- 1Pacific Biological Station, Fisheries and Oceans Canada, Nanaimo, BC, Canada
- 2Parks Canada Agency, Pacific Rim National Park Reserve of Canada, Ucluelet, BC, Canada
- 3Fisheries and Oceans Canada, Institute of Ocean Sciences, Sidney, BC, Canada
The main objective of this study was to ascertain if the 2014 to 2016 spring marine heatwaves, had a significant effect on early summer fish assemblage species composition, abundance and biomass in eelgrass meadows. It was presumed that increased water temperatures above the 90th percentile of the 30y long-term spring temperatures would result in changes to fish growth, survival and ultimately lead to changes in early summer settlement to eelgrass. We assessed this by analyzing the catch per unit effort of 18 common fish species and total fish assemblage biomass in 22 eelgrass meadows from two study areas in the northern California Current during the early summer over an 18 year period from 2004 to 2021. A control chart analysis indicated that eelgrass fish assemblages during heatwave years (2015-16) were significantly higher than the 90th percentile in Bray Curtis dissimilarity of 13 baseline years. Furthermore, analysis of similarity indicated that about one half of the 18 fish species showed variable contributing increases or decreases in catch per unit effort during the heatwave period. In addition, the same fish families from the two study areas only 100km apart did not respond in synchrony to the MHW. We could not however detect any changes in eelgrass fish species composition or assemblage level biomass during the heatwave years. An analysis of the temporal trajectory over the 17y time series in the ordination plots also revealed that changes in fish assemblage dissimilarity during the 2015 and 2016 heatwave returned quickly to levels observed during baseline. Altogether, the results of this study suggest that while the short-term (34-42 d) spring heatwave events resulted in changes fish species abundances, these changes were short-lived. Hence, our results warrant caution about generalizing about the effects of heatwaves on nearshore marine fish.
Introduction
Anomalously warm ocean conditions occurred on the continental shelf in the California Current region from central British Columbia to southern California between 2014 to 2016 (Di Lorenzo and Mantua, 2016; Sanford et al., 2019; Walker et al., 2020). The large mass of anomalously warm offshore water extended into nearshore coastal waters and was due to relaxed winter mixing (Bond et al., 2015). These events have been described as marine heatwaves (MHW) and can be defined as “a discrete prolonged anomalously warm water event in a particular location” (Hobday et al., 2016). Climate modes such as El Niño can exacerbate ocean warming events, and peak MHW conditions observed during 2014-16 coincided with the strong El Niño of 2015-16 (Di Lorenzo and Mantua, 2016).
Episodic and longer-term coastal warming events in the northern California Current region have been linked to specific changes in coastal ecosystems along the continental shelf. Ocean warming has resulted in increased abundance, and change in the timing of arrival, of seasonally migratory fishes such as Sardinops sagax (McFarlane and Beamish, 1981) and Merlucicius productus in the northern California Current (Mcfarlane et al., 1997). At the base of the continental shelf food-web, variability in phytoplankton species blooms (Robinson et al., 2004) and latitudinal shifts in zooplankton communities and production have also been observed (Mackas et al., 2007). More recently, Nielsen et al. (2020) found that the most dominant larval fish taxa were at an all-time low during the 2015-2016 MHW off the northern California Current.
Taken together, the well-documented changes observed in pelagic ecosystems of the northern California Current indicate that MHWs can have rapid and significant effects on continental shelf food webs. Less clear however, is how acute periods of extended coastal higher water temperatures might directly or indirectly affect nearshore ecosystems. Eelgrass meadows (Zostera marina) are one nearshore ecosystem well-known as nursery habitat for young-of-the-year and juvenile stages of more than 70 coastal fish species each spring and summer (Robinson et al., 2011; Robinson and Yakimishyn, 2013).
The survival of pelagic larval phases of many nearshore fishes in later winter and early spring can be enhanced during warmer oceanic conditions before settlement to nursery habitats (Auth et al., 2018). Higher water temperatures can increase growth and result in reduced duration of the larval phase, and a rapid increase in fish size, among other factors, and these changes can ultimately translate into higher larval fish survival rates weeks or months before settlement into nearshore nursery habitats (Rooper et al., 2006). Increased water temperatures would not only have a direct effect of increasing larval growth and therefore contributing to larval survival, but may also be indicative of enhanced physical transport and plankton productivity of nursery habitats (Jenkins and King, 2006).
In this study, we examined the response of resident nearshore fish species in eelgrass habitats to anomalous acute ocean warming events. It was hypothesized that substantially warmer spring ocean conditions would result in significant differences in early summer settled juvenile fish species compared to years with little or no warming in spring. To address this hypothesis, we assessed fish assemblages CPUEs and biomass of 18 common fish species caught by beach seine in 22 eelgrass meadows in early summer from 2004 to 2021 located in two estuaries along the south western coast of Vancouver Island, British Columbia.
Materials and methods
Study region
The south west coast of Vancouver Island (WCVI) is located within the northern portion of the California Current system (Figure 1) and in winter is dominated by southeasterly winds and onshore movement of offshore waters. A Pacific basin-wide transition in atmospheric systems begins between March and June of each year, and results in the prevalence of northwesterly winds blowing nearshore surface waters offshore. These surface waters are replaced by cooler, nutrient rich deeper shelf/slope water, or coastal upwelling (Thomson, 1981).
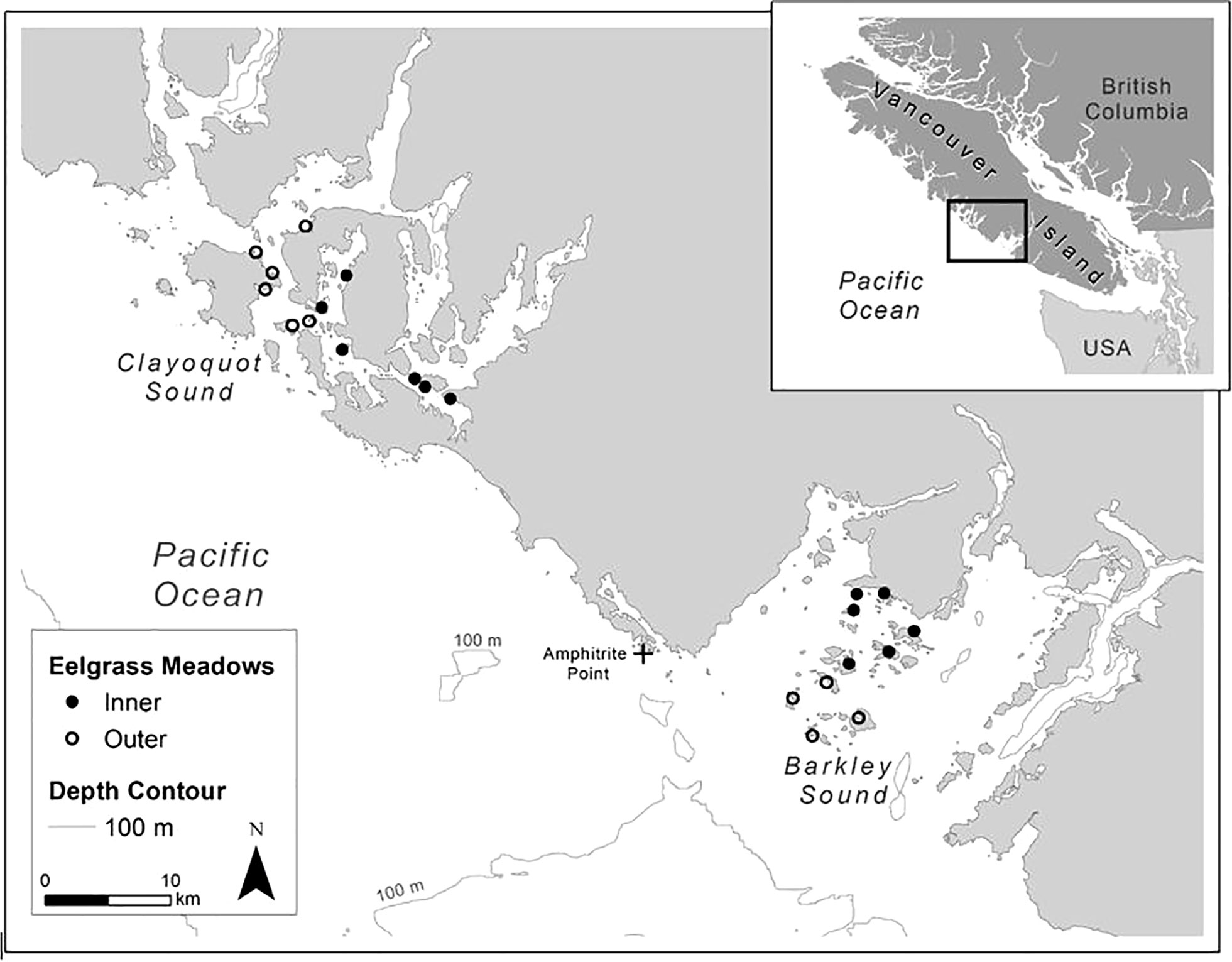
Figure 1 Eelgrass meadows located in inner and outer regions of the two study areas, Clayoquot Sound and Barkley Sound on the south west coast of Vancouver Island, British Columbia, Canada.
Along the WCVI, Barkley Sound is a broad and open embayment that is oceanographically connected to the outer continental shelf; Oceanic waters flow intermittingly into Barkley Sound during winter and spring and have a strong influence on local environmental conditions (Pawlowicz, 2017). In contrast, a second embayment, Clayoquot Sound about 100 km to the north of Barkley Sound, consists of Tofino Inlet whereby the oceanographic properties of the inland portion of Clayoquot Sound are more strongly influenced in spring by freshwater input from the lower Kennedy River rather than by continental shelf marine conditions (Thomson, 1981). Because the local water temperature and salinity conditions differ between Clayoquot and Barkley Sounds, and within each study area depending on marine and freshwater influences, meadows were assigned to belong to either inner of outer subregions of each sound and assessed separately. Fish assemblage data from 6 Clayoquot Sound eelgrass meadows were included in the inner meadow subregion, 6 Clayoquot Sound eelgrass meadows in the outer meadow subregion, while 6 Barkley Sound eelgrass meadows were included in the inner meadow subregion, and 4 Barkley Sound meadows were included in the outer subregion (refer to Figure 1).
Marine heatwaves
The ocean has high heat capacity and its time and space correlation scales are large, and hence temperature measurements at the pre-settlement eelgrass habitats can be less frequently estimated over larger areas (Neuheimer and Taggart, 2007). Long-term daily sea surface temperature (SST) data for Amphitrite Point lighthouse (Figure 1) maintained by Fisheries and Oceans Canada (http://www.pac.dfo-mpo.gc.ca/science/oceans/data-donnees/lighthouses-phares/index-eng.html) were used to describe regional ocean thermal conditions in spring (March to May) prior to settlement in Clayoquot Sound and Barkley Sound eelgrass in early summer.
To characterize the extent of anomalously warm oceanic spring conditions, we used the marine heatwave (MHW) assessment methods discussed by Hobday et al. (2018); Hobday et al. (2016), and implemented via the R package ‘heatwaveR’ (https://rdocumentation.org/packages/heatwaveR/versions/0.4.6; Schlegel and Smit, 2018).
A MHW event in this study was defined using the Hobday et al. (2016) criteria when at least 5 consecutive days exceeded the 90th percentile of the 30y baseline SST climatology for Amphitrite Point lighthouse. Following Hobday et al. (2016), events above this threshold were classified based on their duration (number of days) and their intensity, to give the cumulative number of heatwave days for each spring (March to May) from 2004 to 2021. Three separate time periods were considered in the analyses below: baseline (2004-2013), marine heat wave (2014-2016) and post marine heat wave (2017-2021).
Fish assemblages
Eelgrass meadows were resampled each year for fish on the early morning low tides during early summer to minimize the effects of tide, season, daylight, and spatial factors on catch and occurrence (See Robinson et al., 2011; Robinson and Yakimishyn, 2013 for more details). Each meadow was sampled for fish using a 9.2 m long beach seine with 4mm stretch mess and a 3.1m drop in the centre. Triplicate beach seines sets were made from a small boat at the exposed edge of each meadow during a 2-h window before and after the lowest early morning tide. All fish were removed from the beach seine after each set and held in rubber totes to facilitate processing. The total area of each eelgrass meadow sampled after the three beach seines was about 150-200m2. The species catch per unit effort (CPUE) for each subregion and year was derived by pooling the total number of individuals of each species seined divided by the total number of beach seine sets completed in the group of eelgrass meadows that year. For each subregion and year, the wet weights to the nearest 0.1 gram for each of the 18 fish species were weighed on an Ohaus CS 200 scale. During each beach seining set, in situ sea surface temperature (SST: °C) measurements were taken using a handheld YSI model 30 salinity, conductivity and temperature meter.
Eelgrass fish assemblage biomass was calculated for both study regions and all years by summing the average wet weight of each fish species multiplied by the CPUE for each species and year to give grams wet weight per 200m2. Shiner perch were considered separately from the remaining 17 fish species because it contributed on average 40-60% of total biomass and would overwhelm the assemblage biomass. An annual standardized biomass anomaly was calculated for each study area by subtracting the long-term average 2005-2021 annual fish assemblage biomass (or shiner biomass) from each year and dividing by the long-term standard deviation(SD). The standardized annual anomalies illustrate departures from the long-term average conditions for both a fish assemblage without shiner perch and with shiner perch. The difference between a given year and the long-term mean and anomalies within ± 0.5 SD from the long-term mean indicate near average conditions.
Statistical analyses
A multivariate sequential method, control charts (Anderson and Thompson, 2004), was used to assess for changes in eelgrass fish assemblages in Clayoquot Sound and Barkley Sound inner and outer eelgrass meadows through time. The criterion used was the distance in multivariate space of fish composition and abundance made during the MHW years and post-MHW years compared to the centroid of observations made during the baseline period prior. The assessment criterion was based on the Bray-Curtis dissimilarity measure, and 10,000 bootstrap estimates were used to generate the 90th percentile of the Bray-Curtis dissimilarity for baseline years (Anderson and Thompson, 2004). Bray-Curtis dissimilarity values of MHW and post-MHW years are considered extreme as identified by reference to deviation from the 90th percentile of boot-strapped baseline estimates.
A nonparametric multi-dimensional species ordination (nMDS) using the Bray-Curtis similarity coefficient on fish species CPUE data, was conducted on each study inner and outer subregion and time period. An analysis of similarity (ANOSIM) was used to test for significance among year-group differences by examining the Global R statistic and p-value. If the Global test was significant, a similarity percent analysis (SIMPER) was then used to identify the fish species responsible for the significant difference in community composition between time periods. SIMPER calculates a discrimination index using species presence-absence, which is the ratio of average contribution of similarity between groups to the standard deviation of the similarity between groups. Our analyses focused on species that had a discrimination index value > 1.0 (Ley, 2005), and that contributed to at least 70% of the total annual dissimilarity between time periods. The PRIMER 6.0 software package (Clarke and Gorley, 2006) was used to conduct the nMDS, ANOSIM and SIMPER analyses.
Results
Marine heatwaves
The number of days during the spring (March-May) of each year identified as having MHW events peaked at 42d during 2015, with 2016 having the second highest number of events (34d; Figure 2). Furthermore, the first year of the MHW era, 2014, only had 17d of anomalous temperatures and they occurred at the start of May, while 2015 and 2016 had MHW days occurring from early March until mid April. The average monthly SST observed in MHW years 2015 and 2016 for March and April exceed the 30y long-term SST at Amphitrite Pt lighthouse by ~1.0°C, while the 2014 spring monthly average SST was below the 30y long-term average.
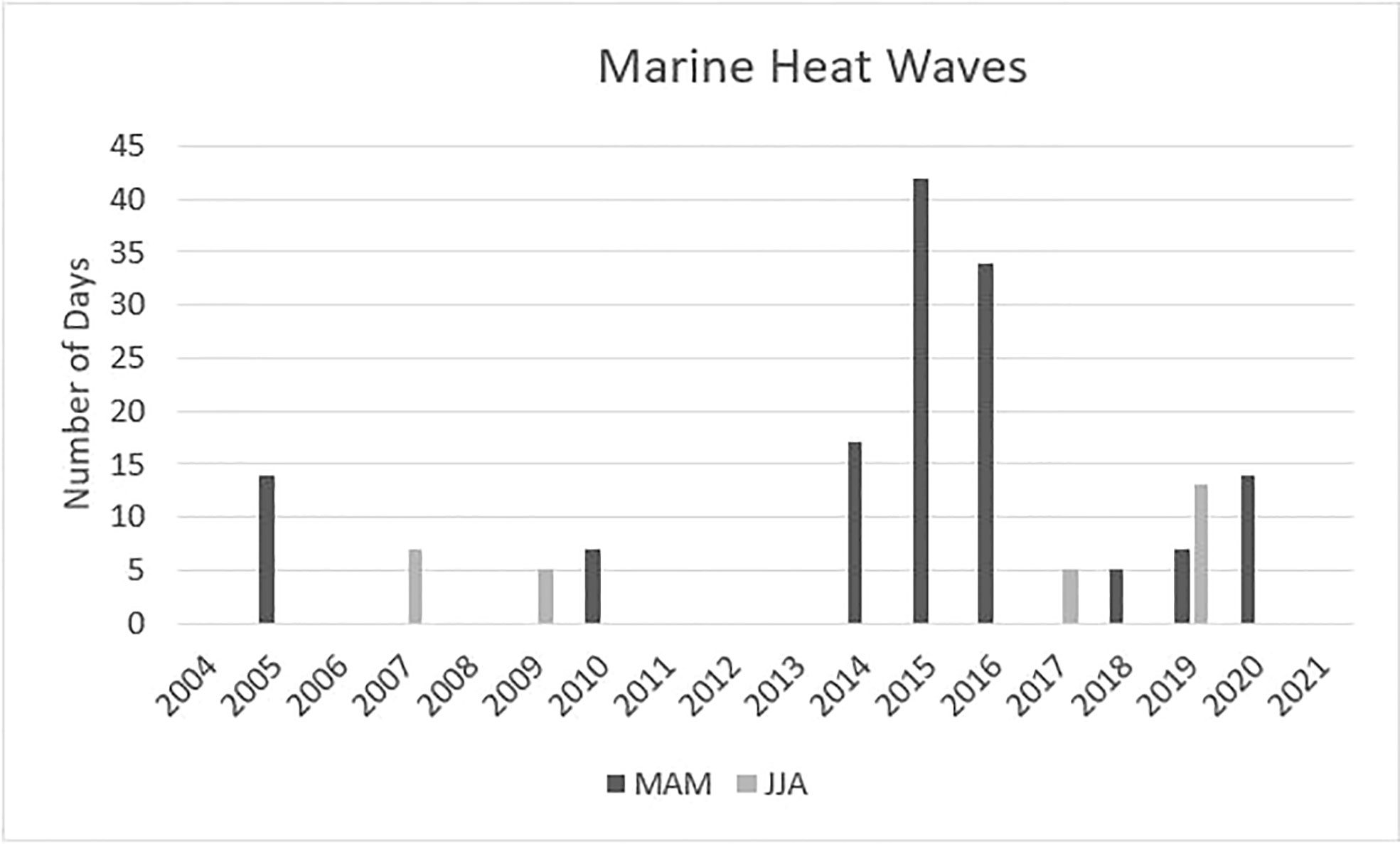
Figure 2 The number of marine heatwave days in the spring (MAM: March to May) and summer (JJA: June to August) of each year from 2004 to 2021. In this study, the baseline period was 2004-2013, MHW era 2014-2016 and 2017-2021 post-MHW years.
Fish assemblages
The 18 nearshore fish species, their overall percent frequency of occurrence, as well as the average number of fish weighed each year is shown in Table 1. In Clayoquot Sound, seven of the seventeen species had their highest or lowest CPUE during the 2015 or 2016 MHW years (Table 2): Scorpaenichthys marmoratus had its two highest CPUEs of the 17y time series in 2015 and 2016, while Sebastes caurinus and Brachyistius frenatus had their highest CPUE in 2015. In Clayoquot Sound, Artedius fenestralis, Apodichthys flavidus, Rimicola muscarum and Blepsias cirrhosus had their lowest CPUEs in the 17y time series in 2016 (Table 2).
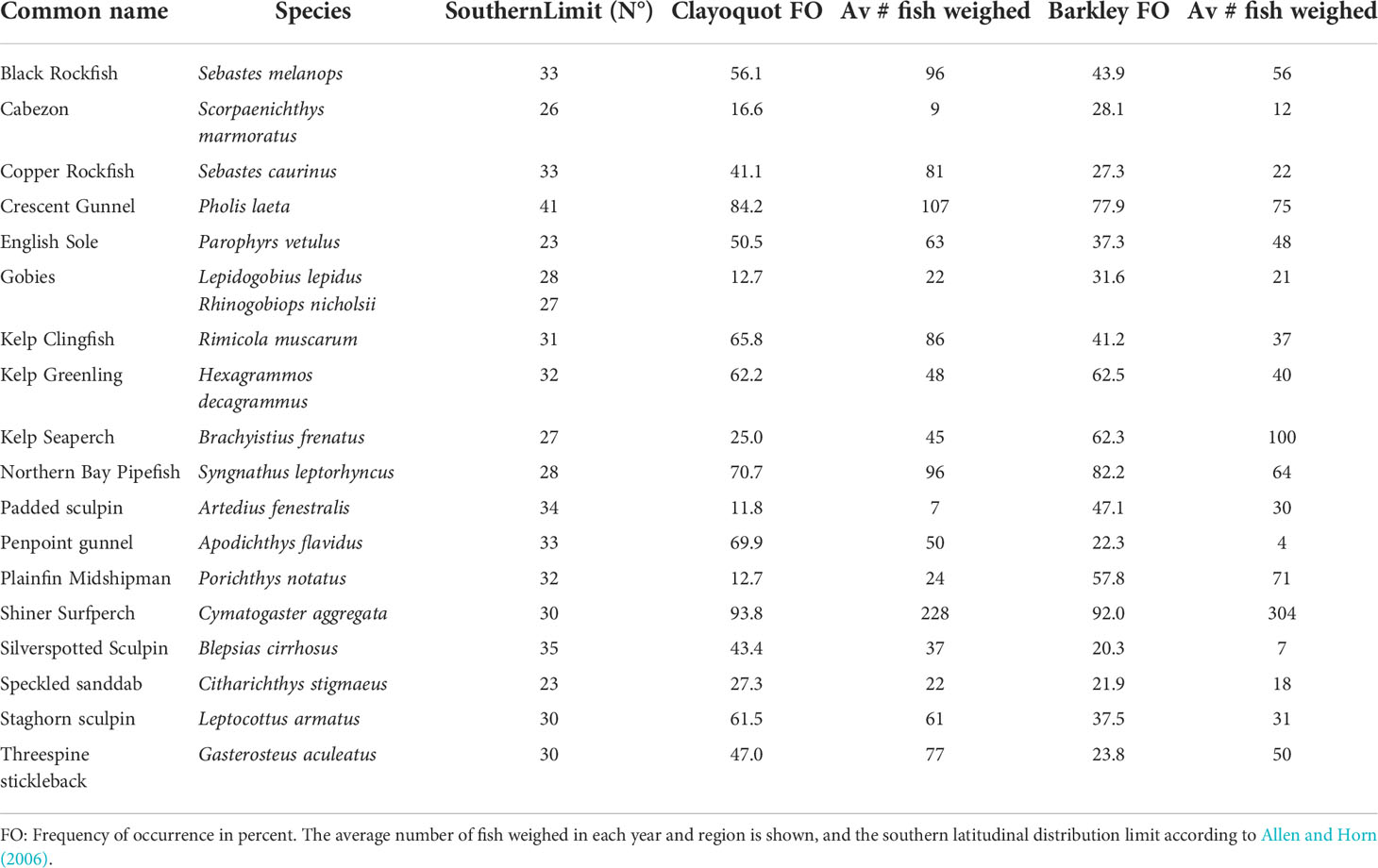
Table 1 List of 18 fish species most frequently caught by beach seine in eelgrass meadows from 2004 to 2021 in Clayoquot Sound (608 sets) and Barkley Sound (488 sets).
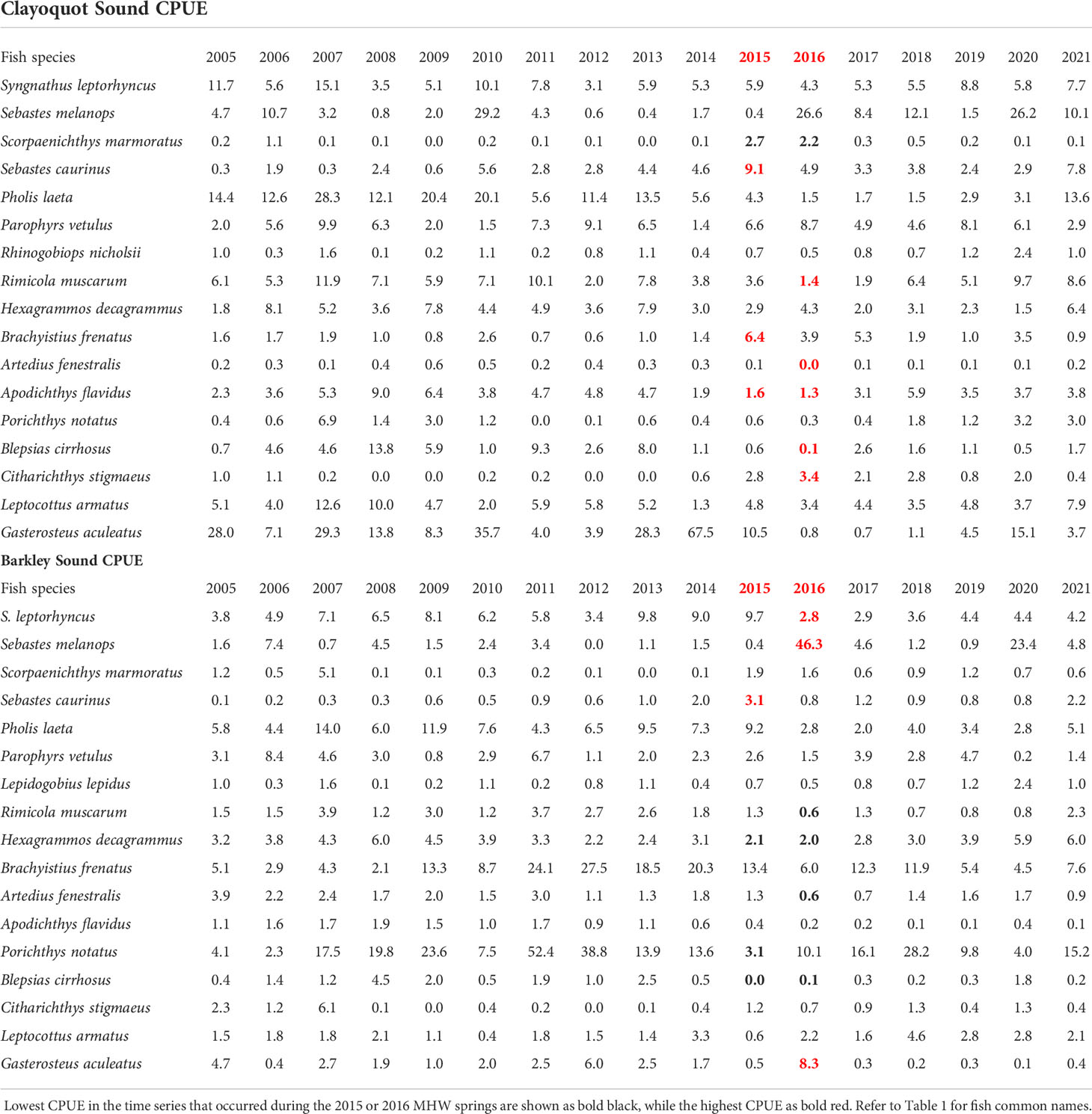
Table 2 Average catch per unit beach seine set for 17 common fish species caught in Clayoquot Sound or Barkley Sound from 2005 until 2021.
In Barkley Sound, nine of the seventeen species had their highest or lowest CPUE during the 2015 or 2016 MHW years (Table 2): S. caurinus had its highest time series CPUE in 2015, while S. melanops and Gasterosteus aculeatus had their highest timeseries CPUE in 2016 (Table 2). Three fish species, Syngnathus leptorhyncus, R. muscarum, and A. fenestralis had their lowest CPUE in the 17yr time series in 2016, while both Hexagrammos decagrammus and Blepsias cirrhosis had their lowest CPUE in both 2015 and 2016 (Table 2).
The eelgrass fish assemblage and Cymatogaster aggregata standardized annual biomass anomalies for Barkley and Clayoquot Sounds are shown in Figure 3, and the MHW years (2015 and 2016) did not result in extreme assemblage biomass anomalies in either region.
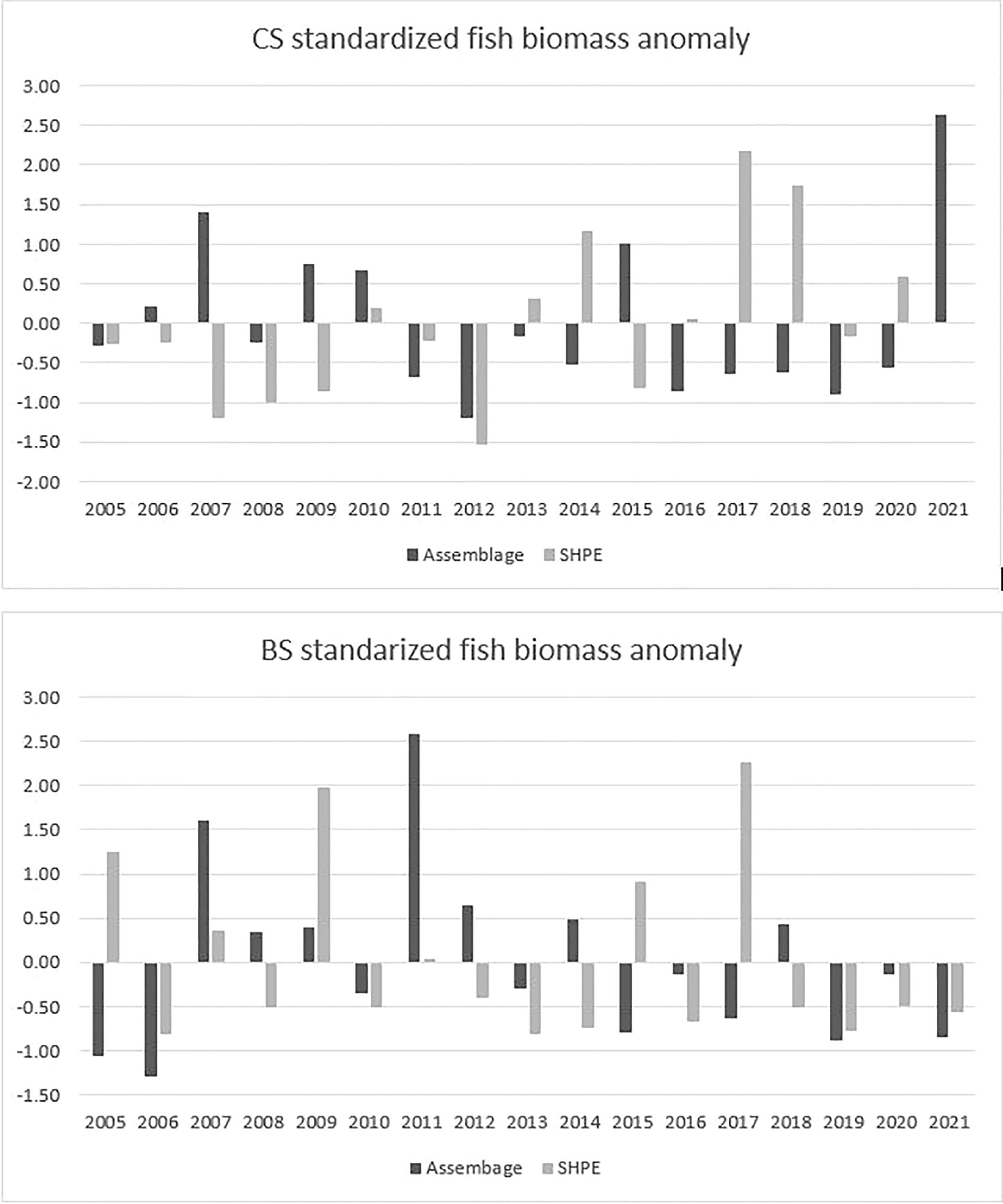
Figure 3 Annual eelgrass fish biomass anomaly (from 2005-2021 av) calculated from 17 species average annual wet weight and catch per unit effort of beach seining in Clayoquot Sound (CS) and Barkley Sound (BS). Note that Shiner perch (SHPE) were not included in the fish assemblage biomass anomaly and shown separately.
The control chart analysis indicated that fish assemblages from all Clayoquot eelgrass meadows combined exceeded the 90th percentile in Bray Curtis dissimilarity of the baseline period in 2016. However, inner Clayoquot eelgrass exceeded baseline in 2015, 2016 and 2017, while outer Clayoquot eelgrass only exceeded the baseline era in 2016 (Figure 4). Fish assemblages from all Barkley Sound eelgrass combined exceeded the baseline 90th percentile in 2015 and 2016, whereas only inner eelgrass exceeded in 2016 and outer eelgrass in both 2015 and 2016 (Figure 4). In both study estuaries, the 2014 fish assemblage dissimilarity did not exceed the 90th percentile baseline dissimilarity and remained between the 50th and 90th (Figure 4). During the post-MHW era (2017-2021), Clayoquot Sound outer meadow fish assemblages exceeded the baseline period 90th percentile in 2020 and inner meadows in 2017. Barkley Sound inner fish assemblages during the post-MHW era exceeded the 90th percentile of baseline similarity once in 2020, while Barkley Sound outer fish assemblages exceeded the 90th percentile in 2017, 2018 and 2022 (Figure 4).
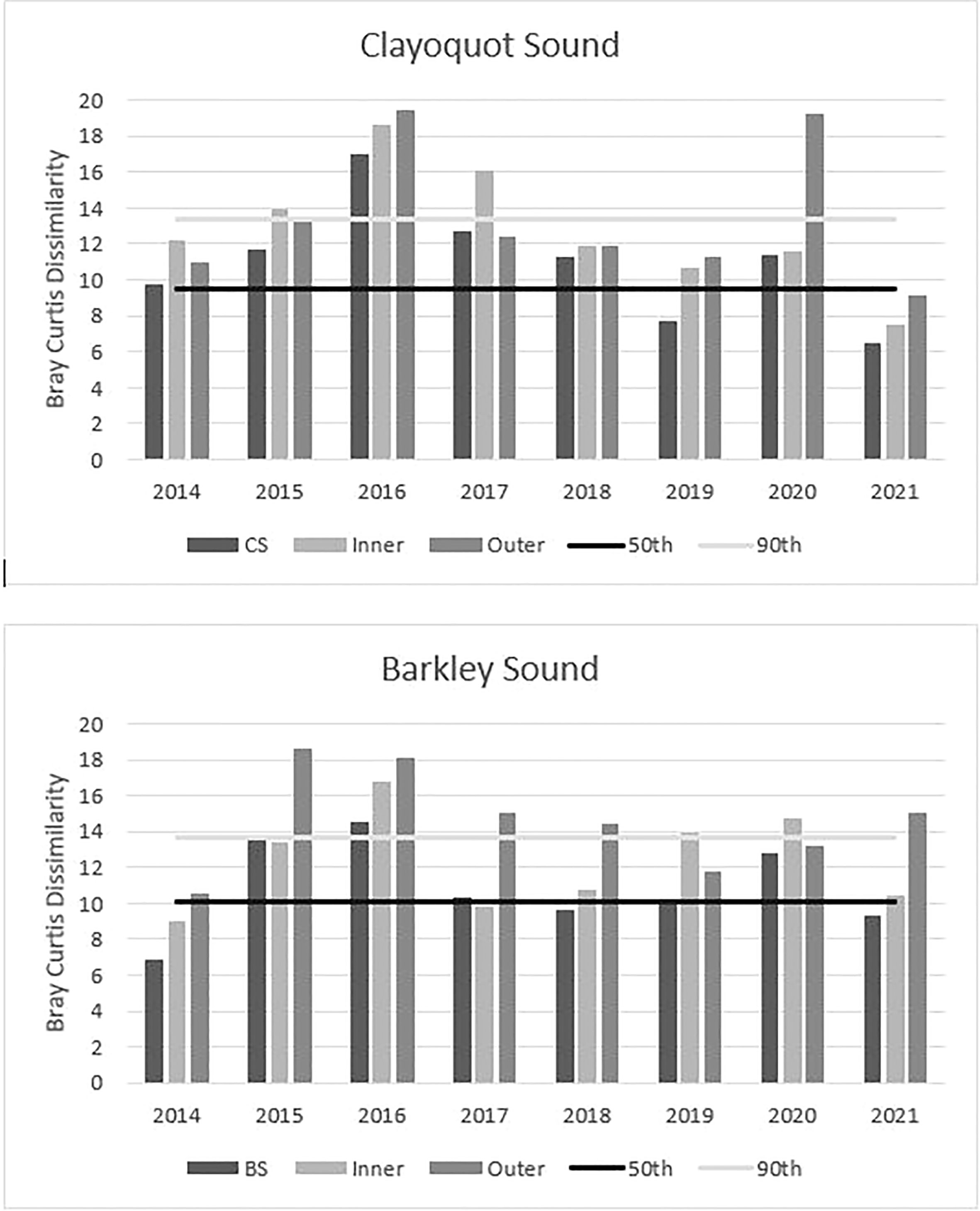
Figure 4 Results of the control chart analysis for inner and outer eelgrass meadows located in Clayoquot Sound and Barkley Sounds (Refer to Figure 1). The red line in each plot represents the 90th percentile and the black line the 50th percentile of Bray Curtis dissimilarities from the baseline pre-MHW 2004-2013. MHW era and post MHW era histograms that rise above the 90th percentile differ significantly from the baseline fish species assemblages.
It is important to note that the 2014 fish assemblages did not exceed the 2004-2013 baseline dissimilarity and that 2014 spring water temperatures were below the 30y long-term monthly averages. Hence, 2014 was re-classed as a baseline year.
An nMDS and ANOSIM of fish assemblages was conducted to determine if fish assemblages observed only during the 2015-2016 MHW springs were significantly different from the baseline era (2004-2014) and the post-MHW era (2017-2021) assemblages. Barkley Sound and Clayoquot Sound baseline fish assemblages were all found to be significantly different from their respective 2015-16 MHW era fish assemblages (Table 3 and Figures 5, 6).

Table 3 Results of analysis of similarity of fish assemblages in each study subregion and pair-wise comparisons between assemblages observed in baseline years (2004-2014), marine heat wave (MHW) years (2015-16) and post MHW years (2017-21). See Figure 5.
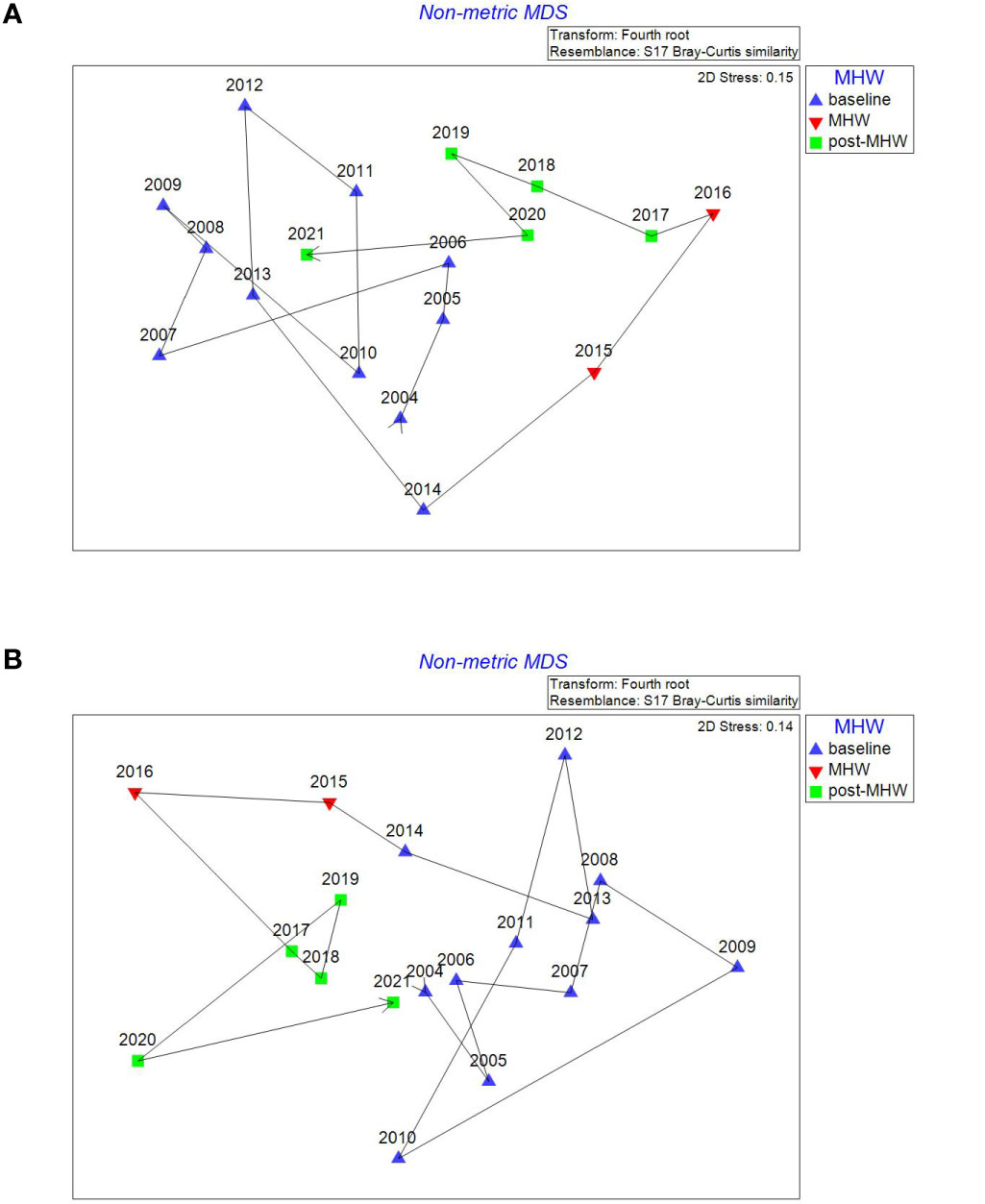
Figure 5 Non metric multidimensional ordination plots of Clayoquot Sound inner (A) and outer (B) eelgrass meadows showing the temporal trajectory in Bray Curtis similarity during the baseline (2004-2014), MHW (2015-2016) and post-MHW (2017-2021) periods. Fish CPUEs were fourth root transformed before the ordination was conducted.
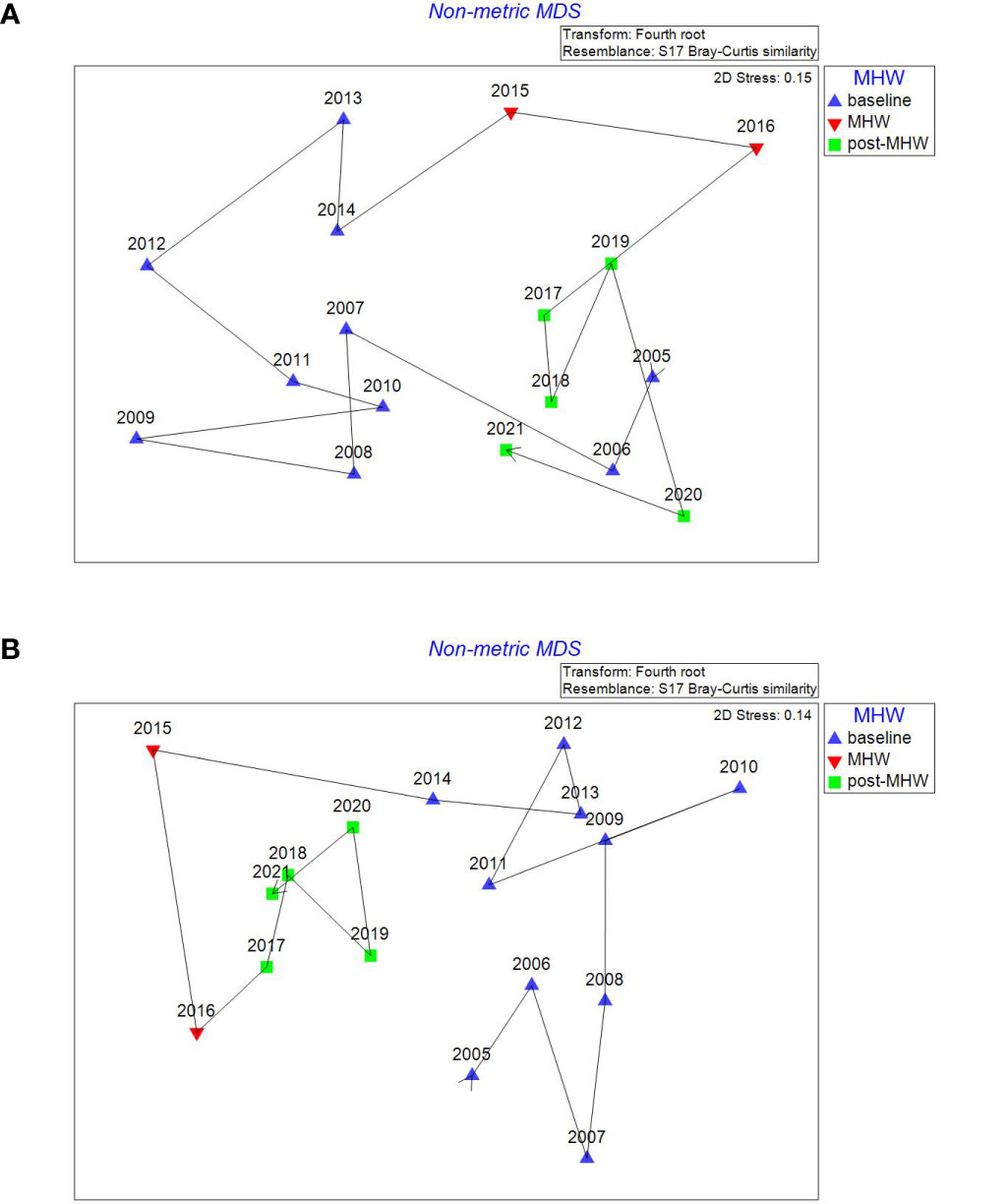
Figure 6 Non metric multidimensional ordination plots of Barkley Sound inner (A) and outer (B) study sub regions showing the temporal trajectory in Bray Curtis similarity during the baseline (2004-2014), MHW (2015-2016) and post-MHW (2017-2021) periods. Fish CPUEs were fourth root transformed before the ordination was conducted.
A temporal trajectory line was added to the fish assemblage ordination plots, to examine for the direction of change in fish assemblage similarity from the baseline era thru the 2015-2016 MHW to the post-MHW period (Figures 5, 6). Years closer together in the ordination plot have more similar fish assemblages than years further apart. The temporal trajectories showed that in both inner and outer Clayoquot Sound eelgrass meadows the 2015-2016 MHW event resulted in a movement of fish assemblage similarity away from the baseline years, followed by a slow return of similarity during the latter post-MHW years (e.g., 2021) back toward the baseline era similarity (Figure 5). Conversely, in both Barkley Sound inner and outer eelgrass meadow fish assemblages, the 2015-2016 MHW events resulted in an initial movement in similarity away from the baseline era, but the post-MHW year similarity appears to remain slightly distinctive from the baseline era (Figure 6). Note that an analysis of similarity indicates that the post-MHW fish assemblages in all 4 study subregions remain significantly dissimilar to the baseline era fish assemblages (Table 4).
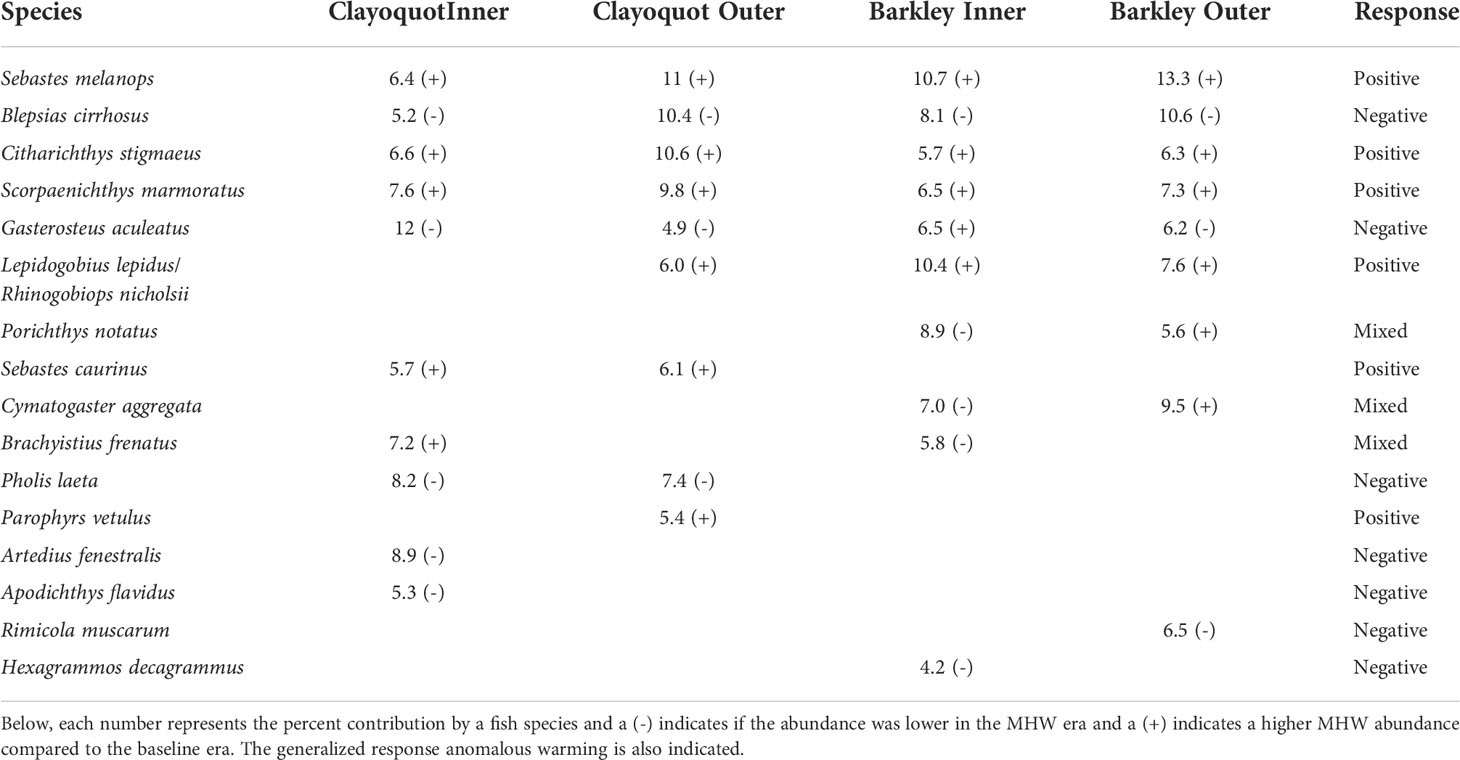
Table 4 Sixteen fish species identified in a SIMPER analysis of baseline (2004-2014) vs MHW (2015-2016) era assemblages that contributed to at least 70% of the separation observed in the nMDS ordination (Figure 5).
A SIMPER analysis indicated that in each subregion, 9-10 fish species contributed to the 70% dissimilarity between baseline and 2015-2016 MHW years. Overall, six fish species showed a positive increase in CPUE during the MHW years, six species showed reduced CPUE, while four species showed a mixed response (Table 4). Five fish species (S. melanops, C. stigmaeus, S. marmoratus, B. cirrhosis, and G. aculeatus) were found to contribute to assemblage dissimilarity across all four study subregions. S. melanops, C. stigmaeus and S. marmoratus had higher CPUEs in MHW years in all four subregions compared to baseline years, while B. cirrhosis CPUEs were consistently lower in MHW years (Table 4).
Discussion
The main objective of this study was to ascertain if 2014 to 2016 spring MHWs had a significant effect on early summer fish assemblage species composition, abundance and biomass in eelgrass meadows. It was presumed that increased water temperatures above the 90th percentile of the 30y long-term average daily spring temperatures would result in changes to fish growth, survival and ultimately leading to changes in early summer settlement to eelgrass. The MHW analysis of SST data showed that the 2014 spring SSTs were less than the 30y average SSTs for the region, and that the 2014 MHW event occurred over a short period (14d) in late May. In addition, there were no discernible affects on eelgrass fish assemblages and we were unable to distinguish spring 2014 assemblages from the study baseline period of 2004 to 2013. Others have noted that the 2014 MHW period did not begin in earnest in southern California until mid summer 2014 and occurred 72% of the time until June 2016 (Cavanaugh et al., 2019; Walker et al., 2020). It is possible that the low number of MHW days observed during late May 2014 were insufficient to modify young fish survival or settlement, or that other factors, such as food supply or changes in predation were more important factors than water temperature in influencing survival of young fish before settlement to eelgrass. In other Pacific regions MHW SST anomalies exceeded long-term water temperatures by 4-5°C and occurred during peak periods of water temperature (i.e., summer) that may already be beyond some fish species upper thermal tolerances (Day et al., 2018).
The control chart analysis did however detect an increase in fish assemblage dissimilarity in both study regions beginning in MHW spring 2015 and peaking in 2016 in both study regions compared to the baseline period. The SIMPER analysis further confirmed that 9-10 species CPUE contributed to the overall assemblage dissimilarity. Several fish species like S. melanops and S. caurinus showed an increase in CPUE, while B. cirrhosis, Artedius fenestralis, G. aculeatus, Pholis laeta, Apodichthys flavidus, Rimicola muscarum, and Hexagrammos decagrammus all showed reduced CPUE during warmer years. Magurran and Henderson (2010) previously noted that abundances of species in an estuarine fish assemblage changes asynchronously through time and that assemblages tend to vary around their mean abundances. Although the CPUE of about one-half of the fish species in the assemblage varied during the MHW 2015 and 2016 springs, the total eelgrass fish biomass was not anomalously different compared to other years in the 17-year time series. It has been well described previously that the consistency of estuarine fish assemblage biomass over time is maintained to a large extent by the presence of few core species, which are most common and contribute a high percentage of overall abundance in a given year (Magurran and Henderson (2010). In our study, a major core fish species, C. aggregata (Robinson and Yakimishyn, 2013) contributed 40-60% to total assemblage biomass but when considered separately did not exhibit any anomalous response in CPUE or biomass of the spring MHW years compared to baseline post-MHW years.
Although the main effect of the spring 2015 and 2016 MHW events observed on early summer WCVI fish assemblages was through changes in CPUE, there was no detected change in fish species composition. This result was not unexpected given that all fish species encountered along the WCVI are typically found in the middle of their latitudinal range, which extends northward by ~3,600km from the warmer waters of Santa Cruz, California to southern Alaska (Allen and Horn, 2006). Elsewhere it has been observed that most reef fish species that disappeared during a MHW in western Australia were characterized by geographic ranges that did not extend to latitudes with temperatures equivalent to the ocean temperature peak during the MHW (Day et al., 2018). Similarly, fish species richness in an area west of Scotland decreased because the number of cold-favoring Boreal species declined in response to warming (Ter Hofstede et al., 2010). In other cases, highly migratory tropical fish are capable of travelling thousands of kilometers northward in response to ocean warming and can temporally increase fish species richness in cooler northern locales (e.g., Cavole et al., 2016). In our study, the 18 common nearshore fish species in eelgrass are non migratory and were found at about 12-14° of latitude north of their southern geographic distribution limits (Table 1). Hence, temperatures experienced during the 2015 and 2016 MHW were well within WCVI species’ thermal tolerances and conditions did not immediately result in unexpected fish species composition.
The influence of the 2015 and 2016 spring MHW on eelgrass fish assemblages off the WCVI was clearly evident in the ordination analysis. However, the temporal response largely subsided through the post MHW era such that by 2021, fish assemblages in 3 of 4 subregions were very similar to baseline assemblages (Figures 5, 6). Nielsen et al. (2020) noted the affect of the 2015-2016 MHW on WCVI ichthyoplankton assemblages was rapid and had diminished by 2017. The more rapid response and recovery of the offshore water column ichthyoplankton assemblage compared to the nearshore eelgrass fish assemblage may be a reflection of the life history of the member species. For instance, high abundances of larval Engraulis mordax, Sardinops sagax and Merluccius productus observed during the MHW period were from highly migratory species with high fecundity rates that respond rapidly to suitable environmental conditions. In our study, the temporal trajectories of the Bray Curtis similarities in each study region from baseline through to post-MHW period (Figures 5, 6), showed the short-term pulse effect of the 2015-2016 spring MHW on early summer eelgrass fish assemblages. Essentially, the 2015-2016 spring MHW events temporarily increased dissimilarity and moved the fish assemblages away from the 2004-2014 baseline era, and in the case of Clayoquot outer and Barkley outer meadows, showed a distinct cluster of assemblages in the post-MHW era. However, this shift in assemblage structure appeared temporary as assemblage similarity moved back towards that observed during the baseline period.
Whilst our study focused on how newly settled eelgrass fish responded to the spring MHWs, there were insufficient data available as to ascertain if Clayoquot or Barkley eelgrass habitat quality or quantity itself had been affected by the 2015-2016 MHW event. It has been noted elsewhere from a controlled experiment that a 3.6°C increase in winter-to-spring temperature conditions in the Baltic Sea had a more severe impact on Z. marina than summertime warming, and resulted in advanced flowering, accelerated consumption of energy reserves and higher plant mortality Sawall et al. (2021). We suspect that because the spring MHW detected in our study region was only 0.5-1.5°C above the 30y long-term average, and that Z. marina in Clayoquot and Barkley Sounds are well within the mid range of SST and SSS experienced by this species over its northeast Pacific range from Bering Alaska to Baja California (Green and Short, 2003), it is unlikely that the spring MHW events would have severely affected this coastal plant. One recent MHW study on algal communities in Alaska did however find that macroalgal foundation species in the rocky intertidal declined and shifted to a heterotroph filter feeding dominated state. These results suggest that more northern populations of algae (and perhaps plant) species in the NE Pacific may be more susceptible to MHW events than mid latitude populations (Weitzman et al., 2021).
In summary, the response of eelgrass fish assemblages along the WCVI during the 2015 and 2016 springtime MHWs showed a milder response from what had been previously observed for pelagic fish species on the adjacent continental shelf. In this study, there were no detectable effects on fish species richness, and the peak anomalous response of the fish assemblage CPUE occurred primarily in early summer of 2016. In addition, the CPUEs of many eelgrass fish species increased and decreased in response to ocean warming, and the same fish families from the two study areas only 100km apart did not respond in synchrony to the MHWs. Overall, our results warrant caution about generalizing the effects of MHWs on nearshore marine fish. Furthermore, it is only through long-term studies of nearshore ecosystems such as this one, that the effects of oceanic perturbations such as MHWs can be more clearly and fully understood.
Data availability statement
The original contributions presented in the study are included in the article/supplementary materials. Further inquiries can be directed to the corresponding author.
Ethics statement
Ethical review and approval was not required for the animal study because fish were only captured held briefly for id and released.
Author contributions
CR and JY conceived the study. CR and RE conducted all data analyses. CR, JY and RE all contributed to the first draft of the manuscript. All authors contributed to the article and approved the submitted version.
Funding
The field component of this research was supported by Parks Canada Agency and the analytical and writing component was supported by the Aquatic Climate Change Adaptation Services Program, Fisheries and Oceans Canada.
Acknowledgments
This investigation into the effects of marine heat waves on nearshore fish species wouldn’t have been possible without the field commitment of many Parks Canada staff, students and volunteers since 2004. B. Proudfoot (DFO) created Figure 1.
Conflict of interest
The authors declare that the research was conducted in the absence of any commercial or financial relationships that could be construed as a potential conflict of interest.
Publisher’s note
All claims expressed in this article are solely those of the authors and do not necessarily represent those of their affiliated organizations, or those of the publisher, the editors and the reviewers. Any product that may be evaluated in this article, or claim that may be made by its manufacturer, is not guaranteed or endorsed by the publisher.
References
Allen L. G., Horn M. H. (Eds.) (2006). The ecology of marine fishes: California and adjacent waters (University of California Press) University of California, 672. doi: 10.1525/9780520932470
Anderson M. J., Thompson A. A. (2004). Multivariate control charts for ecological and environmental monitoring. Ecol. Appl. 14 (6), 1921–1935. doi: 10.1890/03-5379
Auth T. D., Daly E. A., Brodeur R. D., Fisher J. L. (2018). Phenological and distributional shifts in ichthyoplankton associated with recent warming in the northeast pacific ocean. Global Change Biol. 24, 259–272. doi: 10.1111/gcb.13872
Bond N. A., Cronin M. F., Freeland H., Mantua N. (2015). Causes and impacts of the 2014 warm anomaly in the NE pacific. Geophys. Res. Lett. 42 (9), 3414–3420. doi: 10.1002/2015GL063306
Cavanaugh K. C., Reed D. C., Bell T. W., Castorani M. C. N., Beas-Luna R. (2019). Spatial variability in the resistance and resilience of giant kelp in southern and Baja California to a multiyear heatwave. Front. Mar. Sci. 6. doi: 10.3389/fmars.2019.00413
Cavole L.M., Demko A.M., Diner R.E., et al (2016). Biological impacts of the 2013-2015 warm-water anomaly in the northeast Pacific; Winners, Losers, and the Future. Oceanography 29(2). doi: 10.5670/oceanog.2016.32
Day P.B., Stuart-Smith R.D., Edgar G.J., Bates A.E. (2018). Species’ thermal ranges predict changes in reef fish community structure during 8 years of extreme temperature variation. Diversity and Distribution 24(8), 1036–1046. doi: 10.1111/ddi.12753
Di Lorenzo E., Mantua N. (2016). Multi-year persistence of the 2014/15 north pacific marine heatwave. Nat. Climate Change 6, 1,042–1,047. doi: 10.1038/nclimate3082
Green E. P., Short F. T. (2003). ). world atlas of seagrasses. prepared by UNEP world conservation monitoring centre (Berkley, USA: University of California Press)288.
Hobday A. J., Alexander L. V., Perkins S. E., Smale D. A., Straub S. C., Oliver E. C. J., et al. (2016). A hierarchical approach to defining marine heatwaves. Prog. Oceanogr. 141, 227–238. doi: 10.1016/j.pocean.2015.12.014
Hobday A. J., Oliver E. C. J., Sen Gupta A., Benthuysen J. A., Burrows M. T., Donat M. G., et al. (2018). Categorizing and naming marine heatwaves. Oceanography 31 (2), 162–173. doi: 10.5670/oceanog.2018.205
Jenkins G.P., King D. (2006). Variation in larval growth can predict recruitment of a temperate, seagrass-associated fish. Oceanologia 147, 641–649. doi: 10.1007/s00442-005-0336-5
Ley J.A. (2005). Linking fish assemblages and attributes of mangrove estuaries in tropical Australia: criteria for regional marine reserves. Marine Ecology Progress Series 305, 41–57. doi: 10.3354/meps305041
Mackas D. L., Batten S., Trudel M. (2007). Effects on zooplankton of a warmer ocean: Recent evidence from the northeast pacific. Prog. Oceanogr. 75, 223–252. doi: 10.1016/j.pocean.2007.08.010
Magurran A. E., Henderson P. A. (2010). Temporal turnover and the maintenance of diversity in ecological assemblages. Phil. Trans. R. Soc 365, 3611–3620. doi: 10.1098/rstb.2010.0285
McFarlane S., Beamish R. (1981). The re-occurrence of sardines off British Columbia characterises the dynamic nature of regimes. Progress in Oceanography 49, 151–165. doi: 10.1016/S0079-6611(01)00020-9
Mcfarlane G. A., Ware D. M., Thomson R., Mackas D. L., Robinson C. L. K. (1997). Physical, biological and fisheries oceanography of a large ecosystem (west coast of Vancouver island) and implications for management. Oceanologica Acta 20 (1), 191–200. Available at: https://archimer.ifremer.fr/doc/00093/20452/
Neuheimer A. B., Taggart C. T. (2007). The growing degree-day and fish size-at-age: the overlooked metric. Can. J. Fish. Aquat. Sci. 64, 375–385. doi: 10.1139/f07-003
Nielsen J. M., Rogers L. A., Brodeur R. D., Thompson A., Auth T. D., Deary A. L., et al. (2020). Responses of ichthyoplankton assemblages to the recent marine heatwave and previous climate fluctuations in several northeast pacific marine ecosystems. Global Change Biol. 27, 506–520. doi: 10.1111/gcb.15415
van Strein A. (2004) TRIM 3 manual (Trends & indices for monitoring data). Available at: https://pecbms.info/methods/software.
Pawlowicz R. (2017). Seasonal cycles, hypoxia, and renewal in a coastal fjord (Barkley sound, British Columbia). Atmosphere-Ocean 55 (4-5), 264–283. doi: 10.1080/07055900.2017.1374240
Robinson C. L. K., Gower J. F. R., Borstad G. A. (2004). Twenty years of satellite observations describing phytoplankton blooms in seas adjacent to gwaii haanas national park, reserve, canada. J. Remote Sens. 30 (1), 36–43. doi: 10.5589/m03-050
Robinson C. L. K., Yakimishyn J. (2013). The persistence and stability of fish assemblages within eelgrass meadows (Zostera marina) on the pacific coast of Canada. Can. J. Fish. Aquat. Sci. 70, 775–784. doi: 10.1139/cjfas-2012-0339
Robinson C. L. K., Yakimishyn J., Dearden P. (2011). Habitat heterogeneity in eelgrass fish assemblage diversity and turnover. Aquat. Conserv. Mar. Freshw. Ecosyst. 21, 625–635. doi: 10.1002/aqc.1227
Rooper C. N., Gunderson D. R., Hickey B. M. (2006). An examination of the feasibility of passive transport from coastal spawning grounds to estuarine nursery areas for English sole. Estuar. Coast. Shelf Sci. 68, 609–618. doi: 10.1016/j.ecss.2006.03.009
Sanford E., Sones J. L., Garcia-Reyes M., Goddard J. H. R., Largier J. L. (2019). Widespread shifts in the coastal biota of northern California during the 2014-2016 marine heatwaves. Sci. Rep. 9, 4216. doi: 10.1038/s41598-019-40784-3
Sawall Y., Ito M., Pansch C. (2021). Chronically elevated sea surface temperatures revealed high susceptibility of the eelgrass zostera marina to winter and spring warming. Limnol. Oceanogr. 66, 4112–4124. doi: 10.1002/lno.11947
Schlegel R. W., Smit A. J. (2018). “heatwaveR: A central algorithm for the detection of heatwaves and cold-spells”. J. Open Source Softw. 3 (27), 821. doi: 10.21105/joss.00821
Ter Hofstede R., Hiddink J. G., Rijnsdorp A. D. (2010). Regional warming changes fish species richness in the eastern north Atlantic ocean. Mar. Ecol. Prog. Ser. 414, 1–9. doi: 10.3354/meps08753
Thomson R. E. (1981). Oceanography of the British Columbia coast. Can. Spec. Publ. Fish. Aquat. Sci. 56, 291.
Walker H. J., Hastings P. A., Hyde J. R., Lea R. N., Snodgrass O. E., Bellquist L. F. (2020). Unusual occurrences of fishes in the southern California current system during the warm water period of 2014-2018. Estuar. Coast. Shelf Sci. 236, 106634. doi: 10.1016/j.ecss.2020.106634
Weitzman B., Konar B., Iken K., Colett H., Monson D., Suryan R., et al. (2021). Changes in rocky intertidal community structure during a marine heatwave in the northern gulf of Alaska. Front. Mar. Sci. 8, 556820. https://doi.org/10.3389/fmars.2021.556820. doi: 10.3389/fmars.2021.556820
Keywords: marine heatwave (MHW), eelgrass (Zostera marina), fish assemblage, control chart, coastal
Citation: Robinson CLK, Yakimishyn J and Evans R (2022) Minimal effects of the 2014-16 marine heatwave on fish assemblages found in eelgrass meadows on the southwestern coast of Vancouver Island, British Columbia, Canada. Front. Mar. Sci. 9:980703. doi: 10.3389/fmars.2022.980703
Received: 28 June 2022; Accepted: 04 August 2022;
Published: 18 August 2022.
Edited by:
Zeyu Zeng, University of British Columbia, CanadaReviewed by:
David W. Ginsburg, University of Southern California, United StatesKisei R. Tanaka, Pacific Islands Fisheries Science Center (NOAA), United States
Copyright © 2022 Robinson, Yakimishyn and Evans. This is an open-access article distributed under the terms of the Creative Commons Attribution License (CC BY). The use, distribution or reproduction in other forums is permitted, provided the original author(s) and the copyright owner(s) are credited and that the original publication in this journal is cited, in accordance with accepted academic practice. No use, distribution or reproduction is permitted which does not comply with these terms.
*Correspondence: Clifford L.K. Robinson, Cliff.robinson@dfo-mpo.gc.ca
†ORCID: Clifford L.K. Robinson, orcid.org/0000-0001-9199-2233