- 1East China Sea Environmental Monitoring Center, State Oceanic Administration, Shanghai, China
- 2Key Laboratory of Marine ecological monitoring and restoration technologies, The Ministry of Natural Resources, Shanghai, China
- 3Yangtze Delta Estuarine Wetland Ecosystem Observation and Research Station, Ministry of Education & Shanghai Science and Technology Committee, Shanghai, China
- 4Key Laboratory of Ocean Space Resource Management Technology, The Ministry of Natural Resources, Hangzhou, China
- 5Marine Academy of Zhejiang Province, Hangzhou, China
Ecological corridors (ECs) are important management tools to protect biodiversity by linking fragile habitats, especially for highly mobile organisms. ECs in terrestrial landscapes work as passages on land or in water. However, the significance of ECs to migratory species in estuaries has not been well elucidated. Based on annual fishery investigation in the Yangtze estuary and their dominance index rank, three of the top five species, including Larimochthys polyactis, Coilia mystus, and Gobiidae, exhibited absolute dominance in spring during the past 5 years. The temporal and spatial density variance of C. mystus supported its short-distance migration pattern. Redundancy analysis and the MaxEnt model predicted optimum habitats for C. mystus. C. mystus larvae survival was significantly related to salinity, total nitrogen, pH, reactive silicate, dissolved oxygen, surface water temperature, and chlorophyll-a in May and to salinity, surface water temperature, permanganate index, suspended particles, total nitrogen, and total phosphorus in August. The MaxEnt model predicted a broader longitudinal distribution range from offshore to the upstream freshwater area but narrower latitudinal distribution in the southern branch in May than in August. Finally, we delineated migratory corridors connecting optimum habitats for C. mystus using the least-cost route method. Optimum habitats close to the coastlines in the south branch might play a significant role in maintaining population or community connectivity in the Yangtze estuary. Our findings provide a perspective and method to quantify and facilitate the harmonious development of socioeconomy and fishery biodiversity conservation.
Introduction
Ecological corridors (ECs) are landscapes linking spatially isolated and dispersed patches in a linear or strip-like distribution, which are constructed for the movement of individuals such as tigers (Seidensticker et al., 2010), fishes (Benson et al., 2007), birds (Klaasen, 1996; Tong et al., 2012), and butterflies (Runge et al., 2015). They also serve as a buffer with certain similarities to habitual kernels benefiting the recovery and adaptation of native species (Lawler et al., 2013; Degteva et al., 2015; McGuire et al., 2016; Keeley et al., 2018). Such landscapes play important roles in sustaining and restoring biodiversity (Hilty et al., 2019) by promoting the flow of energy and materials and genetic exchange in terrestrial environments (Miklos et al., 2019), freshwater systems (Bastian et al., 2015; Hauer et al., 2016), and marine environments (Gillanders et al., 2003; Cowen and Sponaugle, 2009), or a transboundary of the above-mentioned environments. The methods for investigating ECs (Weeks, 2017; Balbar and Metaxas, 2019) have grown steadily during the past several years. Subsequently, more tools are available to project and map ECs in systematic conservation planning, such as the circuit theory (McRae, 2006), graph theory (Urban and Keitt, 2001), least-cost route method (McRae et al., 2016), and individual-based model (Allen et al., 2016). In general, the following aspects are crucial for modeling ECs: the movement behavior of objectives, the physical conditions of their habitats, the degree of disturbances or pressures, and the persistence and adaptation of objectives. To date, global actions have been taken to sustain ecological connectivity by constructing marine protected area networks (Carr et al., 2017). Most of them are based on specific investigating datasets about objectives; for example, specific megafauna or birds migrating across varied jurisdictions, entire passages, or rivers for anadromous or catadromous fish species. However, research on defining the importance of ECs in fishery biodiversity by projecting the dispersion and distribution of model species in estuaries is lacking.
Estuarine ecosystems are located at the ecological intersection between terrestrial and marine ecosystems. They are socioeconomically and ecologically important benefiting from the economic, demographic, natural resource, transportation, and other advantages (Levin et al., 2001; Cowen and Sponaugle, 2009). In recent years, estuarine ecosystems have been considerably altered by global climate change (Gillanders et al., 2011; Scanes et al., 2020) and intense anthropogenic activities (Wang et al., 2022), such as important habitat reclamation, upstream dam construction (Ferguson et al., 2011), overfishing since the 1990s (Wang et al., 2022), spawning and nursing habitat deterioration, and migratory corridor breakdown (Cowen and Sponaugle, 2009). The coupled effects of natural and human activities have altered the temperature, salinity, pH, and nutrients as well as the heterogeneity of seawater characteristics (depending on the mixing process of fresh and salty water) (Pelage et al., 2021). Additionally, it has adversely accelerated the mixing process and promoted the fragmentation of the physical and chemical properties of water columns (Wu and Wu, 2018). Such variance has driven changes in the physiology, morphology, and genetics of marine organisms (Finn, 2007; Johnston and Roberts, 2009; Garcia and Barria de Cao, 2018). For example, ammonia nitrogen and cadmium could be toxic to fish and may decrease their survival rates and biodiversity (Schram et al., 2014). Ocean acidification has changed the preference of catadromous barramundi for tropical estuarine mangroves (Rossi et al., 2018). Spatial heterogeneity in salinity, temperature, and turbidity gradients have increased in estuaries, simultaneously leading to changes in preferred habitats for some marine organisms or different life history stages of the same marine organism. In past decades, some fishes that are highly dependent on estuarine habitats have been decimated; for example, tapertail anchovies (Coilia nasus) migrates from the sea to the river (Jiang et al., 2014), the Yangtze River dolphin migrates in the descending river (Zhu et al., 2009), and Chinese sturgeon (Wang et al., 2018) larvae require the brackish water of the estuary to balance body fluid osmolality.
Migration is a characteristic developed by fishes in the process of phylogeny and a long-term adaptation of fish to the environment, which enables the population to obtain more favorable conditions for survival and reproduction (Planes et al., 2009; Christie et al., 2010; Sykes and Shrimpton, 2010). Anadromous fishes live in the ocean but spawn in the middle or upstream of rivers. In contrast, catadromous fishes live in freshwater and spawn in the ocean. They have strict adaptations to the ecological conditions of their habitat (Hanson et al., 2010; Wang et al., 2012; Wang et al., 2018; Chaparro-Pedraza and de Roos, 2019). Eggs and larvae play an important role in energy transfer in the marine ecosystem, and their survival rate and the amount of remaining stock determine the abundance of population replenishment resources (Botsford et al., 2009; Saenz-Agudelo et al., 2010; D’Aloia et al., 2015). Hence, eggs and larvae are indispensable elements in the study of sustainable use of fish resources and important indicators for evaluating fish migration (Almany et al., 2017). In recent years, the eutrophication of estuarine waters and increase in pollutant discharges have led to a significant decrease in the suitability of estuarine fish habitats. The larval survival rate and species replenishment have continued to decrease, especially for the migratory fishes. Hence, it is essential to identify the migratory pathways of key species in estuaries and to protect marine biodiversity by maintaining the connectivity of organisms and their habitats. In this study, we aimed to (1) construct a theoretical framework for identifying key migratory species in estuaries; (2) test the suitability of the MaxEnt model to predict optimal habitats and construct resistance surfaces for migratory fish species; and (3) delineate or identify ECs as the minimum management space to maintain population connectivity.
Materials and methods
Study site and ichthyoplankton sampling
The Yangtze River Estuary (YRE) is the largest estuary in China, with three-order bifurcations and four outlets for significant volumes of freshwater discharge from the Yangtze River to the East China Sea (Figure 1A). The annual average water temperature is 15°C–16°C. The YRE is a medium-intensity tidal estuary with regular half-day tides outside the mouth and informal half-day shallow tides inside the mouth. From offshore to upstream of the YRE, the duration of flood tide decreases gradually, whereas that of the ebb tide increases and is longer than that of the flood tide. The tidal range of the southern branch of the YRE decreases upstream. The YRE is located in a subtropical monsoon climate zone with oceanic and monsoon characteristics and an annual average precipitation of approximately 1,030 mm, which is mostly concentrated in June and September. A large amount of freshwater carries sediment discharge into the sea, which influences the water quality, hydrologic dynamics, and topography (Wu et al., 2011; Wu et al., 2013). Furthermore, socioeconomic development, including fishing; shipping; and corresponding channel maintenance, pollutant emission, and coastal reclamation, has led to marine ecological degradation and biodiversity extinction through a trophic cascade or habitat destruction.
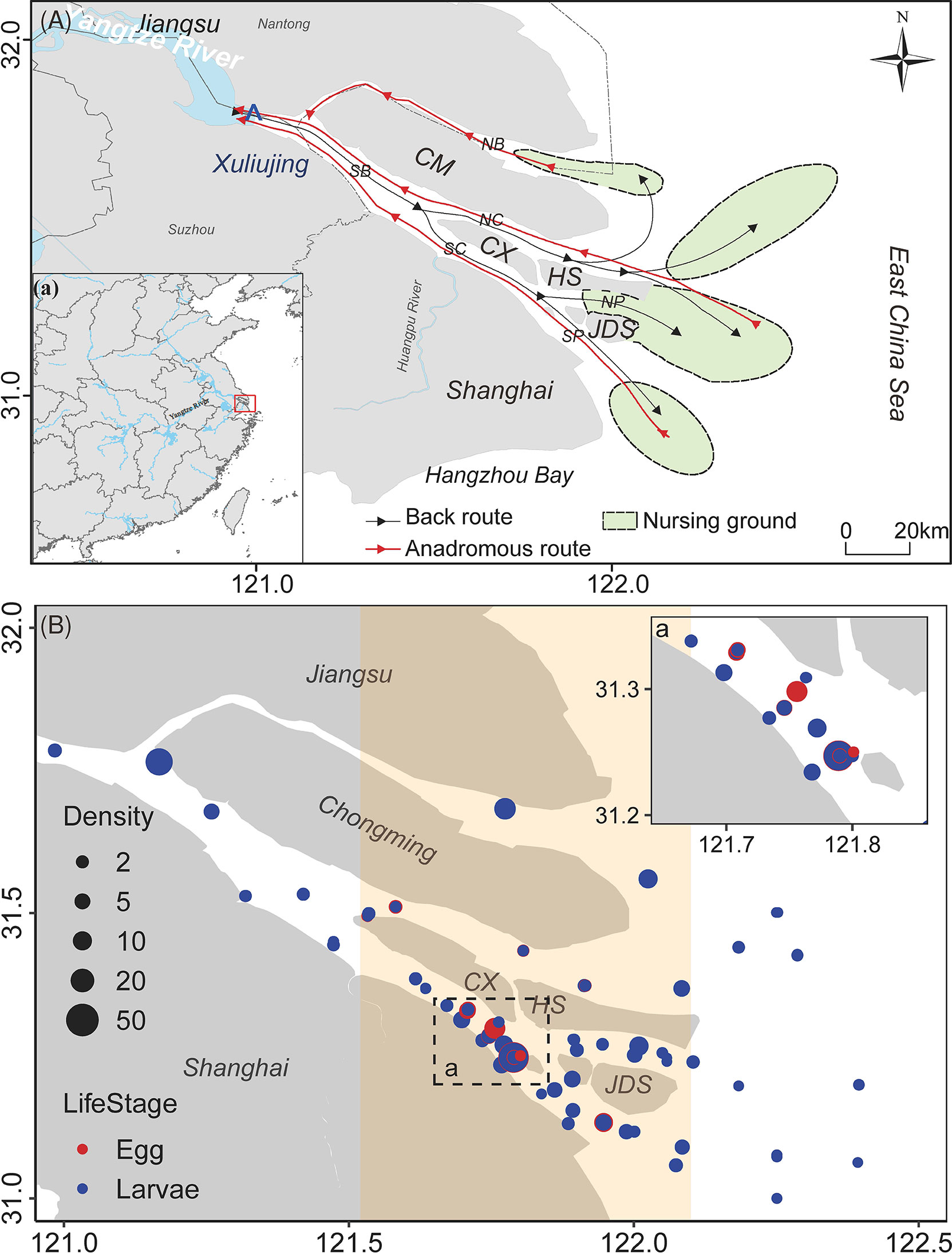
Figure 1 Coilia mystus community at Yangtze estuary. (A) Migration pattern of C mystus; (B) spatial density distribution of C mystus eggs and larvae. Unit of density: ind./m3. NB, north branch; SB, south branch; NC, north channel; SC, south channel; NP, north passage; SP, south passage; CM, Chongming Island; CX, Changxing Island; HS, Hengsha Island; JDS, Jiuduansha Island.
Fish species were surveyed once in spring and summer from 2017 to 2021 during the East China Sea monitoring campaign. In our study area, 65 sites were investigated during each campaign (Figure S1). Ichthyoplankton samples were collected using two kinds of beam trawl: I-trawl and a large plankton trawl. I-trawl is the shallow water type, with a 145 cm length, 50 cm horizontal opening, 0.2 m2 net mouth area, and 0.505 mm screen silk aperture. The I-trawl was trawled once from the bottom to the surface layer at each site. The larvae density of each site was equal to the number of larvae in the sample divided by the volume of the water column, which was defined by the water depth and net mouth area. The large plankton trawl had a net with 280 cm length, 80 cm horizontal opening, 0.5 m2 mouth area, and 0.505 mm screen silk aperture. Larvae density was equal to the number of larvae in the sample divided by the swept area, which was defined by boat speed, trawling time, and the net mouth area. The collected samples were fixed and preserved with a 37% formaldehyde solution. The species were identified and counted using a stereoscopic microscope (Nikon SMZ25, Japan) based on the external morphology of fish eggs and larvae and the individual morphology, tissues, organs, and phylogenetic characteristics at different development stages. The dominance of the main fish species (Y, Li et al., 2022) was calculated using the following equation: Y=ni/N×fi where ni represents the number of individuals of species i, fi is the frequency of species i in each site, and N is the total number of individuals hauled in the campaign. From the five most dominant fish species, only the short-distance migratory species, anchovy (Coilia mystus), was selected for the following analysis.
C. mystus is a short-distance anadromous fish that mainly inhabits coastal shallow water or offshore. Its migratory behavior has been inferred from otolith microchemical analysis (Yang et al., 2006a; Yang et al., 2006b; Jiang et al., 2012; Jiang et al., 2014; Yang et al., 2019; Vu et al., 2022). In general, the breeding period of C. mystus in the Yangtze estuary is from April to September, including the primary spawning season of May and the minor spawning season of August (Hu et al., 2021). In late April, a small number of sexually mature fishes migrate upstream to the brackish water or freshwater areas of the Yangtze estuary to spawn, and some migrate to Zhenjiang, Jiangsu (Zeng and Dong, 1993). The surviving larvae grow in the deep water around Chongming Island and return to the sea in winter (Zeng and Dong, 1993; Yang et al., 2019). Studies on the feeding habits of C. mystus indicated that they mainly fed on crustacean plankton, including Copepods, Mysids, and Decapoda, especially Acanthomysis longirostris, Schmackeria poplesia, Tortanus vermiculus, and Labidocera euchaeta (Liu and Xu, 2011). Compared with long-distance migratory species, the growth and reproduction of C. mystus are more dependent on the marine environment of the Yangtze estuary. Hence, we used C. mystus as the study object to reference the biodiversity conservation of native resident species and short-distance migratory species.
We monitored the environmental factors influencing the community assemblage or distribution of marine fish species. Environmental factors included the concentration of suspended particles (SS), dissolved oxygen (DO), total nitrogen (N), total phosphorus (P), reactive silicate (Si), salinity, estuarine surface water temperature (Temp), pH, permanganate index (CODMn), chlorophyll-a (Chla), and water column depth (depth). Salinity, water temperature, and depth were measured in situ using a conductivity–temperature–depth (CTD) automatic recording analyzer (SBE25plus, Sea-Bird Electronics, Inc. USA). Seawater samples were collected in 5 L bottles from 0.5 m below the surface and at the bottom of each site to measure the other eight physicochemical factors. DO was measured using the iodometric titration method. The water nutrients, including P, Si, and N, were analyzed using an automatic continuous flow analyzer (QuAAtro, SEAL Analytical GmbH, Germany). pH was monitored by a pH meter (JENCO 6010M, JENCO International, Inc. USA). CODMn was measured using the alkaline permanganate method, and Chla was measured using fluorescent spectrophotometry (Agilent Cary Eclipse, Agilent Technologies, Inc. USA). SS samples were filtered by preweighted Whatman GF/F microfiber filters (25 mm) (Gao et al., 2019) and weighted by an electronic scale (QUINTIX224-1CN, Sartorius Aktiengesellschaft, Germany); its concentration was determined using the SS weight divided by the sampling volume.
Predicting potential optimal habitats of migratory species
Maximum entropy (MaxEnt) is a program for modeling species niches and spatial distributions from presence-only species records (Elith et al., 2010). From a set of environmental data and known species occurrence, the model expresses a probability distribution where given grids predict the suitability of conditions for the species by comparing the conditions between present locations and the study area. This model has been widely used in predicting the likely distribution of species in terrestrial (Lamb et al., 2008; Yates et al., 2010) and marine ecosystems (Tittensor et al., 2009; Verbruggen et al., 2009). Considering the complex habitat biogeographical features of C. mystus, the MaxEnt model may be an effective approach to predict their preferred habitats. MaxEnt analysis was performed using the open-source Java software MaxEnt 3.4.1 (Phillips et al., 2006; Phillips et al., 2017). We selected 75% of fishery survey datasets as the training samples to establish the prediction model and the remaining 25% as the test samples to verify the model. Two performance metrics were chosen: (1) the receiver operating characteristic curve, which evaluates the trade-off between prediction sensitivity and specificity and (2) the associated area under the curve, which presents the values of approximately 0.5 representing a random prediction, and values closer to 1 indicate a better performance of the model (Mantas et al., 2022). The analysis was repeated 10 times and averaged to obtain the final simulation results. The prediction results were imported into ArcGIS 10.2 (ESRI, Redlands, California, USA) and converted to a raster format to generate distribution probability prediction maps. Here, we defined the areas with species distribution probability greater than 90% as optimal habitats.
Optimal habitats located at the Yangtze estuarine mouth or offshore were defined as source patches, and those located at upstream freshwater or brackish water area were defined as sink patches. These definitions refer to the two possible behavior patterns of C. mystus. First, C. mystus adults migrate from offshore source habitats to upstream sink habitats to spawn, where the source and sink patches correspond to the feeding ground and spawning ground, respectively. Second, C. mystus egg and larvae have little motor ability, and they drift back and forth with flood and ebb tides between offshore and freshwater areas. Here, source and sink patches represent the origin and destination areas of larvae drifting with the flood tide, respectively.
Delineation of ecological corridors
Ecological resistance refers to the biotic and abiotic factors in a recipient ecosystem that limits population growth (D'Antonio and Thomsen, 2004). Ecological processes such as animals crossing the landscape carry the property of overcoming spatial resistance, which can be measured in terms of accessibility, cost distance, and minimum cumulative resistance. All these measures of resistance can be considered the extensions of the spatial distance concept. In this study, a graph theory algorithm was used to extract animal migration corridors (Pinto and Keitt, 2009). First, the relationship between the model species and the environmental elements was tested using the canonical correspondence analysis, and the explanatory rate values of the environmental factors in the habitats were analyzed. We selected salinity, DO, and surface water temperature with phosphorate concentrations in May and Chla in August to construct a resistance surface. The weights of selected explanatory environmental factors were calculated based on y=(a+b)/2, where a was the predicted contribution value of an environmental factor and b was their explanatory rate in canonical correspondence analysis. Second, kriging interpolation was used to rasterize the point environmental datasets to obtain the resistance surface.
Based on the rasterized resistance surface, the path of cumulative minimum cost distance from the source to the sink patches was generated. The gravity center of source and sink patches were extracted using conventional tools. The cost distance tool was used to calculate the cumulative cost distance from each pixel to the specified source. The cost backlink tool was used to define the direction in which any pixel extends the minimum cost path to the specified source. The cost path tool was used to generate the path with the smallest cumulative cost distance. Finally, 500-m-wide buffer zones were constructed for the theoretical least-cost route to eliminate the spatial redundancy of corridors delineated by the least-cost route method.
Statistical analysis
The nonparametric Kruskal–Wallis test followed by a post hoc comparison was used to analyze the temporal and spatial differences between the average values of life-history characteristics (density, abundance, and maximum and minimum body length) of C. mystus larvae and eggs. Redundancy analysis (RDA) was performed to assess the linear relations between multiple dependent and independent variables in a matrix, which was then incorporated into principal component analysis (Xia, 2020). We used the RDA of life-history characteristics on the environmental factors to extract key factors explaining variations in the investigated sites. This statistical analysis was performed using the statistical software package R (R Core Team, 2014).
Results
Dynamics of fish communities in the Yangtze River Estuary
The YRE is an important spawning and nursery ecosystem for some species. According to the fishery survey of the YRE in May during 2017–2021, this area was dominated by estuarine and marine fishes until 2020; however, freshwater fishes of the family Cyprinidae reached the top five in 2021 (Table 1). Among them, marine fish Larimochthys polyactis, migratory fish C. mystus, and estuarine fish Gobiidae exhibited absolute dominance in spring during the past 5 years. C. mystus larvae or eggs were detected in the Xuliujing hydrological station located upstream of the YRE. Nonetheless, the larvae density around Changxing Island and Jiuduansha Shoal was 2–10 folds higher than offshore and upstream freshwater areas (Figure 1B). Hence, we speculated that C. mystus inhabits coastal or offshore areas and migrates upstream to freshwater or brackish water areas during the breeding season. Their larvae grow around Chongming Island and then migrate back to the sea (Figure 1A). C. mystus has two breeding periods: May is the primary reproductive period, and August is secondary. These life-history characteristics were proven by larger larvae density in May (Wilcoxon test, P < 0.001) than in August. In particular, the density of C. mystus larvae increased significantly from 2020 to 2021 (Figure 2, right panel; Wilcoxon test, P < 0.001), which may benefit fishery production.
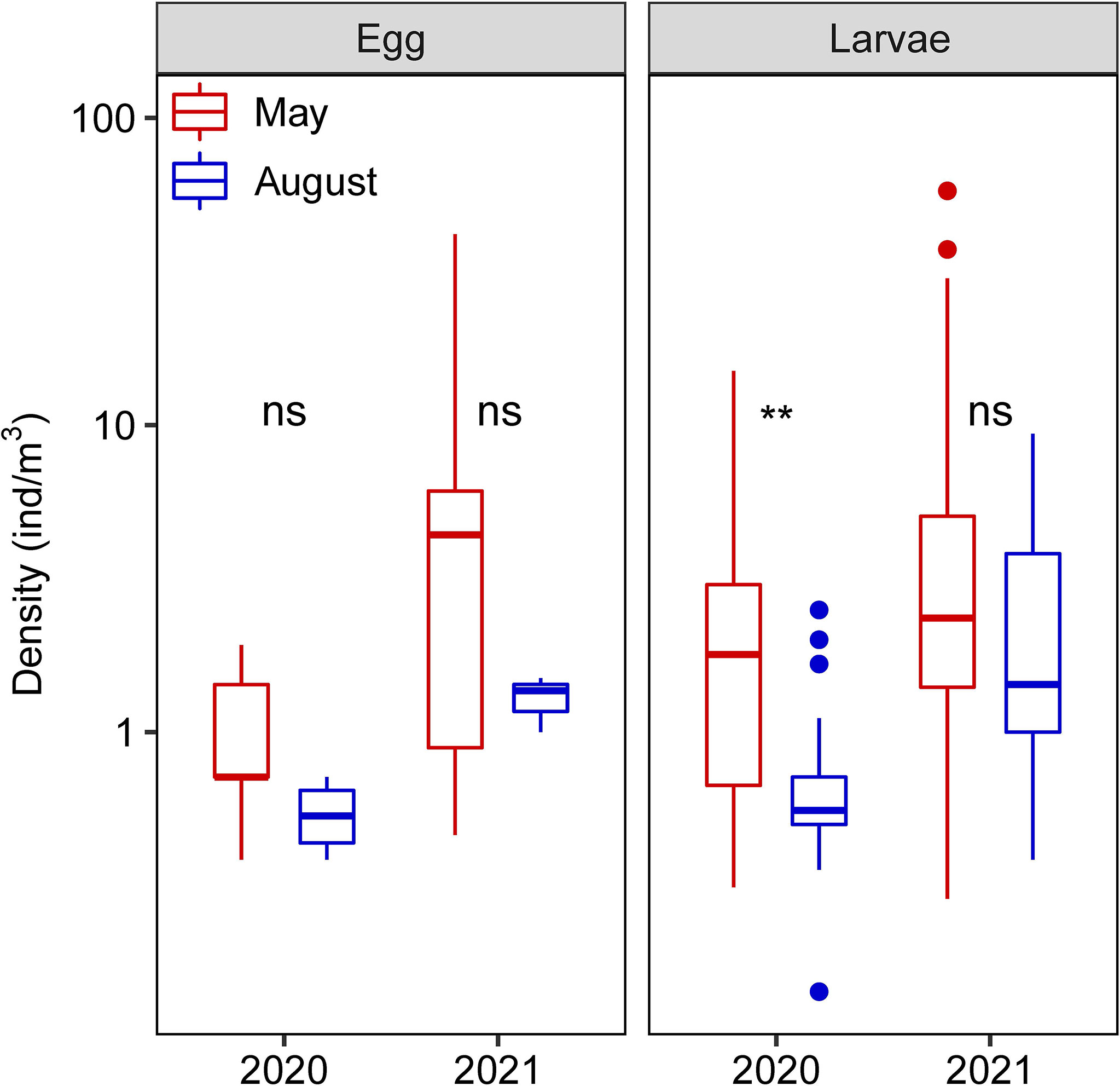
Figure 2 Mean density of C. mystus eggs and larvae in May and August of 2020 and 2021 at the Yangtze estuary. ‘ns’ indicates no significance between compared groups; ** indicates P < 0.01.
Linking life-history characteristics of C. mystus with environmental variables
RDA was used to correlate the life-history characteristics of C. mystus with environmental variables. A total of 11 explanatory variables were considered in this study, including five hydrological variables: Temp, pH, salinity, depth, and DO and six chemical factors: Chla, CODMn, and the contents of N, P, Si, and SS. Forward selection revealed that the correlation between key factors and the life-history characteristics of C. mystus in two breeding seasons varied profoundly (Table S1). In May, 7 of the 11 variables were significant in RDA: salinity, N, pH, Si, DO, surface water temperature, and Chla. However, in August, six variables were significant: salinity, Temp, CODMn, SS, N, and P.
The first and second axes of the RDA plot demonstrated 39.9% and 7.8% variations in the life-history characteristics of C. mystus larvae in May, respectively (Figure 3A; Table S2). The life-history characteristics of C. mystus were positively correlated with Chla, N, Si, P, DO, and surface water temperature, whereas pH and salinity negatively contributed to C. mystus growth. In August, the first and second axes of the RDA plot demonstrated 17.2% and 9.2% variations in the life-history characteristics of C. mystus larvae, respectively (Figure 3B; Table S2). Three chemical factors, P, N, and CODMn, and two physical factors, Temp and SS, were positively correlated with C. mystus growth. Moreover, C. mystus growth was negatively correlated with salinity in May.
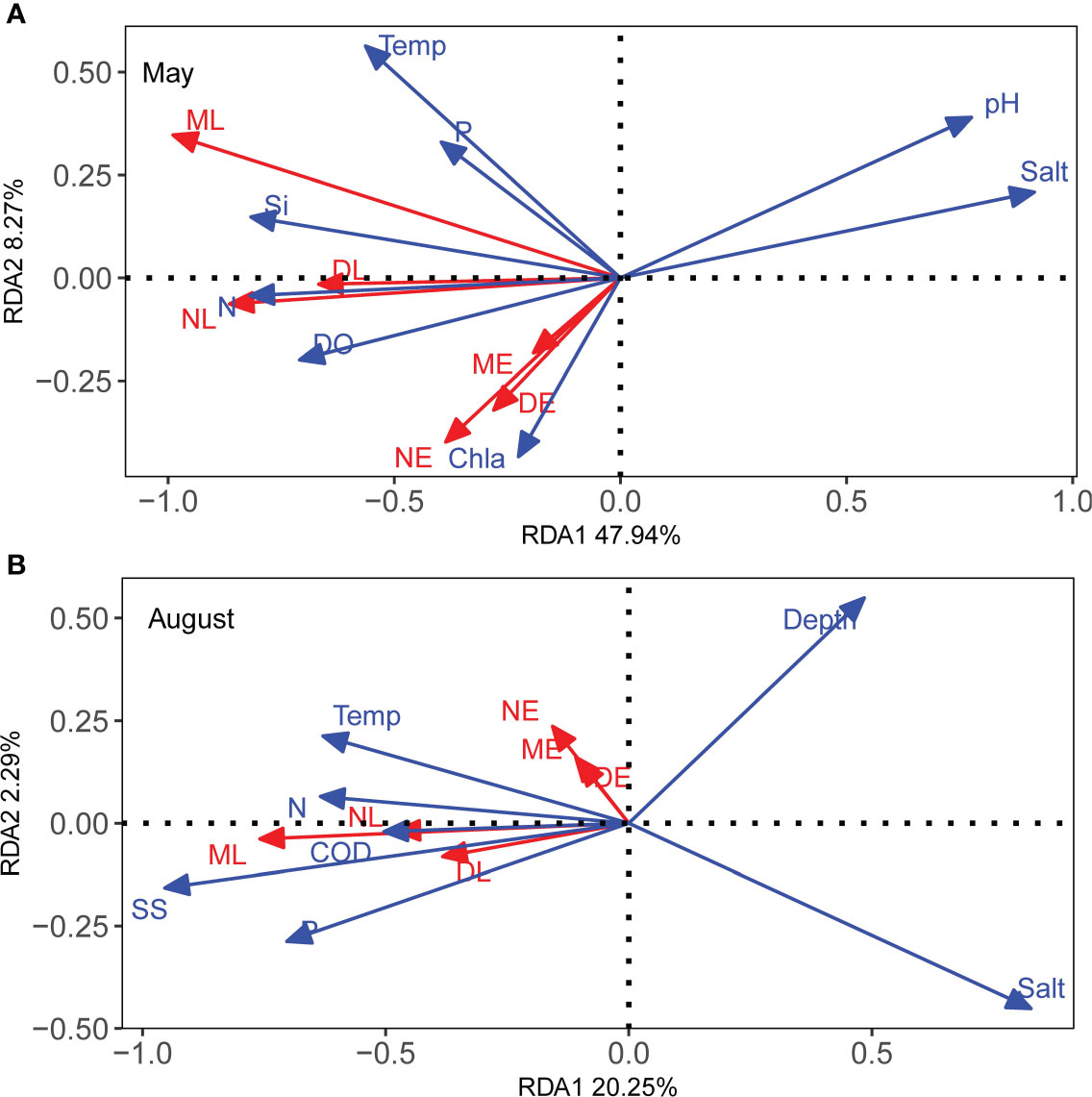
Figure 3 Redundancy analysis of the effect of environmental variables (blue arrows) on the life-history characteristics (red arrows) of C mystus in May (A) and August (B). The response variables include the density, abundance, and mean body length of C mystus larvae (in abbr. DL, NL, and ML, respectively) and egg (in abbr. DE, NE, and ME, respectively). Total variation explained by the redundancy analysis model was 56.21% (A) and 22.54% (B). Si, reactive silicate; N, total nitrogen; P, total phosphorus; SS, suspended particles; Salt, salinity; Temp, temperature of surface water.
Optimal habitats of C. mystus and its migratory corridors in the Yangtze River Estuary
The average area under the curve values of the model training datasets were 0.983 and 0.971 in May and August, respectively (Figures 4A, B), indicating an accurate prediction of the optimal occurrence of C. mystus produced by the MaxEnt model. The predicted C. mystus occurrence hotspots with suitability larger than 0.90 were widely spread in the YRE, where the total area of the hotspots reached 89.9 and 271.2 km2, accounting for 0.41% and 1.53% of the study area in May and August, respectively (Figures 4C, D; Table S3). In May, the predicted occurrence hotspots were mainly concentrated in the south branch (Figure 4C); however, they were almost homogeneously distributed in the south and north branch in August (Figure 4D).
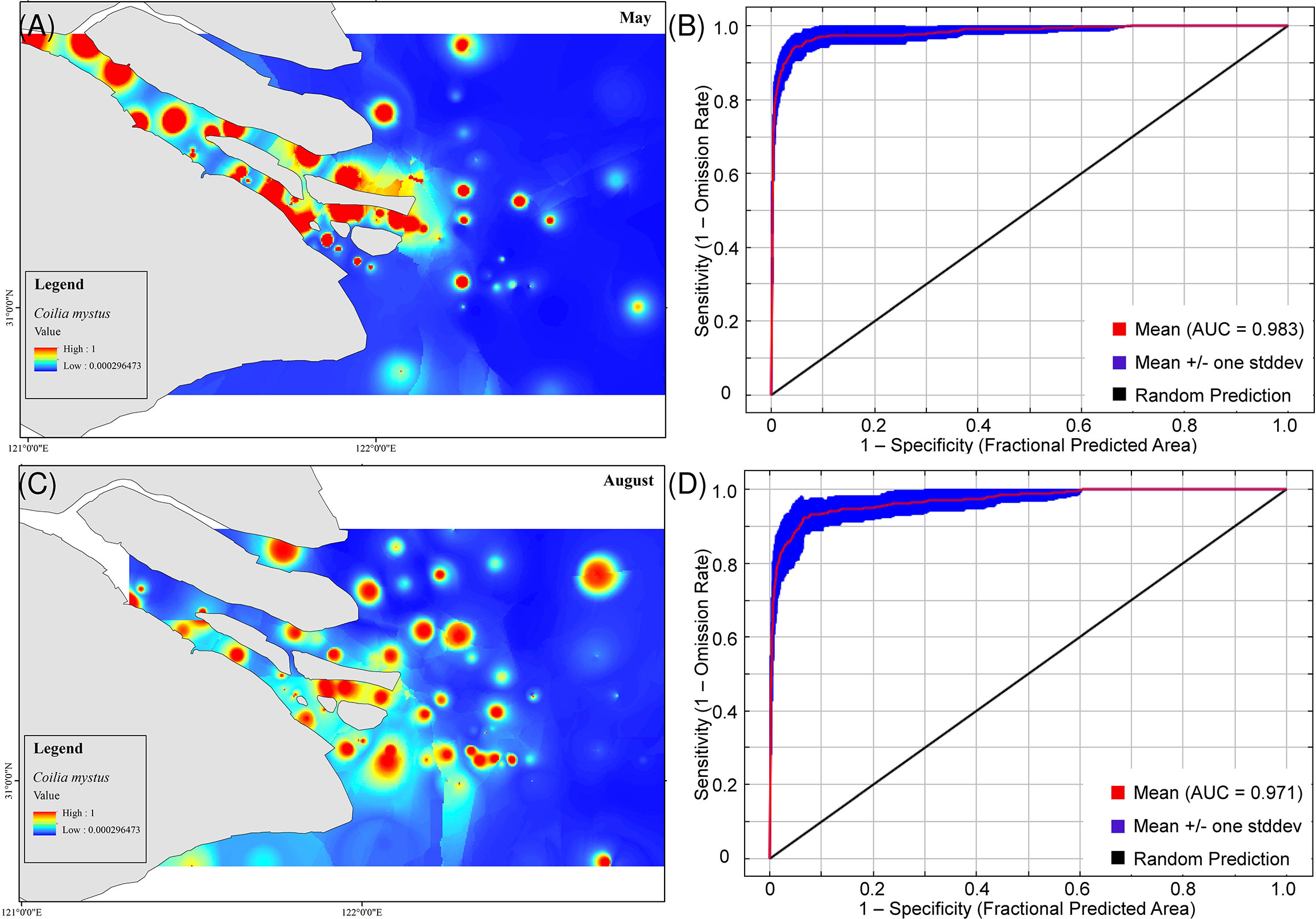
Figure 4 Predicted optimal distribution of C mystus through the MaxEnt model. (A, C) Potential geographical distribution of C mystus in Yangtze Estuarine during the May (A) and August (C) breeding season. (B, D) Area under the curve result of MaxEnt modeling (10 runs) for May (B) and August (D).
The resistance values were 104.73–165.69 in May (Figure 5A) and 47.49–95.93 in August (Figure 5B), generally decreasing from offshore to upstream of the Yangtze River. ECs delineated in this study showed high spatial variances between May and August. Specifically, almost all ECs were concentrated in the south channel, especially around Jiuduansha Shoal (Figure 5A); however, ECs were almost homogeneously spread in the YRE in August (Figure 5B). Furthermore, most ECs were distributed along the coastlines of Changxing Island, Jiuduansha Shoal, and Hengsha Island. The main nodes among EC interactions were accumulated at western Changxing and Chongming islands and around Jiuduansha Shoal, indicating potential conservation priority for YRE fishery biodiversity protection.
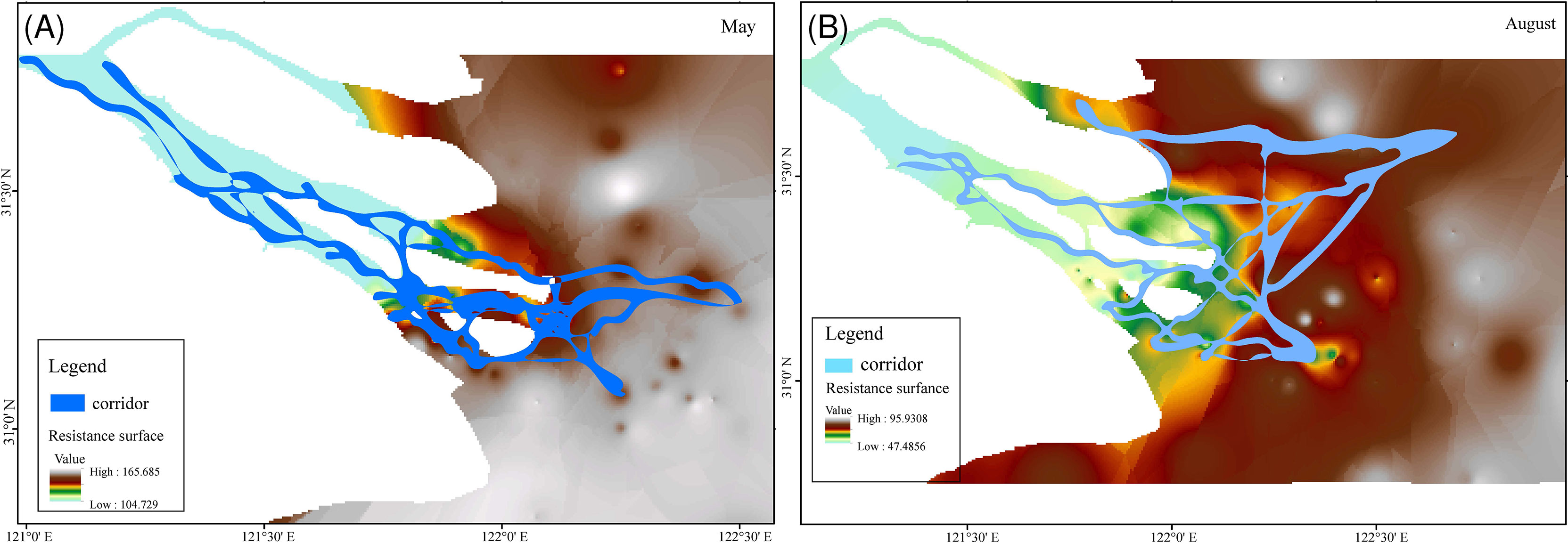
Figure 5 Theoretical migratory corridors of C mystus based on the least-cost route analysis in May (A) and August (B).
Discussion
Life-history characteristics of C. mystus and its fitness to environmental forcing
The life-history characteristics of species are a result of natural evolution and ecological adaptation. The documented spawning ground of C. mystus was in the maximum turbidity zone in the YRE (Hu et al., 2021). Our study extended the spawning ground to the Xuliujing hydrologic station (Figure 1B). This extension may indicate two characteristics of short-distance migratory species. First, adults migrating to the upstream freshwater area were an active selection. Runoff is an unneglectable factor controlling larvae drift. If the spawning ground is close to the estuary front, egg and larvae might be carried into the adjacent sea by the tide, where it will be difficult for them to survive because decreased sea water temperature might inhibit the hatching of eggs, and most larvae may become food sources for other fishes. Therefore, some adults would migrate upstream to freshwater, with weak tide and low salinity. Nonetheless, physiological characteristics may be the key factor controlling the migratory distance of C. mystus. Compared with long-distance migratory species C. nasus, C. mystus allocates most of the body lipid for gonadal development and rapidly consumes trunk lipid (Wu et al., 2017). Moreover, when migrating across a large salinity gradient, C. mystus needs to evolve the ability to rapidly regulate the equilibration of fluid osmotic pressure, potentially raising the cost of population maintenance. Second, some C. mystus adults spawn in the estuary front area, and their larvae drift upstream with tides. In this case, some unknown physiological characteristics may be developed to adapt the ebb and flow tide to sustain their spatial distribution. Some species in the Penaeus family could sense the increase or decrease in water pressure at a depth of 8 m to conduct directional migration. During a flood tide, they enter the estuary along the tide but swim to the bottom to avoid being carried out of the estuary during an ebb tide (Vance and Pendrey, 2008; Wolanski, 2017). Constricted by traditional investigation approaches and the natural characteristics of most marine fish species, providing direct evidence about their migration patterns, life-history characteristics, or adaptative evolution is challenging. Advancements in animal tracking and environmental DNA technology enable greater data collection on migratory behavior and environmental suitability assessment (Xu et al., 2018; Thalinger et al., 2019; Yatsuyanagi and Araki, 2020). Collecting more data using advanced technologies to protect more extinct or dominant species is necessary.
Based on RDA, salinity, CODMn, SS, N, P, pH, Si, DO, Temp, and Chla may considerably affect the breeding and migratory behavior of C. mystus. Among them, physical factors such as salinity, Temp, SS, and DO are correlated with the survival or growth of marine species (Spares et al., 2012; Walsh et al., 2013); however, chemical factors such as Chla, N, P, Si, and CODMn have not been studied adequately. Higher N, P, and Si promote algal growth, and increased Chla indicates high algal bioactivity. Algae produce large amounts of oxygen through photosynthesis and increase the DO content of the water column. As primary producers, the healthy growth of phytoplankton provides the material basis for fish growth and reproduction. Hence, detecting a positive relationship between Chla, N, P, Si, and C. mystus growth is reasonable.
The optimum environmental conditions for C. mystus larvae were as follows: salinity, 5‰–12‰; temperature, 20°C–28°C; and DO concentration, 5.2–8.0 mg/L (Hu et al., 2021), indicating that any event causing environmental variables beyond the range of biological adaptation may lead to biodiversity reduction. For example, saltwater intrusion can carry sexually mature C. mystus to freshwater through the north branch or to brackish water through the south branch. If the upstream freshwater runoff is low, the tide upwells, and brackish water expands upstream, providing a broad survival space for C. mystus larvae; such causality may account for the broader longitudinal distribution of the larvae in May than in August. However, the natural ecosystem is more complex because of the non-linear relationship among all biotic and abiotic factors for the fitness of organisms. Hence, it is necessary to study their fitness to the dynamics of certain or coupled variables.
Marine fishery biodiversity protection through ecological corridors
The YRE is rich in fish biodiversity, supporting 46 freshwater fishes, 53 estuarine sedentary and migratory fishes, and 48 marine fishes (Zhuang et al., 2006). Life-history characteristics revealed that the dominant species varied from season to season, which included Engraulis japonicus, C. mystus, Anchoviella commersonii, Gobiidae, and Liza haematocheilus in spring; C. nasus, C. mystus, and Stolephorus chinensis in summer; Salanx ariakensis, and Benthosema pterotum in autumn; and Neosalanx jordani in winter. Most of these dominant species act as secondary consumers relying on organic detritus or primary consumers (including zooplankton such as copepods, branchiopods, and benthic invertebrates). To date, only a few studies have reported the interspecific competition in the fish community; hence, we speculate that anthropogenic and environmental stressors that directly affect food and habitat quality severely influence the marine fish biodiversity of the YRE.
We investigated the 17 larvae of marine, estuarine, freshwater, and migratory fishes in the YRE during the past few years (Table 1). The spatial distribution of larvae did not match that of adults, which is consistent with the functional orientation of the YRE as migratory passage, spawning, nursing, and feeding grounds. Most larvae species distributed in the maximum turbidity zone developed under the coupled effects of freshwater discharge, tides and currents, the mixture of freshwater and saltwater, and suspended sediment transportation (Shen et al., 1992). Marine fishes use the YRE as a nursing and feeding ground because the convergence of various water masses brings in rich nutrients, and long-distance migratory species need to regulate the equilibration of fluid osmotic pressure here. Some freshwater species, such as those from the Cyprinidae family, can be detected due to complex hydrodynamics here. For example, freshwater discharge from Huangpu River (Figure 1A) southward into the sea along the coastal waters of Shanghai forms a fresh flume. Sometimes, saltwater intrusion might increase salinity in waters near Changxing Island and western Chongming Island. Considering the diversity in the marine species community, their habitat utilization, and the complexity of the marine environment, our study on the short-distance migratory C. mystus may provide a breakthrough for researching multispecies habitat utilization and conservation.
C. mystus, a typical short-distance migratory fish in the YRE, mainly lives in the nearshore area. C. mystus migration began during the spawning period from the nearshore sea area with 25‰ salinity to the estuarine front area with approximately 4% salinity. Some adults migrated further anadromous to the freshwater. Fish larvae were mainly distributed near Jiuduansha Shoal (Figure 1A), which displays a mixture of brackish and fresh waters with the most intense tidal influence. Affected by tides and runoff, the distribution of C. mystus eggs in May was closer to the upstream of the YRE, whereas that in August was closer to the periphery with 4‰–10‰ salinity. In general, migration is an important adaptive evolutionary strategy for fishes to maintain population genetic diversity. Compared with fishes in uniform habitats, migratory fishes need to span different habitats. Highly suitable patches, also called source and sink patches in our study, are important nodes and ecological stepping stones for their migration. The quantity, quality, and connectivity of source and sink patches directly determine whether the species can successfully migrate to their destinations. This study further showed that the estuarine front area was an important ecological aid for anadromous migration, with a larger number of nodes and density of ECs in May and August in this area.
In conclusion, in this study, three priority areas were identified, including the maximum turbidity zone located at the estuarine mouth and two brackish water confluence areas located around Changxing Island and western Chongming Island (Figure 1B). Among these priority areas, C. mystus might prefer migrating through the southern branch, especially the south channel in the May breeding season (Figure 5A), but without any bias in August (Figure 5B). Furthermore, most theoretical corridors are distributed along the coastlines except those in the offshore area, which might infer the more important role of islands in fishery production and biodiversity protection. Aside from that, theoretical ECs also ensure the connectivity of some important nature reserves, such as the C. nasus germplasm resource protection area at western Chongming Island, YRE Chinese sturgeon Nature Reserve to eastern Chongming Island, and some important wetlands located at Chongming Island, Jiuduansha Shoal, and Nanhui Shoal. ECs also provide an accessible approach to fishery management and biodiversity conservation. On one hand, theoretical ECs provide a least-cost movement route for fish migration and inhabitation, indicating that migratory species can sustain their population connectivity at the least cost. On the other hand, from a social management perspective, imposing a ban on an entire estuary is unnecessary, and differential measures must be taken in corridors and priority areas to facilitate the harmonious development of socioeconomic and biodiversity conservation, especially for the high-traffic YRE.
Data availability statement
The raw data supporting the conclusions of this article will be made available by the authors, without undue reservation.
Author contributions
YH, LZ, XZ, and XJ designed research; SL and YW contributed field samples collection and species classification; YH and LZ analyzed data and wrote the paper; All authors contributed to discussion of the content, and reviewed the manuscript. All authors contributed to the article and approved the submitted version.
Funding
This work was supported by the Yangtze Delta Estuarine Wetland Ecosystem Observation and Research Station, Ministry of Education & Shanghai Science and Technology Committee (ECNU-YDEWS-2020), National Natural Science Foundation for Youth Scientists of China (NO.42106171), Open Fund of Key Laboratory of Marine Ecological Monitoring and Restoration Technology, MNR (NO. MEMRT202111) and Open Fund of Key Laboratory of Ocean Space Resource Management Technology, MNR (KF-2021-107).
Conflict of interest
The authors declare that the research was conducted in the absence of any commercial or financial relationships that could be construed as a potential conflict of interest.
Publisher’s note
All claims expressed in this article are solely those of the authors and do not necessarily represent those of their affiliated organizations, or those of the publisher, the editors and the reviewers. Any product that may be evaluated in this article, or claim that may be made by its manufacturer, is not guaranteed or endorsed by the publisher.
Supplementary material
The Supplementary Material for this article can be found online at: https://www.frontiersin.org/articles/10.3389/fmars.2022.966621/full#supplementary-material
Abbreviations
ECs, ecological corridors; YRE, Yangtze River Estuary; SS, suspended particles; DO, dissolved oxygen; N, total nitrogen; P, total phosphorus; Si, reactive silicate; Temp, estuarine surface water temperature; CODMn, permanganate index; Chla, chlorophyll-a; depth, water column depth; RDA, redundancy analysis.
References
Allen C. H., Parrott L., Kyle C. (2016). An individual-based modelling approach to estimate landscape connectivity for bighorn sheep (Ovis canadensis). PeerJ 4, e2001. doi: 10.7717/peerj.2001
Almany G. R., Planes S., Thorrold S. R., Berumen M. L., Bode M., Saenz-Agudelo P., et al. (2017). Larval fish dispersal in a coral-reef seascape. Nat. Ecol. Evol. 1 (6), 148. doi: 10.1038/s41559-017-0148
Balbar A. C., Metaxas A. (2019). The current application of ecological connectivity in the design of marine protected areas. Glob. Ecol. Conserv. 17, e00569. doi: 10.1016/j.gecco.2019.e00569
Bastian O., Grunewald K., Khoroshev A. V. (2015). The significance of geosystem and landscape concepts for the assessment of ecosystem services: exemplified on a case study in Russia. Landscape Ecol. 30, 1145–1164. doi: 10.1007/s10980-015-0200-x
Benson R. L., Turo S., McCovey J. B.W. (2007). Migration and movement patterns of green sturgeon (Acipenser medirostris) in the klamath and trinity rivers, California, USA. Environ. Biol. Fish. 79, 269–279. doi: 10.1007/s10641-006-9023-6
Botsford L. W., Brumbaugh D. R., Grimes C. B., Kellner J. B., Largier J., Michael R., et al. (2009). Connectivity, sustainability, and yield: bridging the gap between conventional fisheries management and marine protected areas. Rev. Fish. Biol. Fish. 19, 69–95. doi: 10.1007/s11160-008-9092-z
Carr M., Robinson S. P., Wahle C., Davis G., Kroll S., Murray S., et al. (2017). The central importance of ecological spatial connectivity to effective coastal marine protected areas and to meeting the challenges of climate change in the marine environment. Aquat. Conserv. 27, 6–29. doi: 10.1002/aqc.2800
Chaparro-Pedraza P. C., de Roos A. M. (2019). Environmental change effects on life-history traits and population dynamics of anadromous fishes. J. Anim. Ecol. 88 (8), 1178–1190. doi: 10.1111/1365-2656.13010
Christie M. R., Tissot B. N., Albins M. A., Beets J. P., Jia Y., Ortiz D. M., et al. (2010). Larval connectivity in an effective network of marine protected areas. PloS One 5 (12), e15715. doi: 10.1371/journal.pone.0015715
Cowen R. K., Sponaugle S. (2009). Larval dispersal and marine population connectivity. Annu. Rev. Mar. Sci. 1, 443–466. doi: 10.1146/annurev.marine.010908.163757
D'Antonio C. M., Thomsen M. (2004). Ecological resistance in theory and practice. Weed Techno. 18, 1572–1577. doi: 10.1614/0890-037X(2004)018[1572:ERITAP]2.0.CO;2
D’Aloia C. C., Bogdanowicz S. M., Francis R. K., Majoris J. E., Harrison R. G., Buston P. M., et al. (2015). Patterns, causes, and consequences of marine larval dispersal. Proc. Natl. Acad. Sci. U.S.A. 112 (45), 13940–19945. doi: 10.1073/pnas.1513754112
Degteva S. V., Ponomarev V. I., Eisenman S. W., Dushenkov V. (2015). Striking the balance: challenges and perspectives for the protected areas network in northeastern European Russia. Ambio 44 (6), 473–490. doi: 10.1007/s13280-015-0636-x
Elith J., Phillips S. J., Hastie T., Dudik M., Chee Y. E., Yates C. J. (2010). A statistical explanation of MaxEnt for ecologists. Diversity Distrib 17 (1), 1–15. doi: 10.1111/j.1472-4642.2010.00725.x
Ferguson J. W., Healey M., Dugan P., Barlow C. (2011). Potential effects of dams on migratory fish in the Mekong river: Lessons from salmon in the fraser and Columbia rivers. Environ. Manage. 47 (1), 141–159. doi: 10.1007/s00267-010-9563-6
Finn R. N. (2007). The physiology and toxicology of salmonid eggs and larvae in relation to water quality criteria. Aquat. Toxicol. 81 (4), 337–354. doi: 10.1016/j.aquatox.2006.12.021
Gao Y.-C., Yang Q., Li H.-J., Wang X.-C., Zhan A.-B. (2019). Anthropogenic pollutant-driven geographical distribution of mesozooplankton communities in estuarine areas of the bohai Sea, China. Sci. Rep. 9, 9668. doi: 10.1038/s41598-019-46047-5
Garcia M. D., Barria de Cao S. M. (2018). Anthropogenic pollution along the coast of a temperate estuary: effects on tintinnid assemblages. Hydrobiologia 809, 201–219. doi: 10.1007/s10750-017-3465-z
Gillanders B. M., Able K. W., Brown J. A., Eggleston D. B., Sheridan P. F. (2003). Evidence of connectivity between juvenile and adult habitats for mobile marine fauna: An important component of nurseries. Mar. Ecol. Prog. Ser. 247, 281–295. doi: 10.3354/meps247281
Gillanders B. M., Elsdon T. S., Halliday I. A., Jenkins G. P., Robins J. B., Valesini F. J. (2011). Potential effects of climate change on Australian estuaries and fish utilizing estuaries: a review. Mar. Fresh. Res. 62, 1115–1131. doi: 10.1071/MF11047
Hanson N., Fogel M., Fong D. W., MacAvoy S. E. (2010). Marine nutrient transport: Anandromous fish migration linked to the freshwater amphipod gammarus fasciatus. Can. J. Zool. 88 (6), 546–552. doi: 10.1139/Z10-030
Hauer F. R., Locke H., Dreitz V. J., Hebblewhite M., Lowe W. H., Muhlfeld C. C., et al. (2016). Gravel-bed river floodplains are the ecological nexus of glaciated mountain landscapes. Sci. Adv. 2 (6), e1600026. doi: 10.1126/sciadv.1600026
Hilty J. A., Keeley A. T. H., Lidicker W. Z. Jr., Merenlender A. M. (2019). Corridor ecology: Linking landscapes for biodiversity conservation and climate adaptation. 2nd ed (Washington, DC: Island Press).
Hu L. J., Song C., Geng Z., Zhao F., Jiang J., Liu R. H., et al. (2021). Temporal and spatial distribution of Coilia mystus larvae and juveniles in the Yangtze estuary during primary breeding season. J. Fish. Sci. China 28 (9), 1152–1161. doi: 10.12264/JFSC2020-0343
Jiang T., Liu H.-B., Shen X.-Q., Shimasaki Y., Oshima Y., Yang J. (2014). Life history variations among different populations of coilia nasus along the Chinese coast inferred from otolith microchemistry. J. Fac. Agr. Kyushu U. 59, 383–389. doi: 10.5109/1467650
Jiang T., Yang J., Liu H., Shen X. (2012). Life history of coilia nasus from the yellow Sea inferred from otolith Sr:Ca ratios. Environ. Biol. Fish. 95 (4), 503–508. doi: 10.1007/s10641-012-0066-6
Johnston E. L., Roberts D. A. (2009). Contaminants reduce the richness and evenness of marine communities: a review and meta-analysis. Environ. pollut. 157 (6), 1745–1752. doi: 10.1016/j.envpol.2009.02.017
Keeley A. T. H., Ackerly D. D., Cameron D. R., Heller N. E., Huber P. R., Schloss C. A., et al. (2018). New concepts, models, and assessments of climate-wise connectivity. Environ. Res. Lett. 13, 073002. doi: 10.1088/1748-9326/aacb85
Klaasen M. (1996). Metabolic constraints on long-distance migration in birds. J. Exp. Biol. 199 (1), 57–64. doi: 10.1242/jeb.199.1.57
Lamb J. M., Ralph T. M. C., Goodman S. M., Bogdanowicz W., Fahr J., Gajewska M., et al (2008). Phylogeography and predicted distribution of African-Arabian and Malagasy populations of giant mastiff bats, Otomops spp. (Chiroptera: Molossidae). Acta Chiropterologica 10 (1), 21–40. doi: 10.3161/150811008X331063
Lawler J. J., Ruesch A. S., Olden J. D., McRae B. H. (2013). Projected climate-driven faunal movement routes. Ecol. Lett. 16, 1014–1022. doi: 10.1111/ele.12132
Levin L. A., Boesch D. F., Covich A., Dahm C., Erséus C., Ewel K. C., et al. (2001). The function of marine critical transition zones and the importance of sediment biodiversity. Ecosystems 4, 430–451. doi: 10.1007/s10021-001-0021-4
Liu S. H., Xu Z. L. (2011). Comparison of zooplankton lists between coilia mystus food contents and collections from the Yangtze river estuary & hangzhou bay. Acta Ecologica Sinica. 31 (8), 2263–2271.
Li Y. X., Yang S. M., Yan Y. T. (2022). Characteristics of phytoplankton assemblages in the central south China Sea. Mar. pollut. Bull. 174, 173189. doi: 10.1016/j.marpolbul.2021.113189
Mantas T. P., CVarotti C., Roveta C., Palma M., Carlo Innocenti I., Giusti M., et al. (2022). Mediterranean Sea Shelters for the gold coral Savalia savaglia: An assessment of potential distribution of a rare parasitic species. Mar. Environ. Res. 179, 1–12. doi: 10.1016/j.marenvres.2022.105686
McGuire J. L., Lawler J. J., McRae B. H., Nuñez T. A., Theobald D. M. (2016). Achieving climate connectivity in a fragmented landscape. Proc. Natl. Acad. Sci. U.S.A. 113 (26), 7195–7200. doi: 10.1073/pnas.160281711
McRae B., Shah V. B., Edelman A. (2016). Circuitscape: modeling landscape connectivity to promote conservation and human health. The Nature Conservancy, Fort Collins, CO, 14. doi: 10.13140/RG.2.1.4265.1126
Miklos L., Diviakova A., Izakovičov. Z. (2019). Ecological networks and territorial systems of ecological stability (London: Springer Nature).
Pelage L., Guazzelli-Gonzalez J. G., Le Loch F., Ferreira V., Munaron J., Lucena-Fredou F., et al. (2021). Importance of estuary morphology for ecological connectivity with their adjacent coast: A case study in Brazilian tropical estuaries. Estuar. Coast. Shelf. S. 251 (1-2), 1–11. doi: 10.1016/j.ecss.2021.107184
Phillips S. J., Anderson R. P., Dudík M., Schapire R. E., Blair M. E. (2017). Opening the black box: an open-source release of maxent. Ecography 40 (7), 887–893. doi: 10.1111/ecog.03049
Phillips S. J., Anderson R. P., Schapire R. E. (2006). Maximum entropy modeling of species geographic distributions. Ecol. Model. 190 (3–4), 231–259. doi: 10.1016/j.ecolmodel.-2005.03.026
Pinto N., Keitt T. H. (2009). Beyond the least-cost path: evaluating corridor redundancy using a graph-theoretic approach. Landscape Ecol. 24 (2), 253–266. doi: 10.1007/s10980-008-9303-y
Planes S., Jones G. P., Thorrold S. R. (2009). Larval dispersal connects fish populations in a network of marine protected areas. Proc. Natl. Acad. Sci. U.S.A. 106 (14), 5693–5697. doi: 10.1073/pnas.0808007106
R Core Team (2014). R: A language and environment for statistical computing (Vienna: R Foundation for Statistical Computing).
Rossi T., Pistevos J. C. A., Connell S. D., Nagelkerken I. (2018). On the wrong track: ocean acidification attracts larval fish to irrelevant environmental cues. Sci. Rep. 8, 5840. doi: 10.1038/s41598-018-24026-6
Runge C. A., Watson J. E. M., Butchart S. H., Hanson J. O., Possingham H. P., Fuller R. A. (2015). Protected areas and global conservation of migratory birds. Science 350, 1266–1258. doi: 10.1126/science.aac9180
Saenz-Agudelo P., Jones G. P., Thorrold S. R., Planes S. (2010). Connectivity dominates larval replenishment in a coastal coral reef fish metapopulation. Proc. R. Soc B. 278, 2954–2961. doi: 10.1098/rspb.2010.2780
Scanes E., Scanes P. R., Ross P. M. (2020). Climate change rapidly warms and acidifies Australian estuaries. Nat. Commun. 11 (1), 1803. doi: 10.1038/s41467-020-15550-z
Schram E., Roques J., van Kuijk T., Abbink W., Heul J., Vries P., et al. (2014). The impact of elevated water ammonia and nitrate concentrations on physiology, growth and feed intake of pikeperch (Sander lucioperca). Aquaculture 420, 95–104. doi: 10.1016/j.aquaculture.2013.10.027
Seidensticker J., Dinerstein E., Goyal S. P., Gurung B., Harihar A., Johnsingh A., et al. (2010). “Tiger range collapse and recovery at the base of the Himalayas: a case study,” in Biology and conservation of wild felids (London: Oxford Unversity Press).
Shen H., He S. L., Pan D. A., Li J. F. (1992). A study of turbidity maximum in the changjiang estuary. Acta Geographica Sinica. 47 (5), 472–479. doi: 10.11821/xb199205010
Spares A. D., Stokesbury M. J. W., O’Dor R. K., Dick T. A. (2012). Temperature, salinity and prey availability shape the marine migration of Arctic char, salvelinus alpinus, in a macrotidal estuary. Mar. Biol. 159 (8), 1633–1646. doi: 10.1007/s00227-012-1949-y
Sykes G. E., Shrimpton J. M. (2010). Effect of temperature and current manipulation on smolting in chinook salmon (Oncorhynchus tshawytscha): The relationship between migratory behaviour and physiological development. Can. J. Fish. Aquat. Sci. 67 (1), 191–201. doi: 10.1139/F09-166
Thalinger B., Wolf E., Traugott M., Wanzenböck J. (2019). Monitoring spawning migrations of potamodromous fish species via eDNA. Sci. Rep. 9 (1), 15388. doi: 10.1038/s41598-019-51398-0
Tittensor D. P., Baco A. R., Brewin P. E., Clark M. R., Consalvey M., Hall-Spencer J., et al. (2009). Predicting global habitat suitability for stony corals on seamounts. J. Biogeogr. 36, 1111–1128. doi: 10.1111/j.1365-2699.2008.02062.x
Tong M.-X., Zhang L., Li J., Zockler C., Clark N. (2012). The critical importance of the rudong mudflats, jiangsu province, China in the annual cycle of the spoon-billed sandpiper calidris pygmeus. Wader Study Group Bulletin. 119 (3), 74–77.
Urban D., Keitt T. H. (2001). Landscape connectivity: A graph-theoretic perspective. Ecology 82, 1205–1218. doi: 10.1890/0012-9658(2001)082[1205:LCAGTP]2.0.CO;2
Vance D. J., Pendrey R. C.. (2008). Vertical migration of postlarval penaeid prawns in two Australian estuaries: the effect of tide and day/night. Mar. Freshwater Res. 59 (8), 671–683. doi: 10.1071/MF07234
Verbruggen H., Tyberghein L., Pauly K., Vlaeminck C., Van Nieuwenhuyze K., Kooistra W., et al. (2009). Macroecology meets macroevolution: evolutionary niche dynamics in the seaweed halimeda. Global Ecol. Biogeogr. 18, 393–405. doi: 10.1111/j.1466-8238.2009.00463.x
Vu A. V., Baumgartner L. J., Limburg K. E., Doran G. S., Mallen-Cooper M., Gillanders B. M., et al. (2022). Life history strategies of mekong pangasiid catfishes revealed by otolith microchemistry. Fish. Res. 249, 106239. doi: 10.1016/j.fishres.2022.106239
Walsh C. T., Reinfelds I. V., Ives M. C., Gray C. A., West R. J., van der Meulen D. E. (2013). Environmental influences on the spatial ecology and spawning behaviour of an estuarine-resident fish, macquaria colonorum. Estuar. Coast. Shelf S. 118, 60–71. doi: 10.1016/j.ecss.2012.12.009
Wang H., Wang P., Xu C., Sun Y.-F., Shi L., Zhou L., et al. (2022). Can the ‘Ten-year fishing ban’ rescue biodiversity of the Yangtze river? Innovation. 3 (3), 100235. doi: 10.1016/j.xinn.2022.100235
Wang C.-Y., Wei Q.-W., Kynard B., Du H., Zhang H. (2012). Migrations and movements of adult Chinese sturgeon acipenser sinensis in the Yangtze river, China. J. Fish Biol. 81 (2), 696–713. doi: 10.1111/j.1095-8649.2012.03365.x
Wang S.-K., Zhang T., Yang G., Wang Y., Zhao F., Zhuang P. (2018). Migration and feeding habits of juvenile Chinese sturgeon (Acipenser sinensis Gray 1835) in the Yangtze estuary: Implications for conservation. Aquat. Conservation: Mar. Freshw. Ecosystems. 28 (6), 1329–1336. doi: 10.1002/aqc.2935
Weeks R. (2017). Incorporating seascape connectivity in conservation prioritization. PloS One 12 (7), e0182396. doi: 10.1371/journal.pone.0182396
Wolanski E. (2017). Bounded and unbounded boundaries-untangling mechanisms for estuarine-marine ecological connectivity: Scales of m to 10,000 km-a review. Estuar. Coast. Shelf S. 198, 378–392. doi: 10.1016/j.ecss.2016.06.022
Wu H., Deng B., Yuan R., Gu J. H., Shen F., Zhu J. R., et al. (2013). Detiding measurement on transport of the changjiang-derived buoyant coastal current. J. Phys. Oceanogr. 43 (11), 2388–2399. doi: 10.1175/JPO-D-12-0158.1
Wu L. H., Tang W. Q., Zhang Y. (2017). Research on the differences of anadromous migratory distance between Coilia mystus and Coilia nasus based on the transfer process of body lipid. J. fisheries China 41 (2), 212–220. doi: 10.11964/jfc.20160310330
Wu T., Wu H. (2018). Tidal mixing sustains a bottom-trapped river plume and buoyant coastal current on an energetic continental shelf. J. Geophys. Res-Oceans 123 (11), 8026–8051. doi: 10.1029/2018JC014105
Wu H., Zhu J. R., Shen J., Wang H. (2011). Tidal modulation on the changjiang river plume in summer. J. Geophys. Res. 116 (C8), 1–21. doi: 10.1029/2011jc007209
Xia Y. L. (2020). Correlation and association analyses in microbiome study integrating multiomics in health and disease. Prog. Mol. Biol. Transl. 171, 309–491. doi: 10.1016/bs.pmbts.2020.04.003
Xu N., Zhu B., Shi F., Shao K., Que Y.-F., Li W. T., et al. (2018). Monitoring seasonal distribution of an endangered anadromous sturgeon in a large river using environmental DNA. Sci. Nat. 105, (11–12). doi: 10.1007/s00114-018-1587-4
Yang J., Arai T., Liu H., Miyazaki N., Tsukamoto K. (2006a). Environmental signature in the otolith elemental fingerprint of the tapertail anchovy, Coilia mystus, from the changjiang estuary, China. J. Appl. Ichthyol. 22 (5), 459–462. doi: 10.1111/j.1439-0426.2006.00776.x
Yang J., Arai T., Liu H., Miyazaki N., Tsukamoto K. (2006b). Reconstructing habitat use of Coilia mystus and Coilia ectenes of the Yangtze river estuary, and of Coilia ectenes of taihu lake, based on otolith strontium and calcium. J. Fish Biol. 69 (4), 1120–1135. doi: 10.1111/j.1095-8649.2006.01186.x
Yang Q., Zhao F., Song C., Zhang T., Zhuang P., Jiang T., et al. (2019). Habitat history reconstruction of coilia mystus from the Yangtze river estuary and its adjacent sea area. J. Fish. Sci. China 26 (6), 1175–1184.
Yates C., McNeill A., Elith J., Midgley G. (2010). Assessing the impacts of climate change and land transformation on banksia in the south West Australian floristic region. Divers. Distrib. 16, 187–201. doi: 10.1111/j.1472-4642.2009.00623.x
Yatsuyanagi T., Araki H. (2020). Understanding seasonal migraton of shishamo smelt in coastal regions using environmental DNA. PloS One 15, e0239912. doi: 10.1371/journal.pone.0239912
Zeng Q., Dong F.-Y. (1993). Study on the biological characteristics and factor correlation of coilia mystus propagating population. J. lake Sci. 5 (2), 164–170.
Zhuang P., Zhang T., Li S.-F., Ni Y., Wang Y.-H., Deng S. M., et al. (2006). Fishes of the Yangtze estuary (Beijing: China agriculture press).
Keywords: ecological corridors, biodiversity, Yangtze Estuary, Coilia mystus, MaxEnt model, fish conservation
Citation: He Y, Zhao L, Liu S, Zhao X, Wang Y and Jiang X (2022) Delineation of estuarine ecological corridors using the MaxEnt model to protect marine fishery biodiversity. Front. Mar. Sci. 9:966621. doi: 10.3389/fmars.2022.966621
Received: 11 June 2022; Accepted: 16 September 2022;
Published: 06 October 2022.
Edited by:
Xi Xiao, Zhejiang University, ChinaCopyright © 2022 He, Zhao, Liu, Zhao, Wang and Jiang. This is an open-access article distributed under the terms of the Creative Commons Attribution License (CC BY). The use, distribution or reproduction in other forums is permitted, provided the original author(s) and the copyright owner(s) are credited and that the original publication in this journal is cited, in accordance with accepted academic practice. No use, distribution or reproduction is permitted which does not comply with these terms.
*Correspondence: Yanlong He, yanlhel@163.com
†These authors contributed equally to this work and share first authorship