- School of Marine Science, Ningbo University, Ningbo, China
Corazonin (Crz) is a neuropeptide that widely distributed in insects and crustaceans. The Crz is proposed to have pleiotropic functions in insects, but its physiological roles in crustaceans are poorly understood. In the present study, Crz and its putative receptor (CrzR) were identified from the swimming crab, Portunus trituberculatus, and their interaction was validated using the Dual-Luciferase reporter assay system. Tissue distribution analysis showed the PtCrz was mainly derived from center nerve system, while its receptor was highly expressed in Y-organ, the main site for ecdysteroids synthesis. Exposure of YO to synthetic Crz and CrzR dsRNA respectively led to the transcriptional changes of two ecdysteroidogenesis genes, further indicating a putative role of Crz signaling on ecdysteroids synthesis. During the ovarian development, the mRNA levels of PtCrz and PtCrzR increased significantly in vitellogenic stages, suggesting a potential role of Crz signaling in vitellogenesis. The hypothesis was further strengthened by in vitro experiments that the expression of vitellogenin (Vg), Vg receptor (VgR), cyclinB, and Cdc2 in ovary explants could be induced by synthetic Crz, whereas reduced by CrzR dsRNA. In addition, since 20-hydroxyecdysone also showed a stimulating effect on Vg expression, an indirect regulation of Crz signaling on ovarian development via ecdysteroids might also exist.
Introduction
Corazonin (Crz) is a neuropeptide that widely distributed in insects and crustaceans (Roch et al., 2011; Patel et al., 2014; Veenstra, 2016). Due to its structural relevance to the vertebrate gonadotropin-releasing hormone (GnRH), Crz is known as a member of the GnRH-related peptides. In arthropods, the GnRH-related peptides also include adipokinetic hormone/corazonin-related peptide (ACP) and red pigment-concentrating hormone (RPCH) (Hauser and Grimmelikhuijzen, 2014; Zandawala et al., 2015). A typical Crz prepropeptide normally consists of a predicted signal peptide, a conserved 11 amino acid corazonin mature peptide, and an additional sequence called corzaonin-precursor-related peptide (CrzRP) (Veenstra, 1994; Baggerman et al., 2002; Verleyen et al., 2006; Predel et al., 2007; Hillyer et al., 2012). In insects, there are 6 isoforms of mature Crzs, differing from each other by only one amino acid (Verleyen et al., 2006; Predel et al., 2007). Among them, the [Arg7]-corazonin is the most common Crz isoform in insects, and the only isoform found in crustaceans (Dircksen et al., 2011; Bao et al., 2015; Veenstra, 2016).
The first Crz was identified as a cardioacceleratory peptide in the cockroach Periplaneta Americana (Veenstra, 1989). Similar function was found in the blood-sucking bug Rhodnius prolixus (Patel et al., 2014), but not in other insects (Predel et al., 1999; Hillyer et al., 2012). Crz was also proposed as a regulator of color polymorphism in some locusts (Tawfik et al., 1999; Roller et al., 2003). Recently, emerging evidence suggests that Crz peptides may have broader physiological roles. For example, injection of [Arg7]-corazonin can reduce the silk spinning rate and prolong the pupal development of the silkworm Bombyx mori (Tanaka et al., 2002). The [Arg7]-corazonin contributes to the early release of ecdysis-triggering hormone (ETH) in the moth Manduca sexta, which initiates the ecdysis behavior (Kim et al., 2004). In the fruit flies, Crz is involved in sperm transfer (Hou et al., 2018) and fertility (Gospocic et al., 2017; Ben-Menahem, 2021), the ethanol intoxication behavior (Varga et al., 2016), and regulation of metabolism (Nassel et al., 2013). Compared with insects, the reports about Crz in crustaceans are limited. In the redclaw crayfish Cherax quadricarinatus, the synthetic Crz showed abilities in inducing some molting behavioral responses (Minh Nhut et al., 2020), while in the green shore crab Carcinus maenas, Crz peptide did not affect heart activity, blood glucose levels, lipid mobilization, or pigment distribution in chromatophores (Alexander et al., 2018).
Most neuropeptides perform their physiological roles by binding to specific G protein-coupled receptors (GPCRs) (Xu et al., 2016). The CrzR is a typical GPCR that was firstly identified is the fruit fly D. melanogaster (Cazzamali et al., 2002). To date, the CrzR has been identified in many insects, including the moth M. sexta (Kim et al., 2004), the malaria mosquito Anopheles gambiae (Belmont et al., 2006), the silkworm B. mori (Yang et al., 2013), the house fly Musca domestica (Sha et al., 2012), the mosquito Aedes aegypti (Oryan et al., 2018), and the blood-sucking bug R. prolixus (Hamoudi et al., 2016). The insect CrzRs are hallmarked with a DRY motif at the border of the cytoplasmic end of the third transmembrane domain and a NSXXNPXXY motif in the seventh transmembrane domain (Oldham and Hamm, 2008). Based on these features, CrzR has been found in several crustaceans, including the green shore crab C. maenas (Alexander et al., 2018), the blackback land crab Gecarcinus lateralis (Tran et al., 2019), and the spiny lobster Sagmariasus verreauxi (Buckley et al., 2016). In C. maenas, the CrzR showed high sensitivity in binding to the Crz with an EC50 value of 0.75 nM (Alexander et al., 2018).
In the present study, Crz and its putative receptor (CrzR) were identified from the swimming crab, Portunus trituberculatus, and their interaction was validated using the Dual-Luciferase reporter assay system. Based on the spatial and temporal expression patterns of PtCrzR, the involvement of Crz signaling in ecdysteroids biosynthesis and ovarian development was speculated. The hypothesis was further strengthened by Crz treatment and PtCrzR silencing in the in vitro assays.
Materials and methods
Ethical care considerations
This study was carried out in accordance with the recommendations of the Institutional Animal Care and Use Committee (IACUC) of the Ningbo University. All experimental procedures were approved by the Committee on the Ethics of Animal Experiments of the Ningbo University.
Animals and tissue sampling
Wild-caught female swimming crabs Portunus trituberculatus (body weight of 120-360 g) were purchased from local fish markets in Zhenhai, Ningbo, China. The ovarian development stage of each crab was determined according to previously reported (Wu et al., 2007). The female crabs of exogenous vitellogenic stage were placed on ice for anesthetization before sacrificed. Tissues including brain (Br), eyestalk ganglion (Es), gill (Gi), hepatopancreas (Hp), heart (Ht), Y-organ (YO), muscles (Ms), thoracic ganglion (TG), and ovaries (Ov) were collected for each crab and stored in RNA preservation fluid (CWBIO, Taizhou, Jiangsu Province, China) at -80°C.
RNA extraction, molecular clone and bioinformatics analysis
Total RNA was extracted from tissues using RNA-Solv® Reagent (Omega, USA) following the manufacturer’s instructions. The quantity and quality of the RNA were determined using a Nanodrop 2000 spectrophotometer (Thermo Scientific, USA), and the genomic DNA was removed with DNase I (RNase-free DNase I, Takara). The first cDNA was synthesized with the Perfect Real-Time PrimerScript®RT reagent Kit (Takara), and 5`/3` RACE-cDNA were synthesized with the BD SMARTer™ RACE cDNA amplification kit (Clontech). The full-length sequence was cloned according to the user manual guide for the BD SMARTer™ 5`/3`RACE kit. All PCR products were 1% agarose gel examined, purified (Sangon, Shanghai, China), cloned into PMD19-T vector (Takara), and sequenced by Zhejiang Youkang Biotechnology Co., Ltd (Hangzhou, Zhejiang Province, China). The open reading frame (ORF) was predicted using ORF finder, and the putative signal peptide was predicted using SignalP 5.0 Server program. For PtCrz, the deduced amino acid sequence was further analyzed using the NeuroPred website to predict the prohormone cleavage sites, the mature peptide and the post-translational modifications. For PtCrzR, the transmembrane regions were predicted by GPCRHMM webserver. Multiple sequence alignment was conducted using Clustal X software. The phylogenetic tree was constructed using the MEGA7.0.14 software with Neighbor-Joining (NJ) algorithm method. GenBank accession numbers of these species are shown in Supplementary Table 1.
Cell culture and transient transfection
The plasmid for transient expression was constructed by inserting the ORF of PtCrzR into the expression vector pEGFP-N1 using restriction enzymes BamHⅠ and EcoRⅠ (NEB, USA). The construct was sequenced to validate the sequence and orientation. Human embryonic kidney 293T cells (HEK293T) were cultured in high glucose dulbecco’s modified eagle medium (DMEM, Corning, USA) supplemented with 10% fetal bovine serum (Bovogen, Australia), 100 U/mL penicillin, and 100 μg/mL streptomycin (Hyclone, USA). Cells were maintained in T25 flasks at 37°C in a humidified incubator containing 5% CO2. Cells were seeded overnight in a 60 mm culture dish and transiently co-transfected with 3 μg of the PtCrzR/pEGFP-N1 plasmid, 3 μg of the reporter gene pCRE-luc, and 1.2 μg of internal control gene pRL-TK using 15 μL Lipofectamine 3000 (GLPBIO, USA) transfection reagent following the manufacturer’s instructions. The empty pEGFP-N1 plasmid was used for mock transfection.
Confocal microscopy
To verify the membrane localization of PtCrzR, the HEK293T cells transiently transfected with PtCrzR/pEGFP-N1 plasmid were used for confocal microscope analysis. The transfected cells were fixed with 4% paraformaldehyde (PFA) for 20 min, and then stained with the cell membrane probe 1,1’-dioctadecyl-3,3,3’,3’-tetramethylindocarbocyanine perchlorate (DiI, Beyotime) at 37°C for 10 min. After removing the DiI solution, the cells were washed three times with PBS and further incubated with a nuclear dye 2-(4-Amidinophenyl)-6-indolecarbamidine dihydrochloride (DAPI, Beyotime) at 37°C for 10 min. After removing the DAPI solution, the cells were washed three times with PBS, mounted in an anti-fade mounting medium (Solarbio), and imaged using a Zeiss laser scanning confocal microscope (LSM880, 294 Carl Zeiss, Oberkochen, Germany). The fluorescence detection was used green channel of 505-550 nm, blue channel of 430-490 nm and red channel of 550-585 nm with excitation of 488 nm, 405 nm and 557 nm respectively.
Dual-luciferase reporter assays
The mature PtCrz peptide (pQTFQYSRGWTN-NH2) was synthesized by Sangon Biotech (Shanghai, China), with a purity of 98%. The transfected HEK293T was co-incubated with synthetic Crz peptide in various concentrations (10-4-10-11 M). After incubation for 8 h, ligand-induced changes in luciferase activity were detected by the Dual-Luciferase® Reporter Assay System kit (Promega, USA). The dose-response curve was established and fitted in the Logistic equation utilizing GraphPad Prism 7.00.
In vitro assays
20-hydroxyecdysone (20E) was purchased from Tokyo Chemical Industry Development Co., Ltd. (Tokyo, Japan), dissolved in 95% ethanol, and then serially diluted to appropriate working concentrations using crab saline. The female crabs of exogenous vitellogenic stage were used for in vitro assays. Hepatopancreas, ovary and YO explants were cultured in 24-well plates as previously described (Xie et al., 2018). After pre-incubation for 1 h, the hepatopancreas and ovary explants were treated with synthetic Crz and 20E respectively, while the YO explants were treated with synthetic Crz. The working concentrations for synthetic Crz were 10-5 M, 10-6 M, and 10-7 M, and 0.05 μM, 0.5 μM and 5 μM for 20E. After incubated for another 8 h, tissues were collected in RNA preservation fluid for qPCR analysis. For Crz treatments, expression levels of PtVg and PtCrzR in hepatopancreas, PtVg, PtVgR, PtCrzR, PtcyclinB and PtCdc2 in ovary, and PtSpo, PtSad and PtCrzR in Y-organs were investigated. For 20E treatments, expression levels of PtVg and PtEcR in hepatopancreas, and PtVg, PtVgR, PtEcR, PtcyclinB and PtCdc2 in ovary were investigated.
RNA interference
cDNA fragments of the PtCrzR transmembrane region (565 bp) and the green fluorescent protein (GFP, 568 bp) were cloned into the pMD19-T vector (Takara), respectively. The dsRNA was prepared as previously described (Xie et al., 2016). The female crabs of exogenous vitellogenic stage were used for this experiment. The RNAi experiments were taken on ovary and YO explants, and were divided into 3 groups. The group 1 and 2 were treated with 5 μg of CrzR dsRNA and the group 3 was treated with 5 μg of GFP dsRNA. For group 1 and 3, the tissues were collected after 8 h incubation for qPCR analysis. For group 2, after 8 h incubation, the culture media was replaced by fresh M199 media containing 10-6 M of synthetic Crz peptide, and the tissues were collected after incubating for another 8 h.
Gene expression analysis
The relatively mRNA levels in the present study were determined by qPCR, using the primers listed in Supplementary Table 2. The amplification efficiency of primers was 95%-105% (Supplementary Table 3). For tissue distribution analysis, RT-PCR was also carried out to test the accuracy of qPCR. The qPCR system and condition were carried out as previously described (Xie et al., 2016). The β-actin gene was used as an internal control. The specificity of the PCR products was verified using melting curve analysis. For each sample, qPCR reactions were repeated three times. The qPCR data were calculated using the 2–ΔΔCT method (Livak and Schmittgen, 2001), and presented as the mean ± standard error (SE). The statistical differences were analyzed by one-way ANOVA followed by Tukey and Duncan test or student`s T-tests (SPSS statistics 25.0). A P value <0.05 was considered to be statistically significant different.
Results
Molecular characterization of PtCrz and PtCrzR
The deduced amino acid sequence of PtCrz consists of a signal peptide, a mature peptide, an RKR cleavage site, and a Crz precursor-related peptide (CrzRP) (Supplementary Figure 1). The predicted Crz mature sequence was pQTFQYSRGWTNamide, which is identical to the insect [Arg7]-corazonins (Supplementary Figure 2). The putative PtCrzR was predicted to have seven transmembrane helix regions (Supplementary Figure 3), and the phylogenetic analysis showed it was clustered with other known CrzR sequences (Supplementary Figure 4), belonging to the rhodopsin-like GPCR family. The sequence of the PtCrz and PtCrzR were deposited in GenBank with accession number OL694705 and OL694706.
Functional Characterization of PtCrzR
Confocal microscopy showed that PtCrzR/pEGFP-N1 mainly localized to the cell membrane (Figure 1), indicating the PtCrzR is a transmembrane protein. For ligand-receptor binding assays, the HEK293T cells were transiently co-transfected with PtCrzR/pEGFP-N1, pCRE-luc, and pRL-TK. The transfected HEK293T cells showed concentration-dependent cAMP responses when activated with Crz peptide. The median effective concentration (EC50) value for Crz was 22.91 nM (Figure 2). HEK293T cells transfected with empty vector showed no response to different concentrations of Crz peptide.
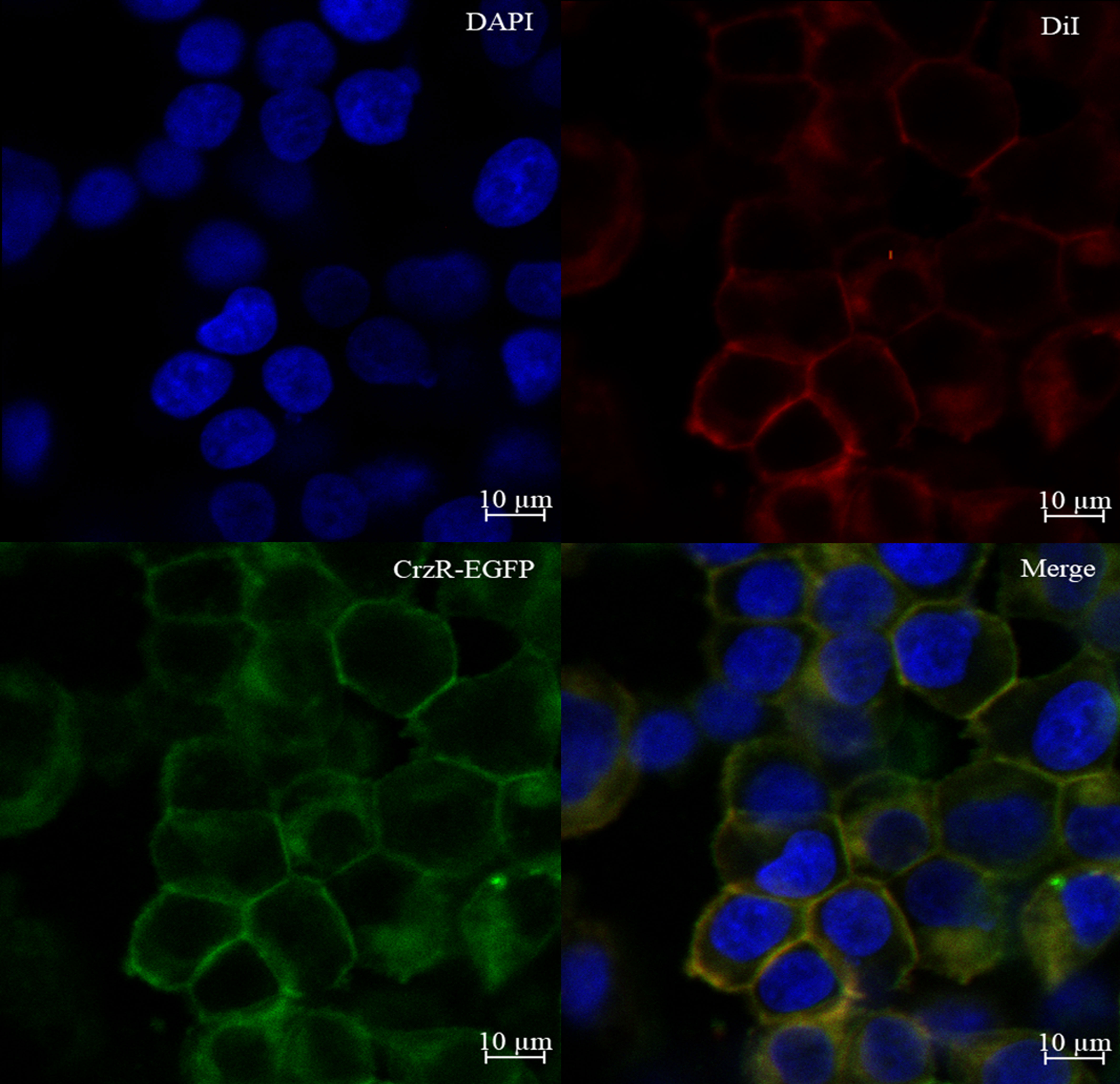
Figure 1 Expression of PtCrzR in HEK293T cells. Cells expressing PtCrzR/pEGFP-N1 fusion protein were stained with a nuclei probe (DAPI) and a membrane plasma probe (DiI).
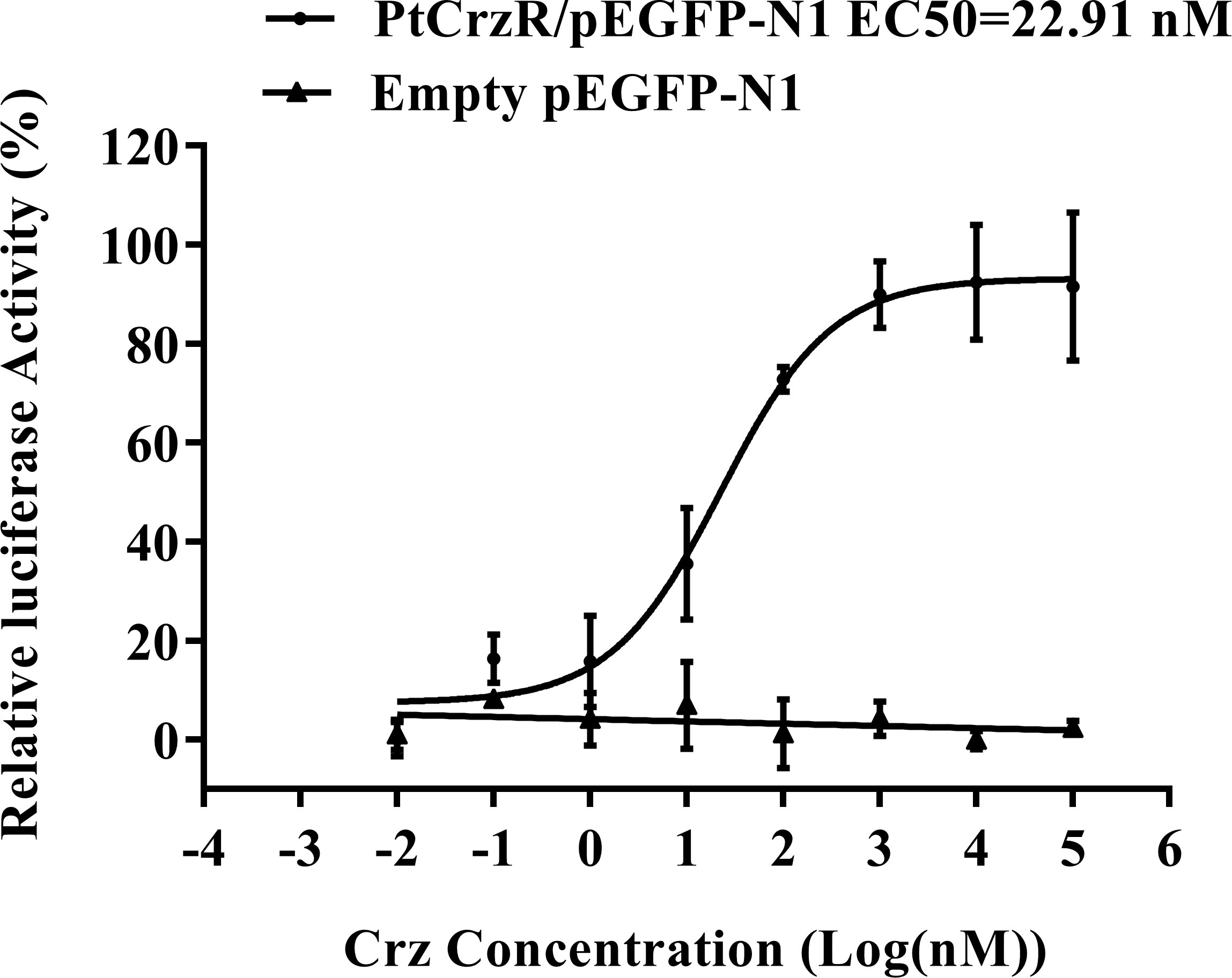
Figure 2 Dose-response curves for PtCrzR heterogeneously expressed in HEK293T cells. Different concentrations of PtCrz peptides were treated and the concentration of cAMP was determined. Each data point was a mean value ± SE from three independent biological replicates.
Spatial and temporal expression of PtCrz and PtCrzR
The mRNA expression levels of PtCrz and PtCrzR were detected in a variety of crab tissues. The level of PtCrz expression was highest in the eyestalk ganglion, and second in the brain (Figures 3A, C). The PtCrzR mRNA was at extremely high level in the Y-organ, while at much lower levels in the eyestalk ganglion, brain, ovary, gill, hepatopancreas, heart, thoracic ganglion and muscle (Figures 3B, D). During the ovarian development, the relative expression level of PtCrz in eyestalk ganglion gradually increased, and reached to a peak in the near-mature stage, while PtCrzR transcripts in Y-organ was expressed at the highest level in the exogenous vitellogenic stage, and decreased in the near-mature stage (Figure 4).
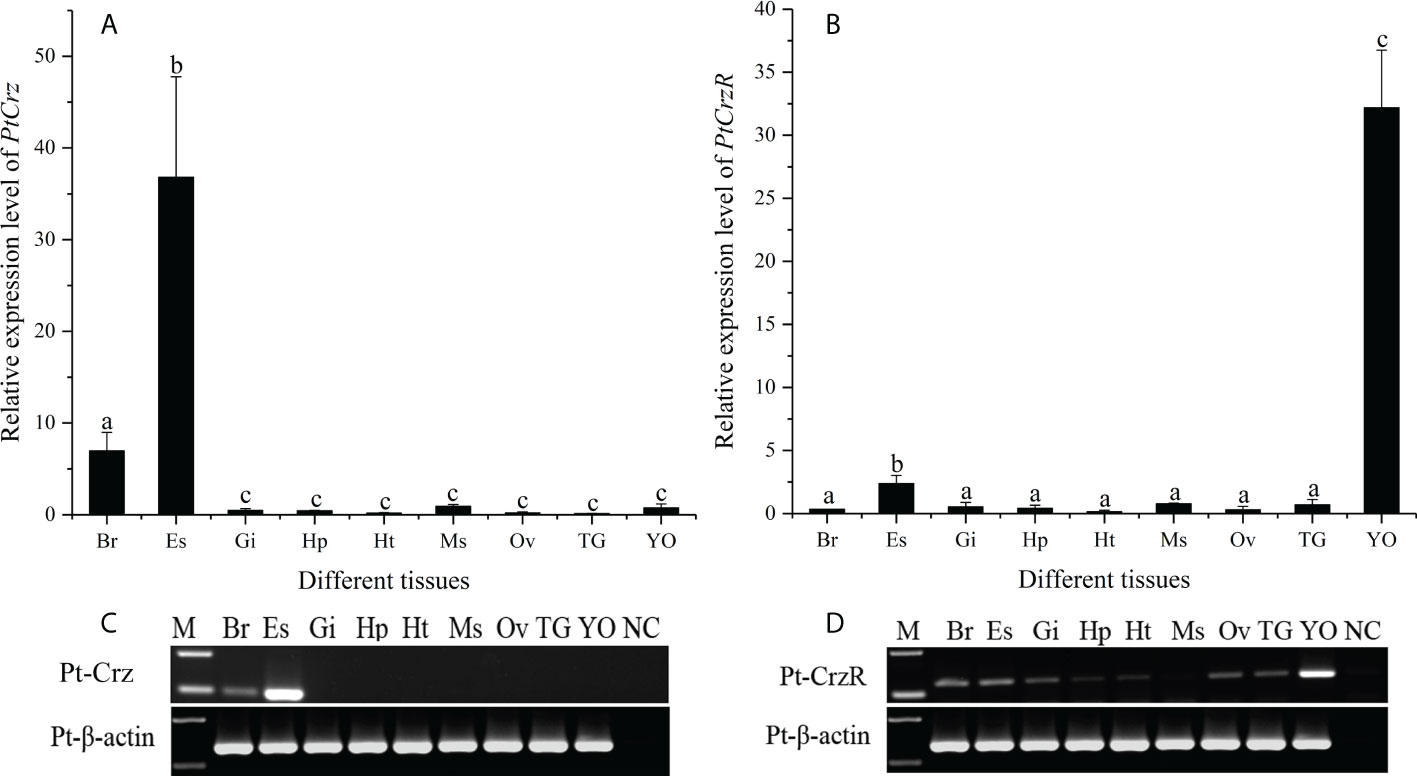
Figure 3 Tissue distribution of PtCrz (A, C) and PtCrzR (B, D) mRNA in the swimming crab (n=5). Different letters indicate statistically significant differences (P < 0.05) within each tissue. Br, brain; Es, eyestalk ganglion; Gi, gill; Hp, hepatopancreas; Ht, heart; Ms, muscle; Ov, ovary; TG, thoracic ganglion; YO, Y-organ.
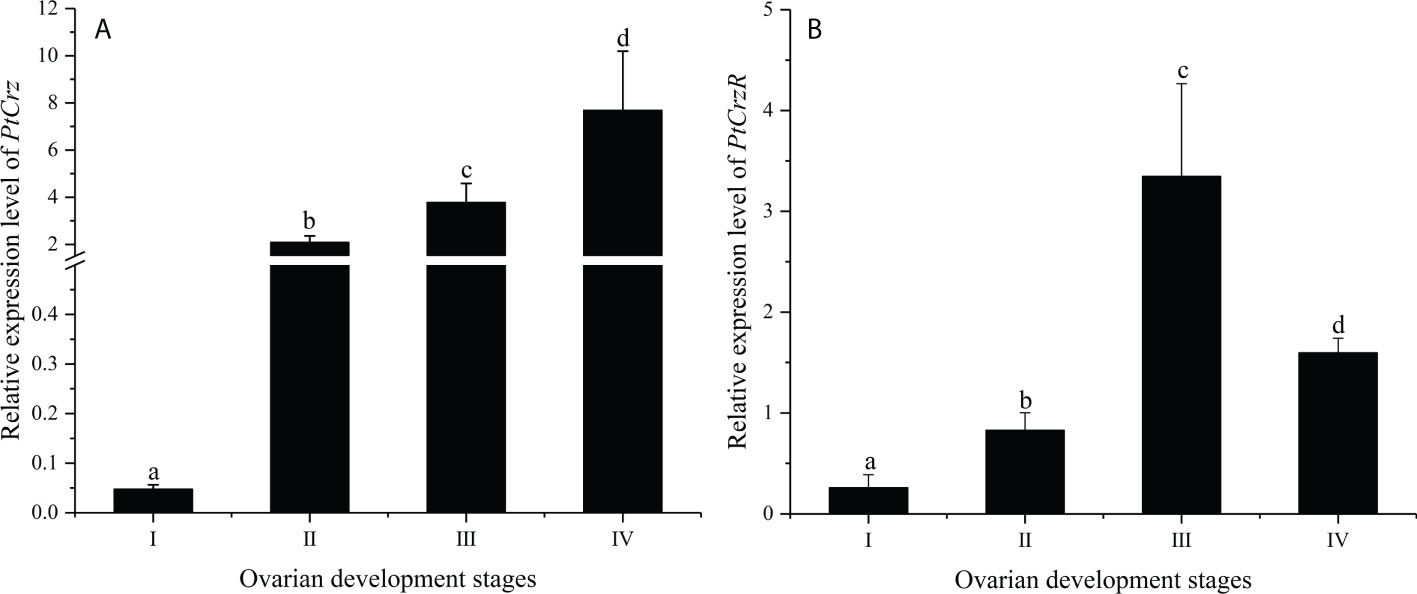
Figure 4 The expression of PtCrz (A) and PtCrzR (B) in the eyestalk ganglion and the Y-organ during the ovarian development were quantified respectively by qPCR analysis (n=4). Different letters indicate statistically significant differences (P < 0.05) within each stage. I: previtellogenic stage; II: endogenous vitellogenic stage; III: exogenous vitellogenic stage; IIV: near-mature stage.
Effects of PtCrz/PtCrzR on ecdysteroidogenesis related genes
Highly expression of PtCrzR in Y-organs suggested the Crz signaling might be involved in ecdysteroids biosynthesis. Therefore, transcriptional changes of two ecdysteroidogenesis related genes, PtSpo and PtSad, were analyzed in the following in vitro assays. The synthetic Crz could induce the expression of PtCrzR, as well as PtSpo and PtSad (Figure 5A). The CrzR dsRNA treatments showed high efficiency in interfering the PtCrzR expression, and significant decrease of the PtSpo and PtSad expression (Figure 5B).
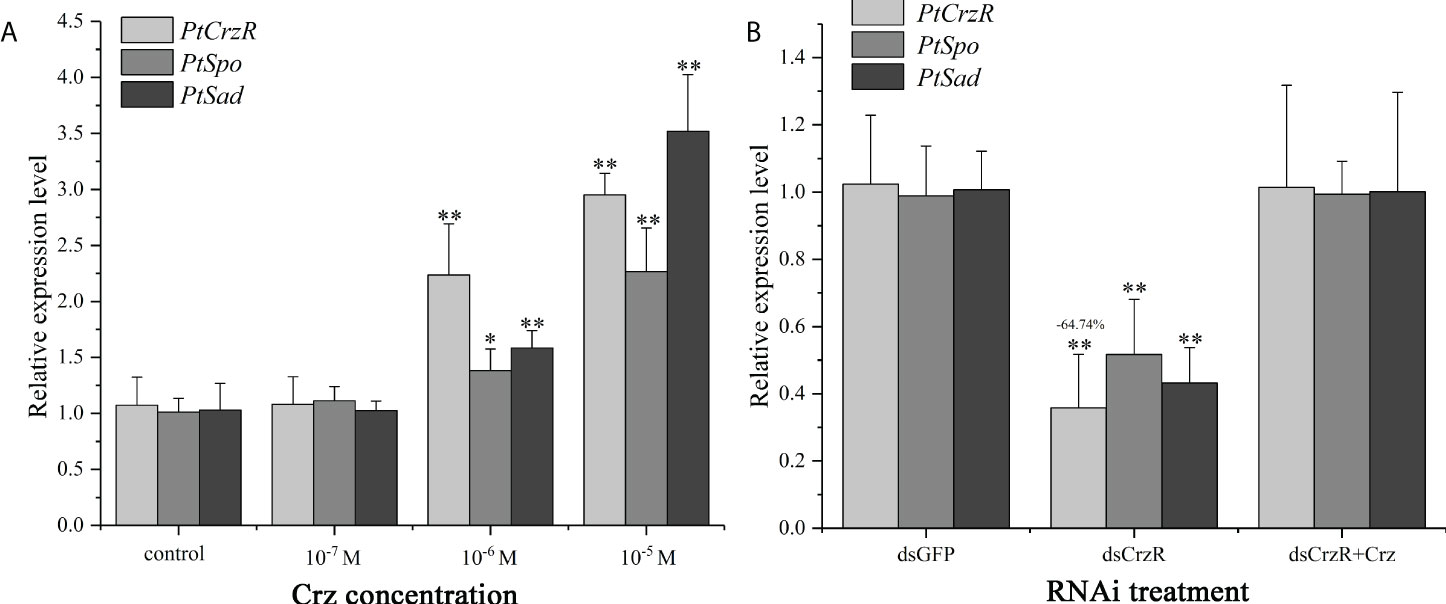
Figure 5 In vitro effect of synthetic Crz (A) or CrzR dsRNA (B) on the expression of PtCrzR, PtSpo, and PtSad in Y-organ explants from P. trituberculatus. Data are shown as mean ± SE (n=4). “*” represents significant differences (P < 0.05) and “**” represents extremely significant differences (P < 0.01) from the control group.
Effects of PtCrz/PtCrzR on genes related to ovarian development
Since the PtCrzR transcripts were also slightly expressed in hepatopancreas and ovaries, the direct effects of Crz signaling on ovarian development were also investigated. Changes in the expression of several related genes were examined in the hepatopancreas and ovary explants after treated with synthetic Crz and CrzR dsRNA, respectively. Treatment with synthetic Crz could induce the expression of PtCrzR in hepatopancreas and ovary, suggesting the Crz signaling is existed in both tissues (Figures 6A, B). However, the synthetic Crz failed to regulate the PtVg in hepatopancreas, but showed stimulatory effects on the expression of PtVg, PtVgR, PtcyclinB, and PtCdc2 in ovaries (Figures 6A, B). Expressions of these genes in ovary explants could be significantly down-regulated by the CrzR dsRNA, but the reduction could be rescued by adding Crz peptide (Figure 7).
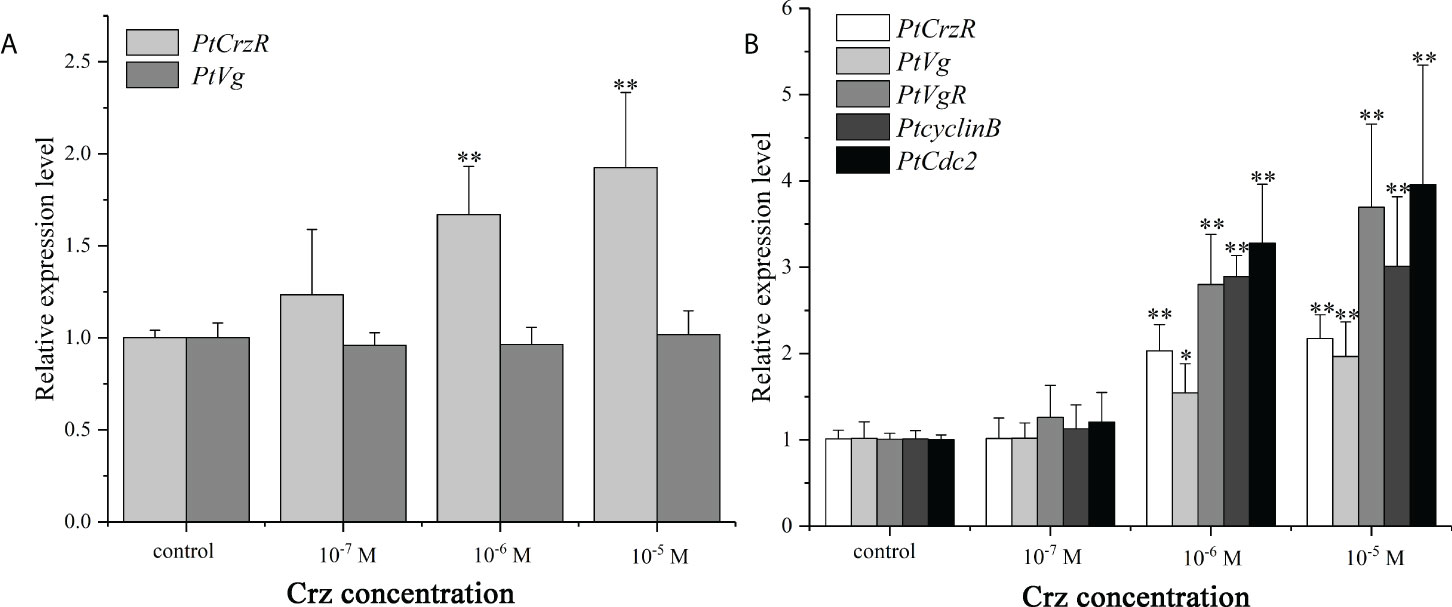
Figure 6 In vitro effect of synthetic Crz on the expression of PtCrzR and PtVg in the hepatopancreas explants (A), and the expression of PtCrzR, PtVg, PtVgR, PtcyclinB, and PtCdc2 in the ovary explants (B). Data are shown as mean ± SE (n=4). “*” represents significant differences (P < 0.05) and “**” represents extremely significant differences (P < 0.01) from the control group.
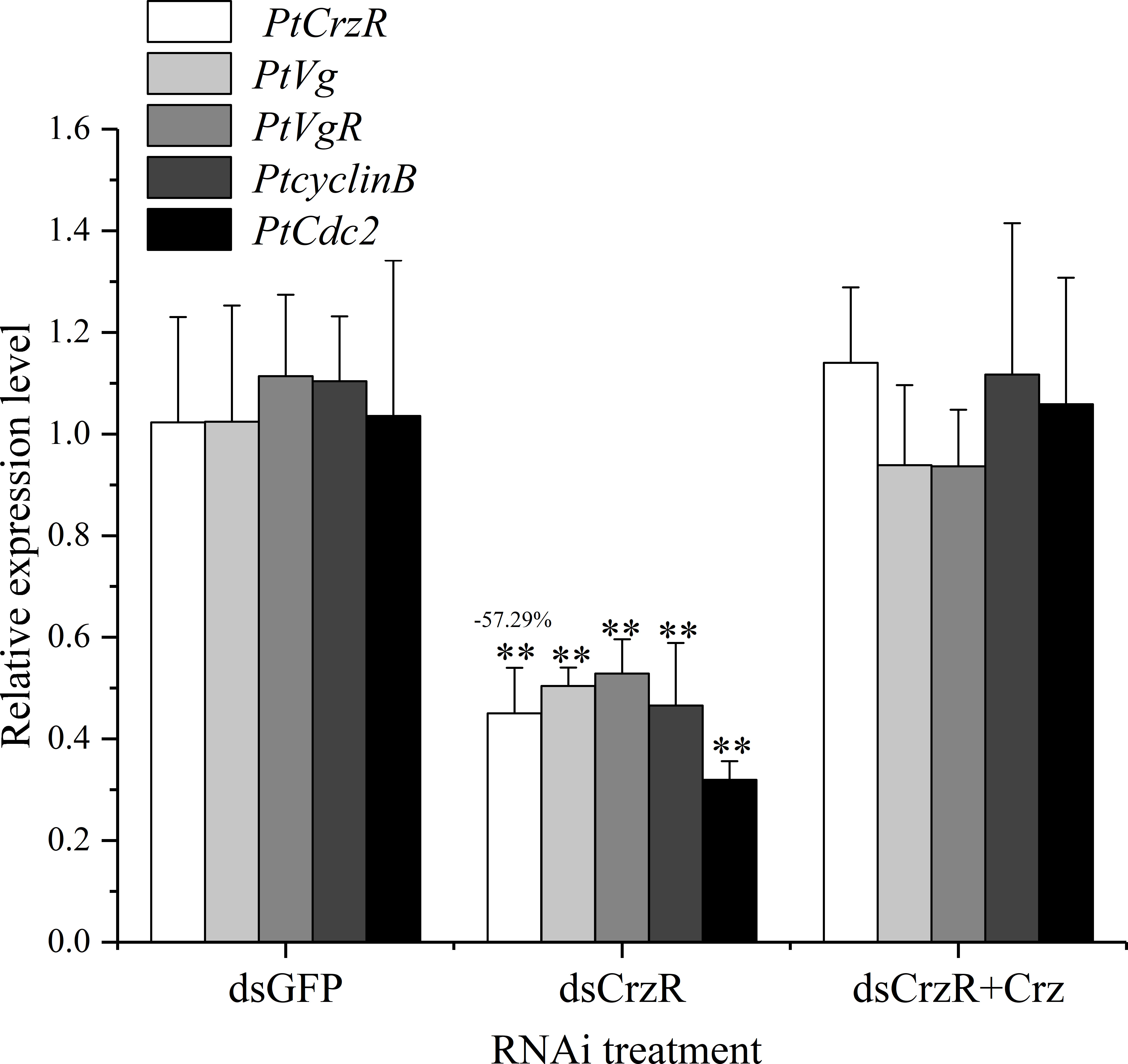
Figure 7 Effect of CrzR dsRNA on the expression of PtCrzR, PtVg, PtVgR, PtcyclinB, and PtCdc2 in the ovary explants from P. trituberculatus. Data are shown as mean ± SE (n=4). “**” represents extremely significant differences (P < 0.01) from the control group.
Effects of 20E on genes related to ovarian development
The effects of 20E on the expression of genes related to ovarian development was verified in hepatopancreas and ovary explants. Compared with the control group, the mRNA expression levels of PtEcR and PtVg were significantly up-regulated in both hepatopancreas and ovary explants. For the ovary explants, exposure to 20E induced the expression of PtcyclinB, but had no effect on PtVgR and PtCdc2 expression (Figure 8).
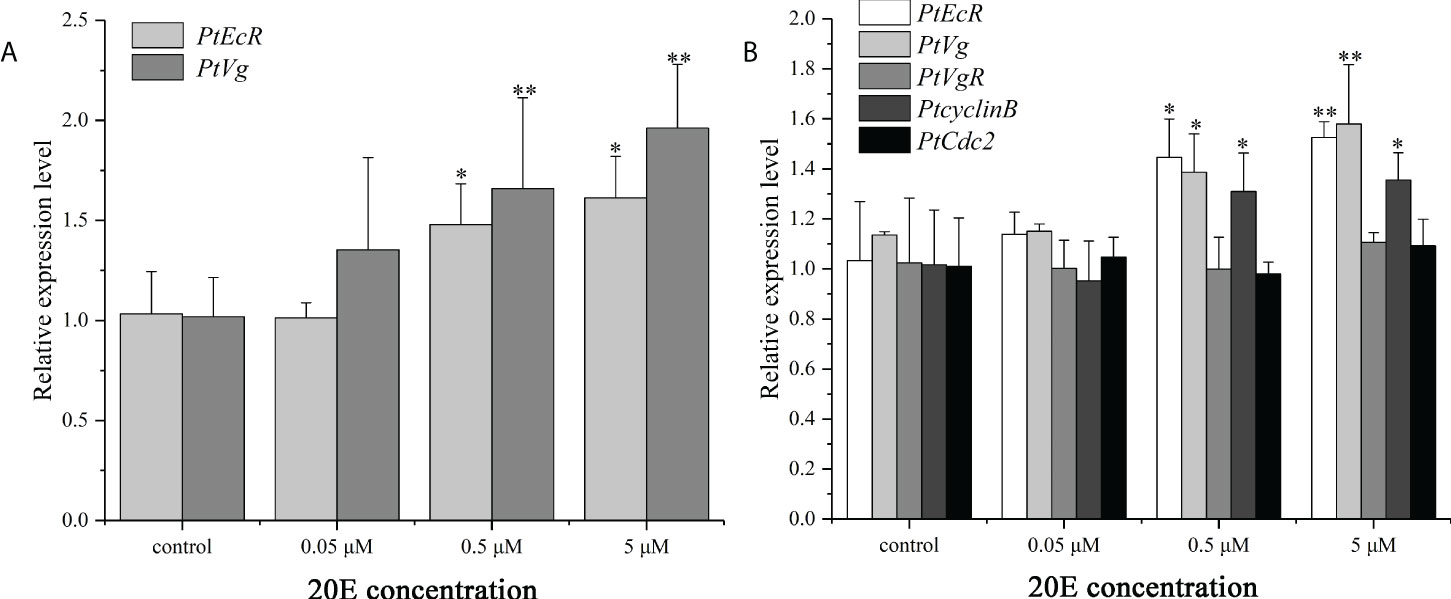
Figure 8 In vitro effect of 20E on the expression of PtEcR and PtVg in the hepatopancreas explants (A), and the expression of PtEcR, PtVg, PtVgR, PtcyclinB, and PtCdc2 in the ovary explants (B). Data are shown as mean ± SE (n=4). “*” represents significant differences (P < 0.05) and “**” represents extremely significant differences (P < 0.01) from the control group.
Discussion
Neuropeptides are the largest and most diverse group of signaling molecules in multicellular organisms, which act as neurotransmitters, neuromodulators, hormones, or growth factor, regulating various physiological processes and myriad behavioral actions (Schoofs et al., 2017). Insects and crustaceans have long been used to study the mode of action of neuropeptides, but only a few of neuropeptides are well characterized in some model species. Especially for crustaceans, the large-scale identification of neuropeptides has only emerged in recent years, relatively little is known about most neuropeptides.
The Crz is a typical peptide that were extensively studied in insects, but poorly understood in crustaceans. The mature peptide sequence of PtCrz was predicted as pQTFQYSRGWTNamide, which is identical to the known crustacean Crzs and insect [Arg7]-corazonins. Since [Arg7]-corazonins is the main isoform for insect Crzs, and since no other Crzs were identified in crustaceans, this type of Crz could be evolutionarily considered as an ancestor Crz. The tissue expression analysis showed that the PtCrz transcripts were mainly expressed in center nerve system, concurred with the CNS dominated expression of Crzs in both insects and crustaceans (Hou et al., 2017; Alexander et al., 2018; Hou et al., 2018). Compared to the conservation in sequence and distribution, the Crz function seems to be divergent between insects and crustaceans. As being a pleiotropic neuropeptide in insects, only its function in the regulation of molting behavior has been revealed in a crustacean (Minh Nhut et al., 2020). In the green shore crab, C. maenas, it was shown that the CmCrz lacks functions that proposed for insect Crzs, such as cardio regulation, glucose metabolism, lipid mobilization, and chromatophore pigment migration (Alexander et al., 2018).
Although the physiological roles of the crustacean Crz remains unclear, its receptor has been found in several species by searching for the conserved DRY and NSXXNPXXY motifs (Veenstra, 2015; Buckley et al., 2016; Alexander et al., 2018). Given the importance of a receptor in signal transduction, the behavioral patterns of CrzR may be indicative for the possible functions of Crz peptides. In this study, the putative PtCrzR sequence was therefore identified. In addition to the membrane location and evolutionary conservation, the obtained PtCrzR could be activated by the synthetic Crz in a dose-dependent manner, with EC50 of 22.91 nM and which resulted in cAMP accumulation. In further in vitro assays, the gene expressions that induced by the synthetic Crz treatment were reduced by PtCrzR silencing, again suggesting the obtained PtCrzR is a bona fide receptor for PtCrz. However, it should be noted that this reduction could be rescued by adding Crz in CrzR dsRNA treatment. Since it is common for a neuropeptide to activate two or more related receptors (Schoofs et al., 2017), our results might suggest the existence of other Crz receptors.
The PtCrzR was strongly expressed in Y-organ, and the level was significantly higher than other tissues. As the molting gland in crustacean, the Y-organ is mainly responsible for the production of ecdysteroids. Therefore, our results elicited an indication that the Crz might be involved in regulating the ecdysteroidogenesis. Similar distribution was also observed for the CrzR of C. maenas (Alexander et al., 2018), but in that study, application of Crz peptide to Y-organ had barely effect on ecdysteroids secretion. In this study, interestingly, contrary evidence might be presented, as the gene expressions of PtSpo and PtSad in Y-organ explants were remarkably induced by Crz treatment, whereas reduced by PtCrzR silencing. As members of Halloween gene family, Spo and Sad encode cytochrome P450 enzymes CYP307A1 and CYP315A1, respectively, which are responsible for the early and late steps of ecdysteroids biosynthesis (Mykles, 2011). However, although the transcription levels of the Halloween genes have been shown to be closely related to ecdysone levels in many studies (Iga et al., 2010; Xie et al., 2016; Yang et al., 2021), future studies to detect the ecdysone levels after Crz treatment are still needed to confirm the stimulatory effects of Crz peptide.
The expressions of PtCrz and PtCrzR were observed to increase during the vitellogenic stages, whereas the PtCrzR expression declined in the near-mature stage, which might suggest a potential role of Crz signaling in vitellogenesis. Combined with the possible effects of Crz on ecdysteroidogenesis, this result could be reminiscent of the vitellogenic promoting role for 20-hydroxyecdysone (20E), the main active form of ecdysteroids (Li et al., 2000; Tiu et al., 2010; Gong et al., 2015; Yang and Liu, 2021). In this study, 20E treatment induced the Vg expression in both hepatopancreas and ovary explants, further illustrating its role in the ovarian development of P. trituberculatus. However, as mentioned in last paragraph, whether Crz signaling can regulate ovarian development via ecdysteroids needs more evidences in hormone levels.
Since the PtCrzR transcripts were also slightly expressed in hepatopancreas and ovaries, the direct effects of Crz signaling on ovarian development should not be ignored. The increase in PtCrzR expression in hepatopancreas and ovary exposed to Crz indicated the existence of Crz signaling in both tissues. However, only the Vg expression in ovaries but not hepatopancreas was induced by Crz exposure, which may suggest other switch pathways for Crz signaling. As a GnRH-like peptide, the effects of Crz on ovarian development were compared with those from RPCH, another member in this group. In the whiteleg shrimp Litopenaeus vannamei, in vivo injection of RPCH caused significant increase in ovarian Vg mRNA levels (Chen et al., 2018). Similar results were also observed in mud crab Scylla paramamosain, but the induction of Vg in vitro required the presence of nerve tissues (Zeng et al., 2016). It was also found in S. paramamosain that in vivo treatment of RPCH could also induced the Vg expression in hepatopancreas, which was not observed in our in vitro studies.
In addition to Vg, several other ovarian-related genes were also selected to investigate their transcriptional response to the Crz exposure. Among them, the VgR is a crucial protein in mediating the endocytosis which incorporating the exogenous Vg into developing oocytes (Zmora et al., 2007). As for cyclinB and Cdc2, which are components of maturation promoting factor (MPF), emerging evidences indicated they are also involved in the oocyte maturation of crustaceans (Fang et al., 2009; Qiu and Liu, 2009; Han et al., 2012). It was observed that the expressions of VgR, cyclinB, and Cdc2 were also up-regulated by synthetic Crz. This induction was further confirmed by treating with CrzR dsRNA, which might suggest an integrative role of Crz signaling during ovarian development. Moreover, this result conforms to the properties of CrzR as a GPCR, as in many studies, the intracellular cAMP level plays pivotal roles in regulating the expression of Vg, cyclinB, and Cdc2 (Chen et al., 2018; Mani et al., 2019; Feng et al., 2021; Wan et al., 2022).
Although the Crz peptides are structurally related to the vertebrate GnRHs (Hauser and Grimmelikhuijzen, 2014; Zandawala et al., 2015), the present study was the first to explore its putative role in ovarian development. Comparably, another GnRH-related peptide RPCH has been reported to be involved in ovarian development in several species (Zandawala et al., 2015; Zeng et al., 2016; Chen et al., 2018; Ben-Menahem, 2021). Interestingly, although the RPCHR has been identified from several crabs (Alexander et al., 2018; Ma et al., 2018), its paralogous sequence cannot be found in the transcriptome (Tu et al., 2021) and the genome of P. trituberculatus (NCBI database: PRJNA555262). In addition, another study in our group has confirmed the ligand activation of PtCrzR by RPCH (data not shown), which suggests that the RPCH might use the PtCrzR as its receptor in P. trituberculatus. It would be intriguing to define the overlapping roles of these two peptides, as well as their crosstalk during signal transduction.
Data availability statement
The datasets presented in this study can be found in online repositories. The names of the repository/repositories and accession number(s) can be found in the article/Supplementary Material.
Ethics statement
The animal study was reviewed and approved by Committee on the Ethics of Animal Experiments of the Ningbo University.
Author contributions
ST: conceptualization, sample collection, experiment, data analysis and writing-original draft. FG and YH: sample collection and experiment. MW: experiment. XX and DZ: editing and supervision. All authors contributed to the article and approved the submitted version.
Funding
This study was supported by the National natural Science Foundation of China (Nos. 41776165 and 31802265), Natural Science Foundation of Zhejiang province (LY20C190004), and the K. C. Wong Magna Fund in Ningbo University.
Conflict of interest
The authors declare that the research was conducted in the absence of any commercial or financial relationships that could be construed as a potential conflict of interest.
Publisher’s note
All claims expressed in this article are solely those of the authors and do not necessarily represent those of their affiliated organizations, or those of the publisher, the editors and the reviewers. Any product that may be evaluated in this article, or claim that may be made by its manufacturer, is not guaranteed or endorsed by the publisher.
Supplementary material
The Supplementary Material for this article can be found online at: https://www.frontiersin.org/articles/10.3389/fmars.2022.976754/full#supplementary-material
References
Alexander J. L., Oliphant A., Wilcockson D. C., Audsley N., Down R. E., Lafont R., et al. (2018). Functional characterization and signaling systems of corazonin and red pigment concentrating hormone in the green shore crab, Carcinus maenas. Front. Neurosci. 11. doi: 10.3389/fnins.2017.00752
Baggerman G., Cerstiaens A., De Loof A., Schoofs L. (2002). Peptidomics of the larval Drosophila melanogaster central nervous system. J. Biol. Chem. 277, 40368–40374. doi: 10.1074/jbc.M206257200
Bao C., Yang Y., Huang H., Ye H. (2015). Neuropeptides in the cerebral ganglia of the mud crab, Scylla paramamosain: Transcriptomic analysis and expression profiles during vitellogenesis. Sci. Rep. 5, 17055. doi: 10.1038/srep17055
Belmont M., Cazzamali G., Williamson M., Hauser F., Grimmelikhuijzen C. J. (2006). Identification of four evolutionarily related G protein-coupled receptors from the malaria mosquito Anopheles gambiae. Biochem. Biophys. Res. Commun. 344, 160–165. doi: 10.1016/j.bbrc.2006.03.117
Ben-Menahem D. (2021). GnRH-related neurohormones in the fruit fly Drosophila melanogaster. Int. J. Molr. Sci. 22, 5035. doi: 10.3390/ijms22095035
Buckley S. J., Fitzgibbon Q. P., Smith G. G., Ventura T. (2016). In silico prediction of the G-protein coupled receptors expressed during the metamorphic molt of Sagmariasus verreauxi (Crustacea: Decapoda) by mining transcriptomic data: RNA-seq to repertoire. Gen. Comp. Endocrinol. 228, 111–127. doi: 10.1016/j.ygcen.2016.02.001
Cazzamali G., Saxild N., Grimmelikhuijzen C. (2002). Molecular cloning and functional expression of a drosophila corazonin receptor. Biochem. Biophys. Res. Commun. 298, 31–36. doi: 10.1016/s0006-291x(02)02398-7
Chen H.-Y., Kang B. J., Sultana Z., Wilder M. N. (2018). Molecular cloning of red pigment-concentrating hormone (RPCH) from eyestalks of the whiteleg shrimp (Litopenaeus vannamei): Evaluation of the effects of the hormone on ovarian growth and the influence of serotonin (5-HT) on its expression. Aquaculture 495, 232–240. doi: 10.1016/j.aquaculture.2018.04.027
Chen T., Ren C., Jiang X., Zhang L., Li H., Huang W., et al. (2018). Mechanisms for type-II vitellogenesis-inhibiting hormone suppression of vitellogenin transcription in shrimp hepatopancreas: Crosstalk of GC/cGMP pathway with different MAPK-dependent cascades. PloS One 13, e0194459. doi: 10.1371/journal.pone.0194459
Dircksen H., Neupert S., Predel R., Verleyen P., Huybrechts J., Strauss J., et al. (2011). Genomics, transcriptomics, and peptidomics of Daphnia pulex neuropeptides and protein hormones. J. Proteome Res. 10, 4478–4504. doi: 10.1021/pr200284e
Fang J. J., Qiu G. F. (2009). Molecular cloning of cyclin b transcript with an unusually long 3' untranslation region and its expression analysis during oogenesis in the Chinese mitten crab, Eriocheir sinensis. Mol. Biol. Rep. 36, 1521–1529. doi: 10.1007/s11033-008-9346-9
Feng Q. M., Liu M. M., Cheng Y. X., Wu X. G. (2021). Comparative proteomics elucidates the dynamics of ovarian development in the Chinese mitten crab Eriocheir sinensis. Comp. Biochem. Physiol. Part D. Genomics Proteomics 40, 100878. doi: 10.1016/j.cbd.2021.100878
Gong J., Ye H., Xie Y., Yang Y., Huang H., Li S., et al. (2015). Ecdysone receptor in the mud crab Scylla paramamosain: A possible role in promoting ovarian development. J. Endocrinol. 224, 273–287. doi: 10.1530/joe-14-0526
Gospocic J., Shields E. J., Glastad K. M., Lin Y., Penick C. A., Yan H., et al. (2017). The neuropeptide corazonin controls social behavior and caste identity in ants. Cell 170, 748–759. doi: 10.1016/j.cell.2017.07.014
Hamoudi Z., Lange A. B., Orchard I. (2016). Identification and characterization of the corazonin receptor and possible physiological roles of the corazonin-signaling pathway in Rhodnius prolixus. Front. Neurosci. 10. doi: 10.3389/fnins.2016.00357
Han K., Dai Y., Zou Z., Fu M., Wang Y., Zhang Z. (2012). Molecular characterization and expression profiles of cdc2 and cyclin b during oogenesis and spermatogenesis in green mud crab (Scylla paramamosain). Comp. Biochem. Physiol. B. Biochem. Mol. Biol. 163, 292–302. doi: 10.1016/j.cbpb.2012.07.001
Hauser F., Grimmelikhuijzen C. J. (2014). Evolution of the AKH/corazonin/ACP/GnRH receptor superfamily and their ligands in the protostomia. Gen. Comp. Endocrinol. 209, 35–49. doi: 10.1016/j.ygcen.2014.07.009
Hillyer J. F., Estevez-Lao T. Y., Funkhouser L. J., Aluoch V. A. (2012). Anopheles gambiae corazonin: Gene structure, expression and effect on mosquito heart physiology. Insect Mol. Biol. 21, 343–355. doi: 10.1111/j.1365-2583.2012.01140.x
Hou Q. L., Chen E. H., Jiang H. B., Yu S. F., Yang P. J., Liu X. Q., et al. (2018). Corazonin signaling is required in the male for sperm transfer in the oriental fruit fly Bactrocera dorsalis. Front. Physiol. 9. doi: 10.3389/fphys.2018.00660
Hou Q.-L., Jiang H.-B., Gui S.-H., Chen E.-H., Wei D.-D., Li H.-M., et al. (2017). A role of corazonin receptor in larval-pupal transition and pupariation in the oriental fruit fly Bactrocera dorsalis (Hendel) (Diptera: Tephritidae). Front. Physiol. 8. doi: 10.3389/fphys.2017.00077
Iga M., Smagghe G. (2010). Identification and expression profile of Halloween genes involved in ecdysteroid biosynthesis in Spodoptera littoralis. Peptides 31, 456–467. doi: 10.1016/j.Peptides.2009.08.002
Kim Y. J., Spalovska-Valachova I., Cho K. H., Zitnanova I., Park Y., Adams M. E., et al. (2004). Corazonin receptor signaling in ecdysis initiation. Proc. Natl. Acad. Sci. U.S.A. 101, 6704–6709. doi: 10.1073/pnas.0305291101
Li C., Kapitskaya M. Z., Zhu J., Miura K., Segraves W., Raikhel A. S. (2000). Conserved molecular mechanism for the stage specificity of the mosquito vitellogenic response to ecdysone. Dev. Biol. 224, 96–110. doi: 10.1006/dbio.2000.9792
Livak K. J., Schmittgen T. D. (2001). Analysis of relative gene expression data using real-time quantitative PCR and the 2(-delta delta C(T)) method. Methods 25, 402–408. doi: 10.1006/meth.2001.1262
Mani T., Subramaniya B. R., Chidambaram Iyer S., Sivasithamparam N. D., Devaraj H. (2019). Modulation of complex coordinated molecular signaling by 5-HT and a cocktail of inhibitors leads to ovarian maturation of Penaeus monodon in captivity. Mol. Reprod. Dev. 86, 576–591. doi: 10.1002/mrd.23135
Ma K. Y., Zhang S. F., Wang S. S., Qiu G. F. (2018). Molecular cloning and characterization of a gonadotropin-releasing hormone receptor homolog in the Chinese mitten crab, Eriocheir sinensis. Gene 665, 111–118. doi: 10.1016/j.gene.2018.05.006
Minh Nhut T., Mykles D. L., Elizur A., Ventura T. (2020). Ecdysis triggering hormone modulates molt behaviour in the redclaw crayfish Cherax quadricarinatus, providing a mechanistic evidence for conserved function in molt regulation across pancrustacea. Gen. Comp. Endocrinol. 298, 113556. doi: 10.1016/j.ygcen.2020.113556
Mykles D. L. (2011). Ecdysteroid metabolism in crustaceans. J Steroid Biochem Mol. Biol. 127, 196–203. doi: 10.1016/j.jsbmb.2010.09.001
Nassel D. R., Kubrak O. I., Liu Y., Luo J., Lushchak O. V. (2013). Factors that regulate insulin producing cells and their output in drosophila. Front. Physiol. 4. doi: 10.3389/fphys.2013.00252
Oldham W. M., Hamm H. E. (2008). Heterotrimeric G protein activation by G-protein-coupled receptors. Nat. Rev. Mol. Cell Biol. 9, 60–71. doi: 10.1038/nrm2299
Oryan A., Wahedi A., Paluzzi J. V. (2018). Functional characterization and quantitative expression analysis of two GnRH-related peptide receptors in the mosquito, Aedes aegypti. Biochem. Biophys. Res. Commun. 497, 550–557. doi: 10.1016/j.bbrc.2018.02.088
Patel H., Orchard I., Veenstra J. A., Lange A. B. (2014). The distribution and physiological effects of three evolutionarily and sequence-related neuropeptides in Rhodnius prolixus: Adipokinetic hormone, corazonin and adipokinetic hormone/corazonin-related peptide. Gen. Comp. Endocrinol. 195, 1–8. doi: 10.1016/j.ygcen.2013.10.012
Predel R., Kellner R., Gade G. (1999). Myotropic neuropeptides from the retrocerebral complex of the stick insect, Carausius morosus (Phasmatodea : Lonchodidae). Eur. J. Entomology. 96, 275–278. doi: 10.1046/j.1570-7458.1999.00427.x
Predel R., Neupert S., Russell W. K., Scheibner O., Nachman R. J. (2007). Corazonin in insects. Peptides 28, 3–10. doi: 10.1016/j.peptides.2006.10.011
Qiu G. F., Liu P. (2009). On the role of Cdc2 kinase during meiotic maturation of oocyte in the Chinese mitten crab, Eriocheir sinensis. Comp. Biochem. Physiol. B. Biochem. Mol. Biol. 152, 243–248. doi: 10.1016/j.cbpb.2008.12.004
Roch G. J., Busby E. R., Sherwood N. M. (2011). Evolution of GnRH: diving deeper. Gen. Comp. Endocrinol. 171, 1–16. doi: 10.1016/j.ygcen.2010.12.014
Roller L., Tanaka Y., Tanaka S. (2003). Corazonin and corazonin-like substances in the central nervous system of the pterygote and apterygote insects. Cell Tissue Res. 312, 393–406. doi: 10.1007/s00441-003-0722-4
Schoofs L., De Loof A., Van Hiel M. B. (2017). Neuropeptides as regulators of behavior in insects. Annu. Rev. Entomol. 62, 35–52. doi: 10.1146/annurev-ento-031616-035500
Sha K., Conner W. C., Choi D. Y., Park J. H. (2012). Characterization, expression, and evolutionary aspects of corazonin neuropeptide and its receptor from the house fly, Musca domestica (Diptera: Muscidae). Gene 497, 191–199. doi: 10.1016/j.gene.2012.01.052
Tanaka Y., Hua Y., Roller L., Tanaka S. (2002). Corazonin reduces the spinning rate in the silkworm, Bombyx mori. J. Insect Physiol. 48, 707–714. doi: 10.1016/s0022-1910(02)00094-x
Tawfik A. I., Tanaka S., De Loof A., Schoofs L., Baggerman G., Waelkens E., et al. (1999). Identification of the gregarization-associated dark-pigmentotropin in locusts through an albino mutant. Proc. Natl. Acad. Sci. U.S.A. 96, 7083–7087. doi: 10.1073/pnas.96.12.7083
Tiu S. H.-K., Chan S.-M., Tobe S. S. (2010). The effects of farnesoic acid and 20-hydroxyecdysone on vitellogenin gene expression in the lobster, Homarus americanus, and possible roles in the reproductive process. Gen. Comp. Endocrinol. 166, 337–345. doi: 10.1016/j.ygcen.2009.11.005
Tran N. M., Mykles D. L., Elizur A., Ventura T. (2019). Characterization of G-protein coupled receptors from the blackback land crab Gecarcinus lateralis y organ transcriptome over the molt cycle. BMC Genomics 20, 74. doi: 10.1186/s12864-018-5363-9
Tu S., Xu R., Wang M., Xie X., Bao C., Zhu D. (2021). Identification and characterization of expression profiles of neuropeptides and their GPCRs in the swimming crab, Portunus trituberculatus. PeerJ 9, e12179. doi: 10.7717/peerj.12179
Varga K., Nagy P., Arsikin Csordas K., Kovacs A. L., Hegedus K., Juhasz G. (2016). Loss of Atg16 delays the alcohol-induced sedation response via regulation of corazonin neuropeptide production in drosophila. Sci. Rep. 6, 34641. doi: 10.1038/srep34641
Veenstra J. A. (1989). Isolation and structure of corazonin, a cardioactive peptide from the american cockroach. FEBS Lett. 250, 231–234. doi: 10.1016/0014-5793(89)80727-6
Veenstra J. A. (1994). Isolation and structure of the drosophila corazonin gene. Biochem. Biophys. Res. Commun. 204, 292–296. doi: 10.1006/bbrc.1994.2458
Veenstra J. A. (2015). The power of next-generation sequencing as illustrated by the neuropeptidome of the crayfish Procambarus clarkii. Gen. Comp. Endocrinol. 224, 84–95. doi: 10.1016/j.ygcen.2015.06.013
Veenstra J. A. (2016). Similarities between decapod and insect neuropeptidomes. PeerJ 4, e2043. doi: 10.7717/peerj.2043
Verleyen P., Baggerman G., Mertens I., Vandersmissen T., Huybrechts J., Van Lommel A., et al. (2006). Cloning and characterization of a third isoform of corazonin in the honey bee Apis mellifera. Peptides 27, 493–499. doi: 10.1016/j.peptides.2005.03.065
Wan H., Zhong J., Zhang Z., Zou P., Wang Y. (2022). Comparative transcriptome reveals the potential modulation mechanisms of spfoxl-2 affecting ovarian development of Scylla paramamosain. Mar. Biotechnol. (NY). 24, 125–135. doi: 10.1007/s10126-022-10091-6
Wu X. G., Yao G. G., Yang X. Z., Cheng Y. X., Wang C. L. (2007). A study on the ovarian development of Portunus trituberculatus in East China Sea during the first reproductive cycle. Acta Oceanologica. Sin. 29, 120–127. doi: 10.3321/j.issn:0253-4193.2007.04.014
Xie X., Liu M., Jiang Q., Zheng H., Zheng L., Zhu D. (2018). Role of kruppel homolog 1 (Kr-h1) in methyl farnesoate-mediated vitellogenesis in the swimming crab Portunus trituberculatus. Gene 679, 260–265. doi: 10.1016/j.gene.2018.08.046
Xie X., Liu Z., Liu M., Tao T., Shen X., Zhu D. (2016). Role of Halloween genes in ecdysteroids biosynthesis of the swimming crab (Portunus trituberculatus): Implications from RNA interference and eyestalk ablation. Comp. Biochem. Physiol. A. Mol. Integr. Physiol. 199, 105–110. doi: 10.1016/j.cbpa.2016.06.001
Xu G., Gu G. X., Teng Z. W., Wu S. F., Huang J., Song Q. S., et al. (2016). Identification and expression profiles of neuropeptides and their G protein-coupled receptors in the rice stem borer Chilo suppressalis. Sci. Rep. 6, 28976. doi: 10.1038/srep28976
Yang J., Huang H., Yang H., He X., Jiang X., Shi Y., et al. (2013). Specific activation of the G protein-coupled receptor BNGR-A21 by the neuropeptide corazonin from the silkworm, Bombyx mori, dually couples to the g(q) and g(s) signaling cascades. J. Biol. Chem. 288, 11662–11675. doi: 10.1074/jbc.M112.441675
Yang X., Liu J. (2021). Effect of 20-hydroxyecdysone on vitellogenesis in the ixodid tick Haemaphysalis longicornis. Int. J. Acarol. 48, 20–26. doi: 10.1080/01647954.2021.2009568
Yang Z. M., Yu N., Wang S. J., Korai S. K., Liu Z. W. (2021). Characterization of ecdysteroid biosynthesis in the pond wolf spider, Pardosa pseudoannulata. Insect Mol. Biol. 30, 71–80. doi: 10.1111/imb.12678
Zandawala M., Haddad A. S., Hamoudi Z., Orchard I. (2015). Identification and characterization of the adipokinetic hormone/corazonin-related peptide signaling system in Rhodnius prolixus. FEBS J. 282, 3603–3617. doi: 10.1111/febs.13366
Zeng H., Bao C., Huang H., Ye H., Li S. (2016). The mechanism of regulation of ovarian maturation by red pigment concentrating hormone in the mud crab Scylla paramamosain. Anim. Reprod. Sci. 164, 152–161. doi: 10.1016/j.anireprosci.2015.11.025
Keywords: corazonin, corazonin receptor, ovarian development, ecdysteroid, Portunus trituberculatus.
Citation: Tu S, Ge F, Han Y, Wang M, Xie X and Zhu D (2022) Putative role of corazonin in the ovarian development of the swimming crab Portunus trituberculatus. Front. Mar. Sci. 9:976754. doi: 10.3389/fmars.2022.976754
Received: 23 June 2022; Accepted: 25 July 2022;
Published: 15 August 2022.
Edited by:
Haihui Ye, Jimei University, ChinaReviewed by:
Sirinart Techa, National Science and Technology Development Agency (NSTDA), ThailandAn Liu, Jimei University, China
Copyright © 2022 Tu, Ge, Han, Wang, Xie and Zhu. This is an open-access article distributed under the terms of the Creative Commons Attribution License (CC BY). The use, distribution or reproduction in other forums is permitted, provided the original author(s) and the copyright owner(s) are credited and that the original publication in this journal is cited, in accordance with accepted academic practice. No use, distribution or reproduction is permitted which does not comply with these terms.
*Correspondence: Xi Xie, eGlleGlAbmJ1LmVkdS5jbg==; Dongfa Zhu, emh1ZG9uZ2ZhQG5idS5lZHUuY24=