- Key Laboratory of Mariculture & Stock Enhancement in North China’s Sea, Ministry of Agriculture and Rural Affairs, Dalian Ocean University, Dalian, China
Improving the aquaculture production efficiency by appropriate diets is an essential approach to meeting the increasing market demand for sea cucumbers. The feces of sea urchins, which contains various enzymes and microorganisms, is a potentially cost-effective food for sea cucumbers. To assess the usability of the fecal diet, a five-week laboratory simulation is conducted to investigate behaviors, digestion ability, growth and resistance ability of the sea cucumber Apostichopus japonicus fed with fecal diet at water temperatures of 15°C and 5°C. In the present study, A. japonicus fed with fecal diet shows an obvious preference to fecal diet rather than prepared feed at water temperatures of both 15°C and 5°C, which suggests that the feces is an applicable diet for A. japonicus. Furthermore, small A. japonicus fed with feces (group F) shows significant advantages in intestinal community richness, community diversity and intestine protease activity to A. japonicus fed with prepared feed (group S) at 15°C. These results indicate that the fecal diet provides benefits to digestion ability of small A. japonicus at 15°C. Weight gaining rate is significantly higher in the A. japonicus fed with feces than that in A. japonicus that were fed with feed or not fed with food (group C), which suggests that the direct improvement of the production efficiency at 15°C. The advantages in intestinal bacteria, protease activity, and growth are consistently found in group F compared with group S at 5°C. In addition, the composition of intestinal bacteria indicates that sea cucumbers may inherit the intestinal bacteria of sea urchins through fecal consumption. This suggests that the fecal diet enhances the digestion ability and enzyme activity at low water temperature and thus improves the growth of sea cucumbers. Furthermore, sea cucumbers fed with sea urchin feces have the highest survival rate among the three groups in exposure to an acute salinity decrease at both 5 and 15°C, indicating a better resistance to low salinity. This provides a new insight into the geographical expansion to low-salinity areas in sea cucumber aquaculture. In conclusion, the present study suggests that sea urchin feces have a great potential for the application in improving the production efficiency of sea cucumber aquaculture.
Introduction
The sea cucumber Apostichopus japonicus is one of the primarily commercialized marine species with high output value and profit in China (Hair et al., 2012; Han et al., 2016). However, the production of A. japonicus does not meet the increasing market demand (Zhang et al., 2015). Therefore, it is essential to increase the production efficiency of A. japonicus aquaculture (An et al., 2007; Yuan et al., 2010).
Seed culture is one of the most important stages in aquaculture of A. japonicus (Chen, 2003; Yang et al., 2004). Small A. japonicus normally stops feeding when water temperature drops to ~5°C in winter (Chen, 2004). The fasting period lasts two months each year, which causes long-term growth retardation in winters in Northern China (Gao et al., 2009; Liu, 2015). Aqua-farmers thus raise the water temperature by burning coal. The method, however, is neither economically nor environmentally friendly. Therefore, it is valuable to develop a diet for overwintering without the need to raise water temperature. Furthermore, A. japonicus generally feed and grow most efficiently at the optimum temperature of 15°C (Yang et al., 2005). Meanwhile, prepared feeds are very expensive (Jiang et al., 2017), accounting for 50% of the total culture cost (Wang, 2015). Therefore, a cost-effective diet is essential for improving the production efficiency of sea cucumber aquaculture.
The feces of sea urchins is an inexpensive natural food source for deposit-feeders (e.g., A. japonicus) (Newell, 1965) Frankenberg & Smith, 1967; Sauchyn & Scheibling, 2009). It is mainly the aggregation of relatively unprocessed vegetative material (e.g., algae) coated in mucus (Peduzzi & Herndl, 1986; Yoon et al., 1996; Povero et al., 2003). The key nutritional values are even higher than the original algal tissues (Dethier et al., 2019). Thus, the feces of sea urchins probably meets the nutritive needs of small A. japonicus (Sui, 1988; Yang et al., 2004). Furthermore, the digestion ability of A. japonicus greatly depends on intestinal microorganisms and digestive enzymes (Jiang et al., 2007; Liu et al., 2013; Wang, 2015; Wang et al., 2016) because of the simple intestinal structure (Yang et al., 2004). The feces is rich in bacteria, protozoa, and enzymes (Robertson, 1982; González & Biddanda, 1990; Thor et al., 2003). Therefore, it probably has the advantage in improving the digestion ability of A. japonicus. Since digestion greatly affects growth (Yang et al., 2004), fecal diet has the great potential to be an alternative food for A. japonicus aquaculture at a water temperature of 15°C. Furthermore, sea cucumbers probably ingest live microorganisms, which enhances enzymatic activity and provides extra benefits (e.g., digestive and resistance benefits) during the feeding process (Moss et al., 2001; Liu et al., 2009; Dethier et al., 2019). The enzyme-rich and microbial-rich feces is even more crucial for improving the digestion ability of sea cucumbers in winter when A. japonicus have poor enzyme activity (Fu, 2004; Gao et al., 2009; Liu et al., 2013).
The present study aims to assess the usability of the fecal diet at water temperatures of 15°C and 5°C in order to improve the production efficiency of A. japonicus aquaculture. We ask: 1) whether the feces of sea urchins is an appreciated diet for A. japonicus, 2) whether the fecal diet improves the growth efficiency of A. japonicus, 3) whether the fecal diet enriches the intestine microbiota and enhances the intestine protease activity of A. japonicus, 4) whether the fecal diet provides resistance benefits to A. japonicus in low salinity at water temperatures of 15°C and 5°C.
Materials and methods
Animals
Small A. japonicus of two sizes (~0.15 g and ~0.5 g) were transported from Dalian Zhuang Yuanhai Ecological Seedling Industry Co., Ltd to the Key Laboratory of Mariculture & Stock Enhancement in the North China’ s Sea, Ministry of Agriculture and Rural Affairs, Dalian Ocean University (121°37′ E, 38°87′ N) and separately cultured at 10°C in two circulating water temperature-control tanks, respectively (length × width × height: 75 × 45 × 40 cm; HXSWT-101, Huixin Co., Dalian, China). Water temperature was increased by 1°C every two days from 10°C to 15°C for the A. japonicus (body weight = 0.16 ± 0.03 g), while water temperature was decreased by 1°C every two days from 10°C to 5°C for A. japonicus (body weight = 0.53 ± 0.12 g). Sea cucumbers were fed with the commercial feed (Anyuan Industrial Co., Ltd) with sea mud (1: 6) for two weeks prior to the feeding trial to make the animals acclimate to the experimental diet and environment.
The sea urchin Strongylocentrotus intermedius were transported from a local aqua-farm to the laboratory (test diameter = 1.38 ± 0.47 cm). To provide fresh feces for sea cucumbers at 15°C and 5°C, sea urchins were randomly collected and cultured in six cylindrical plastic cages (20 cm of diameter, 0.5 cm of mesh size) in two circulating water temperature-control tanks of 15°C and 5°C, respectively (length × width × height: 75 × 45 × 40 cm; HXSWT-101, Huixin Co., Dalian, China). Sea urchins were fed with prepared feed ad libitum . The mesh size of 0.5 cm allowed the feces, while not sea urchins and un-ingested food, to fall to the bottom of the tanks for collection.
There was no major variation in water temperature (5.0 ± 0.5°C, 15.0 ± 0.5°C), salinity (32.5 ± 0.41‰) and pH (8.10 ± 0.11, mean ± SD) during the acclimation period, according to the daily measurement with a YSI probe (YSI Incorporated, OH, USA). The photoperiod was 12D: 12L. The seawater was renewed daily.
Experimental design
Diet was the experimental factor in the following groups: A. japonicus that were not fed (group C), A. japonicus that were fed with the feces of sea urchins (group F), and A. japonicus that were fed with the artificial feed (Anyuan Industrial Co., Ltd, the common food in A. japonicus aquaculture) (group S). Sea cucumbers (body weight = 0.56 ± 0.04 g) were randomly distributed into 18 plastic boxes (length × width × height: 20 × 20 × 15 cm, with fixed shelters on the bottom, Figure 1A). Each box (6 L/tank) was stocked with 20 sea cucumbers. Each diet had six boxes as replicates (N = 6). The water temperature was set at 5 ± 0.5°C using the method of water bath in temperature-controlled tanks (length × width × height: 75 × 45 × 35 cm, HXSWT-101, Huixin Co., Dalian, China). In the seawater at 5°C, the salinity was 32.2–32.5‰, the pH was 8.03–8.26, and dissolved oxygen was 6.2–7.0 mg L−1.
Similarly, 20 sea cucumbers (body weight = 0.16 ± 0.01 g) were put into each experimental plastic box as one replicate, and cultured for five weeks at a water temperature of 15°C. Each group had six boxes as replicates fed with the three different diets (N = 6). The experiment lasted for 5 weeks from December 8, 2020 to January 12, 2021. The seawater in the boxes was aerated and changed every three days. In the seawater at 15°C, the salinity was 31.9–32.2‰, the pH was 7.98–8.42, and dissolved oxygen was 7.0–7.4 mg L−1. The light intensity was 0–5 lx for seawater at both temperatures.
Survival rate
The number of survived sea cucumbers was recorded for all the three groups during the five weeks. Survival rate was calculated as the number of survived individuals divided by the number of all involved sea cucumbers.
Food preference
Food preference was a behavioral experiment to determine which food the sea cucumber prefers after the five-week culture with different diet in seawater at both 15°C and 5°C. Individual sea cucumber was placed in a plastic box with feed at the right side and feces at left side (length × width × height: 17 × 14 × 10 cm, Figure 1B). Each group had six replicates using different sea cucumbers for both 15°C and 5°C (N = 6). We recorded the experiment for an hour using a digital camera (Legria HF20; Canon, Tokyo, Japan). We subsequently calculated the number of grasping times and the staying time in both the feces side and the feed side to determine the food preference of sea cucumbers according to our previous method (Yu et al., 2022).

Figure 1 The conceptual diagrams show the devices for culture (A), for food preference experiment (B), and for the acute salinity decrease treatments (C).
Growth performance
Sea cucumbers were all weighted after one-minute drying time in net using an electric balance (G & G Co., USA) for all the groups at the end of the experiment (N = 6). Weight gain rate was calculated according to the following formula (Wang et al., 2016) WGR (%)=(Wt−W0)/W0×100.
Wt is terminal wet weight of sea cucumbers (g); W0 is initial wet weight of sea cucumbers (g).
Acute salinity decrease treatments
Thirty-two sea cucumbers were randomly selected from each group in seawater at both 15°C and 5°C. The 32 sea cucumbers were equally put into four plastic boxes (length × width × height: 20 × 20 × 15 cm, Figure 1C) for each group at both temperatures (N = 4). Generally, the lethal salinity for A. japonicus is ~15‰ (Sui, 1988; Zhang et al., 2004). Thus, the salinity of acute salinity decrease treatments was decreased from 32.77 ± 0.16‰ to 15.19 ± 0.37‰ within 18 hours at a rate of 1‰ h-1 at 15°C and decreased from 32.53 ± 0.16‰ to 15.05 ± 0.43‰ within 18 hours at 5°C. The number of dead A. japonicus was recorded within 24 hours. Evisceration and autolysis were considered as death. Survival rate was calculated as the number of survived individuals divided by the number of all involved sea cucumbers.
Protease activity
To evaluate the usability of fecal diet in the aquaculture of A. japonicus and understand the effects of fecal diet on digestion ability, we tested the protease activity between sea cucumbers fed with fecal diet and fed with common food in aquaculture. Aseptic instruments were used to dissect the intestines of sea cucumbers. Intestines of two sea cucumbers were pooled for each replicate at 5°C (N = 3), while intestines of three sea cucumbers were pooled for each replicate at 15°C because of the weight requirement of the samples (N = 3) (Wang et al., 2018). The protease activity was determined by using the Protease ELISA Kit (Mlbio, ml560431) (Günay et al., 2020; Zeng et al., 2021). The specific determination method was used according to the instructions of the reagent provided by Bioengineering (Shanghai) Co., LTD.
Intestinal microbiological 16S rRNA gene sequencing
MiSeq high-throughput sequencing technology was used to investigate the intestinal bacterial community structure of A. japonicus fed with prepared feed (group S) and with sea urchin feces (group F) in seawater at both 5°C and 15°C. Sea cucumbers were starved for 24 hours before sampling. Aseptic instruments were used to dissect the intestines of sea cucumbers. For the preparation of 1 mL of intestine samples, the intestines of two sea cucumbers were pooled and mixed as a sample for each of the three replicates for groups F and S at 5°C (N = 3). The intestines of three sea cucumbers were pooled as a mixed sample for each of the three replicates for the two groups at 15°C (N = 3). Then, these pooling samples were thoroughly grounded in a sterile mortar. Genomic DNA was extracted using bacterial DNA extraction kit (Bioengineering (Shanghai) Co., LTD.). High-throughput sequencing was performed using the Illumina MiSeq platform to determine the composition of species of each sample (Yang et al., 2015). The random forest analysis was used to find out which bacteria were responsible for the differences between groups. The post hoc analysis was preformed to compare the abundances in different groups within a species classification. Principal component analysis (PCA) was performed to reveal the relationships among the different samples.
Statistical analysis
Normal distribution and homogeneity of variance were assessed using the Kolmogorov-Smirnov test and Levene test for the WGR, survival rate, the number of grasping times, the staying time in the feces side and the feed side, and the protease activity, respectively.
The number of grasping times of both sides, the staying time in both sides at both 15°C and 5°C were tested by the independent-sample t-test for the three groups, except the grasping times of group C at 5°C. Kruskal-Wallis U test was carried out to compare the survival rate during the whole culturing period among the three groups at both 15°C and 5°C. Mann-Whitney U test was carried out to compare the grasping times of A. japonicus in the two sides of the group C at 5°C and the differences of the WGR between every two groups at both 15°C and 5°C, because the data was non-normal and/or lacked homogeneity in the variance. The protease activity, the OTUs, Chao, ACE, Shannon index, Simpson index, and Coverage for all the groups at both 15°C and 5°C were tested by the independent-sample t-test. Welsh’s t-test corrected with Benjamini-Hochberg FDR for post-hoc multiple comparisons was used to compare the proportion (a possible abundance) of Bacteroidetes among different groups. All data analyses were performed using SPSS 22.0 statistical software. A probability level of P< 0.05 was considered significant.
Results
Food preference
At the water temperature of 5°C, no significant difference between the grasping times of A. japonicus in the two sides was found in sea cucumbers fed with prepared feed (F = 0.040, t = -1.174, P = 0.268). However, sea cucumbers fed with feces had significantly more grasping times when they were in the feces side than the times when they were in the feed side (F = 12.985, t = 2.936, P = 0.022). Sea cucumbers that were not fed had significantly more grasping times when they were in the feed side than the times when they were in the feces side (Mann-Whitney U = 5.000, Z = 2.089, P = 0.037). The sea cucumbers of group F stayed significantly longer in the feces side than the time they stayed in the feed side (F = 0.000, t = 3.234, P = 0.009). The sea cucumbers of groups S and C stayed significantly shorter in the feces side than the time they stayed in the feed side (F = 0.000, t = 3.746, P = 0.004 for group S; F = 0.000, t = 6.543, P < 0.001 for group C).
At the water temperature of 15°C, no significant difference between the grasping times of the two sides was found in group C (F = 0.262, t = 0.525, P = 0.611). Sea cucumbers of group F had significantly more grasping times when they were in the feces side than the times when they were the feed side (F = 0.493, t = 3.673, P = 0.004). Sea cucumbers of group S had significantly more grasping times when they were in the feed side than the times when they were in the feces side (F = 0.800, t = 3.094, P = 0.011). The sea cucumbers of group F stayed significantly longer in the feces side than the time they stayed in the feed side (F = 0.000, t = 3.692, P = 0.004). Sea cucumbers of groups S and C stayed significantly shorter in the feces side than the time they stayed in the feed side (F = 0.000, t = 5.560, P< 0.001 for group S; F = 0.000, t = 5.089, P< 0.001 for group C; Figure 2).
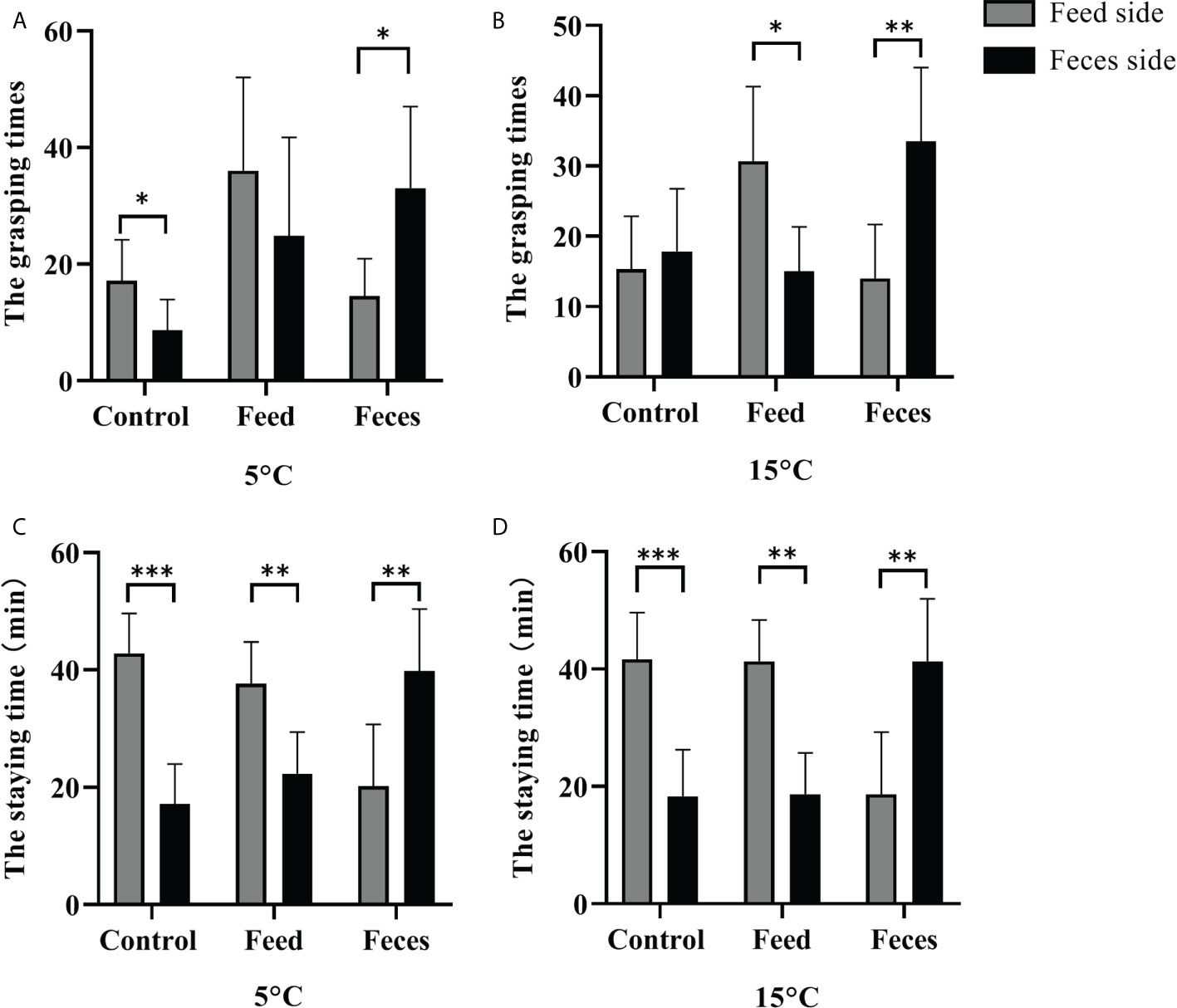
Figure 2 The grasping times of sea cucumbers in the feed side and the feces side at 5°C and at 15°C (mean ± SD, N = 6). The staying time of sea cucumbers in the feces side and the feed side at 5°C and at 15°C (mean ± SD, N = 6). Control, feed, and feces refer to groups C, S, and F, respectively. The asterisks *, ** and ***mean P< 0.05 and P< 0.01 and P< 0.001, respectively.
Survival rate
The sea cucumbers of group C (92.50 ± 6.89%) had significantly lower survival rate than that of sea cucumbers in both group S (100 ± 0.00%, P = 0.007) and group F at the water temperature of 5°C (100 ± 0.00%, P = 0.007). The sea cucumbers of group C (79.17 ± 6.65%) had significantly lower survival rate than that of sea cucumbers in both group S (93.33 ± 5.16%, P = 0.027) and group F at the water temperature of 15°C (98.33 ± 2.58%, P< 0.000; Figure 3).
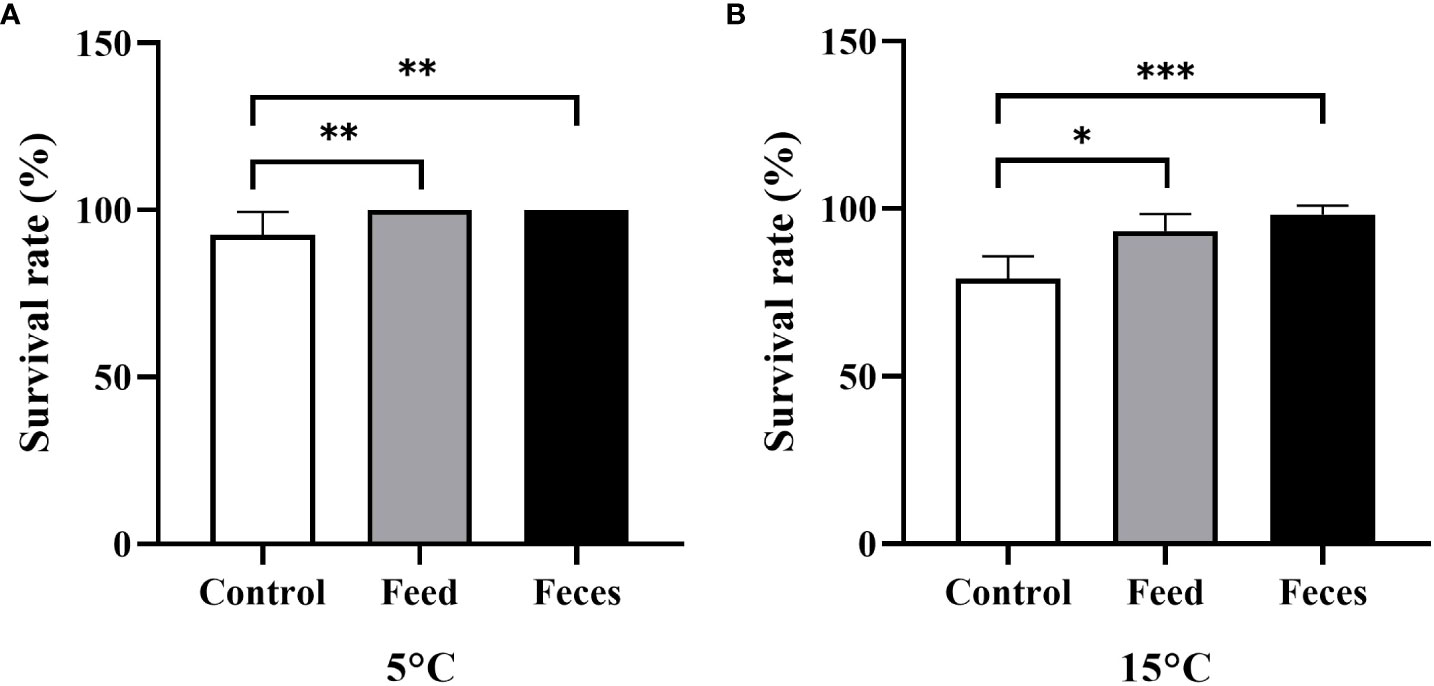
Figure 3 The survival rate of Apostichopus japonicus in the three groups at 5°C (A) and at 15°C (B) (mean ± SD, N = 6). Control, feed, and feces refer to groups C, S, and F, respectively. The asterisks *, ** and *** mean P< 0.05, P< 0.01 and P< 0.001, respectively.
Growth
The WGR of group F (111.15 ± 9.14%) was significantly higher than that in group S (50.62 ± 3.92%, P = 0.002) and that in group C at 5°C (6.23 ± 1.72%, P = 0.002; Figure 4). The sea cucumbers of group S had significantly higher WGR than that of sea cucumbers in group C at 5°C (P = 0.002). The WGR of group F (204.10 ± 33.99%) was significantly higher than that of group S (89.13 ± 15.50%, P = 0.002) and group C at 15°C (-15.28 ± 9.92%, P = 0.002; Figure 4). The WGR of group S was significantly higher than that of group C at 15°C (P = 0.002).
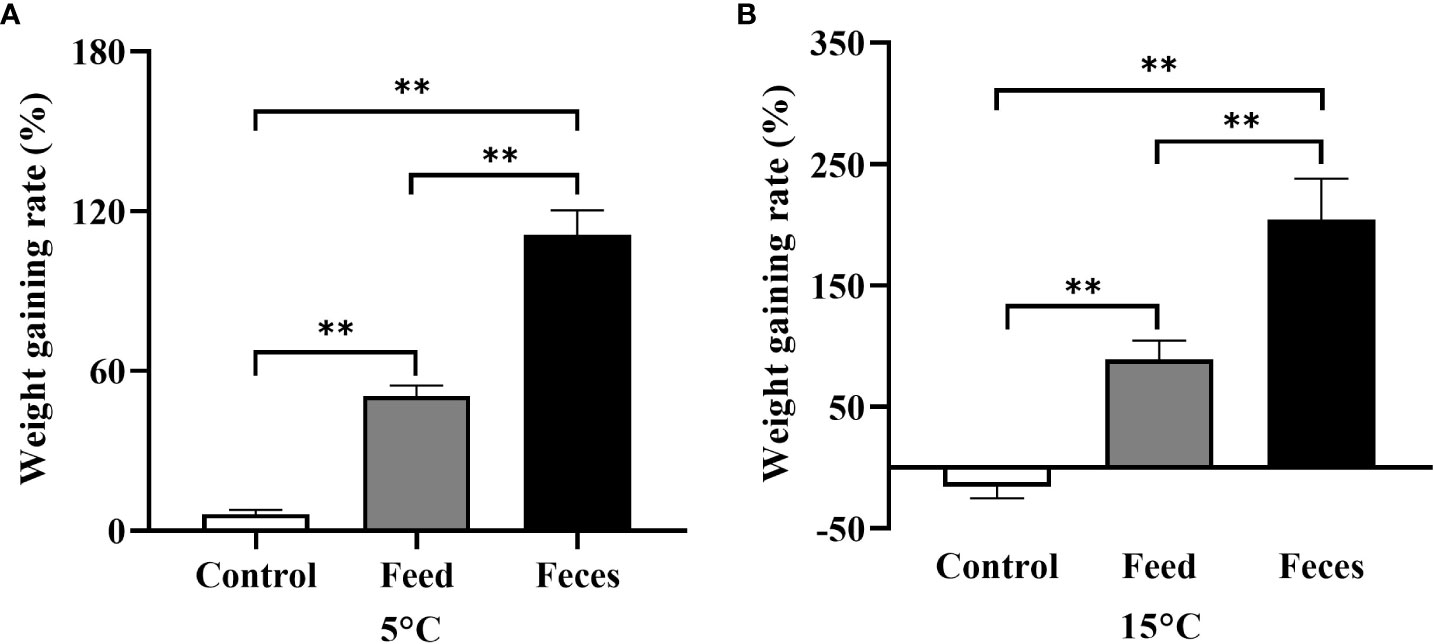
Figure 4 The weight gaining rate (WGR) of Apostichopus japonicus in the three groups at 5°C (A) and at 15°C (B) (mean ± SD, N = 6). Control, feed, and feces refer to groups C, S, and F, respectively. The asterisk ** means P< 0.01.
Microbial composition
At the water temperature of 15°C, small A. japonicus fed with feces had significantly higher OTUs (P = 0.0495) ACE estimator than those fed with feed (P = 0.003, P = 0.024). The sea cucumbers of group F had significantly lower Simpson index and significantly higher Shannon index (P = 0.019, P = 0.025) than those of sea cucumbers in group S. No significant difference of the Simpson index was found between groups S at 5°C and group F at 15°C (P = 0.154; Table. 1). No significant difference of the Good’ s coverage was found between the two groups at 15°C (P = 1.000).
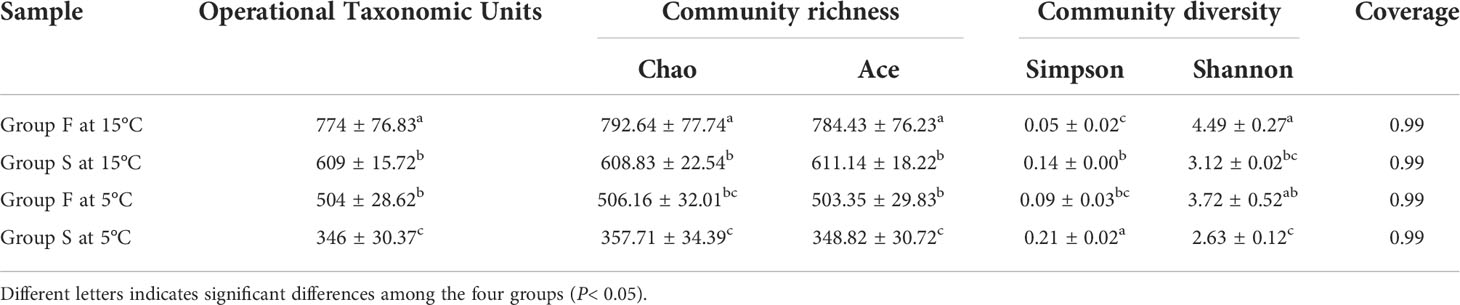
Table 1 Alpha diversity analysis based on operational taxonomic units (OTU) index for groups F and S at 15 and 5°C.
At the water temperature of 5°C, the sea cucumbers fed with feces had significantly higher OTUs (P = 0.003), and ACE estimator than those fed with feed (P = 0.003, P = 0.002). A significantly lower Shannon index and a significantly higher Simpson index were found in group S at 5°C (P = 0.009, P = 0.012) compared with group F at the same temperature (Table. 1). No significant difference of the Good’ s coverage was found between the two groups at 5°C (P = 1.000; Table. 1).
Proteobacteria, Firmicutes, and Actinobacteria predominated in bacterial communities in the groups (Figure 5). Bacteroidetes, Planctomycetes, Gemmatimonadetes, Parcubacteria, and Latescibacteria were the main phylum causing significant differences in between-group variance (Figure 6). Group F at 15°C had significantly higher Bacteroidetes count than group S at both 15°C and 5°C (P = 0.028, P = 0.026), and had no significant difference in Bacteroidetes count from group F at 5°C (P = 0.114; Figure 7).
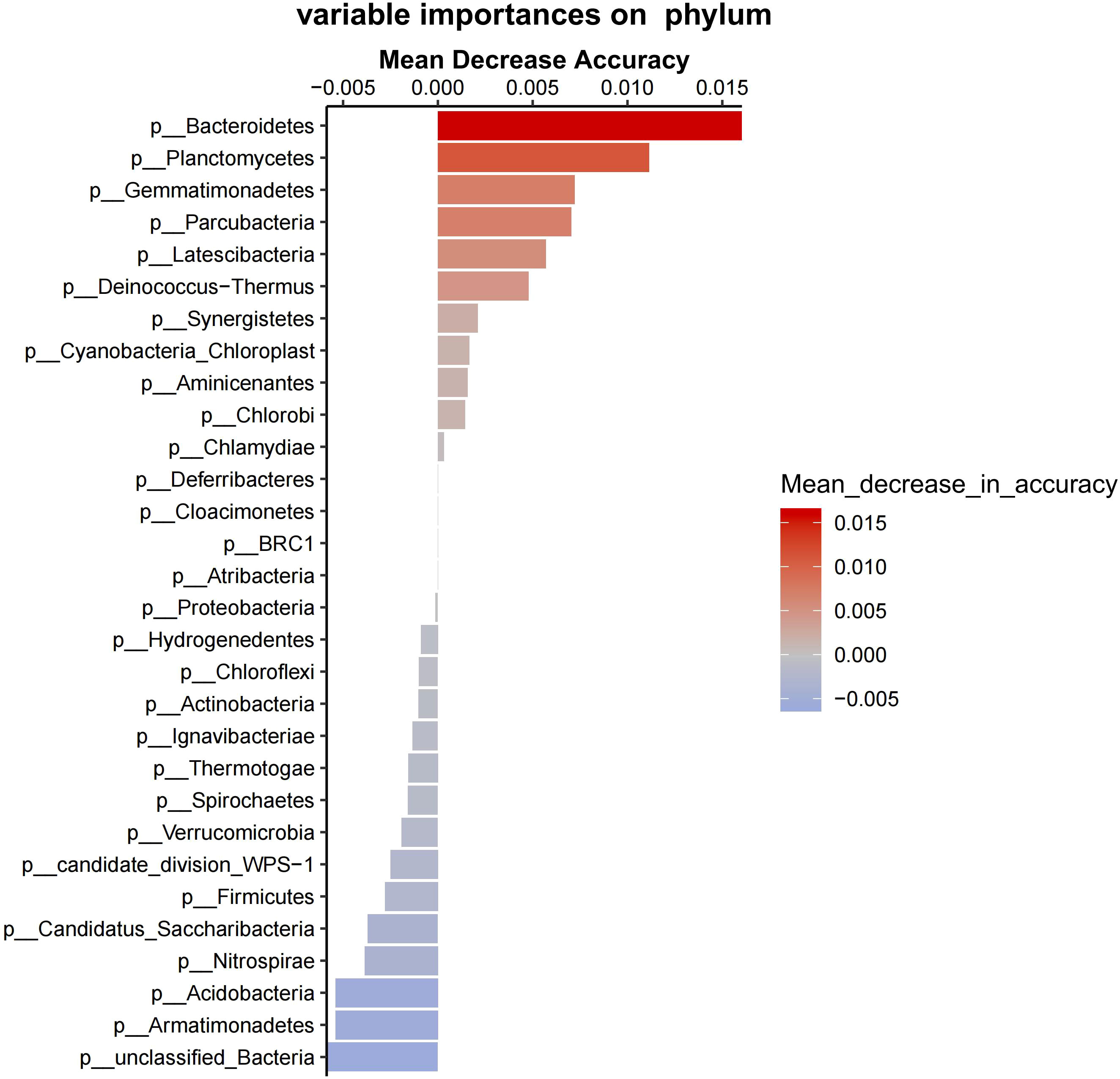
Figure 6 The contribution values of bacterial species to differences between groups. The left side shows the names of different phylum (p means phylum). The accuracy decreases from red to blue (see the right side).
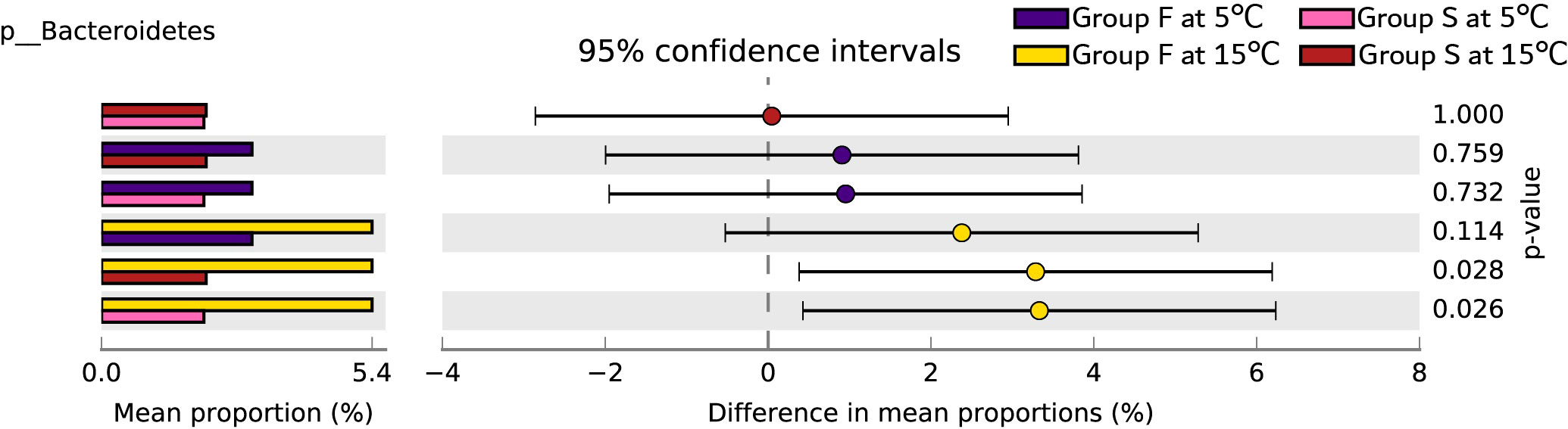
Figure 7 The Post Hoc analysis results of the abundance ratio of Bacteroidetes (phylum) in different groups. Different colors represent different groups (purple for group F at 5°C, pink for group S at 5°C; yellow for group F at 15°C, red for group S at 15°C). Proportion (left side) means a possible abundance of Bacteroidetes. Difference between proportions for each group is indicated by a dot (right side). The q-values are based on Welsh’s t-test and corrected with Benjamini-Hochberg FDR (Phylum).
PCA was performed to reveal the relationships among the different samples. Four groups were distinguished as follows: all the samples of group F and group S at 5°C, all the samples of group F and group S at 15°C (Figure 8). Group F and group S at the water temperature of 5°C were separated distinctly by the first principal component axis (PC1) (P = 0.015), which accounted for 12.24% of the total variations. Consistently, samples of group F and group S at 15°C were also separated distinctly by PC1 (P = 0.002). Furthermore, the second principal component axis (PC2) were accounted for 13.50% of the variance in the bacterial communities. Both the group F samples at 5°C and 15°C (P = 0.049) and both the group S samples at 5°C and 15°C were separated distinctly by PC2 (P = 0.006). Overall, the PC1 and PC2 axes explained 25.74% of the variations between the different bacterial communities.
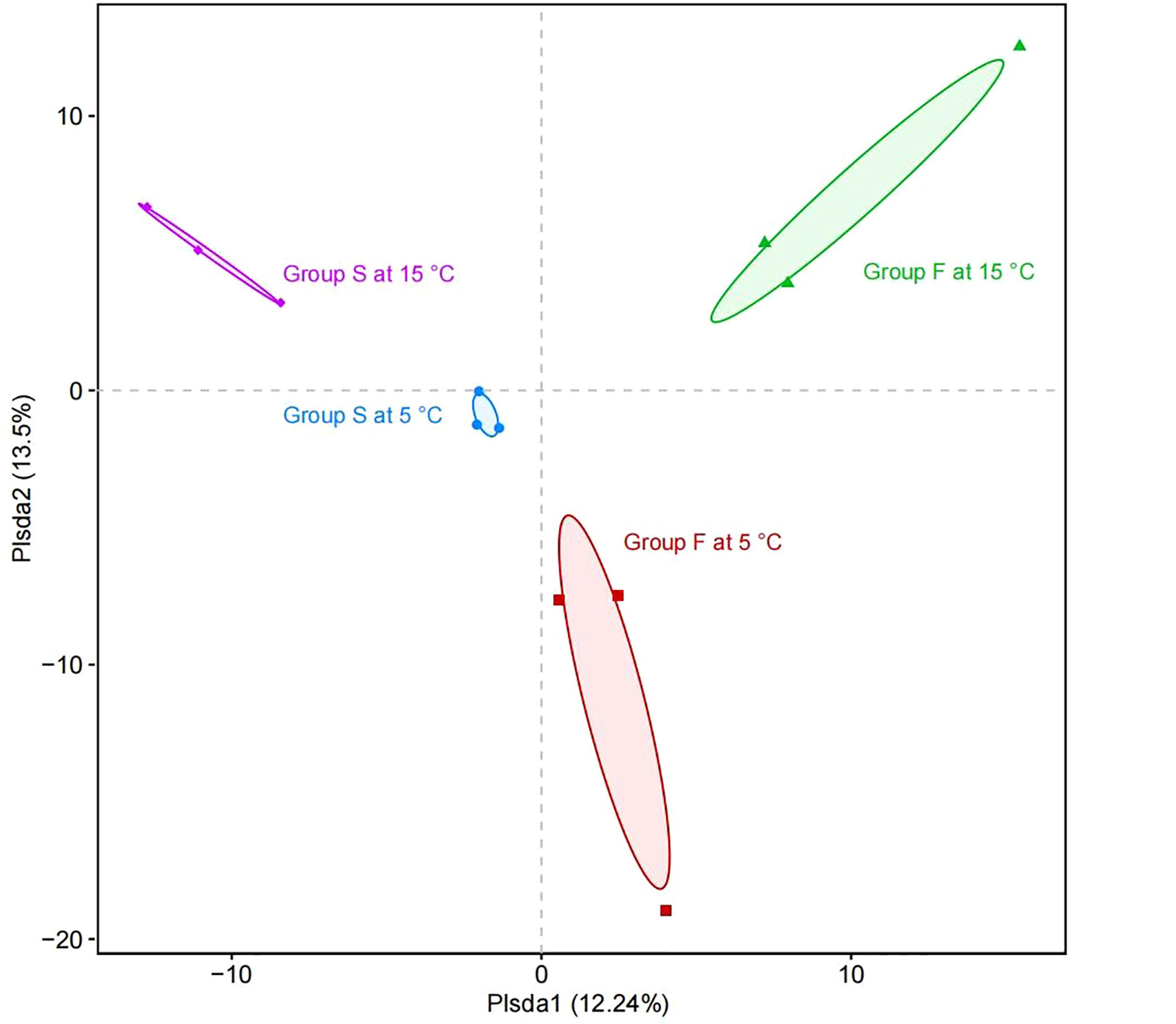
Figure 8 Principal coordinate analysis of the composition of microbial communities. The horizontal axis (PC1) and the vertical axis (PC2) represent the two selected principal component axes. The percentage represents the explanation value of the principal component to the difference in sample composition. Horizontal axis and vertical axis of the scale is the relative distance. Points with different colors or shapes represent the samples of different groups.
Protease activity
Water temperature and diet significantly affected the protease activity (F = 6.105, P< 0.001; F = 4.159, P< 0.001). Sea cucumbers of group S had significantly lower intestine protease activity (511.53 ± 98.03 U mL protein) than those of group F (902.25 ± 36.70 U mL protein) at the water temperature of 5°C (F = 9.232, t = 10.558, P< 0.001; Figure 9A). The protease activity of sea cucumbers of group F (1199.82 ± 101.97 U mL protein) was significantly higher than that of sea cucumbers in group S (997.92 ± 64.76 U mL protein) at the water temperature of 15°C (F = 1.900, t = 4.728, P< 0.001; Figure 9B).
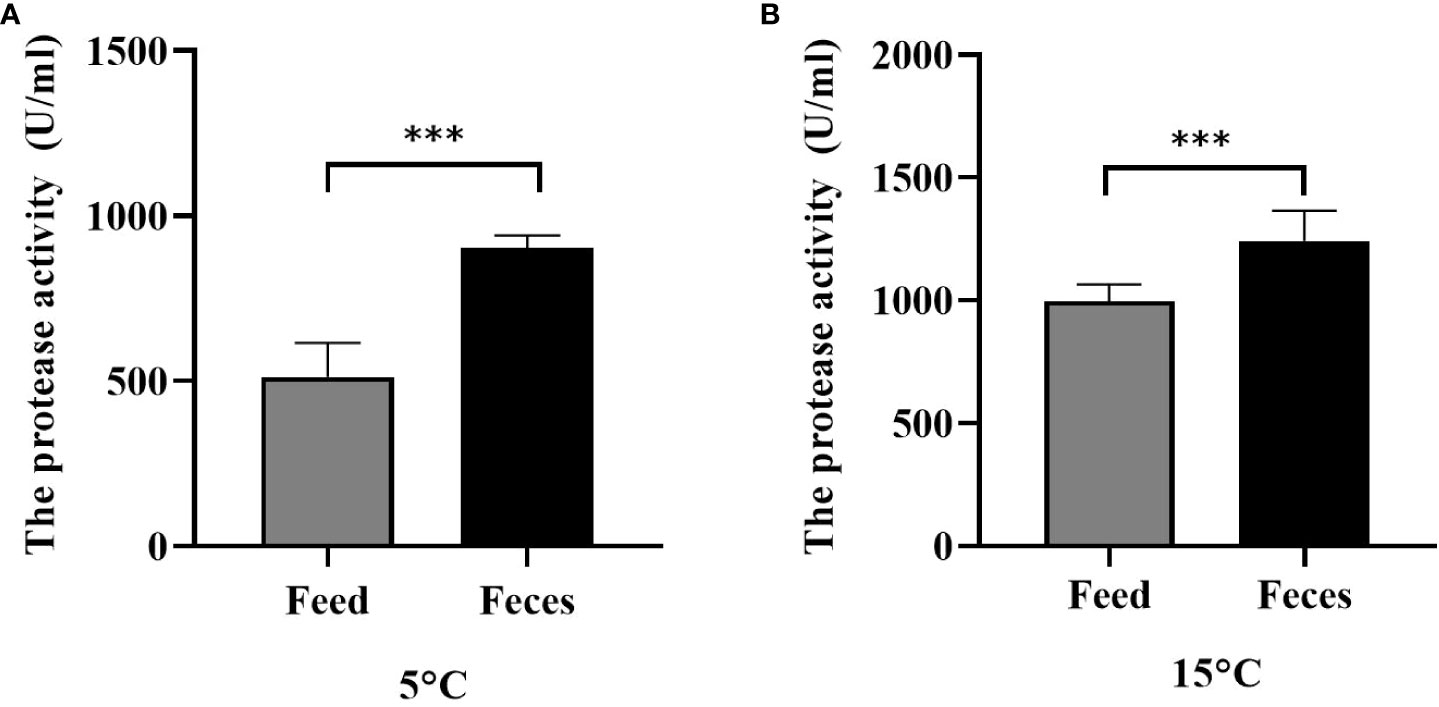
Figure 9 The enteral protease activity of Apostichopus japonicus at 5°C (A) and at 15°C (B) (mean ± SD, N = 3). Feed and feces refer to groups S and F, respectively. The asterisk *** means P< 0.001.
Acute salinity treatments
At the water temperature of 5°C, the survival rate of group F (84.38 ± 11.98%) was significantly higher than that of group C (34.38 ± 25.77%, P = 0.001) and that of group S (40.63 ± 6.25%, P = 0.001). No significant difference in survival rate was found between group S and group C (P = 0.742).
At the water temperature of 15°C, the survival rate of group F (87.50 ± 17.68%) was significantly higher than that of group S (43.75 ± 12.50%, P = 0.002) and that of group C (40.63 ± 6.25%, P = 0.005). No significant difference in survival rate was found between group S and group F (P = 0.611; Figure 10).
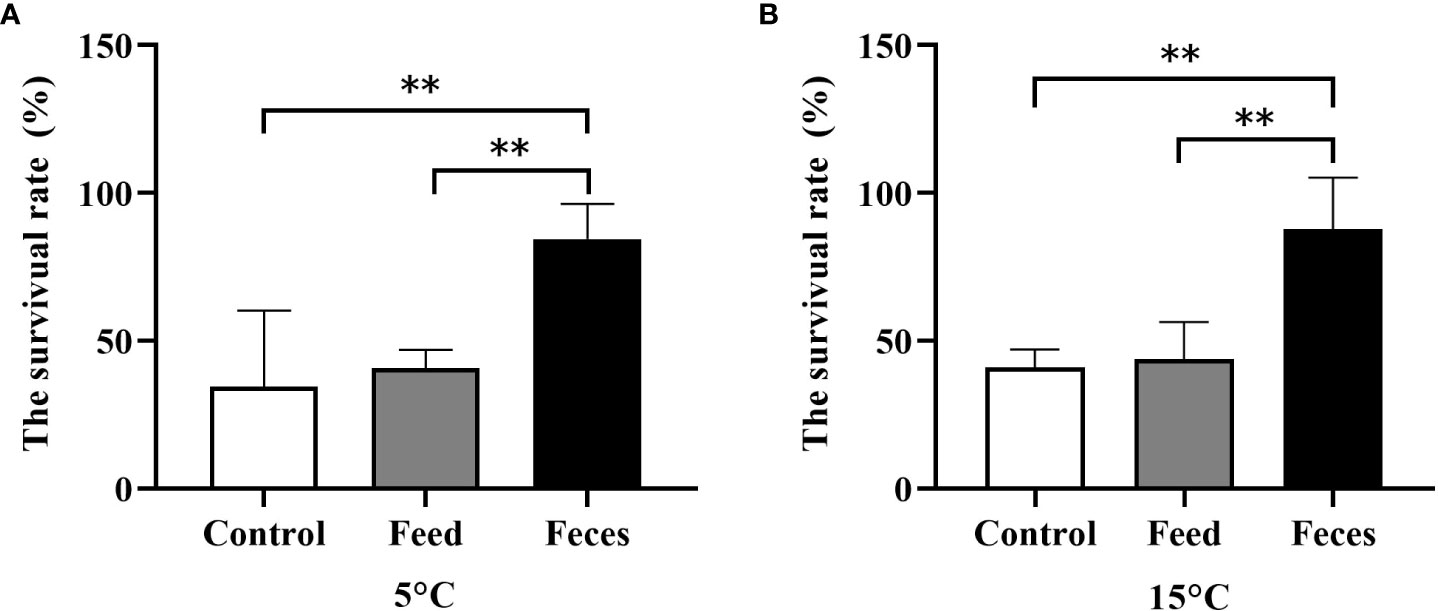
Figure 10 Survival rate of Apostichopus japonicus in three groups exposed to the acute salinity decrease treatments at 5°C (A) and at 15°C (B) (mean ± SD, N = 4). Control, feed, and feces refer to groups C, S, and F, respectively. The asterisk **means P< 0.01.
Discussion
Sea urchin feces is more attractive
Food preference experiment is a useful tool for assessing food usability (Roa, 1992). In the present study, sea cucumbers of group F significantly preferred the feces to the prepared feed at both 5°C and 15°C. However, the sea cucumbers not exposed to feces showed the preference to prepared feed than feces or had no significant preference to feed and feces. Food preference is affected by the nutritional and physiological requirements (Lyons & Scheibling, 2007) and digestive capabilities (Lowe & Lawrence, 1976) of echinoderms. With regular ingestion of natural fresh sea urchin feces that contains readily available live microorganisms, the sea cucumbers intestines possibly became colonized with customized microbial communities to further utilize the remaining nutrients in sea urchin feces (Jensen et al., 2018). Therefore, the feces probably better meets the requirements of digestion and thus is more attractive to small A. japonicus after a short period of aquaculture.
Sea urchin feces improves the survival and growth of A. japonicus
As an ectotherm, A. japonicus have low feeding efficiency, growth rate, and metabolism in winter (Chen, 2004). Sea cucumbers that were not fed had significant lower survival rate than those in groups F and S at 5°C. The present study suggests that failure to feed in winter is associated with the decreased survival rate. Sea cucumbers fed with food (feces or feed) had higher survival rate than that of sea cucumbers in group C at 15°C, which further proves that the potential relationship between death and long-term starvation of small sea cucumbers.
The sea cucumbers fed with the fecal diet had better growth performance than those fed with commercial feed at 15°C. A substantial portion of sea urchin feces is relatively unprocessed vegetative materials colonized by the microbial community (Peduzzi and Herndl, 1986; Yoon et al., 1996; Povero et al., 2003). The nutritional value of these highly digestible feces is probably higher than that of the original diets (Sauchyn and Scheibling, 2009). Consistently, more rapid growth was found in an epibenthic copepod Tigriopus californicus population fed with fecal diet (feces of sea urchins Mesocentrotus franciscanus and Strongylocentrotus droebachiensis who fed with bull kelp) than those fed with bull kelp (Nereocystis luetkeana) (Dethier et al., 2019).
Low water temperature greatly limits the physiological activities of A. japonicus (Yang et al., 2005; Yang et al., 2006; Liu et al., 2013). Hence, the aquaculture of small sea cucumbers suffers a great challenge in winter. Apostichopus japonicus generally starts dormancy at 5°C (Chen, 2004), resulting in feeding cessation, intestine atrophy, decrease of enzyme activity (Tanaka, 1958; Günay et al., 2020) and consequent poor growth (Sui, 1988). The present study found that fecal diet provided ~110% of weight gaining rate for A. japonicus (as twice as the feed diet). Therefore, as an environmentally friendly and cost-effective alternative food, the fecal diet is an efficient approach to improving the production efficiency of sea cucumbers at both the optimal growth temperature and low temperature.
Sea urchin feces enhances the intestinal microbial composition of A. japonicus (α-diversity and β-diversity)
Sea cucumbers do not have specialized physical grinding and chemical digestive organs (Massin, 1982), resulting in poor digestion ability (Yuan et al., 2006). Hence, their digestion and absorption ability highly depend on the intestinal microbiota (Chang et al., 2004; Yang et al., 2004). Whether the diet enhances the intestinal microbial composition is thus important for consideration. In the present study, small A. japonicus fed with feces had significantly higher intestinal bacterial count than those in group S at 15°C, suggesting the fecal diet provides extra microbiota for small sea cucumbers. Since microbiota provides over 70% of energy requirement for A. japonicus (Yang et al., 2004), fecal diet probably provides a high energy supply for small sea cucumbers. Moreover, the intestinal samples of sea cucumbers fed with sea urchin feces showed significantly better community richness (Chao, ACE) and more community diversity (Shannon, Simpson) in intestinal bacteria than that of those that were fed with feed at the optimum temperature. This signifies that fecal diet enhances the intestinal microbial composition of A. japonicus, and thus has the potential to allow small sea cucumbers to achieve the optimal digestibility of nutrients (Wang et al., 2016) and enhance the resilience ability of small sea cucumbers (Li et al., 2009; Thompson et al., 2010).
Since probiotics in food would alter or enhance the digestive ability (Liu et al., 2009; Thompson et al., 2010; Jensen et al., 2018), probiotics is commonly used in aquaculture (Wang et al., 2016). Nevertheless, probiotics serving as allochthonous species showed no significant impact on the composition of fecal microbial community in humans (Bjerg et al., 2014; Hanifi et al., 2014; Laursen et al., 2017). In present study, however, α-diversity analysis of group F showed more OTUs, better community richness (Chao, ACE), and more community diversity (Shannon, Simpson) than that of group S at 5°C. A reasonable explanation is that fecal diet provides a complex microbial ecosystem rather than one or few probiotics. Our results indicate that the fecal diet significantly affects the function composition of the entire microbial community at both 15°C and 5°C. This is a promising method for colonization and regulation of intestinal bacteria. Therefore, the feces of sea urchins is a promising food to provide higher intestinal bacterial count for small A. japonicus and thus enhance the digestion ability of small A. japonicus at both the optimum and low water temperatures. In this way, the aquaculture efficiency is consequently improved.
The two groups fed with different diets at 5°C and two groups fed with different diets at 15°C were both separated by PC1. This suggests that the diet is the main factor leading to the separation of PC1, further proving the ability of transforming microbiome in the fecal diet. Moreover, the sea cucumbers fed with the same diet at different water temperatures were both separated by PC2, suggesting that water temperature is the main factor causing the separation of PC2 due to the reason that different temperatures create different bacterial predominance (Feng et al., 2021). The results of the PCA are consistent with the results of the community richness and community diversity analyses. These analyses indicate that the samples of different diets and water temperatures have different characteristic bacterial communities. The present study clearly indicates that fecal diet significantly influences the intestinal bacteria of sea cucumbers. Similar phenomenon has been documented in a variety of species, indicating that intestinal bacteria shaping mechanisms are evolutionarily conserved (Wilson et al., 2020; Haditomo et al., 2021).
The majority of isolates were affiliated to the phyla Proteobacteria and Firmicutes, according to the molecular identification using 16S rRNA gene sequences. Proteobacteria is the keystone microbe phylum and the main metabolically active microbial populations in the intestine of A. japonicus (Zhang et al., 2012). Allochthonous species could not take dominance in the microbial community (Bjerg et al., 2014; Laursen et al., 2017; Toscano et al., 2017), suggesting that the fecal diet did not enhance the intestinal microbial composition by changing the main dominant colonizing bacteria.
Furthermore, random forest predicted that Bacteroidetes, Planctomycetes, Gemmatimonadetes, Parcubacteria, and Latescibacteria contribute to the uniquely separated microbial ecology in the intestine ecosystem of A. japonicus, which were the main phylum causing significant differences between group variance. Bacteroidetes was the keystone microbe phylum in the intestines of sea urchins, while Planctomycetes was identified in sea urchins fed with macroalgae (Masasa et al., 2021). The finding that Bacteroidetes has significant higher proportion in group F at 15°C than group S at both 15°C and 5°C directly shows that sea cucumbers of group F inherited the intestinal bacteria of sea urchins. Therefore, the fecal diet enhances the intestinal microbial composition of A. japonicus through additional supply of bacteria.
Sea urchin feces improves the protease activity of A. japonicus
Digestion activity is an important indicator for reflecting the nutritional requirements, dietary preference (Boetius & Felbeck, 1995), and digestive physiology of A. japonicus (Yang et al., 2004; Wang et al., 2016). Digestive enzyme activity is closely related to the quality and quantity of nutrients in food (Lee et al., 1984; Wu et al., 2003; Wen et al., 2016). Protease is the most active digestive enzyme in intestine of A. japonicus (Fu, 2004; Jiang et al., 2007). The seasonal pattern of protease activity is that the lowest concentration levels occur in winter, while the highest concentration levels occur at ~15°C (Fu, 2004; Günay et al., 2020). The present study surprisingly found a significantly higher protease activity in sea cucumbers fed with sea urchin feces than that in sea cucumbers in the group S at 5°C. Consistently, the feces significantly improved the growth rate of sea cucumbers at 5°C. This suggests that the feces is not only a valuable food for small sea cucumbers at low temperature, but also an appropriate diet to enhance the enzyme activity and consequently enhance the digestion ability of sea cucumbers in winter. We here encourage aqua-farmers to use the feces as the diet for small A. japonicus without heating the water to achieve appropriate growth, which can reduce economic and environmental costs in winter.
Furthermore, the present study found that sea cucumbers fed with feces had significantly higher intestine protease activity than those of group S at 15°C. It is probably because of the presence of intestinal enzymes of sea urchin feces, which enhances the intestine enzyme activity of sea cucumbers (Mamelona & E´milien, 2005). It can be further explained by the entry of viable bacteria from feces to the intestine of small sea cucumbers, which improves digestive enzyme activities (Sauchyn and Scheibling; 2009); Wang et al., 2016). The opinion is well supported by the result that the better protease activity and the better intestinal microbial composition of A. japonicus (α-diversity and β-diversity) were found in the samples of group F. A higher protease activity reflects a better digestion ability of A. japonicus (Yang et al., 2004; Li, 2013), further proving the feasibility and high developing value of the fecal diet in sea cucumber aquaculture. Considering that enzyme activity is the highest at the optimum temperature for growth and that fecal diet would further increase the growth advantage in this period, we recommend feeding small sea cucumbers with the feces of sea urchins to provide extra digestive benefits at the optimum temperature. Consistently, sea cucumbers had significantly higher growth rate in group F than those of group S at 15°C. This suggests that sea urchin feces probably better meets the need of A. japonicus and results in direct growth benefits for sea cucumbers. Therefore, we recommend that aqua-farmers feed small sea cucumbers with fecal diet to achieve better digestion and growth at the optimal temperature.
Sea urchin feces is an appropriate diet for better resistance to low salinity
Intestinal microbiota plays an important role in the host health because an external organ partakes in nutritional metabolism and affects immunity development of the host (O’Hara and Shanahan, 2006; Wang et al., 2019). Bacterial composition can be affected by environmental and dietary factors (Marques et al., 2010; Zhang et al., 2019). Accordingly, regulating intestinal microbiota is an effective method to improve the health of farmed animals (Chauhan and Singh, 2018). Fecal diet has the potential in aquaculture due to its benefit on homeostasis of intestinal microbiota. Sea cucumber aquaculture continuously expands with the increase of market demands and the decrease of natural resources (Jiang et al., 2017; Li & Hu, 2017; Ciji & Akhtar, 2021). However, the expansion is greatly limited because of the salinity requirements for the survival of A. japonicus (Zhang et al., 2004; Su et al., 2014). In the present study, we found that sea cucumbers fed with sea urchin feces had the highest survival rate among the three groups in exposure to acute salinity decrease at both 15°C and 5°C, indicating a better resistance to low salinity. This novel finding provides a new perspective for the geographical expansion of A. japonicus aquaculture to low salinity areas.
Data availability statement
The datasets presented in this study can be found in online repositories. The data presented in the study are deposited in the NCBI repository, accession number PRJNA859421.
Author contributions
CZ and YY did the investigation, conceived and designed the experiment. YY, YQ, PD, RT, FH, GW performed the experiment. YY, JS performed statistical analysis and visualization. YY, CZ wrote the first draft of the manuscript. CZ reviewed and edited the manuscript. YC provided resources for this study. All authors have read and agreed to the published version of the manuscript.
Funding
This work was funded by the National Natural Science Foundation of China (41506177), a research project for marine economy development in Liaoning province (for Jun Ding), High-level talent support grant for innovation in Dalian (2020RD03), and Liaoning Province “Xingliao Talents Plan” project (XLYC2002107).
Acknowledgments
We appreciate Wei Tang for editorial suggestions.
Conflict of interest
The authors declare that the research was conducted in the absence of any commercial or financial relationships that could be construed as a potential conflict of interest.
Publisher’s note
All claims expressed in this article are solely those of the authors and do not necessarily represent those of their affiliated organizations, or those of the publisher, the editors and the reviewers. Any product that may be evaluated in this article, or claim that may be made by its manufacturer, is not guaranteed or endorsed by the publisher.
References
An Z., Dong Y., Dong S. (2007). Temperature effects on growth-ration relationships of juvenile sea cucumber Apostichopus japonicus (Selenka). Aquaculture 27 (2), 644–648. doi: 10.1016/j.aquaculture.2007.08.038
Bjerg A. T., Sørensen M., Krych L., Hansen L. H., Astrup A., Kristensen M., et al. (2014). The effect of Lactobacillus paracasei subsp. paracasei L. casei W8® on blood levels of triacylglycerol is independent of colonisation. Benef. Microbes 6, 263–269. doi: 10.3920/BM2014.0033
Boetius A., Felbeck H. (1995). Digestive enzymes in marine-invertebrates from hydrothermal vents and other reducing environments. Mar. Biol. 122 (1), 105–113. doi: 10.1007/BF00349283
Chang Y., Yu C., Song X. (2004). “Pond culture of sea cucumbers, apostichopus japonicus, in dalian,” in Advances in Sea cucumber aquaculture and management. Eds. Lovatelli A., Conand C., Purcell S., Uthicke S., Hamel J., Mercier A. (Rome: FAO), 269–272. (In Chinese)
Chauhan A., Singh R. (2018). Probiotics in aquaculture: A promising emerging alternative approach. Symbiosis 77, 99–113. doi: 10.1007/s13199-018-0580-1
Chen J. (2003). Overview of sea cucumber farming and sea ranching practices in China. SPC beche-de-mer Inf. Bull. 18, 18–23.
Chen J. (2004). “Present status and prospects of sea cucumber industry in China,” in Advances in Sea cucumber aquaculture and management. Eds. Lovatelli A., Conand C., Purcell S., Uthicke S., Hamel J., Mercier A. (Rome: FAO), 25–38.
Ciji A., Akhtar M. (2021). Stress management in aquaculture: A review of dietary interventions. Rev. Aquacult. 13 (4), 2190–2247. doi: 10.1111/raq.12565
Dethier M., Hoins G., Kobelt J., Lowed A., Gallowaye A., Schrame J., et al. (2019). Feces as food: The nutritional value of urchin feces and implications for benthic food webs. J. Exp. Mar. Biol. Ecol. 514, 95–102. doi: 10.1016/j.jembe.2019.03.016
Feng J., Zhang L., Tang X., Xia X., Hu W., Zhou P. (2021). Season and geography induced variation in sea cucumber (Stichopus japonicus) nutritional composition and gut microbiota. J. Food Composit. Anal. 101, 103838. doi: 10.1016/j.jfca.2021.103838
Frankenberg D., Smith K. (1967). Coprophagy in marine animals. Limnol. Oceanogr. 12, 443–450. doi: 10.4319/lo.1967.12.3.0443
Fu X. (2004). Study of protease from digestive tract of sea cucumber (Stichopus japonicus). [Master’s thesis] (Qingdao: Ocean Univ. of China).
Gao F., Yang H., Xu Q., Wang F., Wu G. (2009). Effect of water temperature on digestive enzyme activity and intestine mass in sea cucumber Apostichopus japonicus (Selenka), with special reference to aestivation. Chin. J. Ocean. Limnol. 27 (4), 714–722. doi: 10.1007/s00343-009-9202-3
González H., Biddanda B. (1990). Microbial transformation of metazoan (Idotea granulosa) feces. Mar. Biol. 106, 285–295. doi: 10.1007/bf01314812
Günay D., Emiroğlu D., Suzer C. (2020). Seasonal variations of digestive enzymes in sea cucumbers (Holothuria tubulosa, G) under culture conditions. J. Exp. Zool. Part A. 333 (3), 144–150. doi: 10.1002/jez.2336
Haditomo A. H. C., Yonezawa M., Yu J., Mino S., Sakai Y., Sawabe T. (2021). The structure and function of gut microbiomes of two species of sea urchins, Mesocentrotus nudus and Strongylocentrotus intermedius, in japan. front. Mar. Sci. 8. doi: 10.3389/fmars.2021.802754
Hair C., Pickering T., Mill D. (2012). Asia-Pacifific tropical sea cucumber aquaculture, proceedings of an international symposium held in noumea, new Caledonia, 15–17 februar (Noumea, New Caledonia: Australian centre for international agricultural research), 209 pp.
Hanifi A., Culpepper T., Mai V., Anand A., Ford A., Ukhanova M., et al. (2014). Evaluation of Bacillus subtilis R0179 on gastrointestinal viability and general wellness: A randomised, double-blind, placebo-controlled trial in healthy adults. Benefic. Microbes 6, 19–27. doi: 10.3920/BM2014.0031
Han Q., Keesing J., Liu D. (2016). A review of sea cucumber aquaculture, ranching, and stock enhancement in China. Rev. Fish. Sci. Aquac. 24 (4), 326–341. doi: 10.1080/23308249.2016.1193472
Jensen K. E., Taylor J. C., Barry R. J., D’Abramo L., Davis D., Watts S. (2018). The value of sea urchin, Lytechinus variegatus, egesta consumed by shrimp, Litopenaeus vannamei. J. World Aquacult. Soc. 50 (3), 614–621. doi: 10.1111/jwas.12578
Jiang S., Ren Y., Tang B., Li C., Jiang C. (2017). Development status and countermeasures of Apostichopus japonicus culture industry in China. J. Agr. Sci. Tech-Iran 19 (9), 15–23. doi: 10.13304/j.nykjdb.2017.0202
Jiang L., Yang N., Wang W., Wang R., Lu J. (2007). Effects of temperature and pH on the activities of digestive enzyme in Apostichopus japonicus. Oceanol. Limnol. Sin. 38 (5), 476–480. (In Chinese with an English abtract)
Laursen M. F., Laursen R. P., Larnkjær A., Michaelsen K. F., Bahl M. I., Licht T. R. (2017). Administration of two probiotic strains during early childhood does not affect the endogenous gut microbiota composition despite probiotic proliferation. BMC Microbiol. 17, 175. doi: 10.1186/s12866-017-1090-7
Lee P., Smith L., Lawrence A. (1984). Digestive proteases of Penaeus vannamei Boone, relationship between enzyme activity, size and diet. Aquaculture. 42 (3–4), 225–239. doi: 10.1016/0044-8486(84)90103-0
Li X. (2013). Researches on selecting feedstuff and dietary protein requirement of juvenile Apostichopus japonicus. [Master’s thesis] (Yangzhou: Yangzhou University).
Li C., Hu W. (2017). Status, trend and countermeasure in development of sea cucumber Apostichopus japonicus selenka industry in China. Mar. Econo. China 3, 3–20. (In Chinese with an English abstract)
Li J., Tan B., Mai K. (2009). Dietary probiotic Bacillus OJ and isomaltooligosaccharides influence the intestine microbial populations, immune responses and resistance to white spot syndrome virus in shrimp (Litopenaeus vannamei). Aquaculture 291, 35–40. doi: 10.1016/j.aquaculture.2009.03.005
Liu Q. (2015). Characteristics and controlling of water quality during sea cucumber cultivation in the yellow river delta. Hebei Fisheries 3, 11–13. (In Chinese with an English abstract)
Liu C., Chiu C., Ho P., Wang S. (2009). Improvement in the growth performance of white shrimp Litopenaeus vannamei, by a protease-producing probiotic, Bacillus subtilis E20, from natto. J. Appl. Microbiol. 107, 1031–1041. doi: 10.1111/j.1365-2672.2009.04284.x
Liu L., Wang A., Wang Y., Du R., Yang X. (2013). Influence of temperature and body weight on the emptying time of digestive duct and feces quantity of Apostichopus japonicus (Selenka). Mar. Sci. 37 (9), 43–48. (In Chinese with an English abstract)
Lowe E., Lawrence J. (1976). Absorption efficiencies of Lytechinus variegatus (Lamarch) (Echinadermata, echinoidea) for selected marine plants. J. Exp. Mar. Biol. Ecol. 21, 223–234. doi: 10.1016/0022-0981(76)90117-9
Lyons D., Scheibling R. (2007). Effect of dietary history and algal traits on feeding rate and food preference in the green sea urchin Strongylocentrotus droebachiensis. J. Exp. Mar. Biol. Ecol. 349, 194–204. doi: 10.1016/j.jembe.2007.05.012
Mamelona J., E´milien P. (2005). Green urchin as a significant source of fecal particulate organic matter within nearshore benthic ecosystems. Exp. Mar. Biol. Ecol. 314 (2), 163–174. doi: 10.1016/j.jembe.2004.08.026
Marques T. M., Wall R., Ross R. P., Fitzgerald G. F., Ryan C. A., Stanton C. (2010). Programming infant gut microbiota: Influence of dietary and environmental factors. Curr. Opin. Biotech. 21, 149–156. doi: 10.1016/j.copbio.2010.03.020
Masasa M., Kushmaro A., Kramarsky-Winter E., Shpigel M., Barkan R., Golberg A., et al. (2021). Mono-specific algal diets shape microbial networking in the gut of the sea urchin Tripneustes gratilla elatensis. Anim. microbiome 3 (1), 1–21. doi: 10.1186/s42523-021-00140-1
Massin C. (1982). “Echinoderm nutrition,” in Food and feeding mechanisms: Holothuroidea. Eds. Jangou M., Lawrence J.(Rotterdam: Balkema Publ), 43–53.
Moss S., Divakaran S., Kim B. (2001). Stimulating effects of pond water on digestive enzyme activity in the pacific white shrimp Litopenaeus vannamei (Boone). Aquac. Res. 32, 125–131. doi: 10.1046/j.1365-2109.2001.00540.x
Newell R. (1965). The role of detritus in the nutrition of two marine deposit feeders, the prosobranch Hydrobia ulvae and the bivalve Macoma balthica. Proc. Zool. Soc Lond 144, 25–45. doi: 10.1111/j.1469-7998.1965.tb05164.x
O’Hara A. M., Shanahan F. (2006). The gut flora as a forgotten organ. EMBO Rep. 7, 688–693. doi: 10.1038/sj.embor.7400731
Peduzzi P., Herndl G. (1986). Role of bacteria in decomposition of fecal pellets egested by the epiphyte grazing gastropod Gibbula umbilicaris. Mar. Biol. 92, 417–424. doi: 10.1007/BF00392682
Povero P., Misic C., Ossola C., Castellano M., Fabiano M. (2003). The trophic role and ecological implications of oval faecal pellets in Terra Nova bay (Ross Sea). Polar. Biol. 26, 302–310. doi: 10.1007/s00300-003-0485-0
Roa R. (1992). Design and analysis of multiple-choice feeding-preference experiments. Oecologia 89, 509–515. doi: 10.1007/BF00317157
Robertson D. (1982). Fish feces as fish food on a pacific coral reef. Mar. Ecol. Prog. Ser. 7, 253–265. doi: 10.3354/meps007253
Sauchyn L., Scheibling R. (2009). Degradation of sea urchin feces in a rocky subtidal ecosystem: Implications for nutrient cycling and energy flow. Aquac. Biol. 6 (3), 99–108. doi: 10.3354/ab00171
Sui X. (1988). Culture and enhance of sea cucumber (Beijing: China agriculture publishing house), 14–56. (In Chinese)
Su L., Zhou C., Hu L., Xu J. (2014). Development status and sustainable development of Apostichopus japonicus industry in South China. Fish. Sci. Tech. Info. 41 (2), 57–60. (In Chinese with an English abstract)
Tanaka Y. (1958). Feeding and digestive processes of Stichopus japonicus. Bull. Faculty Fisheries Hokkaido Univ. 9, 14–28.
Thompson J., Gregory S., Plummer S., Shields R. J., Rowley A. F. (2010). An in vitro and in vivo assessment of the potential of Vibrio spp. as probiotics for the pacific white shrimp, Litopenaeus vannamei. J. Appl. Microbiol. 109 (4), 1177–1187. doi: 10.1111/j.1365-2672.2010.04743.x
Thor P., Dam H., Rogers D. (2003). Fate of organic carbon released from decomposing copepod fecal pellets in relation to bacterial production and ectoenzymatic activity. Aquat. Microb. Ecol. 33, 279–288. doi: 10.3354/ame033279
Toscano M., De Grandi R., Stronati L., De Vecchi E., Drago L. (2017). Effect of Lactobacillus rhamnosus HN001 and Bifidobacterium longum BB536 on the healthy gut microbiota composition at phyla and species level: A preliminary study. World J. Gastroenterol. 23, 2696. doi: 10.3748/wjg.v23.i15.2696
Wang X. (2015). The effect of microorganism-treated laminaria japonica feedstuff on the growth and immunity of Apostichopus japonicus. [Doctor thesis] (Dalian: Dalian University of Technology).
Wang J., Guo H., Zhang T., Wang H., Liu B., Xiao S. (2016). Growth performance and digestion improvement of juvenile sea cucumber Apostichopus japonicus fed by solid-state fermentation diet. Aquac. Nutr. 23 (6), 1312–1318. doi: 10.1111/anu.12506
Wang Q., Zhang X., Chen M., Zhang P. (2018). Comparison of intestinal microbiota and activities of digestive and immune-related enzymes of sea cucumber Apostichopus japonicus in two habitats. Chin. J. Oceanol. Limn. 36 (3), 990–1001. doi: 10.1007/s00343-018-7075-z
Wang C., Zhou Y., Lv D., Ge Y., Li H., You Y. (2019). Change in the intestinal bacterial community structure associated with environmental microorganisms during the growth of eriocheir sinensis. Microbiol. Open 8, e727. doi: 10.1002/mbo3.727
Wen B., Gao Q., Dong S., Hou Y., Yu H., Li W. (2016). Effect of dietary inclusion of benthic matter on feed utilization, digestive and immune enzyme activities of sea cucumber Apostichopus japonicus (Selenka). Aquaculture 458 (1), 1–7. doi: 10.1016/j.aquaculture.2016.01.028
Wilson A., Koller K. R., Ramaboli M. C., Nesengani L. T., Ocvirk S., Chen C., et al. (2020). Diet and the human gut microbiome: An international review. Dig. Dis. Sci. 65, 723–740. doi: 10.1007/s10620-020-06112-w
Wu Y., Sun J., Zhou Z., Gui Y. (2003). The effects of dietary protein levels on the growth and activities of digestive enzymes in shrimp, Penaeus chinensis. J. Dalian Ocean Univ. 18 (4), 258–262. doi: 10.16535/j.cnki.dlhyxb.2003.04.005
Yang G., Tian X., Dong S., Peng M., Wang D. (2015). Effects of dietary Bacillus cereus G19, B. cereus BC-01 and Paracoccus marcusii DB11 supplementation on the growth, immune response, and expression of immune-related genes in coelomocytes and intestine of the sea cucumber (Apostichopus japonicus selenka). Fish shellfish Immun. 45, 800–807. doi: 10.1016/j.fsi.2015.05.032
Yang H., Yuan X., Zhou Y., Mao Y., Zhang T., Liu Y. (2005). Effects of body size and water temperature on food consumption and growth in the sea cucumber Apostichopus japonicus (Selenka) with special reference to aestivation. Aquac. Res. 36 (11), 1085–1092. doi: 10.1111/j.1365-2109.2005.01325.x
Yang H., Zhou Y., Zhang T. (2004). “Biology of Stichopus japonicus–-Theory and practice,” (Beijing: Science Press). (in Chinese).
Yang H., Zhou Y., Zhang T., Yuan X., Li X., Liu Y., et al. (2006). Metabolic characteristics of sea cucumber Apostichopus japonicus (Selenka) during aestivation. J. Exp. Mar. Biol. Ecol. 330, 505–510. doi: 10.1016/j.jembe.2005.09.010
Yoon W., Marty C., Sylvain D., Nival P. (1996). Degradation of faecal pellets in Pegea confoederata (Salpidae, thaliacea) and its implication in the vertical flux of organic matter. Exp. Mar. Biol. Ecol. 203, 147–177. doi: 10.1016/0022-0981(95)02521-9
Yuan X., Yang H., Wang L., Zhou Y., Gabr H. (2010). Effects of salinity on energy budget in pond cultured sea cucumber Apostichopus japonicus (Selenka) (Echinodermata: Holothuroidea). Aquaculture 306, 348–351. doi: 10.1016/S1872-2032(07)60070-5
Yuan X., Yang H., Zhou Y., Mao Y., Zhang T., Liu Y. (2006). The influence of diets containing dried bivalve feces and/or powdered algae on growth and energy distribution in sea cucumber Apostichopus japonicus (Selenka) (Echinodermata: Holothuroidea). Aquaculture 256, 457–467. doi: 10.1016/j.aquaculture.2006.01.029
Yu Y., Sun J., Zhao Z., Ding P., Yang M., Qiao Y., et al. (2022). Effects of water temperature, age of feces, light intensity and shelter on the consumption of sea urchin feces by the sea cucumber Apostichopus japonicus. Aquaculture 554, 738134. doi: 10.1016/j.aquaculture.2022.738134
Zeng F., Rabbi M., Hu Y., Li Z., Ren X., Han Y., et al. (2021). Synergistic effects of dietary selenomethionine and vitamin c on the immunity, antioxidant status, and intestinal microbiota in sea cucumber (Apostichopus japonicus). Biol. Trace Elem. Res. 199 (10), 3905–3917. doi: 10.1007/s12011-020-02483-3Figurelegends
Zhang X., Nakahara T., Miyazaki M., Nogi Y., Taniyama S., Arakawa O., et al. (2012). Diversity and function of aerobic culturable bacteria in the intestine of the sea cucumber Holothuria leucospilota. J. Gen. Appl. Microbiol. 58 (6), 447–456. doi: 10.1080/09583157.2012.735223
Zhang L., Song X., Hamel J., Mercier A., et al. (2015). “Aquaculture, stock enhancement, and restocking,” in The Sea cucumber Apostichopus japonicus. history, biology and aquaculture. Eds. (Salt Lake City, UT: Academic Press (Elsevier), 289–322.
Zhang Z., Zhang W., Hu Z., Li C., Shao Y., Zhao X., et al. (2019). Environmental factors promote pathogen-induced skin ulceration syndrome outbreak by readjusting the hindgut microbiome of Apostichopus japonicus. Aquaculture 507, 155–163. doi: 10.1016/j.aquaculture.2019.03.054
Keywords: Apostichopus japonicus, sea urchin feces, intestinal bacterial community, protease activity, resistance
Citation: Yu Y, Qiao Y, Ding P, Tian R, Sun J, Hu F, Wu G, Chang Y and Zhao C (2022) Effects of sea urchin feces on behaviors, digestion ability, growth, and resistance of the sea cucumber Apostichopus japonicus. Front. Mar. Sci. 9:967452. doi: 10.3389/fmars.2022.967452
Received: 12 June 2022; Accepted: 25 July 2022;
Published: 26 August 2022.
Edited by:
Chenghua Li, Ningbo University, ChinaReviewed by:
Yanbo Wang, Zhejiang Gongshang University, ChinaZonghe Yu, South China Agricultural University, China
Copyright © 2022 Yu, Qiao, Ding, Tian, Sun, Hu, Wu, Chang and Zhao. This is an open-access article distributed under the terms of the Creative Commons Attribution License (CC BY). The use, distribution or reproduction in other forums is permitted, provided the original author(s) and the copyright owner(s) are credited and that the original publication in this journal is cited, in accordance with accepted academic practice. No use, distribution or reproduction is permitted which does not comply with these terms.
*Correspondence: Chong Zhao, Y2hvbmd6aGFvQGRsb3UuZWR1LmNu