- 1Departamento de Pesca e Aquicultura, Universidade Federal Rural de Pernambuco (UFRPE), Recife, PE, Brazil
- 2MARBEC, Univ Montpellier, CNRS, Ifremer, Institut de Recherche pour le Développement (IRD), Sète, France
- 3IRD, Univ Brest, CNRS, Ifremer, LEMAR, Plouzané, France
- 4Departamento de Oceanografia, Universidade Pernambuco (UFPE), Recife, PE, Brazil
- 5Departamento de Pesca e Aquicultura, Universidade Federal de Sergipe (UFS), São Cristovão, Sergipe, Brazil
Introduction: Competition embodies species struggle for space and food and is, therefore, a critical evolutionary mechanism influencing species spatiotemporal patterns and persistence. One of the main drivers of competition is resource availability. In marine ecosystems, resource availability is determined, among other things, by habitat structural complexity, as it increases biodiversity and species abundance. In this context, our study aims at understanding how the differences in substrate complexity affect potential trophic competition between demersal fishes in Northeast Brazil.
Methods: We selected two zones contrasted in terms of substrates, one dominated by sand (zone A) and the other by complex substrates such as reefs and calcareous algae (zone B). We used Stable Isotope Analysis (SIA) to quantify intraspecific and interspecific interactions of demersal fishes in both zones. We compared the competitive interactions between zones using social network analysis (SNA), a suitable method to quantitatively study a set of interactions.
Results: In the sand-dominated zone, demersal fish showed greater interspecific competition and occupied a larger isotopic niche suggesting that a resource-limited context led to diet diversification in the community. Some species expanded their niche through diet plasticity, while others showed higher intraspecific competition than in Zone B to cope with the greater interspecific competition.
Discussion: Combining SNA and SIA provided a new method to investigate competition. This study potentially gives a perspective on the future ecological response of the demersal fishes at the community and species level. With habitat degradation and climate change, the complex substrates in zone B will probably disappear gradually, transforming this zone into an ecosystem akin to zone A. This would be detrimental to species more vulnerable to interspecific competition, particularly those targeted by fisheries.
1. Introduction
Competition embodies species struggle for space and food and is, therefore, a critical evolutionary mechanism influencing species spatiotemporal patterns and persistence (Cure et al., 2018). Consequently, describing the processes underlying competitive interactions at individual and species levels has received increasing attention from ecologists (Bolnick et al., 2010; Mendes et al., 2019). It has been recognized that environmental conditions determine resource availability, which is one of the main drivers of competition (Zirbel et al., 2017; Huey and Kingsolver, 2019). For instance, habitat structural complexity within marine ecosystems increases species biodiversity and abundance and enhances prey availability for fish (González-Rivero et al., 2017). However, with habitat degradation and climate change, competitive interactions are expected to be profoundly modified, notably due to the increasing loss of complex substrates like coral reefs (Bonin et al., 2015). In a warming ocean with dwindling resources, predicting how species respond to different resource availability contexts is crucial (Kuczynski et al., 2018). As fisheries resources are critical to food security, it is imperative to anticipate how they may be affected by future environmental changes. One of the challenges of fisheries ecology is thus to understand the full range of trophic interactions between fishes for limited resources, including interspecific and intraspecific competition (Xia et al., 2020).
Modelling competition is not easy as it requires finding an adapted proxy to measure interactions. However, the concept of ecological niche, which refers to both the range of environmental conditions necessary for a species to persist and its ecological role in the ecosystem, has shed light on competitive processes (Jackson et al., 2011). In a resource-limited environment, a species trophic niche should be wide enough to avoid intraspecific competition, especially when species density is high, but narrow enough to reduce interspecific competition (Bolnick et al., 2010; Mendes et al., 2019). The Niche Variation Hypothesis (NVH) states that a species niche width is set to maximize individuals foraging efficiency and depends on individual phenotype and resource availability (Van Valen, 1965). Therefore, a species will increase its trophic niche width as much as possible in the context of reduced prey availability or diversity through resource sharing among individuals (Cachera et al., 2017). Although individuals could feed on various items, they will adopt a more specialized diet to avoid intraspecific competition and thus increase species trophic niche. Consequently, trophic niche width can be used as an indicator of species ability to develop strategies (e.g., niche partitioning between juveniles and adults, trophic plasticity) for coping successfully in response to competition and avoiding extinction (Andrades et al., 2019; Cathcart et al., 2019).
Stable isotope analysis (SIA) enables investigating and quantifying trophic niche (Marshall et al., 2019). Hence, SIA can be used to understand intraspecific competition by measuring the isotopic niche width as a proxy of diet diversity (Andrades et al., 2021). In addition, the isotopic niche overlap among species can illustrate interspecific competition by reflecting species capacity to explore isotopically similar sources (Marshall et al., 2019; Ogloff et al., 2019). Thus, trophic niche overlap accounts for the asymmetry in pairwise interspecific competition. For example, species A may overlap with species B entire isotopic niche, while species B may only partially overlap with species A niche, suggesting that species A may eat everything that species B eats but that the reverse is not true (Dominguez Almela et al., 2021). This way, identifying winning and losing species in potential pairwise competitive interactions allows the detection of vulnerable taxa in the context of diminishing resources (Andrades et al., 2021).
However, as ecological dynamics within communities are determined by the set of interactions between species, it is useful to look at the structural properties of the interactions (Olivier et al., 2019; Frelat et al., 2022). Thus, to get a more relevant idea of competition within a community, it is important to represent all pairwise interactions in a system where asymmetric competitive interactions connect species. Social Network Analysis (SNA) is an appropriate method to study a set of species interactions and investigate their structure quantitatively (Runghen et al., 2021). In marine and coastal environments, SNA has been used as a simple way to model predator-prey relationships without needing the mass balance required in ecological network analysis (e. g. Ecopath) (Rakshit et al., 2017; Olivier et al., 2019). Still, networks of non-trophic interactions such as interspecific competition are less studied, even though they could improve our predictive ability of how communities respond to disturbances (Kéfi et al., 2015). This method may help understand how resource availability affects the structure of competitive interactions in a community. The SNA has never been carried out with isotopic data, although these better represent interactions that persist over time than data on stomach contents.
Numerous scenarios of resource availability have already been set up in the laboratory to understand its impact on species metabolism or behaviour (Donelson et al., 2010; Gobler et al., 2018). However, few studies use real examples of contrasting habitats, which are relevant for studying species response without laboratory bias (González-Rivero et al., 2017; Neves et al., 2021). The continental shelf of northeastern Brazil is an interesting case study since it is a classic tropical system composed of a mosaic of sub-systems. This heterogeneity, particularly in terms of substrate diversity (sand, coral, algae), strongly influences the composition of the demersal fish community (Eduardo et al., 2018). Thus, since substrate differences determine the abundance and diversity of demersal fish, it is likely to affect trophic competition.
Tropical ecosystems such as northeastern Brazil are likely to be severely impacted by climate change (França et al., 2020), and it is essential to have information on these regions, which are generally poorly studied. Moreover, the socio-economic importance of demersal fish to local small-scale fisheries (Pelage et al., 2021) raises the need to understand their adaptive capacity to future environmental changes for management purposes. In this context, we combined SNA with SIA to understand how the differences in substrate complexity affect the structure of potential trophic competition among and within demersal fishes in Northeast Brazil. This was an opportunity to test whether 1) species expand their niches in a resource-limited context, as stated by the NVH and 2) whether competitive interactions are enhanced by a resource-limited context (as suggested by Darwin).
2. Material and methods
2.1. Study area
The study area extends along the continental shelf between the states of Rio Grande do Norte and Alagoas (4°–9°S) (Figure 1). This region is characterized by a gentle slope bounded by a sharp shelf break at 50–60 m deep. It is under the influence of the western boundary coastal system dominated by the North Brazil Undercurrent (Dossa et al., 2021). The stratification is low and the thermocline deep (ranging from ∼70 m to ∼170 m), so the shelf area is dominated by a warm (typically 26–29°C) and saline (usually 36.5–37.5) mixed-layer (Assunção et al., 2020). Despite its oligotrophic conditions, the region exhibits relatively high biodiversity (Eduardo et al., 2018; Tosetto et al., 2022). Within the study area, several Marine Protected Areas are established (e.g., “APA dos Corais”, ‘‘APA Costa dos Corais, ‘‘APA Guadalupe’’, ‘‘APA Santa Cruz’’, ‘‘APA Barra de Mamanguape) (Figure 1).
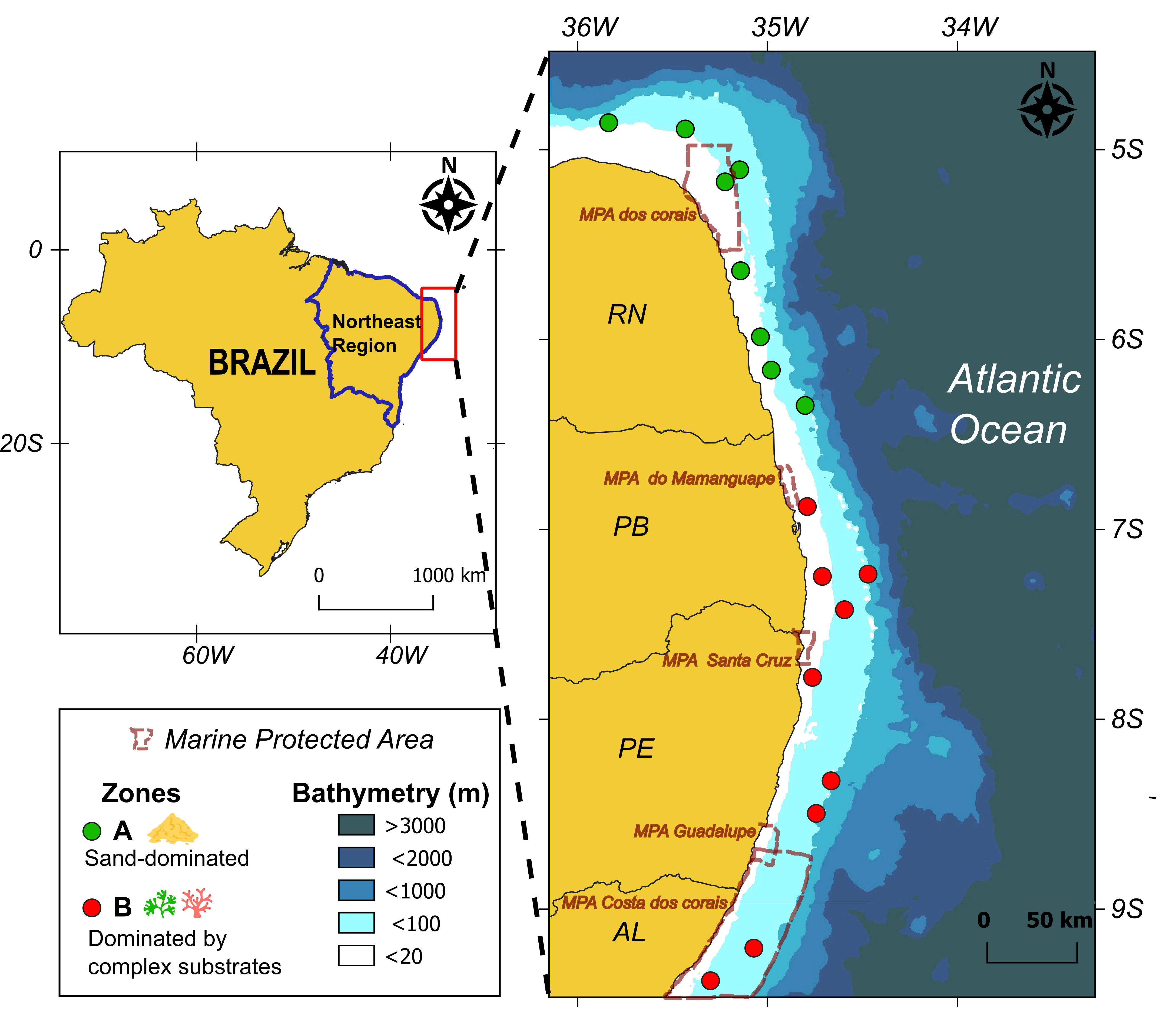
Figure 1 Study area with sampling points in northeastern Brazil, in two contrasting zones: one dominated by sand (green dots) and another with more complex substrates such as algae and coral reefs (red dots).AL, Alagoas; PB, Paraiba; PE, Pernambuco; RN, Rio Grande do Norte.
2.2. Sampling
Data were collected during the second Acoustics Along Brazilian Coast (ABRACOS2) survey performed onboard the R/V ANTEA from April 9 to May 9, 2017 (Bertrand, 2017). Fish were collected using a bottom trawl (body mesh: 40 mm, cod-end mesh: 25 mm, entrance dimensions horizontal x vertical: 28 ×10 m). Trawling was carried out at depths ranging between 15 and 65 m. Each haul lasted approximately 5 min at a mean speed of 3.2 kt. Tow duration was considered the moment of the arrival of the net on the pre-set depth to the lift-off time, recorded with a SCANMAR system. SCANMAR sensors were also used to check the net geometry and give the height, depth, and distance of the wings and doors to ensure that the net was working correctly. Bobbins were added to the bottom rope to reduce the trawling-induced impact on the benthic habitat and avoid damage to the net. Individuals were preserved in formalin (4%) for diet analysis or frozen for stable isotope analysis. At the laboratory, fish were measured (total length, TL, in cm) and weighted (total weight, TW, in g).
An underwater camera (GoPro HERO 3) mounted on the upper part of the net mouth took video footage to classify the bottom substrate. Detailed video analysis was undertaken in the laboratory, where all significant habitats were identified. At the sampling scale, it was possible to distinguish two areas based on the substrate characteristics resulting in differences in the composition (abundance and diversity) of demersal fish communities (Eduardo et al., 2018). Zone B, between 7 and 9°S, dominated by coral and algal formations (Figure 1), offered many ecological advantages, such as greater availability of resources, resulting in a higher abundance and diversity of species than in zone A, characterized by a prevalence of sand (Eduardo et al., 2018). A sediment classification also verified the substrates differences between both areas with data compiled from the Brazilian National Oceanographic Data Bank (BNDO) and literature (Vital et al., 2005; Amaral and dos Santos, 2015; de Assis et al., 2015; de Assis et al., 2016) (Supplementary Figure 1).
2.3. Data analysis
We selected the eight most representative species in the catch (74% of the total catch in number) to study trophic competition within the demersal fish community: Acanthurus chirurgus (Acanthuridae), Haemulon aurolineatum, Haemulon plumierii (Haemulidae), Holocentrus adscensionis (Holocentridae), Hypanus marianae (Dasyatidae), Lutjanus synagris (Lutjanidae), Pseudupeneus maculatus (Mullidae), and Sparisoma axillare (Scaridae). Large piscivorous species, which have a more remarkable ability to avoid fishing gear, were not captured. Still, presumably, this does not unduly bias our results as these fish are likely to have a predatory rather than competitive relationship with the selected species (Vaske and Teixeira Lessa, 2019).
The species were classified into trophic guilds, which were attributed by Ferreira et al. (2019) (Supplementary Table 1). We aimed to identify the main trophic compartments used by the selected species to get a rough idea of their diet in a poor-data context. We used gut content analysis of fish sampled during the cruise combined with literature data from an area as close as possible to our study area (Supplementary Table 2) and constructed a network showing the main trophic compartments and their relative importance in the diet of each selected species.
The catch per unit of effort (CPUE) was calculated considering the number of individuals of fish caught per trawled area (ind.km-2). The trawled area was estimated by multiplying the distance covered by the net through the bottom with the estimated gear mouth opening obtained through the SCANMAR sensors. The SCANMAR system was not operated in six trawls, and the average mouth opening (13 m) was used.
Demersal fish competition in both zones was investigated using the following three steps (Figure 2) (1): Stable isotope analysis (2), Calculation of interspecific and intraspecific competition indices, and (3) Social network analysis.
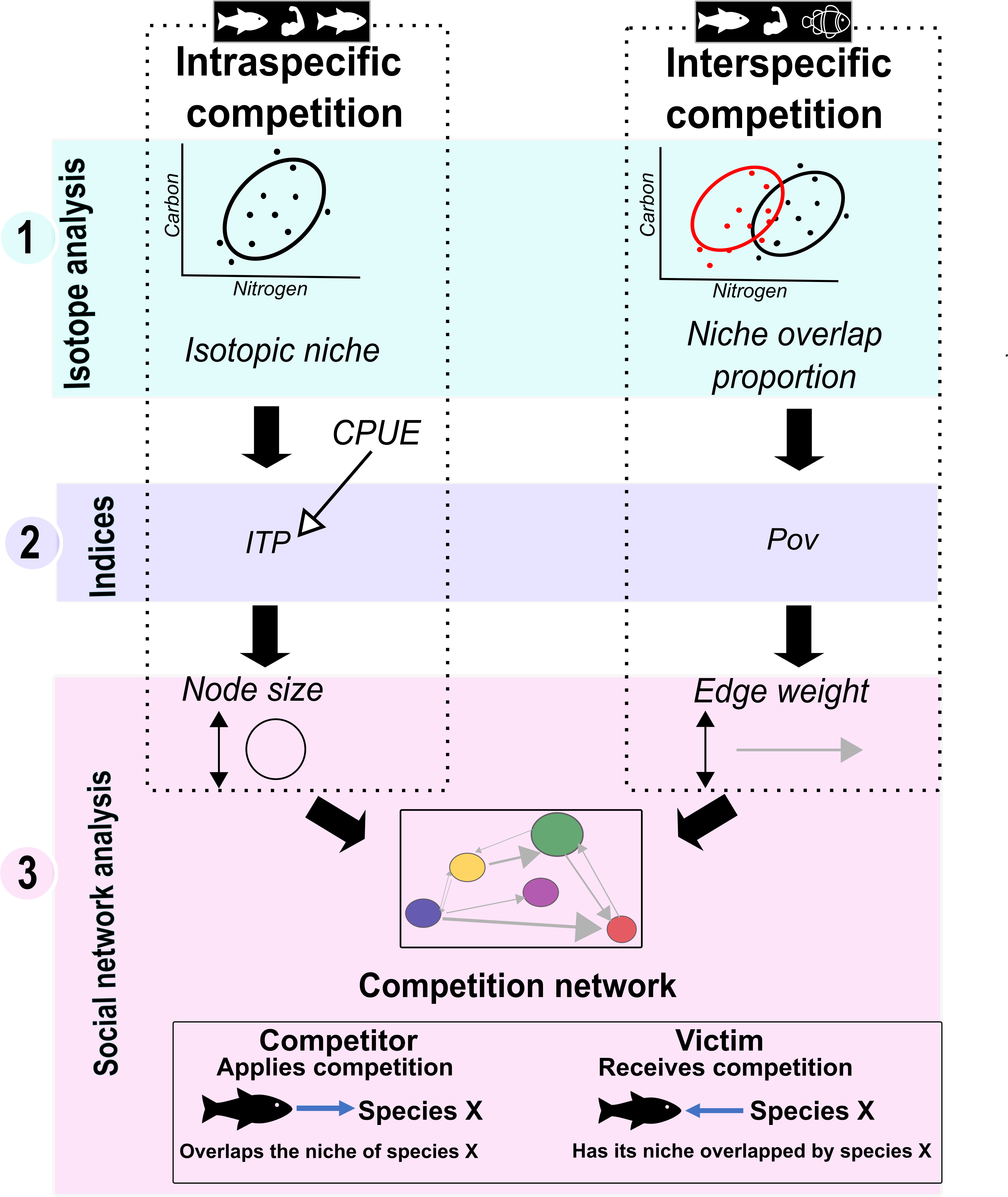
Figure 2 Diagram showing the three main methodological steps of data analysis (stable isotope analysis, index calculation, and modeling) to study competition within and among the selected species.
2.3.1. Stable isotope analysis
Samples were processed following Garcia et al. (2007). A fragment of dorsal white muscle from each fish was extracted and cleaned with distilled water to remove any remaining scales or bones. Thereupon, all samples were oven-dried at 60°C for 48 h. Dried samples were ground into a fine homogeneous powder with a mortar and pestle and then stored in clean Eppendorf tubes.
Analysis of carbon and nitrogen stable isotope ratios were performed at the Pôle de Spectrométrie Océan (PSO - IUEM, Plouzané, France) with an elemental analyzer (Thermo Flash 2000, interface Thermo ConFio IV) interfaced to a mass spectrometer (Thermo Delta V+). The isotope ratios for carbon (δ13C) and nitrogen (δ15N) were calculated from the relation between the sample isotopic value and a known standard:
In this equation, Rsample represents the ratio 13C/12C or 15N/14N. The Rstandard corresponds to the international standards, V-PDB (Vienna PeeDee Belemnite limestone), and the atmospheric nitrogen for carbon and nitrogen, respectively. Experimental precision, based on the standard deviation of repeated measurements of an internal laboratory standard (acetanilide and IVA), was monitored every six samples and defined as ± 0.11‰ (standard error) and ±0.07‰ for carbon and nitrogen, respectively. Since the C/N of all individuals was between 3.2 and 3.4, there was no need for a lipid extraction process to avoid bias in isotopic results for lipid-rich samples (C:N > 3.5) or when comparing species with varying lipid contents (Post, 2002).
The variation in fish length was constrained both within and among species whenever possible to avoid bias caused by ontogeny (Supplementary Table 3). The SIBER (Stable Isotope Bayesian Ellipses in R (Jackson et al., 2011);) package was used to estimate the total isotopic area (TA), the total nitrogen (dN) and carbon (dC) range occupied by the demersal fish community in each zone. We used Bayesian estimates to avoid sampling bias for the TA, dN and dC to propagate sampling error on the estimates of the means of community members and provide measures of uncertainty surrounding these metrics. This Bayesian approach increases the robustness of comparisons between zones by performing resampling simulations within each species and giving a value for each simulation (Jackson et al., 2011). The corrected standard ellipse area (SEAc) of each species, containing the 95% prediction ellipse interval, was calculated. As the SEAc incorporates a correction for sample size bias, the slight differences in sample size between our areas are not expected to influence our results (Supplementary Table 3).
Mean values of δ13C and δ15N, as well as SEAc, are available in Supplementary Table 3. To verify potential niche overlap among species, we calculated the overlap of the maximum likelihood fitted standard ellipses using the function maxLikOverlap based on a 95% prediction ellipse area.
2.3.2. Calculation of trophic competition indices
2.3.2.1. Intraspecific trophic competition
We adapted the Intraspecific Trophic Pressure (ITP) index from Andrades et al. (2021) to estimate the intraspecific competition. The ITP increases with the species density and decreases with species ability to use a wide range of resources. A high ITP indicates an abundant species or a species with limited resource use that is likely to be highly impacted in the context of habitat loss and limited resource availability.
In (Andrades et al., 2021), the ITP was calculated by dividing the transformed (log+1) mean fish density (ind.m−3) of a species by its isotopic niche width. In our case, the fish density was replaced by the CPUE:
where log(CPUEi+1) is the transformed (log+1) CPUE (ind.km−2) of species i and SEAcj i is the corrected standard ellipse area of species, containing the 95% prediction ellipse interval of species i, containing the 95% prediction ellipse interval.
2.3.2.2. Interspecific trophic competition
We investigated the interspecific competition between species pairs by quantifying the proportion of a species isotopic niche overlapped by another species. In the case where species i applied competition and species j received it, we calculated the interspecific competition as follows:
Where Povij is the proportion of species j ellipse overlapped by species i, Ovij is the overlap of the two corrected standard ellipses of species i and j, and SEAcj is the small sample size cored (i.e., core isotopic niche ellipses) of species i, containing the 95% prediction ellipse interval.
2.4. Social network analysis
We built a social network for each studied zone with the ggraph package (Pedersen et al., 2017). This network was constituted of nodes (one for each of the eight selected species) connected by arrows (edges) representing competitive interspecific trophic interactions. The size of the nodes was set according to species intraspecific trophic competition (ITP index), and the width of the arrows was a function of the interspecific trophic index (Pov). To quantify the strength of the competitive trophic interactions within the network of each studied zone, we calculated the shortest, largest, and average paths with the tnet package (Opsahl, 2015). Those metrics, commonly used in social network analysis, help measure network connectivity (Sosa et al., 2020). We used the variant of the metric designed for weighted (when interactions have different intensities) and directed (when interactions have directionality from one node to another) networks (Opsahl et al., 2010). In each zone, we run spearman correlations to seek potential correlations between Intraspecific (ITP) and Interspecific (Pov) trophic competition.
In both zones, we compared the intensity of interspecific trophic competition by representing all pairwise interactions between the selected species in a matrix. For each species, the trophic competition applied as a “competitor” (how much the species overlapped the other species niche) and received as a “victim” (how much of its niche was overlapped by the other species) was shown to visualize the asymmetry of the competitive interactions. This method enables us to infer each species vulnerability to interspecific competition in a system where they are both competitor (overlapping other species niche) and victim (whose niche is overlapped).
3. Results
The species were classified into two trophic guilds: herbivores and zoobenthivores (Figure 3). Crustaceans were the most intensively consumed compartment by the zoobenthivores (Supplementary Table 2). Hypanus marianae was the only species for which polychaetes made up a significant proportion of the diet (over 40%). Overall, most species feed at different trophic levels. Indeed, teleosts were a substantial part of some zoobenthivores diets, such as L. synagris (25%) or H. plumierii (12%) and a minor part of H. aurolineatum and H. marianae diets. Similarly, although herbivores primarily consumed algae and seagrass, animal material was encountered in their gut.
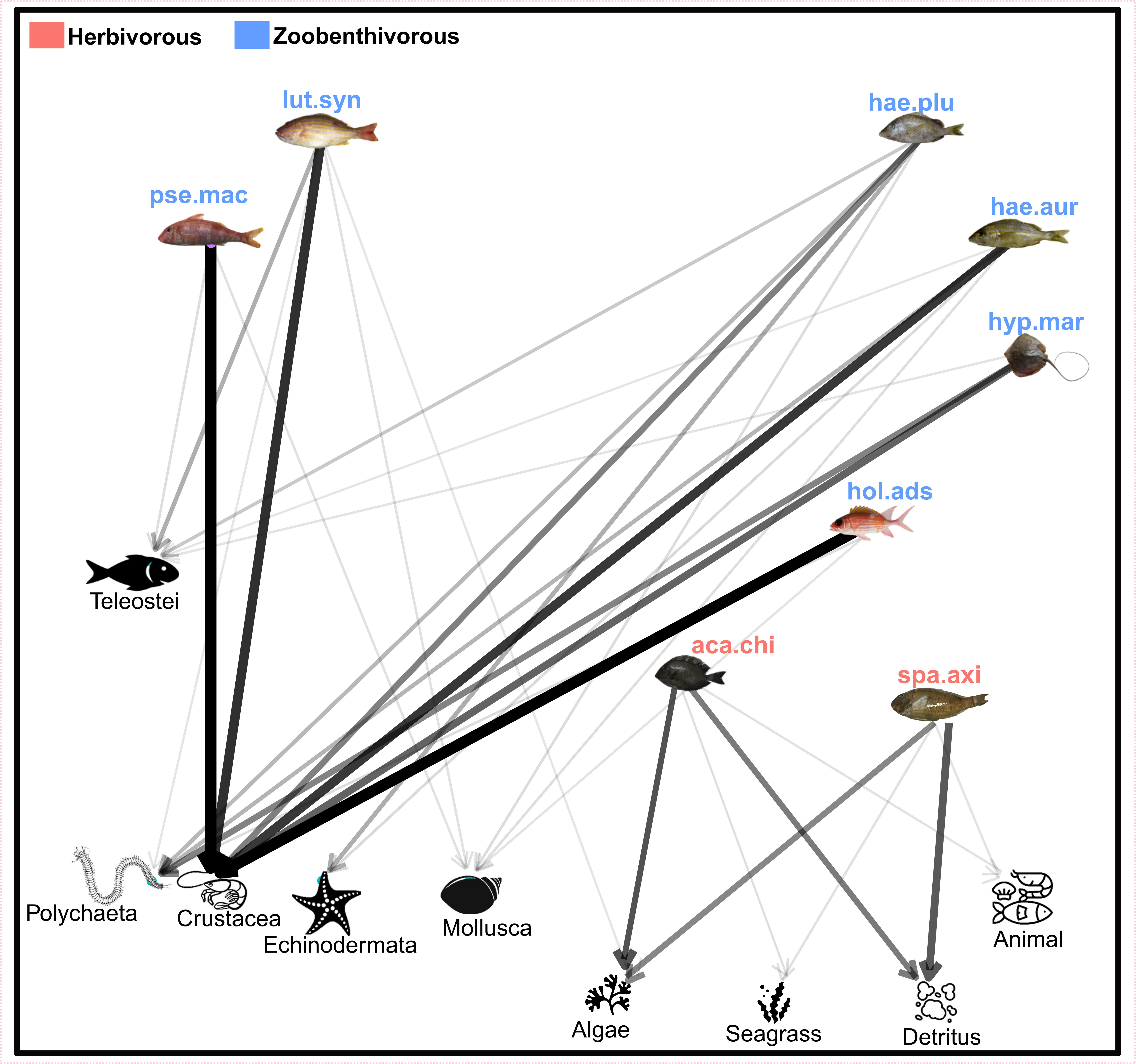
Figure 3 Network representing the main trophic compartments from which each selected species feeds. The thickness of the arrows corresponds to the importance of each item in the diet. lut.syn, Lutjanus synagris; pse.mac, Pseudopenneus maculatus; hae.plu, Haemulon plumierii; hae.aur, Haemulon aurolineatum; hyp.mar, Hypanus marianae; hol.ads, Holocentrus adscencionis; aca.chi, Acanthurus chirurgus; spa.axi, Sparisoma axillare.
The fish communities of the two zones showed different isotopic patterns (Figure 4; Supplementary Figure 2). Zone A, dominated by sand, exhibited a greater total isotopic area (TA) than zone B, where more complex substrates occur (85% of the TA values in zone A obtained with the Bayesian approach were higher than in zone B). Likewise, a greater range of carbon was observed in zone A (94% of the dC values in zone A obtained with the Bayesian approach were higher than in zone B). Conversely, the nitrogen range was slightly higher in zone B (66% of the dN values in zone B obtained with the Bayesian approach were higher than in zone A).
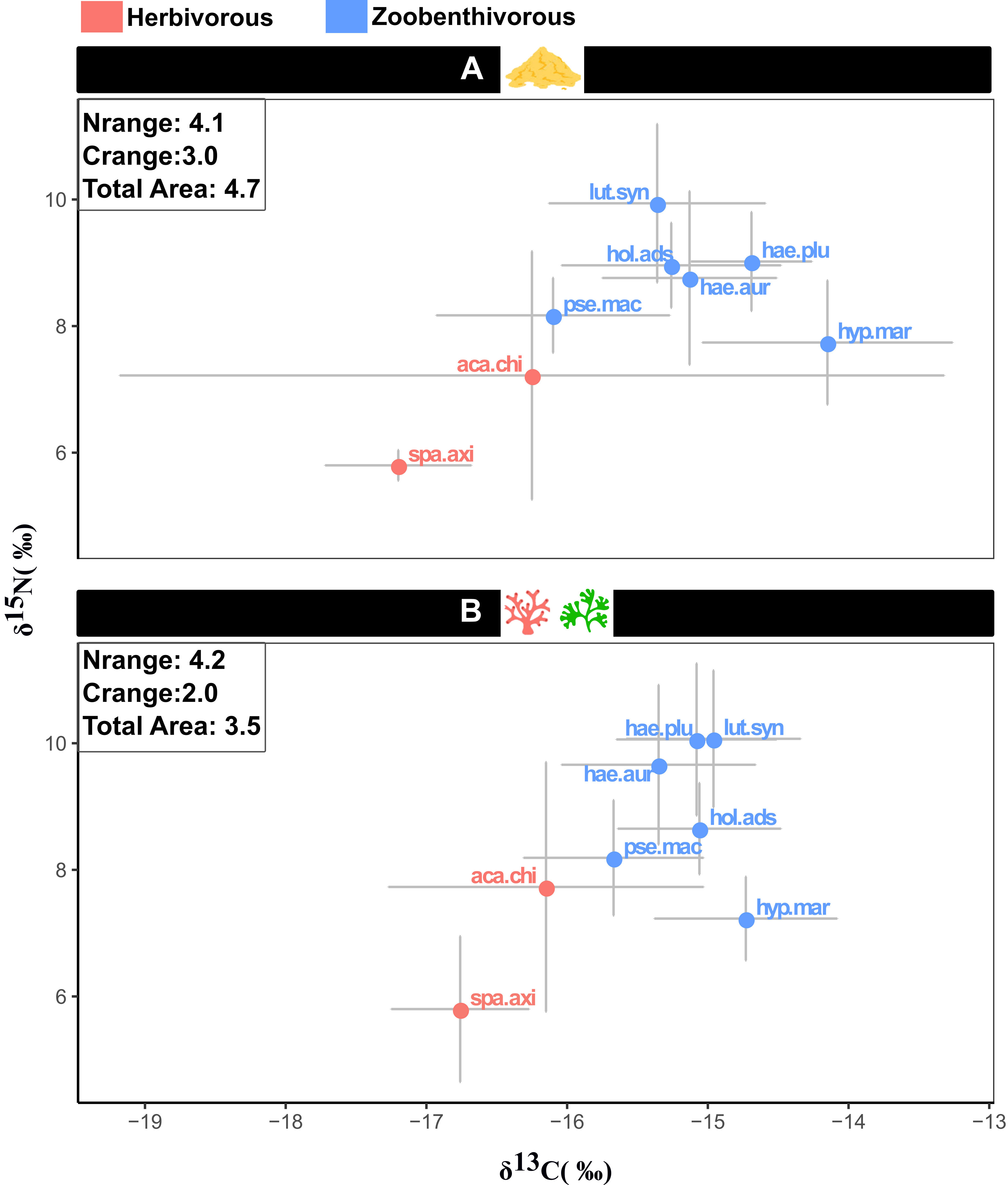
Figure 4 Biplot showing the mean and standard deviation of carbon and nitrogen isotopic compositions of the eight selected species in zones (A) (sand-dominated) and (B) (complex substrates). The fish community’s nitrogen/carbon range and total isotopic area in each zone are shown on the biplots. lut.syn, Lutjanus synagris; pse.mac, Pseudopenneus maculatus; hae.plu, Haemulon plumierii; hae.aur, Haemulon aurolineatum; hyp.mar, Hypanus marianae; hol.ads, Holocentrus adscencionis; aca.chi, Acanthurus chirurgus; spa.axi:, Sparisoma axillare.
SEAc values were highly variable between species and zones (Supplementary Table 3). In zone A, A. chirurgus exhibited the highest SEAc value (98.9 ‰2), followed by L. synagris (18.2 ‰2) and H. marianae (17.4 ‰2) while P. maculatus (9.3 ‰2), H. plumierii (6.6 ‰2), and S. axillare (2.6 ‰2) had the lowest SEAc values. In zone B, the highest SEAc values were exhibited by H. aurolineatum (15.7 ‰2), followed by A. chirurgus (12.9 ‰2) and H. plumierii (11.4 ‰2).
Acanthurus chirurgus presented a vast carbon range, especially in zone A. Moreover, in both zones, this herbivorous species had an extensive nitrogen range that overlapped with the δ15N values of zoobenthivorous fish. The other herbivore, S. axillare, showed a much smaller carbon range in both zones, lower nitrogen values, and a smaller nitrogen range. In zone A, L. synagris exhibited the highest nitrogen values allowing its isotopic niche to be little overlapped by other species. On the other hand, in zone B, its δ15N values were not significantly different from those of the haemulids. In both zones, H. marianae had higher δ13C and lower δ15N values than other zoobenthivores.Both zones exhibited differences in the structure of their competition networks (Figure 5). In zone A, there were fewer interactions (number of edges), but the intensity of the interactions was higher than in zone B. The shortest, largest, and average paths were higher in the sand-dominated zone A.
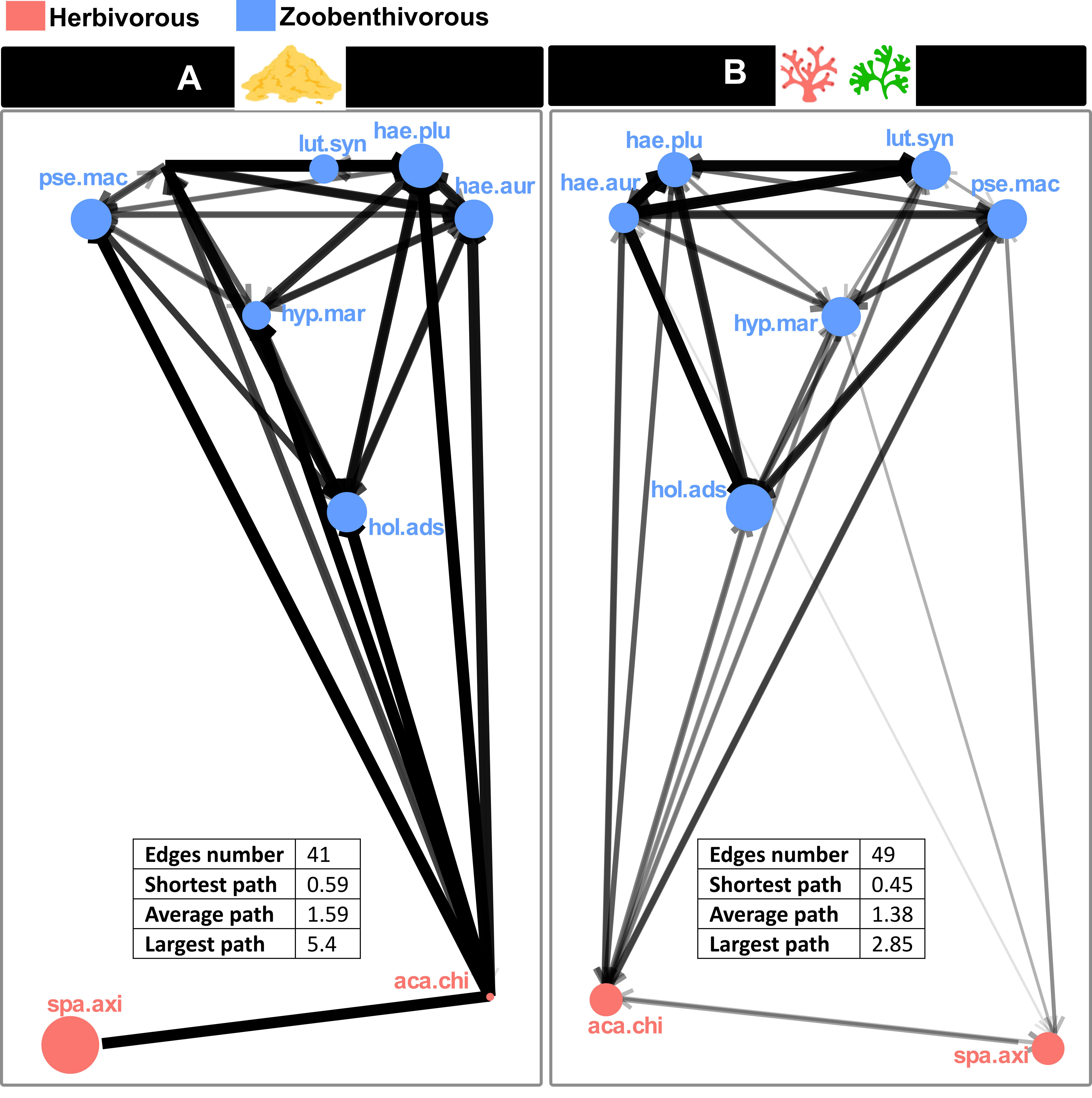
Figure 5 Competition networks in zones (A, B) The networks consist of nodes (one for each of the eight selected species) connected by arrows representing competitive interspecific interactions. The size of the nodes was set according to intraspecific competition (ITP index), and the width of the arrows was a function of the interspecific index (Pov). The number of edges, shortest, largest, and average paths for each zone’s network are indicated. lut.syn, Lutjanus synagris; pse.mac, Pseudopenneus maculatus; hae.plu, Haemulon plumierii; hae.aur, Haemulon aurolineatum; hyp.mar, Hypanus marianae; hol.ads, Holocentrus adscencionis; aca.chi, Acanthurus chirurgus; spa.axi, Sparisoma axillare.
In zone A, species exhibited a wide range of ITP values, and the intensity of competition applied by a given species was negatively correlated with its ITP (p-value< 0.05) (Supplementary Table 3). In this sand-dominated zone, the species with higher ITP was S. axillare (0.87), followed by H. plumierii (0.48), while A. chirurgus exhibited the smallest ITP value (0.03). Conversely, in zone B, no correlation between intraspecific and interspecific competition was found (p-value > 0.05), and species exhibited a smaller range of ITP values between 0.54 (H. adscencionis) and 0.18 (H. aurolineatum). In addition, H. adscenscionis, L. synagris, A. chirurgus and H. marianae had a smaller ITP in zone A. On the other hand, both haemulids and P. maculatus suffered from a higher intraspecific competition in the sandy zone than in zone B.
In addition, both zones were substantially different concerning the competitive interactions between species pairs (Figure 6). In zone B, the competition was mainly from haemulids. In zone A, pairwise interactions between all species were more substantial, so most species received more competition than in zone B. However, A. chirurgus and L. synagris were the only species suffering from less interspecific competition in the sand-dominated zone than in the complex substrates zone. Indeed, in zone A, A. chirurgus competed strongly with all species and received little competition.
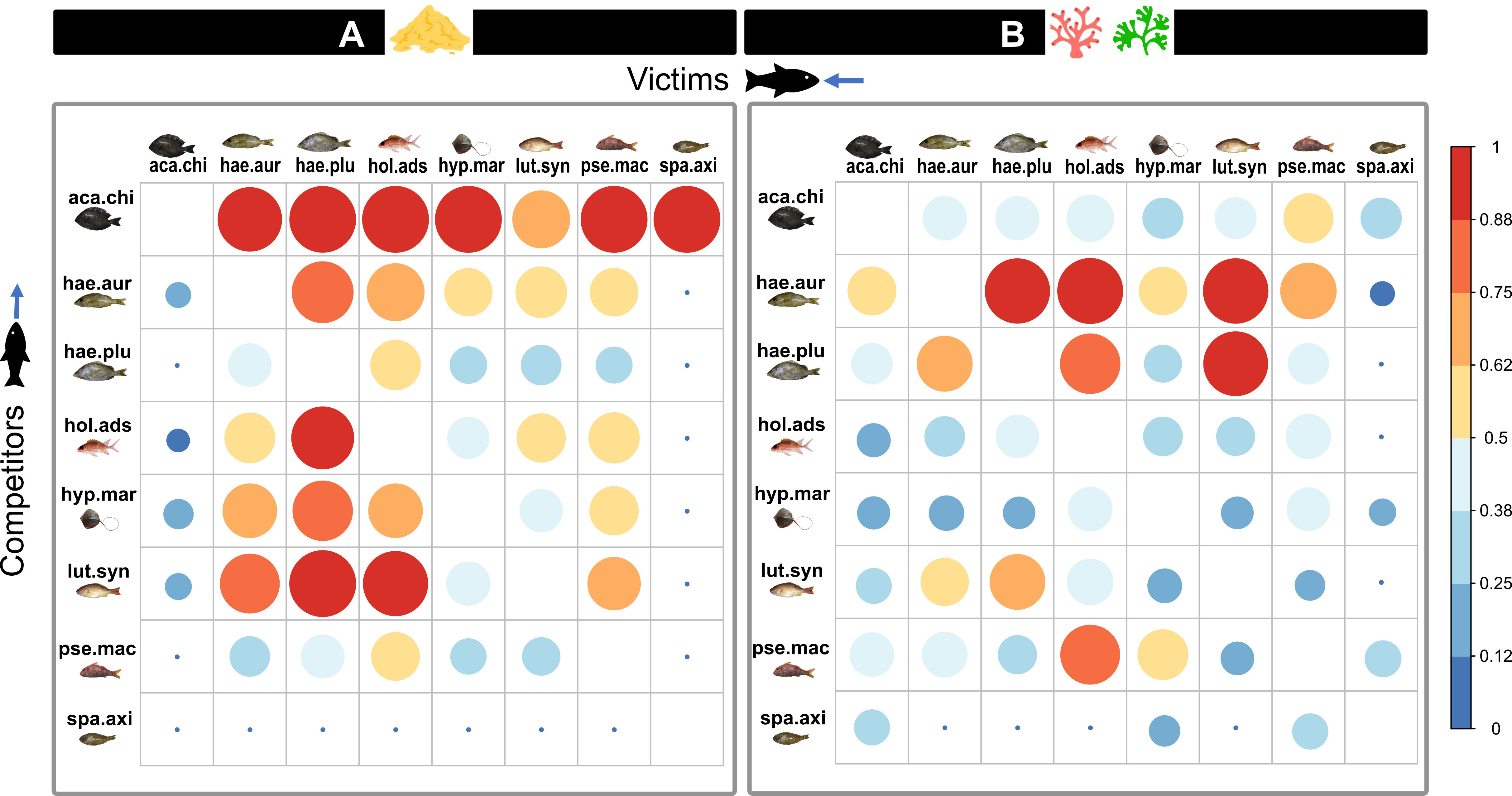
Figure 6 Matrix representing the intensity of the interspecific competition between each species pair in zones (A) (sand-dominated) and (B) (complex substrates). The colour and the size represent the proportion of the victim’s niche (column) overlapped by the competitor (row). lut.syn, Lutjanus synagris; pse.mac, Pseudopenneus maculatus; hae.plu, Haemulon plumierii; hae.aur, Haemulon aurolineatum; hyp.mar, Hypanus marianae; hol.ads, Holocentrus adscencionis; aca.chi, Acanthurus chirurgus; spa.axi, Sparisoma axillare.
Conversely, A. chirurgus applied less competition in zone B with complex substrates. In zone A, L. synagris suffers less competition with the haemulids than in zone B. Moreover, H. marianae was one of the zoobenthivore least impacted by interspecific competition in zone A.
In contrast, some species were strongly affected by the increased interspecific competition in zone A. For instance, H. plumierii was a weaker competitor in the sand-dominated zone, and its niche was heavily overlapped by most species, whereas, in zone B, it was the second strongest competitor after H. aurolineatum. Likewise, P. maculatus received stronger competition from other species than in the complex substrate zone, and in both zones, this species was a weak competitor.
4. Discussion
To our best knowledge, this is the first study combining stable isotope analysis (SIA) and social network analysis (SNA) to investigate trophic competition at the community level. Our results provide a quantitative indication of trophic competition between and among species rather than an exact equivalent since specific trophic interactions cannot be inferred from SIA (Marshall et al., 2019). Still, in our study, the isotopic niche concept is a valid proxy for trophic competition since we selected fish that coexist spatiotemporally, have overlapping diets and/or habitat use, and thus could, under resource limitation, experience competitive exclusion (Cachera et al., 2017). SNA allowed for the simultaneous study of interspecific and intraspecific trophic competition. This method enabled us to approach trophic competition as a set of asymmetric interactions that reflect species ability to efficiently use available resources, as stated by the niche variation hypothesis (NVH) (Costa-Pereira et al., 2018). Conceptualizing a system in which each species is both a victim and a competitor illustrated that received and applied competitions differ in a pairwise interspecific interaction. By applying this approach, we refine the concept of winning and losing taxa developed by Andrades et al. (2021) at a community level.
Our study provided insights into the differences in competition patterns of the demersal community in two ecological contexts representing two scenarios of resource availability. Fish migration possibility did not hamper clear differences in abundance and biodiversity between both zones with contrasted substrates (Eduardo et al., 2018). Hence, although migration between sites could blur the results, it would not likely alter the main patterns found in this study. Species selection greatly influences the overall interaction pattern, so we selected the eight most abundant species (74% of the total catches in number) to give an appropriate overview of the trophic competition in the community. Demersal fishes in the sand-dominated zone (zone A) had a more extensive range of carbon and a greater isotopic area, meaning that they used a broader range of resource use than in the zone with complex substrates (zone B). This niche expansion at the community level, in a resource-limited environment, is in line with the NVH (Bolnick et al., 2010; Cachera et al., 2017). One of the processes increasing niche width at the community level is higher variability in diet among individuals, which results in individuals with more specialized diets (Costa et al., 2015). Because SIA allows for a time-integrated measure of several weeks or months (Fry, 2006; Marshall et al., 2019), this interindividual variability seems to be maintained over time.
Our results reveal that one of the ecological advantages of the complex substrates in zone B is the lower intensity of interspecific competition. This is consistent with Darwin’s theory that a context of limited resources exacerbates competition between individuals (Darwin, 1859). In contrast, in zone A, a higher proportion of most species niches was overlapped by several other species, suggesting that they would be able to compete for the same resources. Interspecific competition is recognized as one of the drivers of natural selection in the context of limited resources (Bolnick et al., 2010). Indeed, without interspecific competition, a species exploiting a declining resource could modify its diet to consume the available resources (Price and Kirkpatrick, 2009). However, it has been observed that this process is hindered by stronger competitors, resulting in weaker species extinction (Bocedi et al., 2013). Thus, the adaptive capacity of species in a resource-limited environment is closely related to that of other competitors consuming the same resources in the region (Neves et al., 2021). In this regard, our study clearly shows that species did not respond unanimously to lower resource availability as some species demonstrated to be stronger competitors.
In zone A, the strongest competitors were the species that suffered the least from intraspecific interactions and had the greatest isotopic niche width. These results align with the NVH and the principle of natural selection, which states that species adaptation ability will determine whether or not they will succeed in competing (Darwin, 1859; Matich et al., 2017; Mendes et al., 2019). The most striking case was A. chirurgus, which had significant inter-individual variability in stable isotopic compositions, especially in the sand-dominated zone. This high inter-individual variability can be explained by the high trophic plasticity of this species. Indeed, despite being herbivorous, A. chirurgus has physiological mechanisms to digest and assimilate flesh (Delgado-Pech et al., 2020). Thus, A. chirurgus can use many resources of different trophic levels. Such remarkable capacity of this species to adapt to various ecological contexts has already been recognized (Vergés et al., 2014).
Another strategy to cope with interspecific competition in a resource-limited context is to explore resources that are less used by others (Kartzinel et al., 2015). This strategy seems to be adopted in zone A by L. synagris, the zoobenthivore species subjected to the least interspecific competition. Indeed, L. synagris has the greater piscivorous tendency among the selected species and can prey on fish or smaller organisms according to food availability (Duarte and Garcia, 1999). In the sand-dominated zone, L. synagris has the highest δ15N values among zoobenthivores, indicating that it feeds on a high trophic level compartment which is probably fish. Fish diversity is lower in the sand-dominated zone (Eduardo et al., 2018), but feeding in this compartment could be advantageous to avoid interspecific competition. In zone B, with a higher diversity of fish, the haemulids, which also can feed on fish, had high δ15N values, similar to those of L. synagris, suggesting that they feed on fish when this resource is available. In zone A, H. marianae also seems to avoid interspecific competition by using different resources, as indicated by its lower nitrogen and higher carbon stable isotopes values. This is corroborated by gut content analysis, which highlighted that the diet of H. marianae was distinct from that of other zoobenthivores fish species because polychaetes were important in its diet (Queiroz et al., 2019).
On the other hand, our study identified some species with a lower capacity to adapt in a context of limited resources. These weaker competitors suffered from the intense intraspecific competition and had a smaller isotopic niche width than in the zone with complex substrates, as predicted by the NVH and the principle of natural selection (Darwin, 1859; Matich et al., 2017; Mendes et al., 2019). This niche reduction, induced by the presence of stronger competitors, could therefore be a mechanism leading to the competitive exclusion of these species (Price and Kirkpatrick, 2009). For instance, P. maculatus showed a smaller niche in zone A, possibly due to a lack of adaptability to the stronger interspecific competition. Similarly, H. plumierii appears vulnerable to resource availability since this species, a strong competitor in the complex substrate zone had the narrowest niche among zoobenthivores in the sand-dominated zone.
This study potentially gives a perspective on the future ecological response at the community and species level of the demersal fishes (Figure 7). With habitat degradation and climate change (Hall-Spencer and Harvey, 2019), the complex substrates in zone B will probably disappear gradually, transforming this zone into an ecosystem akin to zone A. This would lead to a resource-limited context exacerbating competition between species and inducing the resource use diversification shown in our results. According to the principle of natural selection, the intensity of competition should decrease over time among sympatric populations due to species adaptive changes (Darwin, 1859). Some species like A. chirurgus and L. synagris will adapt because of their trophic plasticity or ability to use different resources. Conversely, species like P. maculatus and H. plumierii might suffer from competitive exclusion because they will invest in intraspecific competition (niche width reduction) to cope with the interspecific competition. As these species are of socio-economic importance (De Melo et al., 2020; Soares et al., 2020), fisheries could suffer from this natural selection due to the disappearance of complex substrates.
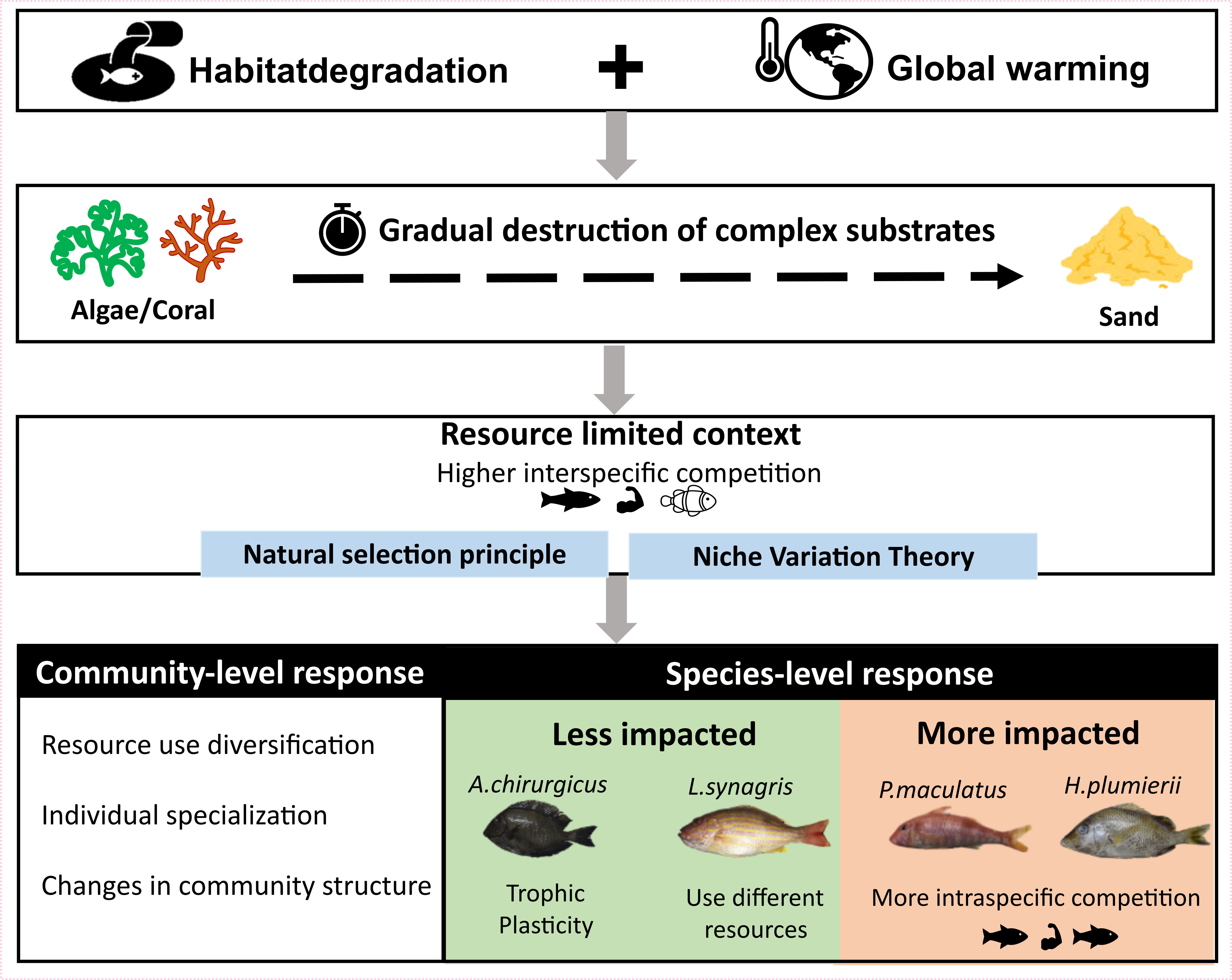
Figure 7 Possible responses of demersal fish to the combined threats of habitat degradation and global warming at community and species levels.
The ecological benefits of habitat complexity go beyond resource availability since they are involved in several ecological functions (such as reproduction, feeding and nursery grounds) that ensure the sustainability of coastal ecosystems (Kovalenko et al., 2012; Perry and Alvarez-Filip, 2019). Complex substrates act as ecosystem engineers, essential in recycling carbon by retaining sediments, thus trapping nutrients (Van Oevelen et al., 2009). In addition, reef proximity influences the ichthyofauna composition of other coastal biomes, such as mangroves (Dubuc et al., 2019). Both threats should be addressed simultaneously, as habitat degradation and climate change go hand in hand. Management policies should adopt approaches with immediate, intermediate, and long-term objectives, local, regional and global, that also benefit the human population (job creation, improved human health…) (Spencer et al., 2017). However, given that the worldwide reduction in carbon emissions needed to protect complex substrates may not happen soon enough and that climate change has already caused irreversible damage, it is necessary to consider how artificial reefs could offer some of the ecological benefits provided by natural reefs. Given that a high abundance and diversity of commercial demersal species have already been observed around wrecks and artificial reefs in our study area (Honório et al., 2010), such structures could be an appropriate mitigation measure in the context of global change.
5. Conclusion
By combining social network analysis and SIA, this study provided a new approach to investigate competition from the perspective of the isotopic niche. Furthermore, this work highlights that complex substrates offer ecological advantages such as less interspecific competition and shows that some species develop adaptations like niche expansion in the context of limited resources, as predicted by the NVH. Conversely, other species with less adaptive capacity would be more vulnerable to competitive exclusion, leading to extinction, as stated by the principle of natural selection. However, competition cannot be limited to the ability of a species to use the same resources as another species. This information helps establish potential competition, but actual competition will also depend on several characteristics of the species that influence its foraging activity, such as body shape, speed, foraging time, and body weight. Network analysis allows assigning characteristics to each compartment of the modelled system, allowing for a trait-based analysis. Several metrics enable the identification of interaction patterns based on specific traits (trophic level, trophic guildsamong) and could thus be relevant in subsequent studies.
Data availability statement
The datasets presented in this article are not readily available because; the data will be soon published in a data paper. Requests to access the datasets should be directed to Arnaud Bertrand, arnaud.bertrand@ird.fr.
Ethics statement
Ethical review and approval was not required for the animal study because It was not experiments with alive organisms we only worked with isotope data.
Author contributions
LP: Data analysis and manuscript writing. AB: Manuscript preparation and revision. FL-F: Sampling procedures and manuscript preparation. FL: Sampling procedures and manuscript preparation. LN: Manuscript preparation and revision. AL: Manuscript preparation and revision. TF: Sampling procedures, data analysis and manuscript preparation. All authors contributed to the article and approved the submitted version.
Funding
This study is a contribution to the LMI TAPIOCA, program CAPES/COFECUB (88881.142689/2017-01), EU H2020 TRIATLAS project (grant agreement 817578).We acknowledge the French oceanographic fleet for funding the at-sea survey ABRACOS 2 (https://dx.doi.org/10.17600/17004100). We thank the CNPq (Brazilian National Council for Scientific and Technological Development), which provided research grant to FL-F.
Acknowledgments
We acknowledge the officers and crew of the RV Antea for their contribution to the success of the operations. Thanks also to the BIOIMPACT (UFRPE) students for their support.
Conflict of interest
The authors declare that the research was conducted in the absence of any commercial or financial relationships that could be construed as a potential conflict of interest.
Publisher’s note
All claims expressed in this article are solely those of the authors and do not necessarily represent those of their affiliated organizations, or those of the publisher, the editors and the reviewers. Any product that may be evaluated in this article, or claim that may be made by its manufacturer, is not guaranteed or endorsed by the publisher.
Supplementary material
The Supplementary Material for this article can be found online at: https://www.frontiersin.org/articles/10.3389/fmars.2022.975091/full#supplementary-material
References
Amaral R. F.d., dos Santos J. R. (2015). Carta textural da plataforma continental rasa do estado de Rio grande do norte-setor touro (CPRM).
Andrades R., Andrade J. M., Jesus-Junior P. S., Macieira R. M., Bernardino A. F., Giarrizzo T., et al. (2019). Multiple niche-based analyses reveal the dual life of an intertidal reef predator. Mar. Ecol. Prog. Series. 624, 131–141. doi: 10.3354/meps13027
Andrades R., Joyeux J. C., Macieira R. M., Godoy B. S., Reis-Filho J. A., Jackson A. L., et al. (2021). Niche-relationships within and among intertidal reef fish species. Front. Mar. Sci. 8, 574. doi: 10.3389/fmars.2021.659579
Assunção R. V., Silva A. C., Roy A., Bourlès B., Silva C. H. S., Ternon J. F., et al. (2020). 3D characterisation of the thermohaline structure in the southwestern tropical Atlantic derived from functional data analysis of in situ profiles. Prog. Oceanogr. 187, 102399. doi: 10.1016/j.pocean.2020.102399
Bocedi G., Atkins K. E., Liao J., Henry R. C., Travis J. M. J., Hellmann J. J. (2013). Effects of local adaptation and interspecific competition on species’ responses to climate change: Interspecific interactions in a changing climate. Ann. NY Acad. Sci. 1297 (1), 83–97. doi: 10.1111/nyas.12211
Bolnick D. I., Ingram T., Stutz W. E., Snowberg L. K., Lau O. L., Paull J. S. (2010). Ecological release from interspecific competition leads to decoupled changes in population and individual niche width. Proc. R. Soc. B: Biol. Sci. 277 (1689), 1789–1797. doi: 10.1098/rspb.2010.0018
Bonin M. C., Boström-Einarsson L., Munday P. L., Jones G. P. (2015). The prevalence and importance of competition among coral reef fishes. Annu. Rev. Ecol Evolution Systematics. 46, 169–190. doi: 10.1146/annurev-ecolsys-112414-054413
Cachera M., Ernande B., Villanueva M. C., Lefebvre S. (2017). Individual diet variation in a marine fish assemblage: Optimal foraging theory, niche variation hypothesis and functional identity. J. Sea Res. 120, 60–71. doi: 10.1016/j.seares.2016.08.004
Cathcart C. N., Dunker K. J., Quinn T. P., Sepulveda A. J., von Hippel F. A., Wizik A., et al. (2019). Trophic plasticity and the invasion of a renowned piscivore: a diet synthesis of northern pike (Esox lucius) from the native and introduced ranges in Alaska, USA. Biol. Invasions. 21 (4), 1379–1392. doi: 10.1007/s10530-018-1909-7
Costa-Pereira R., Rudolf V. H., Souza F. L., Araújo M. S. (2018). Drivers of individual niche variation in coexisting species. J. Anim. Ecol. 87 (5), 1452–1464. doi: 10.1111/1365-2656.12879
Costa A., Salvidio S., Posillico M., Matteucci G., De Cinti B., Romano A. (2015). Generalisation within specialization: Inter-individual diet variation in the only specialized salamander in the world. Sci. Rep. 5 (1), 1–10. doi: 10.1038/srep13260
Cure K., Hobbs J. P. A., Langlois T. J., Fairclough D. V., Thillainath E. C., Harvey E. S. (2018). Spatiotemporal patterns of abundance and ecological requirements of a labrid’s juveniles reveal conditions for establishment success and range shift capacity. J. Exp. Mar. Biol. Ecol. 500, 34–45. doi: 10.1016/j.jembe.2017.12.006
Darwin C. (1859). The origin of species: by means of natural selection or the preservation of favored races in the struggle for life Vol. Vol. 2 (London: Modern library).
de Assis H. M. B., Lopes H., Gomes R. B., dos Salviano K. S., Valle M. M., Oliveira P. R. A., et al. (2016). Carta textural e geomorfológica da plataforma rasa de alagoas, setor maceió oeste (Brasilia, Brazil: Recife CPRM).
de Assis H. M. B., Valle M. M., Gomes R. B., dos Salviano K. S., Pereira L. B. F. (2015). Carta de caracterização dos agregados marinhos da plataforma continental rasa do estado de pernambuco.
Delgado-Pech B., Almazán-Becerril A., Peniche-Pérez J., Caballero-Vázquez J. A. (2020). Facultative scavenging feeding habits in acanthurus chirurgus (Bloch, 1787)(Acanthuriformes: Acanthuridae). Biodiversity Data J. 8, e53712. doi: 10.3897/BDJ.8.e53712
De Melo C. C., Soares A. P. C., Pelage L., Eduardo L. N., Frédou T., Lira A. S., et al. (2020). Haemulidae distribution patterns along the northeastern Brazilian continental shelf and size at first maturity of the most abundant species. Regional Stud. Mar. Sci. 35, 101226. doi: 10.1016/j.rsma.2020.101226
Dominguez Almela V., South J., Britton J. R. (2021). Predicting the competitive interactions and trophic niche consequences of a globally invasive fish with threatened native species. J. Anim. Ecol. 90, 2651–2662. doi: 10.1111/1365-2656.13571
Donelson J., Munday P., McCormick M., Pankhurst N., Pankhurst P. (2010). Effects of elevated water temperature and food availability on the reproductive performance of a coral reef fish. Mar. Ecol. Prog. Ser. 401, 233–243. doi: 10.3354/meps08366
Dossa A. N., Silva A. C., Chaigneau A., Eldin G., Araujo M., Bertrand A. (2021). Near-surface western boundary circulation off northeast Brazil. Prog. Oceanogr. 190, 102475. doi: 10.1016/j.pocean.2020.102475
Duarte L. O., Garcia C. B. (1999). Diet of the lane snapper, lutjanus synagris (Lutjanidae), in the gulf of salamanca, Colombia. Caribbean J. Sci. 35, 54–63.
Dubuc A., Waltham N. J., Baker R., Marchand C., Sheaves M. (2019). Patterns of fish utilisation in a tropical indo-pacific mangrove-coral seascape, new Caledonia. PloS One 14 (4), e0207168. doi: 10.1371/journal.pone.0207168
Eduardo L. N., Frédou T., Lira A. S., Ferreira B. P., Bertrand A., Ménard F., et al. (2018). Identifying key habitat and spatial patterns of fish biodiversity in the tropical Brazilian continental shelf. Continental Shelf Res. 166, 108–118. doi: 10.1016/j.csr.2018.07.002
Ferreira V., Loc’h L., Ménard F., Frédou T., Frédou F. L. (2019). Composition of the fish fauna in a tropical estuary: the ecological guild approach. Scientia Marina. 83 (2), 133–142. doi: 10.3989/scimar.04855.25A
França F. M., Benkwitt C. E., Peralta G., Robinson J. P., Graham N. A., Tylianakis J. M., et al. (2020). Climatic and local stressor interactions threaten tropical forests and coral reefs. Philos. Trans. R. Soc. B. 375 (1794), 20190116. doi: 10.1098/rstb.2019.0116
Frelat R., Kortsch S., Kröncke I., Neumann H., Nordström M. C., Olivier P. E., et al. (2022). Food web structure and community composition: A comparison across space and time in the north Sea. Ecography 2022 (2). doi: 10.1111/ecog.05945
Garcia A. M., Hoeinghaus D. J., Vieira J. P., Winemiller K. O. (2007). Isotopic variation of fishes in freshwater and estuarine zones of a large subtropical coastal lagoon. Estuarine Coast. Shelf Sci. 73 (3–4), 399–408. doi: 10.1016/j.ecss.2007.02.003
Giachini Tosetto E. G., Bertrand A., Neumann-Leitão S., Nogueira Júnior M. (2022). The Amazon river plume, a barrier to animal dispersal in the Western tropical Atlantic. Sci. Rep. 12 (1), 1–12. doi: 10.1038/s41598-021-04165-z
Gobler C. J., Merlo L. R., Morrell B. K., Griffith A. W. (2018). Temperature, acidification, and food supply interact to negatively affect the growth and survival of the forage fish, menidia beryllina (Inland silverside), and cyprinodon variegatus (Sheepshead minnow). Front. Mar. Sci. 5, 86. doi: 10.3389/fmars.2018.00086
González-Rivero M., Harborne A. R., Herrera-Reveles A., Bozec Y. M., Rogers A., Friedman A., et al. (2017). Linking fishes to multiple metrics of coral reef structural complexity using three-dimensional technology. Sci. Rep. 7 (1), 13965. doi: 10.1038/s41598-017-14272-5
Hall-Spencer J. M., Harvey B. P. (2019). Ocean acidification impacts on coastal ecosystem services due to habitat degradation. Emerging Topics Life Sci. 3 (2), 197–206. doi: 10.1042/ETLS20180117
Honório P. P. F., Ramos R. T. C., Feitoza B. M. (2010). Composition and structure of reef fish communities in paraíba state, north-eastern Brazil. J. Fish Biol. 77 (4), 907–926. doi: 10.1111/j.1095-8649.2010.02728.x
Huey R. B., Kingsolver J. G. (2019). Climate warming, resource availability, and the metabolic meltdown of ectotherms. Am. Naturalist. 194 (6), E140–E150. doi: 10.1086/705679
Jackson A. L., Inger R., Parnell A. C., Bearhop S. (2011). Comparing isotopic niche widths among and within communities: SIBER–stable isotope Bayesian ellipses in r. J. Anim. Ecol. 80 (3), 595–602. doi: 10.1111/j.1365-2656.2011.01806.x
Kartzinel T. R., Chen P. A., Coverdale T. C., Erickson D. L., Kress W. J., Kuzmina M. L., et al. (2015). DNA Metabarcoding illuminates dietary niche partitioning by African large herbivores. Proc. Natl. Acad. Sci. 112 (26), 8019–8024. doi: 10.1073/pnas.1503283112
Kéfi S., Berlow E. L., Wieters E. A., Joppa L. N., Wood S. A., Brose U., et al. (2015). Network structure beyond food webs: mapping non-trophic and trophic interactions on Chilean rocky shores. Ecol. 96 (1), 291–303. doi: 10.1890/13-1424.1
Kovalenko K. E., Thomaz S. M., Warfe D. M. (2012). Habitat complexity: approaches and future directions. Hydrobiologia. 685 (1), 1–17. doi: 10.1007/s10750-011-0974-z
Kuczynski L., Legendre P., Grenouillet G. (2018). Concomitant impacts of climate change, fragmentation and non-native species have led to reorganization of fish communities since the 1980s. Global Ecol. Biogeogr. 27 (2), 213–222. doi: 10.1111/geb.12690
Marshall H. H., Inger R., Jackson A. L., McDonald R. A., Thompson F. J., Cant M. A. (2019). Stable isotopes are quantitative indicators of trophic niche. Ecol. letters. 22 (11), 1990–1992. doi: 10.1111/ele.13374
Matich P., Ault J. S., Boucek R. E., Bryan D. R., Gastrich K. R., Harvey C. L., et al. (2017). Ecological niche partitioning within a large predator guild in a nutrient-limited estuary. Limnol Oceanogr. 62 (3), 934–953. doi: 10.1002/lno.10477
Mendes A., Fernandes I. M., Penha J., Mateus L. (2019). Intra and not interspecific competition drives intra-populational variation in resource use by a neotropical fish species. Environ. Biol. Fishes. 102 (8), 1097–1105. doi: 10.1007/s10641-019-00892-5
Neves M. P., Costa-Pereira R., Delariva R. L., Fialho C. B. (2021). Seasonality and interspecific competition shape individual niche variation in co-occurring tetra fish in Neotropical streams. Biotropica. 53 (1), 329–338. doi: 10.1111/btp.12879
Ogloff W. R., Yurkowski D. J., Davoren G. K., Ferguson S. H. (2019). Diet and isotopic niche overlap elucidate competition potential between seasonally sympatric phocids in the Canadian Arctic. Mar. Biol. 166 (8), 1–12. doi: 10.1007/s00227-019-3549-6
Olivier P., Frelat R., Bonsdorff E., Kortsch S., Kröncke I., Möllmann C., et al. (2019). Exploring the temporal variability of a food web using long-term biomonitoring data. Ecography. 42 (12), 2107–2121. doi: 10.1111/ecog.04461
Opsahl T., Opsahl M. T. (2015). R package “tnet”. (version 3.0.16) [Computer software]. Available at: http://toreopsahl.com/tnet/
Opsahl T., Agneessens F., Skvoretz J. (2010). Node centrality in weighted networks: Generalizing degree and shortest paths. Soc. networks. 32 (3), 245–251. doi: 10.1016/j.socnet.2010.03.006
Pedersen T. L., Pedersen M. T. L., LazyData T., Rcpp I., Rcpp L. (2017). Package ‘ggraph’. Retrieved January. 1, 2018.
Pelage L., Bertrand A., Ferreira B. P., Lucena-Frédou F., Justino A. K., Frédou T. (2021). Balanced harvest as a potential management strategy for tropical small-scale fisheries. ICES J. Mar. Sci. 78, 2547–2561. doi: 10.1093/icesjms/fsab136
Perry C. T., Alvarez-Filip L. (2019). Changing geo-ecological functions of coral reefs in the anthropocene. Funct. Ecol. 33 (6), 976–988. doi: 10.1111/1365-2435.13247
Post D. M. (2002). Using stable isotopes to estimate trophic position: models, methods, and assumptions. Ecology. 83 (3), 703–718. doi: 10.1890/0012-9658(2002)083[0703:USITET]2.0.CO;2
Price T. D., Kirkpatrick M. (2009). Evolutionarily stable range limits set by interspecific competition. Proc. R. Soc. B: Biol. Sci. 276 (1661), 1429–1434. doi: 10.1098/rspb.2008.1199
Queiroz A. P., Araújo M. L., Lessa R. (2019). Dietary composition and trophic level of hypanus marianae (Myliobatiformes: Dasyatidae), captured off pernambuco coast, Brazil. Latin Am. J. Aquat. Res. 47 (5), 808–817. doi: 10.3856/vol47-issue5-fulltext-10
Rakshit N., Banerjee A., Mukherjee J., Chakrabarty M., Borrett S. R., Ray S. (2017). Comparative study of food webs from two different time periods of hooghly matla estuarine system, India through network analysis. Ecol. Modelling. 356, 25–37. doi: 10.1016/j.ecolmodel.2017.04.003
Runghen R., Poulin R., Monlleó-Borrull C., Llopis-Belenguer C. (2021). Network analysis: ten years shining light on host–parasite interactions. Trends Parasitology. 37, 445–455. doi: 10.1016/j.pt.2021.01.005
Soares A., Lira A. S., Gonzalez J. G., Eduardo L. N., Lucena-Frédou F., Le Loc’h F., et al. (2020). Feeding habits and population aspects of the spotted goatfish, pseudupeneus maculatus (Perciformes: Mullidae), on the continental shelf of northeast Brazil. Scientia Marina. 84 (2), 119–131. doi: 10.3989/scimar.04958.24A
Sosa S., Sueur C., Puga-Gonzalez I. (2020). Network measures in animal social network analysis: Their strengths, limits, interpretations and uses. Methods Ecol. Evolution. 12, 10–21. doi: 10.1111/2041-210X.13366
Spencer B., Lawler J., Lowe C., Thompson L., Hinckley T., Kim S. H., et al. (2017). Case studies in co-benefits approaches to climate change mitigation and adaptation. J. Environ. Plann. Management. 60 (4), 647–667. doi: 10.1080/09640568.2016.1168287
Van Oevelen D., Duineveld G., Lavaleye M., Mienis F., Soetaert K., Heip C. H. (2009). The cold-water coral community as hotspot of carbon cycling on continental margins: A food-web analysis from rockall bank (northeast Atlantic). Limnol Oceanogr. 54 (6), 1829–1844. doi: 10.4319/lo.2009.54.6.1829
Van Valen L. (1965). Morphological variation and width of ecological niche. Am. Naturalist. 99 (908), 377–390. doi: 10.1086/282379
Vaske T., Teixeira Lessa R. P. (2019). Occurrence of brephoepipelagic fishes in the stomach contents of large pelagic predators in the southwestern equatorial Atlantic ocean. Cahiers Biologie Mar. 60, 235–241.
Vergés A., Steinberg P. D., Hay M. E., Poore A. G., Campbell A. H., Ballesteros E., et al. (2014). The tropicalization of temperate marine ecosystems: climate-mediated changes in herbivory and community phase shifts. Proc. R. Soc. B: Biol. Sci. 281 (1789), 20140846. doi: 10.1098/rspb.2014.0846
Vital H., da Silveira I. M., Amaro V. E. (2005). Carta sedimentólogica da plataforma continental brasileira: Area guamaré a Macau (NE brasil), utilizando integração de dados geológicos e sensoriamento remoto. Rev. Bras. Geofísica. 23 (3), 233–241.
Xia Y., Li Y., Zhu S., Li J., Li S., Li X. (2020). Individual dietary specialization reduces intraspecific competition, rather than feeding activity, in black amur bream (Megalobrama terminalis). Sci. Rep. 10 (1), 1–11. doi: 10.1038/s41598-020-74997-8
Keywords: social network analysis (SNA), isotopic niche, trophic competition, climate change, tropical ecosystems
Citation: Pelage L, Lucena-Frédou F, Eduardo LN, Le Loc’h F, Bertrand A, Lira AS and Frédou T (2022) Competing with each other: Fish isotopic niche in two resource availability contexts. Front. Mar. Sci. 9:975091. doi: 10.3389/fmars.2022.975091
Received: 21 June 2022; Accepted: 18 November 2022;
Published: 09 December 2022.
Edited by:
Lynne Jane Shannon, University of Cape Town, South AfricaReviewed by:
Stuart James Kininmonth, The University of Queensland, AustraliaVitor H. Paiva, University of Coimbra, Portugal
Copyright © 2022 Pelage, Lucena-Frédou, Eduardo, Le Loc’h, Bertrand, Lira and Frédou. This is an open-access article distributed under the terms of the Creative Commons Attribution License (CC BY). The use, distribution or reproduction in other forums is permitted, provided the original author(s) and the copyright owner(s) are credited and that the original publication in this journal is cited, in accordance with accepted academic practice. No use, distribution or reproduction is permitted which does not comply with these terms.
*Correspondence: Latifa Pelage, latifa.pelage3@gmail.com