-682
Zhang Y.-L., Shang Z.-H., Wang G.-Y., You K., Mi D. (2021). High concentrations of environmental ammonia induced changes in large-scale loach (Paramisgurnus dabryanus) immunity. Ecol. Evol. 11, 8614–9622. doi: 10.1002/ece3.7675
Zhang Y.-L., Wang G.-Y., Zhang Z.-H., Xie Y.-Y., Jin H., Dong Z.-R. (2019). Partial amino acid metabolism and glutamine synthesis as the ammonia defensive strategies during aerial exposure in Chinese loach Paramisgurnus dabryanus. Front. Physiol 10. doi: 10.3389/fphys.2019.00014
Zhang Y., Wu Q., Hu W., Wang F., Shao W., Zhang C., et al. (2016). Morphological changes and allometric growth in hatchery-reared Chinese loach Paramisgurnus dabryanus (Dabry de thiersant 1872). Chin. J. Oceanol. Limnol. 34, 757–762. doi: 10.1007/s00343-016-5079-0
Keywords: alkaline water, Rh glycoproteins, Aquaporins, air-breathing fish, osmoregulation, amonia excretion
Citation: Huang M, Wu M-X, Zhang L-J, Mi D and Zhang Y-L (2022) Effects of carbonate alkalinity on branchial gene expression in the large-scale loach (Paramisgurnus dabryanus). Front. Mar. Sci. 9:983615. doi: 10.3389/fmars.2022.983615
Received: 01 July 2022; Accepted: 25 July 2022;
Published: 11 August 2022.
Edited by:
Mohamed Ashour, National Institute of Oceanography and Fisheries (NIOF), EgyptReviewed by:
Mohammed A. E. Naiel, Zagazig University, EgyptEman Abbas, National Institute of Oceanography and Fisheries (NIOF), Egypt
Gamal Ammar, City of Scientific Research and Technological Applications, Egypt
Copyright © 2022 Huang, Wu, Zhang, Mi and Zhang. This is an open-access article distributed under the terms of the Creative Commons Attribution License (CC BY). The use, distribution or reproduction in other forums is permitted, provided the original author(s) and the copyright owner(s) are credited and that the original publication in this journal is cited, in accordance with accepted academic practice. No use, distribution or reproduction is permitted which does not comply with these terms.
*Correspondence: Yun-Long Zhang, emhhbmd5dW5sb25nQGFoYXUuZWR1LmNu