- 1Department of Oceanography, Dalhousie University, Halifax, NS, Canada
- 2Fisheries and Oceans Canada, Bedford Institute of Oceanography, Dartmouth, NS, Canada
- 3JASCO Applied Sciences, Halifax, NS, Canada
- 4Fisheries and Oceans Canada, Northwest Atlantic Fisheries Center, St. John’s, NL, Canada
- 5Department of Biological Sciences, University of New Brunswick, Saint John, NB, Canada
Northward range shifts are increasingly being identified in mobile animals that are responding to climate change. Range shifts are consequential to animal ecology, ecosystem function, and conservation goals, yet for many species these cannot be characterised without means of synoptically measuring their distribution. The distribution of critically endangered North Atlantic right whale (Eubalaena glacialis; NARW) north of 45°N has been largely unknown due to a lack of systematic monitoring. The objectives of this study were to characterize the spatial and temporal variation in NARW acoustic occurrence in the northern portion of their foraging range. In addition, we sought to identify relevant NARW migratory corridors and explore potential previously unidentified high-use habitats beyond the highly surveyed Gulf of St. Lawrence (GSL). To achieve this, passive acoustic monitoring data were collected and analyzed from 67 moorings and 13 gliders deployed (across 38 recording stations) throughout the Atlantic Canadian continental shelf, between 42°N and 58°N during 2015 through 2017. The results support that while a portion of the population has moved northward into the GSL, this shift was constrained to temperate latitudinal ranges < 52°N during the study period. NARWs were not detected in the Labrador Sea and Newfoundland Shelf, despite their preferred prey occurring in those areas. NARWs were present on the Scotian Shelf (45°N) nearly year-round, but only from May through December in the Cabot Strait (50°N). These results indicate that the northern range of the population is probably influenced by energetic requirements to minimize the distance between suitable foraging habitat and low latitude calving grounds, rather than an absence of suitable foraging conditions in high latitude waters, or other environmental or physiological factors. This work provides critical information to conserve the species and mitigate human-induced risks.
Introduction
Unprecedented shifts in the distribution of marine species in response to climate change are increasingly being identified around the world (Perry et al., 2005; Pinsky et al., 2013; Greene, 2016). In the Northern Hemisphere, large-scale climate variations have been associated with the weakening of the Atlantic Meridional Overturning Circulation (AMOC), a regional circulation system characterized by the northward flow of warm, saline water and the southward flow of cool water that has an important role in global thermohaline circulation (Saba et al., 2016; Caesar et al., 2018; Smeed et al., 2018). Processes that alter circulation patterns can have downstream impacts on foraging resources for predators, such as fish and whales (Greene et al., 2003; Greene et al., 2013; Meyer-Gutbrod and Greene, 2017; Meyer-Gutbrod et al., 2021). For example, the weakening of the AMOC has been associated with a northward shift in the warm Gulf Stream and subsequent reduction in copepod biomass indices in the Gulf of Maine (Meyer-Gutbrod et al., 2021). It is critical to observe how mobile predators respond to such bottom-up ecosystem changes driven by altered circulation patterns. One prevailing hypothesis, supported by observations, is that predators in the North Atlantic will shift their ranges north in response to a northward shift in the distribution of their prey.
It is challenging to measure distributional shifts in wide-ranging taxa such as baleen whales due, in part, to their cryptic and migratory nature. Passive acoustic monitoring (PAM) is now commonly used to study the distribution of many whale species over various temporal (hours to years) and spatial (10 to 10,000 km) scales. PAM can provide information on whale acoustic occurrence in remote locations, during hours of darkness, and during weather conditions that limit visual monitoring methods (e.g., Stafford et al., 1999; Nieukirk et al., 2004; Širović et al., 2004; Munger et al., 2005; Stafford et al., 2007). PAM has provided valuable insights into the distribution of whales by, for example, identifying migratory routes (e.g., Stevick et al., 2011), characterizing the distribution of cryptic species (e.g., Klinck et al., 2012; Stanistreet et al., 2017; Hildebrand et al., 2019), and explaining variability in habitat use (e.g., Wiggins, 2003; Moore et al., 2006; Baumgartner and Fratantoni, 2008; Bittencourt et al., 2016; Davis et al., 2017).
Information about variation in the distribution of North Atlantic right whales (NARWs), Eubalaena glacialis, throughout their range is essential for the mitigation of anthropogenic threats that pose significant barriers to the survival of the species (Pace et al., 2017). This species suffers from low reproductive rates, likely driven by a combination of climate-induced reduction in prey availability, anthropogenic stressors and high mortality predominantly caused by vessel strikes and fishing gear entanglements (Knowlton and Kraus, 2001; Vanderlaan and Taggart, 2007; Conn and Silber, 2013; van der Hoop et al., 2013; Meyer-Gutbrod et al., 2015; Daoust et al., 2017; van der Hoop et al., 2017; Meyer-Gutbrod and Greene, 2017; Bourque et al., 2020; Moore et al., 2021). In July 2020, the International Union for the Conservation of Nature changed the NARW status to Critically Endangered due to the declining population trend and consistently high mortality rate from human activities (Cooke, 2020). If these high mortality and low reproductive rates continue, the rate of population decline of ~5.3% per year is not sustainable and the species could be functionally extinct within the next 30 years (i.e., no more breeding females; Meyer-Gutbrod et al., 2018).
NARW survey efforts span the eastern Continental Shelf of North America from the calving grounds off southeast USA (i.e., Georgia and Florida) to the feeding grounds off Northeast USA (i.e., New England) and Atlantic Canada. However, the historically observed and modeled geographic distribution extends north of Newfoundland to Greenland, Iceland, and Norway (Lien et al., 1989; Knowlton et al., 1992; Reeves, 2001; Jacobsen et al., 2004; Mellinger et al., 2011; Monsarrat et al., 2015). NARWs were acoustically detected in southern Greenland waters between May and December of 2007 and 2008, but most consistently between July and August (Mellinger et al., 2011; Davis et al., 2017). Although NARWs have been sighted on the continental shelf along the Labrador and Newfoundland coasts (Hay, 1982; Lien et al., 1989), two large-scale aerial visual surveys of marine megafauna in Canadian waters in the northwest Atlantic in 2007 and 2015 yielded no NARW sightings (Lawson and Gosselin, 2009). Since then, no comprehensive NARW survey efforts (visual or acoustic) have been conducted in these northern Canadian waters.
NARW occurrence and movement from the Gulf of Maine northward are strongly influenced by the abundance and distribution of their preferred prey (Pendleton et al., 2009; Pershing et al., 2009; Record et al., 2019), lipid-rich copepods of the genus Calanus (Kann and Wishner, 1995; Baumgartner et al., 2003). The reduced NARW occurrence observed in Great South Channel and Roseway Basin in the 1990s (Kenney, 2001; Patrician and Kenney, 2010a; Davies et al., 2015; Davies et al., 2019; Meyer-Gutbrod et al., 2022) and in the Gulf of Maine, Bay of Fundy, and Roseway Basin, in the 2010s (Davis et al., 2017; Grieve et al., 2017; Johnson et al., 2017; Meyer-Gutbrod and Greene, 2017; Hayes et al., 2018; Record et al., 2019; Meyer-Gutbrod et al., 2021; Meyer-Gutbrod et al., 2022) has been linked to decadal-scale reductions in the availability of NARW temperate prey, Calanus finmarchicus, in and around these habitats. Since 2010, NARW occurrence decreased precipitously in their critical habitats in the Gulf of Maine and on the Scotian Shelf, and increased in the Gulf of St. Lawrence (GSL; Khan et al., 2014; Pettis and Hamilton, 2015; Pettis and Hamilton, 2016; Meyer-Gutbrod et al., 2018; Davies et al., 2019; Record et al., 2019; Simard et al., 2019). It is possible that the declining availability of prey of the 2010s has driven the northward expansion of NARW range into more suitable foraging areas (Meyer-Gutbrod et al., 2021; 2022).
Since 2015, NARW occurrence increased in the Gulf of St. Lawrence (GSL), a previously underutilized habitat (Khan et al., 2014; Pettis and Hamilton, 2015; Pettis and Hamilton, 2016; Meyer-Gutbrod et al., 2018; Davies et al., 2019; Record et al., 2019; Simard et al., 2019). Between 2015 and 2019, 40% of the population has been observed in the GSL annually between May and December, but in higher numbers between June and October (Crowe et al., 2021). The whereabouts of the remainder of the population are largely unknown, but could include other regions in Atlantic Canada, such as the more northern waters of Newfoundland and Labrador. Although visual and acoustic survey efforts have drastically increased since 2015, between 2015 and 2017 they were largely concentrated when and where NARW are known to occur. Therefore, NARW distribution outside known Canadian Critical Habitats (i.e., Roseway Basin and Grand Manan Basin) and the GSL is poorly described for this period and remains so today. Unmitigated risks, such as those from fisheries and vessel traffic, in unidentified high-use habitats, may pose a significant barrier to NARW survival and recovery. For example, the increase in NARW presence in the GSL and the initial lack of NARW protection measures amplified the risk of entanglement and vessel strike in the region. This contributed to an unusually severe mortality event in 2017, when at least 12 whales died and five were entangled in fishing gear. Since then, at least 9 additional NARWs have died in Canadian waters (Daoust et al., 2017; Bourque et al., 2020). Identifying where and when NARWs occur within the species’ range is needed to respond rapidly to distributional changes and improve our ability to successfully mitigate anthropogenic threats to the species.
The objective of this study is to provide information on the large-scale spatial and temporal variation in NARW occurrence in Atlantic Canadian waters between 2015 through 2017 using a comprehensive PAM network with coverage spanning multiple ecosystems, from the north-temperate Scotian Shelf to the subarctic Labrador Sea. In addition, this work aims to identify previously unknown NARW migratory corridors and high-use habitats.
Methods
Data collection
Acoustic data were collected in Atlantic Canadian waters during 2015 through 2017. Various acoustic recording devices were deployed by five different organizations: Autonomous Multichannel Acoustic Recorders (AMARs; JASCO Applied Sciences) were deployed by Fisheries and Oceans Canada (DFO) Maritimes Region, JASCO Applied Sciences, and Dalhousie University; Multi-Electronic Autonomous Underwater Recorders for Acoustic Listening (AURALs; Multi-Électronique) were deployed by DFO Newfoundland and Labrador Region; Slocum gliders (Teledyne-Webb Research) equipped with a digital acoustic monitoring instrument (DMON; Johnson and Hurst, 2007) were deployed by Dalhousie University. Recorders collected data either continuously or were duty cycled using various sampling rates and recording schedules (Supplementary Table 1). Some PAM moorings were placed to investigate NARW occurrence in known habitats (e.g., Roseway Basin and Emerald Basin; Figure 1), while other locations were chosen to monitor cetacean species at risk in general (e.g., Moors-Murphy et al., 2018), or to characterize broad-scale ambient and anthropogenic noise and address knowledge gaps in marine mammal distribution off eastern Canada, as was the case for the 38 deployments funded by the Environmental Studies Research Fund program (ESRF; Delarue et al., 2018). Although the detection range of each recording system will vary based on make, location and oceanographic conditions, we assumed a detection range of 10-30 km (Laurinolli et al., 2003; Baumgartner et al., 2019; Baumgartner et al., 2020; Johnson et al., 2020).
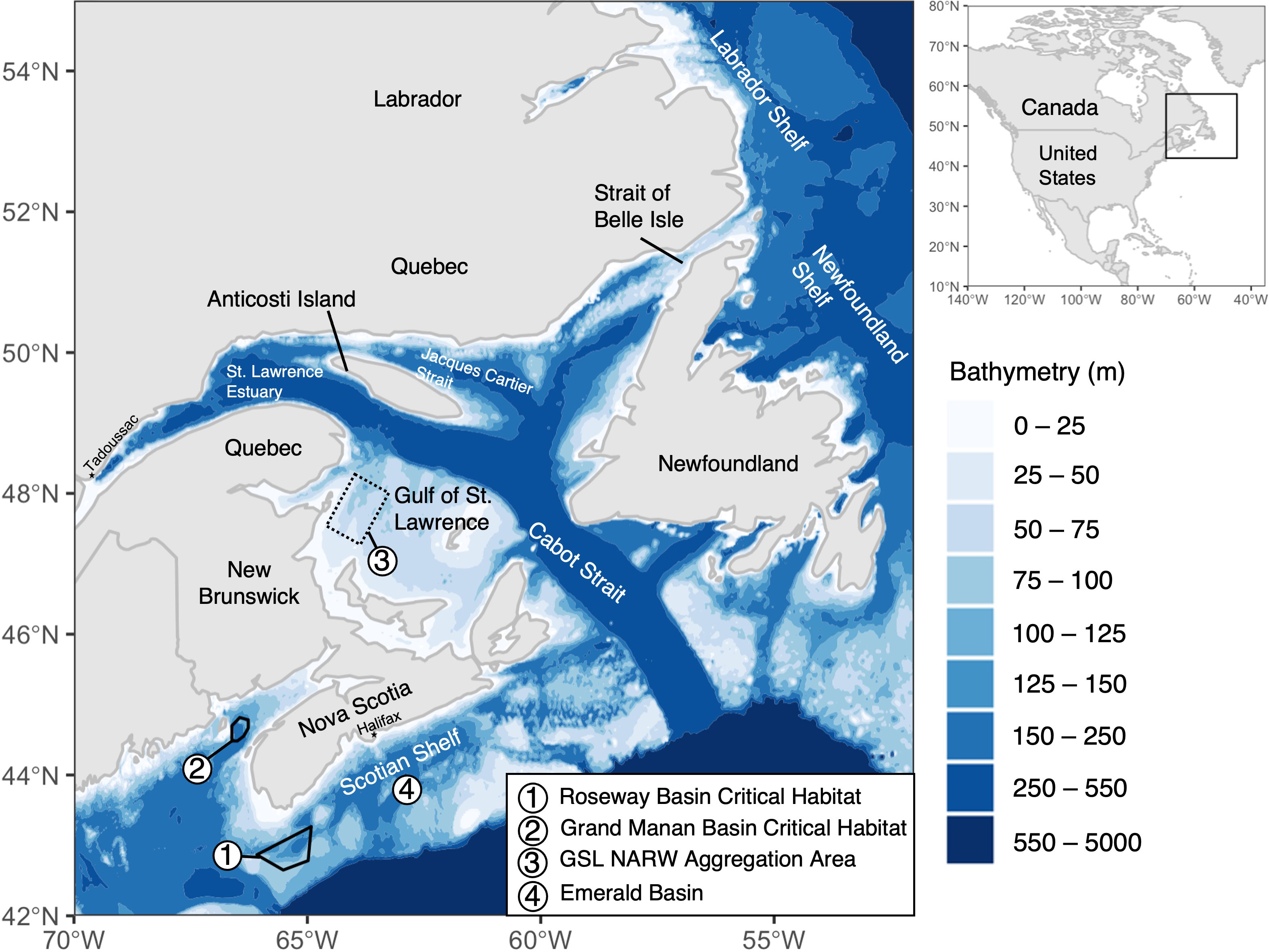
Figure 1 Areas discussed in this paper, including Canadian Critical Habitats designated for the North Atlantic right whale (DFO 2014) and general aggregation area in the southern Gulf of Saint Lawrence (sGSL). Bathymetry data sourced from the NOAA National Geophysical Data Center. 2009: ETOPO1 1 Arc-Minute Global Relief Model. NOAA National Centers for Environmental Information. Accessed 2022-02-06.
Data were analysed for 80 deployments from two types of PAM platforms: 13 from acoustic gliders and 67 from PAM moorings (Supplementary Table 1). These deployments spanned 38 unique recording stations; some stations had multiple deployments over the study period (Figure 2 and Supplementary Figure 1). The recording periods of each deployment include the first through to the last full 24 hr days of available recordings for the deployment (i.e., if the deployment started midday, the first full-recording day starts at midnight after the deployment). All ESRF and DFO Maritimes Region moorings spanned the 2015-2016 and 2016-2017 recording periods, with a few exceptions due to equipment failure or loss at sea (Supplementary Table 1). For stations spanning multiple recording periods, these recording systems were deployed in the same location, except for Station 7, which was moved 210 km east of the 2015-2016 location, north of the Flemish Pass to provide additional monitoring of northern bottlenose whales (Delarue et al., 2018). Additionally, one glider deployment traversed both Emerald and Roseway Basin and thus was divided into Stations 33 and 36 (Figure 2). The 38 stations were divided among 11 geographic recording regions of Atlantic Canadian waters based on areas potentially important to NARWs, such as feeding habitats and/or migratory corridors, as well as geographical context (Figure 2). The geographic recording regions are presented, south to north and west to east, relative to the coastline as follows: Bay of Fundy (BOF), Western Scotian Shelf (WSS), Eastern Scotian Shelf (ESS), Grand Bank (GB), Southern Newfoundland (SNL), Cabot Strait (CSt), Southern GSL (sGSL), Western Newfoundland (WNL), Strait of Belle Isle (StBI), Eastern Newfoundland (ENL), and Labrador Coast (Lab). Due to the large-scale collaborative effort of this study, the available recording effort in each region is temporally and spatially inconsistent; the number of recording systems in each region ranged from one to 16 over the study period (Supplementary Figure 1).
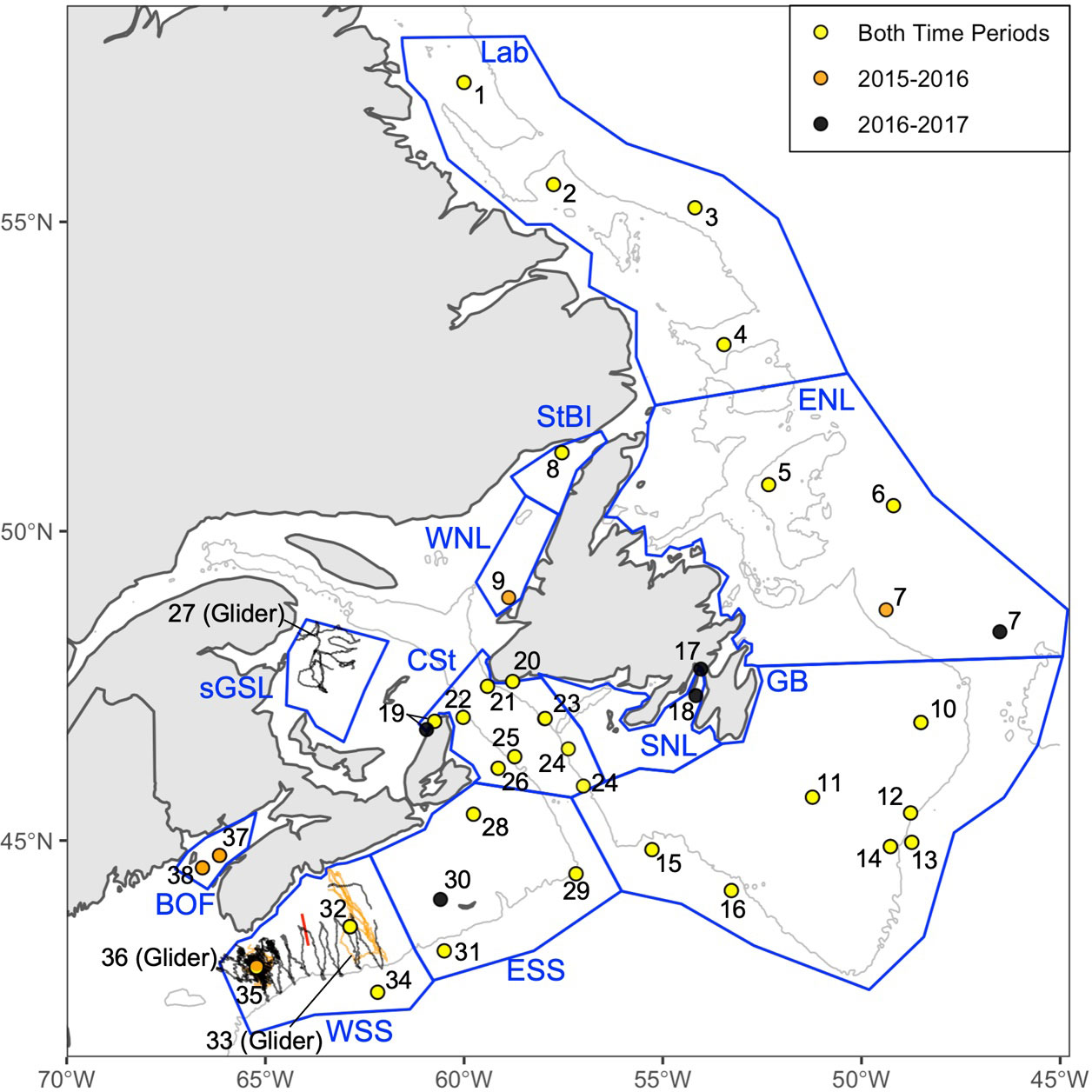
Figure 2 The 38 PAM recording stations analysed in this study. Orange, black and yellow closed circles indicate moored stations available for the 2015-2016, 2016-2017 periods, or both, respectively. Black and orange lines indicate glider survey tracks (stations 27, 33, and 36). The glider survey spanning stations 33 and 36 was divided by the red line observed on the map. Stations 38, 35, and 32 are located in Grand Manan Critical Habitat, Roseway Basin Critical Habitat and Emerald Basins, respectively. The recording regions are outlined by blue polygons and labeled from North to South as follows; Lab, Labrador Coast; ENL, Eastern Newfoundland; StBI, Strait of Belle Isle; WNL, Western Newfoundland; sGSL, Southern Gulf of Saint Lawrence; CSt, Cabot Strait; SNL, Southern Newfoundland; GB, Grand Bank; ESS, Eastern Scotian Shelf; WSS, Western Scotian Shelf and BOF, Bay of Fundy. The 300 m isobath is shown in light grey.
Data processing and analysis
The acoustic recordings were processed and analysed for the presence of NARW upcalls. The upcall has been used extensively to detect the presence of NARWs across their range (e.g., Van Parijs et al., 2009; Davis et al., 2017). It is a communication sound between 50-300Hz and is produced by both sexes and all age classes of the species (Parks and Tyack, 2005). Data processing followed the protocol described by Davis et al. (2017); recordings were processed using the low-frequency detection and classification system (LFDCS; Baumgartner and Mussoline, 2011), software designed to automate the detection and classification of NARW upcalls (‘auto-detections’) from the raw acoustic data. In brief, the LFDCS down-samples raw acoustic data to a 2 kHz sampling rate, computes a spectrogram, conditions the spectrogram by removing continuous noise, draws pitch tracks through tonal sounds, and then uses discriminant function analysis to compare pitch tracks to a library of known upcalls. This call library was that used in Davis et al. (2017) and did not include upcalls recorded in Canadian waters. However, this protocol and library have been used in Canadian waters since 2014 to classify and detect NARW upcalls (Johnson, 2022) with no indication that the characteristics of NARW upcalls differ among Canadian and United States’ waters. All auto-detections were reviewed by trained analysts (two of the authors) through aural and visual inspection of spectrograms using the LFDCS user interface.
Given the overlap in the sound repertoires of the NARW and humpback whale (Megaptera novaeangliae; Waite et al., 2003), we co-opted the standardized and documented LFDCS analyst protocol (see reference guide dcs.whoi.edu/#protocol) developed by the National Oceanic and Atmospheric Administration’s Northeast Fisheries Science Center Passive Acoustics Group and Woods Hole Oceanographic Institution (Baumgartner et al., 2019; Baumgartner et al., 2020) to distinguish between the two species. NARW upcalls tend to occur irregularly while humpback sounds often occur in repetitive patterns sometimes associated with songs, especially from fall to spring (Waite et al., 2003). These songs can include upsweep sounds very similar to the NARW upcalls. Although humpback whales produce sounds that are not always in a pattern, the protocol characterized humpback whale occurrence by the presence of patterned tonal and upsweep sounds. If potential upcalls coincided with humpback whale presence within a 24 hr period, upcalls were validated as a NARW detection if, and only if, they were out of pattern with the other tonal/upsweep sounds, at a different amplitude, and/or if other characteristic NARW sounds were present (e.g., gunshots; Clark, 1982; Parks, 2003; Parks and Tyack, 2005; Mellinger et al., 2007b). If the potential upcall could not be discerned from the pattern, then the call was dismissed as “incorrect” or conservatively as “unknown” and was not included in further analysis. Only the auto-detections confirmed to be true NARW upcall, hereafter ‘detections’, were used in the subsequent analysis; false auto-detections (false positives) were discarded. With every auto-detection manually validated and all false positives removed, all remaining detections were considered correct.
To evaluate the rate of false negatives (i.e., how often the LFDCS missed NARW upcalls), we calculated the LFDCS Recall using previous analysis of the ESRF recordings (Delarue et al., 2022). Determining Recall for all recordings via a systematic analysis was beyond the scope of the present work, however great insight can be gained by utilizing previous work. Delarue et al. (2018; 2022) manually reviewed a subset (825 hrs) of the ESRF recordings, spread across stations and time of year, for the presence of multiple species, including NARWs (ADSV method; Kowarski et al., 2021). The LFDCS upcall detections were compared to these manual results to calculate per file Recall for each ESRF recording station and recording period (Equation 1), where acoustic files with both manual and auto-detections were true positives and files with manual detections, but no auto-detections, were false negatives.
The daily presence of NARW upcalls, defined as any day with at least one validated NARW upcall, was used as the unit of measurement for NARW presence in this study. The temporal variability in recording effort was assessed using recording days, defined as the total number of active recorders on a given calendar day at a defined spatial scale (e.g., recording region). For example, if there were three active recorders on 01 January 2016 within a recording region, there were three recording days for that calendar day. Statistics on NARW presence were derived over three spatial scales (recording station, recording region, or aggregated across the entire study area) and two temporal scales (weekly, monthly). To do this, all recording days within the defined spatial and temporal scales were tallied to calculate the number of calendar days with at least one recording day with a NARW detection. For example, if there were three active recorders on 01 January 2016 within a recording region all of which detected NARW upcalls, this would count as only one day with NARW presence in that region.
Temporal and spatial patterns in NARW presence were explored in several ways. The timing and duration of NARW occupancy were defined using the number of consecutive months with acoustic presence at each spatial scale. Seasonal NARW occurrence was first examined over the entire study area, all years combined, and a sensitivity analysis was performed to assess the effect of variable recording effort (i.e., recording days) on NARW occurrence across the study area (see Supplementary 2). Variability in the weekly and monthly NARW presence, among years, was compared among regions to examine seasonal distribution and potential migratory patterns along a latitudinal gradient between 42°N and 57°N. Variability in weekly presence, among years, was then compared among recording stations within each recording region to explore potential unknown high-use habitats. Periods of NARW acoustic presence were categorised as persistent (two consecutive weeks each with at least three days with NARW presence), sporadic (two consecutive weeks each with at least one day with presence), or rare (only one day with presence during non-consecutive weeks).
Seasonal and spatial distribution patterns were further examined by calculating the detection rate of NARWs during the different quarters of the calendar year (Q1: January, February, March; Q2: April, May, June; Q3: July, August, September; Q4: October, November, December). For this analysis we assume years are independent. As above, these statistics were calculated by aggregating (i.e., tallying) the data at the spatial scale selected. The number of days per month with at least one recording day with a detection was divided by the number of days with at least one recording day (across all recorders included). Quarterly medians were then calculated over all months with recording effort independent of the recording years. This quarterly NARW detection rate was calculated for each of the 11 recording regions and among three broad-scale shelf domains where NARWs were detected, defined below. The ‘known’ NARW domain, where NARWs have been known to occur regularly, included three regions: ESS, WSS, and BOF. These southern regions included NARW Canadian Critical Habitats: Grand Manan Basin and Roseway Basin (Figure 1). The sGSL was omitted from this final analysis due to limited recording effort, but its occupancy period was previously defined by Simard et al. (2019). The ‘transition’ domain, the hypothesized migratory corridor where NARW presence is sporadic, included one region, CSt. The ‘northern’ domain, where NARWs are rarely present, included two regions: StBI, and GB.
Results
A total of 18,400 recording days were included in this study; NARWs were acoustically present on 589 of these recording days (3%; Figure 3). NARW were present on 42 of the 80 deployments analysed (52%), on 24 of the 38 PAM recording stations (63%), and in eight of the 11 recording regions (Figure 4). NARWs were detected in Atlantic Canadian waters during all months of the year (Figure 3A). However, the increased acoustic presence in the latter half of the calendar year suggests NARW presence varies with season; the majority of upcalls were detected from June through December (seven consecutive months with >50% daily presence; Figure 3A). A test with multiple random permutations of the daily presence data showed that the seasonal signal was not sensitive to the effort variation across all recording regions but was sensitive to the limited recording effort in the sGSL (Supplementary 2; Supplementary Figure 2; Figure 4). The period with the greatest spatial extent of acoustic presence throughout the entire study area was from mid-August to mid-October (Figure 5).
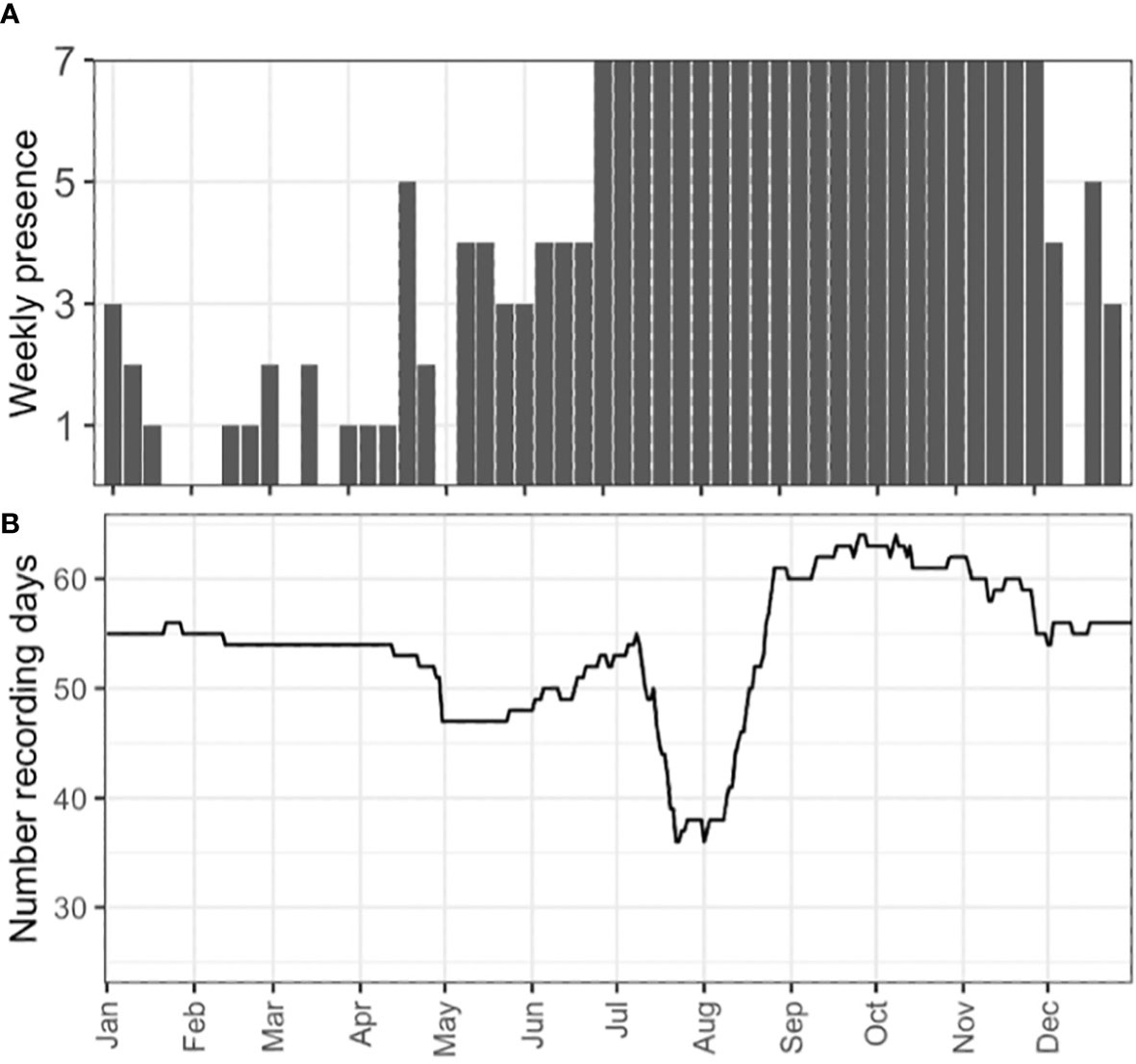
Figure 3 (A) The number of days per week with at least one NARW upcall detection across all recorders and years analysed for this study, (B) Daily number of recording days across all recorders and years analysed for this study. Recording days are defined as the total number of active recorders on a given day (e.g., if there were three active recorders on 1 January 2016, there were three recording days for that day).
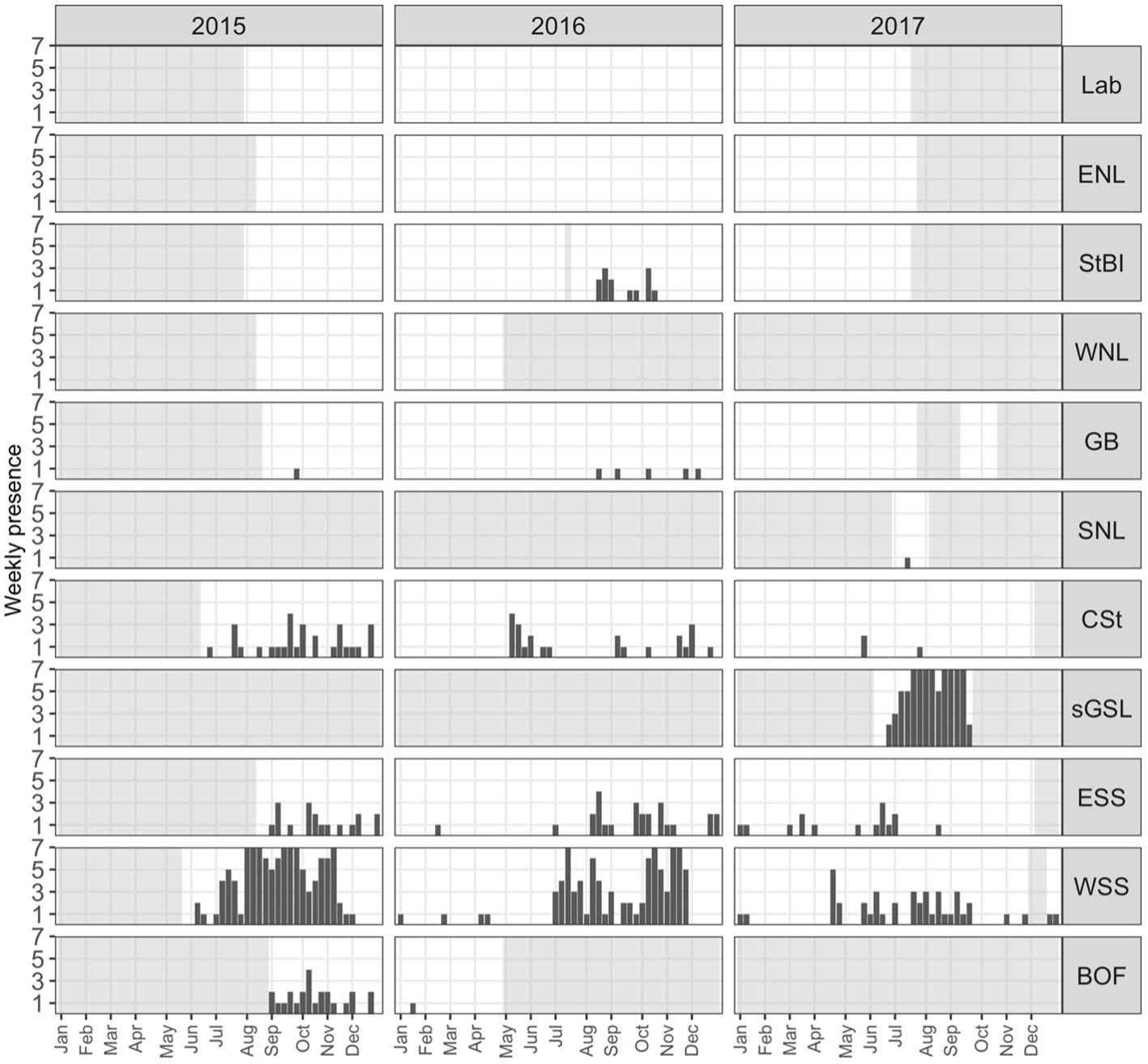
Figure 4 The number of days per week with at least one NARW upcall detection by region (rows) and year (columns). Light grey polygons indicate periods with no recording effort in that region. Refer to Figure 2 for the location of regions; Labrador Coast (Lab), Eastern Newfoundland (ENL), Strait of Belle Isle (StBI), Western Newfoundland (WNL), Grand Bank (GB), Southern Newfoundland (SNL), Cabot Strait (CSt), southern Gulf of Saint Lawrence (sGSL), Eastern Scotian Shelf (ESS), Western Scotian Shelf (WSS), and Bay of Fundy (BOF).
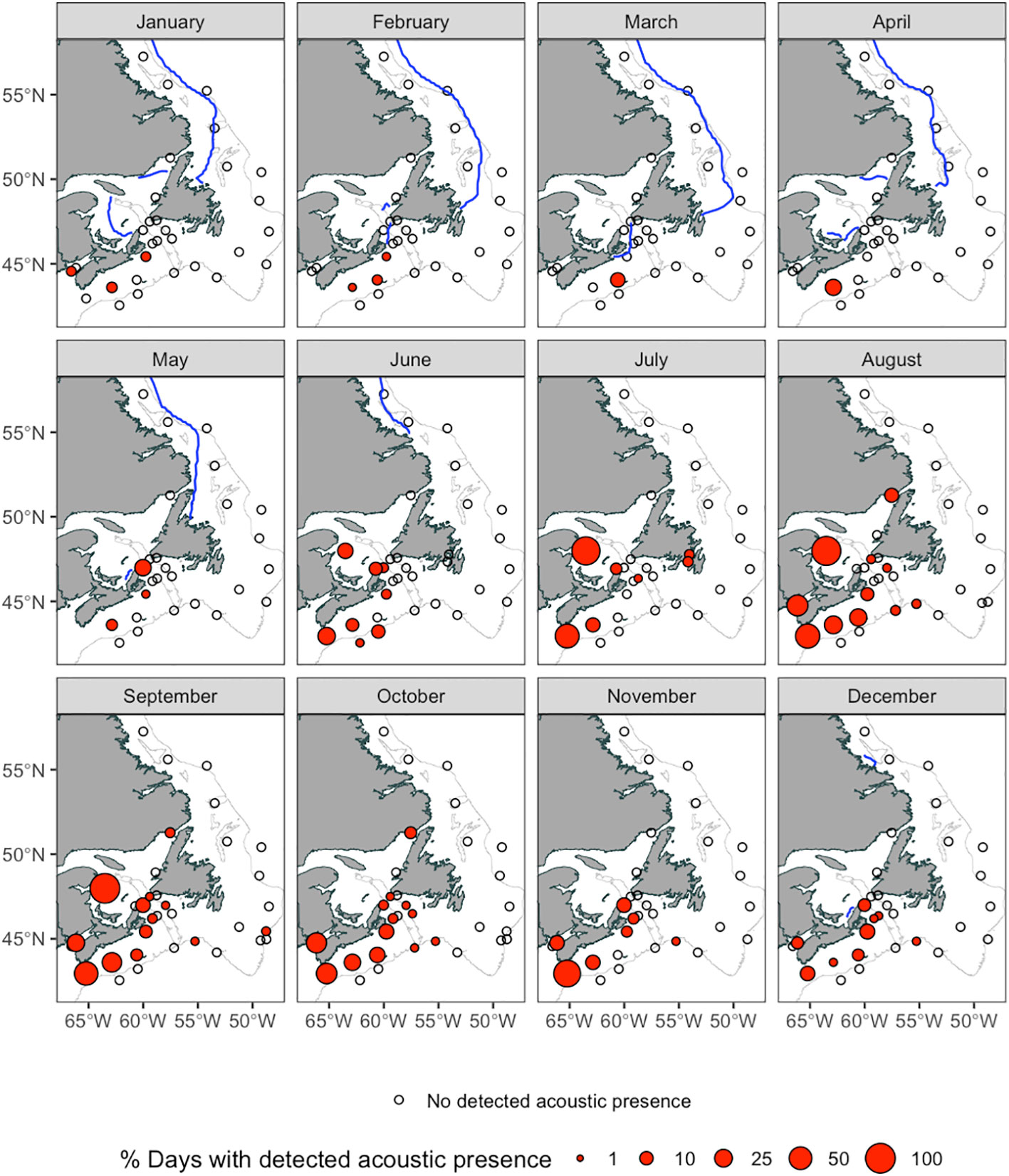
Figure 5 Spatial distribution of NARW presence in Canadian waters per calendar month. Red symbols denote deployment locations where NARW were present, and open symbols denote deployment locations where NARWs were not detected. The size of each symbol denotes the percent of recording days within each month, across all years, when NARW were present. Blue contours denote monthly median extent of ice-cover from the shoreline during 1987 to 2010, data taken from the National Snow & Ice Data Center (Environment Canada, C.I.Service, 2011). The 300 m isobath is shown in light grey.
Within a calendar year, the earliest NARW detections occurred in the most southerly regions: ESS, WSS and BOF (Figures 4, 5). In the BOF, 481 recording days were analyzed from two Stations (37 and 38) with the same recording effort from 27 August 2015 to 27 April 2016. Daily presence at Station 37 was sporadic from the start of the deployment through November 2015 (10% of recording days with presence; Supplementary Figure 4). NARWs were detected on one day (21 January 2016) at Station 38. NARWs were present in every month on the Scotian Shelf, except for March on the WSS and April on the ESS. Furthermore, within both Scotian Shelf regions, presence was lower in 2017 relative to 2015 and 2016, especially from August through December.
Daily presence in the WSS was sporadic during the first five months of the calendar year but persistent from June through December (Figure 4). The WSS recording effort consisted of a mooring and glider deployment in Emerald Basin (EB; Station 32 and 33), Roseway Basin (RB; Station 35 and 36), and Station 34 on the continental slope (>1500 m; Figure 2, Supplementary Figure 1). Daily presence in RB was more persistent during the periods of recording in 2015 and 2016 compared to 2017 (73, 68 and 13% of recording days, respectively; Supplementary Figure 4). In contrast, daily presence in EB was generally sporadic throughout 2015, 2016, and 2017 (24, 11, and 7% of recording days with NARW, respectively; Supplementary Figure 4). Glider and moored hydrophone data showed similar patterns within periods of overlapping effort among RB (Station 35 and 36; 29 July 2015 to 03 September 2015) and EB (Stations 32 and 33; 28 July 2015 to 22 August 2015, 11 September 2015 to 03 October 2015, 28 October 2015 to 16 November 2015, 23 January 2016 to 10 February 2016, and 17 September 2016 to 11 October 2016). The only exception was the first EB-glider deployment in Station 33 in 2015, during which NARWs were detected on more days compared to the EB moored deployment in Station 32 during the same period (28 July 2015 to 22 August 2015). The January-February 2016 deployment in Station 33 was the only glider deployment that did not detect NARWs, however NARWs also were not detected in Station 32 during this period (January 2016 to 10 February 2016). Station 34 on the continental slope had only one day in June 2017 with acoustic detections.
Daily presence was lower in the ESS, despite the greater recording effort compared to the WSS (2,226 recording days compared to 2,008). NARW occurrence was concentrated at Stations 30 and 28 (on 6 and 5% of recording days, respectively; Supplementary Figure 4) where daily presence ranged from sporadic to at times rare. Stations 31 on the continental slope (>1500 m) recorded 6 days of acoustic presence in June 2017.
Daily presence in the CSt started in early May and was sporadic until mid-December (Figure 4). Though more recorders were deployed in this region (4,335 recording days) relative to other recording regions, NARW presence was never persistent, with only 1% of recording days with NARW presence. In 2017, NARWs were detected in this region on only two days in May and one day in July.
NARWs were detected in the sGSL and StBI, two of the three regions within the GSL. The only acoustic data available for this study in the sGSL was one 109 day-long glider deployment in June through mid-September 2017 (Station 27). Like the glider deployments on the WSS, acoustic occurrence in the sGSL was persistent, from June through September, on 78% of recording days (Figure 4). At the entrance of the GSL in the CSt, Station 19 had only two short deployments at the end of June through July 2015 and in July through August 2017 for a total of 96 recording days (Supplementary Figure 1); NARWs were detected in June and July on 5 recording days. At the northern entrance of the GSL, NARW presence in the StBI (Station 8) occurred as early as mid-August and as late as mid-October, corresponding to the period with the greatest spatial extent of acoustic presence throughout the entire study area (Figure 5). Despite the continuous recording effort in the StBI, NARWs were only detected on 2% of 699 recording days and were not detected in 2015 or in 2017. In the WNL (Station 9), no NARWs were detected during the 2015-2016 recording period (253 recording days).
In and around the Newfoundland and Labrador regions, NARWs were only detected on 14 July 2017 on both month-long recordings at Stations 17 and 18 in the SNL (end of June to end of August 2017 and end of June to end of July 2017 respectively; Figure 4). However, there were detections on six days in the GB (0.2% of recording days) between August and early December. Acoustic presence was detected at two of the 10 Stations in this region, Stations 15 and 12 (Supplementary Figure 4). Station 15 was one of three in GB at a depth >300 m but the only one with NARW presence. The two most northerly regions in this analysis, the ENL and Lab on the Labrador Shelf, contained no confirmed NARW acoustic occurrence (Figure 4). Of the seven Stations in the ENL and Lab, four were located off the continental shelf at depths >300 m. The Lab Stations 1 and 2 cumulatively contained three days in March and two in April of 2016 as well as three days in April and one day in February of 2017 with ‘possible’ NARW upcalls among sea-ice ambient noise. The ‘possible’ score was assigned due to the presence of other marine mammals that make calls similar to and/or within the same frequency band as NARWs (e.g., humpback whales, bowhead whales, and bearded seals; Delarue et al., 2018).
The quarterly medians of the NARW detection rate further indicate spatial variation and seasonal patterns among recording regions and among the three broad-scale domains (Figure 6). Of the 11 regions, seven had at least one quarter with a median above zero (Figure 6A). The highest medians were during Q3 (July through September) in the sGSL, WSS, and BOF (0.94, 0.48, and 0.37 respectively). Despite the variability in recording effort (Supplementary Figure 1), NARW acoustic occurrence was generally highest in the ‘known’ domain (ESS, WSS, and BOF), diminished in the ‘transition’ domain (CSt), and was rare north of CSt in the ‘northern domain’ (Figure 6B).
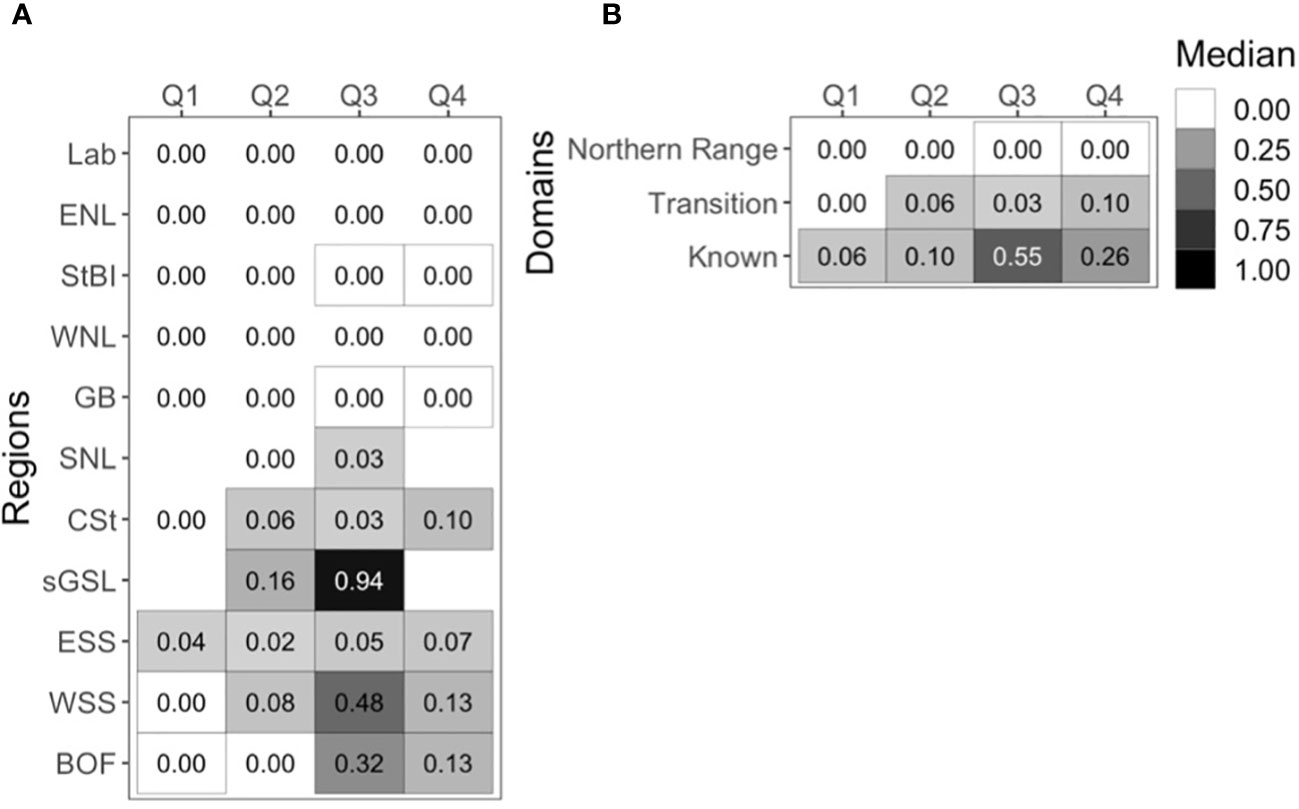
Figure 6 Quarterly median of monthly effort-corrected NARW acoustic detection rate among (A) different regions and (B) the different domains; “Northern Range” domain included StBI, WNL, GB, “Transition” domain included CSt, and the “Known” domain included ESS, WSS, and BOF. Yearly quarters defined as Q1; January – March, Q2; April – June, Q3; July – September, Q4; October – December. Bordered cells highlight quarters when and where NARW were detected on at least one recording day.
The LFDCS per file Recall could only be calculated at stations that contained confirmed NARW occurrence during the manual analysis of the subsampled ESRF recording stations. The Recall was 1.00 (i.e., all files containing NARW upcalls were captured by the LFDCS) for all stations but Station 22 during the 2015-2016 recording period (Supplementary Table 2). Within the subsampled manual analysis at this station, NARWs were manually detected on 16 and 17 September 2015, but only detected by the LFDCS on 16 September.
Discussion
In this study, we document spatial and temporal variation of NARW presence in Canadian waters during a period in which the NARW distribution, northward of the Gulf of Maine, was changing, with relatively little visual survey effort in Canadian waters and only a portion of the known NARW population sighted. It therefore provides critical insights into what may have occurred during the transition period towards more consistent NARW distribution in Canadian waters. In this study, from 2015-2017, NARWs were detected throughout the calendar year, with the greatest occurrence on the Scotian Shelf, year-round, and in the central recording regions of eastern Canada (such as around the CSt and in the GSL) from May to December. Higher overall acoustic presence relative to the StBI suggests that the CSt is likely the primary NARW migratory corridor into and out of the GSL. The lack of acoustic presence off northern Newfoundland and eastern Labrador in this study suggests that the geographic distribution of the species between 2015 and 2017 was constrained to temperate regions.
Detector performance and assumptions
While the data provide novel insights into NARW distribution, there are important caveats. The current analysis assumes an equal probability of detecting a NARW among all regions and monitoring platforms. Variation may exist as a result of differences among monitoring platforms (gliders versus moorings provide different coverage in time and space), recording systems, their detection ranges, and deployment locations, bathymetry and environmental conditions (including anthropogenic activities and associated noise), the vocal behaviours and calling rates of the animals, and the accuracy and reliability of the auto-detector (Baumgartner and Mussoline, 2011; Davis et al., 2017; Johnson et al., 2020).
With all false positives manually removed, the auto-detector-guided results presented here can be considered a true minimum representation of NARW acoustic occurrence, but the likelihood of underestimating the species’ occurrence should be considered. Davis et al. (2017) found the LFDCS to have a daily missed NARW auto-detection rate of ~25%, but acknowledged that such auto-detector performance metrics vary by stations. We found that the LFDCS auto-detector does not always capture every instance of manually confirmed NARW, depending on the year and station analyzed, and that this may be accentuated in areas where whale vocalizations are sporadic or rare (as in migratory corridors). While we did not determine Recall for all deployments included in the present study, false negatives are not expected to be an important issue for this broad-scale analysis because NARW presence is quantified at daily, weekly, monthly, and seasonal time scales and Recall tends to increase with longer timeframes (e.g., Kowarski, 2020). In fact, although the LFDCS auto-detector did not detect NARW upcalls on one of two days of the subsampled manual analysis, NARW upcalls were auto-detected by the LFDCS on both days during periods that were not included in the subsampled analysis. Furthermore, in an analysis of 825 hr of ESRF recordings for the acoustic presence of marine mammals, Delarue et al. (2018) similarly concluded NARWs were rare or absent from the more northern regions. This further illustrates that the patterns observed in the current study were not an artifact of detector performance.
It is possible that NARW presence was missed if the whales were not producing upcalls near the recorders during the recording periods, were producing other vocalization types not targeted in the present analysis (e.g., gunshots, moans; Van Parijs et al., 2009; Durette-Morin et al., 2019), or were outside of the detection range of the recorders. NARW calling rates may vary by season and behavioral state (e.g., travelling, socializing, feeding, calving, group size; Clark, 1983; Parks et al., 2011; Durette-Morin et al., 2019; Franklin, 2022). However it is not possible to control for these factors in this study. Furthermore, although the general trends of NARW distribution in this study are similar to those from NARW sightings, stations with rare detections (e.g., Station 12 in the GB) should be interpreted with caution as there could be confounding presence of humpback whales call types, or other species, that were not considered with the analysis protocol used.
This study aggregated data from multiple research programs with variable survey objectives and equipment, therefore, we could not control for the recording periods and duty cycles that varied among deployments. However, we assume each recorder has the minimum recording effort each day needed to detect minimum NARW presence as the majority (71 of 81) deployments were duty cycled at 50% or more (mean = 0.535, var = 0.093). The ten other deployments were sampled between 0.33 and 0.40 (Stations 14, 20, 23, and 24), with only one notable exception sampled at 0.16 (Station 12). Subsampling is a consequence of duty cycle, and therefore can reduce the probability of detecting whales (Sousa-Lima et al., 2013; Thomisch et al., 2015), however, we assume that the reduction in detection is negligible given that the units of measurement for NARW presence in this study were on daily, weekly and monthly scales, rather than an hourly rate. Furthermore, in the present study, only one true NARW upcall was needed as a criterion to characterize NARW presence on any day, as was done for duty cycled recorders by Davis et al. (2017). Nevertheless, the results must be interpreted with caution, especially for recorders in areas where calling activity may be rare.
Due to the finite number of recording systems contributed from various projects, the defined region polygons (Figure 2) include areas not covered by the assumed detection range of the recorders (i.e., 10-30 km; Laurinolli et al., 2003; Baumgartner et al., 2019; Baumgartner et al., 2020; Johnson et al., 2020). For example, there was no coverage of the inshore waters of Newfoundland and Labrador (ENL and LAB) while some regions contained only one to two recording stations (e.g., StBI, WNL, and SNL). In such cases, it is possible whale presence was missed if the whales were calling outside of the recording range of the recorders. In the WSS, glider deployments surveyed in and around the Roseway and Emerald Basins and are assumed to be representative of the surveyed area in general. Given whale movement, the effective monitoring range over the recording periods at each station will be much larger than the assumed detection range of the recorders as whales continuously move in and out of the detection range over time (Baumgartner et al., 2019; Johnson et al., 2020). Additionally, it is possible that the glider moves in and out of the detection range relative to the whales. Nevertheless, although the recording systems do not cover all possible areas where whales could be present, the data presented in this study remain the most comprehensive effort in Canadian waters for that period.
Seasonal distribution in northern Canadian waters
The monitoring efforts in this study are complementary to that of Davis et al. (2017), which characterized NARW distribution primarily along the eastern seaboard of the United States. Although the northernmost recording stations of the Davis et al. (2017) study (Iceland and Greenland in 2007) were in historical habitats of the NARW northern range, the coverage into Canadian waters extended only as far north as the Scotian Shelf in 2014. The influx of NARW into the GSL, since 2015, likely represents a northward expansion of the NARW distribution into a previously less-utilized portion of the foraging range (Simard et al., 2019; Meyer-Gutbrod et al., 2021), but little is known of NARW occurrence in Canadian waters, north of the GSL. Our study shows that in Canadian waters during 2015 and 2017, NARWs occurred consistently as far north as the GSL, west of 55°W longitude, but were not detected on the Newfoundland Shelf. This was true even during the summertime peak (July-September) NARW occupancy period in Canadian waters when subarctic waters are ice-free and can produce high abundances of NARW prey (Environment Canada, 2011; Head et al., 2013; Pepin et al., 2013). Although, the study does not cover all possible areas on the Newfoundland and Labrador shelves, particularly the inshore waters of the north and east coasts of Newfoundland, it is probable that NARWs were not present on the shelf in large numbers during this study, but that dispersed roaming individuals travel through these waters. Alternatively, NARWs may have started frequenting the Newfoundland and Labrador shelves more regularly after 2017; at least one NARW was sighted along the northern coast of Newfoundland on 29 September 2019 (Lawson unpubl. data), four near the Newfoundland north and east coasts in November 2021 (Lawson unpubl. data), and one along the southwest coast of Iceland on 23 July 2018 (Johnson et al., 2021).
Although this data did not contain recorders outside Canadian waters, the lack of definite acoustic presence reported in this study in subarctic waters around northern Newfoundland and Labrador suggests that the northern range of the species was largely focused on temperate waters during this period. This suggests that few NARWs travel as far north as the estimated distribution of their preferred prey, Calanus spp., which extends into arctic waters (Conover, 1988; Melle et al., 2014; Record et al., 2018). Documented forays by individuals into sub-zero Celsius waters of the GSL and higher latitudes indicates thermal tolerance should not be a constraint, which is corroborated by models suggesting healthy baleen whales are over-insulated (Kanwisher and Ridgway, 1983; Hokkanen, 1990; Favilla et al., 2021). However, NARWs have thinner blubber layers and poorer body conditions compared to the southern right whale species (Christiansen et al., 2022). NARWs may only travel north until they find the first suitable feeding habitat. Further prey search may be too energetically costly and reduce the potential for social interactions or, in the case of mother-calf pairs, expose the calf to potential predators.
The ability of NARWs to meet their metabolic requirements is critically dependent on the presence of high-density patches of their prey (Baumgartner et al., 2007). These prey aggregations are influenced by processes that affect supply (e.g., advection, production; Sorochan et al., 2021). Climate change is altering these processes dramatically in the North Atlantic, which is affecting the spatial and temporal variation of prey abundance (Richardson, 2008; Grieve et al., 2017; Record et al., 2019; Pershing and Stamieszkin, 2020). If the regional declining trend in C. finmarchicus abundance continues, NARWs may migrate further north in search of prey, which would represent a shift in the ecological niche for this species.
Given that less than half of the NARW population has been sighted in the GSL beginning in May through December, locating the remaining portion of the population during the summer season (June-August) is critical (Crowe et al., 2021). While this present study did not show evidence of unidentified high-use habitats in Canadian waters, summertime (August) NARW aggregations have been observed south of the Gulf of Maine in the Nantucket Shoals region, suggesting the population could be spread out both north and south of the Gulf of Maine (RWSAS, n.d. Northeast Fisheries Science Center, 2020; Quintana-Rizzo et al., 2021). Continued persistent and improved monitoring is crucial to locate as yet unidentified habitats where whales may be aggregating (Meyer-Gutbrod et al., 2018).
Variation in presence within the known foraging range
Following the distributional shift in 2010, NARW sightings per unit effort decreased in the Canadian NARW critical habitats (Meyer-Gutbrod et al., 2021). This has framed an oft-repeated narrative that NARWs almost completely abandoned the Grand Manan Basin and Roseway Basin Critical Habitats (Hamilton et al., 2007; Batten and Burkill, 2010; Patrician and Kenney, 2010a; van der Hoop et al., 2012; Brillant et al., 2015; Davies et al., 2015). Davis et al. (2017) reported year-round NARW occurrence on the Scotian Shelf between 2004 and 2014, but the occurrence between August and December was lower during the 2010-2014 period. Our study shows there was year-round NARW acoustic presence on the Scotian Shelf, particularly persistent in Roseway Basin during the recording periods in 2015 and 2016. This demonstrates that NARW distribution can be highly dynamic such that a habitat apparently abandoned in one year can be fully occupied the next, as was shown by Davis et al. (2017). This has important implications when interpreting species occurrence data for management purposes. More consistent monitoring of Grand Manan Basin Critical Habitat is needed to understand the current seasonal use of that area.
The persistent NARW occurrence in Emerald Basin in August and September support previous works suggesting Emerald Basin is an important NARW habitat (Mellinger et al., 2007a; Moore, 2017). Additionally, while no other recording stations had persistent NARW presence to suggest the existence of a previously unknown high-use habitat, Station 28, had higher NARW presence compared to the rest of the ESS deployments. Notably, this station is located near an area predicted to be a suitable feeding habitat by Plourde et al. (2019).
Migratory corridors to the Gulf of St. Lawrence
The more sustained acoustic presence of NARWs in the CSt, compared to the StBI, suggests that the CSt is the primary migratory corridor used by NARWs to enter and exit the GSL. Though recording effort was higher in the CSt compared to the StBI, most acoustic presence in the CSt occurred at Station 22. The similar occupancy period between CSt and GSL indicate NARWs continually transit via the CSt throughout the foraging season and that likely most NARWs identified in the sGSL habitat have used this corridor each year since at least 2015. Furthermore, the geographic position of the CSt makes it the shortest route from the Scotian Shelf into the GSL. Given the narrow entrance to the GSL, the CSt is a bottleneck for medium to large-sized vessels and the NARWs, as well as other whale species, and thus, should be considered as an area of high concern for noise (e.g., Cominelli et al., 2020) and vessel strike threat mitigation.
NARW presence was rare to sporadic in migratory corridors such as the StBI and CSt, as well as the ESS and GB, despite continuous recording effort. NARW behaviour is the primary determinant of calling rates (Matthews et al., 2001; Parks et al., 2005; Parks et al., 2011; Matthews et al., 2014). Although calling rates of travelling NARWs remain largely unknown, travelling may lower the probability of social interactions, which can yield high calling rates and detections, or reduce the time spent within the detection range of each hydrophone. Further insights into acoustic behavior of travelling whales could be obtained by studying concurrent PAM and visual observations (e.g., Clark et al., 2010; Durette-Morin et al., 2019; Franklin, 2020; 2022). Alternatively, it is possible that the local noise (i.e., vessel noise) reduced detectability. In such regions, NARWs are far more likely to be detected with continuous monitoring as that provided by PAM. Additionally, a greater spatial extent for PAM in the area would increase the probability of NARW detection.
Conclusions
This study provides a broad-scale characterization of NARW distribution in Canadian waters for the 2015-2017 period. While we found no evidence of additional NARW high-use habitats in Canadian waters outside of previously identified Critical Habitats and the GSL, NARWs were acoustically detected as far south as Bay of Fundy and as far north as the Strait of Belle Isle. This is crucial information for management of marine activities and the protection of this species as it indicates that NARWs are present in these waters and could be at risk of anthropogenic activities. Although NARWs were sighted off the Newfoundland coast in 2019 and 2021, they were not detected in our analysis, suggesting the geographic distribution of the species between 2015 through 2017 may have been constrained to temperate ecosystems. Given the large proportion of the NARW population that is not seen each year, sporadic detections could be important indicators of further range expansion or represent remnants of historical range. This study provides a baseline for interpreting future broad-scale acoustic monitoring in Atlantic Canada, which is necessary to assess how such predator distribution may respond to climate-driven shifts of their prey. NARWs occurred between May and December in the CSt and throughout the year on the Scotian Shelf, therefore considerations should be given for protection measures to overlap with these periods of NARW occurrence in these areas. Considering this species has a primarily coastal distribution, future evaluation of migration corridors or presence near shore in Canadian waters would be best performed with recorder lines extending from the shore to shelf breaks (e.g., Davis et al., 2017). It is difficult to quantify distributional shifts in wide-ranging predators due to a lack of synoptic data across their range, this study highlights the advantages of PAM in defining broad spatial and temporal scale distribution of mobile animals in changing oceans.
Data availability statement
The datasets presented in this study can be found in the online repositories below: https://datadryad.org/stash/share/AcJ7Hiae286LoDNAjmXX-W9thM9fF4RST6TziI44r9M Durette-Morin, Delphine (2022), North Atlantic Right Whale Detections from Passive Acoustic Monitoring Network across Atlantic Canada during 2015-2017, Dryad, Dataset, https://doi.org/10.5061/dryad.stqjq2c5s. Detection data can also be viewed on the NOAA Passive Acoustic Cetacean Map through https://apps-nefsc.fisheries.noaa.gov/pacm/#/narw and accessed through the North Atlantic Right Whale Catalogue through https://www.narwc.org.
Author contributions
DDM and KD contributed to conception and design of the study. DDM organized the data. DDM and CE processed and validated the acoustic recordings. JL, HMM, JD, KK, and EM either deployed, recovered, organized, processed recordings and/or contributed these to the study. HJ contributed to the analysis by providing code to process the large quantities of data, and provided help with the software. DDM wrote the first draft of the manuscript. KD supervised and provided feedback throughout the study. All authors contributed to the article and approved the submitted version.
Funding
This research was supported the funding by a National Science and Engineering Research Council of Canada (NSERC) CGS M scholarship to Delphine Durette-Morin, the Government of Canada's Marine Environmental Observation, Prediction and Response (MEOPAR) Network of Centers of Excellence funding to the Whales, Habitat, and Listening Experiment (WHaLE), the Habitat Stewardship Program of Environment and Climate Change Canada, and the Canadian Whale Institute. The DFO Maritimes Region deployments were supported through funding by DFO’s Species at Risk Implementation Funds and National Conservation Program. The DFO Northwest Atlantic Fisheries Center deployments were supported by DFO’s Species at Risk Implementation Funds and the Ocean Protection Plan’s MEQ program. The JASCO deployments were supported by a Collaborative Agreement with the Environmental Studies Research Fund.
Acknowledgments
We thank those involved in the field work, data collection and database management of the DFO deployments over the years. We thank those at DFO who built the PAM moorings, DFO and the CCG for their help in coordinating and conducting deployments and retrievals of the PAM systems. We thank all those at Jasco Applied Sciences for collecting, sharing and maintaining the PAM data over the years. This work benefitted from those involved in the field work, data collection and database management of the Dalhousie University deployments over the years; the Ocean Tracking Network and CEOTR glider group for the deployment, recovery and maintenance of gliders, Adam Comeau, Jude Van der Meer, Tyler Byrne, Richard Davis, among many. We thank you to all groups who contributed NARW sightings and effort data; namely the North Atlantic right whale Consortium, Anderson Cabot Centre for Marine Life at the New England Aquarium, Canadian Whale Institute, Mingan Island Cetacean Study, National Atmospheric and Oceanic Administration Northeast Fisheries Science Center, Transport Canada and Fisheries and Oceans Canada. We are indebted to Mark Baumgartner, for the use of the LFDCS and Genevieve Davis and Julianne Gurnee for the much-appreciated assistance in NARW call classification.
Conflict of interest
The authors declare that the research was conducted in the absence of any commercial or financial relationships that could be construed as a potential conflict of interest.
Publisher’s note
All claims expressed in this article are solely those of the authors and do not necessarily represent those of their affiliated organizations, or those of the publisher, the editors and the reviewers. Any product that may be evaluated in this article, or claim that may be made by its manufacturer, is not guaranteed or endorsed by the publisher.
Supplementary material
The Supplementary Material for this article can be found online at: https://www.frontiersin.org/articles/10.3389/fmars.2022.976044/full#supplementary-material
References
Batten S. D., Burkill P. H. (2010). The continuous plankton recorder: towards a global perspective. J. Plankton Res. 32, 1619–1621. doi: 10.1093/plankt/fbq140
Baumgartner M. F., Bonnell J., Corkeron P. J., Van Parijs S. M., Hotchkin C., Hodges B. A., et al. (2020). Slocum Gliders provide accurate near real-time estimates of baleen whale presence from human-reviewed passive acoustic detection information. Front. Mar. Sci. 7. doi: 10.3389/fmars.2020.00100
Baumgartner M. F., Bonnell J., Van Parijs S. M., Corkeron P. J., Hotchkin C., Ball K., et al. (2019). Persistent near real-time passive acoustic monitoring for baleen whales from a moored buoy: System description and evaluation. Methods Ecol. Evol. 10, 1476–1489. doi: 10.1111/2041-210x.13244
Baumgartner M. F., Cole T. V. N., Campbell R. G., Teegarden G. J., Durbin E. G. (2003). “Associations between north Atlantic right whales and their prey, calanus finmarchicus, over diel and tidal time scales,” in Marine ecology progress series 264, 155–166. doi: 10.3354/meps264155
Baumgartner M. F., Fratantoni D. M. (2008). Diel periodicity in both sei whale vocalization rates and the vertical migration of their copepod prey observed from ocean gliders. Limnol. Oceanogr. 53, 2197–2209. doi: 10.4319/lo.2008.53.5_part_2.2197
Baumgartner M. F., Mayo C. A., Kenney R. D. (2007). “Enormous carnivores, microscopic food, and a restaurant that’s hard to find,” in Urban whale n. atl. right whales crossroads (Camb. MA: Harv. Univ. Press), 138–171.
Baumgartner M. F., Mussoline S. E. (2011). A generalized baleen whale call detection and classification system. J. Acoust. Soc Am. 129, 2889–2902. doi: 10.1121/1.3562166
Bittencourt L., Barbosa M., Secchi E., Lailson-Brito J., Azevedo A. (2016). Acoustic habitat of an oceanic archipelago in the southwestern Atlantic. Deep Sea Res. Part Oceanogr. Res. Pap. 115, 103–111. doi: 10.1016/j.dsr.2016.06.001
Bourque L., Wimmer T., Lair S., Jones M., Daoust P. (2020). Incident report: North Atlantic right whale mortality event in Eastern Canada 2019. Collab. Rep. Prod. Can. Wildl. Health Coop. Mar. Anim. Response Soc 210 p.
Brillant S. W., Vanderlaan A. S. M., Rangeley R. W., Taggart C. T. (2015). Quantitative estimates of the movement and distribution of north Atlantic right whales along the northeast coast of north America. Endanger. Species Res. 27, 141–154. doi: 10.3354/esr00651
Caesar L., Rahmstorf S., Robinson A., Feulner G., Saba V. (2018). Observed fingerprint of a weakening Atlantic ocean overturning circulation. Nature 556, 191–196. doi: 10.1038/s41586-018-0006-5
Christiansen F., Bejder L., Burnell S., Ward R., Charlton C. (2022). Estimating the cost of growth in southern right whales from drone photogrammetry data and long-term sighting histories. Mar. Ecol. Prog. Ser. 687, 173–194. doi: 10.3354/meps14009
Clark C. W. (1982). The acoustic repertoire of the southern right whale, a quantitative analysis. Anim. Behav. 30, 1060–1071. doi: 10.1016/S0003-3472(82)80196-6
Clark C. W. (1983). Acoustic communication and behavior of the southern right whale (Eubalaena australis). Commun. Behav. Whales 163–198.
Clark C. W., Brown M. W., Corkeron P. J. (2010). Visual and acoustic surveys for north Atlantic right whales, eubalaena glacialis, in cape cod bay, Massachusetts 2001-2005: Management implications. Mar. Mammal. Sci. 26, 837–854. doi: 10.1111/j.1748-7692.2010.00376.x
Cominelli S., Halliday W. D., Pine M. K., Hilliard R. C., Lawson J. W., Duman N. I., et al. (2020). Vessel noise in spatially constricted areas: Modeling acoustic footprints of large vessels in the Cabot strait, Eastern Canada. Ocean Coast. Manage. 194, 105255. doi: 10.1016/j.ocecoaman.2020.105255
Conn P. B., Silber G. K. (2013). Vessel speed restrictions reduce risk of collision-related mortality for north Atlantic right whales. Ecosphere, 4 (4), 1–16. doi: 10.1890/ES13-00004.1
Conover R. J. (1988). Comparative life histories in the genera calanus and neocalanus in high latitudes of the northern hemisphere. Hydrobiologia, 167 (1), 127–142. doi: 10.1007/BF00026299
Cooke J. (2020)IUCN red list of threatened species: Eubalaena glacialis [WWW document]. In: IUCN red list threat. species. Available at: https://www.iucnredlist.org/en (Accessed 9.21.20).
Crowe L. M., Brown M. W., Corkeron P. J., Hamilton P. K., Ramp C., Ratelle S., et al. (2021). In plane sight: a mark-recapture analysis of north Atlantic right whales in the gulf of st. Lawrence. Endanger. Species Res. 46, 227–251. doi: 10.3354/esr01156
Daoust P., Couture E., Wimmer T., Bourque L. (2017). Incident report: North Atlantic right whale mortality event in the gulf of st. Lawrence 2017. Can. Wildlife Health Cooperative Mar. Anim. Response Society Fisheries Oceans Canada. 256.
Davies K. T. A., Brown M., Hamilton P., Knowlton A., Taggart C., Vanderlaan A. (2019). Variation in north Atlantic right whale eubalaena glacialis occurrence in the bay of fundy, Canada, over three decades. Endanger. Species Res. 39, 159–171. doi: 10.3354/esr00951
Davies K. T. A., Vanderlaan A. S. M., Smedbol R. K., Taggart C. T. (2015). Oceanographic connectivity between right whale critical habitats in Canada and its influence on whale abundance indices during 1987–2009. J. Mar. Syst. 150, 80–90. doi: 10.1016/j.jmarsys.2015.05.005
Davis G. E., Baumgartner M. F., Bonnell J. M., Bell J., Berchok C., Bort Thornton J., et al. (2017). Long-term passive acoustic recordings track the changing distribution of north Atlantic right whales (Eubalaena glacialis) from 2004 to 2014. Sci. Rep. 7, 13460. doi: 10.1038/s41598-017-13359-3
Delarue J., Kowarski K., Maxner E., MAcDonnell J. T., Martin B. (2018). “Acoustic monitoring along canada’s East coast: August 2015 to July 2017,” in Document number 01279, environmental studies research funds report number 215, version 1.0 (Dartmouth, NS, Canada: JASCO Applied Sciences for Environmental Studies Research Fund), 120 pp + appendices.
Delarue J., Moors-Murphy H., Kowarski K., Davis G., Urazghildiiev I., Martin S. (2022). Acoustic occurrence of baleen whales, particularly blue, fin, and humpback whales, off eastern Canada 2015-2017. Endanger. Species Res. 47, 265–289. doi: 10.3354/esr01176
Durette-Morin D., Davies K. T. A., Johnson H. D., Brown M. W., Moors-Murphy H., Martin B., et al. (2019). Passive acoustic monitoring predicts daily variation in north Atlantic right whale presence and relative abundance in roseway basin, Canada. Mar. Mammal. Sci. 35, 1280–1303. doi: 10.1111/mms.12602
Environment Canada, Canadian Ice Service (2011). Sea Ice climatic atlas (East Coast of Canada: Government of Canada Publications), 1981–2010.
Favilla A. B., Horning M., Costa D. P. (2021). Advances in thermal physiology of diving marine mammals: The dual role of peripheral perfusion. Temperature 0 null 9 (1), 46–66. doi: 10.1080/23328940.2021.1988817
Franklin K. J. (2020). Using sonobuoys and visual surveys to characterize north Atlantic right whale (Eubalaena glacialis) acoustic ecology in the gulf of st. Lawrence (Undergraduate honour’s thesis) (Canada: Dalhousie University).
Franklin K. J., Cole T. V., Cholewiak D. M., Duley P. A., Crowe L. M., Hamilton P. K., et al (2022). Using sonobuoys and visual surveys to characterize North Atlantic right whale (Eubalaena glacialis) calling behavior in the Gulf of St. Lawrence (Endangered Species Research). 49, 159–174. doi: 10.3354/esr01208
Greene C. (2016). North america’s iconic marine species at risk due to unprecedented ocean warming. Oceanography 29, 14–17. doi: 10.5670/oceanog.2016.67
Greene C. H., Meyer-Gutbrod E., Monger B. C., McGarry L. P., Pershing A. J., Belkin I. M., et al. (2013). Remote climate forcing of decadal-scale regime shifts in Northwest Atlantic shelf ecosystems. Limnol. Oceanogr. 58, 803–816. doi: 10.4319/lo.2013.58.3.0803
Greene C. H., Pershing A. J., Conversi A., Planque B., Hannah C., Sameoto D., et al. (2003). Trans-Atlantic responses of calanus finmarchicus populations to basin-scale forcing associated with the north Atlantic oscillation. Prog. Oceanogr. 58, 301–312. doi: 10.1016/j.pocean.2003.08.009
Grieve B. D., Hare J. A., Saba V. S. (2017). Projecting the effects of climate change on calanus finmarchicus distribution within the U.S. Northeast Continental Shelf. Sci. Rep. 7, 6264. doi: 10.1038/s41598-017-06524-1
Hamilton P. K., Knowlton A. R., Marx M. K. (2007). “Right whales tell their own stories: The photo-identification catalog,” in Chapter 1 in the urban whale: North Atlantic right whales at a crossroads. Eds. Kraus S. D., Rolland R. M. (Cambridge, MA: Harvard University Press).
Hay K. (1982). Aerial line-transect estimates of abundance of humpback, fin, and long-finned pilot whales in the Newfoundland-Labrador area. Rep. Int. Whal. Commun. 32, 475–486.
Hayes S. A., Gardner S., Garrison L. P., Henry A., Leandro L. (2018). North Atlantic right whales - evaluating their recovery challenges in 2018. doi: 10.25923/w9cy-5844
Head E. J. H., Melle W., Pepin P., Bagøien E., Broms C. (2013). On the ecology of calanus finmarchicus in the subarctic north Atlantic: A comparison of population dynamics and environmental conditions in areas of the Labrador Sea-Labrador/Newfoundland shelf and Norwegian Sea Atlantic and coastal waters. Prog. Oceanogr. Norway-Canada Comparison Mar. Ecosyst. (NORCAN) 114, 46–63. doi: 10.1016/j.pocean.2013.05.004
Hildebrand J. A., Frasier K. E., Baumann-Pickering S., Wiggins S. M., Merkens K. P., Garrison L. P., et al. (2019). Assessing seasonality and density from passive acoustic monitoring of signals presumed to be from pygmy and dwarf sperm whales in the gulf of Mexico. Front. Mar. Sci. 6. doi: 10.3389/fmars.2019.00066
Hokkanen J. E. (1990). Temperature regulation of marine mammals. J. Theor. Biol. 145, 465–485. doi: 10.1016/S0022-5193(05)80482-5
Jacobsen K.-O., Marx M., Oien N. (2004). Two-way trans-Atlantic migration of a north Atlantic right whale (Eubalaena glacialis). Mar. Mammal. Sci. 20, 161–166. doi: 10.1111/j.1748-7692.2004.tb01147.x
Johnson H. D. (2022). Advancing baleen whale acoustic and habitat monitoring in the Northwest Atlantic (PhD thesis) ( Canada: Dalhousie University).
Johnson H. D., Baumgartner M. F., Taggart C. T. (2020). Estimating north Atlantic right whale (Eubalaena glacialis) location uncertainty following visual or acoustic detection to inform dynamic management. Conserv. Sci. Pract. 2, e267. doi: 10.1111/csp2.267
Johnson C., Devred E., Casault B., Head E., Spry J. (2017). Optical, chemical, and biological oceanographic conditions on the scotian shelf and in the Eastern gulf of Maine in 2015 Sci. Advis. Sec. Res. Doc. 58, 53.
Johnson M., Hurst T. (2007). “The DMON: an open-hardware/open-software passive acoustic detector,” in 3rd international workshop on the detection and classification of marine mammals using passive acoustics, Boston, Massachusetts, USA 12.
Johnson H., Morrison D., Taggart C. (2021). WhaleMap: a tool to collate and display whale survey results in near real-time. J. Open Source Software 6, 3094. doi: 10.21105/joss.03094
Kann L. M., Wishner K. (1995). Spatial and temporal patterns of zooplankton on baleen whale feeding grounds in the southern gulf of Maine. J. Plankton Res. 17, 235–262. doi: 10.1093/plankt/17.2.235
Kanwisher J. W., Ridgway S. H. (1983). The physiological ecology of whales and porpoises. Sci. Am. 248, 110–120. doi: 10.1038/scientificamerican0683-110
Kenney R. D. (2001). Anomalous 1992 spring and summer right whale (Eubalaena glacialis) distributions in the gulf of Maine. J. Cetacean Res. Manage, 209–223. doi: 10.47536/jcrm.vi.287
Khan C. B., Duley P., Henry A., Gatzke J., Crowe L., Cole T. V. N. (2014). North Atlantic right whale sighting survey (NARWSS). (NOAA). Available at: https://repository.library.noaa.gov/view/noaa/9051.
Klinck H., Mellinger D. K., Klinck K., Bogue N. M., Luby J. C., Jump W. A., et al. (2012). Near-Real-Time acoustic monitoring of beaked whales and other cetaceans using a SeagliderTM. PLos One 7, e36128. doi: 10.1371/journal.pone.0036128
Knowlton A., Kraus S. (2020). Mortality and serious injury of northern right whales (Eubalaena glacialis) in the western north Atlantic ocean. J. Cetacean Res. Ldots 2, 193–208. doi: 10.47536/jcrm.vi.288
Knowlton A. R., Sigukjosson J., Ciano J. N., Kraus S. D. (1992). Long-distance movements of north Atlantic right whales (Eubalaena glacialis). Mar. Mammal. Sci. 8, 397–405. doi: 10.1111/j.1748-7692.1992.tb00054.x
Kowarski K. (2020). Humpback whale signing behaviour in the Western north Atlantic: from methods for analysing passive acoustic monitoring data to understanding humpback whale song ontogeny (PhD thesis) (Canada: Dalhousie University).
Kowarski K. A., Delarue J. J.-Y., Gaudet B. J., Martin S. B. (2021). Automatic data selection for validation: A method to determine cetacean occurrence in large acoustic data sets. JASA Express Lett. 1, 051201. doi: 10.1121/10.0004851
Laurinolli M. H., Hay A. E., Desharnais F., Taggart C. T. (2003). Locatization of north Atlantic right whale sonunds in the bay of fundy using a sonobuoy array. Mar. Mammal. Sci. 19, 708–723. doi: 10.1111/j.1748-7692.2003.tb01126.x
Lawson J. W., Gosselin J.-F. (2009). Distribution and preliminary abundance estimates for cetaceans seen during canada’s marine megafauna survey - a component of the 2007 TNASS. DFO Can. Sci. Advis. Sec. Res. Doc. 2009/031, iv + 29.
Lien J., Sears R., Stenson G. B., Jones P. W., Ni I.-H. (1989). Right whale, eubalaena glacialis, sightings in waters off Newfoundland and Labrador and the gulf of st. lawrence 1978-1987. Can. Field-Nat. 103 (1), 91–93.
Matthews J., Brown S., Gillespie D. (2001). Vocalisation rates of the north Atlantic right whale (Eubalaena glacialis). J. Cetacean Ldots. 3 (3), 271–282. doi: 10.1121/1.4824682
Matthews L. P., McCordic J., Parks S. E. (2014). Remote acoustic monitoring of north Atlantic right whales (Eubalaena glacialis) reveals seasonal and diel variations in acoustic behavior. PLos One 9, e91367. doi: 10.1371/journal.pone.0091367
Melle W., Runge J., Head E., Plourde S., Castellani C., Licandro P., et al. (2014). The north Atlantic ocean as habitat for calanus finmarchicus: Environmental factors and life history traits. Prog. Oceanogr. North Atlantic Ecosystems role Climate anthropogenic forcing their structure Funct. 129, 244–284. doi: 10.1016/j.pocean.2014.04.026
Mellinger D. K., Nieukirk S. L., Klinck K., Klinck H., Dziak R. P., Clapham P. J., et al. (2011). Confirmation of right whales near a nineteenth-century whaling ground east of southern Greenland. Biol. Lett. 7, 411–413. doi: 10.1098/rsbl.2010.1191
Mellinger D. K., Nieukirk S. L., Matsumoto H., Heimlich S. L., Dziak R. P., Haxel J., et al. (2007a). Seasonal occurrence of north Atlantic right whale (Eubalaena glacialis) vocalizations at two sites on the scotian shelf. Mar. Mammal. Sci. 23, 856–867. doi: 10.1111/j.1748-7692.2007.00144.x
Mellinger D. K., Stafford K. M., Moore S. E., Dziak R. P., Matsumoto H. (2007b). An overview of fixed passive acoustic observation methods for cetaceans. Oceanography 20, 36–45. doi: 10.5670/oceanog.2007.03
Meyer-Gutbrod E. L., Greene C. H. (2018). Uncertain recovery of the north Atlantic right whale in a changing ocean. Glob. Change Biol 24 (1), 455–464. doi: 10.1111/gcb.13929
Meyer-Gutbrod E. L., Greene C. H., Davies K. T. A. (2018). Marine species range shifts necessitate advanced policy planning: the case of the north Atlantic right whale. Oceanography 31 (2), 19–23. doi: 10.5670/oceanog.2018.209
Meyer-Gutbrod E. L., Greene C. H., Davies K. T., Johns D. G. (2021). Ocean regime shift is driving collapse of the north Atlantic right whale population. Oceanography 34 (3), 22–31. doi: 10.5670/oceanog.2021.308
Meyer-Gutbrod E. L., Greene C., Sullivan P., Pershing A. (2015). Climate-associated changes in prey availability drive reproductive dynamics of the north Atlantic right whale population. Mar. Ecol. Prog. Ser. 535, 243–258. doi: 10.3354/meps11372
Meyer-Gutbrod E., Davies K. T. A., Johnson C. L., Pluorde S., Sorochan K., Kenney R., et al (2022). Redefining North Atlantic right whale habitat use patterns under climate change. Limnology and Oceanography. 9999, 1–164. doi: 10.3354/esr01208
Monsarrat S., Pennino M. G., Smith T. D., Reeves R. R., Meynard C. N., Kaplan D. M., et al. (2015). Historical summer distribution of the endangered north Atlantic right whale (Eubalaena glacialis): a hypothesis based on environmental preferences of a congeneric species. Divers. Distrib. 21, 925–937. doi: 10.1111/ddi.12314
Moore D. (2017). Is emerald basin, scotian shelf, Canada, a north Atlantic right whale (Eubalaena glacialis) feeding habitat? (Undergraduate honour’s thesis) (Halifax, Nova Scotia: Dalhousie University).
Moore M. J., Rowles T. K., Fauquier D. A., Baker J. D., Biedron I., Durban J. W., et al. (2021). REVIEW assessing north Atlantic right whale health: threats, and development of tools critical for conservation of the species. Dis. Aquat. Organ. 143, 205–226. doi: 10.3354/dao03578
Moore S. E., Stafford K. M., Mellinger D. K., Hildebrand J. A. (2006). Listening for Large whales in the offshore waters of Alaska. BioScience 56, 49. doi: 10.1641/0006-3568(2006)056[0049:LFLWIT]2.0.CO;2
Moors-Murphy H. B., Lawson J. W., Rubin B., Marotte E., Renaud G., Fuentes-Yaco C. (2018). Occurrence of blue whales (Balaenoptera musculus) off Nova Scotia, Newfoundland, and Labrador. DFO Can. Sci. Advis Sec Res. Doc. 2018/007. iv + 55, 59.
Munger L. M., Mellinger D. K., Wiggins S. M., Moore S. E., Hildebrand J. A. (2005). Performance of spectrogram cross-correlation in detecting right whale calls in long-term recordings from the Bering Sea. Can. Acoust. 33, 25–34.
Nieukirk S. L., Stafford K. M., Mellinger D. K., Dziak R. P., Fox C. G. (2004). Low-frequency whale and seismic airgun sounds recorded in the mid-Atlantic ocean. J. Acoust. Soc Am. 115, 1832–1843. doi: 10.1121/1.1675816
Pace R. M. III, Corkeron P. J., Kraus S. D. (2017). State-space mark-recapture estimates reveal a recent decline in abundance of north Atlantic right whales. Ecol. Evol. 7 (21), 8730–8741. doi: 10.1002/ece3.3406
Parks S. E. (2003). Acoustic communication in the north Atlantic right whale (Eubalaena glacialis) (PhD thesis) (Campbridge: Massachusetts inst of tech cambridge).
Parks S. E., Hamilton P. K., Kraus S. D., Tyack P. L. (2005). The gunshot sounds produced by male north Atlantic right whales (Eubalaenae glacialis) and it’s potential function in reproductive advertisement. Mar. Mammal. Sci. 21, 458–475. doi: 10.1111/j.1748-7692.2005.tb01244.x
Parks S. E., Searby A., Célérier A., Johnson M. P., Nowacek D. P., Tyack P. L. (2011). Sound production behavior of individual north Atlantic right whales: Implications for passive acoustic monitoring. Endanger. Species Res. 15, 63–76. doi: 10.3354/esr00368
Parks S. E., Tyack P. L. (2005). Sound production by north Atlantic right whales (Eubalaena glacialis) in surface active groups. J. Acoust. Soc Am. 117, 3297–3306. doi: 10.1121/1.1882946
Patrician M. R., Kenney R. D. (2010a). Using the continuous plankton recorder to investigate the absence of north Atlantic right whales (Eubalaena glacialis) from the roseway basin foraging ground. J. Plankton Res. 32, 1685–1695. doi: 10.1093/plankt/fbq073
Pendleton D., Pershing A., Brown M., Mayo C., Kenney R., Record N., et al. (2009). Regional-scale mean copepod concentration indicates relative abundance of north Atlantic right whales. Mar. Ecol. Prog. Ser. 378, 211–225. doi: 10.3354/meps07832
Pepin P., Han G., Head E. J. (2013). Modelling the dispersal of calanus finmarchicus on the Newfoundland shelf: implications for the analysis of population dynamics from a high frequency monitoring site. Fish. Oceanogr. 22, 371–387. doi: 10.1111/fog.12028
Perry A. L., Low P. J., Ellis J. R., Reynolds J. D. (2005). Climate change and distribution shifts in marine fishes. science 308, 1912–1915. doi: 10.1126/science.1111322
Pershing A. J., Record N. R., Monger B. C., Mayo C. A., Brown M. W., Cole T. V. N., et al. (2009). Model-based estimates of right whale habitat use in the gulf of Maine. Mar. Ecol. Prog. Ser. 378, 245–257. doi: 10.3354/meps07829
Pershing A. J., Stamieszkin K. (2020). The north Atlantic ecosystem, from plankton to whales. Annu. Rev. Mar. Sci. 12, 339–359. doi: 10.1146/annurev-marine-010419-010752
Pettis H. M., Hamilton P. K. (2015). North Atlantic right whale consortium 2015 annual report card. Rep. N. Atl. Right Whale Consort. Novemb. 2015.
Pettis H. M., Hamilton P. K. (2016). North Atlantic right whale consortium annual report card. Rep. N. Atl. Right Whale Consort. Novemb. 2016.
Pinsky M. L., Worm B., Fogarty M. J., Sarmiento J. L., Levin S. A. (2013). Marine taxa track local climate velocities. Science 341, 1239–1242. doi: 10.1126/science.1239352
Plourde S., Lehoux C., Johnson C. L., Perrin G., Lesage V. (2019). North Atlantic right whale (Eubalaena glacialis) and its food: (I) a spatial climatology of calanus biomass and potential foraging habitats in Canadian waters. J. Plankton Res. 41, 667–685. doi: 10.1093/plankt/fbz024
Quintana-Rizzo E., Leiter S., Cole T. V. N., Hagbloom M. N., Knowlton A. R., Nagelkirk P., et al. (2021). Residency, demographics, and movement patterns of north Atlantic right whales eubalaena glacialis in an offshore wind energy development area in southern new England, USA. Endanger. Species Res. 45, 251–268. doi: 10.3354/esr01137
Record N. R., Ji R., Maps F., Varpe Ø., Runge J. A., Petrik C. M., et al. (2018). Copepod diapause and the biogeography of the marine lipidscape. J. Biogeogr. 45, 2238–2251. doi: 10.1111/jbi.13414
Record N., Runge J., Pendleton D., Balch W., Davies K. T. A., Pershing A., et al. (2019). Rapid climate-driven circulation changes threaten conservation of endangered north Atlantic right whales. Oceanography 32 (1), 162–169. doi: 10.5670/oceanog.2019.201
Reeves R. R. (2001). Overview of catch history, historic abundance and distribution of right whales in the western north Atlantic and in cintra bay, West Africa J. Cetacean Res. Manage. 6, 187–192. doi: 10.47536/jcrm.vi.289
Richardson A. J. (2008). In hot water: zooplankton and climate change. ICES J. Mar. Sci. 65, 279–295. doi: 10.1093/icesjms/fsn028
RWSAS, n.d. Northeast Fisheries Science Center. (2020). Right whale sightings advisory system (RWSAS). Available at: https://www.fisheries.noaa.gov/inport/item/23305https://www.fisheries.noaa.gov/inport/item/23305 (Accessed 12.12.20).
Saba V. S., Griffies S. M., Anderson W. G., Winton M., Alexander M. A., Delworth T. L., et al. (2016). Enhanced warming of the Northwest Atlantic ocean under climate change. J. Geophys. Res. Oceans 121, 118–132. doi: 10.1002/2015JC011346
Simard Y., Roy N., Giard S., Aulanier F. (2019). North Atlantic right whale shift to the gulf of st. Lawrence in 2015, revealed by long-term passive acoustics. Endanger. Species Res. 40, 271–284. doi: 10.3354/esr01005
Širović A., Hildebrand J. A., Wiggins S. M., McDonald M. A., Moore S. E., Thiele D. (2004). Seasonality of blue and fin whale calls and the influence of sea ice in the Western Antarctic peninsula. Deep Sea Res. Part II Top. Stud. Oceanogr. Integrated Ecosystem Stud. Western Antarctic Peninsula Continental Shelf Waters Related South. Ocean Regions 51, 2327–2344. doi: 10.1016/j.dsr2.2004.08.005
Smeed D. A., Josey S. A., Beaulieu C., Johns W. E., Moat B. I., Frajka-Williams E., et al. (2018). The north Atlantic ocean is in a state of reduced overturning. Geophys. Res. Lett. 45, 1527–1533. doi: 10.1002/2017GL076350
Sorochan K. A., Plourde S., Baumgartner M. F., Johnson C. L. (2021). Availability, supply, and aggregation of prey (Calanus spp.) in foraging areas of the north Atlantic right whale (Eubalaena glacialis). ICES J. Mar. Sci. 78 (10), 3498–3520. doi: 10.1093/icesjms/fsab200
Sousa-Lima R. S., Norris T. F., Oswald J. N., Fernandes D. P. (2013). A review and inventory of fixed autonomous recorders for passive acoustic monitoring of marine mammals. Aquatic Mammals 39, (1), 23–53. doi': 10.1109/RIOAcoustics.2013.6683984
Stafford K. M., Moore S. E., Spillane M., Wiggins S. (2007). Gray Whale calls recorded near Barrow, Alaska, throughout the winter of 2003-04. Arctic 60, 167–172. doi: 10.14430/arctic241
Stafford K. M., Nieukirk S. L., Fox C. G. (1999). Low-frequency whale sounds recorded on hydrophones moored in the eastern tropical pacific. J. Acoust. Soc Am. 106, 3687–3698. doi: 10.1121/1.428220
Stanistreet J. E., Nowacek D. P., Baumann-Pickering S., Bell J. T., Cholewiak D. M., Hildebrand J. A., et al. (2017). Using passive acoustic monitoring to document the distribution of beaked whale species in the western north Atlantic ocean. Can. J. Fish. Aquat. Sci. 74, 2098–2109. doi: 10.1139/cjfas-2016-0503
Stevick P. T., Neves M. C., Johansen F., Engel M. H., Allen J., Marcondes M. C. C., et al. (2011). A quarter of a world away: female humpback whale moves 10 000 km between breeding areas. Biol. Lett. 7, 299–302. doi: 10.1098/rsbl.2010.0717
Thomisch K., Boebel O., Zitterbart D. D. P., Samaran F., Van Parijs S. M., Van Opzeeland I. (2015). Effects of subsampling of passive acoustic recordings on acoustic metrics. J. Acoust. Soc Am. 138, 267–278. doi: 10.1121/1.4922703
van der Hoop J., Corkeron P., Moore M. (2017). Entanglement is a costly life-history stage in large whales. Ecol. Evol. 7, 92–106. doi: 10.1002/ece3.2615
van der Hoop J. M., Moore M. J., Barco S. G., Cole T. V. N., Daoust P. Y., Henry A. G., et al. (2013). Assessment of management to mitigate anthropogenic effects on large whales. Conserv. Biol. 27, 121–133. doi: 10.1111/j.1523-1739.2012.01934.x
van der Hoop J. M., Vanderlaan A. S. M., Taggart C. T. (2012). Absolute estimates of lethal probability to north Atlantic right whales in roseway vessel basin, strikes scotian shelf. Ecol. Appl. 22, 2021–2033. doi: 10.2307/1942049
Vanderlaan A. S. M., Taggart C. T. (2007). Vessel collisions with whales: The probability of lethal injury based on vessel speed. Mar. Mammal. Sci. 23, 144–156. doi: 10.1111/j.1748-7692.2006.00098.x
Van Parijs S. M., Clark C. W., Sousa-Lima R. S., Parks S. E., Rankin S., Risch D., et al. (2009). Management and research applications of real-time and archival passive acoustic sensors over varying temporal and spatial scales. Mar. Ecol. Prog. Ser. 395, 21–36. doi: 10.3354/meps08123
Waite J. M., Wynne K., Mellinger D. K. (2003). Documented sighting of a north pacific right whale in the gulf of Alaska and post-sighting acoustic monitoring. Northwest. Nat. 84 (1), 38–43. doi: 10.2307/3536721
Keywords: North Atlantic right whale (Eubalaena glacialis), passive acoustic monitoring (PAM), distribution, range expansion, regime shift
Citation: Durette-Morin D, Evers C, Johnson HD, Kowarski K, Delarue J, Moors-Murphy H, Maxner E, Lawson JW and Davies KTA (2022) The distribution of North Atlantic right whales in Canadian waters from 2015-2017 revealed by passive acoustic monitoring. Front. Mar. Sci. 9:976044. doi: 10.3389/fmars.2022.976044
Received: 22 June 2022; Accepted: 07 November 2022;
Published: 08 December 2022.
Edited by:
Ian David Jonsen, Macquarie University, AustraliaReviewed by:
Genevieve Davis, Northeast Fisheries Science Center (NOAA), United StatesRhianne Ward, Curtin University, Australia
Copyright © 2022 Durette-Morin, Evers, Johnson, Kowarski, Delarue, Moors-Murphy, Maxner, Lawson and Davies. This is an open-access article distributed under the terms of the Creative Commons Attribution License (CC BY). The use, distribution or reproduction in other forums is permitted, provided the original author(s) and the copyright owner(s) are credited and that the original publication in this journal is cited, in accordance with accepted academic practice. No use, distribution or reproduction is permitted which does not comply with these terms.
*Correspondence: Delphine Durette-Morin, ZC5kdXJldHRlbW9yaW5AZ21haWwuY29t