- College of Fisheries, Key Laboratory of Freshwater Fish Reproduction and Development, Ministry of Education, and Research Center of Fishery Resources and Environment, Southwest University, Chongqing, China
Hyriopsis cumingii has attracted attention because of its pearl production performance and water purification capacity. Realizing sustainable industrialized culture of H. cumingii or applying it to bivalve biomanipulation for controlling water eutrophication needs urgent studies about the selection of suitable algae and the effects of different microalgae on mussel physiology. To contrast molecular and biochemical effects of high-quality microalgal diets (Chlorella vulgaris, Navicula pelliculosa, and Cyclotella sp.) with toxic Microcystis aeruginosa on metabolism and immune physiology of H. cumingii, levels of related enzymes and genes were analyzed during the 28-day exposure period. Results showed that the Cyclotella sp. diet could significantly (p < 0.05) maintain higher levels of metabolic enzymes (glutamic oxaloacetate transaminase (GOT), glutamic pyruvate transaminase (GPT), pyruvate kinase (PK), and hexokinase (HK)) and genes (CPT1 and LDLR). C. vulgaris and N. pelliculosa treatments significantly (p < 0.05) reduced activities of these metabolic parameters. The M. aeruginosa treatment significantly (p < 0.05) enhanced levels of immune enzymes (alkaline phosphatase (AKP), superoxide dismutase (SOD), and catalase (CAT)) and genes (HcIL-17 and IAP) on day 1 or 7, and there was a significant (p < 0.05) reduction on day 28. Results suggested that Cyclotella sp. was the suitable algae for H. cumingii, followed by C. vulgaris and N. pelliculosa, and toxic algae caused metabolic disorders, immune injury, and poor physiological status. The study has practical significance in the sustainable cultivation of H. cumingii and provides a theoretical basis for bivalve biomanipulation in eutrophic water.
Introduction
Global freshwater pearl production accounting for more than 75% is achieved by the triangle sail mussel Hyriopsis cumingii from China (Tang et al., 2015). On the one hand, water pollution, disease outbreaks, and benthic ecological destruction often occur in the traditional cultivation of H. cumingii because of the use of massive amounts of organic and chemical fertilizers (Hu et al., 2015). The development and exploitation of a new sustainable cultivation mode such as industrialized culture are urgent, and the selection of microalgae with high quality and nutritional value is the key. On the other hand, freshwater bivalves have been considered a biomanipulation tool to control water eutrophication based on ecological functions such as biofiltration, biodeposition, and bioturbation (Gulati et al., 2008; Gu et al., 2020). In small-scale bivalve biomanipulation experiments, H. cumingii can effectively reduce concentrations of total suspended solids and the number of cyanobacteria, increase transparency, and change the community structure of phytoplankton in water (He et al., 2014; Wang et al., 2018). Toxic algae Microcystis aeruginosa is the most common species from cyanobacterial blooms, which causes adverse effects on the physiological responses of H. cumingii (Hu et al., 2016). Therefore, urgent studies about contrasting molecular and biochemical effects of high-quality microalgal diets with the toxic M. aeruginosa on the metabolism and immune physiology of H. cumingii are needed.
As the main food for bivalves, algae is the natural nutritional base and primary source of nutrients. Diatoms such as Navicula sp., Cyclotella sp., and green algae Chlorella vulgaris, which are rich in nutrients including carbohydrate, lipid, and protein, have been applied to bivalve culture. Previous studies have shown that different algae affect the growth, pearl production, fatty acid composition, and energy metabolism of H. cumingii (Chen et al., 2021). Mussels have sensitive physiological responses such as changes in enzyme and gene levels to different algal species or concentrations (Blanco-Rayon et al., 2019; Sun et al., 2021). Metabolic enzymes including glutamic oxaloacetate transaminase (GOT), glutamic pyruvate transaminase (GPT), pyruvate kinase (PK), and hexokinase (HK) have a key role in protein, carbohydrate, and glucose metabolism (Xia et al., 2015). Low-density lipoprotein receptor (LDLR) and carnitine palmitoyltransferase 1 (CPT1) have a crucial function in lipid uptake, transport, and catabolic metabolism (Liu et al., 2018). Hydrolases alkaline phosphatase (AKP) and antioxidase superoxide dismutase (SOD), and catalase (CAT), as non-specific humoral immune factors, participate in immune responses (Hu et al., 2015). Interleukin-17 (HcIL-17) and inhibitor of apoptosis protein (IAP) have crucial functions in the inflammatory reaction, immune responses, and defense of bivalves (Rosani et al., 2015; Zhu et al., 2021). Bivalve gill is the tissue that first comes into contact with foreign particles, and changes in the hemolymph can reflect the process of oxidative damage and the metabolism of pollutants (Pan et al., 2006). Functional features of the bivalve liver include the metabolic reserve site and the helper of transferring these reserves to other organs (Albentosa et al., 2012).
Therefore, detecting the above molecular and biochemical parameters could reflect the health and physiology status of mussels when exposed to three microalgal diets and the toxic M. aeruginosa. This study provides new considerations for technical issues such as the sustainable cultivation of bivalves and the implementation of bivalve biomanipulation in eutrophic water.
Materials and methods
Experimental mussels and microalgal cultivation
One-year-old and unisex triangle sail mussels H. cumingii (8.26 ± 0.46 cm in shell length and 48.54 ± 4.02 g in wet weight with shell) were obtained from the laboratory culture base (Dahonghu lake, Changshou, Chongqing, China). Healthy mussels after removing shell surface attachments were placed in 20-L aquarium tanks (18 mussels in each tank, 12 tanks in total) with aeration indoors. Mussels were subjected to starvation treatment for 2 days at 23.0°C ± 0.2°C, pH 7.8 ± 0.3, and DO 8.0 ± 0.2 mg L−1. Full water was changed daily.
Microalgae was bought from the Freshwater Algae Culture Collection of the Institute of Hydrobiology, Chinese Academy of Sciences, Wuhan, China. C. vulgaris (clone FACHB-8) and M. aeruginosa (clone FACHB-315) used BG11 medium; Navicula pelliculosa (clone FACHB-2262) and Cyclotella sp. (clone FACHB-1638) used CSI medium. Microalgae was cultured in 0.45-μm, filtered, natural freshwater, at 25°C with a 12-h light:12-h dark cycle. Algal cells had been harvested during the late exponential growth phase, and algal cell densities were counted using a hemocytometer under a light microscope.
Experimental design
To select the optimal microalgal diet and contrast the physiological effects of different microalgae on the metabolism and immunity of H. cumingii, a single-factor experiment had been performed involving four microalgal treatments, with three replicates (i.e., three tanks) per treatment (the culture conditions were the same as above performed during the acclimatization period). Green algae C. vulgaris commonly used in production, diatoms N. pelliculosa and Cyclotella sp. with high nutritional value, and toxic cyanobacteria M. aeruginosa forming algal blooms had been adopted in this study. According to previous research data from our laboratory (in the treatment of biomass at 12.5 mg L−1, mussels of this size produced more real feces and a small amount of pseudo-feces after 1 day) (Sun et al., 2021), each treatment was reared with the suitable microalgae concentration (C. vulgaris, 6.2 × 105 cells ml−1; N. pelliculosa, 4.0 × 104 cells ml−1; Cyclotella sp., 3.1 × 104 cells ml−1; M. aeruginosa, 4.5 × 106 cells ml−1; ca. 12.5 mg L−1). Full water renewal and microalgae additions were conducted once a day at 9:00 a.m. Three mussels from each tank were collected for molecular and biochemical measurements on days 1, 7, 14, 21, and 28. Growth data were measured at the 28th day including shell length and wet weight of mussels (Table S2 in the Supplementary Material).
Sample collection
Gill and liver tissues of mussels had been collected on ice, after the collection of hemolymph from the adductor muscle sinus. Samples were immediately frozen in liquid nitrogen, transported, and stored at −80°C for further enzyme activities and gene expression analysis. Tissues were later defrosted on ice and homogenized at a ratio of 1:10 (w/v) of saline solution in an ice bath, using a hand-held glass homogenizer. The homogenized sample was centrifuged at 8,000 × g for 10 min at 4°C. The supernatant was used for the determination of parameters.
Determination of molecular and biochemical parameters
Metabolic and immune enzyme activities were respectively evaluated by commercial test kits (Nanjing Jiancheng Bioengineering Institute, Jiangsu, China) (Yang et al., 2021) following the manufacturer’s protocols.
Quantitative PCR was used to analyze relative expression levels of metabolic genes such as CPT1 and LDLR, and immune genes HcIL-17 and IAP in the liver of mussels. The qPCR was performed in a CFX96 Real-Time PCR Detection System (Bio-Rad, Hercules, CA, USA) using Hieff UNICON® Universal Blue qPCR SYBR Green Master Mix (Yeasen, Shanghai, China) to detect double-stranded cDNA synthesis. Reactions were performed in a volume of 20 μl containing 10 μl of qPCR SYBR Green Master Mix, 0.4 μl of 10 mM of each forward and reverse primer (Table S1 in the Supplementary Material), and 2 μl of cDNA and 7.2 μl of RNAse-free water. Each qRT-PCR assay was performed in triplicate for analysis, and dissociation curves for each amplicon were carefully examined to confirm the specificity of the primer pairs used. The mean PCR efficiency per pair of primers, the threshold cycle (Ct) value per sample, and the fluorescence threshold set to determine the Ct value were performed using the LinRegPCR software (Ruijter et al., 2009). Relative expression levels for each sample were obtained using the comparative Ct method. The Ct value obtained after each reaction was normalized to the Ct value of EF1a, whose expression was consistent across all conditions. Relative expression levels were obtained by calibrating ΔΔCt values.
Statistical analysis
Statistical analysis was performed using SPSS 26 software (SPSS Inc., Chicago, IL, USA). Values of tested parameters were means of three replicates expressed as mean ± SD. One-way ANOVA was used to evaluate the effects of four algae on mussels, followed by a Student’s t-test to determine significant variation among different groups. The significance was set at p < 0.05. Principal component analysis (PCA) was visualized using XLSTAT®2020 (Addinsoft Inc., New York, NY, USA).
Results
Effects of four microalgae on metabolic-related enzymes and genes in Hyriopsis cumingii
Higher GOT activities were observed from the Cyclotella sp. treatment (7.50 ± 0.23 U g protein−1) on days 7, 14, 21, and 28 (Figure 1A). On day 28, the GOT activity of the M. aeruginosa treatment (3.56 ± 0.28 U g protein−1) was the lowest. GOT activities of C. vulgaris and M. aeruginosa treatments gradually reduced along with time during the experimental period. GPT activities (6.41 ± 0.17 U g protein−1) of the Cyclotella sp. treatment were significantly (p < 0.05) higher than other treatments on days 14, 21, and 28 (Figure 1B). The lowest GPT activity was observed from the M. aeruginosa treatment on day 28 (3.09 ± 0.05 U g protein−1). There was a significant reduction in GPT activities of C. vulgaris, N. pelliculosa, and M. aeruginosa treatments over time.
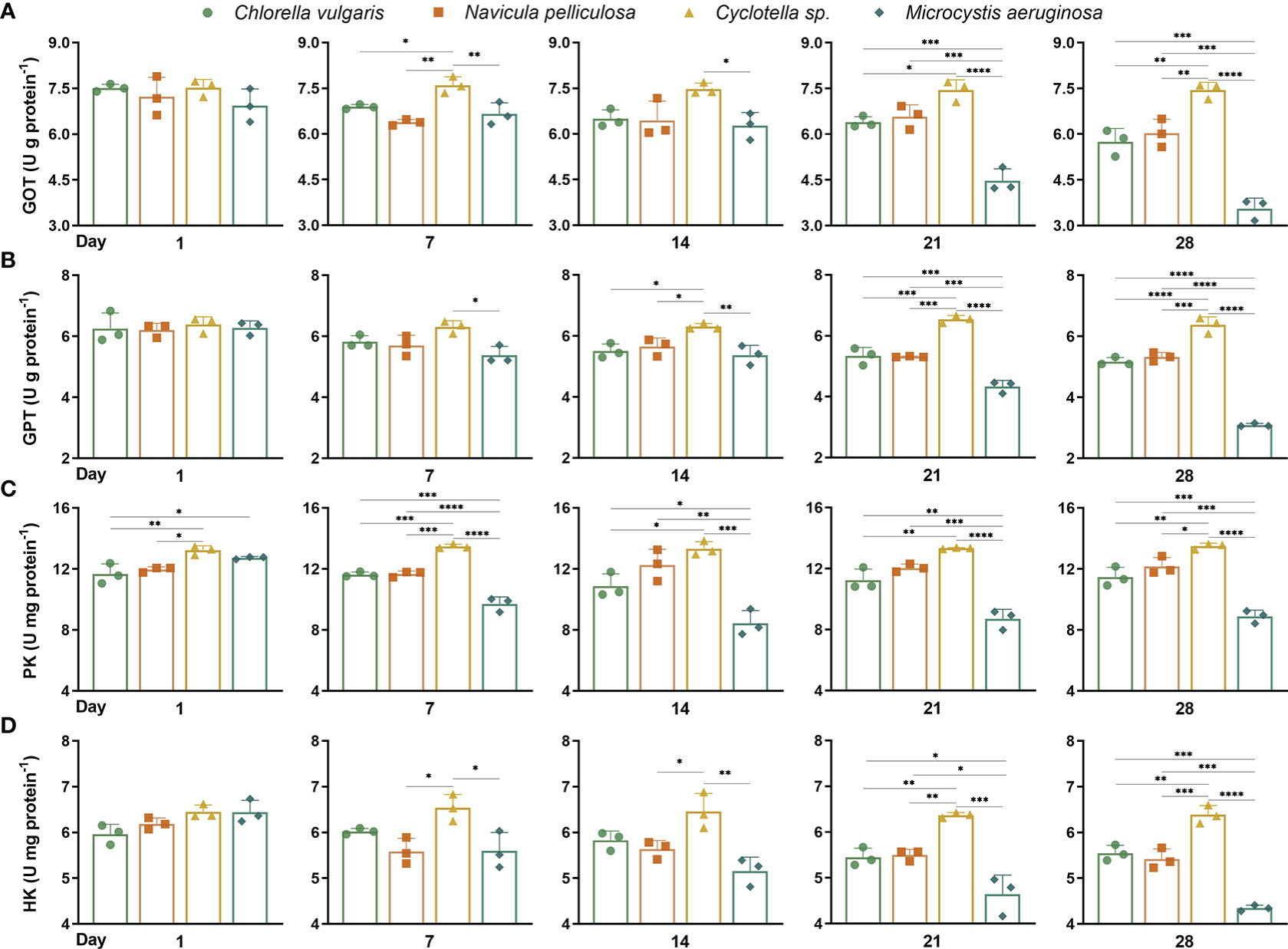
Figure 1 Activities of metabolic enzymes in liver of Hyriopsis cumingii. Mussels exposed to four different microalgae treatments on days 1, 7, 14, 21, and 28. (A) GOT, (B) GPT, (C) PK, and (D) HK. The asterisk (*) indicates significant differences between four microalgae treatments at each sampling time (Tukey test, p < 0.05). Values are expressed as mean ± SD (n = 3). GOT, glutamic oxaloacetate transaminase; GPT, glutamic pyruvate transaminase; PK, pyruvate kinase; HK, hexokinase. The symbols *, ** , *** and **** represent p < 0.05, p < 0.01, p < 0.001 and p < 0.0001 respectively.
Higher PK activities were observed from the Cyclotella sp. treatment (13.37 ± 0.24 U mg protein−1) during the experimental period (Figure 1C). The PK activity of mussels in the M. aeruginosa treatment (8.92 ± 0.69 U mg protein−1) significantly (p < 0.05) decreased after day 1. HK activities of mussels in the Cyclotella sp. treatment (13.37 ± 0.24 U mg protein−1) were significantly (p < 0.05) higher than in other treatments on days 21 and 28 (Figure 1D). The lowest HK activity was observed from the M. aeruginosa treatment on day 28 (4.35 ± 0.05 U mg protein−1).
As shown in Figure 2, transcript levels of metabolism-related genes such as CPT1 and LDLR in the liver of mussels were significantly (p < 0.05) affected by four algae treatments on days 1, 14, and 28. Consistent with the results of metabolic enzyme activities, there were no significant (p < 0.05) differences in relative expression levels of CPT1 and LDLR among all treatments on day 1 (Figures 2A, B). Relative expression levels on days 14 and 28 could be arranged in the following order: Cyclotella sp. treatment > C. vulgaris and N. pelliculosa treatments > M. aeruginosa treatment. In the M. aeruginosa treatment, CPT1 expression levels reduced along with time. Relative expression levels of LDLR in C. vulgaris, N. pelliculosa, and M. aeruginosa treatments on day 28 significantly (p < 0.05) decreased as compared with expression levels on day 1.
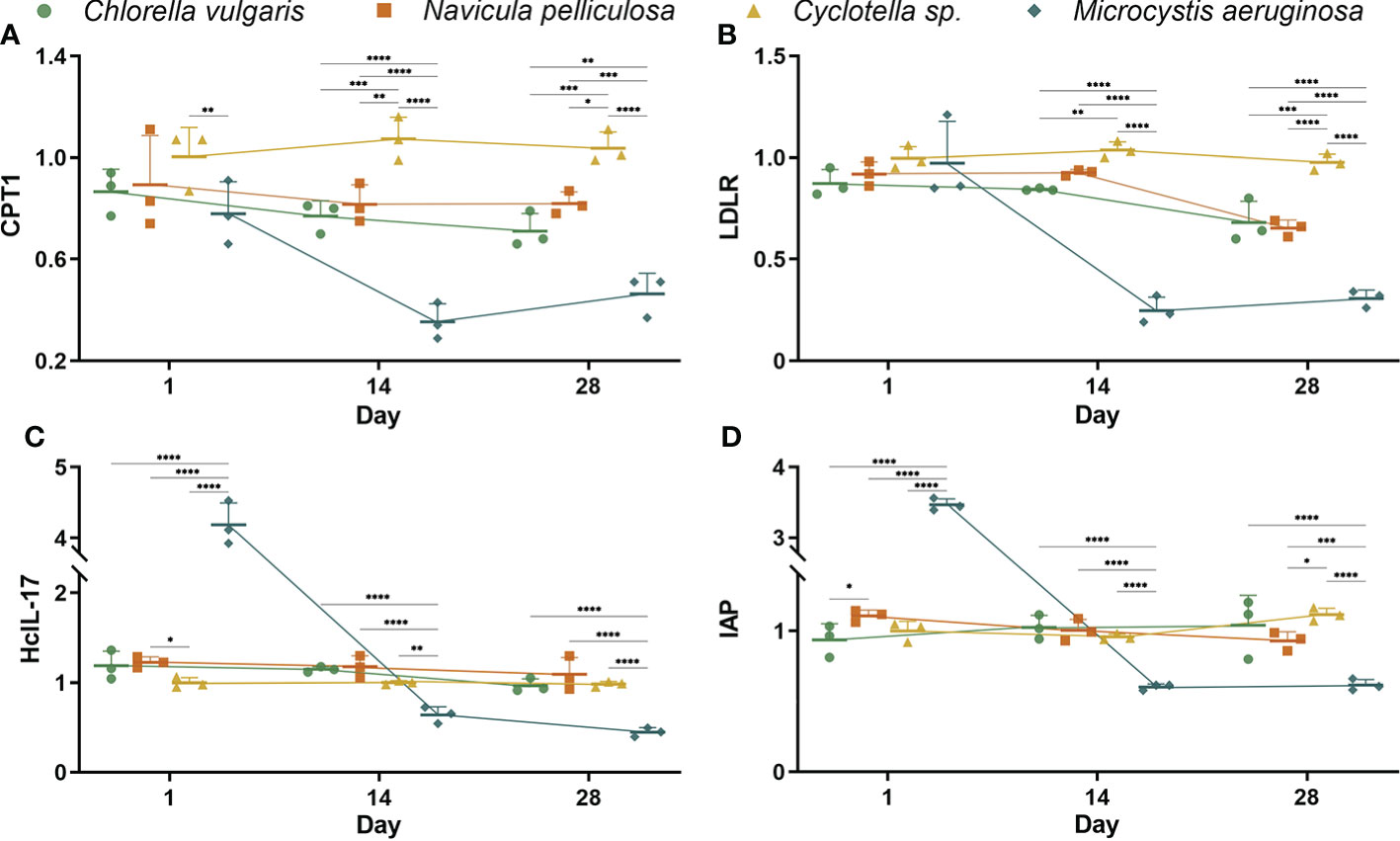
Figure 2 Relative expression levels of metabolic and immune-related genes in liver of Hyriopsis cumingii. Mussels exposed to four different microalgae treatments on days 1, 14, and 28. Metabolic genes: (A) CPT1 and (B) LDLR. Immune genes: (C) HcIL-17 and (D) IAP. The asterisk (*) indicates significant differences between four microalgae treatments at each sampling time (Tukey test, p < 0.05). Values are expressed as mean ± SD (n = 3).
Effects of four microalgae on immune-related enzymes and genes in Hyriopsis cumingii
AKP activities in the serum of the C. vulgaris treatment on days 7, 14, and 28 were significantly (p < 0.05) lower than those of the Cyclotella sp. treatment (Figure 3A). AKP activities in the serum of the N. pelliculosa treatment decreased on day 7 and then recovered. The AKP activity in the serum of the M. aeruginosa treatment on day 7 (2.46 ± 0.11 U ml−1) was significantly (p < 0.05) higher than in the other three treatments. On day 28, the AKP activity in the serum of the Cyclotella sp. treatment (2.05 ± 0.09 U ml−1) was higher than that of C. vulgaris (1.64 ± 0.19 U ml−1) and M. aeruginosa (1.60 ± 0.03 U ml−1) treatments. AKP activities in the gill of C. vulgaris, N. pelliculosa, and Cyclotella sp. treatments were significantly (p < 0.05) higher than the M. aeruginosa treatment on days 14, 21, and 28 (Figure 3B).
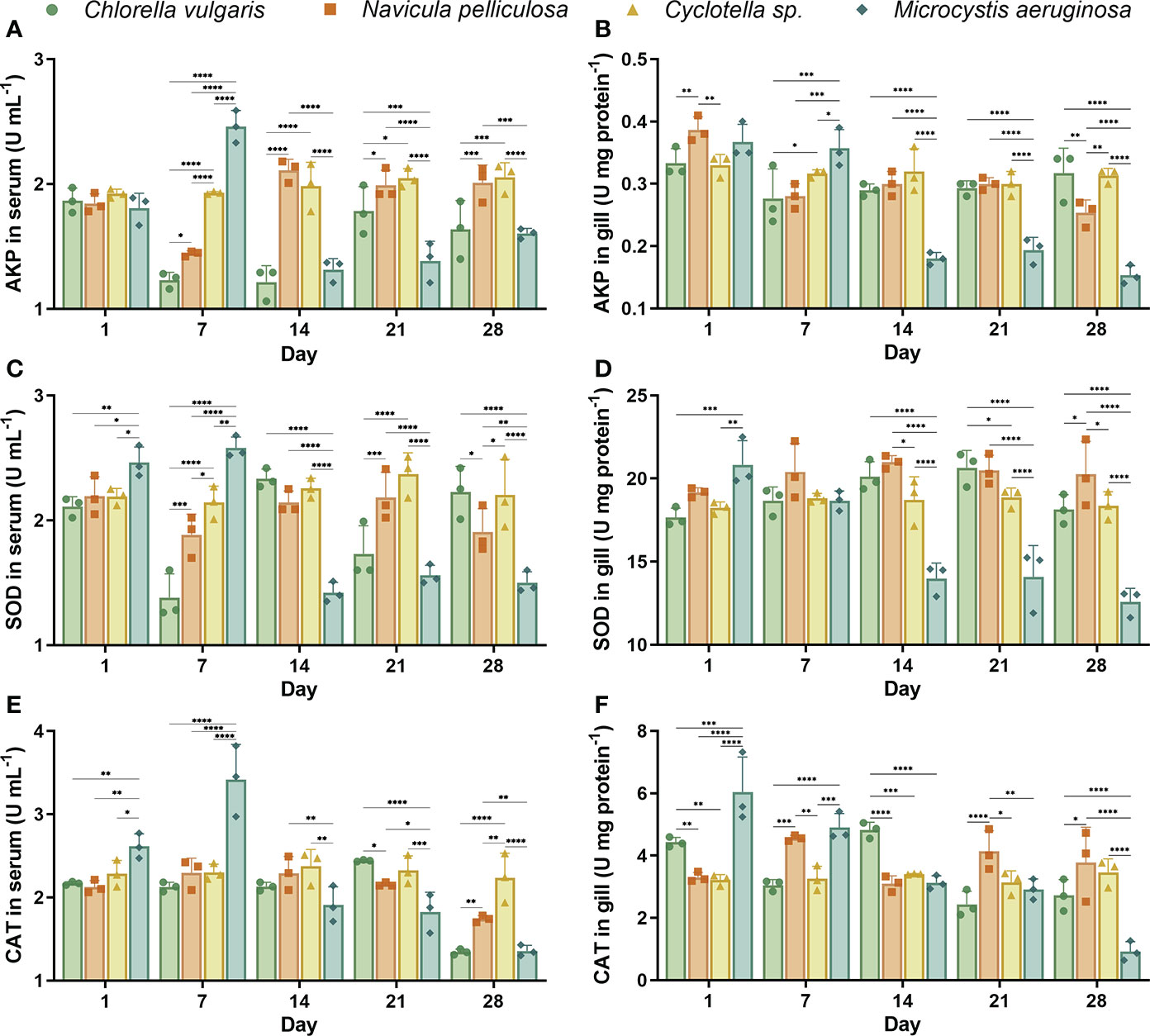
Figure 3 Activities of immune enzymes in serum and gill of Hyriopsis cumingii. Mussels exposed to four different microalgae treatments on days 1, 7, 14, 21, and 28. Immune enzyme activities in serum: (A) AKP, (C) SOD, and (E) CAT. Immune enzyme activities in gill: (B) AKP, (D) SOD, and (F) CAT. The asterisk (*) indicates significant differences between four microalgae treatments at each sampling time (Tukey test, p < 0.05). Values are expressed as mean ± SD (n = 3). AKP, alkaline phosphatase; SOD, superoxide dismutase; CAT, catalase. The symbols *, ** , *** and **** represent p < 0.05, p < 0.01, p < 0.001 and p < 0.0001 respectively.
The SOD activity in the serum of the M. aeruginosa treatment on day 7 (2.58 ± 0.07 U ml−1) was significantly (p < 0.05) higher than in the other three treatments (Figure 3C). SOD activities in the serum of the C. vulgaris treatment on days 7 and 21 and the M. aeruginosa treatment on days 14, 21, and 28 were lower than those of the Cyclotella sp. treatment. The SOD activity in the gill of the M. aeruginosa treatment on day 1 (20.84 ± 1.18 U mg protein−1) was significantly (p < 0.05) higher than that of C. vulgaris (17.66 ± 0.46 U mg protein−1) and Cyclotella sp. (18.84 ± 0.28 U mg protein−1) treatments. SOD activities in the gill of C. vulgaris, N. pelliculosa, and Cyclotella sp. treatments were significantly (p < 0.05) higher than those of the M. aeruginosa treatment on days 14, 21, and 28 (Figure 3D).
CAT activities in the serum of the M. aeruginosa treatment on days 1 and 7 were significantly (p < 0.05) higher than those of the other three treatments (Figure 3E). On day 28, there was a significant reduction in CAT activities in the serum of the C. vulgaris and N. pelliculosa treatments, and the CAT activity in the serum of the Cyclotella sp. treatment (2.24 ± 0.24 U ml−1) was higher than that of the other three treatments. CAT activities in the gill of the M. aeruginosa treatment on days 1 and 7, the N. pelliculosa treatment on day 7, and the C. vulgaris treatment on day 14 were higher than those of the other treatments at the same sampling time (Figure 3F). The CAT activity in the gill of the M. aeruginosa treatment on day 28 (0.92 ± 0.26 U mg protein−1) was significantly (p < 0.05) lower than that of the other three treatments.
Transcript levels of immune-related genes such as HcIL-17 and IAP in the liver of mussels were significantly (p < 0.05) affected by four algae treatments during the experimental period (Figures 2C, D). Relative expression levels of HcIL-17 and IAP in the M. aeruginosa treatment increased on day 1 but gradually decreased on days 14 and 28 (significantly lower than other treatments). Time had no effects on HcIL-17 and IAP expression levels in C. vulgaris, N. pelliculosa, and Cyclotella sp. treatments during the experimental period.
Discussion
The cultivation mode of H. cumingii urgently needs transformation and upgrading, and industrialized culture has become a new direction for the sustainable development of freshwater shellfish in the future because of its characteristics of environmental protection, high yield, and easy operation. The key to success in industrialized culture is the exploration of preferred microalgal diets of H. cumingii. In our study, enzyme and gene expression levels of digestion (showed in Figure S1 in the Supplementary Material), metabolism and immunity in H. cumingii maintained higher levels in the Cyclotella sp. treatment on day 28, indicating that Cyclotella sp. was suitable algae for H. cumingii, and mussels in this treatment had better metabolic abilities, immune function, and physiological status. In C. vulgaris and N. pelliculosa treatments, enzyme and gene expression levels of metabolism were lower than those in the Cyclotella sp. treatment. This is because the effects of algal species on bivalves are critically related to their abilities to supply essential nutrients to bivalves, in addition to physical properties of algae such as size and shape. The nutritional value of algae is mainly reflected in differences in biochemical components, and compared with proteins and carbohydrates, the lipid composition is more likely to lead to differences (Geng et al., 2016). Because of the low ability to synthesize and biotransform essential fatty acids, bivalves must obtain these nutrients from diets. Similar to our findings, a study proves that Cyclotella sp. is the optimum microalgal diet for H. cumingii from the perspective of the greatest increases in growth and pearl weight, saturated fatty acids, and monounsaturated fatty acids of mussels.
In addition to physical and chemical methods, different nature-based biomanipulation methods are used in a large number of practical cases to control algal blooms (Triest et al., 2016). With the emergence of shortcomings in fish manipulation (an inadequate reduction in the standing stock of planktivorous fish and the inability of piscivorous fish to control small fish) (Gulati et al., 2008), utilizing interactions of bivalves and algae makes bivalves a potential biomanipulation tool to effectively control eutrophication of water bodies. In small-scale bivalve biomanipulation experiments for mostly less than 30 days, freshwater bivalves can effectively reduce concentrations of total suspended solids, chlorophyll content, and concentrations of total phosphorus and increase transparency and concentrations of soluble reactive phosphorus in water (Wu et al., 2018; Gu et al., 2020). A 14-day exposure experiment of M. aeruginosa (1.7 × 107 cells ml−1) found that short-term ingestion of toxic M. aeruginosa is not fatal to H. cumingii and that mussels also can recover to health (Liu et al., 2020). In our study, M. aeruginosa stimulates immune responses of H. cumingii in a short-term exposure (7 days), and there was a significant reduction in the metabolism and immune abilities of mussels on the 28th day of this treatment. On the one hand, there were no dead mussels, and the concentration of M. aeruginosa used in the experiment (4.5 × 106 cells ml−1) was higher than that in natural environment (about 2 × 105 cells ml−1 in China Lake Taihu and Dianchi), indicating that H. cumingii has a high tolerance to M. aeruginosa and the ability to control eutrophication in the natural environment. On the other hand, it should deserve attention that the toxic M. aeruginosa exhibits negative effects on the physiology such as the clearance rate and absorption efficiency of bivalves (Hu et al., 2016). The same as the results of our study, exposure to M. aeruginosa still influences the metabolism and the immunological mechanism of bivalves (Hu et al., 2015). Therefore, more studies are needed to use H. cumingii for bivalve biomanipulation. The purpose of this study was to help screen suitable algal diets for H. cumingii in industrialized cultures and provide supporting theories for bivalve biomanipulation by contrasting the effects of high-quality microalgal diets and the toxic M. aeruginosa on the physiological state of H. cumingii. The following is a specific analysis of how these microalgae affected the metabolism, immune enzyme, and gene expression levels of H. cumingii.
Transaminases have a key role in mobilizing amino acids for gluconeogenesis and function in carbohydrate and protein metabolism (Ramaswamy et al., 1999). Levels of GOT and GPT can reflect the turnover efficiency of amino acids, and they are usually used as biochemical markers to determine tissue damage in the liver when aquatic animals face changes in physiology and pathology induced by environmental conditions (Hong et al., 2015). The ability of aquatic animals to use carbohydrates depends on their ability of glucose oxidation, glycogen biosynthesis, and lipogenesis (Guo et al., 2006). HK and PK are two key glycolytic regulatory enzymes, and they can reflect the capacity to metabolize glucose in aquatic animals (Xia et al., 2015). A study about effects on aminotransferases activities of sea cucumber Apostichopus japonicus fed with different diets such as brown algae, red algae, and green algae and addition of benthic matter (composed of benthic diatoms, dinoflagellates, detritus, and fine clay particles) for 70 days shows that the mixture of red algae and benthic matter significantly increases GOT and GPT activities, transamination, and protein metabolism, and A. japonicus has a higher specific growth rate (Wen et al., 2016). To assess the influence of food type on biomarkers, the PK activity of mussels Mytilus galloprovincialis fed with commercial food is much lower than those fed with live microalgae (Isochrysis galbana and Tetraselmis chuii), suggesting decreased aerobic scope, increased gluconeogenesis, and lower nutritional status of mussels (Blanco-Rayon et al., 2019). In our study, GOT, GPT, PK, and HK activities of mussels in the Cyclotella sp. treatment remained stable and were significantly higher than those in C. vulgaris and N. pelliculosa treatments after 7 days. The 28-day toxic M. aeruginosa treatment significantly reduced these metabolic enzyme activities in the liver of H. cumingii. Results suggested that mussels in the Cyclotella sp. treatment had a higher capacity to metabolize protein and glucose, and decreased metabolic enzyme activities in mussels from the M. aeruginosa treatment might be caused by liver tissue damage.
LDLR has a crucial function in lipid uptake and transport into liver cells, then lipids are targeted to catabolic and anabolic pathways, and CPT1 has a key role in lipid catabolic metabolism (Liu et al., 2018). Similar to the results of the above metabolize enzymes, mussels in the Cyclotella sp. treatment had higher expression levels of LDLR and CPT1 than mussels in C. vulgaris and N. pelliculosa treatments, suggesting that these mussels had higher lipid uptake and metabolic efficiency. The 28-day toxic M. aeruginosa treatment led to metabolism dysfunction and affected the expression of related genes in mussels.
How to boost the immunity of mussels through nutritional methods should be a concern. Bivalves rely on innate immunity to provide defense, and this immunity involves both cell-mediated and humoral systems. The phosphomonoesterase AKP has a crucial function in the digestion and degradation of foreign proteins, carbohydrates, and lipids in the lysosome, participating in non-specific immune actions of aquatic animals (Liang et al., 2014). Under oxidative stress, when the production of free radicals exceeds the level of natural antioxidant defense regulation, it triggers the oxidation of DNA, protein, and lipids, resulting in multiple disease conditions (Ighodaro and Akinloye, 2018). Antioxidant enzymes such as SOD and CAT are the first and most important line of defense in the antioxidant system, respectively, dismutase superoxide radicals into H2O2 and water and breakdown of H2O2 into O2 and water. Hydrolases AKP and antioxidase SOD and CAT, as non-specific humoral immune factors, participate in immune responses, and they are often used as reliable indicators in assessing the immune status of bivalves (Hu et al., 2015). Our results showed that AKP, SOD, and CAT activities of H. cumingii in the Cyclotella sp. treatment remained stable, and mussels in this treatment had higher immunity. The fluctuation of these immune enzyme activities in C. vulgaris and N. pelliculosa treatments may be related to changes in immune responses and physiological instability of H. cumingii caused by these algae. Similar to our study, after 45 days, feeding diets such as Chlorella sp. powder and Spirulina platensis powder significantly increased AKP, SOD, and CAT activities and absolute growth rates of pearl oyster Pinctada fucata martensii as compared with the corn gluten diet, suggesting that immunity of P. f. martensii can be improved by these algal diets. However, most AKP, SOD, and CAT activities in the M. aeruginosa treatment significantly increased at first and then decreased. It suggested that toxic algae stimulated immune responses of mussels in a short time (Hu et al., 2015) and then caused immune damage and enzyme inactivation.
HcIL-17 is a key inducer of proinflammatory cytokine secretion, IAP has a crucial function in regulating apoptosis and immune defense, and these genes have been verified to be involved in the bivalve immune responses (Rosani et al., 2015; Zhu et al., 2021). HcIL-17 and IAP expression levels of H. cumingii in the M. aeruginosa treatment increased on day 1 and then decreased after 14 days. Toxic algae created an inflammatory milieu in liver tissues and stimulated the immune response of mussels (Dou et al., 2020), and long-term stress of toxic algae caused immune disorders and metabolic imbalance and reduced the expression of related immune genes at last.
Results of PCA (Figure 4) visually supported that the suitable algal diet played a significant role in metabolic abilities, immune function, and physiological status, shown by the strong clustering of the side with higher enzyme activities in the Cyclotella sp. treatment during the experimental period. When accompanied with the 28-day toxic M. aeruginosa treatment, the clustering effect weakened, suggesting that toxic algae caused metabolic disorders, immune injury, and poor physiological status.
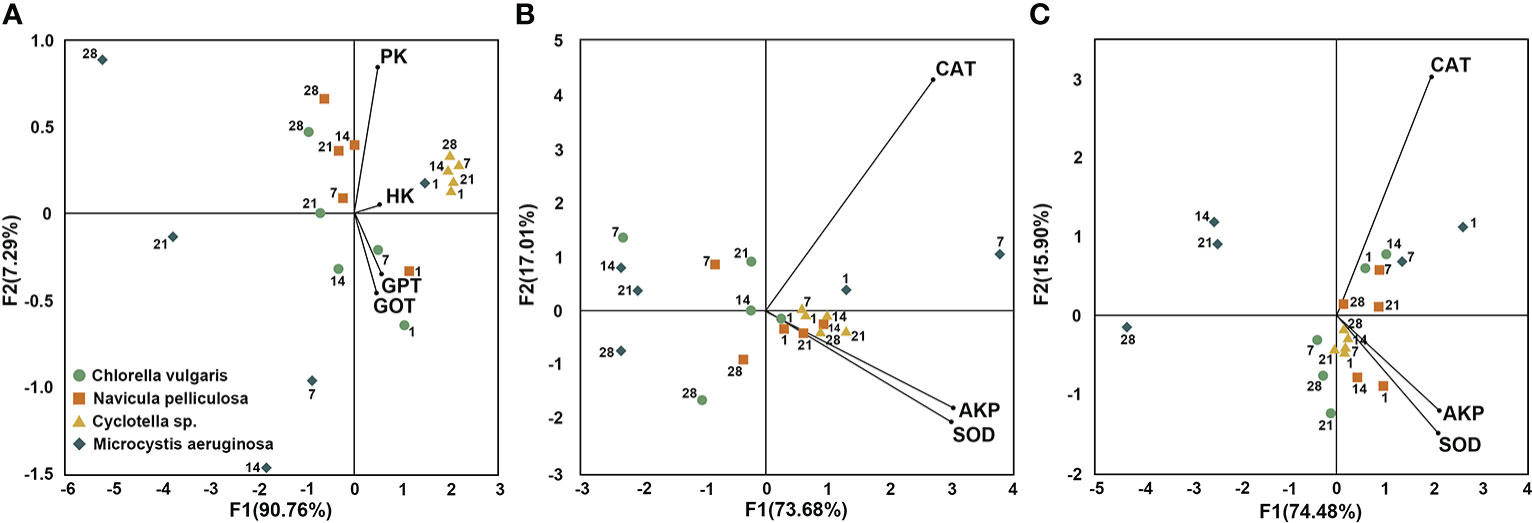
Figure 4 Biplot of principal component analysis (PCA) integrating metabolic and immune enzyme activities of Hyriopsis cumingii. (A) PCA for metabolic enzymes in liver, (B) PCA for immune enzymes in serum, and (C) PCA for immune enzymes in gill. Metabolic enzymes: GOT, GPT, PK, and HK. Immune enzymes: AKP, SOD, and CAT. Four microalgae treatments: Chlorella vulgaris, Navicula pelliculosa, Cyclotella sp., and Microcystis aeruginosa. Sampling time points: days 1, 7, 14, 21, and 28. GOT, glutamic oxaloacetate transaminase; GPT, glutamic pyruvate transaminase; PK, pyruvate kinase; HK, hexokinase; AKP, alkaline phosphatase; SOD, superoxide dismutase; CAT, catalase.
Conclusions
Metabolism, immune enzyme, and gene expression levels in H. cumingii had no significant changes and remained at a high level in the Cyclotella sp. treatment on day 28, suggesting that Cyclotella sp. was the suitable algae for H. cumingii, followed by C. vulgaris and N. pelliculosa. The toxic M. aeruginosa treatment resulted in metabolic disorders and immune injury, though no mussels died. These findings provide basic information on metabolism and immune mechanisms in H. cumingii exposed to different algae. How the suitable algae can apply to practices of the long-term industrialized culture of H. cumingii by considering mussel growth and the pearl quality should be studied in the future. How to apply H. cumingii to bivalve biomanipulation in a large-scale or whole ecosystem needs more studies about the tolerance of mussels to M. aeruginosa, specific stocking methods, and adjusting measures to local conditions.
Data availability statement
The original contributions presented in the study are included in the article/Supplementary Material. Further inquiries can be directed to the corresponding author.
Ethics statement
All applicable international, national, and/or institutional guidelines for the care and use of animals were followed by the authors.
Funding
This work was supported by the earmarked fund for CARS (Grant No. CARS-49), the National Natural Science Foundation of China (Grant No. 31770965), and the Chongqing Natural Science Foundation Project (Grant No. cstc2020jcyj-msxmX0971).
Conflict of interest
The authors declare that the research was conducted in the absence of any commercial or financial relationships that could be construed as a potential conflict of interest.
Publisher’s note
All claims expressed in this article are solely those of the authors and do not necessarily represent those of their affiliated organizations, or those of the publisher, the editors and the reviewers. Any product that may be evaluated in this article, or claim that may be made by its manufacturer, is not guaranteed or endorsed by the publisher.
Supplementary Material
The Supplementary Material for this article can be found online at: https://www.frontiersin.org/articles/10.3389/fmars.2022.970781/full#supplementary-material
References
Albentosa M., Sanchez-Hernandez M., Campillo J. A., Moyano F. J. (2012). Relationship between physiological measurements (SFG -scope for growth-) and the functionality of the digestive gland in mytilus galloprovincialis. Comp. Biochem. Physiol. a-Molecular Integr. Physiol. 163, 286–295. doi: 10.1016/j.cbpa.2012.07.019
Blanco-Rayon E., Ivanina A. V., Sokolova I. M., Marigomez I., Izagirre U. (2019). Food-type may jeopardize biomarker interpretation in mussels used in aquatic toxicological experimentation. PloS One 14, e0220661. doi: 10.1371/journal.pone.0220661
Chen Q., Jiang X. M., Han Q. X., Sheng P., Chai Y. H., Peng R. B., et al. (2021). Growth, calcium content, proximate composition, and fatty acid composition of triangle sail mussel (Hyriopsis cumingii) fed five different microalgal diets. Aquaculture 530, 735719. doi: 10.1016/j.aquaculture.2020.735719
Dou M., Jiao Y. H., Zheng J. W., Zhang G., Li H. Y., Liu J. S., et al. (2020). De novo transcriptome analysis of the mussel perna viridis after exposure to the toxic dinoflagellate prorocentrum lima. Ecotoxicol. Environ. Saf. 192, 110265. doi: 10.1016/j.ecoenv.2020.110265
Geng S. S., Zhou C. X., Chen W. B., Yu S. S., Huang W., Huan T., et al. (2016). Fatty acid and sterol composition reveal food selectivity of juvenile ark shell tegillarca granosa Linnaeus after feeding with mixed microalgae. Aquaculture 455, 109–117. doi: 10.1016/j.aquaculture.2016.01.012
Gulati R. D., Pires L. M. D., Van Donk E. (2008). Lake restoration studies: Failures, bottlenecks and prospects of new ecotechnological measures. Limnologica 38, 233–247. doi: 10.1016/j.limno.2008.05.008
Gu J., Li K. Y., Jeppesen E., Han Y. Q., Jin H., He H., et al. (2020). Using freshwater bivalves (Corbicula fluminea) to alleviate harmful effects of small-sized crucian carp (Carassius carassius) on growth of submerged macrophytes during lake restoration by biomanipulation. Water 12, 3161. doi: 10.3390/w12113161
Guo R., Liu Y. J., Tian L. X., Huang J. W. (2006). Effect of dietary cornstarch levels on growth performance, digestibility and microscopic structure in the white shrimp, litopenaeus vannamei reared in brackish water. Aquacult. Nutr. 12, 83–88. doi: 10.1111/j.1365-2095.2006.00384.x
He H., Liu X. B., Liu X. L., Yu J. L., Li K. Y., Guan B. H., et al. (2014). Effects of cyanobacterial blooms on submerged macrophytes alleviated by the native Chinese bivalve hyriopsis cumingii: A mesocosm experiment study. Ecol. Eng. 71, 363–367. doi: 10.1016/j.ecoleng.2014.07.015
Hong Y., Jiang W. D., Kuang S. Y., Hu K., Tang L., Liu Y., et al. (2015). Growth, digestive and absorptive capacity and antioxidant status in intestine and hepatopancreas of sub-adult grass carp ctenopharyngodonidella fed graded levels of dietary threonine. J. Anim. Sci. Biotechnol. 6, 34. doi: 10.1186/s40104-015-0032-1
Hu M. H., Wu F. L., Yuan M. Z., Li Q. Z., Gu Y. D., Wang Y. J., et al. (2015). Antioxidant responses of triangle sail mussel hyriopsis cumingii exposed to harmful algae microcystis aeruginosa and hypoxia. Chemosphere 139, 541–549. doi: 10.1016/j.chemosphere.2015.07.074
Hu M. H., Wu F. L., Yuan M. Z., Liu Q. G., Wang Y. J. (2016). Combined effects of toxic cyanobacteria microcystis aeruginosa and hypoxia on the physiological responses of triangle sail mussel hyriopsis cumingii. J. Hazardous Materials 306, 24–33. doi: 10.1016/j.jhazmat.2015.11.052
Ighodaro O. M., Akinloye O. A. (2018). First line defence antioxidants-superoxide dismutase (SOD), catalase (CAT) and glutathione peroxidase (GPX): Their fundamental role in the entire antioxidant defence grid. Alexandria J. Med. 54, 287–293. doi: 10.1016/j.ajme.2017.09.001
Liang S., Luo X., You W. W., Luo L. Z., Ke C. H. (2014). The role of hybridization in improving the immune response and thermal tolerance of abalone. Fish Shellfish Immunol. 39, 69–77. doi: 10.1016/j.fsi.2014.04.014
Liu C., Kim Y. S., Kim J., Pattison J., Kamaid A., Miller Y. I. (2018). Modeling hypercholesterolemia and vascular lipid accumulation in LDL receptor mutant zebrafish. J. Lipid Res. 59, 391–399. doi: 10.1194/jlr.D081521
Liu Y. M., Li L., Zheng L., Fu P., Wang Y., Haidang N., et al. (2020). Antioxidant responses of triangle sail mussel hyriopsis cumingii exposed to harmful algae microcystis aeruginosa and high pH. Chemosphere 243, 125241. doi: 10.1016/j.chemosphere.2019.125241
Pan L. Q., Ren J. Y., Liu J. (2006). Responses of antioxidant systems and LPO level to benzo(a)pyrene and benzo(k)fluoranthene in the haemolymph of the scallop chlamys ferrari. Environ. Pollut. 141, 443–451. doi: 10.1016/j.envpol.2005.08.069
Ramaswamy M., Thangavel P., Selvam N. P. (1999). Glutamic oxaloacetic transaminase (GOT) and glutamic pyruvic transaminase (GPT) enzyme activities in different tissues of sarotherodon mossambicus (Peters) exposed to a carbamate pesticide, carbaryl. Pesticide Sci. 55, 1217–1221. doi: 10.1002/ps.2780551214
Rosani U., Varotto L., Gerdol M., Pallavicini A., Venier P. (2015). IL-17 signaling components in bivalves: Comparative sequence analysis and involvement in the immune responses. Dev. Comp. Immunol. 52, 255–268. doi: 10.1016/j.dci.2015.05.001
Ruijter J. M., Ramakers C., Hoogaars W. M. H., Karlen Y., Bakker O., van den Hoff M. J. B., et al. (2009). Amplification efficiency: linking baseline and bias in the analysis of quantitative PCR data. Nucleic Acids Res. 37, e45. doi: 10.1093/nar/gkp045
Sun Y., Liu Y., Yu X. B., Wang T., Wang Q., Yao W. Z., et al. (2021). Combined effects of ration levels and temperature on immune responses of the triangle sail mussel hyriopsis cumingii. Aquacult. Res. 53, 440–452. doi: 10.1111/are.15586
Tang J. Y., Dai Y. X., Wang Y., Qin J. G., Li Y. M. (2015). Improvement of fish and pearl yields and nutrient utilization efficiency through fish-mussel integration and feed supplementation. Aquaculture 448, 321–326. doi: 10.1016/j.aquaculture.2015.05.028
Triest L., Stiers I., Van Onsem S. (2016). Biomanipulation as a nature-based solution to reduce cyanobacterial blooms. Aquat. Ecol. 50, 461–483. doi: 10.1007/s10452-015-9548-x
Wang L., Ma L., Sun J., Zhang Y., Zhou Q. H., Wu Z. B., et al. (2018). Effects of different aquaculture methods for introduced bivalves (Hyriopsis cumingii) on seston removal and phosphorus balance at the water-sediment interface. J. Freshw. Ecol. 33, 251–265. doi: 10.1080/02705060.2018.1429328
Wen B., Gao Q. F., Dong S. L., Hou Y. R., Yu H. B., Li W. D. (2016). Effects of different feed ingredients on growth, fatty acid profiles, lipid peroxidation and aminotransferases activities of sea cucumber apostichopus japonicus (Selenka). Aquaculture 454, 176–183. doi: 10.1016/j.aquaculture.2015.12.027
Wu Z. K., Qiu X. C., Zhang X. F., Liu Z. W., Tang Y. L. (2018). Effects of anodonta woodiana on water quality improvement in restoration of eutrophic shallow lakes. Hupo Kexue 30, 1610–1615. doi: 10.18307/2018.0612
Xia B., Gao Q. F., Wang J. Y., Li P. Y., Zhang L. M., Zhang Z. D. (2015). Effects of dietary carbohydrate level on growth, biochemical composition and glucose metabolism of juvenile sea cucumber apostichopus japonicus (Selenka). Aquaculture 448, 63–70. doi: 10.1016/j.aquaculture.2015.05.038
Yang Q. L., Yu X. B., Du C. Y., Ni X., Li W. Y., Yao W. Z., et al. (2021). Bacterial challenge undermines the innate immune response in hyriopsis cumingii. Aquaculture 530, 735783. doi: 10.1016/j.aquaculture.2020.735783
Keywords: hyriopsis cumingii, microalgae, metabolism, non-specific immunity, gene expression
Citation: Sun Y, Guo K, Yu X, Li Y, Yao W and Wu Z (2022) Molecular and biochemical effects on metabolism and immunity of Hyriopsis cumingii fed with four different microalgae. Front. Mar. Sci. 9:970781. doi: 10.3389/fmars.2022.970781
Received: 16 June 2022; Accepted: 11 July 2022;
Published: 04 August 2022.
Edited by:
Dr. Shivendra Kumar, Dr. Rajendra Prasad Central Agricultural University, IndiaReviewed by:
Srijit Chakravarty, Central Institute of Fisheries Education (ICAR), IndiaSarvendra Kumar, College of Fisheries Kishanganj, India
Copyright © 2022 Sun, Guo, Yu, Li, Yao and Wu. This is an open-access article distributed under the terms of the Creative Commons Attribution License (CC BY). The use, distribution or reproduction in other forums is permitted, provided the original author(s) and the copyright owner(s) are credited and that the original publication in this journal is cited, in accordance with accepted academic practice. No use, distribution or reproduction is permitted which does not comply with these terms.
*Correspondence: Zhengli Wu, zh20140202@swu.edu.cn