- 1School of Life Sciences, Arizona State University, Tempe, AZ, United States
- 2School of Mathematical and Natural Sciences, New College, Arizona State University, Tempe, AZ, United States
- 3Center for Biodiversity Outcomes, Global Institute of Sustainability and Innovation, College of Global Futures, Arizona State University, Tempe, AZ, United States
Marine plastic pollution (MPP) has emerged as a global sustainability challenge with environmental, social, and economic consequences. This has inspired action at every scale of governance—from the local level to international institutions. However, policy and management efforts have been reactive and ad hoc, resulting in concerns about their efficacy, cost, and unintended consequences. To adequately address MPP and its global impacts, a systematic, evidence-based approach is needed. Seascape ecology, a subdiscipline of landscape ecology, is an interdisciplinary system science focused on the reciprocal relationship between the patterns and processes that shape seascapes. In this paper, we define the plastic-scape as all the social-ecological systems that interact with plastic (as a product and pollutant), the drivers and pathways of MPP, and the natural and human environments impacted by MPP. We then demonstrate the ways in which principles, methods, tools, and transdisciplinary research approaches from seascape ecology can be applied to better understand the plastic-scape, inform future MPP research and improve management strategies.
1 Introduction
Marine plastic pollution (MPP) is an urgent sustainability challenge. In 2016 alone, between 19.3 and 23.4 million metric tons of plastic entered aquatic ecosystems (Borrelle et al., 2020). This pollution has environmental, economic, and social consequences (Beaumont et al., 2019), which have inspired global stakeholder action (Xanthos and Walker, 2017; Schnurr et al., 2018). Still, even if these ambitious actions are achieved, plastic pollution emissions will continue to rise due to increased production (Borrelle et al., 2020). As MPP continues to increase, so will its social, ecological, and economic consequences (Beaumont et al., 2019).
Current management efforts for MPP are often ad hoc, without consideration for decision-makers’ goals, scale of governance, context of implementation, or systematic coordination across scales and sectors (Excell et al., 2018). Intervention efficacy is rarely evaluated and evaluated interventions report mixed outcomes (Excell et al., 2018). For example, bag regulations are among the most popular policies for plastics across the globe, yet less than half have been evaluated for effectiveness in reducing bag consumption, and 40% of evaluated policies have achieved little to no impact (Excell et al., 2018). In general, the effectiveness of popular interventions—bag bans and levies, deposit refund schemes, and dumping fines—are conditional on the context of implementation, including governance, socio-economic status, and environmental conditions (Lavee, 2010; McIlgorm et al., 2011; Oosterhuis et al., 2014; Excell et al., 2018).
Effectively implemented policies may still fail to reduce MPP. Research has shown that even if the most ambitious global commitments are achieved, annual plastic emissions will continue increase due to increased production driven by global development and population growth (Borrelle et al., 2020). This indicates that the suite of solutions being implemented are largely insufficient for addressing the primary sources and environmental pathways of MPP.
Finally, effective policy must ultimately reduce the social and ecological consequences of MPP, which depend on how MPP interacts with social and ecological communities. Not all ecosystems are equally vulnerable to MPP, and marine regions vary in their social and economical importance (Murphy et al. in review; Beaumont et al., 2019; Armoškaitė et al., 2020). As a result, policy effectiveness should not only be measured by MPP reduction, but also by social-ecological outcomes.
Failure to mitigate MPP and its consequences through current efforts has fueled calls for transformative, system-wide change along the entire plastics’ life cycle (Borrelle et al., 2020; Raubenheimer and Urho, 2020). This will require action across scales of governance that not only consider policy objectives, but also feasibility, cost, trade-offs, and efficacy for mitigating the social, ecological, and economic consequences of MPP (Tessnow-von Wysocki and Le Billon, 2019; Murphy et al., 2021; Helm et al., 2022). This approach must 1) be transdisciplinary, 2) be multi-scale, 3) be spatially-explicit, and 4) encompass the entire plastic-scape—which includes all the governance systems, human actors, and ecological components (i.e., abiotic, and biotic processes) that contribute to patterns of plastic production, use, and pollution, as well as the interactions between MPP and human and natural communities that drive its social and ecological consequences (Figure 1).
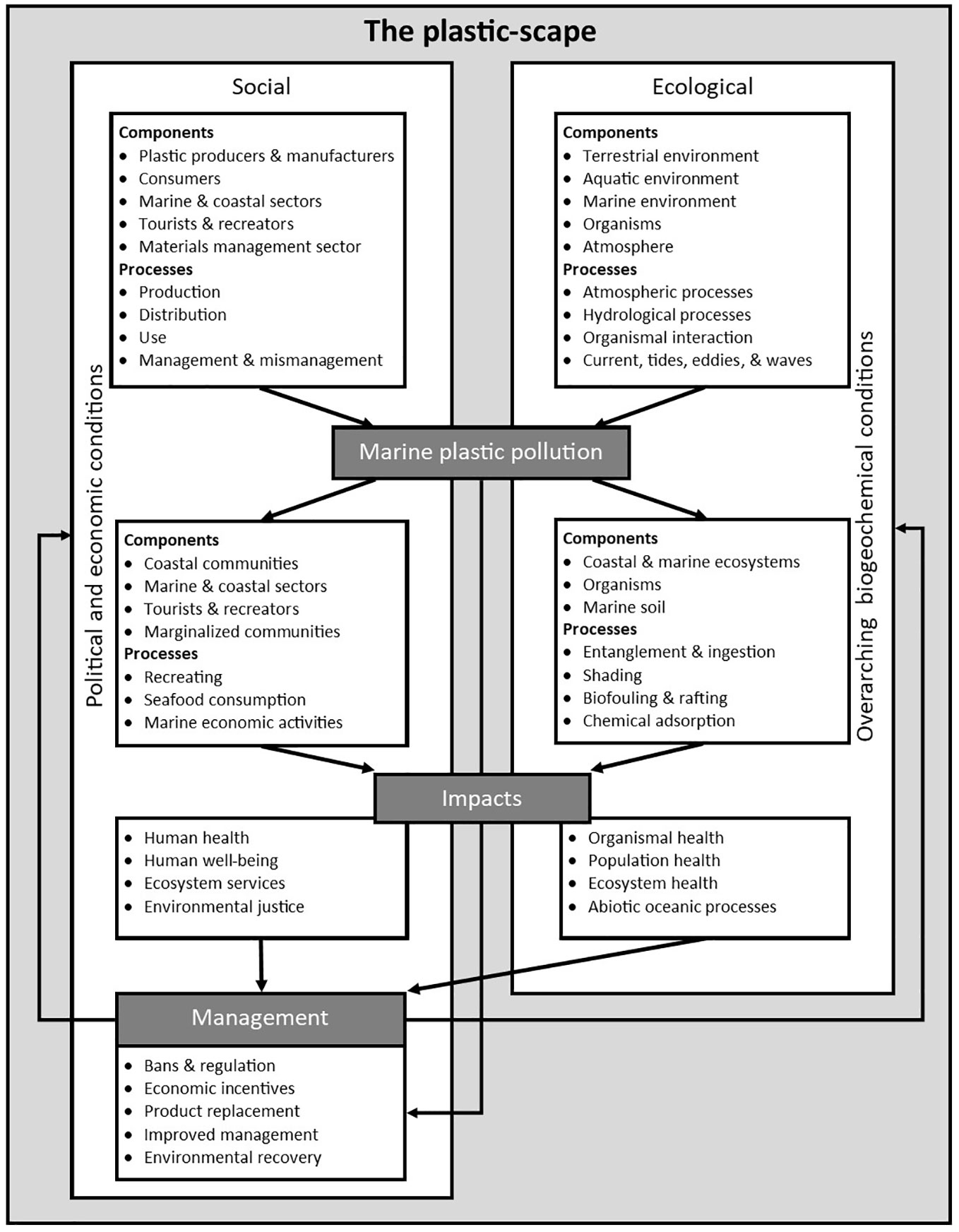
Figure 1 A conceptual model of the plastic-scape. The first set of social and ecological components and processes drive the creation and distribution of marine plastic pollution. The second interact with marine plastic pollution to drive the social-and ecological impacts of marine plastic pollution. Finally, marine plastic pollution and its impacts drive management actions that can act along the entire plastic-scape. The social and ecological components of the plastic-scape also interact with and influence each other.
Landscape ecology (LE) provides a spatially explicit, multi-scale approach for understanding social-ecological landscapes that is well-suited for MPP research and management (Wu, 2013; Opdam et al., 2018). LE draws on natural and human ecology, geography, history, economics, and wildlife management to understand the relationship between pattern and process in the environment (Risser et al., 1984; Wu, 2013). Historically, European LE focused on human landscapes and solutions-oriented questions, while North American LE aimed to advance quantitative methods for understanding natural systems (Wu and Hobbs, 2002). The integration of these approaches provides theory, principles, methods, and tools for studying complex and spatially explicit environmental challenges (Wu, 2013). Additionally, LE’s contributions to sustainability science, environmental management, and conservation demonstrate its value in achieving conservation outcomes (Wu, 2006; Opdam et al., 2018).
More recently, seascape ecology (SE) has emerged (Pittman, 2018). Like LE, it is well-suited to support sustainability science and has informed several marine conservation issues (e.g., habitat restoration, marine planning), but its application to MPP has been limited (Fraschetti et al., 2009; Stamoulis and Friedlander, 2013; Rees et al., 2018).
SE offers a multi-scale approach for understanding and evaluating the plastic-scape (Cumming et al., 2017; Opdam et al., 2018). Below, we explore opportunities for applying SE to MPP research and management.
2 The seascape ecology approach
A seascape ecology approach can help address the shortcomings of the current approach by providing a framework that 1) is spatially explicit, to account for context of implementation, 2) is holistic and multi-scale, to ensure that the sum of individual interventions is enough to address this global challenge, and 3) integrates social and ecological outcomes.
The maturation of SE has promoted the emergence of seascape specific principles, tools, and methods to capture the dynamic and three-dimensional structure of the seascape, which is necessary for understanding MPP (Wedding et al., 2011; Kavanaugh et al., 2016; Lepczyk et al., 2021; Swanborn et al., 2022). It has also sparked interest in novel research priorities—seascape connectivity; seascape goods and services; ecosystem-based management; and applications for marine management (Pittman et al., 2021). This has driven novel approaches for evaluating these seascape components, which are important aspects of the plastic-scape that have been difficult to quantify (Grober-Dunsmore et al., 2009; Halpern et al., 2010; Barbier and Lee, 2014; Urlich et al., 2022).
Landscape sustainability science, another emerging subdiscipline, aims to understand how landscape structure and elements influence the sustainability of real-world landscapes, including biodiversity, ecological processes, ecosystem services, and human wellbeing (Wu, 2021). To center human dimensions of the landscape, the landscape sustainability science framework captures a broader set of landscape pattern drivers than traditional LE—socioeconomic, political, technological, natural, and cultural—all of which are important in the plastic-scape (Bürgi et al., 2005). Further, landscape sustainability science is inherently transdisciplinary and applied. Therefore, approaches from this field can be used to inform transdisciplinary research and management approaches for the plastic-scape (Wu, 2021).
Below, we describe the ways SE principles can inform our understanding of the plastic-scape, describe applicable methods and tools for evaluating the plastic-scape, and discuss how LE and SE transdisciplinary research approaches can improve research and management.
2.1 Concepts from seascape ecology
2.1.1 Heterogeneity and pattern-process relationships
Heterogeneity is the spatial variation—or patterns—in a seascape, represented as patches or gradients (Wu, 2012; Pittman, 2018). Composition relates to the number and proportion of patch types, while configuration relates to their spatial arrangement (Gustafson, 1998).
The plastic-scape is heterogenous in both its social and ecological dimensions. Patterns in MPP configuration exist, such as gradients throughout the water column and high-density patches in the gyres and coastal zones (Eriksen et al., 2014; Hardesty et al., 2017; Brignac et al., 2019). These patterns are well-represented in the MPP literature; however, the social-ecological components of the plastic-scape also have patterns, making the impacts of MPP on biodiversity, human health, marine ecosystem services, and human well-being heterogenous (Barbier and Lee, 2014; Bucci et al., 2020; Phelan et al., 2020). Heterogeneity in these other dimensions must also be considered to effectively address MPP and its consequences more broadly.
Processes are dynamic features that create and are influenced by seascape patterns (Turner, 1989; Boström et al., 2011; Fu et al., 2011). Seascape connectivity—the movement of living and non-living material from one location to another—is an important component of these pattern-process relationships (Hyndes et al., 2014; Olds et al., 2016; Olds et al., 2018). Most MPP is derived from land-based sources, which makes understanding land-sea connectivity and connectivity between human-dominated and natural ecosystems critical (Napper and Thompson, 2020).
Processes influencing the plastic-scape include all five drivers from landscape sustainability science—socioeconomic, political, technological, natural, and cultural (Bürgi et al., 2005). Socioeconomic, technological, cultural, and political processes affect the patterns of plastic production, use, management, and mismanagement in our environment, ultimately shaping the pathways of plastic leakage (Napper and Thompson, 2020; Thushari and Senevirathna, 2020). They also influence patterns of plastic type, shape, and chemical composition in the ocean (Napper and Thompson, 2020; Thushari and Senevirathna, 2020). The human processes driving patterns in the plastic-scape are influenced by the overarching geopolitical and socio-economic context, such as patterns of human population density, wealth, and governance (Jambeck et al., 2015; Borrelle et al., 2020).
Natural processes also drive patterns in the plastic-scape. Ecological processes (e.g., rainfall, animal movements) influence patterns of plastic leakage from management sites, such as landfills (Axelsson and van Sebille, 2017; Ballejo et al., 2021). Once in the environment, hydrological processes are one of the primary pathways for transporting terrestrial plastic pollution to the ocean, making watershed patterns important for informing patterns of MPP (Lebreton et al., 2017; Windsor et al., 2019; Correa-Araneda et al., 2022).
Oceanographic processes—currents, tides, and eddies—are the primary processes driving MP transportation and deposition in the ocean (Eriksen et al., 2014; Brignac et al., 2019). Interactions with animals (e.g., ingestion), plants (e.g., entanglement), bacteria (e.g., biofouling), and human activities (e.g., clean-ups) also contribute (Ocean Conservancy, 2016; Kaiser et al., 2017; Jacquin et al., 2019; Ryan, 2020; Sanchez-Vidal et al., 2021). Understanding the relationship between these processes and patterns, and which are most important across contexts, is critical for effective management.
Beyond exploring processes that drive MPP patterns, the plastic-scape must also integrate the pattern-process relationships of MPP impacts on human and natural communities. Considering patterns within the human and natural components of the plastic-scape can provide insight into the processes that drive patterns of impacts. For instance, overlaying patterns of MPP and human use of seascapes (e.g., tourist beaches or fishing areas), may inform patterns of high MPP impact (Mouat et al., 2010; Leggett et al., 2018; Beaumont et al., 2019). Currently, this is a significant gap in MPP research, which would benefit from place-based, seascape ecology approaches. Ultimately, as the impacts of MPP drive action, these pattern-process relationships should be centered in management approaches.
2.1.2 Scale and hierarchy organization
Scale is the grain (finest resolution) and extent (total area) of a seascape. As scale changes, dominant processes and patterns change (Wu, 2012). To fully understand the plastic-scape, processes and patterns must be studied across spatial and temporal scales, and the correct scale for analysis will depend on the patterns or processes of interest (Figure 2).
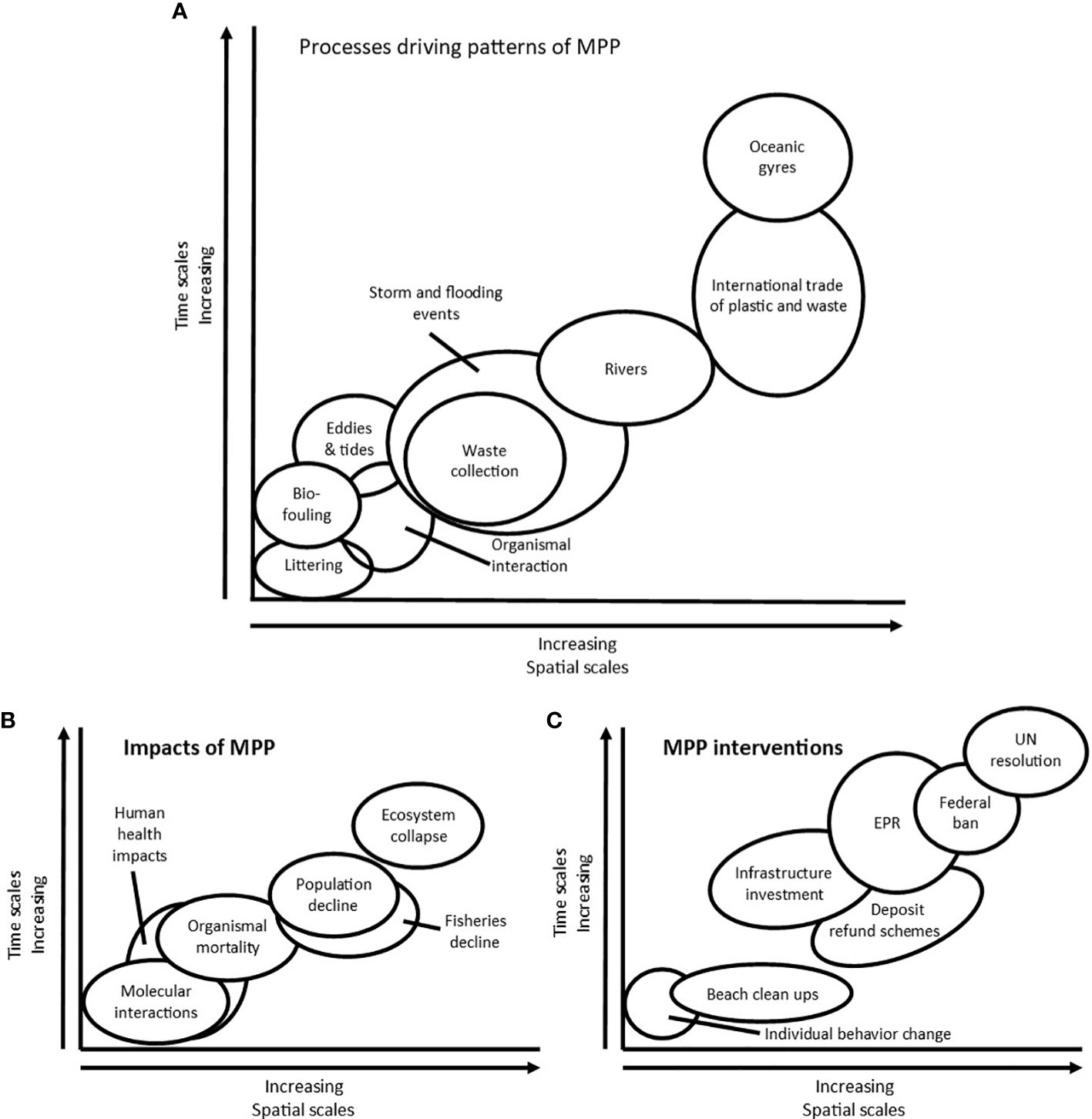
Figure 2 Time-space diagrams showcasing the multi-scale nature of the plastic-scape. (A) Provides examples of anthropogenic and natural processes that drive the spatial arrangement of marine plastic pollution. (B) Provides examples of the social-ecological impacts of plastic pollution. (C) Provides examples of marine plastic pollution management strategies.
At the global scale, particular nations have been identified as MPP sources, but at finer scales different leakage patterns emerge, such as high MPP densities near urban centers, rivers, and landfills (Eriksen et al., 2014; Huang et al., 2020). The dominant processes driving national leakage patterns are wealth, governance, and socio-economic status, while infrastructure, municipal management practices, and local hydrology are more important locally (Jambeck et al., 2015; Lebreton et al., 2017; Thushari and Senevirathna, 2020). Spatial and temporal scales are often linked, with change occurring faster at finer scales (Westley et al., 2002). Current-driven accumulation of MPP in oceanographic gyres is a global pattern-process relationship occurring on the time scale of years to decades, while finer scale patterns are driven by smaller and faster oceanographic processes—wave action, eddies, or tides (Eriksen et al., 2014; Brignac et al., 2019).
Hierarchy theory assumes systems can be divided into nested levels, where patterns and processes occurring across scales are part of a single system with cross-scale effects (Kavanaugh et al., 2016; Allen and Starr, 2017).
Patterns and processes that emerge at different temporal and spatial scales of the plastic-scape influence each other. For example, global oceanographic processes are the dominant processes driving patterns of MPP associated with the gyres. However, these currents also contribute to local heterogeneity, such as the variation in MPP density between windward and leeward coasts (Brignac et al., 2019). Another cross-scale impact is the influence of national governance and socio-economic status on local plastic waste management strategies. National governance and wealth influence the resources, technology, and funding available to implement local waste management, ultimately changing local leakage rates (Helm et al., 2022).
Hierarchy theory can also be implemented to understand management across scales. Policies introduced at one scale of governance will influence others. For example, China’s National Sword Policy, which regulates the import of recyclables, affected U.S. municipalities by decreasing the demand for plastic waste, ultimately driving local action (Murphy et al., 2020; Vedantam et al., 2022). Therefore, hierarchy theory provides an approach for understanding the plastic-scape as a whole and understanding the influence of interventions across levels of governance.
2.2 Methods and tools
SE provides tools, metrics, and methods that can be applied to the plastic-scape (Wedding et al., 2011; Costa et al., 2018). Additionally, it provides an ecological framework, technical skills, and best practices for applying them (Grober-Dunsmore et al., 2009; Lepczyk et al., 2021; Cumming et al., 2022).
Seascape ecologists employ a breadth of imaging tools—satellites and aerial photography, drones, boat-based sensors (e.g., LIDAR), autonomous vehicles, underwater imaging, benthic mapping, and semi-automated image classification—that can be used to map and monitor plastics (Costa et al., 2018; D’Urban Jackson et al., 2020). However, their limited use has focused on characterizing MPP transport and deposition (Lebreton et al., 2017; Salgado-Hernanz et al., 2021). MPP researchers have already called for the broader application of these methods, in the form of the integrated marine debris observing system, to develop global MPP maps for long-term monitoring and management (Maximenko et al., 2019).
SE also provides metrics to quantify characteristics of the plastic-scape. Spatial pattern metrics are applied to maps to quantify, characterize, and interpret patterns and pattern-process relationships (Boström et al., 2011; Wedding et al., 2011; Pittman et al., 2021). These metrics can be applied to the plastic-scape to quantify and interpret the distribution of MPP, the configuration of its social-ecological consequences, and the effects of management on these patterns.
Finally, SE provides modelling approaches. Network models, predictive spatial models, neutral seascape models and dynamic models have been applied to better understand marine conservation issues, characterize complex connectivity patterns at management appropriate scales, and simulate management outcomes under various scenarios (Pittman et al., 2007; Engelhard et al., 2017; Costa et al., 2018; Stamoulis et al., 2018; Treml and Kool, 2018; Wedding et al., 2019). We have seen the value of modeling MPP to understand patterns of MPP leakage (Lebreton et al., 2017; Borrelle et al., 2020). The application of SE models will improve the evaluation of interventions, provide spatially explicit outputs, and allow for multi-scale models.
2.3 Transdisciplinary research for management
SE transdisciplinary approaches can inform more effective MPP research and management (Pittman et al., 2021; Wu, 2021). First, research agendas should be co-produced. In SE, practitioners are being included in discussions about future research agendas, with their priorities deemed equally important to academics (Pittman et al., 2021). Though differences between these two groups remain, areas of agreement provide clear opportunities for collaboration (Cvitanovic et al., 2016; Dey et al., 2020). Setting a co-produced research agenda presents an opportunity for aligning the goals of the diverse group of stakeholders addressing MPP.
SE also provides methods for transdisciplinary research, including management specific metrics, predictive models to inform decision making, monitoring approaches, and tools to evaluate management outcomes (Nassauer and Opdam, 2008; Pressey and Bottrill, 2009; Olds et al., 2016; Pittman, 2018). The benefits of these approaches are exemplified by their rapid adoption in biodiversity conservation, restoration, and sustainable development (Choi et al., 2008; Opdam et al., 2018; Balbar and Metaxas, 2019).
3 Future research
Generally, an SE approach should be applied to answer spatially explicit, place-based questions about patterns in the plastic-scape, and the processes that drive them, with a focus on informing management. Since MPP is primarily land-based, characterizing connectivity between terrestrial and marine systems is critical. Hydrological models have already been applied to identify MPP leakage patterns and particular rivers as management priorities (Lebreton et al., 2017; Windsor et al., 2019; Correa-Araneda et al., 2022). Future research could explore different scales and processes to identify other contributors to leakage patterns.
Researchers should also explore how seascape configuration influences MPP pathways and patterns. For example, certain habitats act as plastic sinks (Martin et al., 2020; Sanchez-Vidal et al., 2021). Research on the relationship between seascape configuration and MPP deposition can be used to predict MPP patterns and inform management priorities.
Future work could also employ social sensing—the characterization of human components of the plastic-scape (Liu et al., 2015). Integration of human activity and social data into MPP maps and models could provide more insight into anthropogenic pathways of MPP leakage and the efficacy of different management efforts.
Finally, research to inform and evaluate management should be prioritized. For example, researchers can employ predictive spatial models to compare outcomes associated with various intervention strategies and inform a multi-scale management plan that integrates action across levels of governance. SE approaches could also provide baselines, allowing researchers to better monitor changes in plastic-scape patterns to evaluate management efficacy (Maximenko et al., 2019).
4 Limitations
Using the tools of SE, researchers can better understand the plastic-scape; however, this approach has limitations. The primary limitation is technological. To date, remote sensing has only been used to quantify surficial MPP (Goddijn-Murphy and Williamson, 2019). Additionally, satellite data typically has a resolution of >1 meter, which is too coarse to detect most MPP. Though alternatives exist, they can be expensive (e.g., aerial imaging and high spectral sensors), inconsistent (e.g., thermal infrared sensing), or range limited (e.g., drones) (Goddijn-Murphy and Williamson, 2019; Salgado-Hernanz et al., 2021). However, as technology improves and data collection becomes easier, the value of employing an SE approach will continue to increase.
Second, land-based pollution is not a research priority in SE (Pittman et al., 2021). Further, plastic pollution is a non-point source pollutant with a complex life cycle largely driven by human activity (Napper and Thompson, 2020). Identifying the appropriate scope and scale of analyses and actions may prove challenging. MPP also represents a breadth of pollutants that have different patterns, processes, and social-ecological consequences as they degrade, making MPP less predictable than other pollutants (Eriksen et al., 2014; Luo et al., 2022).
Finally, more research is needed on integrating human dimensions (e.g., ecosystem services) into SE models (Barbier and Lee, 2014; Pittman et al., 2021). Still, LE and SE continuously adapt to better address applied research questions. Therefore, as SE is further applied to MPP research and management, many of these limitations could be addressed.
5 Conclusion
The plastic-scape includes all the human (i.e., governance systems and actors) and ecological components (i.e., abiotic, and biotic processes) of the system that contribute to patterns of plastic production, use, and pollution, as well as the interactions between MPP and human and natural communities that drive its social and ecological consequences. Failures to effectively mitigate MPP and its consequences are exacerbated by the complexity of this system and the ad hoc, reactive nature of many management efforts. SE provides a novel approach for researching the plastic-scape informing effective management.
Data availability statement
The original contributions presented in the study are included in the article/supplementary material. Further inquiries can be directed to the corresponding author.
Author contributions
EM: Conceptualization, led writing. BP: writing and editing. LG: writing and editing. All authors contributed to the article and approved the submitted version.
Funding
The publication of this article was funded by the Arizona State University Graduate and Professional Student Association's Publication Research Grant.
Conflict of interest
The authors declare that the research was conducted in the absence of any commercial or financial relationships that could be construed as a potential conflict of interest. The publication of this article was funded by the Arizona State University Graduate and Professional Student Association's Publication Research Grant.
Publisher’s note
All claims expressed in this article are solely those of the authors and do not necessarily represent those of their affiliated organizations, or those of the publisher, the editors and the reviewers. Any product that may be evaluated in this article, or claim that may be made by its manufacturer, is not guaranteed or endorsed by the publisher.
References
Allen T. F. H., Starr T. B. (2017). Hierarchy: Perspectives for ecological complexity (Chicago: University of Chicago Press). doi: 10.7208/9780226489711
Armoškaitė A., Puriņa I., Aigars J., Strāķe S., Pakalniete K., Frederiksen P., et al. (2020). Establishing the links between marine ecosystem components, functions and services: An ecosystem service assessment tool. Ocean. Coast. Manag. 193, 105229. doi: 10.1016/j.ocecoaman.2020.105229
Axelsson C., van Sebille E. (2017). Prevention through policy: Urban macroplastic leakages to the marine environment during extreme rainfall events. Mar. Poll. Bull. 124 (1), 211–227. doi: 10.1016/j.marpolbul.2017.07.024
Balbar A. C., Metaxas A. (2019). The current application of ecological connectivity in the design of marine protected areas. Glob. Ecol. Conserv. 17, e00569. doi: 10.1016/j.gecco.2019.e00569
Ballejo F., Plaza P., Speziale K. L., Lambertucci A. P., Lambertucci S. A. (2021). Plastic ingestion and dispersion by vultures may produce plastic islands in natural areas. Sci. Tot. Envir. 755, 142421. doi: 10.1016/j.scitotenv.2020.142421
Barbier E. B., Lee K. D. (2014). Economics of the marine seascape. Int. Rev. Envir. Res. Econ. 7 (1), 35–65. doi: 10.1561/101.00000056
Beaumont N. J., Aanesen M., Austen M. C., Börger T., Clark J. R., Cole M., et al. (2019). Global ecological, social and economic impacts of marine plastic. Mar. Poll. Bull. 142, 189–195. doi: 10.1016/j.marpolbul.2019.03.022
Borrelle S. B., Ringma J., Law K. L., Monnahan C. C., Lebreton L., McGivern A., et al. (2020). Global plastic waste generation exceeds efforts to mitigate plastic pollution. Sci. 369 (6510), 1515–1518. doi: 10.1126/science.aba3656
Boström C., Pittman S. J., Simenstad C., Kneib R. T. (2011). Seascape ecology of coastal biogenic habitats: Advances, gaps, and challenges. Mar. Eco. Pro. Ser. 427, 191–217. doi: 10.3354/meps09051
Brignac K. C., Jung M. R., King C., Royer S. J., Blickley L., Lamson M. R., et al. (2019). Marine debris polymers on main Hawaiian island beaches, sea surface, and seafloor. Enviro. Sci. Tech. 53 (21), 12218–12226. doi: 10.1021/acs.est.9b03561
Bucci K., Tulio M., Rochman C. M. (2020). What is known and unknown about the effects of plastic pollution: A meta-analysis and systematic review. Ecol. App. 30 (2), e02044. doi: 10.1002/eap.2044
Bürgi M., Hersperger A. M., Schneeberger N. (2005). Driving forces of landscape change - current and new directions. Land. Ecol. 19, 857–868. doi: 10.1007/s10980-005-0245-3
Choi Y. D., Temperton V. M., Allen E. B., Grootjans A. P., Halassy M., Hobbs R. J., et al. (2008). Ecological restoration for future sustainability in a changing environment. Ecosci. 15 (1), 53–64. doi: 10.2980/1195-6860(2008)15[53:ERFFSI]2.0.CO;2
Correa-Araneda F., Pérez J., Tonin A. M., Esse C., Boyero L., Díaz M. E., et al. (2022). Microplastic concentration, distribution and dynamics along one of the largest Mediterranean-climate rivers: A whole watershed approach. Enviro. Res. 209, 112808. doi: 10.1016/j.envres.2022.112808
Costa B., Walker B. K., Dijkstra J. A. (2018). “Mapping and quantifying seascape patterns,” in Seascape ecology. Ed. Pittman S. (Hoboken, NJ: John Wiley & Sons), 27–56.
Cumming G. S., Morrison T. H., Hughes T. P. (2017). New directions for understanding the spatial resilience of social–ecological systems. Eco. 20, 649–664. doi: 10.1007/s10021-016-0089-5
Cumming G. S., Magris R. A., Maciejewski K. (2022). Quantifying cross-scale patch contributions to spatial connectivity. Land. Eco. 37(9), 2255–2272. doi: 10.1007/s10980-022-01497-7
Cvitanovic C., McDonald J., Hobday A. J. (2016). From science to action: principles for undertaking environmental research that enables knowledge exchange and evidence-based decision-making. J. Environ. Manage. 183, 864–874. doi: 10.1016/j.jenvman.2016.09.038
Dey C. J., Rego A. I., Midwood J. D., Koops M. A. (2020). A review and meta-analysis of collaborative research prioritization studies in ecology, biodiversity conservation and environmental science. Proc. R. Soc Bio. 287, 20200012. doi: 10.1098/rspb.2020.0012
D’Urban Jackson T., Williams G. J., Walker-Springett G., Davies A. J. (2020). Three-dimensional digital mapping of ecosystems: A new era in spatial ecology. Pro. R. Soc Bio. 287 (1920), 20192383. doi: 10.1098/rspb.2019.2383
Engelhard S. L., Huijbers C. M., Stewart-Koster B., Olds A. D., Schlacher T. A., Connolly R. M. (2017). Prioritising seascape connectivity in conservation using network analysis. J. App. Ecol. 54 (4), 1130–1141. doi: 10.1111/1365-2664.12824
Eriksen M., Lebreton L. C., Carson H. S., Thiel M., Moore C. J., Borerro J. C., et al. (2014). Plastic pollution in the world’s oceans: More than 5 trillion plastic pieces weighing over 250,000 tons afloat at sea. PloS One 9 (12), e111913. doi: 10.1371/journal.pone.0111913
Excell C., Salcedo-La Viña C., Worker J., Moses E. (2018). Legal limits on single-use plastics and microplastics: A global review of national laws and regulations (Nairobi, Kenya: UNEP and WRI).
Fraschetti S., D’Ambrosio P., Micheli F., Pizzolante F., Bussotti S., Terlizzi A. (2009). Design of marine protected areas in a human-dominated seascape. Mar. Ecol. Prog. Ser. 375, 13–24. doi: 10.3354/meps07781
Fu B., Liang D., Lu N. (2011). Landscape ecology: Coupling of pattern, process, and scale. Chin. Geo. Sci. 21 (4), 385–391. doi: 10.1007/s11769-011-0480-2
Goddijn-Murphy L., Williamson B. (2019). On thermal infrared remote sensing of plastic pollution in natural waters. Rem. Sens. 11 (18), 2159. doi: 10.3390/rs11182159
Grober-Dunsmore R., Pittman S. J., Caldow C., Kendall M. S., Frazer T. K. (2009). “A landscape ecology approach for the study of ecological connectivity across tropical marine seascapes,” in Ecological connectivity among tropical coastal ecosystems (Dordrecht: Springer), 493–530. doi: 10.1007/978-90-481-2406-0_14
Gustafson E. J. (1998). Quantifying landscape spatial pattern: What is the state of the art? Eco. 1 (2), 143–156. doi: 10.1007/s100219900011
Halpern B. S., Lester S. E., McLeod K. L. (2010). Placing marine protected areas onto the ecosystem-based management seascape. Proc. Nat. Acad. Sci. 107 (43), 18312–18317. doi: 10.1073/pnas.0908503107
Hardesty B. D., Harari J., Isobe A., Lebreton L., Maximenko N., Potemra J., et al. (2017). Using numerical model simulations to improve the understanding of micro-plastic distribution and pathways in the marine environment. Fron. Mar. Sci. 4. doi: 10.3389/fmars.2017.00030
Helm L. T., Murphy E. L., McGivern A., Borrelle S. B. (2022). Impacts of plastic waste management strategies. Enviro. Rev., (ja). doi: 10.1139/er-2021-0117
Huang Y., Tian M., Jin F., Chen M., Liu Z., He S., et al. (2020). Coupled effects of urbanization level and dam on microplastics in surface waters in a coastal watershed of southeast China. Mar. Poll. Bull. 154, 111089. doi: 10.1016/j.marpolbul.2020.111089
Hyndes G. A., Nagelkerken I., McLeod R. J., Connolly R. M., Lavery P. S., Vanderklift M. A. (2014). Mechanisms and ecological role of carbon transfer within coastal seascapes. Bio. Rev. 89 (1), 232–254. doi: 10.1111/brv.12055
Jacquin J., Cheng J., Odobel C., Pandin C., Conan P., Pujo-Pay M., et al. (2019). Microbial ecotoxicology of marine plastic debris: A review on colonization and biodegradation by the “Plastisphere”. Front. Micro. 10. doi: 10.3389/fmicb.2019.00865
Jambeck J. R., Geyer R., Wilcox C., Siegler T. R., Perryman M., Andrady A., et al. (2015). Plastic waste inputs from land into the ocean. Sci. 347 (6223), 768–771. doi: 10.1126/science.1260352
Kaiser D., Kowalski N., Waniek J. J. (2017). Effects of biofouling on the sinking behavior of microplastics. Env. Res. Lett. 12 (12), 124003. doi: 10.1088/1748-9326/aa8e8b
Kavanaugh M. T., Oliver M. J., Chavez F. P., Letelier R. M., Muller-Karger F. E., Doney S. C. (2016). Seascapes as a new vernacular for pelagic ocean monitoring, management and conservation. ICES J. Mar. Sci. 73 (7), 1839–1850. doi: 10.1093/icesjms/fsw086
Lavee D. (2010). A cost-benefit analysis of a deposit-refund program for beverage containers in Israel. Waste. Manag. 30(2), 338–345. doi: 10.1016/j.wasman.2009.09.026
Lebreton L., van der Zwet J., Damsteeg J. W., Slat B., Andrady A., Reisser J. (2017). River plastic emissions to the world’s oceans. Nat. Commun. 8 (1), 1–10. doi: 10.1038/ncomms15611
Leggett C. G., Scherer N., Haab T. C., Bailey R., Landrum J. P., Domanski A. (2018). Assessing the economic benefits of reductions in marine debris at southern California beaches: a random utility travel cost model. Mar. Res. Econ. 33 (2), 133–153. doi: 10.1086/697152
Lepczyk C. A., Wedding L. M., Asner G. P., Pittman S. J., Goulden T., Linderman M. A., et al. (2021). Advancing landscape and seascape ecology from a 2D to a 3D science. BioS. 71 (6), 596–608. doi: 10.1093/biosci/biab001
Liu J., Mooney H., Hull V., Davis S. J., Gaskell J., Hertel T., et al. (2015). Systems integration for global sustainability. Sci. 347 (6225), 1258832. doi: 10.1126/science.1258832
Luo H., Liu C., He D., Xu J., Sun J., Li J., et al. (2022). Environmental behaviors of microplastics in aquatic systems: A systematic review on degradation, adsorption, toxicity and biofilm under aging conditions. J. Haz. Mat. 423, 126915. doi: 10.1016/j.jhazmat.2021.126915
Martin C., Baalkhuyur F., Valluzzi L., Saderne V., Cusack M., Almahasheer H., et al. (2020). Exponential increase of plastic burial in mangrove sediments as a major plastic sink. Sci. Adv. 6 (44), eaaz5593. doi: 10.1126/sciadv.aaz5593
Maximenko N., Corradi P., Law K. L., Van Sebille E., Garaba S. P., Lampitt R. S., et al. (2019). Toward the integrated marine debris observing system. Front. Mar. Sci. 6. doi: 10.3389/fmars.2019.00447
McIlgorm A., Campbell H. F., Rule M. J. (2011). The economic cost and control of marine debris damage in the Asia-Pacific region. Ocean Coast. Manag. 54(9), 643–651. doi: 10.1016/j.ocecoaman.2011.05.007
Mouat J., Lozano R. L., Bateson H. (2010) Economic impacts of marine litter. KIMO international. Available at: https://www.kimointernational.org/wp/wp-content/uploads/2017/09/KIMO_Economic-Impacts-of-Marine-Litter.pdf.
Murphy E. L., Bernard M. L., Helm L., Hill I., Tuñas-Corzón Á. (2020). Policy recommendations to reinvigorate recycling in Arizona. J. Sci. Pol. Gov. 17. doi: 10.38126/JSPG170115
Murphy E. L., Bernard M., Iacona G., Borrelle S. B., Barnes M., McGivern A., et al. (2021). A decision framework for estimating the cost of marine plastic pollution interventions. Con. Bio. 36 (2), e13827. doi: 10.1111/cobi.13827
Murphy E. L., Fredette-Roman C., Rochman C., Gerber L. R., Polidoro B. (in review). Assessing the relative vulnerability of marine biodiversity to macroplastics. Front. Eco. Enviro.
Napper I. E., Thompson R. C. (2020). Plastic debris in the marine environment: history and future challenges. Glob. Chall. 4 (6), 1900081. doi: 10.1002/gch2.201900081
Nassauer J., Opdam P. (2008). Design in science: Extending the landscape ecology paradigm. Land. Ecol. 23, 633–644. doi: 10.1007/s10980-008-9226-7
Ocean Conservancy (2016) 30th anniversary international coastal cleanup. Available at: https://oceanconservancy.org/wp-content/uploads/2017/04/2016-Ocean-Conservancy-ICC-Report.pdf.
Olds A. D., Connolly R. M., Pitt K. A., Pittman S. J., Maxwell P. S., Huijbers C. M., et al. (2016). Quantifying the conservation value of seascape connectivity: A global synthesis. Glob. Ecol. Biog. 25 (1), 3–15. doi: 10.1111/geb.12388
Olds A. D., Nagelkerken I., Huijbers C. M., Gilby B. L., Pittman S. J., Schlacher T. A. (2018). “Connectivity in coastal seascapes,” in Seascape ecology. Ed. Pittman S. (Hoboken, NJ: John Wiley & Sons), 261–292.
Oosterhuis F., Papyrakis E., Boteler B. (2014). Economic instruments and marine litter control. Ocean .Coast. Manag. 102, 47–54. doi: 10.1016/j.ocecoaman.2014.08.005
Opdam P., Luque S., Nassauer J., Verburg P. H., Wu J. (2018). How can landscape ecology contribute to sustainability science? Land. Ecol. 33 (1), 1–7. doi: 10.1007/s10980-018-0610-7
Phelan A., Ross H., Setianto N. A., Fielding K., Pradipta L. (2020). Ocean plastic crisis–mental models of plastic pollution from remote Indonesian coastal communities. PloS One 15 (7), e0236149. doi: 10.1371/journal.pone.0236149
Pittman S. J., Christensen J. D., Caldow C., Menza C., Monaco M. E. (2007). Predictive mapping of fish species richness across shallow-water seascapes in the Caribbean. Ecol. Mod. 204 (1-2), 9–21. doi: 10.1016/j.ecolmodel.2006.12.017
Pittman S. J., Yates K. L., Bouchet P. J., Alvarez-Berastegui D., Andréfouët S., Bell S. S., et al. (2021). Seascape ecology: identifying research priorities for an emerging ocean sustainability science. Mar. Ecol. Pro. Ser. 663, 1–29. doi: 10.3354/meps13661
Pressey R. L., Bottrill M. C. (2009). Approaches to landscape-and seascape-scale conservation planning: convergence, contrasts and challenges. Oryx 43 (4), 464–475. doi: 10.1017/S0030605309990500
Raubenheimer K., Urho N. (2020). Possible elements of a new global agreement to prevent plastic pollution (Copenhagen, Denmark: Nordic Council of Ministers).
Rees M. J., Knott N. A., Davis A. R. (2018). Habitat and seascape patterns drive spatial variability in temperate fish assemblages: Implications for marine protected areas. Mar. Ecol. Pro. Ser. 607, 171–186. doi: 10.3354/meps12790
Risser P. G., Karr J. R., Forman R. T. T. (1984) Landscape ecology: directions and approaches. Available at: https://www.ideals.illinois.edu/handle/2142/111627.
Ryan P. G. (2020). Using photographs to record plastic in seabird nests. Mar. Poll. Bull. 156, 111262. doi: 10.1016/j.marpolbul.2020.111262
Salgado-Hernanz P. M., Bauzà J., Alomar C., Compa M., Romero L., Deudero S. (2021). Assessment of marine litter through remote sensing: recent approaches and future goals. Mar. Poll. Bull. 168, 112347. doi: 10.1016/j.marpolbul.2021.112347
Sanchez-Vidal A., Canals M., de Haan W. P., Romero J., Veny M. (2021). Seagrasses provide a novel ecosystem service by trapping marine plastics. Sci. Rep. 11 (1), 1–7. doi: 10.1038/s41598-020-79370-3
Schnurr R. E., Alboiu V., Chaudhary M., Corbett R. A., Quanz M. E., Sankar K., et al. (2018). Reducing marine pollution from single-use plastics (SUPs): A review. Mar. Poll. Bull. 137, 157–171. doi: 10.1016/j.marpolbul.2018.10.001
Stamoulis K. A., Delevaux J. M., Williams I. D., Poti M., Lecky J., Costa B., et al. (2018). Seascape models reveal places to focus coastal fisheries management. Ecol. App.s 28 (4), 910–925. doi: 10.1002/eap.1696
Stamoulis K. A., Friedlander A. M. (2013). A seascape approach to investigating fish spillover across a marine protected area boundary in hawai ‘i. Fish. Res. 144, 2–14. doi: 10.1016/j.fishres.2012.09.016
Swanborn D. J., Huvenne V. A., Pittman S. J., Woodall L. C. (2022). Bringing seascape ecology to the deep seabed: A review and framework for its application. Lim. Ocean. 67 (1), 66–88. doi: 10.1002/lno.11976
Tessnow-von Wysocki I., Le Billon P. (2019). Plastics at sea: Treaty design for a global solution to marine plastic pollution. Enviro. Sci. Pol. 100, 94–104. doi: 10.1016/j.envsci.2019.06.005
Thushari G. G. N., Senevirathna J. D. M. (2020). Plastic pollution in the marine environment. Heli. 6 (8), e04709. doi: 10.1016/j.heliyon.2020.e04709
Treml E. A., Kool J. (2018). “Networks for quantifying and analysing seascape connectivity,” in Seascape ecology. Ed. Pittman S. (Hoboken, NJ: John Wiley & Sons), 293–318.
Turner M. G. (1989). Landscape ecology: The effect of pattern on process. Ann. Rev. Ecol. Syst. 20 (1), 171–197. doi: 10.1146/annurev.es.20.110189.001131
Urlich S. C., White F. R., Rennie H. G. (2022). Characterising the regulatory seascape in aotearoa new Zealand: Bridging local, regional and national scales for marine ecosystem-based management. Oc. Coast. Man. 224, 106193. doi: 10.1016/j.ocecoaman.2022.106193
Vedantam A., Suresh N. C., Ajmal K., Shelly M. (2022). Impact of china’s national sword policy on the US landfill and plastics recycling industry. Sust. 14 (4), 2456. doi: 10.3390/su14042456
Wedding L. M., Jorgensen S., Lepczyk C. A., Friedlander A. M. (2019). Remote sensing of three-dimensional coral reef structure enhances predictive modeling of fish assemblages. Rem. Sens. Ecol. Cons. 5 (2), 150–159. doi: 10.1002/rse2.115
Wedding L. M., Lepczyk C. A., Pittman S. J., Friedlander A. M., Jorgensen S. (2011). Quantifying seascape structure: Extending terrestrial spatial pattern metrics to the marine realm. Mar. Ecol. Pro. Ser. 427, 219–232. doi: 10.3354/meps09119
Westley F., Carpenter S. R., Brock W. A., Holling C. S., Gunderson L. H. (2002). “Why systems of people and nature are not just social and ecological systems,” in Panarchy: Understanding transformations in human and natural systems. Eds. Gunderson L. H., Holling C. S. (Washington, DC: Island Press), 103–120.
Windsor F. M., Durance I., Horton A. A., Thompson R. C., Tyler C. R., Ormerod S. J. (2019). A catchment-scale perspective of plastic pollution. Glob. Cha. Bio. 25 (4), 1207–1221. doi: 10.1111/gcb.14572
Wu J. J. (2006). Landscape ecology, cross-disciplinarity, and sustainability science. Land. Ecol. 21 (1), 1–4. doi: 10.1007/s10980-006-7195-2
Wu J. (2012). “Landscape ecology,” in Encyclopedia of theoretical ecology. Eds. Hastings A., Gross L. (Berkeley, CA: University of California Press), 392–396.
Wu J. (2013). Key concepts and research topics in landscape ecology revisited: 30 years after the allerton park workshop. Land. Ecol. 28 (1), 1–11. doi: 10.1007/s10980-012-9836-y
Wu J. (2021). Landscape sustainability science (II): Core questions and key approaches. Land. Ecol. 36 (8), 2453–2485. doi: 10.1007/s10980-021-01245-3
Wu J., Hobbs R. (2002). Key issues and research priorities in landscape ecology: an idiosyncratic synthesis. Land. Ecol. 17 (4), 355–365. doi: 10.1023/A:1020561630963
Keywords: landscape ecology, plastic, pollution, policy, mitigation, marine, seascape ecology
Citation: Murphy EL, Polidoro B and Gerber LR (2022) The plastic-scape: Applying seascape ecology to marine plastic pollution. Front. Mar. Sci. 9:980835. doi: 10.3389/fmars.2022.980835
Received: 29 June 2022; Accepted: 03 October 2022;
Published: 03 November 2022.
Edited by:
Heng-Xiang Li, South China Sea Institute of Oceanology (CAS), ChinaReviewed by:
Prabhu Kolandhasamy, Bharathidasan University, IndiaCopyright © 2022 Murphy, Polidoro and Gerber. This is an open-access article distributed under the terms of the Creative Commons Attribution License (CC BY). The use, distribution or reproduction in other forums is permitted, provided the original author(s) and the copyright owner(s) are credited and that the original publication in this journal is cited, in accordance with accepted academic practice. No use, distribution or reproduction is permitted which does not comply with these terms.
*Correspondence: Erin L. Murphy, ZWxtdXJwaDFAYXN1LmVkdQ==