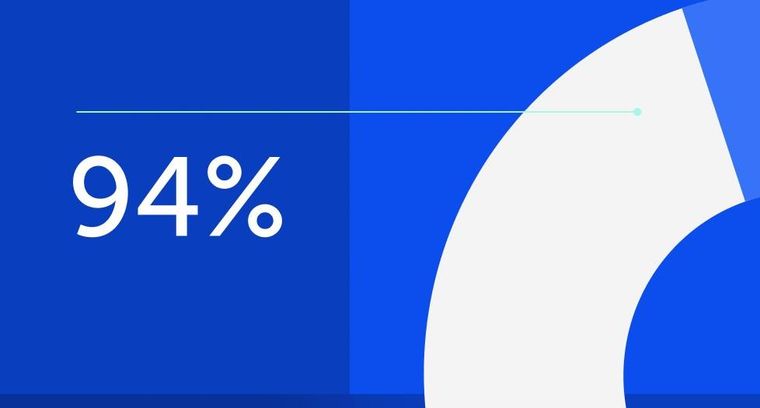
94% of researchers rate our articles as excellent or good
Learn more about the work of our research integrity team to safeguard the quality of each article we publish.
Find out more
ORIGINAL RESEARCH article
Front. Mar. Sci., 17 August 2022
Sec. Aquatic Physiology
Volume 9 - 2022 | https://doi.org/10.3389/fmars.2022.977719
This article is part of the Research TopicRising Stars in Aquatic Physiology: 2022View all 5 articles
The perception of an acute stressor (short-duration; high-intensity) induces a physiological response that activates the hypothalamic-pituitary-interrenal (HPI) axis and the subsequent release of cortisol. Cortisol carries out its effect at the molecular level through its recognition by the mineralocorticoid receptor (MR) and the glucocorticoid receptor (GR). Recently, we unveiled the nucleotide sequence of the glucocorticoid receptor 1 (gr1) and gr2 in gilthead sea bream (Sparus aurata). Importantly, GR1 and GR2 respond to different levels of cortisol concentration in fish and, consequently, play a differential role in the stress response. To date, and despite their relevance, no data describes the modulation of these receptors in response to an acute stressor in gilthead sea bream (S. aurata). In this study, we evaluated the kinetics of modulation of cortisol receptors expression (gr1, gr2, mr), and its similarity with the expression pattern of selected genes associated with stress (hsp70; enolase) and immune response (lysozyme; c3; il-1β; tnf-α; il-10; tgf-β1) in gilthead sea bream mucosal tissues (skin; gills; anterior gut). To do it, fish were acutely stressed by three-minute air exposure, and the expression profile was evaluated at zero, 1 h, 6 h, and 24 h post-stress (hps). The cortisol level in plasma and skin mucus peaked at 1 hps. All the mucosal tissues showed a time-dependent and tissue-specific upregulation of gr1 and mr. The immune-related genes showed the upregulation of il-1β at 6 hps (gills; anterior gut), and tnf-α and c3 at 24 hps (anterior gut). Taking together, our study concludes that fish subjected to three-minute air exposure modulated the expression of gr1 but not gr2 in mucosal tissues (skin; gills; anterior gut). Furthermore, our data reinforce the idea of a stimulatory effect induced in genes associated with the innate immune response after acute stress but focused at the mucosal level and in a time- and tissue-dependent manner.
Stress is a situation in which an organism is subjected to an alteration (exposure to a stressor) that can result in real or symbolic challenge to its integrity (Tort, 2011). Depending on the time that the organism is exposed, stress can be classified as acute (short-term and high-intensity) or chronic (sustained over time). Acute stress situations provoke “fight or flight reactions” that imply the survival or death of the individual. In aquaculture, fish can be subjected to acute stressors due to intrinsic processes of production, and to the use of intensive systems associated to culture strategies (exposure to air, chase, catch, handling) (Iversen et al., 2014; Khansari et al., 2017c).
In teleosts, cortisol is the main corticosteroid and plays an important role in many physiological areas including growth, immunoregulation, and energy maintenance (Tsalafouta et al., 2018). A stress situation causes the activation of a coordinated system of two physiological axes: the HPI axis (Hypothalamus-Pituitary-Interrenal axis) where the head kidney interrenal cells produce corticosteroids (cortisol), and the HSC axis (Hypothalamus-Sympathetic-Chromaffin axis) where the chromaffin cells produce catecholamines (epinephrine and norepinephrine) (Khansari et al., 2019). Immediately after sensing the stressful stimulus, catecholamines activate cardiovascular, respiratory, and metabolic responses, aimed at mitigating the effects of the acute stressors. This mechanism of response is immediately activated once the stimulus is perceived, while the release of glucocorticoid hormones is slightly delayed by minutes (Schreck and Tort, 2016). Then, the HPI axis involves the immediate secretion of corticosteroid-releasing hormone (CRH) by the preoptic nucleus of the hypothalamus (Parra et al., 2015). Stimulated CRH receptors of corticotropic cells of the pituitary gland induce the release of adrenocorticotropic hormone (ACTH) into the circulation. It subsequently stimulates the release of cortisol from the interrenal cells of the head kidney, as well as melanocyte-stimulating hormone (α-MSH) (Tort, 2011).
Cortisol induces relevant effects on immune responses such as an increase in neutrophils, a reduction in the number of lymphocytes and antibody responses, or a decrease in the production of some cytokines, such as TNF-α, TGF-β, and IL-6 (Parra et al., 2015). However, it seems difficult to conclusively state that acute stress has a definitive immunoenhancing effect in fish (Guo and Dixon, 2021). There is consensus that the greater energy demand required in response to the activation of the stress response produces a lack of energy resources destined to other processes, which compromises the immune response (Tort, 2011). In teleosts, the head kidney is a lymphohematopoietic organ with endocrine functions (Parra et al., 2015). The head kidney acts as an endocrine gland responsible for the secretion of stress hormones and also as an intermediary tissue for the regulation of immune processes in response to stressors (Khansari et al., 2017b; Khansari et al., 2017a). Thus, the activation of the stress response, and consequently the increase in cortisol, produces a series of secondary physiological responses in fish (changes in the metabolism and osmoregulation, and immunosuppression, among others) (Schreck and Tort, 2016).
Mucosal surfaces have a pivotal role in fish health. The mucosal surfaces play a key role in protecting the body from threats and infections. They act as the first body barrier when contacting with the external agents, with the benefits and threats that this implies, such as the interaction with pathogens. Thus, mucosal tissues register a wide repertoire of responses both at the innate and specific immunity level (Esteban and Cerezuela, 2015; Koppang et al., 2015; Merrifield and Rodiles, 2015; Salinas and Parra, 2015). For this reason, they have been described as mucosal-associated lymphoid tissues (MALT), including the nose-associated lymphoid tissue (NALT), skin-associated lymphoid tissue (SALT), gill-associated lymphoid tissue (GIALT), and gut-associated lymphoid tissue (GALT). The skin, gills, and gut are the mucosal tissues in which special emphasis has been placed on the evaluation of the stress response at the hormone and gene expression levels. The presence of stress hormones in mucosa-associated tissues has attracted special interest because of its relevance as a potential non-invasive biomarker but also due to the modulation of genes associated with stress and immune response at the local level (Khansari et al., 2018). In this way, the measurement of cortisol in blood has been the traditional method for assessing the fish stress response performance. In the last years, new sample collection strategies have been used to measure cortisol including skin mucus (Khansari et al., 2018; Franco-Martinez et al., 2022). Importantly, it has been shown in rainbow trout that the measurement of cortisol levels in skin mucus accurately reflects plasma responses to stress at early times of exposure (Carbajal et al., 2019). In other words, the cortisol detected in skin mucus would be the reflection of the high circulating concentrations at systemic (bloodstream) level (Carbajal et al., 2019). Importantly, it has been recently proposed that the cortisol secreted after an intense activation of the HPI axis but not the exogenous cortisol sensed from the environment would be responsible of the changes in the expression profile in fish mucosa (Vallejos-Vidal et al., 2022). This acquires special relevance when considering the immunoprotective and defense role of mucosa-associated tissues (Parra et al., 2015), and the repercussions of the presence of cortisol in the modulation over time of the expression of genes involved in the immune response to a given stressor stimulus.
Cortisol is recognized by the mineralocorticoid receptor (MR) and the glucocorticoid receptors 1 (GR1) and 2 (GR2), thus carrying out its mechanism of action at the physiological level. The identification of glucocorticoid receptors in the different species of teleost has been a challenge and a matter of interest given their relevance in the activation of the stress response by different levels of cortisol (Bury et al., 2003; Stolte et al., 2006). Thus, GR1 would be responsible for the recognition of cortisol at higher concentrations, meanwhile the GR2 would act at a very low concentration of cortisol (Vazzana et al., 2010). In the case of gilthead sea bream, our research group reported in 2007 (Acerete et al., 2007) a single GR. Years later, in 2018, another study indicated that such gr nucleotide sequence corresponded to gr1 (Tsalafouta et al., 2018). A recent study made by our research team determined that the difference at nucleotide sequence level between gr1 and gr2 resides in an insertion/deletion in the DNA binding region of gilthead sea bream (S. aurata), thus unveiling the uncertainty that existed around the glucocorticoid receptors in gilthead sea bream. At the same time, this finding evidences the lack of knowledge regarding the pattern of gene expression of gr1 and gr2 in gilthead sea bream in the presence of a stressor. Thus, in this study, we aimed to evaluate the gene expression profile for glucocorticoid receptors (gr1, gr2) in gilthead sea bream. We subjected fish to air exposure as a model of stress response induction by the activation of the HSC and HPI axes (Arends et al., 1999; Khansari et al., 2018; Skrzynska et al., 2018; Khansari et al., 2019; Liu et al., 2019). We also evaluated the expression pattern of mineralocorticoid receptor (mr) and genes associated with stress (hsp70; enolase) and immune response (lysozyme; c3; il-1β; tnf-α; il-10; tgf-β1) in gilthead sea bream mucosal tissues (skin; gills; anterior gut). The acute stress response was analyzed at zero, 1 h, 6 h, and 24 h post-stress (hps). Taking together, our study concludes that fish subjected to three-minute air exposure modulated the expression of gr1 but not gr2 in mucosal tissues (skin; gills; anterior gut).
Gilthead sea bream (S. aurata, Linnaeus, 1758) were obtained from a local fish farm (Culmarex, Puerto de Sagunto, Valencia, Spain), with a length of 23.59 ± 0.83 cm (mean ± standard deviation) and a body weight of 150 ± 5.2 g (n total= 32). The fish were acclimatized to the laboratory conditions in the same tank for 30 days. Fish were kept in a closed recirculating seawater system at 21-22°C, on a 12-h light/12-h dark cycle, and fed a commercial diet at 1.5% of total body weight per day (Skretting). They were kept in 300-liter tanks at a density of 2 kg/m3. The fish were fasted 24 h before the experimental procedures. Dissolved oxygen (7.1 ± 0.1 mg/l), pH (7.1 ± 0.1), nitrite (<0.5 mg/l), nitrate (<10 mg/l), and total ammonia (≤ 0.5) were analyzed periodically.
All procedures were carried out in accordance with the ethical guidelines for animal experimentation (EEC regulation 86/609), and authorized and supervised by the ethics committee of the “Universitat Autònoma de Barcelona” (Ref. OH4218_4219).
Fish were fasted 24 h before the experimental procedure. Gilthead sea bream (total n = 24) were stressed by exposure to the air for 3 minutes using a net because it has been shown to be effective in inducing the stress response (Arends et al., 1999; Khansari et al., 2018; Skrzynska et al., 2018; Khansari et al., 2019; Liu et al., 2019). Then, fish were placed in three independent tanks (n=8 fish in each tank; one tank per sampling time-point) at a culture density of 2 kg/m3. Each tank has a closed recirculation system consisting of a water pump, solid filter, and a mature biofilter. Non-stressed fish (n=8) were used as a control group and maintained at a density of 2 kg/m3.
Fish were sampled at zero (control group), and 1 h, 6 h, and 24 hours post-stress (hps) (n=8 per time-point, coming all sampled fish from the same tank). All animals were sacrificed by anesthetic overdose (MS222 (Sigma), 200 mg/l). For the collection of plasma samples, the tubes and syringes were previously heparinized. Once collected, the blood sample was centrifuged at 3000 x g and 4°C for 10 minutes. Each sample of plasma obtained (supernatant) was stored at -20°C. On the other hand, the skin mucus samples were collected as described elsewhere (Khansari et al., 2018), and immediately placed on ice. The samples were homogenized in a 1:1 volume of TBS buffer and centrifuged at 3500 x g at 4°C for 10 min. The collected supernatant was stored at -80°C. Skin tissue samples were taken from the upper lateral line area behind the dorsal fin, the left side, and avoiding muscle contamination (Vallejos-Vidal et al., 2022). Then, the body cavity was opened laterally and the anterior gut was sampled removing the feces and mucus carefully with forceps, and then rinsed once with PBS. For gills, the first lamella from the left side was sampled (Khansari et al., 2018). Tissue samples were immediately frozen in liquid nitrogen and stored at -80°C for further processing.
Cortisol level was measured by radioimmunoassay (RIA) and radioactivity was quantified using a liquid scintillation counter (Scintillation Counter Wallac 1409; PerkinElmer), as described elsewhere (Vargas et al., 2018). Anti-cortisol antibody (ref. 07-121016, MP Biomedicals, Solon, OH, USA) was used for the assay at the final dilution of 1:4,500. The cross-reactivity of the antibody with cortisol was 100%, and the lowest detection limit of the assay was 0.16 ng/ml. Cross-reactivity with other steroid hormones ranged from 1.6% for corticosterone to less than 0.7% for other steroids tested. The analysis was conducted in duplicate for each sample analyzed (n=8).
The total RNA was extracted from each individual tissue sample using the TriReagent (Sigma) according to the manufacturer’s instructions. In brief, 50-100 mg of tissue was homogenized in 1 ml TriReagent® (Sigma) with a Polytron and then incubated at room temperature for 5 min. Subsequently, 0.1 mL of BCP (Sigma) was added to each sample, vortexed, and incubated at room temperature for 15 min. The samples were centrifuged at 12,000 x g for 15 minutes at 4°C to separate the phases. After transferring the aqueous phase to a new tube, the RNA was precipitated by adding 0.5 ml of 2-propanol (Sigma). The samples were incubated at -20°C overnight and subsequently centrifuged at 12,000 x g for 10 min at 4°C. Finally, the RNA pellet was washed twice with 1 ml of 75% ethanol (prepared with DEPC-water), air-dried for 10 min, resuspended in DEPC-water, and then incubated in a thermoblock at 55°C for 10 min. The RNA sample was quantified with a Nanodrop ND-1000 (Thermo Scientific) and the quality of the RNA was verified by Bioanalyzer (Agilent Technologies). All the RNA integrity number (RIN) values obtained were > 7.0, which indicates the integrity and quality of the RNA suitable for transcriptomics analysis. Subsequently, cDNA was prepared from 1000 ng of total RNA, using the iScript cDNA kit (Bio-Rad Laboratories) according to the manufacturer’s instructions.
The immunological gene expression profiling in gilthead sea bream subjected to air-exposure acute stress was assessed in the skin, gills, and anterior gut. Thus, the transcript level of interleukin 1-beta (il-1β), tumor necrosis factor-alpha (tnf-α), interleukin 10 (il-10), tumor growth factor-beta 1 (tgf-β1), lysozyme (lys), complement protein c3 (c3), the glucocorticoid receptor (gr) 1 (gr1) and gr2, the mineralocorticoid receptor (mr); and stress-related genes including the heat shock protein 70 (hsp70), and enolase were analyzed by real-time PCR (RT-qPCR). The reference genes elongation factor 1-alpha (ef1-α) and the ribosomal protein L27 (rpl27) were evaluated in gilthead sea bream based on previous evidence (Khansari et al., 2018; Khansari et al., 2019) to determine the gene with the lower variation among all the samples included in the analysis. Thus, in our study, the rpl27 was used as a reference gene. All primers used in this study were designed and checked for their specificity with the Primer-Blast Primer software. Table 1 details the list of primers. The potential development of secondary structure and self/heterodimer were verified with OligoAnalyzer (version 3.1). The primer efficiency was checked for all the primer sets included in the study. The RT-qPCR reactions were performed with iTaq universal sybr green supermix (Bio-Rad Laboratories) using 2 μl of 1:40 dilution of cDNA (for the reference genes) and 2 μl of 1:10 dilution of cDNA (for the rest of the genes) as optimum cDNA dilution for each tissue based on the set of serial dilutions contained in the primer efficiency curve. Primers for all genes were used at a final concentration of 500 nM. The thermal conditions used were 3 min at 95°C for preincubation followed by 40 cycles at 95°C for 30 s, and 60°C for 30 s. All reactions were performed in duplicate using the CFX384 Touch real-time PCR detection system (Bio-Rad Laboratories). Quantification was performed according to the Pfaffl method and corrected for the efficiency of each set of primers (Pfaffl, 2001). The value of the normalized relative expression for each experimental condition was calculated in relation to values obtained for the control group and the rpl27 (reference gene).
All statistical analyzes were performed with GraphPad Prism 9 software. Statistical differences between all experimental groups were determined by one-way ANOVA, and specific differences for multiple comparisons were performed with Turkey’s test (p<0.05). These differences were considered statistically significant when a p-value <0.05 between the groups.
In our study, exposure of gilthead sea bream to air caused a rapid increase in plasma cortisol levels at 1 hps. The cortisol value remained high at 6 hps to subsequently decrease at 24 hps. Like in the plasma, in the skin mucus the cortisol level increased at 1 hps. However, a rapid decrease in concentration was observed at 6 hps and then returned to basal level at 24 hps (Figure 1).
Figure 1 Detection of cortisol levels in plasma and skin mucus for gilthead sea bream (Sparus aurata) subjected to three-minute air exposure stress. Values for (A) plasma and (B) skin mucus at 0, 1, 6, and 24 hours post-stress (hps) are plotted. Values (ng/ml) are presented as mean ± standard error (n = 8 fish per sample type and time evaluated). Significant differences between times are represented in capital letters (One-way Anova; p<0.05). Different colored bars indicate different treatments: black (control group) and red (air exposure-stressed group).
The expression of gr1 registered a slight but non-significant increase at 1 hps in gilthead sea bream subjected to air exposure acute stress. Then, the value returns to the basal level of expression. The same expression pattern was observed for the mr, noting an upregulation at 1 hps to then decrease to the control level at 6 hps. A time-dependent increase in gr1 was again detected at 24 hps. This modulation was also registered on mr at 24 hps compared with 6 hps. Importantly, the gr2 showed no sign of modulation at any time-point evaluated (Figure 2).
Figure 2 Normalized gene expression (NRE) profile in the skin of gilthead sea bream subjected to acute stress by air exposure. Modulation of cortisol receptors (gr1, gr2, mr), immune (il-1β; tnf-α; il-10; tgf-β1; lys; c3), and stress response (hsp70; enolase) were evaluated by RT-qPCR. Bar values represent the mean ± standard error (n = 8 fish per treatment and time evaluated). Time zero represents the control group (non-stressed fish; black bar). The modulation of gene expression at 1 hour, 6 hours, and 24 hours post-stress (red bars) is represented. The rpl27 was used as reference gene. The significant differences between the different times are presented with a capital letter on each bar. The absence of letters indicates that there are no significant differences in the times evaluated (one-way Anova; p<0.05). gr1, glucocorticoid receptor 1; gr2, glucocorticoid receptor 2; mr, mineralocorticoid receptor; il-1β, Interleukin 1-beta; tnf-α, tumor necrosis factor-alpha; il-10, interleukin 10; tgf-β1, tumor growth factor-beta 1; lys, lysozyme; c3, complement protein c3; hsp70, heat shock protein 70.
The immune-related genes showed no differences between the time points analyzed. However, the same modulatory behavior observed for gr1 and mr was identified for the pro-inflammatory genes il-1β, lysozyme, c3, and the anti-inflammatory genes il-10 and tgf-β1. No modulation was either observed for hsp70 and enolase (Figure 2).
The glucocorticoid receptors in gills showed the same trend of expression over time as those observed in the skin. In detail, the gr1 was upregulated at 1 hps compared to time-zero (control group). The mr also registered an upregulation at 1 hps, although not significant. Then, the kinetics of expression showed the downregulation of gr1 at 6 hps, to then increase (but not significantly) its value at 24 hps. This time-dependent pattern is also observed for mr, noting a diminishing in the expression at 6 hps, to then increase its value at 24 hps. Like in the skin, no modulation of gr2 was observed (Figure 3).
Figure 3 Normalized gene expression (NRE) profile in the gills of gilthead sea bream subjected to acute stress by air exposure. Modulation of cortisol receptors (gr1, gr2, mr), immune (il-1β; tnf-α; il-10; tgf-β1; lys; c3), and stress response (hsp70; enolase) were evaluated by RT-qPCR. Bar values represent the mean ± standard error (n = 8 fish per treatment and time evaluated). Time zero represents the control group (non-stressed fish; black bar). The modulation of gene expression at 1 hour, 6 hours, and 24 hours post-stress (red bars) is represented. The rpl27 was used as reference gene. The significant differences between the different times are presented with a capital letter on each bar. The absence of letters indicates that there are no significant differences in the times evaluated (one-way Anova; p<0.05). gr1, glucocorticoid receptor 1; gr2, glucocorticoid receptor 2; mr, mineralocorticoid receptor; il-1β, Interleukin 1-beta; tnf-α, tumor necrosis factor-alpha; il-10, interleukin 10; tgf-β1, tumor growth factor-beta; lys, lysozyme; c3, complement protein c3; hsp70, heat shock protein 70.
At the immunity level, a marked upregulation of il-1β was identified at 6 hps to then return to the basal expression value of the control. No modulation was registered for the other immune genes analyzed. From them, only tgf-β1 represented a similar trend of expression than the observed for gr1 and mr. The stress-related genes hsp70 and enolase registered no modulation of their expression profile (Figure 3).
The glucocorticoid receptors showed a different expression pattern of modulation in the anterior gut than in the skin and gills. The gr1 was no modulated at 1 and 6 hps, noting the upregulated only at 24 hps. The mr also showed an upregulation that took place at 6 hps to then drop to the value of the non-stressed fish (Figure 4).
Figure 4 Normalized gene expression (NRE) profile in the anterior gut of gilthead sea bream subjected to acute stress by air exposure. Modulation of cortisol receptors (gr1, gr2, mr), immune (il-1β; tnf-α; il-10; tgf-β1; lys; c3), and stress response (hsp70; enolase) were evaluated by RT-qPCR. Bar values represent the mean ± standard error (n = 8 fish per treatment and time evaluated). Time zero represents the control group (non-stressed fish; black bar). The modulation of gene expression at 1 hour, 6 hours, and 24 hours post-stress (red bars) is represented. The rpl27 was used as reference gene. The significant differences between the different times are presented with a capital letter on each bar. The absence of letters indicates that there are no significant differences in the times evaluated (one-way Anova; p<0.05). gr1, glucocorticoid receptor 1; gr2, glucocorticoid receptor 2; mr, mineralocorticoid receptor; il-1β, Interleukin 1-beta; tnf-α, tumor necrosis factor-alpha; il-10, interleukin 10; tgf-β1, tumor growth factor-beta; lys, lysozyme; c3, complement protein c3; hsp70, heat shock protein 70.
When we look at genes associated with immune response, three types of modulation are observed. First, there is an upregulation of pro-inflammatory genes only at 6 hps (il-1β), like the mr expression profile. Other genes (il-10; hsp70) showed the same trend although not significant. Secondly, in the same way as gr1, we observed an upregulation exclusively at 24 hps for pro-inflammatory genes (tnf-α; c3). Third, the other genes were not significant (tgf-β1; enolase), although lysozyme registered a similar not significant profile to gr2 (Figure 4).
Taking together, the data suggest that the stress response in gilthead sea bream could modulate the expression of immune- and stress-related genes preferentially by the action of one of these glucocorticoid receptors at the mucosal level.
Gilthead sea bream (S. aurata) is one of the most relevant Mediterranean fish species in terms of volume and economic value (Salomón et al., 2021). Thus, the analysis of the stress response and the modulatory mechanisms of the glucocorticoid receptors deserve attention. In this study, we evaluated for the first time the modulation of the expression of cortisol receptors (gr1; gr2; mr) in gilthead sea bream subjected to an acute stressor (three minutes of air exposure), and its eventual similarity with the expression profile of a set of stress- and immune-related genes in mucosal tissues (skin; gills; anterior gut).
Our data indicate that gr1 but not gr2 is modulated in a time-dependent manner in gilthead sea bream subjected to air exposure. This modulation is also tissue-specific, confirming previous evidence of differential responses between mucosal tissues subjected to the same stressor (Khansari et al., 2018).
In this study, we reported the differential expression profile of the gr1 and gr2 in response to three-minute air exposure stress for the first time in gilthead sea bream. We registered the upregulation of gr1 in all the mucosal tissues evaluated (skin; gills; anterior gut) in a time- and tissue-specific dependent manner. By contrast, no modulation of gr2 was detected in any of the tissues and the time-points evaluated. The glucocorticoid receptors are responsible of the cortisol recognition and its subsequent effect. The cortisol-GR complex is transported to the nucleus, where it binds to DNA by glucocorticoid response elements (GREs) present in the promoter region of several genes, interacting with co-activator molecules. In this way, the cortisol concentration is of great relevance. In fact, GR1 and GR2 can respond to different levels of cortisol concentration in fish (Bury et al., 2003; Stolte et al., 2006). It has been postulated that GR2 and MR have a higher affinity for cortisol, making possible the ligand-receptor interaction at a very low concentration of cortisol; by contrast, GR1 would be responsible for the recognition of cortisol at higher concentrations (Vazzana et al., 2010). Thus, the modulation of gr1 but not gr2 in our study might reside in the marked increase of cortisol after 1 hps, which even persists high at 6 hps in plasma. Thus, we speculate that the high level of cortisol could stimulate its recognition of cortisol by GR1 but not by GR2 in our stress model. In this way, the differential expression pattern between gr1 and gr2 observed in our study is in line with the hypothesis that neo-functionalization likely facilitated the maintenance of duplicate GRs in teleosts (Arterbery et al., 2011). The effect of the early stress response within the first hour (0-1hps) on the modulation of gr1 and gr2 remains to be elucidated.
On the other hand, the expression of mr was also modulated in a time- and tissue-dependent manner. This result should be expected taking into consideration the role of MR in the ion and water transport (McCormick and Bradshaw, 2006; Takahashi and Sakamoto, 2013). This evidence rises particular consideration taking into account the relevance of such functional processes and the permeability characteristic of the mucosal tissues (Parra et al., 2015). On the other hand, special attention requires the similarity in the expression pattern observed for gr1 and mr, and some of the genes associated with the immune and stress response. The association in the expression pattern of such genes should be analyzed in future functional works.
The measurement of cortisol in plasma and skin mucus was assessed as an indicator of the presence of the hormone at systemic and local level, respectively. The quantification of plasmatic cortisol has been the traditional method used to evaluate the response to stress in fish (Schreck and Tort, 2016). Previous evidence demonstrated that air exposure stimulates both the HPI and HSC axes in gilthead sea bream, activating the transcriptomic response and the transitory enhancement of cortisol (Skrzynska et al., 2018). The analysis of cortisol in skin mucus appears as a very useful tool to less-invasively evaluate the activity of the HPI axis of fish (Carbajal et al., 2019; Franco-Martinez et al., 2022). The identification of cortisol in skin mucus is possible because of the lipophilic property that makes it feasible to diffuse it easily through cell membranes, thus reaching different biological matrices (i.e. skin mucus) in proportion to the amount of circulating hormone when high amounts of cortisol are released in the bloodstream (Carbajal et al., 2019). In a previous study, it was observed that exposing fish to air for one minute induces the release of cortisol in plasma and skin mucus, with a peak of maximum release after 1h and 6hps, respectively (Khansari et al., 2018). In this way, the comparative stress response of gilthead sea bream with other fish species indicates that gilthead seabream has a lower activation threshold to acute stress by one-minute air exposure (Khansari et al., 2018). Thus, it is not surprising that in our results the highest duration of the acute stressor stimulus (3-minutes air exposure) could keep augmented the level of cortisol at 1 and 6 hps. In skin mucus, the cortisol peaked at 1hps and then began to decrease to reach the control level at 24 hps. The augmented but not significant cortisol level at 6 hps could be also the result of a delay in the peak of cortisol in epidermal mucus, as it has been suggested in previous studies for gilthead sea bream (Guardiola et al., 2016) and other fish species (Kroska et al., 2021). In line with this idea, the very low concentrations of cortisol detected in skin mucus makes it very sensitive to the determination of significant differences. In any case, the cortisol release profile in skin mucus registered in our study matches the level previously found in this fish species under acute stress (Khansari et al., 2019).
There is a consensus that when the stressor is acute and short-term, the response pattern is somehow stimulatory and the fish immune response shows an activating phase that especially enhances innate responses (Tort, 2011), including the upregulation of pro-inflammatory genes like complement component c3 (Demers and Bayne, 2020; Qian et al., 2020), il-1β and tnf-α (Metz et al., 2006; Hoseini et al., 2019; Su et al., 2020). Accordingly, in our study we observed the upregulation of the complement protein c3 (anterior gut), and the pro-inflammatory genes il-1β (gills; anterior gut) and tnf-α (anterior gut) in mucosal tissues. Importantly, the same il-1β expression pattern, as observed in our study at 6 hps for the anterior gut, was also registered in another study using one-minute air exposure as acute stressor (Khansari et al., 2018). However, the magnitude of the upregulation detected in our study was much higher, probably due to the application of a stressor of greater intensity (three-minute of air exposure). In the same way, our study determined a higher expression value for c3 in the anterior gut at 24 hps than the study subjecting gilthead sea bream to one-minute air exposure stress (upregulated at 6 hps) (Khansari et al., 2018). This displacement of the upregulated expression profile observed in our study might explain the marked upregulation trend but not the significant differences registered for skin at the time-points evaluated. Moreover, the higher expression magnitude of pattern for il-1β was even more marked at 6 hps for gills applying three-minute of air exposure stress. We hypothesize that this much higher magnitude of upregulation observed in our study for gills could be due to the vascular anatomy and blood-flow because of its multifunctional characteristics (i.e., respiratory, osmoregulatory, and immune processes). Thus, the high cortisol content detected in plasma at 1 hps and slightly higher at 6 hps in gilthead sea bream subjected to three-minute of air exposure stress, might promote a stronger stimulatory effect upon il-1β in gills. Anyway, it is important to take into consideration that this augment on the expression of increase of il-1β does not necessarily increase the protection against pathogenic challenges (Fast et al., 2008).
Collectively, our results suggest that gilthead sea bream subjected to an acute stressor of short duration (three minutes of air exposure) and high intensity (high cortisol level at 1hps and peaked at 6 hps) modulated the expression of gr1 but not gr2. This result agrees with previous evidence that assigns GR1 the responsibility for the recognition of cortisol at higher concentrations (Vazzana et al., 2010). In addition, our data reinforce the stimulatory effect that acute stress induces mainly in genes associated with the innate immune response but at the mucosal level, and in a time- and tissue-dependent manner.
The original contributions presented in the study are included in the article/supplementary material. Further inquiries can be directed to the corresponding authors.
The animal study was reviewed and approved by Universitat Autònoma de Barcelona.
EV-V, LT, and FER-L performed the conceptualization of the study. EV-V, BS-M, MT, LT, and FER-L carried out the experiments. EV-V, BS-M, and FER-L conducted the sampling processing for RT-qPCR. EV-V, BS-M, SR-C, JM, LT, and FER-L performed the data analysis. EV-V and FER-L conceptualized the figures. EV-V and FER-L wrote the original draft. All authors contributed to the article and approved the submitted version.
The authors thank the funding by the AGL2016-76069-C2-2- R, PID2020-117557RB-C21, and PID2020-117557RB-C22, AEI-MINECO (Spain). EV-V thanks the support of Fondecyt iniciación grant (project number 11221308; Agencia Nacional de Investigación y Desarrollo de Chile, Government of Chile). MT thanks the support of the post-doctoral fellowship “Ramón y Cajal” (ref. RYC2019-026841-I) (Ministerio de Ciencia e Innovación, Spanish Government). FER-L thanks the support of Fondecyt regular grant (project number: 1211841; Agencia Nacional de Investigación y Desarrollo de Chile, Government of Chile).
The authors declare that the research was conducted in the absence of any commercial or financial relationships that could be construed as a potential conflict of interest.
All claims expressed in this article are solely those of the authors and do not necessarily represent those of their affiliated organizations, or those of the publisher, the editors and the reviewers. Any product that may be evaluated in this article, or claim that may be made by its manufacturer, is not guaranteed or endorsed by the publisher.
Acerete L., Balasch J. C., Castellana B., Redruello B., Roher N., Canario A. V., et al. (2007). Cloning of the glucocorticoid receptor (GR) in gilthead seabream (Sparus aurata). differential expression of GR and immune genes in gilthead seabream after an immune challenge. Comp. Biochem. Physiol. - B Biochem. Mol. Biol. 148, 32–43. doi: 10.1016/j.cbpb.2007.04.015
Arends R. J., Mancera J. M., Muñoz J. L., Wendelaar Bonga S. E., Flik G. (1999). The stress response of the gilthead sea bream (Sparus aurata l.) to air exposure and confinement. J. Endocrinol. 163, 149–157. doi: 10.1677/joe.0.1630149
Arterbery A. S., Fergus D. J., Fogarty E. A., Mayberry J., Deitcher D. L., Kraus W. L., et al. (2011). Evolution of ligand specificity in vertebrate corticosteroid receptors. BMC Evol. Biol. 11, 14. doi: 10.1186/1471-2148-11-14
Bury N. R., Sturm A., Le Rouzic P., Lethimonier C., Ducouret B., Guiguen Y., et al. (2003). Evidence for two distinct functional glucocorticoid receptors in teleost fish. J. Mol. Endocrinol. 31, 141–156. doi: 10.1677/jme.0.0310141
Carbajal A., Reyes-López F. E., Tallo-Parra O., Lopez-Bejar M., Tort L. (2019). Comparative assessment of cortisol in plasma, skin mucus and scales as a measure of the hypothalamic-pituitary-interrenal axis activity in fish. Aquaculture 506, 410–416. doi: 10.1016/j.aquaculture.2019.04.005
Demers N., Bayne C. (2020). Immediate increase of plasma protein complement C3 in response to an acute stressor. Fish. Shellfish Immunol. 107, 411–413. doi: 10.1016/j.fsi.2020.10.022
Esteban M.Á., Cerezuela R. (2015). “Fish mucosal immunity: Skin,” in Mucosal health in aquaculture. Eds. Beck B. H., Peatman E. (Elsevier Inc), 67–92. doi: 10.1016/B978-0-12-417186-2.00005-4
Fast M. D., Hosoya S., Johnson S. C., Afonso L. O. B. (2008). Cortisol response and immune-related effects of Atlantic salmon ( salmo salar Linnaeus ) subjected to short- and long-term stress. Fish. Shellfish Immunol. 24, 194–204. doi: 10.1016/j.fsi.2007.10.009
Franco-Martinez L., Brandts I., Reyes-López F., Tort L., Tvarijonaviciute A., Teles M. (2022). Skin mucus as a relevant low-invasive biological matrix for the measurement of an acute stress response in rainbow trout (Oncorhynchus mykiss). Water 14, 1754. doi: 10.3390/w14111754
Guardiola F. A., Cuesta A., Esteban M. A. (2016). Using skin mucus to evaluate stress in gilthead seabream (Sparus aurata l.). Fish. Shellfish Immunol. 59, 323–330. doi: 10.1016/j.fsi.2016.11.005
Guo H., Dixon B. (2021). Understanding acute stress-mediated immunity in teleost fish. Fish. Shellfish Immunol. Rep. 2, 100010. doi: 10.1016/j.fsirep.2021.100010
Hoseini S. M., Yousefi M., Hoseinifar S. H., Van Doan H. (2019). Cytokines’ gene expression, humoral immune and biochemical responses of common carp (Cyprinus carpio, linnaeus, 1758) to transportation density and recovery in brackish water. Aquaculture 504, 13–21. doi: 10.1016/j.aquaculture.2019.01.049
Iversen M.H., Eliassen R.A. (2014). The effect of allostatic load on hypothalamic-pituitary-interrenal (HPI) axis before and after secondary vaccination in Atlantic salmon postsmolts (Salmo salar L.). Fish Physiol. Biochem. 40, 527–538. doi: 10.1007/s10695-013-9863-x
Khansari A. R., Balasch J. C., Reyes-lópez F. E., Tort L. (2017a). Stressing the inflammatory Network: Immuno-endocrine responses to allostatic load in fish. J. Mar. Sci. Res. Technol. 1–14.
Khansari A. R., Balasch J. C., Vallejos-Vidal E., Parra D., Reyes-López F. E., Tort L. (2018). Comparative immune- and stress-related transcript response induced by air exposure and vibrio anguillarum bacterin in rainbow trout (Oncorhynchus mykiss) and gilthead seabream (Sparus aurata) mucosal surfaces. Front. Immunol. 9. doi: 10.3389/fimmu.2018.00856
Khansari A. R., Balasch J. C., Vallejos-Vidal E., Teles M., Fierro-Castro C., Tort L., et al. (2019). Comparative study of stress and immune-related transcript outcomes triggered by vibrio anguillarum bacterin and air exposure stress in liver and spleen of gilthead seabream (Sparus aurata), zebrafish (Danio rerio) and rainbow trout (Oncorhynchus mykiss). Fish. Shellfish Immunol. 86, 436–448. doi: 10.1016/j.fsi.2018.11.063
Khansari A. R., Parra D., Reyes-López F. E., Tort L. (2017b). Cytokine modulation by stress hormones and antagonist specific hormonal inhibition in rainbow trout (Oncorhynchus mykiss) and gilthead sea bream (Sparus aurata) head kidney primary cell culture. Gen. Comp. Endocrinol. 250, 122–135. doi: 10.1016/j.ygcen.2017.06.005
Khansari A. R., Parra D., Reyes-López F. E., Tort L. (2017c). Modulatory in vitro effect of stress hormones on the cytokine response of rainbow trout and gilthead sea bream head kidney stimulated with vibrio anguillarum bacterin. Fish. Shellfish Immunol. 70, 736–749. doi: 10.1016/j.fsi.2017.09.009
Koppang E. O., Kvellestad A., Fischer U. (2015). “Fish mucosal immunity: Gill,” in Mucosal health in aquaculture. Eds. Beck B. H., Peatman E. (Elsevier Inc), 93–133. doi: 10.1016/B978-0-12-417186-2.00005-4
Kroska A. C., Wolf N., Planas J. V., Baker M. R., Smeltz T. S., Harris B. P. (2021). Controlled experiments to explore the use of a multi-tissue approach to characterizing stress in wild-caught pacific halibut (Hippoglossus stenolepis). Conserv. Physiol. 9, 1–9. doi: 10.1093/conphys/coab001
Liu X. H., Khansari A. R., Teles M., Martínez-Rodríguez G., Zhang Y. G., Mancera J. M., et al. (2019). Brain and pituitary response to vaccination in gilthead seabream (Sparus aurata l.). Front. Physiol. 10. doi: 10.3389/fphys.2019.00717
McCormick S. D., Bradshaw D. (2006). Hormonal control of salt and water balance in vertebrates. Gen. Comp. Endocrinol. 147, 3–8. doi: 10.1016/j.ygcen.2005.12.009
Merrifield D. L., Rodiles A. (2015). “The fish microbiome and its interactions with mucosal tissues,” in Mucosal health in aquaculture (Elsevier Inc), 273–295. doi: 10.1016/B978-0-12-417186-2/00010-8
Metz J. R., Huising M. O., Leon K., Verburg-van Kemenade B. M. L., Flik G. (2006). Central and peripheral interleukin-1β and interleukin-1 receptor I expression and their role in the acute stress response of common carp, cyprinus carpio l. J. Endocrinol. 191, 25–35. doi: 10.1677/joe.1.06640
Parra D., Reyes-Lopez F. E., Tort L. (2015). Mucosal immunity and b cells in teleosts: Effect of vaccination and stress. Front. Immunol. 6. doi: 10.3389/fimmu.2015.00354
Pfaffl M. W. (2001). A new mathematical model for relative quantification in real-time RT-PCR. Nucleic Acids Res. 29, e45. doi: 10.1093/nar/29.9.e45
Qian B., Xue L., Qi X., Bai Y., Wu Y. (2020). Gene networks and toxicity/detoxification pathways in juvenile largemouth bass (Micropterus salmoides) liver induced by acute lead stress. Genomics 112, 20–31. doi: 10.1016/j.ygeno.2019.06.023
Salinas I., Parra D. (2015). Fish mucosal immunity: intestine (Elsevier Inc). doi: 10.1016/B978-0-12-417186-2/00006-6
Salomón R., Reyes-López F. E., Tort L., Firmino J. P., Sarasquete C., Ortiz-Delgado J. B., et al. (2021). Medicinal plant leaf extract from sage and lemon verbena promotes intestinal immunity and barrier function in gilthead seabream (sparus aurata). Front. Immunol. 12. doi: 10.3389/fimmu.2021.670279
Schreck C. B., Tort L. (2016). Biology of stress in fish. Eds. Farrell A. P., Brauner C. J. (London: Academic Press).
Skrzynska A. K., Maiorano E., Bastaroli M., Naderi F., Míguez J. M., Martínez-Rodríguez G., et al. (2018). Impact of air exposure on vasotocinergic and isotocinergic systems in gilthead sea bream (Sparus aurata): New insights on fish stress response. Front. Physiol. 9. doi: 10.3389/fphys.2018.00096
Stolte E. H., Van Kemenade B. M. L. V., Savelkoul H. F. J., Flik G. (2006). Evolution of glucocorticoid receptors with different glucocorticoid sensitivity. J. Endocrinol. 190, 17–28. doi: 10.1677/joe.1.06703
Su M., Zhang R., Liu N., Zhang J. (2020). Modulation of inflammatory response by cortisol in the kidney of spotted scat (Scatophagus argus) in vitro under different osmotic stresses. Fish. Shellfish Immunol. 104, 46–54. doi: 10.1016/j.fsi.2020.05.060
Takahashi H., Sakamoto T. (2013). The role of ‘mineralocorticoids’ in teleost fish : Relative importance of glucocorticoid signaling in the osmoregulation and ‘central’ actions of mineralocorticoid receptor. Gen. Comp. Endocrinol. 181, 223–228. doi: 10.1016/j.ygcen.2012.11.016
Tort L. (2011). Stress and immune modulation in fish. Dev. Comp. Immunol. 35, 1366–1375. doi: 10.1016/j.dci.2011.07.002
Tsalafouta A., Sarropoulou E., Papandroulakis N., Pavlidis M. (2018). Characterization and expression dynamics of key genes involved in the gilthead Sea bream (Sparus aurata) cortisol stress response during early ontogeny. Mar. Biotechnol. 20, 611–622. doi: 10.1007/s10126-018-9833-5
Vallejos-Vidal E., Khansari A. R., Soliva-Dueso L., Balasch J. C., Tort L., Reyes-López F. E. (2022). The direct exposure of cortisol does not modulate the expression of immune-related genes on tissue explants of mucosal surfaces in rainbow trout (oncorhynchus mykiss) nor in gilthead Sea bream (sparus aurata). Front. Mar. Sci. 9. doi: 10.3389/fmars.2022.828050
Vargas R., Balasch J. C., Brandts I., Reyes-López F., Tort L., Teles M. (2018). Variations in the immune and metabolic response of proactive and reactive sparus aurata under stimulation with vibrio anguillarum vaccine. Sci. Rep. 8, 17352. doi: 10.1038/s41598-018-35863-w
Vazzana M., Vizzini A., Sanfratello M. A., Celi M., Salerno G., Parrinello N. (2010). Differential expression of two glucocorticoid receptors in seabass (teleost fish) head kidney after exogeneous cortisol inoculation. Comp. Biochem. Physiol. - A Mol. Integr. Physiol. 157, 49–54. doi: 10.1016/j.cbpa.2010.05.003
Keywords: Mucosal-associated lymphoid tissues (MALT), cortisol, glucocorticoid receptors (GRs), fish skin mucosa, acute stress response, fish immune response
Citation: Vallejos-Vidal E, Sanz-Milián B, Teles M, Reyes-Cerpa S, Mancera JM, Tort L and Reyes-López FE (2022) The gene expression profile of the glucocorticoid receptor 1 (gr1) but not gr2 is modulated in mucosal tissues of gilthead sea bream (Sparus aurata) exposed to acute air-exposure stress. Front. Mar. Sci. 9:977719. doi: 10.3389/fmars.2022.977719
Received: 24 June 2022; Accepted: 25 July 2022;
Published: 17 August 2022.
Edited by:
Kang-le Lu, Jimei University, ChinaReviewed by:
Carlo C. Lazado, Norwegian Institute of Food, Fisheries and Aquaculture Research (Nofima), NorwayCopyright © 2022 Vallejos-Vidal, Sanz-Milián, Teles, Reyes-Cerpa, Mancera, Tort and Reyes-López. This is an open-access article distributed under the terms of the Creative Commons Attribution License (CC BY). The use, distribution or reproduction in other forums is permitted, provided the original author(s) and the copyright owner(s) are credited and that the original publication in this journal is cited, in accordance with accepted academic practice. No use, distribution or reproduction is permitted which does not comply with these terms.
*Correspondence: Lluis Tort, TGx1aXMuVG9ydEB1YWIuZXM=; Felipe E. Reyes-López, ZmVsaXBlLnJleWVzLmxAdXNhY2guY2w=
Disclaimer: All claims expressed in this article are solely those of the authors and do not necessarily represent those of their affiliated organizations, or those of the publisher, the editors and the reviewers. Any product that may be evaluated in this article or claim that may be made by its manufacturer is not guaranteed or endorsed by the publisher.
Research integrity at Frontiers
Learn more about the work of our research integrity team to safeguard the quality of each article we publish.