- 1Graduate School of Human Science and Environment, University of Hyogo, Himeji, Japan
- 2Himeji EcoTech Co., Ltd., Himeji, Japan
- 3Sustainable System Research Laboratory, Central Research Institute of Electric Power Industry, Abiko, Japan
- 4School of Marine Science, Kitasato University, Sagamihara-Minami, Japan
- 5Geological Survey of Japan, National Institute of Advanced Industrial Science and Technology (AIST), Tsukuba, Japan
- 6Institute of Natural and Environmental Sciences, University of Hyogo, Sanda, Japan
- 7Division of Nature and Environmental Management, Museum of Nature and Human Activities, Sanda, Japan
Larval settlement drives population and community dynamics and evolution of marine invertebrates. While it is well known that larvae sense a variety of biological and environmental factors, there is limited information on how they react to specific factors so as to locate settlement sites. Proteinaceous Waterborne Settlement Pheromone (WSP) was purified from adult barnacles, Amphibalanus amphitrite, in a previous study, but it is unclear how WSP guides barnacle cyprids to the source. Here, a series of settlement assays were conducted to investigate the concentration dependence of recombinant WSP. We report that low concentrations of barnacle WSP decrease the probability of settlement of conspecific cyprids, whereas high concentrations have the opposite effect. We suggest that this is because weak WSP informs cyprids that suitable settlement sites with adult barnacles are distant, inducing them to extend the larval phase. The present study clearly shows that larvae use WSP concentration, rather than presence/absence information. In barnacles, several other conspecific chemical/visual cues also attract larvae. This study opens the door to future research to determine how these cues influence larval behavior in nature.
Introduction
Drastic transitions from planktonic to benthic phases are very common in marine invertebrates, planktonic larvae of which drift to suitable adult habitats nearby for settlement (Pawlik, 1992; Hodin et al., 2018; Bilodeau and Hay, 2021). Planktonic larvae sense a wide array of physico-chemical and biological factors, such as settlement pheromones, to find suitable settlement sites (Pawlik, 1992; Hodin et al., 2018; Bilodeau and Hay, 2021). These govern the structure and function of benthic communities.
Coastal barnacles, which are models for studies of settlement mechanisms, are generally hermaphroditic and exchange gametes with close conspecifics. The planktonic phase starts when nauplius larvae, released by an adult, disperse in the water column. Then, the nauplii metamorphose into cyprids and cyprids explore possible substrates using a pair of appendages that bear chemo/mechano-sensory setae (Bielecki et al., 2009; Moyse, 1995; Maruzzo et al., 2011). If cyprids judge that a site is suitable, they settle and commence benthic life. Otherwise, they swim using thoracopods and continue exploration of other sites.
Adult barnacles induce settlement of conspecific cyprids nearby to facilitate reproduction. Peptide released into the seawater from adult barnacles induce settlement (Rittschof, 1990; Rittschof, 1993; Rittschof and Cohen, 2004). The active components are reportedly 3-5 kDa (Rittschof, 1985; Tegtmeyer and Rittschof, 1988) or < 500 Da (Clare and Matsumura, 2000). In addition, di- and tri-peptides with arginine or lysine at the N-terminus induce settlement (Tegtmeyer and Rittschof, 1988) although this is not supported by another study (Clare and Yamazaki, 2000). Two other proteinaceous settlement pheromones, Settlement-Inducing Protein Complex (SIPC) (Matsumura et al., 1998b) and Waterborne Settlement Pheromone (WSP) (Endo et al., 2009), are thought to induce settlement of cyprids (reviewed by Clare, 2010). Understanding how such conspecific cues guide larvae to settlement sites illuminates mechanisms underlying population/community dynamics and evolution of marine invertebrates (Jenkins, 2005; Dreanno et al., 2006b; Zimmer et al., 2016). SIPC is presumed to be adsorbed on substrate surfaces and on adult barnacle shells; therefore, it functions in the vicinity of adult barnacles (Matsumura et al., 1998b; Clare and Matsumura, 2000; Endo et al., 2009). In addition, cyprids leave SIPC footprints on the substratum during exploration, which promote larva-larva interactions (Matsumura et al., 1998c; Dreanno et al., 2006a). A recent study revealed that SIPC functions in a concentration-dependent manner (Kotsiri et al., 2018). Settlement is induced at lower SIPC concentrations, whereas it is inhibited at higher concentrations. This bimodal response of barnacle cyprids to SIPC suggests that cyprids judge the density of barnacles on the substrate and do not settle if the site is overcrowded (Kotsiri et al., 2018).
Another pheromone, WSP, purified from adults of A. amphitrite diffuses into seawater when embedded in an agarose gel, suggesting that it attracts cyprids on a relatively larger spatial scale (Endo et al., 2009; Clare, 2010). In addition, WSP was identified from cement of adult A. amphitrite (So et al., 2017; Schultzhaus et al., 2019; Schultzhaus et al., 2020; Schultzhaus et al., 2021), which indicates that WSP exists in the adhesive layer of adult barnacles. Although it remains to be confirmed that WSP exists in seawater, these findings suggest that WSP is released into the environment. WSP was also detected from a stalked barnacle, Pollicipes pollicipes (Schultzhaus et al., 2021), suggesting that a phylogenetically wide variety of barnacles use WSP to attract conspecific cyprids. Recent studies indicate that there are several homologs of WSP (So et al., 2017; Abramova et al., 2019; Schultzhaus et al., 2019; Schultzhaus et al., 2020; Schultzhaus et al., 2021). Endo et al. (2009) reported N-terminal amino acid sequences of the “original WSP”. In 2012, we sequenced more peptide fragments and determined the full-length cDNA sequence deposited in EMBL/Genbank/DDBJ databases as unpublished data (accession number AB695090), which is reported in this paper. Among the WSP homologs in A. amphitrite, this one is highly expressed during settlement (Abramova et al., 2019) and most concentrated in the cement of adult barnacles (So et al., 2017; Schultzhaus et al., 2019; Schultzhaus et al., 2020), supporting the idea that the “original WSP” is a major cyprid attractant among WSP homologs. In the present study, we extended our studies on the “original WSP” (hereafter, “WSP”). The original study showed a linear response of cyprids to WSP concentrations. High concentrations of WSP induced settlement, but low concentrations did not (Endo et al., 2009). Interpretation of these results requires caution, because larval densities in the test arena affect settlement rates (Clare et al., 1994; Head et al., 2003; Elbourne et al., 2008). This is because larva-larva interactions and settlement induction by pre-settled individuals occur when multiple larvae are added to a test arena; therefore, single-larva settlement assays are recommended to investigate factors stimulating larval settlement (Elbourne et al., 2008).
To understand how cyprids use settlement cues to find suitable settlement sites, it is essential to investigate the effect of WSP by itself. Here, we performed a series of settlement assays to investigate the effect of WSP concentration on individual cyprids using recombinant WSP.
Methods
Partial amino acid sequence analysis
WSP was purified from adult A. amphitrite according to a previous study (Endo et al., 2009). Purified protein was reduced and S-carboxymethylated with dithiothreitol and iodoacetic acid. The protein solution was dialyzed against 0.5 M Tris-HCl buffer (pH 8.5) that contained 7 M guanidine and 10 mM EDTA. After addition of 1 mM dithiothreitol, the solution was bubbled with nitrogen, and incubated for 2 h at 60°C. After incubation, 2.2 mg iodoacetic acid were added and the solution was incubated for 30 min at room temperature. Then, it was dialyzed against 50 mM Tris-HCl buffer (pH 9.0) containing 4 M urea. The carboxymethylated protein was then digested at 37°C for 15 h using lysyl endopeptidase (Achromobacter proteinase I; Wako Pure Chemicals) at an enzyme/substrate ratio of 1:200 (mol/mol). Peptide fragments generated were separated by reverse phase high-performance liquid chromatography (HPLC) using a TSKgel® ODS-120T column (4.6×250 mm; Tosoh Co., Tokyo, Japan). The column was eluted at a flow rate of 1 mL/min using a linear gradient of acetonitrile (0-80% in 120 min) in 0.1% trifluoroacetic acid. Amino acid sequences of peptide fragments were determined using a PPSQ-21A automated gas-phase protein/peptide sequencer (Shimadzu Co., Kyoto, Japan) and the following sequences were obtained (LEP1-8).
LEP1: FNVMVPK
LEP2: PEVHHEDGYFEEHGH
LEP3: EVILGNPIYHK
LEP4: MAGPRFVSPVK
LEP5: GTFNGVEEPTFSYVNNM
LEP6: VHMLTSGGIHK
LEP7: QLELQDALDIHWREGK
LEP8: NTYCIFESLSEEESTFSSFV
Based on these partial amino acid sequences, degenerate primers for cDNA amplification were designed as follows.
5′-GAGCCNCANCCNGGNGTNCCNWSNACNCCNCAGCTNGCCGG-3′
5′-TTNACNGGNSWNACRAANCKNGGNCCNGCC-3′
cDNA cloning
Adult A. amphitrite were collected in Hamana-ko, Japan, and total RNA was extracted from muscle tissue using a QuickGene-Mini80 with a QuickGene RNA tissue kit SII (FUJIFILM Co., Tokyo, Japan). mRNA was purified using an Oligotex™-dT30<Super> mRNA purification kit (TaKaRa Bio Inc., Shiga, Japan), and first strand cDNA was synthesized using a SMART™ RACE cDNA Amplification Kit (Clontech Laboratories, Inc., CA, USA) and PrimeScript™ Reverse Transcriptase (TaKaRa Bio Inc.). PCR was carried out with the cDNA template, primers described above, and an Advantage® 2 PCR Kit (Clontech Laboratories, Inc.), with initial denaturation at 95°C for 1 min, followed by 35 cycles of 95°C for 30 s, 55°C for 30 s, and 68°C for 30 s with a final extension at 68°C for 3 min. The PCR product was subjected to electrophoresis on 2% agarose gels and then extracted using a QIAquick® Gel Extraction Kit (Qiagen, GmbH, Germany). Purified PCR product was subcloned into pCR® 2.1-TOPO® vectors (Invitrogen Corporation, CA, USA), and One Shot® TOP10 Chemically Competent Escherichia coli (Invitrogen Corporation) transformants harboring plasmid constructs were screened on Luria-Bertani agar plates containing 50 μg/mL of ampicillin. Plasmid DNA was purified using a Miniprep DNA Purification Kit (TaKaRa Bio Inc), and the inserted DNA was sequenced with M13 forward (-20) and reverse primers using an ABI Prism® 3130xl Genetic Analyzer (Applied Biosystems). Complete cDNA sequences of WSP were determined using the following primers for 5’ and 3’ RACE designed from partial cDNA sequences, respectively.
5′-GTTGGTCAGCACGCCGGCCAGCTGCG-3
5′-CCAACCTGTACCGCCGCTTCCTGCACAC-3′
Amplified DNA was subcloned and sequenced as described above. The presence and locations of signal peptide cleavage sites were predicted using SignalP 3.0 (Bendtsen et al., 2004). Nucleotide sequence data reported in this paper are available from the EMBL/GenBank/DDBJ databases under accession number AB695090.
Recombinant protein
DNA fragments of the full-length ORF of WSP that lacked its signal peptide were synthesized with an Ndel site (5′-CATATG-3′) and a His-Tag (5′-CACCATCATCATCATCAT-3′) at the 5′ end and a Hind III site (5′-AAGCTT-3′) added at the 3′ end (Figure S1). Then it was ligated into pET30a vectors, and transformed into E. coli. After the IPTG incubation of E. coli, protein solution was obtained from the E. coli pellet and the recombinant WSP was purified on His Trap. A quality check and estimation of relative molecular mass were conducted using SDS-PAGE. All procedures were conducted with GenScript Japan Inc. (Tokyo, Japan).
Adult barnacles and larval culture
Adult A. amphitrite were collected in Kobe, Japan during 2021-2022, and maintained in aquaria by feeding them with brine shrimp. Nauplii released from adults were cultured in filtered sea water (FSW) containing penicillin/streptomycin (FUJIFILM Wako Pure Chemical Co. Osaka, Japan) and fed with Chaetoceros calcitrans at 22°C under a 12h:12h light cycle. Nauplii metamorphosed into cyprids after 5-6 days. Only swimming cyprids were collected. These were rinsed with FSW, and kept at 5°C for 1 day.
Larval settlement assays
At first, we conducted a multi-larva settlement assay to confirm that settlement-inducing activities of recombinant WSP were identical to those of native WSP by comparing the results with previous research (Endo et al., 2009). Recombinant protein (1, 10 and 100 nmol/L) and 10 cyprids were added to wells of 48-well polystyrene plates with 80% FSW at a final volume of 600 µL with 10 replicates. 50 mM Tris-HCl buffer (pH 7.5) was added for the control. After incubation for 5 h at 22°C in dark, the number of settled individuals were counted. Each treatment comprised 10 replicates from two batches of larvae. Binominal Generalized Linear Mixed Model (GLMM) was performed to assess the effect of WSP concentration on cyprid responses among treatments with larval batch as a random effect.
Larval settlement-inducing activities were also determined in single-larva settlement assays using 48-well polystyrene plates. Recombinant WSP (1, 10 and 100 nmol/L) and single cyprids were placed in the wells with 80% FSW at a final volume of 600 μL. After incubation for 24 h at 22°C in dark, the number of settled cyprids was counted. 50 mM Tris-HCl buffer (pH 7.5) was added for the control. Each treatment had about 48 replicates from six batches of larvae. Binominal GLMM was performed to assess the effect of WSP concentration on cyprid response among treatments with larval batch as a random effect. All statistical analyses were performed with R 4.0.4 (R Core Team, 2021). GLMM and post-hoc tests were performed using lem4 (Bates et al., 2015) and the Anova function of the car (Fox and Weisberg, 2018) package, respectively. The Holm-Bonferroni method (Holm, 1979) was used to adjust P values.
Results
cDNA cloning and recombinant protein
We determined full-length cDNA sequences that consisted of 907 bp and encoded 251 amino acids. This amino acid sequence contained a signal peptide with the N-terminal 15 residues, and the sequence was consistent with that of a previous study (Endo et al., 2009). All peptide sequences determined by amino acid sequencing were contained in the structure of WSP. Our sequence has already been cited in a recent study (Abramova et al., 2019) that showed discussed gene structure of WSP and its homologs in A. amphitrite and A. improvisus. Recombinant WSP showed a single band in SDS-PAGE with a relative molecular mass of 28.1 kDa (Figure S1).
Settlement assay with multiple larvae
Binominal Generalized Linear Mixed Model (GLMM) indicated that settlement rate was significantly higher in 100 nmol/L WSP (=2.81 µg/mL) than at lower concentrations (Figure 1). These results are consistent with a previous study (Endo et al., 2009) that showed 1.0 and 10 µg/mL of native purified WSP increased settlement rate while 0.1 µg/mL did not.
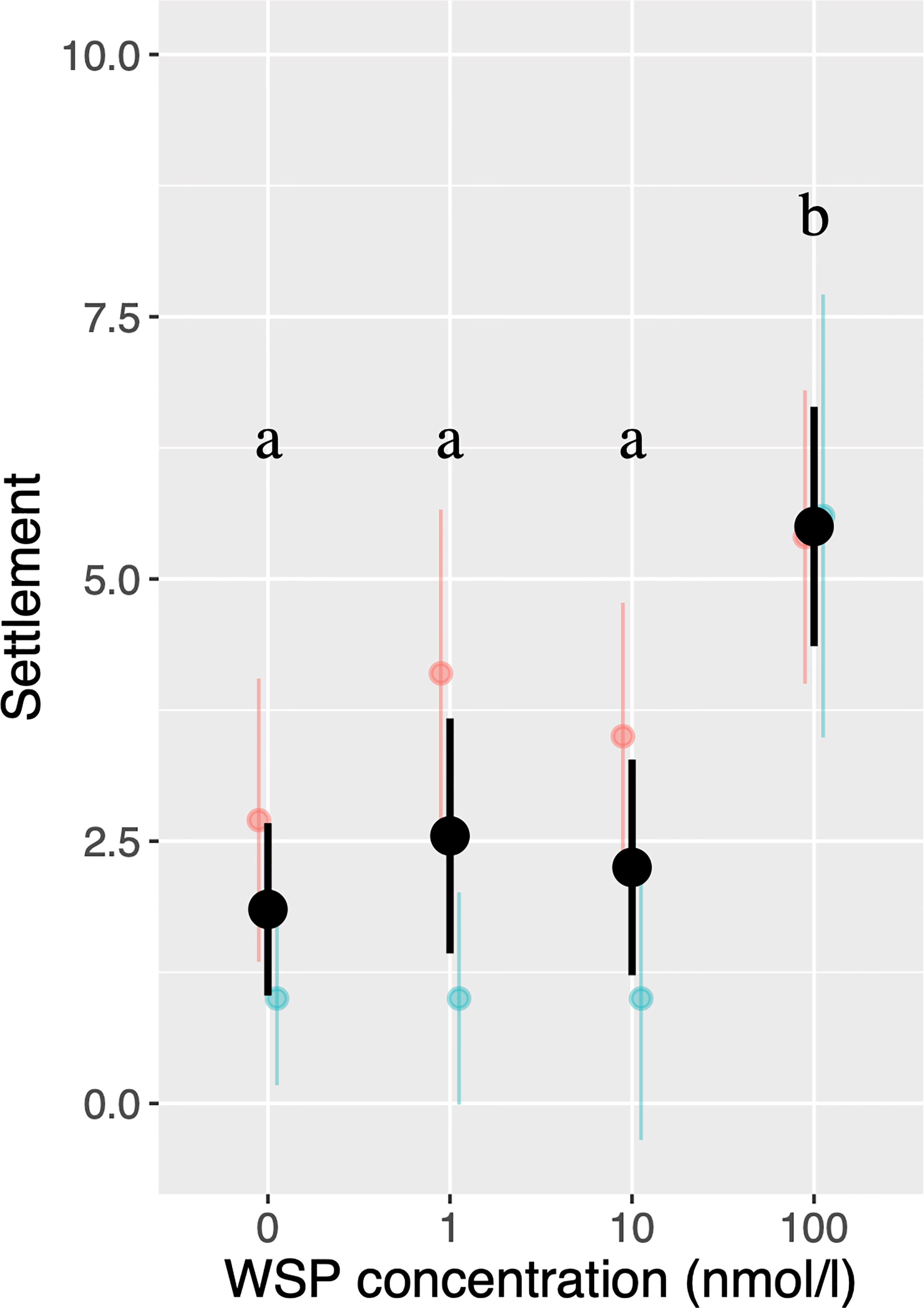
Figure 1 The number of settled cyprids in settlement assays using multiple larvae. Colors correspond to larval batches with black bars indicating results of pooled data. Bars indicate 95% confidence intervals. Different letters indicate significant differences (P < 0.05).
Single-larva settlement assay
Amphibalanus amphitrite cyprids showed a non-linear response to conspecific WSP concentration (Figure 2). While variances of settlement probabilities are high among larval batches, binominal GLMM indicated that the probability of settlement was significantly lower in 1 nmol/L WSP than in controls or in 10 nmol/L WSP. The probability of settlement was significantly higher in the 100 nmol/L treatment than in the other treatments. Out of six replicate batches, a single batch showed lower settlement probability in controls than in 1 nmol/L treatment.
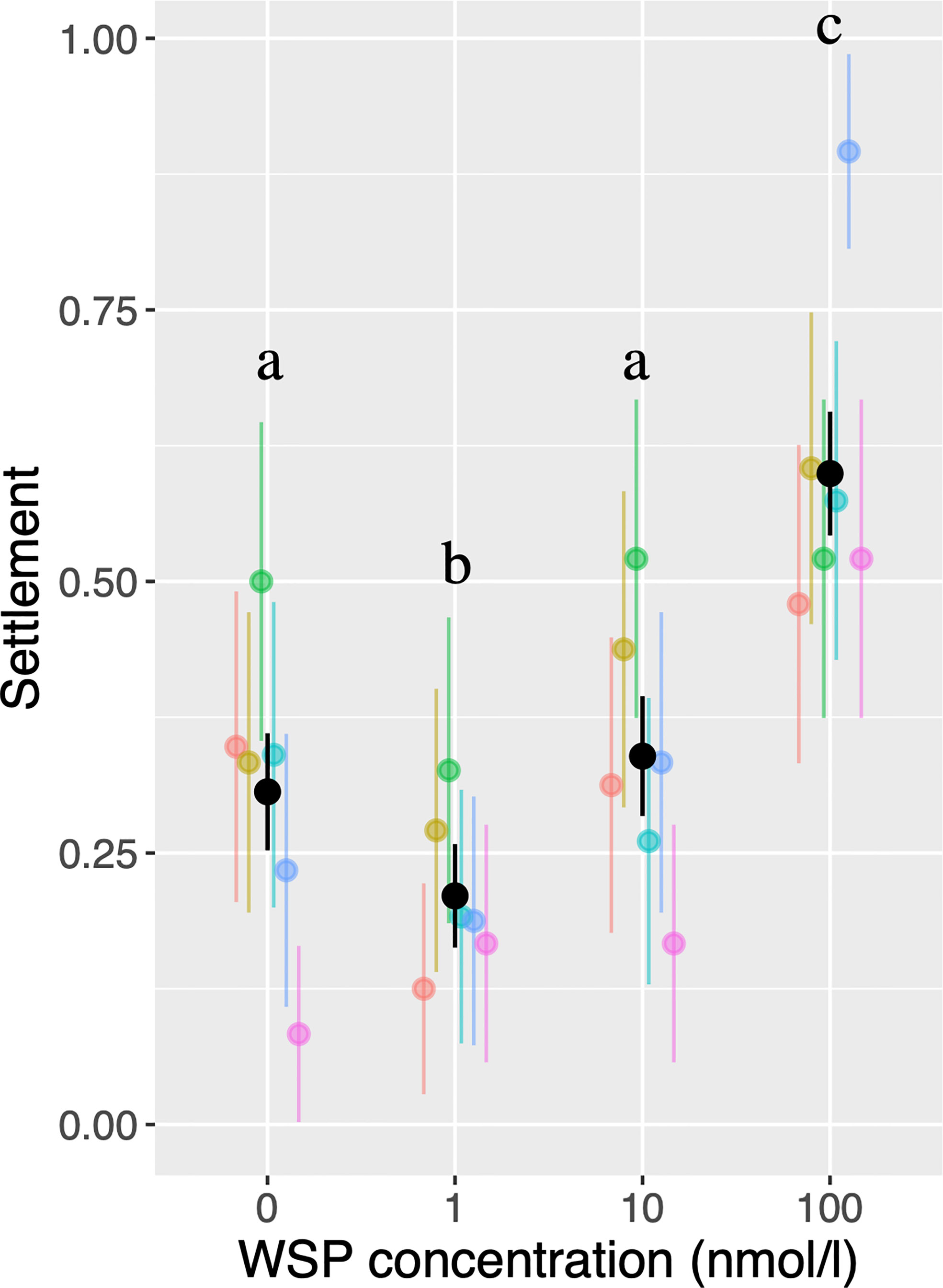
Figure 2 Probability of settlement based on single-larva assays. Colors correspond to larval batches, with black bars indicating results from pooled data. Bars indicate 95% confidence intervals. Different letters indicate significant differences (P < 0.05).
Discussion
Recombinant WSP had a relative molecular mass of 28.1 kDa, which is about 4 kDa smaller than native WSP (Endo et al., 2009). This suggests post-translational modifications, such as glycosylation in native WSP. Larval settlement-inducing activity of another pheromone, SIPC, was inhibited by lentil lectin, suggesting that sugar chains are functionally important in SIPC (Matsumura et al., 1998a). In contrast, treatments with lentil lectin had no effect on function of native WSP (Endo et al., 2009). Additionally, our assay using multiple larvae with recombinant WSP showed settlement-inducing activities that were identical to those of natural WSP reported in a previous study (Endo et al., 2009). These results suggest that sugar chains are not important for settlement-inducing activity of WSP.
A previous study showed that the settlement-inducing activity of seawater collected 1 cm from adult barnacle habitats induced settlement, while that collected 19 cm away did not (Elbourne and Clare., 2010). This suggests that WSP exists at “high” concentration levels within a few centimeters from adult habitats while it is diluted to “low” concentration levels over tens of centimeters. However, it remains unknown how WSP disperses in the field, much less how cyprids sense WSP and orient to its source. Studies on symbiotic and parasitic barnacles suggested that larvae may need to sense only the presence or absence of the host-derived chemical attractant (Pasternak et al., 2004a; Pasternak et al., 2004b). Our single-larva assays, however, clearly show that barnacle cyprids monitor the WSP concentration. If cyprids detect low levels of WSP, they may recognize that suitable adult habitat is distant from their location, and they extend their pelagic phase in an attempt to find better habitat. If cyprids detect high levels of WSP, they may recognize that adult habitat exists nearby, and are highly likely to settle. Such scenarios are consistent with a nonlinear cyprid response to WSP concentration. In all probability, WSP has an important role in guiding cyprids to suitable settlement sites, and not only in directly inducing settlement. However, these results must be interpreted with caution because the variance of settlement probability is high among larval batches, suggesting that larval responses can vary depending upon their physiological conditions (Tremblay et al., 2007). Additional study is needed to identify WSP in seawater, and to determine how cyprids orient to its source.
SIPC works as an avoidance cue when its concentration is high, indicating overcrowding (Kotsiri et al., 2018). In addition, a homolog of Balanus glandula SIPC, which has been named MULTIFUNCIN, attracts predator dogwhelks, which indicates that expression of SIPC/MULTIFUNCIN increases the predation risk for barnacle prey (Zimmer et al., 2016). In addition, waterborne peptide pheromones attract barnacle predators (Rittschof et al., 1984). In contrast to SIPC, WSP induces settlement when the concentration is high. Here, we suggest that high WSP with intermediate conspecific SIPC informs cyprids that a site has an optimal population density and reduced predation risk. However, this requires further study to clarify whether WSP also attracts predators.
Recent transcriptome and proteome studies identified homologs of WSP and SIPC/MULTIFUNCIN (So et al., 2017; Abramova et al., 2019; Schultzhaus et al., 2019; Schultzhaus et al., 2020; Schultzhaus et al., 2021). Abramova et al. (2019) suggested that the blend ratio of WSP homologs may determine conspecificity. Additionally, a distance tree of amino acid sequences of WSP homologs from A. amphitrite and Pollicipes pollicipes indicated that these proteins separate by species rather than WSP family, which suggests that WSPs have species specificity in settlement inducing activity (Schultzhaus et al., 2021). Peptides obtained from seawater conditioned by Semibalanus balanoides induced settlement of A. amphitrite cyprids (Rittschof, 1985), which suggests that peptide pheromones do not have con-specificity. In contrast, SIPC has variable regions that may contribute to species specificity (Yorisue et al., 2012) and it is suggested to function in a species-specific manner (Matsumura et al., 2000; Dreanno et al., 2007). Additional studies are required to clarify how cyprids use multiple settlement pheromones and the role of homologs for species recognition and habitat selection.
Data availability statement
The datasets presented in this study can be found in online repositories. The names of the repository/repositories and accession number(s) can be found below: EMBL/Gen-Bank/DDBJ databases under accession number AB695090.
Author contributions
SK, NE, YN, KM, and TY designed research; SK, NE, YN, and TY performed research; SK, NE, and TY analyzed data; KY and AI provided recombinant protein; SK, NE, and TY wrote the paper. All authors contributed to the article and approved the submitted version.
Funding
This study was supported by JSPS KAKENHI (Grant number 20K15576); the Research Laboratory on Environmentally-Conscious Developments and Technologies (E-code) at the National Institute of Advanced Industrial Science and Technology.
Conflict of interest
Author NE was employed by Himeji EcoTech Co., Ltd., Himeji, Japan.
The remaining authors declare that the research was conducted in the absence of any commercial or financial relationships that could be construed as a potential conflict of interest.
Publisher’s note
All claims expressed in this article are solely those of the authors and do not necessarily represent those of their affiliated organizations, or those of the publisher, the editors and the reviewers. Any product that may be evaluated in this article, or claim that may be made by its manufacturer, is not guaranteed or endorsed by the publisher.
Supplementary material
The Supplementary Material for this article can be found online at: https://www.frontiersin.org/articles/10.3389/fmars.2022.983389/full#supplementary-material
References
Abramova A., Lind U., Blomberg A., Rosenblad M. A. (2019). The complex barnacle perfume: Identification of waterborne pheromone homologues in Balanus improvisus and their differential expression during settlement. Biofouling 35, 416–428. doi: 10.1080/08927014.2019.160212
Bates D., Maechler M., Bolker B., Walker S. (2015). lme4: Linear mixed-effects models using S4 classes. J. Stat. Software 67, 1–48. doi: 10.18637/jss.v067.i01
Bendtsen J. D., Nielsen H., Von Heijne G., Brunak S. (2004). Improved prediction of signal peptides: SignalP 3.0. J. Mol. Boil. 340, 783–795. doi: 10.1016/j.jmb.2004.05.028
Bielecki J., Chan B. K. K., Høeg J. T., Sari A. (2009). Antennular sensory organs in cyprids of balanomorphan cirripedes: standardizing terminology using Megabalanus rosa. Biofouling 25, 203–214. doi: 10.1080/08927010802688087
Bilodeau S. M., Hay M. E. (2021). Chemical cues affecting recruitment and juvenile habitat selection in marine versus freshwater systems. Aquat. Ecol. 56, 1–22. doi: 10.1007/s10452-021-09905-x
Clare A. S. (2010). “Toward a characterization of the chemical cue to barnacle gregariousness” in Chemical communication in crustaceans. Eds. Breithaupt T., Thiel M. (New York: Springer), 431–450.
Clare A. S., Freet R. K., McClary M. (1994). On the antennular secretion of the cyprid of Balanus amphitrite amphitrite, and its role as a settlement pheromone. J. Mar. Biol. Assoc. U. K. 74, 243–250. doi: 10.1017/s0025315400035803
Clare A. S., Matsumura K. (2000). Nature and perception of barnacle settlement pheromones. Biofouling 15, 57–71. doi: 10.1080/08927010009386298
Clare A. S., Yamazaki M. (2000). Inactivity of glycyl-glycyl-arginine and two putative (QSAR) peptide analogues of barnacle waterborne settlement pheromone. J. Mar. Biol. Assoc. U. K. 80, 945–946. doi: 10.1017/S0025315400002952
Dreanno C., Kirby R. R., Clare A. S. (2006a). Smelly feet are not always a bad thing: The relationship between cyprid footprint protein and the barnacle settlement pheromone. Biol. Lett. 2, 423–425. doi: 10.1098/rsbl.2006.0503
Dreanno C., Kirby R. R., Clare A. S. (2007). Involvement of the barnacle settlement-inducing protein complex (SIPC) in species recognition at settlement. J. Exp. Mar. Biol. Ecol. 351, 276–282. doi: 10.1016/j.jembe.2007.07.003
Dreanno C., Matsumura K., Dohmae N., Takio K., Hirota H., Kirby R. R., et al. (2006b). An α2-macroglobulin-like protein is the cue to gregarious settlement of the barnacle balanus amphitrite. Proc. Natl. Acad. Sci. U.S.A. 103, 14396–14401. doi: 10.1073/pnas.060276310
Elbourne P. D., Clare A. S. (2010). Ecological relevance of a conspecific, waterborne settlement cue in Balanus amphitrite (Cirripedia). J. Exp. Mar. Biol. Ecol. 392, 99–106. doi: 10.1016/j.jembe.2010.04.013
Elbourne P. D., Veater R. A., Clare A. S. (2008). Interaction of conspecific cues in Balanus amphitrite Darwin (Cirripedia) settlement assays: Continued argument for the single-larva assay. Biofouling 24, 87–96. doi: 10.1080/08927010701802565
Endo N., Nogata Y., Yoshimura E., Matsumura K. (2009). Purification and partial amino acid sequence analysis of the larval settlement-inducing pheromone from adult extracts of the barnacle, Balanus amphitrite (= Amphibalanus amphitrite). Biofouling 25, 429–434. doi: 10.1080/08927010902875113
Head R., Overbeke K., Klijnstra J., Biersteker R., Thomason J. (2003). The effect of gregariousness in cyprid settlement assays. Biofouling 19, 269–278. doi: 10.1080/0892701031000101502
Hodin J., Ferner M. C., Heyland A., Gaylord B. (2018). “I feel that! fluid dynamics and sensory aspects of larval settlement across scales” in Evolutionary ecology of marine invertebrate larvae. Eds. Carrier T. J., Reitzel A. M., Heyland A. (Oxford: Oxford University Press), 190–207. doi: 10.1093/oso/9780198786962.003.0013
Holm S. A. (1979). A simple sequentially rejective multiple test procedure. Scand. J. Stat. 6, 65–70.
Jenkins S. R. (2005). Larval habitat selection, not larval supply, determines settlement patterns and adult distribution in two chthamalid barnacles. J. Anim. Ecol. 74, 893–904. doi: 10.1111/j.1365-2656.2005.00985.x
Kotsiri M., Protopapa M., Mouratidis S., Zachariadis M., Vassilakos D., Kleidas I., et al. (2018). Should I stay or should I go? The settlement-inducing protein complex guides barnacle settlement decisions. J. Exp. Biol. 221, jeb185348. doi: 10.1242/jeb.185348
Maruzzo D., Conlan S., Aldred N., Clare A. S., Høeg J. T. (2011). Video observation of surface exploration in cyprids of Balanus amphitrite: The movements of antennular sensory setae. Biofouling 27, 225–239. doi: 10.1080/08927014.2011.555534
Matsumura K., Hills J. M., Thomason P. O., Thomason J. C., Clare A. S. (2000). Discrimination at settlement in barnacles: Laboratory and field experiments on settlement behaviour in response to settlement-inducing protein complexes. Biofouling 16, 181–190. doi: 10.1080/08927010009378443
Matsumura K., Mori S., Nagano M., Fusetani N. (1998a). Lentil lectin inhibits adult extract-induced settlement of the barnacle. Balanus amphitrite. J. Exp. Zool. 280, 213–219. doi: 10.1002/(SICI)1097-010X(19980215)280:3<213::AID-JEZ2>3.0.CO;2-Q
Matsumura K., Nagano M., Fusetani N. (1998b). Purification of a larval settlement-inducing protein complex (SIPC) of the barnacle, Balanus amphitrite. J. Exp. Zool. 281, 12–20. doi: 10.1002/(SICI)1097-010X(19980501)281:1<12::AID-JEZ3>3.0.CO;2-F
Matsumura K., Nagano M., Kato-Yoshinaga Y., Yamazaki M., Clare A. S., Fusetani N. (1998c). Immunological studies on the settlement–inducing protein complex (SIPC) of the barnacle Balanus amphitrite and its possible involvement in larva–larva interactions. Proc. R. Soc London Ser. B 265, 1825–1830. doi: 10.1098/rspb.1998.0508
Moyse J. (1995). Attachment organs in cypris larvae: Using scanning electron microscopy. Crustacean Issues 10, 153–177.
Pasternak Z., Blasius B., Abelson A. (2004a). Host location by larvae of a parasitic barnacle: Larval chemotaxis and plume tracking in flow. J. Plankton Res. 26, 487–493. doi: 10.1093/plankt/fbh040
Pasternak Z., Blasius B., Achituv Y., Abelson A. (2004b). Host location in flow by larvae of the symbiotic barnacle Trevathana dentata using odour–gated rheotaxis. Proc. R. Soc London Ser. B 271, 1745–1750. doi: 10.1098/rspb.2004.2765
Pawlik J. P. (1992). Chemical ecology of the settlement of benthic marine invertebrates. Oceanogr. Mar. Biol. Annu. Rev. 30, 273–335.
R Core Team (2021). R: A language and environment for statistical computing (Vienna, Austria: R Foundation for Statistical Computing).
Rittschof D. (1985). Oyster drills and the frontiers of chemical ecology: Unsettling ideas. Bull. Am. Mar. Union 1, 111–116.
Rittschof D. (1990). Peptide-mediated behaviors in marine organisms evidence for a common theme. J. Chem. Ecol. 16, 261–272. doi: 10.1007/BF01021283
Rittschof D. (1993). Body odors and neutral-basic peptide mimics: A review of responses by marine organisms. Am. Zool 33, 487–493. doi: 10.1093/icb/33.6.487-a
Rittschof D., Cohen J. H. (2004). Crustacean peptide and peptide-like pheromones and kairomones. Peptides 25, 1503–1516. doi: 10.1016/j.peptides.2003.10.024
Rittschof D., Shepherd R., Williams L. G. (1984). Concentration and preliminary characterization of a chemical attractant of the oyster drill, Urosalpinx cinerea. J. Chem. Ecol. 10, 63–79. doi: 10.1007/BF00987644
Schultzhaus J. N., Dean S. N., Leary D. H., Hervey W. J., Fears K. P., Wahl K. J., et al. (2019). Pressure cycling technology for challenging proteomic sample processing: Application to barnacle adhesive. Integr. Biol. 11, 235–247. doi: 10.1093/intbio/zyz020
Schultzhaus J. N., Hervey W. J., Taitt C. R., So C. R., Leary D. H., Wahl K. J., et al. (2021). Comparative analysis of stalked and acorn barnacle adhesive proteomes. Open Biol. 11, 210142. doi: 10.1098/rsob.210142
Schultzhaus J. N., Wang C., Patel S., Smerchansky M., Phillips D., Taitt C. R., et al. (2020). Distribution of select cement proteins in the acorn barnacle Amphibalanus amphitrite. Front. Mar. Sci. 7. doi: 10.3389/fmars.2020.586281
So C. R., Scancella J. M., Fears K. P., Essock-Burns T., Haynes S. E., Leary D. H., et al. (2017). Oxidase activity of the barnacle adhesive interface involves peroxide-dependent catechol oxidase and lysyl oxidase enzymes. ACS Appl. Mater. Interfaces 9, 11493–11505. doi: 10.1021/acsami.7b01185
Tegtmeyer K., Rittschof D. (1988). Synthetic peptide analogs to barnacle settlement pheromone. Peptides 9, 1403–1406. doi: 10.1016/0196-9781(88)90209-4
Tremblay R., Olivier F., Bourget E., Rittschof D. (2007). Physiological condition of Balanus amphitrite cyprid larvae determines habitat selection success. Mar. Ecol. Prog. Ser. 340, 1–8. doi: 10.3354/meps340001
Yorisue T., Matsumura K., Hirota H., Dohmae N., Kojima S. (2012). Possible molecular mechanisms of species recognition by barnacle larvae inferred from multi-specific sequencing analysis of proteinaceous settlement-inducing pheromone. Biofouling 28, 605–611. doi: 10.1080/08927014.2012.695776
Keywords: larva, settlement, waterborne settlement pheromone, barnacle, cyprid
Citation: Kitade S, Endo N, Nogata Y, Matsumura K, Yasumoto K, Iguchi A and Yorisue T (2022) Faint chemical traces of conspecifics delay settlement of barnacle larvae. Front. Mar. Sci. 9:983389. doi: 10.3389/fmars.2022.983389
Received: 01 July 2022; Accepted: 24 August 2022;
Published: 29 September 2022.
Edited by:
Daniel Rittschof, Duke University, United StatesReviewed by:
Gary H. Dickinson, The College of New Jersey, United StatesChristopher So, United States Naval Research Laboratory, United States
Anthony Clare, Newcastle University, United Kingdom
Copyright © 2022 Kitade, Endo, Nogata, Matsumura, Yasumoto, Iguchi and Yorisue. This is an open-access article distributed under the terms of the Creative Commons Attribution License (CC BY). The use, distribution or reproduction in other forums is permitted, provided the original author(s) and the copyright owner(s) are credited and that the original publication in this journal is cited, in accordance with accepted academic practice. No use, distribution or reproduction is permitted which does not comply with these terms.
*Correspondence: Takefumi Yorisue, yorisue@gmail.com