- 1Nicholas School of the Environment, Duke University, Durham, United States
- 2Laboratoire Environnement Ressources Bretagne Occidentale (LER/BO), Ifremer, Concarneau, France
- 3Department of Medicine, Duke University Medical Center, Durham, United States
- 4Department of Orthopaedics, Duke University Medical Center, Durham, United States
The global accumulation of plastic waste has reached crisis levels. The diverse and multilayered impacts of plastic on biological health prompts an evaluation of these effects from a One Health perspective, through which the complexity of these processes can be integrated and more clearly understood. Plastic particles ranging from nanometers to meters in size are found throughout every ecosystem on Earth, from the deepest marine trenches to the highest mountains. Plastic waste affects all layers of biological organization, from the molecular and cellular to the organismal, community, and ecosystem-levels. These effects are not only mediated by the physical properties of plastics, but also by the chemical properties of the plastic polymers, the thousands of additives combined with plastics during manufacturing, and the sorbed chemicals and microbes that are transported by the plastic waste. Using a One Health framework we provide an overview of the following themes: 1) ways in which plastic impacts global health across levels of biological organization, 2) how the effects of plastic interact between layers of biology, and 3) what knowledge gaps exist in understanding the effects of plastic within and between biological scales. We also propose potential solutions to address this growing crisis, with an emphasis on One Health perspectives that consider the oneness of animals, humans, and the environment.
Plastic is ubiquitous and interacts with all aspects of the biosphere
Plastics are ubiquitous in our society. Demand for plastic has skyrocketed since the 1950s due its inexpensive, strong, durable, and lightweight properties (Thompson et al., 2009). As a result, plastic pollution is now found all across the planet, including along coastlines (Kwon et al., 2014; Courtene-Jones et al., 2021), in the open ocean (Eriksen et al., 2013; Cózar et al., 2014), the deep sea (Bergmann et al., 2017; Barrett et al., 2020), soils (Fuller and Gautam, 2016), and the atmosphere (González-Pleiter et al., 2021). Current estimates suggest that a minimum of 5.25 trillion plastic particles are present in the world’s oceans, a number that is expected to grow (Eriksen et al., 2014). Indeed, the amount of plastic pollution entering the terrestrial and aquatic environment is predicted to grow by an additional 710 million metric tons between 2016 and 2040, even if immediate action is taken to reduce waste (Lau et al., 2020). These plastics are degraded by biotic and abiotic processes in the environment, such as bacterial activity, UV light, temperature, and abrasion, resulting in smaller fragments with altered surface properties. These smaller plastics, classified as microplastics (<5 mm) and nanoplastics (<1 µm), are the most prevalent type of solid waste, especially in the aquatic environment (Jambeck et al., 2015; Gigault et al., 2018). Additionally, both types of small plastics (referred to as micro- and nanoplastics) can be found in commercial and industrial items.
The ubiquity of plastics in the biosphere has made interactions with animals and humans inevitable. Vast numbers of marine species are impacted by plastics (Gall and Thompson, 2015). Microplastics are found in fish, clams, mussels, oysters, and crabs destined for human consumption (Van Cauwenberghe and Janssen, 2014; Li et al., 2015; Rochman et al., 2015; Karami et al., 2017; Su et al., 2018; Waite et al., 2018), as well as table and sea salt (Yang et al., 2015; Zarus et al., 2021), seaweed (Baini et al., 2017), honey (Liebezeit and Liebezeit, 2013; Liebezeit and Liebezeit, 2015), tea (Hernandez et al., 2019), beer (Kosuth et al., 2018), and tap and bottled water (Kosuth et al., 2018; Zuccarello et al., 2019; Kankanige and Babel, 2020). Microplastics have also been documented in the human body, (e.g., in lung tissues (Amato-Lourenço et al., 2021), stool (Schwabl et al., 2019; Ibrahim et al., 2021), blood (Leslie et al., 2022), and even placentas (Ragusa et al., 2021).
There is perhaps no other single anthropogenic contaminant that has had such a wide spectrum of direct exposure ranging across all levels of biology. Plastics disrupt homeostasis at the individual organismal level via ingestion of plastic debris (Gall and Thompson, 2015). Plastic pollution can also disrupt ecosystem functioning by changing and damaging habitats (Aloy et al., 2011; Carson et al., 2011; Richards and Beger, 2011) and altering the balance of species across ecosystems (Barnes and Milner, 2005; Goldstein et al., 2012). Such changes, in turn, inevitably have unknown effects upon health. Mitigating plastic impacts on the health of people, animals, and ecosystems requires an approach that transcends traditional species-level risk assessments. One such framework is the concept of One Health (Figure 1). One Health recognizes the interconnectedness of people, animals, and plants, and how their individual health is itself dependent on the health of their shared environment (One Health, 2021). The One Health perspective calls for a multi-sectoral, transdisciplinary, and collaborative approach to solving health issues at the local, national, and global levels (One Health, 2022). While the origins of One Health research stem from the study of zoonotic diseases, this framework provides a transdisciplinary lens to (i) examine the imminent threat to human, animal, and ecosystem health imposed by plastic pollution, (ii) elucidate socio-economic ramifications of plastic pollution and (iii) implement mitigation strategies interlining with the public and private sectors.
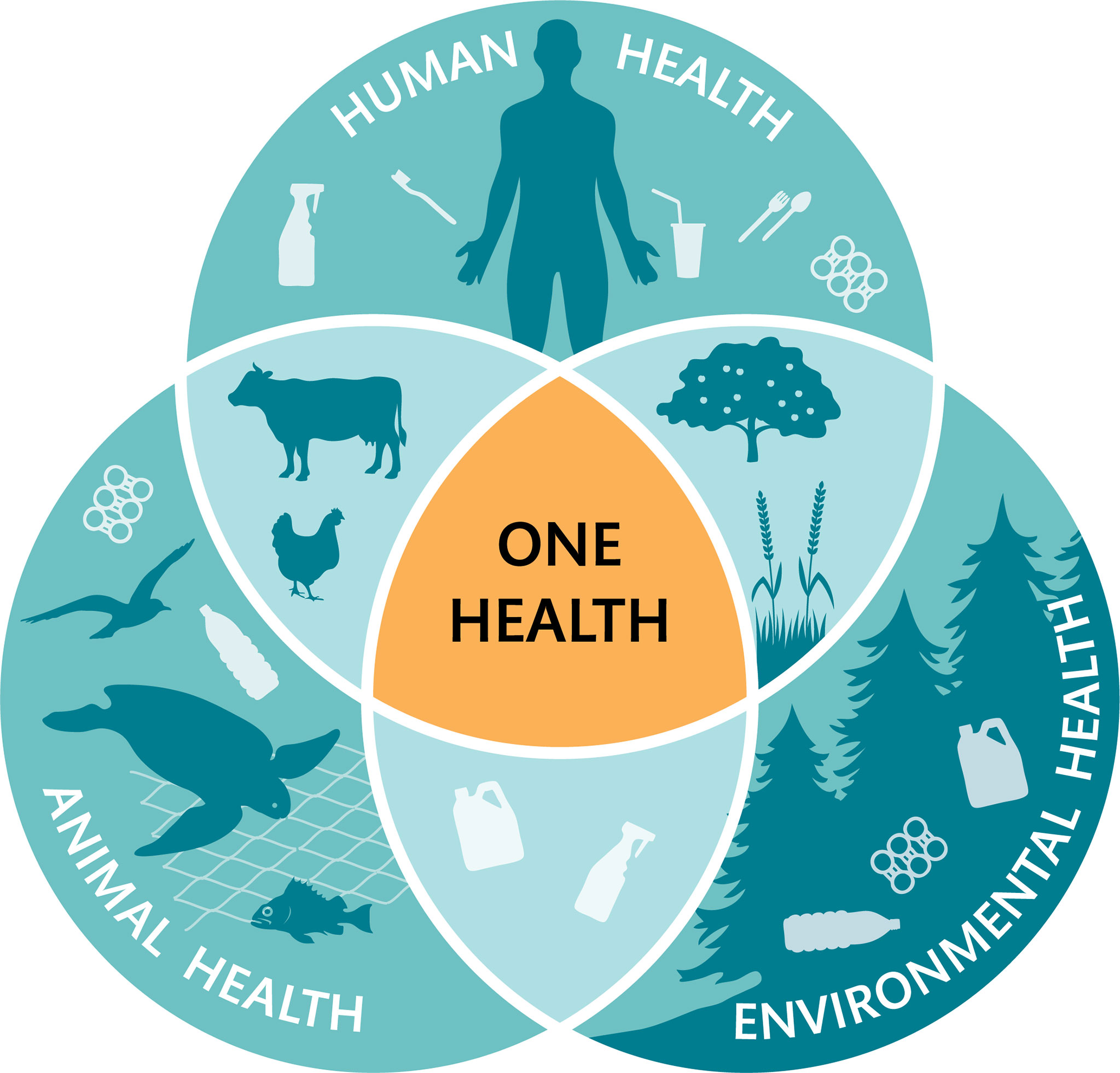
Figure 1 Plastic impacts every facet of the One Health paradigm. One Health views animal, human, and environmental health as a single, interconnected entity, with impacts on one sphere affecting all others, both directly and indirectly. Plastic pollution has multiple potential effects on every aspect of global health.
To establish the need for an integrated assessment, here, we focus on routes of exposure and the health threats at the cellular, individual organismal, population, and ecosystem levels to highlight plastic pollution impacts across layers of biological organization. The goals of this review are to i) summarize our understanding of how plastic affects layers of biological organization, ii) provide rationale for the use of a One Health paradigm to understand and investigate plastic’s consequences on health, and iii) illuminate gaps in existing knowledge and research on the impacts of plastics within the One Health paradigm.
Routes of exposures
Humans and other organisms encounter plastics in a variety of ways, including ingestion, inhalation, and physical contact with plastics and plastic additive chemicals (Cox et al., 2020; World Health Organization, 2022). Humans in the United States are estimated to consume between 39,000 to 52,000 microplastic particles per year from food and beverages alone (Cox et al., 2020) or an average of 0.1-5g of microplastics weekly (Senathirajah et al., 2021). Plastic ingestion is also well documented in other species, including zooplankton (Desforges et al., 2015), fish (Barboza et al., 2020), turtles (Duncan et al., 2019), seabirds (Wilcox et al., 2015), and marine mammals (Nelms et al., 2019).
In addition to ingestion, humans and other terrestrial organisms can also inhale plastics. Micro- and nanoplastics (MNPs) and plastic fibers are released into the atmosphere via the washing of synthetic textiles, rubber tires, dried sludge, agriculture, and city and household dust (Wright and Kelly, 2017; Karbalaei et al., 2018; World Health Organization, 2022). MNPs can even be generated through simple tasks, such as opening and cutting plastic packaging and containers (Sobhani et al., 2020). While the fate of inhaled MNPs and their subsequent uptake in lung tissue is currently unknown (Amato-Lourenço et al., 2021), airborne exposures can occur both indoors, via household items and clothing, as well as outdoors from particulate matter (Kasirajan and Ngouajio, 2012; Wright and Kelly, 2017; Catarino et al., 2018). Occupational exposure, exposure to medical devices, and contact exposure to items such as personal care products and plastic toys also contribute to human exposures (Karbalaei et al., 2018; Zarus et al., 2021).
Exposure to plastics is inexorably associated with exposure to plastic additives—compounds added to plastic to improve the functionality of the polymers (Hahladakis et al., 2018; Wiesinger et al., 2021). Additives include plasticizers, flame retardants, heat and light stabilizers, antioxidants, lubricants, pigments, antistatic agents, slip agents, biocides, and thermal stabilizers (Groh et al., 2019). While these additives are helpful to enhance the performance of plastics, there is great potential for additives to contaminate soil, air, water, and food (Hahladakis et al., 2018), with poorly-understood consequences to the environment and to health.
In addition to the chemicals intentionally added, plastics can carry environmental pollutants and microbes. Collected plastic litter has been associated with diverse bacterial species, including human pathogens, suggesting that plastic may lead to transmission of infectious diseases and may contribute to antimicrobial resistance (Rasool et al., 2021). Plastics accumulate persistent organic pollutants and heavy metals (Thompson et al., 2009; Rochman et al., 2014), though more work is needed to understand if these “Trojan horse” or “vector” effects of adsorption are physiologically relevant (Koelmans et al., 2016). In addition to plastic polymers, we must also consider the potential exposure to a variety of chemicals and microbes, when evaluating impacts of plastics on health.
Cross-species comparisons of cellular and organismal effects of plastics
Effects of plastics on animal health
The wide ranging effects of plastic have been assessed multiple organ impacts have been assessed across taxa, including in fish, phytoplankton, zooplankton, and bivalves (Figure 2). Due to their small size, MNPs have the potential to affect organisms on a cellular level (Prinz and Korez, 2020; Banerjee and Shelver, 2021). For example, in fish, MNPs lead to formation of reactive oxygen species (ROS) and increased oxidative stress, inducing cellular damage in liver, blood cells, gills, digestive tract, and brain (Pitt et al., 2018b; Hu and Palić, 2020; Buwono et al., 2022; Capó et al., 2022; Hoyo-Alvarez et al., 2022; Rangasamy et al., 2022). In fish, MNPs induced mitochondrial stress, altered hormonal regulation of energy metabolism, and catabolic and anabolic processes, which can limit the ability and flexibility of the organism to respond to future stresses (Brun et al., 2019; Trevisan et al., 2019; Trevisan et al., 2020; Wang et al., 2022). Such alterations affect animal fitness, reproduction, and success (Dreier et al., 2019).
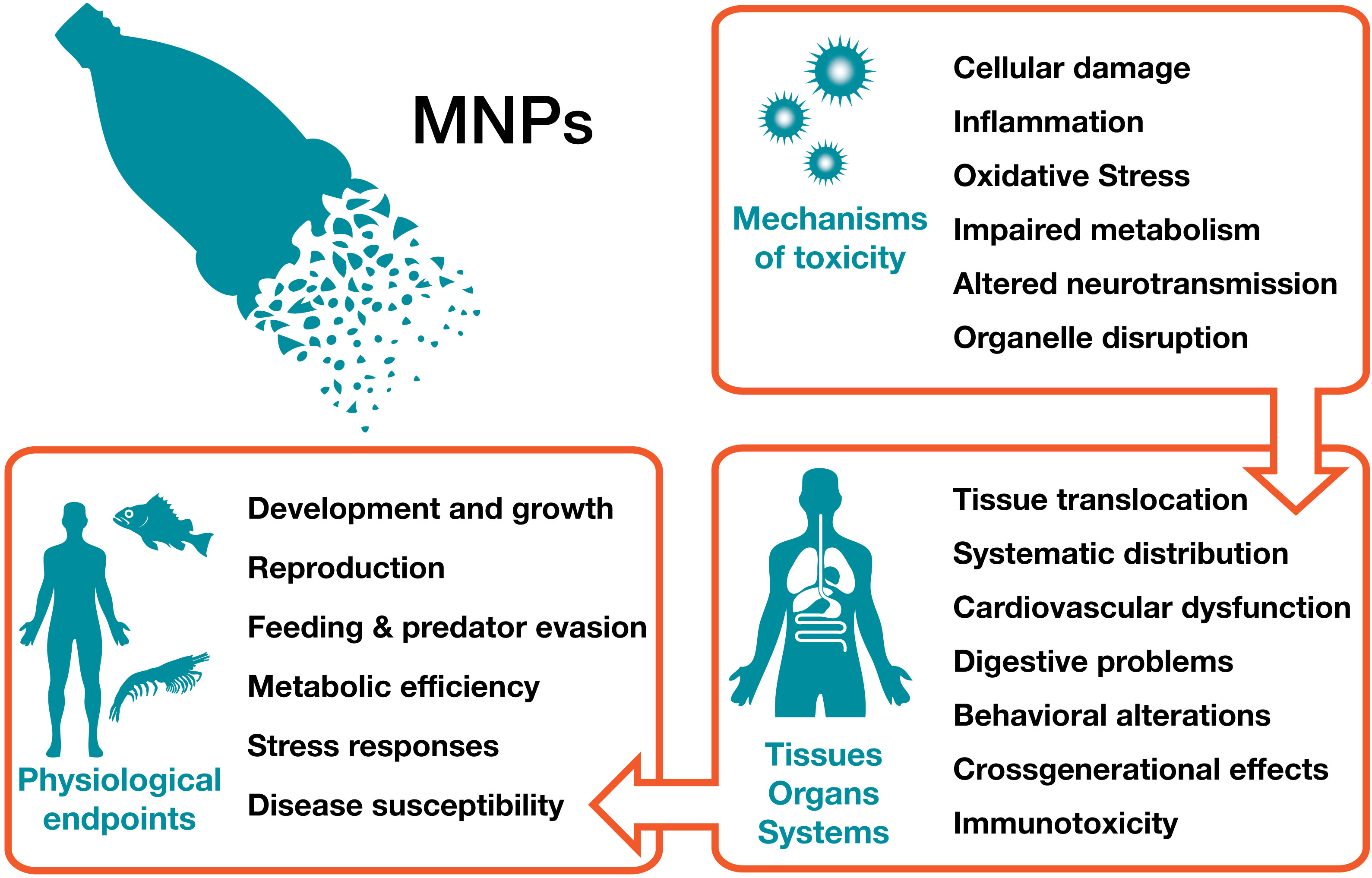
Figure 2 The impacts of plastic across all levels of biological organization. Micro- and nanoplastics (MNPs) affect molecular and cellular responses, tissue and organ systems, and physiological/behavioral responses in multiple species. Adapted from Trevisan et al., 2022.
In algae, MNPs can adsorb to cell walls (Nam et al., 2022) and can increase the formation of ROS, reduce cell viability, modify the activity of antioxidant enzymes, and promote lipid peroxidation (Das et al., 2022). These cellular changes are linked to plastic impacts on organisms’ growth rates and energy demands. For example, nanoplastics may impact freshwater algae development rates (Huang et al., 2019) and metabolism, chlorophyll-a concentrations (Zhang et al., 2018), and maximal quantum yield of photosynthetic system II (Das et al., 2022), although direct effects of plastics are not always evident (Seoane et al., 2019).
MNP exposure also affects growth, reproduction, and fitness of many invertebrates. For example, MNP exposure studies in copepods [e.g., Calanus finmarchicus (Cole et al., 2019), Daphnia magna (An et al., 2021), and Artemia parthenogenetica)] (Wang et al., 2019), in clams [Corbicula fluminea (Oliveira et al., 2018)], and mussels [Mytilus galloprovincialis (Avio et al., 2015; Abidli et al., 2021)] show effects on mitochondrial gene expression, prey preference, lipid content, molting, feeding rates, filter feeding, survival, growth, and reproduction. In mussels (Mytilus edulis), high-density polyethylene exposure resulted in the accumulation of microplastics in the digestive gland, inflammatory reactions, and lysosomal membrane damage (von Moos et al., 2012). On the other hand, oysters exposed to polystyrene microplastics had a 3% higher algal consumption rate and an 11% higher absorption efficiency of organic matter from ingested food rates (Sussarellu et al., 2016), possibly induced as a compensation for the higher energy demand caused by the detected digestive interference of microplastics. Sampling of gametes from oysters (Crassostrea gigas) showed that exposure to 2 μm and 6 μm polystyrene particles led to a 38% drop in oocyte counts and a 23% decrease in the mobility rate of spermatozoa. The offspring produced by artificial fertilization using gametes from exposed parents had a 20% reduction in D-larval yield, an 18% reduction in larval size at 17 days post-fertilization, and a 6-day delay in the time necessary to complete metamorphosis (Sussarellu et al., 2016). These results demonstrate that small plastic particles can affect the health of bivalves at the subcellular and physiological levels.
The intestinal microbiota of vertebrates is critical for health, and disturbance to the microbiota leads to increased risk of disease; however, few studies have investigated the impact of plastic exposure on the gut microbiome. One study exposed male zebrafish to polystyrene microplastics and observed greater mucus levels and significant changes to the species richness and diversity of microbiota in the polystyrene microplastic-exposed zebrafish (Jin et al., 2018). In another study, adult zebrafish (Danio rerio) co-exposed to titanium dioxide nanoparticles and the plasticizer bisphenol A (BPA) shifted the intestinal microbial community (Chen et al., 2018).
Exposure to MNPs can cause disruption in the immune and antioxidant systems, as well as the nervous and reproductive systems. For example, mussels exposed to a combination of 2 and 6 μm polystyrene microplastics exhibited disturbance of cellular homeostasis in hemocytes, infiltration of these cells into digestive system tissues, and changes in the activity or gene expression of antioxidant enzymes in gills and digestive glands (Paul-Pont et al., 2016). Sheepshead minnows (Cyprinodon variegatus) exposed to irregularly-shaped microplastics led to upregulation of Cxcr5 and Tnfsf13b, both of which are involved in B cell development (Choi et al., 2018). Other studies have demonstrated increased inflammation in fish when fed irregularly-shaped microplastics (Jiang et al., 2016; Tao et al., 2016). In contrast, exposure to PVC microplastics in gilthead seabream (Sparus aurata L.) did not alter humoral or cellular immunity, but produced cellular and oxidative stress. These results agree with altered albumin, total proteins and globulin levels observed in juvenile Clarias gariepinus serum after virgin microplastic ingestion (Karami et al., 2016).
At the level of the nervous system, exposure of MNPs have also been to affect fish behavior, including reduced swim speed and erratic swimming (Barboza et al., 2018); reduced locomotor activity (Chen et al., 2017); reduced predator avoidance behavior and dysregulated circadian rhythm locomotion (Sarasamma et al., 2020); increased shoal formation and feeding time and less exploration (Mattsson et al., 2017); and reduced predatory performance (Carlos de Sá et al., 2015; Wen et al., 2018). Alterations to locomotory behavior (Bergami et al., 2016) and burrowing kinetics (Silva et al., 2020) have been observed in brine shrimp larvae and polychaeteas upon exposure to nanoplastics.
Ingested MNPs at the lower micron range (<5 µm) can cross the gastrointestinal barrier, reaching the blood and potentially moving to other body compartments (Roch et al., 2020). Very small MNPs can also cross other biological barriers, including the egg chorion (usually particles smaller than a few hundred nm) and the blood brain barrier (particles smaller than a few dozen µm) (Guerrera et al., 2021). This potential for translocation across tissue barriers poses an additional threat to multicellular organisms, as multiple physiological systems could potentially be affected by the plastic particles. Early developmental stages can be particularly susceptible to the translocation of plastics to different organs, as many of these biological barriers are not fully developed, thereby facilitating the distribution of plastic to multiple organs (Pitt et al., 2018a).
Another source of concern, particularly in fish and other marine organisms, is the transfer of plastics through the food web and between generations. MNPs can interact with phytoplankton and zooplankton, which can then be consumed by small fish and passed up the food chain (Benson et al., 2022). Dietary exposure of fish to MNPs can reduce growth rate, cause liver damage, impair swimming performance, and create behavioral abnormalities (da Costa Araújo et al., 2020; Kim et al., 2022). Recent research has also revealed that the translocation of plastics to the gonads of fish can result in the cross-generational transfer of these particles to the offspring, as well as developmental and physiological damage (Pitt et al., 2018b; Zhao et al., 2021). Because most plastic particles are hydrophobic, oocytes may be important targets for the bioaccumulation of MNPs due to their larger size and higher lipid content, suggesting that female fish may be important vectors for the cross-generational transfer of plastics (Pitt et al., 2018b). The interaction of plastic particles with blood proteins, such as vitelogenin, which has already been found with polystyrene nanoplastics, can promote the transportation of plastics to the female gonads and oocytes (Rossi et al., 2014), a topic that requires further investigation.
It is clear from these studies that plastics induce substantial effects on the biology and fitness of these keystone species. Crustacean zooplankton, such as copepods, daphnids, and brine shrimp, play a key role in community structure and act as a critical connection in the trophic web between primary producers and secondary consumers. Bivalves are primary consumers at the base of the food chain that also offer habitat, can improve the diversity and complexity of coastal ecosystems, link the benthic and pelagic systems through their filter-feeding activity, and are an essential nutrient source for other species. Fish are an important food source globally, and play a large role in the ocean food web. Microalgae are vital to the productivity of aquatic environments and play a crucial role in community structure. Plastic exposure could alter the health and abundance of this critically important group of species. While the ecological repercussions of such changes to coastal ecosystems still need to be determined, the ubiquity and volume of plastics and their numerous negative impacts across species call for an urgent need to better understand these consequences and how to combat them.
Effects of plastics on humans
While it is evident that humans have regular exposure to plastics and their byproducts, the impact of these exposures on human health is not currently well understood. Research to date suggests that the potential health effects of exposure to plastics include respiratory irritation, dyspnea, decreased lung capacity, coughing, obesity, increased phlegm production, cardiovascular disease, asthma, and cancer (Wright and Kelly, 2017; Karbalaei et al., 2018; World Health Organization, 2022). It has also been postulated that MNPs may cause inflammation, immune dysfunction, neurotoxicity, neoplasia, and changes in metabolism (Wang et al., 2020; Banerjee and Shelver, 2021; Coffin et al., 2022; World Health Organization, 2022). Furthermore, as observed in fish, human ingestion of microplastics has the potential to impact gut health. Exposure to microplastics can cause inflammation in the gut and destruction of the gut epithelium, which can lead to intestinal leakage and could pose a significant health threat (Huang et al., 2021). This inflammation is thought to be driven by an increase in oxidative stress in intestinal epithelial cells. Additionally, microplastics can reduce the mucus layer in the intestines, which serves as an important chemical barrier in the gut (Huang et al., 2021). Studies have also shown that microplastics affect the microbiota in the gut, which can destabilize the intestinal microenvironment (Yong et al., 2020; Huang et al., 2021).
Much of the research that has explored the health impacts of plastics and plastic additives in humans has focused on the effects of BPA and phthalates. BPA and phthalates are known endocrine disrupting chemicals, and therefore affect development and reproduction. In men, this can manifest as declined reproductive capacity or increased risk of testicular and prostate cancer, whereas in women this can manifest as increased risk for endometriosis, reproductive related cancers, and impaired ovarian function and menstrual cycling (Meeker et al., 2009; Kim and Kim, 2020). Exposure to endocrine disrupting chemicals in utero may contribute to diseases of the testis, prostate, kidney, immune system, and cause tumors (Basak et al., 2020). Additionally, exposure to phthalates is positively correlated with shorter gestational age at delivery and worse in vitro fertilization outcomes (Latini et al., 2003; Machtinger and Orvieto, 2014; Basak et al., 2020). BPA levels in blood have also been shown to be associated with impaired thyroid functioning (Kwon et al., 2020).
BPA and phthalates may also have neurological impacts by inducing changes in the neuroendocrine system and inflammatory signaling (Solleiro-Villavicencio et al., 2020; Nadeem et al., 2021). For example, BPA can pass through the blood-brain barrier, and BPA exposure is linked with neuropsychological dysfunction, neurobehavioral disorders, and neurodegenerative disease (Wang et al., 2019). Exposure to BPA and phthalates is also associated with alterations to the cardiovascular system and metabolism, with studies showing a positive relationship between BPA and phthalates and cardiovascular disease, type 2 diabetes, and increased blood pressure (Lang et al., 2008; Gong et al., 2013; Haq et al., 2020; Mariana and Cairrao, 2020). BPA has also been shown to have epigenetic impacts, such as affecting DNA methylation in first trimester trophoblast cells, sperm cells, prostate carcinoma cells, and neuroblastoma cells (Manikkam et al., 2013; Senyildiz et al., 2017; Basak et al., 2018; Fatma Karaman et al., 2019). It has also been demonstrated that BPA can cause epigenetic alterations that impact cardiac development and metabolic dysfunction (Lombó et al., 2015; Junge et al., 2018). Many of the above studies examined correlations between BPA and phthalate concentrations in humans and the increased risk of certain health impacts, which highlights the potential health effects of exposure to environmentally-relevant doses of these chemicals.
While the vast majority of research on the health impacts of plastics has focused on BPA and phthalates, recent studies have identified more than 10,000 substances related to the manufacture of plastics, including over 2,400 substances that are identified as substances of potential concern (Hahladakis et al., 2018; Groh et al., 2019; Wiesinger et al., 2021). Clearly, the current research has focused on only a small fraction of the additives to which we are likely exposed on a regular basis, demonstrating a clear dearth of knowledge surrounding the full health risks posed by plastics. When these studies expand beyond just a few chemicals, clear exposures are identified. For example, a study that tested estrogenic and androgenic activity in the saliva from children exposed to 18 toys found nine of the 18 toys to have estrogenic effects (Kirchnawy et al., 2020). Of the nine toys that induced an estrogenic response, seven could not be explained by analysis for 41 known endocrine disrupting chemicals, suggesting that other unknown plastic additives existed in these toys with potential to threaten human health.
Furthermore, the ability for plastic additives to leach out of plastic remains a matter of continued debate. Several studies have examined the leachability of certain additives from items such as plastic water bottles, kitchen utensils, and plastic water pipes with mixed results. While some studies have found estrogenic activity in drinking water resulting from plastic bottles and pipes (Wagner and Oehlmann, 2011; Liu et al., 2017), others have determined that the levels of leached additives are below those that would pose a threat to human health (Corea-Téllez et al., 2008; Aneck-Hahn et al., 2018; Wang et al., 2019). However, these studies fail to consider the cumulative exposure that an individual may have across sources and over time. When added together, the total exposure to these chemicals may very well exceed the acceptable thresholds; however, current research has yet to quantify such cumulative exposures. The effect of simultaneous co-exposures to these chemicals on human health is also poorly understood, despite the fact that human exposures to complex mixtures of compounds are well documented (Meeker et al., 2009). Furthermore, these studies do not account for the possibility for increased leaching over time, since factors such as UV exposure, mechanical abrasion, hydrolysis, and oxidation cause plastics to break down and release chemicals (Walker et al., 2021). With plastic production and use steadily on the rise, human exposure to plastic will continue to increase. Further, efforts towards waste reduction are driving growth in the reuse of plastic materials, which may also increase health risks due to potential increased chemical leaching (Muncke et al., 2020).
There is also evidence that the impacts of plastic on human health are not readily reversible, given that exposure to plastic additives may continue even after removal of plastics from one’s environment. For example, BPA was detected in 23 out of 29 urinary samples from workers in a hazardous waste incinerator, despite the implementation of BPA regulations after a certain time (González et al., 2019). Additionally, an intervention study that removed all sources of plastics from a family’s household failed to lead to a clear reduction of phthalate metabolites in urine in all family members even after two months (Hutter et al., 2016). It has also been shown that in office spaces where phthalate-containing materials or sources have been removed, phthalates were still present in dust in non-negligible concentrations (Hutter et al., 2006). This underscores how widespread plastics and plastic additives are in our environment and how difficult it is to avoid such exposures, even with local mitigation or rigorous avoidance strategies.
A recent report released by the World Health Organization highlights the urgent need for improved research on the health effects of MNPs, as research to date is “incomplete and insufficient for an assessment of human risk” (World Health Organization, 2022). While research on the health impacts of plastic is lagging woefully behind human consumption of plastic products, it is clear that plastics have the potential to affect human health in multiple ways. The physical properties of plastics have the potential to damage organs, such as the gastrointestinal and respiratory systems, and chemical exposures from these plastics can have systemic effects, ranging from cellular effects on oxidative stress and apoptosis, to impacts on reproduction, development, metabolism, and even intergenerational effects through epigenetic modifications. As a result, there is an “overwhelming consensus” that measures should be taken to mitigate exposure to MNPs (World Health Organization, 2022).
Disparities exist in causes and consequences of plastic exposure
As with many societal challenges, the impacts of plastic pollution are not distributed evenly across populations. Since the late 1980s, high-income countries have been the primary exporters of plastic pollution, accounting for 87% of all exports (Brooks et al., 2018). Six of the top 20 plastic polluters are high-income countries (United States, Japan, Kuwait, Oman, Argentina, and Italy) (Law et al., 2020) These exports are primarily to lower-income countries in Asia and the Pacific (Brooks et al., 2018). The waste-management infrastructure in the countries receiving these exports cannot handle the excess burden of the exports, which contributes to the disproportional impacts of plastic pollution in these countries (Ncube et al., 2021). The excess burden of plastic waste in specific communities is further compounded by housing shortages and unemployment, both of which can lead to circumstances where humans are prompted to deliberately stay in these areas to better adapt to the more urgent challenges of poverty. For example, the Smokey Mountain in the Philippines, an unregulated dumpsite no less than 20 meters high, housed 30,000 homeless or scavenging Filipino families for 40 years before it was closed in the 1990s (Galarpe, 2015)
Plastic pollution exacerbates the climate-instigated downturn of agriculture and fishery industries that serve as the primary economic activities for certain societies. For example, approximately 10% of the world’s population relies heavily on marine environments for their diet and livelihood, with the vast majority (95%) from developing nations (Food and Agriculture Organization, 2014; Taylor et al., 2019). Low-lying Pacific islands with limited arable land bear the brunt of the plastic crisis. Tuvalu, for instance, clings to “blue economy” policies contingent on the use of marine resources to keep their economy and people afloat (International Organization for Migration and International Labour Organization, 2021). These circumstances make the island nation among the hardest hit by plastic accumulation in marine environments and the climate effects of plastic production and incineration. The disproportionate impact of plastic waste on specific communities should be interrogated through a holistic exploration of geo-economic, environmental, structural, and socio-political underpinnings.
Ecosystem-wide effects of plastic
Ecosystem health, function, and services are critically linked with human physical health as well as societal, cultural, and economic well-being (Summers et al., 2012). The various consequences of plastic across all levels of biological organization from cells to populations portend a grim future with respect to the constitution of the natural world, inclusive of humans, and can be exemplified by sentinel species. Among these sentinel species, many marine apex predators, such as marine mammals, have long life spans, amplify trophic information across multiple spatiotemporal scales, and share food resources of commercial and subsistence importance to humans, making them efficacious harbingers of negative impacts to both individual- and population-level animal and human well-being (Bossart, 2011; Hazen et al., 2019). Trophic transfer of microplastic particles to marine mammals from contaminated prey who have consumed microplastics is thought to be the primary route of microplastic exposure for both filter and raptorial predators (Zantis et al., 2022). The direct link between humans and marine mammals is self-evident: as top predators with shared resources, exposure to microplastics in humans via consumption is concerning. However, a larger question of indirect consequences looms: does plastic pollution threaten whole ecosystem collapse?
Whether or not plastic threatens the functionality of whole ecosystems is poorly studied (Bucci et al., 2020); however, the potential downstream consequences of plastic to marine mammals and the ecosystems they inhabit are not difficult to imagine, particularly when contextualized through a framework of population consequences of disturbance (Ocean Studies Board et al., 2017; Bucci et al., 2020). Interaction with macroplastic, such as ingestion or entanglement, can lead to physiological and behavioral changes that induce acute or lethal consequences impacting vital rates and subsequently population dynamics (Ocean Studies Board et al., 2017). Similarly, both micro and macroplastics may have chronic, sublethal impacts on individual health, which may also lead to alterations in vital rates (Ocean Studies Board et al., 2017). As instrumental players in nutrient cycling (Roman et al., 2016), the reduction of a whale population, for example, may result in a catastrophic depletion of energy at lower trophic levels that rely on whale excrement and carcasses. This disruption to energy availability at the lower trophic levels could potentially reverberate up each trophic level, including those with cultural, subsistence, and commercial importance to humans, resulting in whole ecosystem remodeling or collapse. Indeed, marine mammals are of great cultural and subsistence importance to indigenous communities (Huntington et al., 2016). For most of the contemporary global human population, marine mammals serve as clear sentinels for a variety of environmental and ecological threats (Bossart, 2011; Hazen et al., 2019). But for some native peoples who consume them, the meat from contaminated marine mammals may have direct consequences to users’ health. Ingestion of plastic by whales, seals, sea lions, and polar bears is well documented and may either translocate to, or leach toxic substances into, consumable tissues (Law, 2017; Zantis et al., 2021). Plastic consumed by marine mammals therefore threatens a critical life line, and a way of life, for several indigenous communities world-wide.
Of course, many factors influence the proper functioning of an ecosystem, and processes like emigration/immigration, prey-switching, shifts in species assemblages and niche partitioning among others may all affect the ultimate ecosystem-level consequence of disturbances resulting from plastic exposure. In addition, ecosystems contend with many anthropogenic stressors apart from plastic. Consequently, the interactions between exposure to plastic and climate change, habitat loss/degradation, exploitation, etc. need to be explored, and safeguarding regular and proper functioning of ecosystems from plastic pollution is critical to optimal human, organism, and environmental vitality.
Solutions, adaptations, and future research efforts
As human demand for plastic continues, new solutions will be needed that span the entirety of societal structure, including novel technological innovations to degrade or recycle plastic, campaigns directed at consumer behavior, and implementation of bold policies at all levels of government. These solutions must be implemented across the entire lifecycle of plastic, from reducing the amount of new plastic entering the environment to removing existing plastic pollution. Technological innovations that are underway for clean-up and remediation efforts include a variety of plastic capture approaches. These tools are summarized in “The Inventory,” a summary of 52 inventions, such as ocean plastic skimmers, beach cleaning robots, and river and ocean debris filters, that are focused on preventing plastic leakage or collecting marine plastics (Schmaltz et al., 2020). Although these technologies are a necessary component of our efforts to mitigate plastic pollution, their scalability and effectiveness to date does not match the enormity of the plastic pollution problem.
Another novel approach to prevent plastic pollution is the utilization of plastic-degrading bacteria as a mechanism to create a “circular economy of plastic”. As plastic has increased in the environment over the past century, microorganisms have evolved enzymes to degrade plastic [reviewed in (Sheth et al., 2019)]. While there may be hundreds of bacterial strains that have evolved plastic-degrading properties, none have been able to do so rapidly; however, further refinement of these naturally-evolved enzymes has led to increasingly-efficient microbially-mediated plastic bioremediation systems (Tournier et al., 2020; Lu et al., 2022). In addition to these substantial improvements in bacterially-mediated degradation of plastic, it will be important to process plastic waste into forms that are readily and fully biodegradable, such as through amorphization of micronization.
Concomitant with the development of new technologies, governments around the world are increasingly using policy, laws, and ordinances to target the plastic pollution issue. Policies can target plastic pollution in a variety of ways through the implementation of regulatory, economic, and educational instruments. A recent review of plastic policies around the world found that international policies primarily focus on plans and future actions, while national and subnational policies most frequently use plastic bans to achieve a reduction in plastic pollution (Diana et al., 2022). Despite this increasing trend, substantial gaps still remain across the policy space, including the types of plastic targeted by these policies. For example, within national policies throughout the world, macroplastics were the most common plastic type targeted, followed by plastic bags (Diana et al., 2022), while only 3 of the 147 national policies to date solely target microplastics. Furthermore, only 5% of national policies have effectiveness studies in the peer-reviewed literature, highlighting the need for more evidenced-based policy development in the future (Diana et al., 2022). Finally, notably lacking from global policy is a binding global treaty targeting plastic pollution (Karasik et al., 2020). Despite an increasing trend of policy implementation to combat plastic waste, progress has been stymied by the COVID-19 pandemic, which prompted a pause in many policies around the world due to safety concerns regarding reusable materials (Karasik et al., 2020). Existing policy limitations, compounded by COVID-19 impacts, call for improved and coordinated policy efforts globally.
To help guide global policy efforts, a planetary boundaries approach has been proposed to first define the limits of waste production that ensure that Earth remains a “safe operating space” for humanity (Folke et al., 2021). To date, planetary boundaries have been defined for climate change, genetic diversity, land-system change, freshwater use, biochemical flows (phosphorus and nitrogen), ocean acidification, and the depletion of stratospheric ozone depletion (Steffen et al., 2015). However, experts have not yet defined planetary boundaries for plastics or other novel entities. Quantifying the planetary boundary for plastic pollution can help society to understand whether or not plastic pollution is driving large-scale and irreversible harm to the planet and identify measures to prevent exceeding the boundary. By changing ecosystems, generating greenhouse gasses, and impacting the health of people and animals, it remains unclear whether plastic pollution could reach levels that would render the planet inhospitable. Recent efforts have sought to characterize the dangerous pathways that plastic could lead to such irreversible impacts in order to better understand the cumulative and planetary impacts of plastic pollution (Diana et al., 2022). These efforts are the first step towards defining a limit for plastic pollution, which can then facilitate the development of global policy to keep society within the identified boundary.
Finally, in addition to improved technologies and policies that target plastic pollution, increased research on the impacts of plastic are also needed. A recent review of studies examining impacts of plastic pollution highlighted several important gaps in research to date (Bucci et al., 2020). Observational or manipulative field experiments have largely focused on macroplastics (97%), while manipulative laboratory experiments have largely focused on microplastics (96%). Of the experiments that researched microplastics, the majority used polyethylene and polystyrene, and only a few investigated other polymer types such as PVC, PET, polypropylene, and others. Finally, 76% of all studies focused only on the marine environment, whereas relatively little research has been conducted on freshwater and terrestrial ecosystems. Understanding the effects of different plastic types, different sizes and shapes of plastics, as well as the effects in different ecosystems is critical to gaining a complete understanding of the health impacts of plastic pollution globally.
Conclusions
Mounting evidence suggests that plastic can impact multiple layers of biological organization, from molecular and cellular to organismal and population levels. These impacts are wide-ranging, inducing alterations to inflammation and oxidative stress, metabolic function, neurologic function, behavior, reproduction and development, and the microbiome. These effects are mediated both by the physical impacts of ingested or absorbed plastic particles and by the chemicals and microbes present in or on the plastics.
Despite the growing body of research on the impacts of plastics on global human, animal, plant, and overall ecosystem health, many questions remain. For one, more systematic and comprehensive studies are needed to account for the widespread differences in polymer type, plastic particle size, and additive mixtures. Additionally, there is a notable lack of research that integrates cell, organismal, population- and ecosystem-level impacts of plastic pollution, and little is understood about the cumulative exposure to plastics and additives over time across these levels of biology. Furthermore, the pace of global policy response and the adoption of plastic-reducing technologies is lagging substantially behind the rate of plastic consumption and production. A One Health approach can help address these knowledge gaps by providing a framework in which to integrate across biological scales, promote transdisciplinary partnerships, and engage stakeholders from diverse perspectives in an effort to mitigate and prevent the accelerating global plastic pollution crisis for the protection of all life on Earth.
Author contributions
MM, RT, PR, GM, JS, NJ, WE and AH each wrote sections of the manuscript. JS, NJ and WE helped supervise the project. All authors contributed to the article and approved the submitted version.
Funding
The authors acknowledge funding support from the Nicholas Institute for Environmental Policy Solutions (pre-catalyst grant to JAS) and the Duke Bass Connections program (JAS). The authors thank members of the Duke Plastic Pollution Working Group, particularly Zoie Diana and Dr. Meagan Dunphy-Daly, for helpful discussions. The authors acknowledge Uko Gorter for providing figure illustrations.
Conflict of interest
The authors declare that the research was conducted in the absence of any commercial or financial relationships that could be construed as a potential conflict of interest.
Publisher’s note
All claims expressed in this article are solely those of the authors and do not necessarily represent those of their affiliated organizations, or those of the publisher, the editors and the reviewers. Any product that may be evaluated in this article, or claim that may be made by its manufacturer, is not guaranteed or endorsed by the publisher.
References
Abidli S., Pinheiro M., Lahbib Y., Neuparth T., Santos M. M., Trigui El Menif N. (2021). Effects of environmentally relevant levels of polyethylene microplastic on mytilus galloprovincialis (Mollusca: Bivalvia): filtration rate and oxidative stress. Environ. Sci. pollut. Res. 28, 26643–26652. doi: 10.1007/s11356-021-12506-8
Aloy A. B., Vallejo B. M., Juinio-Meñez M. A. (2011). Increased plastic litter cover affects the foraging activity of the sandy intertidal gastropod nassarius pullus. Mar. pollut. Bull. 62, 1772–1779. C.OMMAJ.R.X.X.X. doi: 10.1016/j.marpolbul.2011.05.021
Amato-Lourenço L. F., Carvalho-Oliveira R., Júnior G. R., Dos Santos Galvão L., Ando R. A., Mauad T. (2021). Presence of airborne microplastics in human lung tissue. J. Hazard. Mater. 416, 126124. doi: 10.1016/j.jhazmat.2021.126124
Aneck-Hahn N. H., Van Zijl M. C., Swart P., Truebody B., Genthe B., Charmier J., et al. (2018). Estrogenic activity, selected plasticizers and potential health risks associated with bottled water in south Africa. J. Water Health 16, 253–262. doi: 10.2166/wh.2018.043
An D., Na J., Song J., Jung J. (2021). Size-dependent chronic toxicity of fragmented polyethylene microplastics to daphnia magna. Chemosphere 271, 129591. doi: 10.1016/j.chemosphere.2021.129591
Avio C. G., Gorbi S., Milan M., Benedetti M., Fattorini D., d’Errico G., et al. (2015). Pollutants bioavailability and toxicological risk from microplastics to marine mussels. Environ. pollut. 198, 211–222. doi: 10.1016/j.envpol.2014.12.021
Baini M., Martellini T., Cincinelli A., Campani T., Minutoli R., Panti C., et al. (2017). First detection of seven phthalate esters (PAEs) as plastic tracers in superficial neustonic/planktonic samples and cetacean blubber. Anal. Methods 9, 1512–1520. doi: 10.1039/C6AY02674E
Banerjee A., Shelver W. L. (2021). Micro- and nanoplastic induced cellular toxicity in mammals: A review. Sci. Total Environ. 755, 142518. doi: 10.1016/j.scitotenv.2020.142518
Barboza L. G. A., Lopes C., Oliveira P., Bessa F., Otero V., Henriques B., et al. (2020). Microplastics in wild fish from north East Atlantic ocean and its potential for causing neurotoxic effects, lipid oxidative damage, and human health risks associated with ingestion exposure. Sci. Total Environ. 717, 134625. doi: 10.1016/j.scitotenv.2019.134625
Barboza L. G. A., Vieira L. R., Guilhermino L. (2018). Single and combined effects of microplastics and mercury on juveniles of the European seabass (Dicentrarchus labrax): Changes in behavioural responses and reduction of swimming velocity and resistance time. Environ. pollut. 236, 1014–1019. doi: 10.1016/j.envpol.2017.12.082
Barnes D. K. A., Milner P. (2005). Drifting plastic and its consequences for sessile organism dispersal in the Atlantic ocean. Mar. Biol. 146, 815–825. doi: 10.1007/s00227-004-1474-8
Barrett J., Chase Z., Zhang J., Holl M. M. B., Willis K., Williams A., et al. (2020). Microplastic pollution in deep-sea sediments from the great Australian bight. Front. Mar. Sci. 7. doi: 10.3389/fmars.2020.576170
Basak S., Das M. K., Duttaroy A. K. (2020). Plastics derived endocrine-disrupting compounds and their effects on early development. Birth Defects Res. 112, 1308–1325. doi: 10.1002/bdr2.1741
Basak S., Srinivas V., Duttaroy A. K. (2018). Bisphenol-a impairs cellular function and alters DNA methylation of stress pathway genes in first trimester trophoblast cells. Reprod. Toxicol. 82, 72–79. doi: 10.1016/j.reprotox.2018.10.009
Benson N. U., Agboola O. D., Fred-Ahmadu O. H., De-la-Torre G. E., Oluwalana A., Williams A. B. (2022). Micro(nano)plastics prevalence, food web interactions, and toxicity assessment in aquatic organisms: A review. Front. Mar. Sci. 9. doi: 10.3389/fmars.2022.851281
Bergami E., Bocci E., Vannuccini M. L., Monopoli M., Salvati A., Dawson K. A., et al. (2016). Nano-sized polystyrene affects feeding, behavior and physiology of brine shrimp artemia franciscana larvae. Ecotoxicol. Environ. Saf. 123, 18–25. doi: 10.1016/j.ecoenv.2015.09.021
Bergmann M., Wirzberger V., Krumpen T., Lorenz C., Primpke S., Tekman M. B., et al. (2017). High quantities of microplastic in Arctic deep-Sea sediments from the HAUSGARTEN observatory. Environ. Sci. Technol. 51, 11000–11010. doi: 10.1021/acs.est.7b03331
Bossart G. D. (2011). Marine mammals as sentinel species for oceans and human health. Vet. Pathol. 48, 676–690. doi: 10.1177/0300985810388525
Brooks A. L., Wang S., Jambeck J. R. (2018). The Chinese import ban and its impact on global plastic waste trade. Sci. Adv. 4, eaat0131. doi: 10.1126/sciadv.aat0131
Brun N. R., van Hage P., Hunting E. R., Haramis A.-P. G., Vink S. C., Vijver M. G., et al. (2019). Polystyrene nanoplastics disrupt glucose metabolism and cortisol levels with a possible link to behavioural changes in larval zebrafish. Commun. Biol. 2, 382. doi: 10.1038/s42003-019-0629-6
Bucci K., Tulio M., Rochman C. M. (2020). What is known and unknown about the effects of plastic pollution: A meta-analysis and systematic review. Ecol. Appl. 30, e02044. doi: 10.1002/eap.2044
Buwono N. R., Risjani Y., Soegianto A. (2022). Oxidative stress responses of microplastic-contaminated gambusia affinis obtained from the brantas river in East Java, Indonesia. Chemosphere 293, 133543. doi: 10.1016/j.chemosphere.2022.133543
Capó X., Alomar C., Compa M., Sole M., Sanahuja I., Soliz Rojas D. L., et al. (2022). Quantification of differential tissue biomarker responses to microplastic ingestion and plasticizer bioaccumulation in aquaculture reared sea bream sparus aurata. Environ. Res. 211, 113063. doi: 10.1016/j.envres.2022.113063
Carlos de Sá L., Luís L. G., Guilhermino L. (2015). Effects of microplastics on juveniles of the common goby (Pomatoschistus microps): confusion with prey, reduction of the predatory performance and efficiency, and possible influence of developmental conditions. Environ. pollut. 196, 359–362. doi: 10.1016/j.envpol.2014.10.026
Carson H. S., Colbert S. L., Kaylor M. J., McDermid K. J. (2011). Small plastic debris changes water movement and heat transfer through beach sediments. Mar. pollut. Bull. 62, 1708–1713. doi: 10.1016/j.marpolbul.2011.05.032
Catarino A. I., Macchia V., Sanderson W. G., Thompson R. C., Henry T. B. (2018). Low levels of microplastics (MP) in wild mussels indicate that MP ingestion by humans is minimal compared to exposure via household fibres fallout during a meal. Environ. pollut. 237, 675–684. doi: 10.1016/j.envpol.2018.02.069
Chen Q., Gundlach M., Yang S., Jiang J., Velki M., Yin D., et al. (2017). Quantitative investigation of the mechanisms of microplastics and nanoplastics toward zebrafish larvae locomotor activity. Sci. Total Environ., 584–585, 1022–1031. doi: 10.1016/j.scitotenv.2017.01.156
Chen L., Guo Y., Hu C., Lam P. K. S., Lam J. C. W., Zhou B. (2018). Dysbiosis of gut microbiota by chronic coexposure to titanium dioxide nanoparticles and bisphenol a: Implications for host health in zebrafish. Environ. pollut. 234, 307–317. doi: 10.1016/j.envpol.2017.11.074
Choi J. S., Jung Y.-J., Hong N.-H., Hong S. H., Park J.-W. (2018). Toxicological effects of irregularly shaped and spherical microplastics in a marine teleost, the sheepshead minnow (Cyprinodon variegatus). Mar. pollut. Bull. 129, 231–240. doi: 10.1016/j.marpolbul.2018.02.039
Coffin S., Bouwmeester H., Brander S., Damdimopoulou P., Gouin T., Hermabessiere L., et al. (2022). Development and application of a health-based framework for informing regulatory action in relation to exposure of microplastic particles in California drinking water. Microplastics Nanoplastics 2, 12. doi: 10.1186/s43591-022-00030-6
Cole M., Coppock R., Lindeque P. K., Altin D., Reed S., Pond D. W., et al. (2019). Effects of nylon microplastic on feeding, lipid accumulation, and moulting in a coldwater copepod. Environ. Sci. Technol. 53, 7075–7082. doi: 10.1021/acs.est.9b01853
Corea-Téllez K. S., Bustamante-Montes P., García-Fábila M., Hernández-Valero M. A., Vázquez-Moreno F. (2008). Estimated risks of water and saliva contamination by phthalate diffusion from plasticized polyvinyl chloride. J. Environ. Health 71, 34–9, 45. Available at: https://www.ncbi.nlm.nih.gov/pmc/articles/PMC5633929/
Courtene-Jones W., Maddalene T., James M. K., Smith N. S., Youngblood K., Jambeck J. R., et al. (2021). Source, sea and sink–a holistic approach to understanding plastic pollution in the southern Caribbean. Sci. Total Environ. 797, 149098. doi: 10.1016/j.scitotenv.2021.149098
Cox K. D., Covernton G. A., Davies H. L., Dower J. F., Juanes F., Dudas S. E. (2020). Correction to human consumption of microplastics. Environ. Sci. Technol. 54, 10974. doi: 10.1021/acs.est.0c04032
Cózar A., Echevarría F., González-Gordillo J. I., Irigoien X., Ubeda B., Hernández-León S., et al. (2014). Plastic debris in the open ocean. Proc. Natl. Acad. Sci. U. S. A. 111, 10239–10244. doi: 10.1073/pnas.1314705111
da Costa Araújo A. P., de Andrade Vieira J. E., Malafaia G. (2020). Toxicity and trophic transfer of polyethylene microplastics from poecilia reticulata to danio rerio. Sci. Total Environ. 742, 140217. doi: 10.1016/j.scitotenv.2020.140217
Das S., Thiagarajan V., Chandrasekaran N., Ravindran B., Mukherjee A. (2022). Nanoplastics enhance the toxic effects of titanium dioxide nanoparticle in freshwater algae scenedesmus obliquus. Comp. Biochem. Physiol. C. Toxicol. Pharmacol. 256, 109305. doi: 10.1016/j.cbpc.2022.109305
Desforges J.-P. W., Galbraith M., Ross P. S. (2015). Ingestion of microplastics by zooplankton in the northeast pacific ocean. Arch. Environ. Contam. Toxicol. 69, 320–330. doi: 10.1007/s00244-015-0172-5
Diana Z., Vegh T., Karasik R., Bering J., Llano Caldas J. D., Pickle A., et al. (2022). The evolving global plastics policy landscape: An inventory and effectiveness review. Environ. Sci. Policy 134, 34–45. doi: 10.1016/j.envsci.2022.03.028
Diana Z. T., Karasik R., Merril GB., Morrison M., Corcoran KA., Vermeer D., et al. (2022). A transdisciplinary approach to reducing global plastic pollution. Frontiers in Marine Science. doi: 10.3389/fmars.2022.1032381
Dreier D. A., Mello D. F., Meyer J. N., Martyniuk C. J. (2019). Linking mitochondrial dysfunction to organismal and population health in the context of environmental pollutants: Progress and considerations for mitochondrial adverse outcome pathways. Environ. Toxicol. Chem. 38, 1625–1634. doi: 10.1002/etc.4453
Duncan E. M., Broderick A. C., Fuller W. J., Galloway T. S., Godfrey M. H., Hamann M., et al. (2019). Microplastic ingestion ubiquitous in marine turtles. Glob. Change Biol. 25, 744–752. doi: 10.1111/gcb.14519
Eriksen M., Lebreton L. C. M., Carson H. S., Thiel M., Moore C. J., Borerro J. C., et al. (2014). Plastic pollution in the world’s oceans: More than 5 trillion plastic pieces weighing over 250,000 tons afloat at Sea. PloS One 9, e111913. doi: 10.1371/journal.pone.0111913
Eriksen M., Maximenko N., Thiel M., Cummins A., Lattin G., Wilson S., et al. (2013). Plastic pollution in the south pacific subtropical gyre. Mar. pollut. Bull. 68, 71–76. doi: 10.1016/j.marpolbul.2012.12.021
Fatma Karaman E., Caglayan M., Sancar-Bas S., Ozal-Coskun C., Arda-Pirincci P., Ozden S. (2019). Global and region-specific post-transcriptional and post-translational modifications of bisphenol a in human prostate cancer cells. Environ. pollut. 255, 113318. doi: 10.1016/j.envpol.2019.113318
Folke C., Polasky S., Rockström J., Galaz V., Westley F., Lamont M., et al. (2021). Our future in the anthropocene biosphere. Ambio 50, 834–869. doi: 10.1007/s13280-021-01544-8
Food and Agriculture Organization (2014). The state of world fisheries and aquaculture 2014: opportunities and challenges (Rome, Italy: Food & Agriculture Organization of the United Nations (FAO).
Fuller S., Gautam A. (2016). A procedure for measuring microplastics using pressurized fluid extraction. Environ. Sci. Technol. 50, 5774–5780. doi: 10.1021/acs.est.6b00816
Galarpe V. R. K. (2015). Review on the impacts of waste disposal sites in the Philippines. Sci. Int. 29(2) , 379–385.
Gall S. C., Thompson R. C. (2015). The impact of debris on marine life. Mar. pollut. Bull. 92, 170–179. doi: 10.1016/j.marpolbul.2014.12.041
Gigault J., Halle A. T., Baudrimont M., Pascal P.-Y., Gauffre F., Phi T.-L., et al. (2018). Current opinion: What is a nanoplastic? Environ. pollut. 235, 1030–1034. doi: 10.1016/j.envpol.2018.01.024
Goldstein M. C., Rosenberg M., Cheng L. (2012). Increased oceanic microplastic debris enhances oviposition in an endemic pelagic insect. Biol. Lett. 8, 817–820. doi: 10.1098/rsbl.2012.0298
Gong H., Zhang X., Cheng B., Sun Y., Li C., Li T., et al. (2013). Bisphenol a accelerates toxic amyloid formation of human islet amyloid polypeptide: a possible link between bisphenol a exposure and type 2 diabetes. PloS One 8, e54198. doi: 10.1371/journal.pone.0054198
González N., Cunha S. C., Monteiro C., Fernandes J. O., Marquès M., Domingo J. L., et al. (2019). Quantification of eight bisphenol analogues in blood and urine samples of workers in a hazardous waste incinerator. Environ. Res. 176, 108576. doi: 10.1016/j.envres.2019.108576
González-Pleiter M., Edo C., Aguilera Á., Viúdez-Moreiras D., Pulido-Reyes G., González-Toril E., et al. (2021). Occurrence and transport of microplastics sampled within and above the planetary boundary layer. Sci. Total Environ. 761, 143213. doi: 10.1016/j.scitotenv.2020.143213
Groh K. J., Backhaus T., Carney-Almroth B., Geueke B., Inostroza P. A., Lennquist A., et al. (2019). Overview of known plastic packaging-associated chemicals and their hazards. Sci. Total Environ. 651, 3253–3268. doi: 10.1016/j.scitotenv.2018.10.015
Guerrera M. C., Aragona M., Porcino C., Fazio F., Laurà R., Levanti M., et al. (2021). Micro and nano plastics distribution in fish as model organisms: Histopathology, blood response and bioaccumulation in different organs. Appl. Sci. 11, 5768. doi: 10.3390/app11135768
Hahladakis J. N., Velis C. A., Weber R., Iacovidou E., Purnell P. (2018). An overview of chemical additives present in plastics: Migration, release, fate and environmental impact during their use, disposal and recycling. J. Hazard. Mater. 344, 179–199. doi: 10.1016/j.jhazmat.2017.10.014
Haq M. E. U., Akash M. S. H., Sabir S., Mahmood M. H., Rehman K. (2020). Human exposure to bisphenol a through dietary sources and development of diabetes mellitus: a cross-sectional study in Pakistani population. Environ. Sci. pollut. Res. Int. 27, 26262–26275. doi: 10.1007/s11356-020-09044-0
Hazen E. L., Abrahms B., Brodie S., Carroll G., Jacox M. G., Savoca M. S., et al. (2019). Marine top predators as climate and ecosystem sentinels. Front. Ecol. Environ. 17, 565–574. doi: 10.1002/fee.2125
Hernandez L. M., Xu E. G., Larsson H. C. E., Tahara R., Maisuria V. B., Tufenkji N. (2019). Plastic teabags release billions of microparticles and nanoparticles into tea. Environ. Sci. Technol. 53, 12300–12310. doi: 10.1021/acs.est.9b02540
Hoyo-Alvarez E., Arechavala-Lopez P., Jiménez-García M., Solomando A., Alomar C., Sureda A., et al. (2022). Effects of pollutants and microplastics ingestion on oxidative stress and monoaminergic activity of seabream brains. Aquat. Toxicol. 242, 106048. doi: 10.1016/j.aquatox.2021.106048
Huang B., Wei Z.-B., Yang L.-Y., Pan K., Miao A.-J. (2019). Combined toxicity of silver nanoparticles with hematite or plastic nanoparticles toward two freshwater algae. Environ. Sci. Technol. 53, 3871–3879. doi: 10.1021/acs.est.8b07001
Huang Z., Weng Y., Shen Q., Zhao Y., Jin Y. (2021). Microplastic: A potential threat to human and animal health by interfering with the intestinal barrier function and changing the intestinal microenvironment. Sci. Total Environ. 785, 147365. doi: 10.1016/j.scitotenv.2021.147365
Huntington H. P., Quakenbush L. T., Nelson M. (2016). Effects of changing sea ice on marine mammals and subsistence hunters in northern Alaska from traditional knowledge interviews. Biol. Lett. 12, 20160198. doi: 10.1098/rsbl.2016.0198
Hu M., Palić D. (2020). Micro- and nano-plastics activation of oxidative and inflammatory adverse outcome pathways. Redox Biol. 37, 101620. doi: 10.1016/j.redox.2020.101620
Hutter H.-P., Kundi M., Hohenblum P., Scharf S., Shelton J. F., Piegler K., et al. (2016). Life without plastic: A family experiment and biomonitoring study. Environ. Res. 150, 639–644. doi: 10.1016/j.envres.2016.05.028
Hutter H.-P., Moshammer H., Wallner P., Damberger B., Tappler P., Kundi M. (2006). Health complaints and annoyances after moving into a new office building: a multidisciplinary approach including analysis of questionnaires, air and house dust samples. Int. J. Hyg. Environ. Health 209, 65–68. doi: 10.1016/j.ijheh.2005.08.010
Ibrahim Y. S., Tuan Anuar S., Azmi A. A., Wan Mohd Khalik W. M. A., Lehata S., Hamzah S. R., et al. (2021). Detection of microplastics in human colectomy specimens. JGH Open 5, 116–121. doi: 10.1002/jgh3.12457
International Organization for Migration and International Labour Organization (2021). Powering past the pandemic: Bolstering tuvalu’s socioeconomic resilience in a covid-19 world. Available at: https://publications.iom.int/books/powering-past-pandemic-bolstering-tuvalus-socioeconomic-resilience-covid-19-world
Jambeck J. R., Geyer R., Wilcox C., Siegler T. R., Perryman M., Andrady A., et al. (2015). Marine pollution. plastic waste inputs from land into the ocean. Science 347, 768–771. doi: 10.1126/science.1260352
Jiang J., Chen Y., Yu R., Zhao X., Wang Q., Cai L. (2016). Pretilachlor has the potential to induce endocrine disruption, oxidative stress, apoptosis and immunotoxicity during zebrafish embryo development. Environ. Toxicol. Pharmacol. 42, 125–134. doi: 10.1016/j.etap.2016.01.006
Jin Y., Xia J., Pan Z., Yang J., Wang W., Fu Z. (2018). Polystyrene microplastics induce microbiota dysbiosis and inflammation in the gut of adult zebrafish. Environ. pollut. 235, 322–329. doi: 10.1016/j.envpol.2017.12.088
Junge K. M., Leppert B., Jahreis S., Wissenbach D. K., Feltens R., Grützmann K., et al. (2018). Mediates the impact of prenatal bisphenol a exposure on long-term body weight development. Clin. Epigenet. 10, 58. doi: 10.1186/s13148-018-0478-z
Kankanige D., Babel S. (2020). Smaller-sized micro-plastics (MPs) contamination in single-use PET-bottled water in Thailand. Sci. Total Environ. 717, 137232. doi: 10.1016/j.scitotenv.2020.137232
Karami A., Romano N., Galloway T., Hamzah H. (2016). Virgin microplastics cause toxicity and modulate the impacts of phenanthrene on biomarker responses in African catfish (Clarias gariepinus). Environmental Research 151, 58–70. doi: 10.1016/j.envres.2016.07.024
Karami A., Golieskardi A., Ho Y. B., Larat V., Salamatinia B. (2017). Microplastics in eviscerated flesh and excised organs of dried fish. Sci. Rep. 7, 1–9. doi: 10.1038/s41598-017-05828-6
Karasik R., Vegh T., Diana Z., Bering J., Caldas J., Pickle A., et al. (2020) 20 years of government responses to the global plastic pollution problem: The plastics policy inventory. Available at: https://nicholasinstitute.duke.edu/sites/default/files/publications/20-Years-of-Government-Responses-to-the-Global-Plastic-Pollution-Problem-New_1.pdf.
Karbalaei S., Hanachi P., Walker T. R., Cole M. (2018). Occurrence, sources, human health impacts and mitigation of microplastic pollution. Environ. Sci. pollut. Res. Int. 25, 36046–36063. doi: 10.1007/s11356-018-3508-7
Kasirajan S., Ngouajio M. (2012). Polyethylene and biodegradable mulches for agricultural applications: a review. Agron. Sustain. Dev. 32, 501–529. doi: 10.1007/s13593-011-0068-3
Kim L., Cui R., Kwak J. I., An Y.-J. (2022). Sub-Acute exposure to nanoplastics via two-chain trophic transfer: From brine shrimp artemia franciscana to small yellow croaker larimichthys polyactis. Mar. pollut. Bull. 175, 113314. doi: 10.1016/j.marpolbul.2021.113314
Kim J. H., Kim S. H. (2020). Exposure to phthalate esters and the risk of endometriosis. Dev. Reprod. 24, 71–78. doi: 10.12717/DR.2020.24.2.71
Kirchnawy C., Hager F., Osorio Piniella V., Jeschko M., Washüttl M., Mertl J., et al. (2020). Potential endocrine disrupting properties of toys for babies and infants. PloS One 15, e0231171. doi: 10.1371/journal.pone.0231171
Koelmans A. A., Bakir A., Burton G. A., Janssen C. R. (2016). Microplastic as a vector for chemicals in the aquatic environment: Critical review and model-supported reinterpretation of empirical studies. Environ. Sci. Technol. 50, 3315–3326. doi: 10.1021/acs.est.5b06069
Kosuth M., Mason S. A., Wattenberg E. V. (2018). Anthropogenic contamination of tap water, beer, and sea salt. PloS One 13, e0194970. doi: 10.1371/journal.pone.0194970
Kwon B. G., Saido K., Koizumi K., Sato H., Ogawa N., Chung S.-Y., et al. (2014). Regional distribution of styrene analogues generated from polystyrene degradation along the coastlines of the north-East pacific ocean and Hawaii. Environ. pollut. 188, 45–49. doi: 10.1016/j.envpol.2014.01.019
Kwon J. A., Shin B., Kim B. (2020). Urinary bisphenol a and thyroid function by BMI in the Korean national environmental health survey (KoNEHS) 2012-2014. Chemosphere 240, 124918. doi: 10.1016/j.chemosphere.2019.124918
Lang I. A., Galloway T. S., Scarlett A., Henley W. E., Depledge M., Wallace R. B., et al. (2008). Association of urinary bisphenol a concentration with medical disorders and laboratory abnormalities in adults. JAMA 300, 1303–1310. doi: 10.1001/jama.300.11.1303
Latini G., De Felice C., Presta G., Del Vecchio A., Paris I., Ruggieri F., et al. (2003). In utero exposure to di-(2-ethylhexyl)phthalate and duration of human pregnancy. Environ. Health Perspect. 111, 1783–1785. doi: 10.1289/ehp.6202
Lau W. W. Y., Shiran Y., Bailey R. M., Cook E., Stuchtey M. R., Koskella J., et al. (2020). Evaluating scenarios toward zero plastic pollution. Science 369, 1455–1461. doi: 10.1126/science.aba9475
Law K. L. (2017). Plastics in the marine environment. Ann. Rev. Mar. Sci. 9, 205–229. doi: 10.1146/annurev-marine-010816-060409
Law K. L., Starr N., Siegler T. R., Jambeck J. R., Mallos N. J., Leonard G. H. (2020). The united states’ contribution of plastic waste to land and ocean. Sci. Adv. 6, eabd0288. doi: 10.1126/sciadv.abd0288
Leslie H. A., van Velzen M. J. M., Brandsma S. H., Vethaak A. D., Garcia-Vallejo J. J., Lamoree M. H. (2022). Discovery and quantification of plastic particle pollution in human blood. Environ. Int. 163, 107199. doi: 10.1016/j.envint.2022.107199
Liebezeit G., Liebezeit E. (2013). Non-pollen particulates in honey and sugar. Food Addit. Contam. Part A Chem. Anal. Control Expo. Risk Assess. 30, 2136–2140. doi: 10.1080/19440049.2013.843025
Liebezeit G., Liebezeit E. (2015). Origin of synthetic particles in honeys. Pol. J. Food Nutr. Sci. 65, 143–147. doi: 10.1515/pjfns-2015-0025
Liu Z.-H., Yin H., Dang Z. (2017). Do estrogenic compounds in drinking water migrating from plastic pipe distribution system pose adverse effects to human? an analysis of scientific literature. Environ. Sci. pollut. Res. Int. 24, 2126–2134. doi: 10.1007/s11356-016-8032-z
Li J., Yang D., Li L., Jabeen K., Shi H. (2015). Microplastics in commercial bivalves from China. Environ. pollut. 207, 190–195. doi: 10.1016/j.envpol.2015.09.018
Lombó M., Fernández-Díez C., González-Rojo S., Navarro C., Robles V., Herráez M. P. (2015). Transgenerational inheritance of heart disorders caused by paternal bisphenol a exposure. Environ. pollut. 206, 667–678. doi: 10.1016/j.envpol.2015.08.016
Lu H., Diaz D. J., Czarnecki N. J., Zhu C., Kim W., Shroff R., et al. (2022). Machine learning-aided engineering of hydrolases for PET depolymerization. Nature 604, 662–667. doi: 10.1038/s41586-022-04599-z
Machtinger R., Orvieto R. (2014). Bisphenol a, oocyte maturation, implantation, and IVF outcome: review of animal and human data. Reprod. Biomed. Online 29, 404–410. doi: 10.1016/j.rbmo.2014.06.013
Manikkam M., Tracey R., Guerrero-Bosagna C., Skinner M. K. (2013). Plastics derived endocrine disruptors (BPA, DEHP and DBP) induce epigenetic transgenerational inheritance of obesity, reproductive disease and sperm epimutations. PloS One 8, e55387. doi: 10.1371/journal.pone.0055387
Mariana M., Cairrao E. (2020). Phthalates implications in the cardiovascular system. J. Cardiovasc. Dev. Dis. 7. doi: 10.3390/jcdd7030026
Mattsson K., Johnson E. V., Malmendal A., Linse S., Hansson L.-A., Cedervall T. (2017). Brain damage and behavioural disorders in fish induced by plastic nanoparticles delivered through the food chain. Sci. Rep. 7, 11452. doi: 10.1038/s41598-017-10813-0
Meeker J. D., Sathyanarayana S., Swan S. H. (2009). Phthalates and other additives in plastics: human exposure and associated health outcomes. Philos. Trans. R. Soc Lond. B Biol. Sci. 364, 2097–2113. doi: 10.1098/rstb.2008.0268
Muncke J., Andersson A.-M., Backhaus T., Boucher J. M., Carney Almroth B., Castillo A., et al. (2020). Impacts of food contact chemicals on human health: a consensus statement. Environ. Health 19, 25. doi: 10.1186/s12940-020-0572-5
Nadeem A., Ahmad S. F., Al-Harbi N. O., Attia S. M., Bakheet S. A., Alsanea S., et al. (2021). Aggravation of autism-like behavior in BTBR T+tf/J mice by environmental pollutant, di-(2-ethylhexyl) phthalate: Role of nuclear factor erythroid 2-related factor 2 and oxidative enzymes in innate immune cells and cerebellum. Int. Immunopharmacol. 91, 107323. doi: 10.1016/j.intimp.2020.107323
Nam S.-H., Lee J., An Y.-J. (2022). Towards understanding the impact of plastics on freshwater and marine microalgae: A review of the mechanisms and toxicity endpoints. J. Hazard. Mater. 423, 127174. doi: 10.1016/j.jhazmat.2021.127174
Ncube L. K., Ude A. U., Ogunmuyiwa E. N., Zulkifli R., Beas I. N. (2021). An overview of plastic waste generation and management in food packaging industries. Recycl. Today 6, 12. doi: 10.3390/recycling6010012
Nelms S. E., Barnett J., Brownlow A., Davison N. J., Deaville R., Galloway T. S., et al. (2019). Microplastics in marine mammals stranded around the British coast: ubiquitous but transitory? Sci. Rep. 9, 1075. doi: 10.1038/s41598-018-37428-3
Ocean Studies Board, Division on Earth and Life Studies, National Academies of Sciences Engineering and Medicine, Committee On The Assessment Of The Cumulative Effects Of Anthropogenic Stressors On Marine Mammals (2017). Approaches to understanding the cumulative effects of stressors on marine mammals (Washington, D.C., DC: National Academies Press).
Oliveira P., Barboza L. G. A., Branco V., Figueiredo N., Carvalho C., Guilhermino L. (2018). Effects of microplastics and mercury in the freshwater bivalve corbicula fluminea (Müller 1774): Filtration rate, biochemical biomarkers and mercury bioconcentration. Ecotoxicol. Environ. Saf. 164, 155–163. doi: 10.1016/j.ecoenv.2018.07.062
One Health (2021) OIE - world organisation for animal health. Available at: https://www.oie.int/en/what-we-do/global-initiatives/one-health/ (Accessed May 13, 2022).
One Health (2022). Available at: https://www.cdc.gov/onehealth/index.html (Accessed May 13, 2022).
Paul-Pont I., Lacroix C., González Fernández C., Hégaret H., Lambert C., Le Goïc N., et al. (2016). Exposure of marine mussels mytilus spp. to polystyrene microplastics: Toxicity and influence on fluoranthene bioaccumulation. Environ. pollut. 216, 724–737. doi: 10.1016/j.envpol.2016.06.039
Pitt J. A., Kozal J. S., Jayasundara N., Massarsky A., Trevisan R., Geitner N., et al. (2018a). Uptake, tissue distribution, and toxicity of polystyrene nanoparticles in developing zebrafish (Danio rerio). Aquat. Toxicol. 194, 185–194. doi: 10.1016/j.aquatox.2017.11.017
Pitt J. A., Trevisan R., Massarsky A., Kozal J. S., Levin E. D., Di Giulio R. T. (2018b). Maternal transfer of nanoplastics to offspring in zebrafish (Danio rerio): A case study with nanopolystyrene. Sci. Total Environ. 643, 324–334. doi: 10.1016/j.scitotenv.2018.06.186
Prinz N., Korez Š. (2020). “Understanding how microplastics affect marine biota on the cellular level is important for assessing ecosystem function: A review,” in YOUMARES 9 - the oceans: Our research, our future (Cham: Springer International Publishing), 101–120.
Ragusa A., Svelato A., Santacroce C., Catalano P., Notarstefano V., Carnevali O., et al. (2021). Plasticenta: First evidence of microplastics in human placenta. Environ. Int. 146, 106274. doi: 10.1016/j.envint.2020.106274
Rangasamy B., Malafaia G., Maheswaran R. (2022). Evaluation of antioxidant response and Na-K-ATPase activity in zebrafish exposed to polyethylene microplastics: Shedding light on a physiological adaptation. J. Hazard. Mater. 426, 127789. doi: 10.1016/j.jhazmat.2021.127789
Rasool F. N., Saavedra M. A., Pamba S., Perold V., Mmochi A. J., Maalim M., et al. (2021). Isolation and characterization of human pathogenic multidrug resistant bacteria associated with plastic litter collected in Zanzibar. J. Hazard. Mater. 405, 124591. doi: 10.1016/j.jhazmat.2020.124591
Richards Z. T., Beger M. (2011). A quantification of the standing stock of macro-debris in Majuro lagoon and its effect on hard coral communities. Mar. pollut. Bull. 62, 1693–1701. doi: 10.1016/j.marpolbul.2011.06.003
Roch S., Friedrich C., Brinker A. (2020). Uptake routes of microplastics in fishes: practical and theoretical approaches to test existing theories. Sci. Rep. 10, 3896. doi: 10.1038/s41598-020-60630-1
Rochman C. M., Hentschel B. T., Teh S. J. (2014). Long-term sorption of metals is similar among plastic types: implications for plastic debris in aquatic environments. PloS One 9, e85433. doi: 10.1371/journal.pone.0085433
Rochman C. M., Tahir A., Williams S. L., Baxa D. V., Lam R., Miller J. T., et al. (2015). Anthropogenic debris in seafood: Plastic debris and fibers from textiles in fish and bivalves sold for human consumption. Sci. Rep. 5, 14340. doi: 10.1038/srep14340
Roman J., Nevins J., Altabet M., Koopman H., McCarthy J. (2016). Endangered right whales enhance primary productivity in the bay of fundy. PloS One 11, e0156553. doi: 10.1371/journal.pone.0156553
Rossi G., Barnoud J., Monticelli L. (2014). Polystyrene nanoparticles perturb lipid membranes. J. Phys. Chem. Lett. 5, 241–246. doi: 10.1021/jz402234c
Sarasamma S., Audira G., Siregar P., Malhotra N., Lai Y.-H., Liang S.-T., et al. (2020). Nanoplastics cause neurobehavioral impairments, reproductive and oxidative damages, and biomarker responses in zebrafish: Throwing up alarms of wide spread health risk of exposure. Int. J. Mol. Sci. 21, 1410. doi: 10.3390/ijms21041410
Schmaltz E., Melvin E. C., Diana Z., Gunady E. F., Rittschof D., Somarelli J. A., et al. (2020). Plastic pollution solutions: emerging technologies to prevent and collectmarineplastic pollution. Environ. Int. 144, 106067. doi: 10.1016/j.envint.2020.106067
Schwabl P., Köppel S., Königshofer P., Bucsics T., Trauner M., Reiberger T., et al. (2019). Detection of various microplastics in human stool: A prospective case series. Ann. Intern. Med. 171, 453–457. doi: 10.7326/M19-0618
Senathirajah K., Attwood S., Bhagwat G., Carbery M., Wilson S., Palanisami T. (2021). Estimation of the mass of microplastics ingested - a pivotal first step towards human health risk assessment. J. Hazard. Mater. 404, 124004. doi: 10.1016/j.jhazmat.2020.124004
Senyildiz M., Karaman E. F., Bas S. S., Pirincci P. A., Ozden S. (2017). Effects of BPA on global DNA methylation and global histone 3 lysine modifications in SH-SY5Y cells: An epigenetic mechanism linking the regulation of chromatin modifiying genes. Toxicol. In Vitro 44, 313–321. doi: 10.1016/j.tiv.2017.07.028
Seoane M., González-Fernández C., Soudant P., Huvet A., Esperanza M., Cid Á., et al. (2019). Polystyrene microbeads modulate the energy metabolism of the marine diatom chaetoceros neogracile. Environ. pollut. 251, 363–371. doi: 10.1016/j.envpol.2019.04.142
Sheth M. U., Kwartler S. K., Schmaltz E. R., Hoskinson S. M., Martz E. J., Dunphy-Daly M. M., et al. (2019). Bioengineering a future free of marine plastic waste. Front. Mar. Sci. 6. doi: 10.3389/fmars.2019.00624
Silva M. S. S., Oliveira M., Valente P., Figueira E., Martins M., Pires A. (2020). Behavior and biochemical responses of the polychaeta hediste diversicolor to polystyrene nanoplastics. Sci. Total Environ. 707, 134434. doi: 10.1016/j.scitotenv.2019.134434
Sobhani Z., Lei Y., Tang Y., Wu L., Zhang X., Naidu R., et al. (2020). Microplastics generated when opening plastic packaging. Sci. Rep. 10, 1–7. doi: 10.1038/s41598-020-61146-4
Solleiro-Villavicencio H., Gomez-De León C. T., Del Río-Araiza V. H., Morales-Montor J. (2020). The detrimental effect of microplastics on critical periods of development in the neuroendocrine system. Birth Defects Res. 112, 1326–1340. doi: 10.1002/bdr2.1776
Steffen W., Richardson K., Rockström J., Cornell S. E., Fetzer I., Bennett E. M., et al. (2015). Sustainability. planetary boundaries: guiding human development on a changing planet. Science 347, 1259855. doi: 10.1126/science.1259855
Su L., Cai H., Kolandhasamy P., Wu C., Rochman C. M., Shi H. (2018). Using the Asian clam as an indicator of microplastic pollution in freshwater ecosystems. Environ. pollut. 234, 347–355. doi: 10.1016/j.envpol.2017.11.075
Summers J. K., Smith L. M., Case J. L., Linthurst R. A. (2012). A review of the elements of human well-being with an emphasis on the contribution of ecosystem services. Ambio 41, 327–340. doi: 10.1007/s13280-012-0256-7
Sussarellu R., Suquet M., Thomas Y., Lambert C., Fabioux C., Pernet M. E. J., et al. (2016). Oyster reproduction is affected by exposure to polystyrene microplastics. Proc. Natl. Acad. Sci. U. S. A. 113, 2430–2435. doi: 10.1073/pnas.1519019113
Tao S., Zhang Y., Yuan C., Gao J., Wu F., Wang Z. (2016). Oxidative stress and immunotoxic effects of bisphenol a on the larvae of rare minnow gobiocypris rarus. Ecotoxicol. Environ. Saf. 124, 377–385. doi: 10.1016/j.ecoenv.2015.11.014
Taylor S. F. W., Roberts M. J., Milligan B., Ncwadi R. (2019). Measurement and implications of marine food security in the Western Indian ocean: an impending crisis? Food Secur. 11, 1395–1415. doi: 10.1007/s12571-019-00971-6
Thompson R. C., Moore C. J., vom Saal F. S., Swan S. H. (2009). Plastics, the environment and human health: current consensus and future trends. Philos. Trans. R. Soc Lond. B Biol. Sci. 364, 2153–2166. doi: 10.1098/rstb.2009.0053
Tournier V., Topham C. M., Gilles A., David B., Folgoas C., Moya-Leclair E., et al. (2020). An engineered PET depolymerase to break down and recycle plastic bottles. Nature 580, 216–219. doi: 10.1038/s41586-020-2149-4
Trevisan R., Ranasinghe P., Jayasundara N., Di Giulio R. T. (2022). Nanoplastics in aquatic environments: Impacts on aquatic species and interactions with environmental factors and pollutants. Toxics 10, 326. doi: 10.3390/toxics10060326
Trevisan R., Uzochukwu D., Di Giulio R. T. (2020). PAH sorption to nanoplastics and the trojan horse effect as drivers of mitochondrial toxicity and PAH localization in zebrafish. Front. Environ. Sci. Eng. China 8. doi: 10.3389/fenvs.2020.00078
Trevisan R., Voy C., Chen S., Di Giulio R. T. (2019). Nanoplastics decrease the toxicity of a complex PAH mixture but impair mitochondrial energy production in developing zebrafish. Environ. Sci. Technol. 53, 8405–8415. doi: 10.1021/acs.est.9b02003
Van Cauwenberghe L., Janssen C. R. (2014). Microplastics in bivalves cultured for human consumption. Environ. pollut. 193, 65–70. doi: 10.1016/j.envpol.2014.06.010
von Moos N., Burkhardt-Holm P., Köhler A. (2012). Uptake and effects of microplastics on cells and tissue of the blue mussel mytilus edulis l. after an experimental exposure. Environ. Sci. Technol. 46, 11327–11335. doi: 10.1021/es302332w
Wagner M., Oehlmann J. (2011). Endocrine disruptors in bottled mineral water: estrogenic activity in the e-screen. J. Steroid Biochem. Mol. Biol. 127, 128–135. doi: 10.1016/j.jsbmb.2010.10.007
Waite H. R., Donnelly M. J., Walters L. J. (2018). Quantity and types of microplastics in the organic tissues of the eastern oyster crassostrea virginica and Atlantic mud crab panopeus herbstii from a Florida estuary. Mar. pollut. Bull. 129, 179–185. doi: 10.1016/j.marpolbul.2018.02.026
Walker I., Montaño M. D., Lankone R. S., Fairbrother D. H., Ferguson P. L. (2021). Influence of CNT loading and environmental stressors on leaching of polymer-associated chemicals from epoxy and polycarbonate nanocomposites. Environ. Chem. 18, 131. doi: 10.1071/EN21043
Wang Q., Bai J., Ning B., Fan L., Sun T., Fang Y., et al. (2020). Effects of bisphenol a and nanoscale and microscale polystyrene plastic exposure on particle uptake and toxicity in human caco-2 cells. Chemosphere 254, 126788. doi: 10.1016/j.chemosphere.2020.126788
Wang C., Gao W., Liang Y., Jiang Y., Wang Y., Zhang Q., et al. (2019). Migration of chlorinated paraffins from plastic food packaging into food simulants: Concentrations and differences in congener profiles. Chemosphere 225, 557–564. doi: 10.1016/j.chemosphere.2019.03.039
Wang C., Hou M., Shang K., Wang H., Wang J. (2022). Microplastics (Polystyrene) exposure induces metabolic changes in the liver of rare minnow (). Molecules 27, 584. doi: 10.3390/molecules27030584
Wang H., Zhao P., Huang Q., Chi Y., Dong S., Fan J. (2019). Bisphenol-a induces neurodegeneration through disturbance of intracellular calcium homeostasis in human embryonic stem cells-derived cortical neurons. Chemosphere 229, 618–630.
Wang Y., Mao Z., Zhang M., Ding G., Sun J., Du M. (2019c). The uptake and elimination of polystyrene microplastics by the brine shrimp, Artemia parthenogenetica, and its impact on its feeding behavior and intestinal histology. Chemosphere 234, 123–131. doi: 10.1016/j.chemosphere.2019.05.267
Wen B., Zhang N., Jin S.-R., Chen Z.-Z., Gao J.-Z., Liu Y., et al. (2018). Microplastics have a more profound impact than elevated temperatures on the predatory performance, digestion and energy metabolism of an Amazonian cichlid. Aquat. Toxicol. 195, 67–76. doi: 10.1016/j.aquatox.2017.12.010
Wiesinger H., Wang Z., Hellweg S. (2021). Deep dive into plastic monomers, additives, and processing aids. Environ. Sci. Technol. 55, 9339–9351. doi: 10.1021/acs.est.1c00976
Wilcox C., Van Sebille E., Hardesty B. D. (2015). Threat of plastic pollution to seabirds is global, pervasive, and increasing. Proc. Natl. Acad. Sci. U. S. A. 112, 11899–11904. doi: 10.1073/pnas.1502108112
World Health Organization (2022) Dietary and inhalation exposure to nano- and microplastic particles and potential implications for human health. Available at: https://www.who.int/publications/i/item/9789240054608.
Wright S. L., Kelly F. J. (2017). Plastic and human health: A micro issue? Environ. Sci. Technol. 51, 6634–6647. doi: 10.1021/acs.est.7b00423
Yang D., Shi H., Li L., Li J., Jabeen K., Kolandhasamy P. (2015). Microplastic pollution in table salts from China. Environ. Sci. Technol. 49, 13622–13627. doi: 10.1021/acs.est.5b03163
Yong C. Q. Y., Valiyaveettil S., Tang B. L. (2020). Toxicity of microplastics and nanoplastics in mammalian systems. Int. J. Environ. Res. Public Health 17, 1509. doi: 10.3390/ijerph17051509
Zantis L. J., Bosker T., Lawler F., Nelms S. E., O’Rorke R., Constantine R., et al. (2022). Assessing microplastic exposure of large marine filter-feeders. Sci. Total Environ. 818, 151815. doi: 10.1016/j.scitotenv.2021.151815
Zantis L. J., Carroll E. L., Nelms S. E., Bosker T. (2021). Marine mammals and microplastics: A systematic review and call for standardisation. Environ. pollut. 269, 116142. doi: 10.1016/j.envpol.2020.116142
Zarus G. M., Muianga C., Hunter C. M., Pappas R. S. (2021). A review of data for quantifying human exposures to micro and nanoplastics and potential health risks. Sci. Total Environ. 756, 144010. doi: 10.1016/j.scitotenv.2020.144010
Zhang Q., Qu Q., Lu T., Ke M., Zhu Y., Zhang M., et al. (2018). The combined toxicity effect of nanoplastics and glyphosate on microcystis aeruginosa growth. Environ. pollut. 243, 1106–1112. doi: 10.1016/j.envpol.2018.09.073
Zhao X., Liu Z., Ren X., Duan X. (2021). Parental transfer of nanopolystyrene-enhanced tris(1,3-dichloro-2-propyl) phosphate induces transgenerational thyroid disruption in zebrafish. Aquat. Toxicol. 236, 105871. doi: 10.1016/j.aquatox.2021.105871
Keywords: microplastic toxicity, marine plastic, ecosystem health and pollution, human health, nanoplastic (NP)
Citation: Morrison M, Trevisan R, Ranasinghe P, Merrill GB, Santos J, Hong A, Edward WC, Jayasundara N and Somarelli JA (2022) A growing crisis for One Health: Impacts of plastic pollution across layers of biological function. Front. Mar. Sci. 9:980705. doi: 10.3389/fmars.2022.980705
Received: 28 June 2022; Accepted: 10 October 2022;
Published: 02 November 2022.
Edited by:
Suresh Valiyaveettil, National University of Singapore, SingaporeReviewed by:
Camilo Dias Seabra Pereira, Federal University of São Paulo, BrazilPolina Lemenkova, Université libre de Bruxelles, Belgium
Copyright © 2022 Morrison, Trevisan, Ranasinghe, Merrill, Santos, Hong, Edward, Jayasundara and Somarelli. This is an open-access article distributed under the terms of the Creative Commons Attribution License (CC BY). The use, distribution or reproduction in other forums is permitted, provided the original author(s) and the copyright owner(s) are credited and that the original publication in this journal is cited, in accordance with accepted academic practice. No use, distribution or reproduction is permitted which does not comply with these terms.
*Correspondence: Nishad Jayasundara, nishad.jayasundara@duke.edu; Jason A. Somarelli, jason.somarelli@duke.edu