- 1Department of Aquaculture and Poultry, Institute of Animal Sciences, Agricultural Research Organization, Volcani Center, Rishon Letziyon, Israel
- 2Department of Animal Sciences, The Robert H. Smith Faculty of Agriculture Food and Environment, Hebrew University of Jerusalem, Rehovot, Israel
The flathead grey mullet (Mugil cephalus) is at the focus of an intense domestication effort. However, despite significant advances, aquaculture of grey mullets is still based on capture of wild fingerlings in estuaries and stocking them into ponds. Such reliance on wild seed limits aquaculture production since it is highly seasonal, unpredictable, hinders genetic improvement programs and may negatively affect local capture fisheries. As captive breeding has been optimized for the species, to date, larval rearing remains the main challenge hindering the commercial production of captive-bred mullets. Here we set out to characterize larval development of grey mullets under captive conditions and to identify key developmental bottlenecks. By analyzing four larval batches from a commercial hatchery, we defined ten distinct developmental stages from hatching to the end of metamorphosis based on macroscopically observable morphological features. Using this developmental atlas to study development dynamics, we found that mullet larvae exhibit a biphasic growth profile with a 17-fold increase in growth rates at the onset of metamorphosis. Moreover, we found that within rearing batches, size variation almost doubles at metamorphosis, suggesting that the onset of metamorphosis is a critical step that increases variation by dictating different growth trajectories to early and late-metamorphosing larvae. By plotting the developmental stages against different morphometric parameters, we show here that age is a poor predictor of larval development due to large variations in development rates both within and between batches. We found that mullet larvae begin metamorphosis at a set size threshold of ~4.5 mm, however age at this threshold varies greatly even within batches. This age and size variance is further amplified by the aforementioned enhanced growth rates at metamorphosis and results in highly non-uniform fry, which complicate the rearing and weaning procedures. Understanding and treating the underlying causes for delayed metamorphosis are therefore considered important steps for the full domestication of the grey mullet.
Introduction
Throughout most of their natural range, grey mullets (Mugil cephalus) are consumed for their flesh as well as for their ovaries, which are used in the roe industry and are in high demand in many Asian and Mediterranean markets. As a result, mullets have been fished and cultured for centuries mostly in Europe, Asia and Africa (Lovatteli and Holthus, 2008). In aquaculture, grey mullets are raised in extensive and semi intensive ponds systems and as monoculture or polyculture with carp and tilapia (Sadek and Mires, 2000; Saleh, 2008; Whitfield et al., 2012; Gisbert et al., 2016). Several attributes including high meat quality, their tolerance to a wide range of salinities and their omnivorous diet (De Silva and Wijeyaratne, 1977; Wells, 1984) make grey mullets particularly desirable as an aquaculture species. To date, Egypt is the world’s largest producer of grey mullets, with production of 156,400 tons in 2005 (Saleh, 2008; Shaalan et al., 2018). Other producers include Korea, Israel, Italy, Greece and Taiwan.
However, despite the considerable popularity as a food fish, captive bred grey mullets account for a negligible proportion of total mullet production. Instead, the aquaculture of the species is based on the capture of wild fry as they migrate from the open sea to estuaries and stocking them into growth ponds (Sadek and Mires, 2000; Saleh, 2008; Shaalan et al., 2018). From an aquaculture point of view, this dependence on wild fry is a major bottleneck that hampers the expansion of grey mullet farming since it is inherently seasonal and fluctuates widely between years. Limitation on hatchery production of fry also prevents selective breeding and genetic improvement programs (Whitfield et al., 2012). In addition, collection of wild fry creates severe environmental pressure on the natural ecosystem and affects resources that are critical for capture fisheries of the species (Whitfield et al., 2012). There is therefore an urgent need to develop reliable protocols for closing the entire life cycle of grey mullets in captivity that will enable year-round supply of adequate numbers of high-quality mullet fry for sustainable aquaculture.
Efforts invested in trying to close the life cycle of the grey mullet in captivity have been going on since the early 1970’s, mainly in experimental and semi-commercial facilities (Finucane et al., 1978; Kuo et al., 1972; Shehadeh et al., 1973; Liao, 1975; Aizen et al., 2005; Ramos-Júdez et al., 2022), but captive breeding on a commercial scale did not ripen so far. In fact, even today, large producers of cultured mullet such as Egypt and Italy still produce only several hundred thousand of fry annually, far less than the demand of the local aquaculture industries. In Israel, induced spawning protocols were published almost two decades ago (Aizen et al., 2005) and were further optimized by commercial hatcheries that are now able to spawn brood-stock year round by manipulating the photoperiod and temperature to create shifted spawning seasons. Nevertheless, hatchery raised mullet fry account for only 7% of Israeli market demands (Levi et al., 2018).
With the development of reliable captive breeding protocols, the main factor limiting commercial production of grey mullet fingerlings remains poor larval survival and development (Vallainc et al., 2021). This critical production stage still holds many challenges due to the scarce knowledge regarding larval development requirements of the species in captivity. In general, larval development spans the period from hatching until the completion of metamorphosis, upon which the larvae attain the full characteristics of the juvenile fish. This critical period is also exceptionally fragile and losses often exceed 99% in natural settings (Hjort, 1914) and over 70% in captivity (Planas and Cunha, 1999). Larval survival rates under hatchery conditions are particularly low in species at the beginning of their domestication process, such as the grey mullet, since the precise conditions required for their rearing have not yet been fully established. The two main periods where most larval losses occur under hatchery conditions are at first free feeding and around metamorphosis. After metamorphosis mortality rates decrease sharply and fingerlings can be switched to adult diets and become more resilient to handling and transportation. Therefore, raising larvae through metamorphosis constitutes a significant bottleneck in many larval rearing operations.
As a step towards removing bottlenecks in grey mullet larviculture, we set out to generate a developmental atlas of the species that will allow the precise staging of larval development. Such a tool is important as a reference for diagnosing and treating developmental delays. It is also useful for normalizing growth under different rearing conditions and for the transfer of larval rearing technologies to new end-users. Here, we provide a detailed description of grey mullet larval development under captive conditions, based upon morphological features, and investigate how discrete morphological traits characterizing developmental stages correlate with continuous morphometric traits. Using these tools, we identify metamorphosis as a major bottleneck in larval development and as a main source for non-uniform juvenile growth.
Materials and methods
Larval rearing procedures
All procedures were conducted according to the instructions and under the authorization of the ARO ethics committee (authorization no. 909/21). Larvae were sampled from batches spawned and reared in the commercial hatchery of Dagon in Kibbutz Ma’agan Michael in Israel.
Brood stock were held in an isolated facility with photoperiod control to simulate either natural or shifted spawning seasons. Spawning, according to photoperiod season, was induced by a single injection of gonadotropin releasing hormone analog (Aizen et al., 2005), followed by transfer of one female and four males to a four cubic meter-spawning tank. Fish spawned 36 h after injection and eggs were collected in external 1 cubic meter tanks. Twelve hours later, 450-550 ml of eggs were transferred to indoor 10 cubic meter tanks for hatching and rearing, corresponding to an initial density of 170-200 larvae per liter. Hatching of the eggs occurred 34-38 hours after fertilization. During the entire larval rearing period, larvae were maintained in Mediterranean seawater (25-26°C, 38-40 ppt) sterilized by uv light and ozone and then passed through activated carbon to eliminate ozone traces.
Larval rearing protocols were largely similar to those described previously (Thieme et al., 2021; Vallainc et al., 2021). Feeding of larvae was initiated at day 2 post hatching (dph) with rotifers (Brachionus rotundiformis, 10–15 rotifer/mL) enriched with unicellular algae (Nannochloropsis sp.). Algal paste (Nannochloropsis sp., Reed Mariculture, CA.) was also added to the larval rearing tanks to increase turbidity (Koven et al., 2019; Besbes et al., 2020) and maintain the nutritional quality of the rotifers. Ten days post-hatching, newly hatched Artemia nauplii were incorporated into the larval feeding regime and 6 days later, they were substituted with enriched instar II Artemia. Application of dry food (56% protein, 14% fat) began at 5 dph and larvae were completely weaned onto dry food at 30 dph. Survival, calculated as number of juveniles moved to grow-out of initial eggs stocked, was 1.2, 4.35, 1.5 and 1.2% for batches A, B, C, and D, respectively.
Sampling
Larvae from four different spawning batches were sampled at different and overlapping time points from hatching (0 dph) to 30 dph, by which metamorphosis was completed and fry were moved to further grow-out. Group A were reared in July 2020, groups B,C and D were all spawned and reared in April-May 2021. Samples from each batch were collected from a single rearing tank. Sampling details are listed in Table 1.
Morphometric measurements and stage definition
Larvae were euthanized using tricaine overdose, fixed in 4% paraformaldehyde overnight at 4°C, and then transferred to phosphate buffered saline for preservation. Age of larvae was determined as days post hatching (dph). Ten to 46 larvae from each sampling point were imaged under a stereomicroscope (Nikon SMZ25). The standard length (SL) and eye diameter (ED) were measured to the nearest 10 µm using ImageJ software on the acquired larval images. For further analysis, larvae were weighed to the nearest 1 mg. Larvae were then inspected under a stereomicroscope to describe changes that occur during development in the following morphological features: eyes, yolk sac, oil globule, head region, fins, spinal cord, and pigmentation. These changes were used to define distinct stages and generate the developmental atlas. Onset of metamorphosis was defined as beginning of flexion followed by fin formation and fin rays ossification (Mcmenamin and Parichy, 2013; Schnitzler et al., 2017; Roux et al., 2019; Gajbhiye et al., 2022).
Statistics
All data was plotted using Prism 8 software (GraphPad, San Diego, CA). To correlate continuous measurements (age, standard length, eye diameter and weight), we used a bi-phasic linear regression model. To correlate stage with age, standard length, eye diameter, or weight, we treated stage as a continuous parameter and applied Pearson’s correlation analysis, using Prism 8 software (GraphPad). Coefficient of variance (CV) analysis was conducted using JMP pro 16 (SAS, Cary, NC).
Results
Larval developmental stages
Based on visible morphological features we defined 10 distinct developmental stages of grey mullet larval development (Figure 1 and Supplementary Table 1). Since many of the ontogenetic changes that we recorded change over a wide continuum, it was difficult to divide them into distinct stages. Therefore, for each developmental stage we chose one or two prominent morphological features that were designated as “stage defining” and which distinguish it clearly from its adjacent stages. These stage defining features include eye pigmentation changes (stages A to C), opening of the mouth (stage C), absorption of the yolk sac and oil globule (stages A to D), flexion angle (stages E to G), fin development (stages E to J) and the appearance of a silvery body color in the final stages of metamorphosis (stages I and J). All stage-defining features are detailed and illustrated in Figure 1.
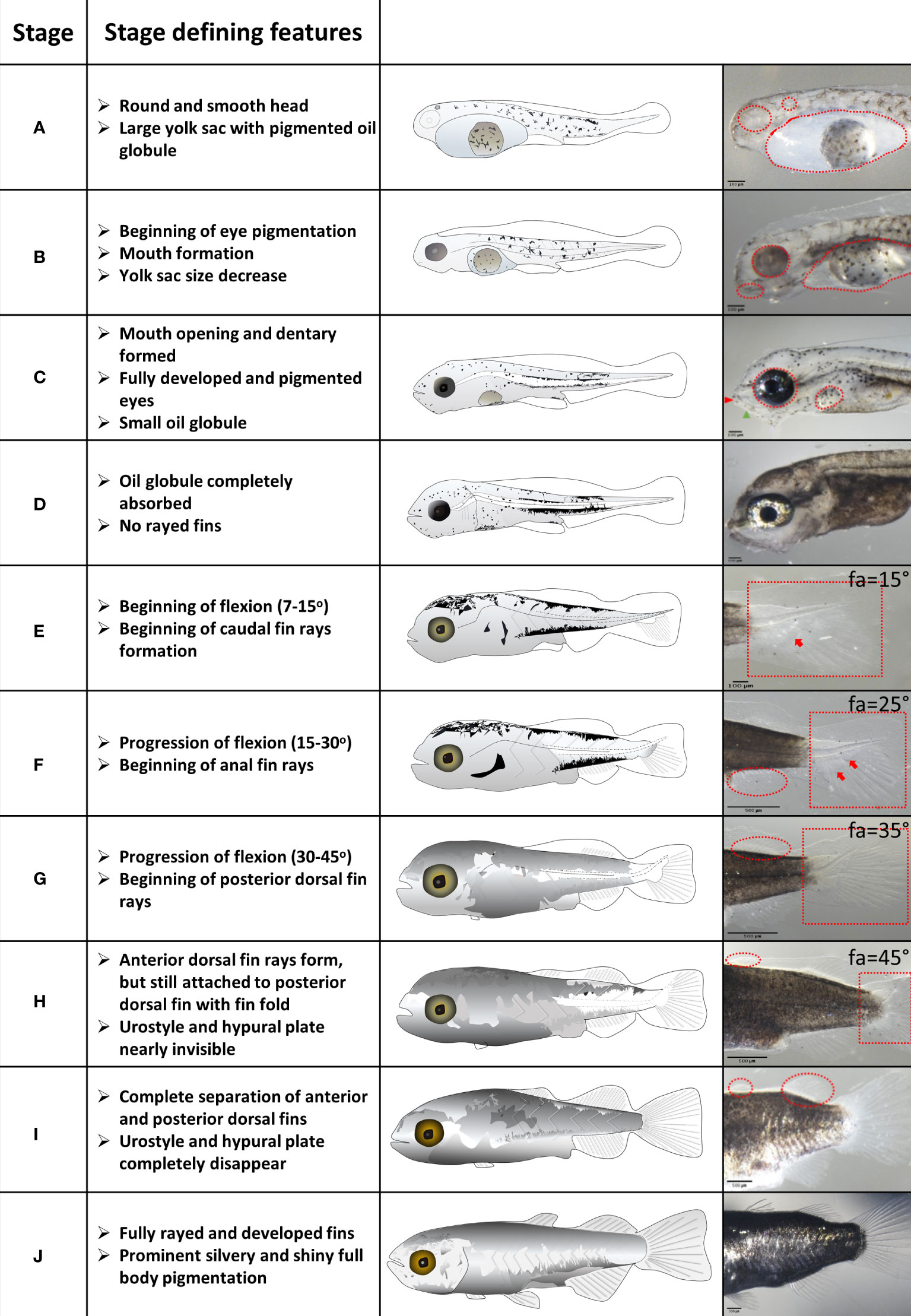
Figure 1 Stages of grey mullet larval development and metamorphosis. Stage defining features are described for each developmental stage. Important features are marked in red on the images in the right column. Fa – Flexion angle. Scale bar: Stages A to E – 100 µm; stages F to J – 500 µm.
Larvae nutrition and eye development
Grey mullet larvae hatched 34-38 hours post fertilization at 25-26°C, with a large yolk sac and a big, round oil globule (Figure 1 and Supplementary Figure 1; stage A). During stage B, the yolk sac was mostly depleted, whereas the oil globule largely maintained its size (Figure 1 and Supplementary Figure 1; stage B). The yolk sac was completely absorbed by stage C, and the oil globule was depleted by stage D (Figure 1 and Supplementary Figure 1). There is an overlap in stages between the depletion of the maternal nutritional stores in the form of the yolk sac and oil globule (stages A–D) and the onset of external feeding (stage C). Since mullet larvae are visual hunters, eye development needs to complete before the onset of external feeding. Larvae hatched with unpigmented eyes and an apparent otic vesicle (Figure 1 and Supplementary Figure 2, stage A). At stage B pigmentation of the eye began and by stage C, a well pigmented retina was developed, coinciding with mouth opening and development of the optic tectum and olfactory pits (Figure 1 and Supplementary Figure 2; stage C), allowing larvae to detect prey items.
Fin development
At hatching, grey mullet larvae were surrounded by a continuous fin fold from the mid-dorsal region to the ventral posterior part of the yolk sac (Figure 1 and Supplementary Figure 3; stage A). As the yolk sac began its absorption and the larvae attained a slender shape, the fin fold covered also the head (Figure 1 and Supplementary Figure 3; stage B). Pectoral fin buds were apparent at stage C and by stage D, the fin fold receded to the dorsal posterior two thirds of body (Figure 1 and Supplementary Figure 3; stage D). Development of fin rays is an important step in fins development since they form the mechanical cantilever that provides the required rigidity. As the anal and caudal fins began to differentiate, the caudal fin began developing ventral rays, coinciding with the beginning of notochord flexion (Figure 1 and Supplementary Figure 4, stage E). As the fin rays at the caudal fin continued to develop and began to shift horizontally, rays began developing in the anal fin (Figure 1 and Supplementary Figure 5, stage F). As the caudal fin developed rays in its central region, the second (posterior) dorsal fin began to ray and the pelvic fins began to form (Stage G, Figure 1 and Supplementary Figure 6). The last fin to differentiate was the first (anterior) dorsal fin beginning at stage H (Figure 1 and Supplementary Figure 7).
Notochord flexion and hypural plates development
Notochord flexion (changes in the angle of the posterior part of notochord) is a key feature of the initiation of metamorphosis (Mcmenamin and Parichy, 2013; Roux et al., 2019). In grey mullet, flexion began at stage E along with the initial development of the caudal fin rays and the appearance of the inferior hypural plate (Figure 1 and Supplementary Figure 4). As flexion progressed, both inferior and superior hypural plates became visible (stage F). The urostyle nearly disappeared by stage H; at this stage the hypural plates were also not visible any more (Figure 1 and Supplementary Figure 4). Notochord flexion was completed by stage I.
Body pigmentation
Grey mullet larvae hatched with stellate melanophores on the oil globule and body, excluding the posterior region (Figure 2, stage A). By stage C, melanophores developed and spread along the superior part of the head, trunk and covered a part of the body from the line of the anal pore towards the posterior region, but most of caudal region remained unpigmented. By stage D, all of the body was uniformly pigmented, except for the caudal region which acquired its pigmentation at stage E. Shiny silver iridophores first appeared at the onset of metamorphosis (stage E), in the middle of the body. Later, the iridophores spread anteriorly and posteriorly until the larvae was fully covered by shiny silver color at stage J (Figure 2).
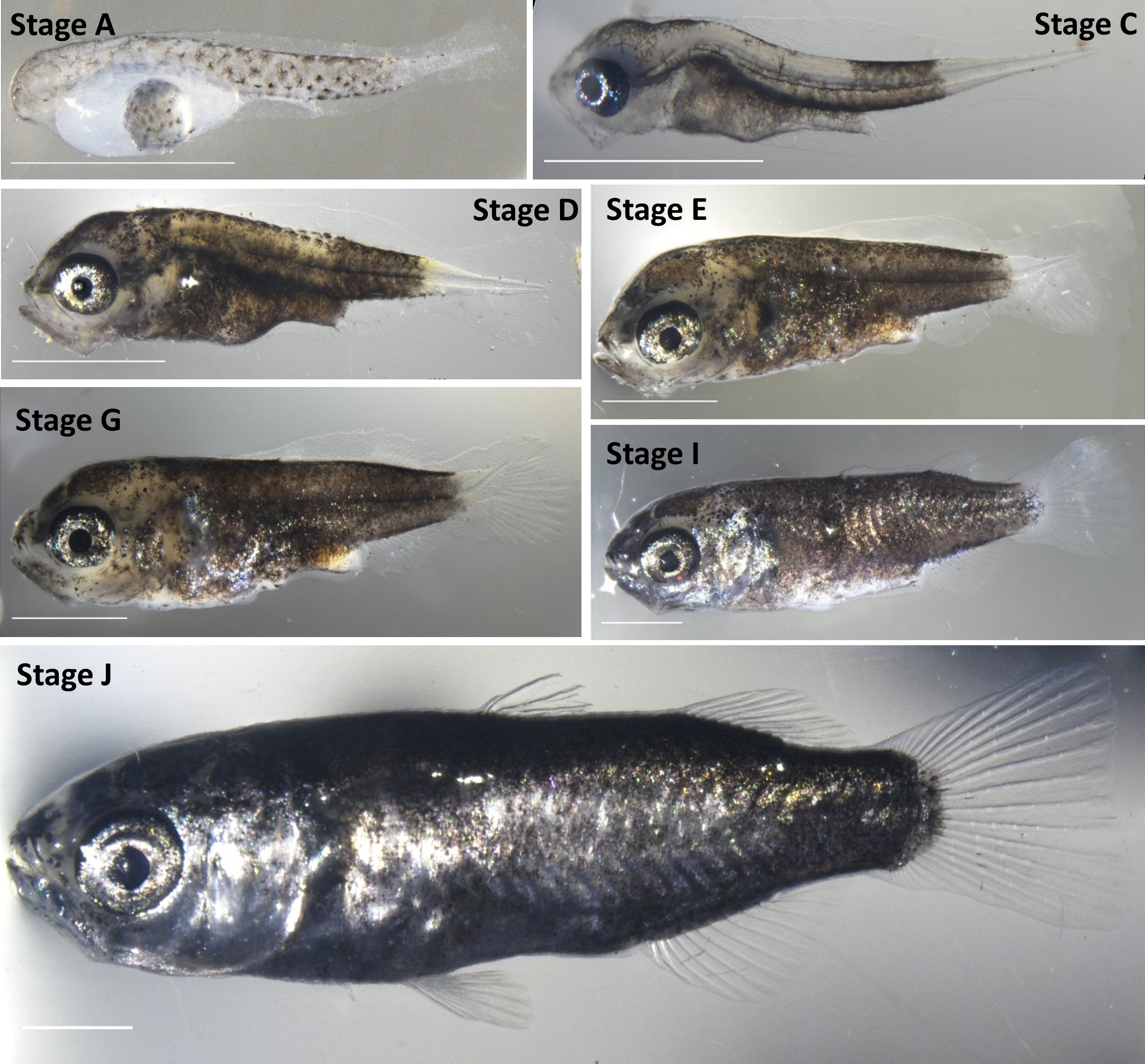
Figure 2 Development of pigmentation. Note that stages A to C are largely transparent. Dark pigmentations covers the body except for the caudal region from stage D to G. Silver iridophores first appear at stage E and gradually cover the body until the attainment of a full silver body at stage J. Scale bar-1 mm.
Larval growth
We used 4 quantitative parameters, namely age, standard length (SL), eye diameter (ED) and weight to follow the development of grey mullet larvae (Figure 3 and Supplementary Figure 8). Our larvae were sampled from four different rearing batches derived from two different spawning seasons and four different sets of parents, but all were reared under similar environmental conditions. Grey mullet larvae hatched relatively uniform in size, with a standard length of 2.36 ± 0.22 mm and eye diameter of 0.19 ± 0.02 mm. After yolk sac and oil globule were fully absorbed (stage D), the larvae attained a SL of 3.2 ± 0.48 mm and ED of 0.36 ± 0.06. We identify two main periods of development that differ in growth rates of the larvae. During the first period, until the onset of metamorphosis (12 dph, stage E) the growth was relatively slow (slope, SL= 24 μm/day, ED=15.7 μm/day). In comparison, after the onset of metamorphosis; fish growth rates increased by approximately 17-fold (Figures 3C, D; slope, SL= 411 μm/day, ED=42 μm/day). Notably, while growth and development during the first 12 days were relatively uniform both within and between batches, after metamorphosis (stage E/12 dph and onward), larger differences between individual fish began to appear, both within and between batches (Figures 3A–D). To quantify the variance between the fish within a particular age group we analyzed the coefficient of variance (CV), a normalized measure of the variation between the fish, for SL and ED. Our analysis shows a rise in variation within the age group in both parameters beginning around day 12 of development (Figures 3E, F). Consequently, as development proceeds, variance increases within the groups and their growth trajectories become less uniform. The trend is particularly evident in the SL values which are ~4-5 fold less uniform at the peak of metamorphosis (~18 dph) than during the early stages of development (Figure 3E).
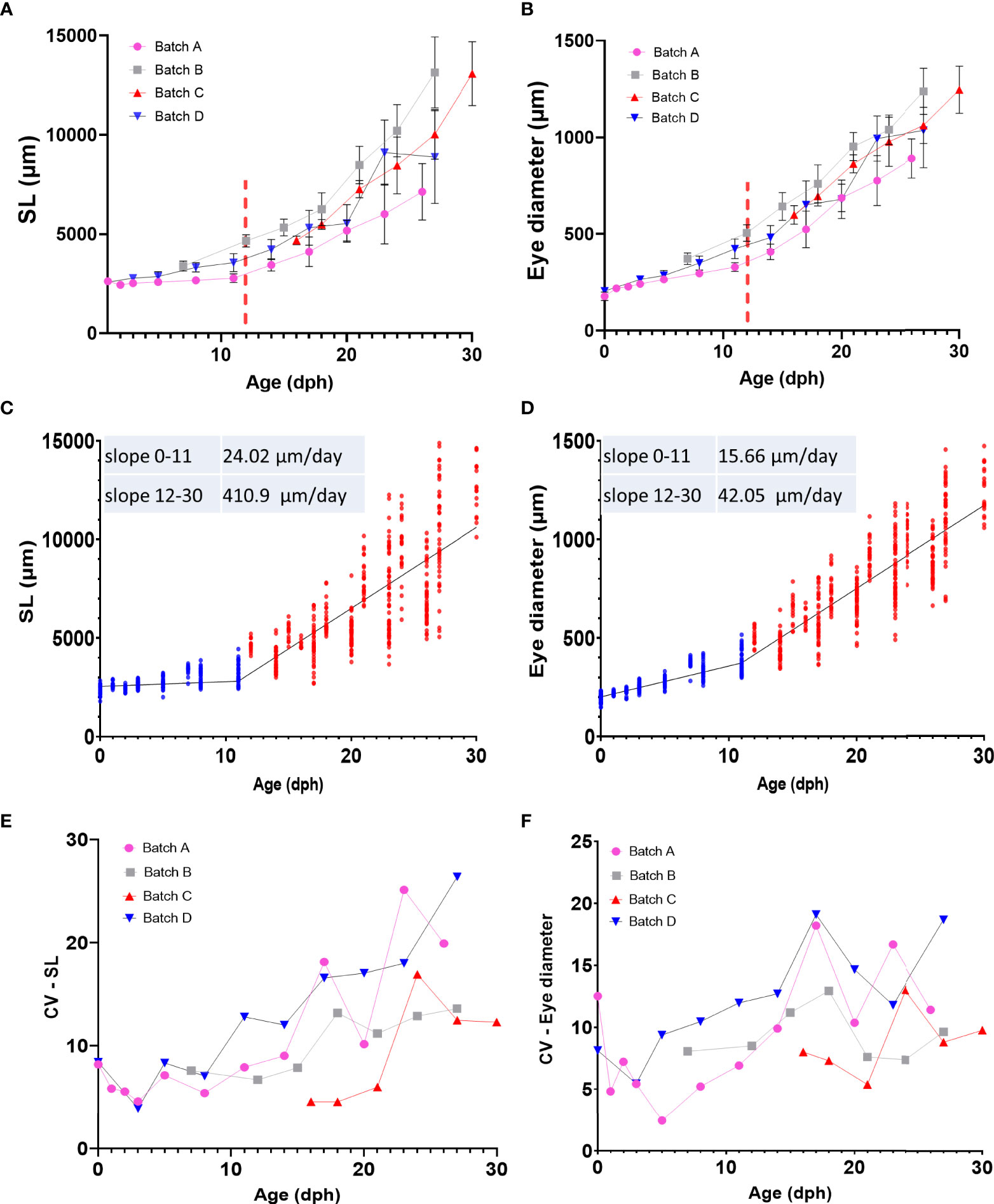
Figure 3 Growth pattern of grey mullet larvae (A) Age-standard length (SL) relationship (B) Age-Eye diameter (ED) relationship. In A and B, each batch is represented by a different color, Red dashed bar marks approximate onset of metamorphosis. (C, D) Linear regression of standard length (C) and eye diameter (D) by age. Both parameters show a biphasic growth pattern. Blue dots represent individual fish from 0 to 11 dph. Red dots represent individual fish from 12 to 30 dph. (E, F) Changes in variance (CV) of SL (E) and ED (F) in relationship to age in each of the sampling batches.
Effects of growth on developmental stage
To gain more insight into drivers of metamorphosis we next analyzed the relationship between age, weight, SL and ED on the developmental stage of the larvae (Figure 4 and Supplementary Figure 9). We first used Pearson correlation to check which parameter best correlates with the developmental stage. As expected, all the parameters correlated positively with developmental stage although r2 values slightly differed between parameters. In our data, age received the best score along with eye diameter and SL (r2 = 0.95, 0.95, 0. 83 for age, ED and SL respectively, Figures 4A–C), considerably better than weight (r2 = 0.53, Supplementary Figure 9). However, although in our Pearson correlation analyses all parameters were shown to positively affect developmental stage, not all relationships are necessarily linear and the large variations found within and between batches (Figure 4 and Supplementary Figure 9) suggest that not all parameters are equally accurate as predictors of developmental stage. We therefore tested how each parameter varies within and between larval batches by plotting the coefficient of variance of each parameter against the developmental stage. As expected, SL and ED shows consistent and significantly lower values than age (Figures 5A, B), suggesting that these parameters are considerably more accurate predictors of developmental stage.
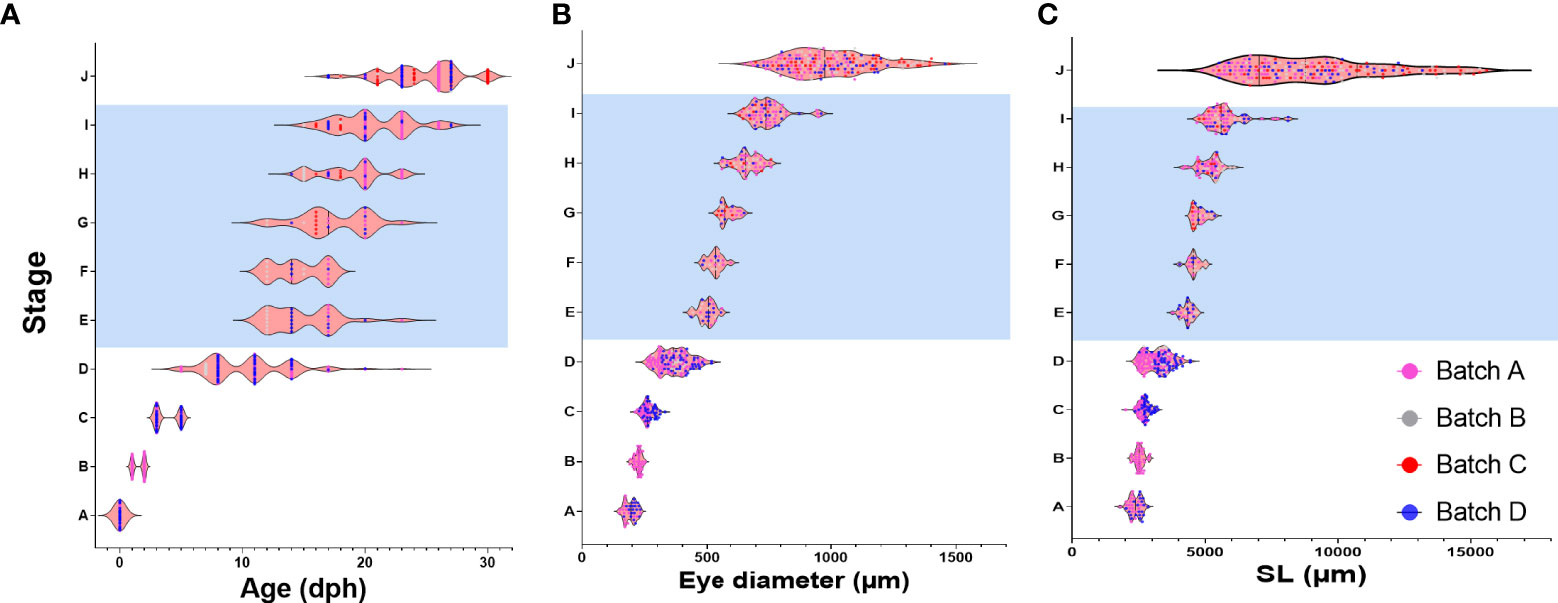
Figure 4 Relationship between age, ED and SL and developmental stage. Graphs depict developmental stage as a function of age (A), eye diameter (B) and standard length (C). Each dot represents a single larva. Dots are color-coded according to the batch of individual larvae. Shaded area marks the metamorphosis period.
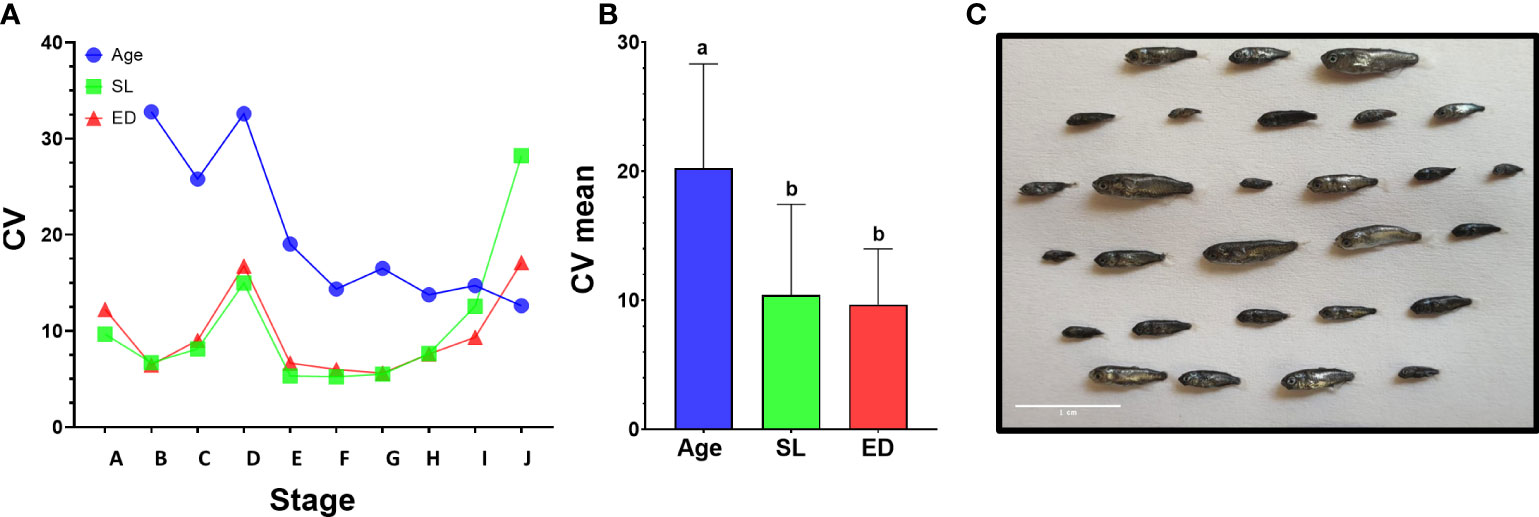
Figure 5 Variance of morphometric parameters during development. (A) Coefficient of variance analysis of age, SL and ED. CV was calculated on larvae from the same developmental stage in batches A and D that encompass the entire larval period. In stages A to I, age shows higher CV values than SL or ED. (B) Comparison of mean CV for all stages using Tukey-Kramer hsd. Different letters mark means which differ significantly (α=0.05). (C) Size variation of mullet larvae at 26 dph (batch A). Scale bar-1 cm.
Notably, CV values peak in all parameters at stage D, just before the onset of metamorphosis, and then drop sharply as the larvae begin metamorphosis at stage E (Figures 4 and 5A). This reflects the fact that stage D is a relatively long stage in which the larvae invest mostly in somatic growth rather than in the acquisition of new morphological features. It also suggests that in order to begin metamorphosis, the larvae must pass a specific threshold (SL = ~4.3mm, ED = ~0.5mm). Until this threshold is reached, larvae will remain at stage D, regardless of their age. Moreover, the CV values remain small throughout metamorphosis (stages E to I), indicating that these stages are also gated by specific size thresholds. CV values rise at reaching the juvenile stage (stage J) as the fish age and grow without adding new developmental features. The wide variation in age at the onset of metamorphosis, coupled with the increased growth rates of the metamorphosis period, amplifies the differences in size of the fish and leads to a wide range of growth trajectories within batches (Figure 5C).
Discussion
In recent years, much effort has been invested in the domestication of the grey mullet as an important aquaculture target species (https://www.diversifyfish.eu/). This effort has resulted in the development of captive breeding technologies (Aizen et al., 2005; Besbes et al., 2020; Ramos-Júdez et al., 2022), genomic and transcriptomic resources (Dor et al., 2020; Curzon et al., 2021) and increased production of captive bred mullet fry (Besbes et al., 2020; Vallainc et al., 2021). Yet, despite these advancements, larval rearing remains a significant hurdle that limits the numbers of captive fry produced and therefore restricts the expansion of mullet aquaculture.
As a step towards resolving this bottleneck, we generated a detailed developmental atlas for the flathead grey mullet under captive conditions. Our staging system largely correspond to the mullet staging table developed by Thieme and co-authors (Thieme et al., 2021), yet we defined an additional stage of metamorphosis (stage F), that is characterized by mid-flexion and the beginning of anal fin ray formation. This resource can now be used to follow development in larval batches and precisely identify developmental delays in real time, allowing hatcheries to evaluate their advancement and treat underlying causes.
As in other larval fish species, we found that in the grey mullet opening of the mouth occurs before the full absorption of the yolk sac and oil globule, providing an overlap between the maternally-supplied nutrition and external feeding. This overlap ensures adequate nutrition as the larvae develop and perfect their hunting skills during the first days of external feeding (Rønnestad et al., 2013). Fish larvae are often visual hunters and therefore, the development of visual sensing is crucial for feeding, orientation and predator avoidance (Semmelhack et al., 2014; Förster et al., 2020). In many teleosts, the larvae hatch with unpigmented and non-functional eyes and the development of the eye correlates with the beginning of exogenous feeding (Hubbs and Blaxter, 1986). In grey mullet, vision begins with the appearance of first cones and correlates with mouth opening (Loi et al., 2020). At the same developmental stage, the olfactory and the optic lobes are formed and are important for the processing of the visual and olfactory cues to enable prey identification and capture (Hubbs and Blaxter, 1986; Semmelhack et al., 2014; Förster et al., 2020; Gajbhiye et al., 2022). Unlike bass larvae, that can feed in early life stages in low light levels (MacIntosh and Duston, 2007; Gajbhiye et al., 2022), mullet larvae, in indoor rearing conditions, require continuous light in order to identify and capture live prey (Loi et al., 2020). With the development of the first retinal rods [17 dph according to (Loi et al., 2020), larvae develop the ability to identify food at lower light conditions and do not require continues light (Loi et al., 2020).
Using our larval developmental atlas, we tested the relationship between several morphometric parameters (age, weight, SL and ED) with larval developmental stage and found that all parameters positively correlate with stage. However, since many biotic (egg quality, genetics, etc.) and abiotic (water temperature, water quality, rearing density, etc.) factors affect the growth and developmental rates of each rearing batch, age is not usually a good universal predictor for developmental stage as it varies widely between batches and conditions (Parichy et al., 2009; Chambers and Leggett, 2011; Kingsford et al., 2022). This phenomenon is also manifested in the high CV scores of age in our data (Figures 5A, B). Therefore, the use of quantitative morphometric parameters, such as SL and ED is considered superior as it can be used to normalize development staging across different rearing conditions (Parichy et al., 2009; Roux et al., 2019; Gajbhiye et al., 2022). Moreover, our data shows that transition between stages is dependent on reaching defined morphometric thresholds as was described for amphibians (Wilbur and Collins, 1973; Day and Rowe, 2002) and fish (Chambers and Leggett, 2011; Kingsford et al., 2022), further supporting the use of SL and ED as better predictors of developmental stage than age.
Our data suggests that in the grey mullet the metamorphosis period is a major modifier of larval development trajectories as growth rates increase dramatically during metamorphosis. This sharp change in growth rates (Figures 3C, 3D) along with the rise in CV values around the onset of metamorphosis, was also observed by others (Besbes et al., 2020; Vallainc et al., 2021; Ramos-Júdez et al., 2022) and marks the metamorphosis period as an important contributor of variation to mullet growth. We show here that larvae enter metamorphosis only after reaching a specific size threshold and that the age at which this threshold is reached varies widely both within and between rearing batches. While a rise in heterogeneity with age was reported in several species (Lugert et al., 2016; Ibarra-Castro et al., 2020; Vallainc et al., 2021; Gajbhiye et al., 2022), the phenomenon is particularly marked in the mullet as their non-cannibalistic nature ensures that developmentally delayed larvae are not removed via predation and therefore are well represented in the population even at later developmental stages. The variation in age at the onset of metamorphosis (stage D), combined with the increased growth rates of the metamorphosis period, generates an enormous size variance in the larval batches. This heterogeneity not only complicates the rearing procedures and decision making for hatcheries which cannot apply a uniform protocol for the batch, but may also cause long term effects on performance of late-metamorphosing fry and increase mortalities as they are moved to weaning.
Taken together, our results imply that future research should focus on understanding the mechanisms that drive mullet metamorphosis. Revealing these drivers will advance our ability to understand the underlying causes for the variation and develop effective treatments to increase the uniformity, improve growth rates and increase survival. Removing the mullet larval rearing bottleneck is expected to open the way for a wide range of aquaculture opportunities that are largely reserved for domesticated species. These include genetic selection programs aimed at improving growth, stress resilience and disease resistance, year-round production of fry for stocking, generation of all-female populations and decreased pressure on wild fish stocks.
Data Availability Statement
The raw data supporting the conclusions of this article will be made available by the authors, without undue reservation.
Ethics Statement
The animal study was reviewed and approved by ARO ethics committee.
Author Contributions
IO, LD, and MG designed the research. IO, DG, and YC-S performed experiments. DG illustrated the larvae. IO, LD, and MG wrote the paper. All authors contributed to the article and approved the submitted version.
Funding
The work of IO was partly funded by the Chief Scientist of the Ministry of Agriculture grant no. 20-04-0008 to MG and LD. DG was partly funded by the India-Israel ARO Postdoctoral Fellowship Program.
Acknowledgments
This study would not have been possible without the generous support of the Dagon marine finfish hatchery in Kibbutz Ma’agan Michael (Israel), which provided the mullet larvae for the project. We thank Boaz Ginzburg, Yoav Magnus, Moti Shlomovich, Itay Taieb, and Gilad Hasson for kindly providing the mullet larvae and for their help during the sampling process.
Conflict of Interest
The authors declare that the research was conducted in the absence of any commercial or financial relationships that could be construed as a potential conflict of interest.
Publisher’s Note
All claims expressed in this article are solely those of the authors and do not necessarily represent those of their affiliated organizations, or those of the publisher, the editors and the reviewers. Any product that may be evaluated in this article, or claim that may be made by its manufacturer, is not guaranteed or endorsed by the publisher.
Supplementary Material
The Supplementary Material for this article can be found online at: https://www.frontiersin.org/articles/10.3389/fmars.2022.967984/full#supplementary-material.
References
Aizen J., Meiri I., Tzchori I., Levavi-Sivan B., Rosenfeld H. (2005). Enhancing spawning in the grey mullet (Mugil cephalus) by removal of dopaminergic inhibition. Gen. Comp. Endocrinol. 142, 212–221. doi: 10.1016/j.ygcen.2005.01.002
Besbes R., Benseddik A. B., Kokokiris L., Changeux T., Hamza A., Kammoun F., et al. (2020). Thicklip (Chelon labrosus) and flathead (Mugil cephalus) grey mullets fry production in Tunisian aquaculture. Aquac. Rep. 17, 100380. doi: 10.1016/J.AQREP.2020.100380
Chambers R. C., Leggett W. C. (2011). Size and age at metamorphosis in marine fishes: An analysis of laboratory-reared winter flounder (Pseudopleutonectes americanus) with a review of variation in other species. Can. J. Fish. Aquat. Sci. 44, 1936–1947. doi: 10.1139/F87-238
Curzon A. Y., Dor L., Shirak A., Meiri-Ashkenazi I., Rosenfeld H., Ron M., et al. (2021). A novel c.1759T>G variant in follicle-stimulating hormone-receptor gene is concordant with male determination in the flathead grey mullet (Mugil cephalus). G3 Genes|Genomes|Genetics 11, 1–10. doi: 10.1093/G3JOURNAL/JKAA044
Day T., Rowe L. (2002). Developmental thresholds and the evolution of reaction norms for age and size at life-history transitions. Am. Nat. 159, 338–350. doi: 10.1086/338989/ASSET/IMAGES/LARGE/FG4.JPEG
De Silva S. S., Wijeyaratne M. J. S. (1977). Studies on the biology of young grey mullet, mugil cephalus l. II. food and feeding. Aquaculture 12, 157–167. doi: 10.1016/0044-8486(77)90183-1
Dor L., Shirak A., Curzon A. Y., Rosenfeld H., Ashkenazi I. M., Nixon O., et al. (2020). Preferential mapping of sex-biased differentially-expressed genes of larvae to the sex-determining region of Flathead grey mullet (Mugil cephalus). Front. Genet. 11. doi: 10.3389/FGENE.2020.00839/BIBTEX
Finucane J. H., Collins L. A., Barger L. E. (1978). Spawning of the striped mullet, mugil cephalus, in the northwestern gulf of Mexico. Northeast Gulf Science 2. doi: 10.18785/negs.0202.11
Förster D., Helmbrecht T. O., Mearns D. S., Jordan L., Mokayes N., Baier H. (2020). Retinotectal circuitry of larval zebrafish is adapted to detection and pursuit of prey. Elife 9, 1–26. doi: 10.7554/ELIFE.58596
Gajbhiye D. S., Oz I., Columbus-shenkar Y. Y., Golan M. (2022). Larval development staging table for hatchery-reared sunshine bass ( morone chrysops ♀ x morone saxatilis ♂ ). Aquaculture 546, 737379. doi: 10.1016/j.aquaculture.2021.737379
Gisbert E., Mozanzadeh M. T., Kotzamanis Y., Estévez A. (2016). Weaning wild flathead grey mullet (Mugil cephalus) fry with diets with different levels of fish meal substitution. Aquaculture 462, 92–100. doi: 10.1016/j.aquaculture.2016.04.035
Hjort J. (1914). Fluctuations in the great fisheries of northern Europe. Rapp. Procés-Verbaux 20 ,1–128.
Hubbs C., Blaxter J. H. S. (1986). Development of sense organs and behaviour of teleost larvae with special reference to feeding and predator avoidance. Trans. Am. Fish. Soc 115, 98–114. doi: 10.1577/1548-8659(1986)115<98:NLFCDO>2.0.CO;2
Ibarra-Castro L., Ochoa-Bojórquez M. O., Sánchez-Téllez J. L., Rojo-Cebreros A. H., Alvarez-Lajonchère L. (2020). A new efficient method for the mass production of juvenile spotted rose snapper lutjanus guttatus. Aquac. Rep. 18, 100550. doi: 10.1016/J.AQREP.2020.100550
Kingsford M. J., Krunes E. A., Hall A. E. (2022). Testing the critical size at settlement hypothesis for two species of coral reef fish. Mar. Ecol. Prog. Ser. 681, 87–101. doi: 10.3354/MEPS13920
Koven W., Gisbert E., Nixon O., Solovyev M. M., Gaon A., Allon G., et al. (2019). The effect of algal turbidity on larval performance and the ontogeny of digestive enzymes in the grey mullet (Mugil cephalus). Comp. Biochem. Physiol. Part A. Mol. Integr. Physiol. 228, 71–80. doi: 10.1016/J.CBPA.2018.11.005
Kuo C. M., Shehadeh Z. H., Nash C. E. (1972). Induced spawning of captive grey mullet (Mugil cephalus l.) females by injection of human chorionic gonadotropin (HCG). Aquaculture 1, 429–432. doi: 10.1016/0044-8486(72)90046-4
Levi A., Belmaker J., Heinisch G., Mozes N., Milstein D., Froiman N., et al. (2018). The collection of mugilidae fingerlings from estuaries – experts’ opinion. Isr. Soc Ecol. Environ. Sci., 38.
Liao I. C. (1975). Experiments on induced breeding of the grey mullet in Taiwan from 1963 to 1973. Aquaculture 6, 31–58. doi: 10.1016/0044-8486(75)90088-5
Loi B., Papadakis I. E., Leggieri F., Giménez Papiol G., Vallainc D. (2020). Ontogeny of the digestive system and eye of reared flathead grey mullet, mugil cephalus (Linnaeus 1758), and evaluation of lipid deposition in the liver according to the feeding protocol. Aquaculture 526, 735386. doi: 10.1016/j.aquaculture.2020.735386
Lovatteli A., Holthus P. F. (2008). Capture based aquaculture - global overview. FAO Fish. Tech. Pap. 508:1–298.
Lugert V., Thaller G., Tetens J., Schulz C., Krieter J. (2016). A review on fish growth calculation: multiple functions in fish production and their specific application. Rev. Aquac. 8, 30–42. doi: 10.1111/RAQ.12071
MacIntosh K. E., Duston J. (2007). Effect of light intensity and eye development on prey capture by larval striped bass morone saxatilis. J. Fish Biol. 71, 725–736. doi: 10.1111/J.1095-8649.2007.01539.X
Mcmenamin S. K., Parichy D. M. (2013). Metamorphosis in teleosts. Curr. Top. Dev. Biol. 103, 127–165. doi: 10.1016/B978-0-12-385979-2.00005-8
Parichy D. M., Elizondo M. R., Mills M. G., Gordon T. N., Engeszer R. E. (2009). Normal table of postembryonic zebrafish development: Staging by externally visible anatomy of the living fish. Dev. Dyn. 238, 2975–3015. doi: 10.1002/dvdy.22113
Planas M., Cunha I. (1999). Larviculture of marine fish: problems and perspectives. Aquaculture 177, 171–190. doi: 10.1016/S0044-8486(99)00079-4
Rønnestad I., Yúfera M., Ueberschär B., Ribeiro L., Saele Ø., Boglione C. (2013). Feeding behaviour and digestive physiology in larval fish: current knowledge, and gaps and bottlenecks in research. Rev. Aquac. 5, S59–S98. doi: 10.1111/raq.12010
Ramos-Júdez S., Giménez I., Gumbau-Pous J., Arnold-Cruañes L. S., Estévez A., Duncan N. (2022). Recombinant fsh and lh therapy for spawning induction of previtellogenic and early spermatogenic arrested teleost, the flathead grey mullet (Mugil cephalus). Sci. Rep. 12, 1–15. doi: 10.1038/s41598-022-10371-0
Roux N., Salis P., Lambert A., Logeux V., Soulat O., Romans P., et al. (2019). Staging and normal table of postembryonic development of the clownfish (Amphiprion ocellaris). Dev. Dyn. 248, 545–568. doi: 10.1002/dvdy.46
Sadek S., Mires D. (2000). Capture of wild finfish fry in Mediterranean coastal areas and possible impact on aquaculture development and marine genetic resources. lsraeli J. Aquac. 52, 77–88.
Saleh M. (2008). Capture-based aquaculture of mullets in Egypt. Capture-based Aquac. Glob. Overv. FAO Fish. Tech. Pap. No. 508, 109–126.
Schnitzler J. G., Dussenne M., Frédérich B., Das K. (2017). Post-embryonic development of sheepshead minnow cyprinodon variegatus: a staging tool based on externally visible anatomical traits. Ichthyol. Res. 64, 29–36. doi: 10.1007/s10228-016-0534-7
Semmelhack J. L., Donovan J. C., Thiele T. R., Kuehn E., Laurell E., Baier H. (2014). A dedicated visual pathway for prey detection in larval zebrafish. Elife 3, 1–19. doi: 10.7554/ELIFE.04878
Shaalan M., El-Mahdy M., Saleh M., El-Matbouli M. (2018). Aquaculture in Egypt: Insights on the current trends and future perspectives for sustainable development. Rev. Fish. Sci. Aquac. 26, 99–110. doi: 10.1080/23308249.2017.1358696
Shehadeh Z. H., Kuo C.-M., Milisen K. K. (1973). Induced spawning of grey mullet mugil cephalus l. with fractionated salmon pituitary extract. J. Fish Biol. 5, 471–478. doi: 10.1111/J.1095-8649.1973.TB04476.X
Thieme P., Vallainc D., Moritz T. (2021). Postcranial skeletal development of mugil cephalus (Teleostei: Mugiliformes): morphological and life-history implications for mugiliformes. Zool. J. Linn. Soc 192, 1071–1089. doi: 10.1093/zoolinnean/zlaa123
Vallainc D., Concu D., Papiol G. G., Loi B., Leggieri F., Brundu G., et al. (2021). Producing flat-head grey mullet mugil cephalus (Linnaeus 1758) fries in captivity from sexually mature adults collected in sardinian lagoons. Aquac. Rep. 21, 100844. doi: 10.1016/J.AQREP.2021.100844
Wells R. D. S. (1984). The food of the grey mullet (Mugil cephalus l.) in lake waahi and the Waikato river at huntly the food of the grey mullet (Mugil cephalus l.) in lake waahi and the Waikato river at huntly. New Zeal. J. Mar. Freshw. Res. 18, 13–19. doi: 10.1080/00288330.1984.9516024
Whitfield A. K., Panfili J., Durand J.-D. (2012). A global review of the cosmopolitan flathead mullet mugil cephalus Linnaeus 1758 (Teleostei: Mugilidae), with emphasis on the biology, genetics, ecology and fisheries aspects of this apparent species complex. Rev. Fish Biol. Fish. 22, 641–681. doi: 10.1007/S11160-012-9263-9
Keywords: mugil cephalus, metamorphosis, larvae – development, growth rate, developmental atlas
Citation: Oz I, Gajbhiye DS, Columbus-Shenkar YY, David L and Golan M (2022) Non-uniform metamorphosis underlies different development trajectories in hatchery-reared flathead grey mullet (Mugil cephalus). Front. Mar. Sci. 9:967984. doi: 10.3389/fmars.2022.967984
Received: 13 June 2022; Accepted: 01 July 2022;
Published: 01 August 2022.
Edited by:
Miquel Planas, Institute of Marine Research (CSIC), SpainReviewed by:
Alicia Estevez Garcia, IRTA Sant Carles de la Ràpita, SpainDario Vallainc, International Marine Centre Foundation, Italy
Copyright © 2022 Oz, Gajbhiye, Columbus-Shenkar, David and Golan. This is an open-access article distributed under the terms of the Creative Commons Attribution License (CC BY). The use, distribution or reproduction in other forums is permitted, provided the original author(s) and the copyright owner(s) are credited and that the original publication in this journal is cited, in accordance with accepted academic practice. No use, distribution or reproduction is permitted which does not comply with these terms.
*Correspondence: Matan Golan, bWF0YW4uZ29sYW5AbWFpbC5odWppLmFjLmls; bWF0YW5nQHZvbGNhbmkuYWdyaS5nb3YuaWw=