- 1Sydney School of Veterinary Science, Faculty of Science, The University of Sydney, Camperdown, NSW, Australia
- 2School of Animal and Veterinary Sciences, Faculty of Science, The University of Adelaide, Roseworthy, SA, Australia
Toxoplasma gondii is a ubiquitous parasite increasingly detected in marine mammals and suspected to contribute to limited recovery of endangered populations. This study reports on the exposure of the Australian sea lion (Neophoca cinerea) to this protozoon using archived adult and pup sera from three island colonies in South Australia. Modified agglutination testing (MAT) detected a seroprevalence of 30.4% (95% CI 13.2-52.9: n = 23) and high antibody titers (512 to > 2048 IU/ml) in adult females (median age 9.5 y, range 5.5-14.5 y) at Dangerous Reef, a felid-free island. Antibodies weren’t detected in any surveyed pup (97.5% CI 0.0-2.0%, n = 184) at two felid-free islands (Dangerous Reef: n = 21; Olive Island: n = 65), nor at Kangaroo Island (n = 98), which has a high-density feral cat population. Kangaroo Island pups of known age were 7-104 d old, while standard length and bodyweight comparison suggested younger and older pup cohorts at Dangerous Reef and Olive Island, respectively. This study provides the first quantification of disease risk in this endangered species from T. gondii exposure. The absence of detectable pup seroconversion supports the lack of detectable congenital transmission, maternal antibody persistence or early post-natal infection in the sampled cohort yet to commence foraging. An extended serosurvey of N. cinerea colonies is recommended to confirm the hypothesis of predominantly forage-associated exposure to T. gondii in this species. Findings have implications for parasitic disease risk in wildlife inhabiting Australia’s islands and for the feral cat control program on Kangaroo Island.
Introduction
Toxoplasma gondii is an emerging pathogen in marine mammals, with substantial worldwide seroprevalence in pinniped (seal and sea lion), cetacean (dolphin and whale) and mustelid (sea otter) species, (reviewed in Dubey et al., 2020) and documented mortalities in critically endangered populations (Roe et al., 2013). Understanding of the parasite’s potential to modulate marine mammal population size and geographical range comes from surveys of European and North American cetaceans and marine mustelids (Ahmadpour et al., 2022). In the threatened southern sea otter (Enhydra lutris nereis), for instance, T. gondii-associated encephalitis was considered a primary or contributing cause of more than a quarter of deaths (1998-2001: n = 105), predominantly in adults (Kreuder et al., 2003). In one spatial cluster, it was the primary cause of half of all deaths (n = 16). A longer-term study showed nearly two-thirds of sea otters (1998-2012: n = 542) were infected with T. gondii and the parasite contributed to at least one in ten deaths, while infectious disease more generally was the leading cause of death in this threatened species (Miller et al., 2020).
In pinnipeds, and specifically otariids (eared seals), T. gondii infection and clinical toxoplasmosis are reported in free-ranging populations worldwide (Jensen et al., 2012; Carlson-Bremer et al., 2015; Sepulveda et al., 2015; Michael et al., 2016). Dependent on serological method and selected cut-off value, seroprevalence can exceed 50% (Jensen et al., 2012). In comparison, surveying for T. gondii exposure in Australian otariid species is limited to Australian fur seals (Arctocephalus pusillus doriferus) in Bass Strait, where in 2007-2008 an absence of T. gondii antibodies were detected in adult females of unspecified age (n = 104) (Lynch et al., 2011). Reporting of sporadic cases of disseminated toxoplasmosis is also rare: the most recent in Australia was a subadult male long-nosed fur seal (Arctocephalus forsteri) that hauled out on the east coast in 2012 (Donahoe et al., 2014). For the endangered Australian sea lion (Neophoca cinerea), toxoplasmosis is listed as a potential threat to the species’ recovery (Threatened Species Scientific Committee, 2020), based on observations in other marine mammals and documented disease susceptibility (Kabay, 1996). To date there has not been any serological survey for T. gondii (or other infectious pathogen) exposure reported in this declining species. This is of particular importance given the species’ relatively low genetic diversity potentially associated with an increased susceptibility to infectious disease (Bilgmann et al., 2021), and a vulnerable population structure of approximately 80 effectively closed sub-populations resulting from extreme female natal site fidelity (Campbell et al., 2008). Consequently, disease-induced mortality outbreaks pose the greatest risk to the species’ resilience based on modelling of future population decline (Bilgmann et al., 2021).
Domestic (Felis catus) and wild (including feral) felids are the only definitive hosts of T. gondii, an intracellular and zoonotic apicomplexan protozoan parasite capable of infecting all warm-blooded animals. Across these species, the mammals and birds, parasite transmission is related either to foraging – ingestion of oocysts in contaminated food or water, or of tissue cysts in prey species – or to transplacental transmission from recently infected mother to offspring (Dubey, 2022). In the marine mammals, the former can contribute directly to illness or death from localized or disseminated infection, reported for instance in the California sea lion (Zalophus californianus) (Carlson-Bremer et al., 2015), Mediterranean monk seal (Monachus monachus) (Mazzariol et al., 2021) and multiple other seal species (van de Velde et al., 2016; Reisfeld et al., 2019). Additionally, infection can increase the risk of death from other causes such as predation and conspecific trauma, as observed in the southern sea otter (Kreuder et al., 2003) and New Zealand sea lion (Phocarctos hookeri) (Roe et al., 2017). Transplacental transmission, placental inflammation and disseminated congenital fetal infection, resulting in early or late-term abortion or stillbirth have also been documented in multiple species, among them Risso’s dolphin (Grampus griseus) (Resendes et al., 2002), the California sea lion (Carlson-Bremer et al., 2015) and the southern sea otter (Shapiro et al., 2016).
The infection of marine mammals indicates contamination of coastal and marine environments with oocysts shed in cat feces, by terrestrial water run-off or from ocean sewage discharge (Miller et al., 2002; Fayer et al., 2004) (Figure 1). For species frequenting coastal habitats, such as pinnipeds, infection could also result from direct contamination of haul out sites by cat feces. In any case, infection is most likely to result from oocyst (versus tissue cyst) ingestion, given the cold-blooded nature of their prey species (e.g., fish and marine invertebrates) precludes susceptibility to active T. gondii infection. Oocysts are environmentally resistant, capable of sporulation (becoming infectious) and temperature-dependent maintenance of infectivity in sea water for at least 6-24 months (Lindsay et al., 2003; Lindsay and Dubey, 2009). Increased exposure of marine mammals is likely facilitated by parasite carriage and maintenance of viability in cold-blooded prey species acting as phoretic hosts – including filter-feeding bivalve mollusks (e.g., blue (Mytilus edulis) (Bigot-Clivot et al., 2022) and green-lipped (Perna canaliculus) mussels (Coupe et al., 2018)) and fish species (Marino et al., 2019), the latter already a suggested source of infection for coastal and pelagic seabirds (Poulle et al., 2021; Campbell et al., 2022). Independent of prey species, the initial entry of the parasite into the marine food chain is likely enabled by aggregation in gelatinous marine polymers and by adhesion to algal biofilms on kelps grazed by marine invertebrates (Shapiro et al., 2014).
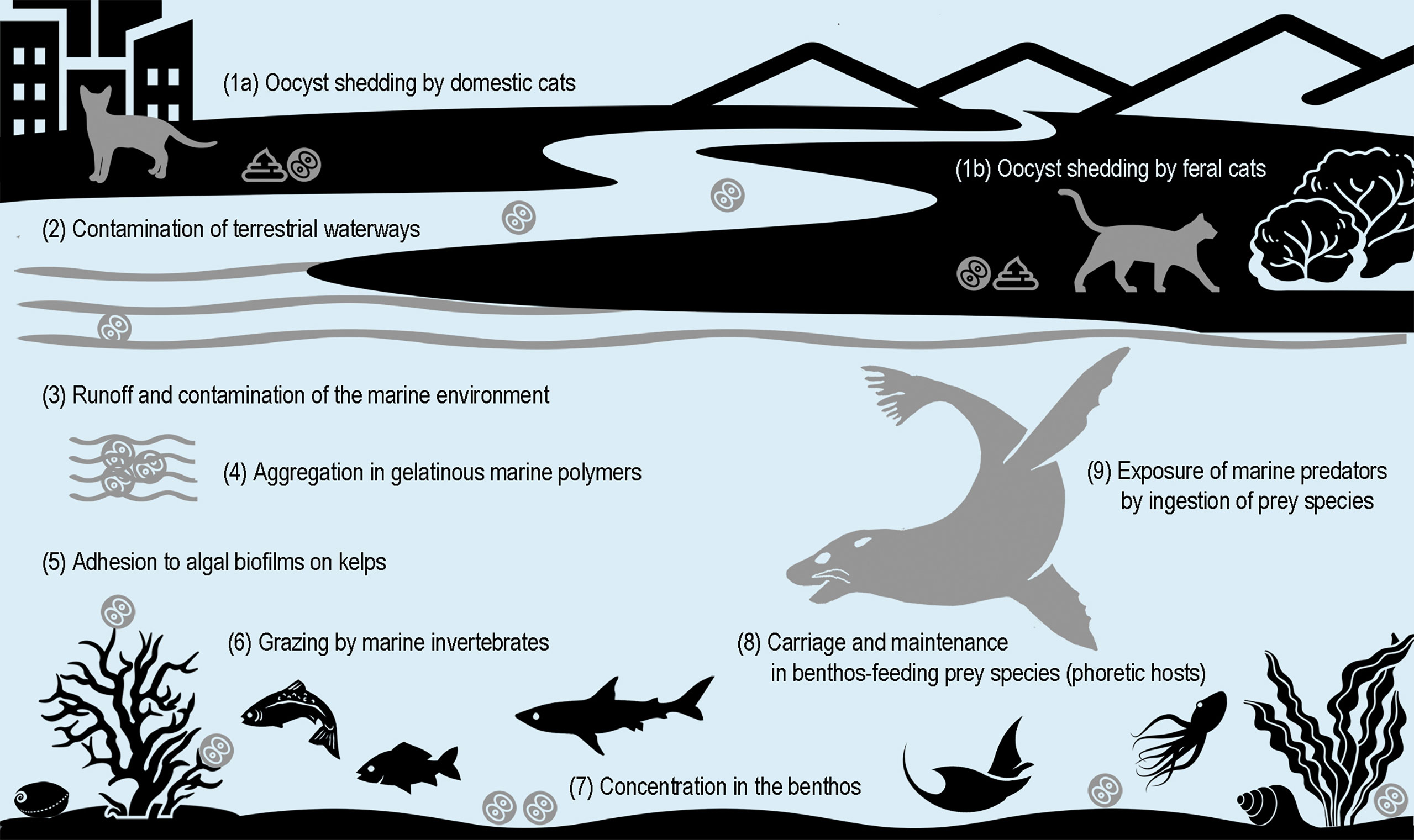
Figure 1 Proposed pathways for exposure of marine mammals to Toxoplasma gondii oocysts shed by terrestrial-bound definitive felid hosts.
The Australian sea lion inhabits the shores and islands of Australia’s southern and south-western coast (Gales et al., 1994), much of which is arid coastline of relatively low human population density. Australia’s abundant feral cat population, estimated to be 2.1-6.3 million animals dispersed across 99.8% of the continent (including the arid regions) (Legge et al., 2017) is a likely source of environmental contamination by T. gondii oocysts. Furthermore, while some Australian sea lion colonies exist on felid-free offshore islands (e.g., Olive Island and Dangerous Reef, South Australia), others (e.g., Seal Bay, Kangaroo Island, South Australia) are exposed to a feral cat density estimated to be 10 times greater than that of the adjacent mainland (Taggart et al., 2019a). For colonies on feral cat-inhabited islands there are potential health implications for pups (and adults) from increased exposure risk, given pups are mainly terrestrial until their first molt at 4-5 months of age, and are not weaned until 15-18 months of age. However, pups commence exploration of the adult foraging range by 6 months of age and obtain hunting skills at least 8 months prior to weaning (Lowther and Goldsworthy, 2012) at which age, their foraging-associated exposure risk (ingestion to T. gondii oocysts present in prey species) is likely to be similar to that of an adult animal.
This study aimed to provide the first estimate of T. gondii exposure in N. cinerea, and to infer route of infection in neonatal animals. We hypothesized that adult seroprevalence would parallel rates reported in other otariids, and that seroconversion would not be detected in young, pre-weaned pups yet to commence foraging. The latter investigation would also enable assessment of the persistence of any transplacental or colostrum-derived maternal antibodies.
Materials and methods
Study colonies
Archived sera from adult female Australian sea lions and pups were collected from Dangerous Reef (-34.817, 136.217), a small, uninhabited, and felid-free rocky island in the Spencer Gulf, South Australia (Figure 2), located 17.5 km from Lincoln National Park on the east coast of the Eyre Peninsula and 33 km from the coastal town of Port Lincoln (population 16,400). Pups were also sampled on Olive Island (-32.717, 133.983), a small, uninhabited, and felid-free island located 5 km from Cape Bauer on the west coast of the Eyre Peninsula and 25 km from the small regional coastal town of Streaky Bay (population 1,400), and at Seal Bay (-35.995, 137.317) on the south coast of Kangaroo Island, Australia’s third largest (4,405 km2) inhabited island (population 4,700). Kangaroo Island, located 13.5 km from the southern tip of the Fleurieu Peninsula and 112 km southwest of the state capital city of Adelaide (regional population 1,359,760), has a high-density feral cat population (Taggart et al., 2019a).
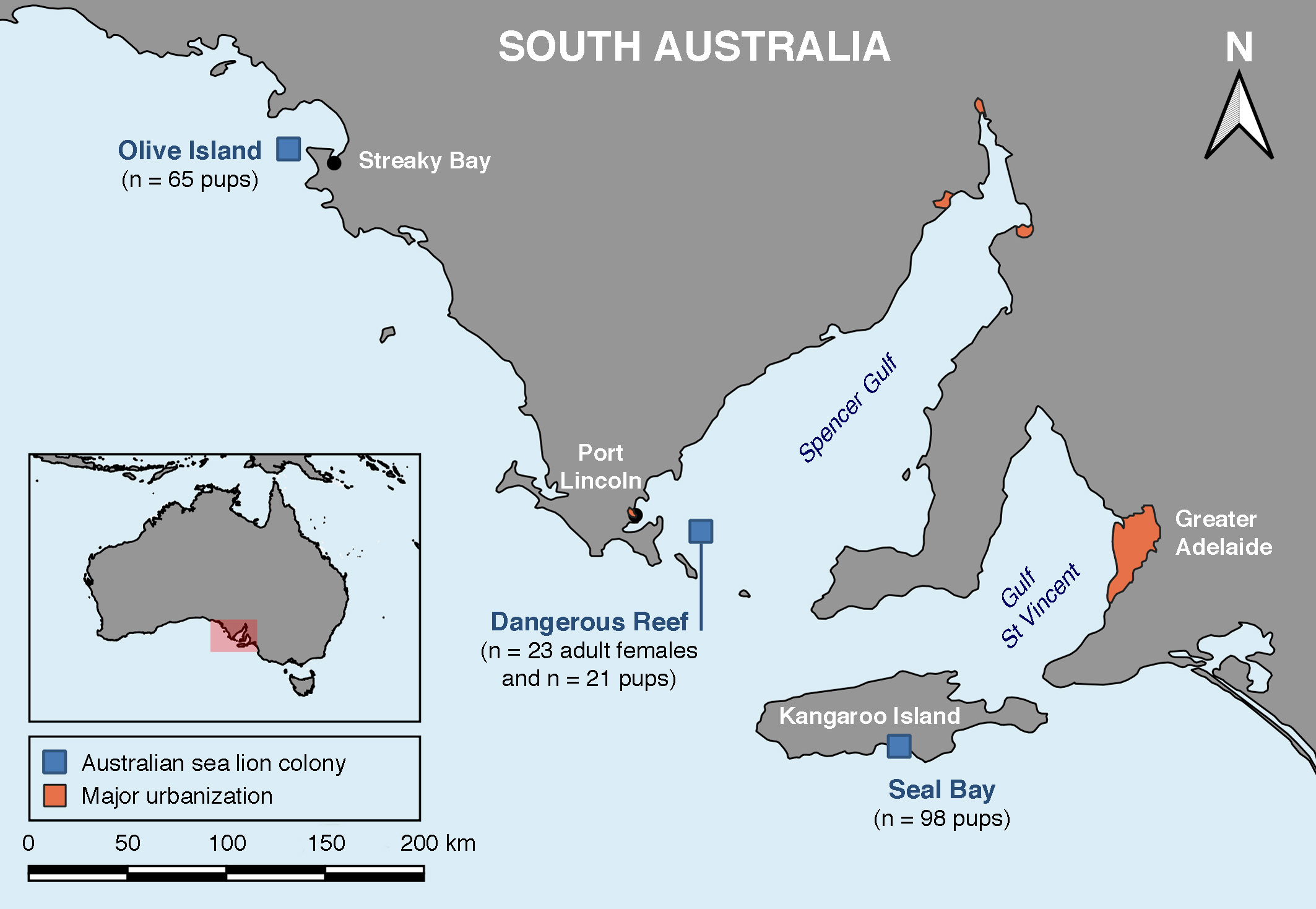
Figure 2 Location of the three Australian sea lion (Neophoca cinerea) colonies sampled for the serological survey of T. gondii antibody presence in adult females and pups.
Sample collection and age estimation
Australian sea lion pup samples were collected with approval of South Australian Department of Environment and Water (Permit/License Number: MR00073-2-R; Scientific Research Permit Number: A25088-12) and the study protocol was approved by The University of Sydney Animal Ethics Committee (Protocol Number 2014/726). Adult Australian sea lion samples were collected with approval of the South Australian Department for Environment and Heritage (DEH) (Permit Number U24630) and the study protocol was approved by La Trobe University Animal Ethics Committee (Permit AEC00/42(L)). Adult southern sea lion samples were collected with approval of the Falkland Islands Government (Research Permit R16/2012). Adult female samples (n = 23) from Dangerous Reef were collected from 17 September to 21 November 2003, for a demographic study of the female breeding population (McIntosh, 2007). All but one female had recently given birth (pup age range 4-60 d). Dental aging was available for 20 animals (median age 9.5 y, range 5.5-14.5 y). Additional morphometric data were bodyweight (median 84.5 kg, range 65.5-103.5 kg) and standard length (median 159 cm, range 142-169 cm). Blood samples were collected into clot activation tubes and the separated serum stored in liquid nitrogen (-196° C) in the field before longer-term freezing (-80° C). Pup samples were collected as previously described (Lindsay et al., 2021), for health monitoring and hookworm disease investigations. Pup morphometric data included bodyweight (kg) and linear standard length (cm), both proxies for pup age. At Seal Bay, known pup age based on observation of birth or detection of the pup as a newborn, was recorded when available and repeat sampling was performed on a subset of pups. Known-age pups provided a reference to determine indicative pup ages for other cohorts. Simple linear regressions of the age proxies of standard length and bodyweight were made between six cohorts, one each from Dangerous Reef and Olive Island, and four from Seal Bay (initial and repeat samples from pups of known age, initial and repeat samples from pups of unknown age). A Bonferroni correction was used to adjust for multiple comparisons between pairs of the above cohorts.
Sample collection dates for Dangerous Reef (n = 21), Olive Island (n = 65) and Seal Bay (n = 126) were 21 July to 29 August 2017, 15 to 19 September 2017, and 5 March to 8 May 2018, respectively. Serum was separated within 12 h of blood collection after centrifuging plain blood tubes for 2 min at 13,700 g (StatSpin MP, StatSpin Technologies, Norwood, USA). Samples were stored in the field in liquid nitrogen (Dangerous Reef and Olive Island) or frozen at -20° C (Seal Bay), before longer term freezing at -80° C.
Serological testing and analysis
A commercially available modified agglutination test (MAT) (Toxo-Screen DA®, Biomerieux, Marcy-l’Étoile, France) was used to screen sera for T. gondii tachyzoite immunoglobulin G (IgG) antibodies at 1:40 and 1:4,000 dilutions, as per manufacturer directions. Samples (freeze-thaw cycles: adults (1), pups (≤3)) were analyzed between 11 July and 27 September 2018 at the Veterinary Diagnostic Laboratory, The University of Adelaide. Non-specific agglutination was suppressed by including 2-mercaptoethanol (0.2 mol/L) in the dilution buffer. Manufacturer supplied positive and negative goat control sera and phosphate-buffered saline control were included with each MAT test plate. Quantitative assessment of antibody titer was determined for positive sera using sequential doubling of sample dilution (from a 1:40 baseline and commencing at 1:640). Antibody titer was converted to IU/ml as per the manufacturer’s instructions (equivalent to one-tenth of the reciprocal of the highest dilution giving a positive reaction – a higher titer indicating a higher level of antibody).
When seropositive adult females were detected, the association of demographic factors (age, bodyweight, and standard length) with seropositivity was explored using simple logistic regressions. Binomial exact 95% confidence intervals were reported for estimated seroprevalences in this study and calculated for comparative studies using published data. Seroprevalence analyses were conducted using version 17.0 of the Stata software package (StataCorp LLC., College Station, Texas, USA). For pup groups that tested all seronegative, a one-sided 97.5% confidence interval was reported. For these cohorts, the survey sensitivity (SSe) was calculated post-hoc according to the achieved sample size using the online AusVet EpiTools calculator (Sergeant, 2018a). Assumptions were representative sampling, 100% diagnostic test sensitivity and specificity, as well as a 5% design seroprevalence (minimum assumed seroprevalence in an exposed animal group). The population size was considered finite according to referenced pup production estimates for each colony. Calculation of the SSe used a modified hypergeometric exact method and was then used to estimate the probability of pup population freedom from T. gondii exposure (Sergeant, 2018b). Assumptions for probability of freedom estimations were 100% diagnostic (and therefore survey) specificity, as well as a non-informative 50% pre-survey probability of freedom from exposure.
Results
Seven adult female Australian sea lion serum samples (n = 23) collected from Dangerous Reef were seropositive for T. gondii (30.4%; 95% CI 13.2-52.9%). All animals screening positive were positive at the 1:4,000 dilution and the result was confirmed in all cases by subsequent titration. Antibody titers of 512 (n = 3), 1024 (n = 2) and >2048 IU/ml (n = 2) were determined. There was no significant association between age (OR 0.95 [0.67-1.36]), bodyweight (OR 0.96 [0.88-1.05]) or standard length (OR 1.06 [0.92-1.23]), and T. gondii seropositivity.
None of the Australian sea lion pup serum samples (n = 212 samples from 184 animals) collected from Dangerous Reef, Olive Island (both felid-free), and Kangaroo Island (felid-inhabited), were seropositive (0.0%; 97.5% CI 0.0-2.0% for pooled initial sampling: Table 1). None of the pups sampled from any colony was likely to have commenced foraging, based on subjective assessment of age (R. Gray, pers comm). One-third (n = 33) of the 98 pups initially sampled from Seal Bay were of known age (median age 26 d, age range 7-73 d). From these 98 pups there were 28 pups subsequently resampled approximately four weeks later, and half (n = 15) of these pups were of known age (median age 62 d, age range 34-104 d). Multiple pair-wise comparisons of age proxies (bodyweight and standard length: Table 1) found that using either parameter: 1. at initial sampling of both Seal Bay cohorts, the pups of unknown age were significantly larger (older) than the pups of known age (Bonferroni corrected P < 0.05: Supplementary Figure 1); 2. at their initial sampling, the Seal Bay pups of unknown age did not differ significantly from the Seal Bay pups of known age at their subsequent resampling (P > 0.05); 3. the Dangerous Reef pups did not differ significantly from the Seal Bay pups of known age at their initial sampling (Bonferroni corrected P > 0.05):; 4. the Olive Island pups did not differ significantly from the Seal Bay pups of known age at their subsequent resampling (P > 0.05).
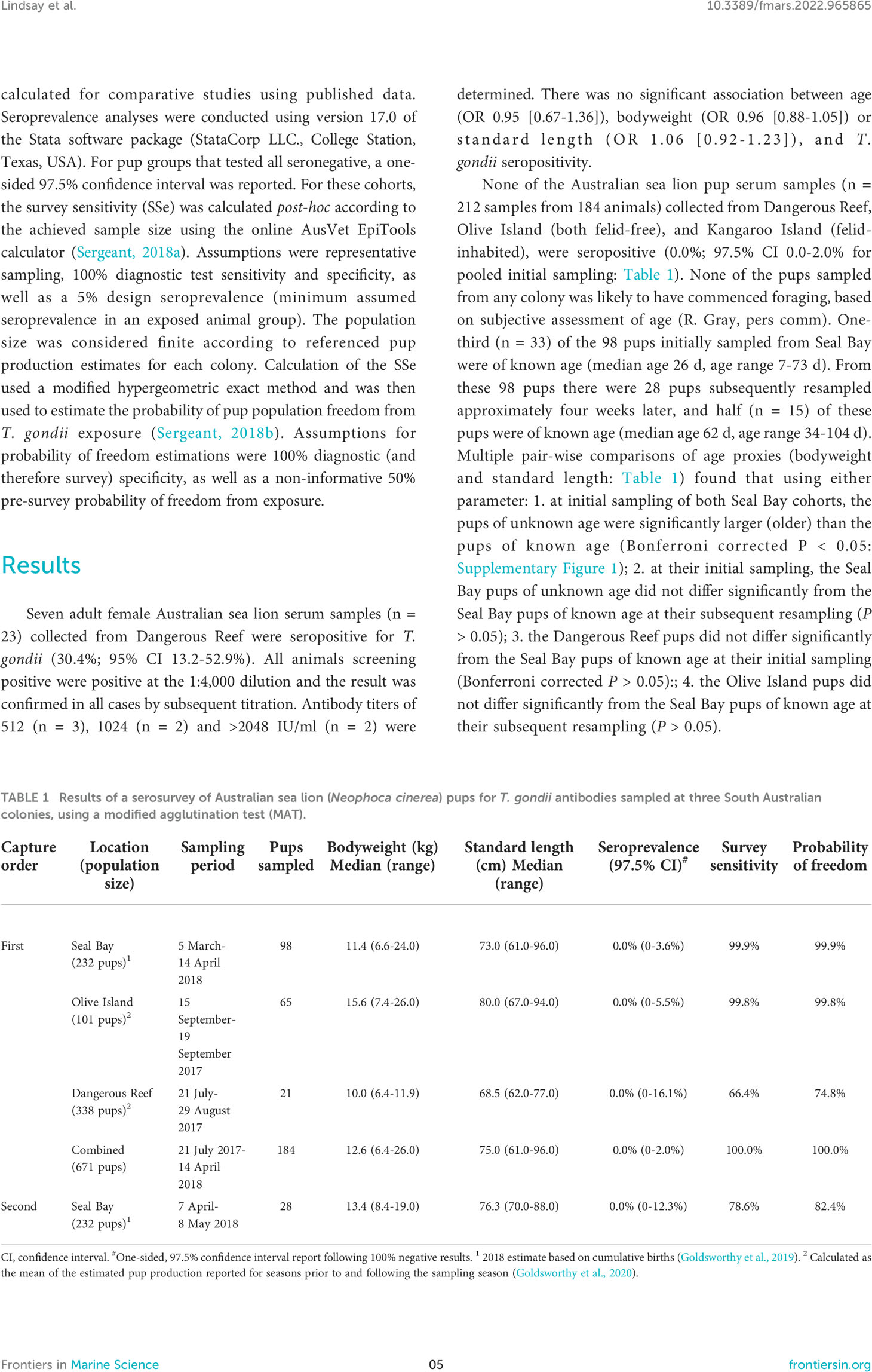
Table 1 Results of a serosurvey of Australian sea lion (Neophoca cinerea) pups for T. gondii antibodies sampled at three South Australian colonies, using a modified agglutination test (MAT).
The probabilities of detecting at least one seropositive pup if the pup cohort had been exposed to T. gondii are reported in Table 1. The resultant probabilities of the seasonal pup population freedom from T. gondii exposure at Dangerous Reef, Olive Island and Seal Bay (initial and subsequent sampling) were 74.8%, 99.8%, 99.9% and 82.4%, respectively. Pooling all first capture negative results provided 100% probability of the combined pre-weaned seasonal pup population (indicative age range 7-104 d) freedom from T. gondii exposure.
Discussion
Disease surveillance in an extensively distributed otariid population such as the Australian sea lion is logistically challenging. Sampling of subadult and adult animals is difficult, requiring either chemical restraint with its safety risks or the unlikely sampling of recently deceased individuals. Access to archived sera from 23 adult females sampled in 2003 was a unique opportunity to explore T. gondii exposure in a mature cohort. Evidence of T. gondii seroconversion in approximately a third of adult females suggests that, even 20 years ago in a felid-free island colony (Dangerous Reef), this cohort’s exposure to T. gondii was frequent although not systematic. Adapting calculations reported by Taggart et al. (2020), the prevalence of prey carrying T. gondii could be as low as 0.001% to achieve an adult female seal seroprevalence of 30.4% (assuming a median cumulative exposure time of 9.0 y and a conservative daily consumption of 10 prey: Supplementary data). That equates to one in every 100,000 prey carrying T. gondii, recognizing that higher prevalence is likely to be clustered in areas where contamination of the marine environment may be higher. By comparison, none of the colony’s pups (n = 21) sampled 14 years later showed evidence of T. gondii exposure. Ideally, paired mother and pup serology would have been analyzed, however, this was not logistically or ethically feasible. Cumulatively across the three surveyed colonies (n = 184) the available serological evidence provided us with approximately absolute confidence (rounded to 100%) that no pups from these colonies had seroconverted and therefore were yet to be exposed to T. gondii.
The estimated 30.4% seroprevalence in adult Australian sea lions approximates that reported in other otariids, including the 32.9% (binomial exact 95% CI 30.6-35.2%: n = 1,630) reported in free-ranging California sea lions stranding along the central California coast between 1998 and 2009 (Carlson-Bremer et al., 2015; Dubey et al., 2020). That assessment, using a 1:40 cut-off and indirect fluorescent antibody methodology was reduced to 2.8% (binomial exact 95% CI 2.1-3.7%) at a cut-off titer of 1:640, determined from confirmed cases (Carlson-Bremer et al., 2015). A similar cut-off more specific for diseased (versus infected) animals remains to be determined for the Australian sea lion. In a regional context, the seroprevalence of antibodies to T. gondii in New Zealand sea lions using latex agglutination testing and a 1:32 cut-off was found to be 20.0% (binomial exact 95% CI 2.5-55.6%: n = 10) on the Otago Peninsula of the New Zealand South Island, and 8.3% (binomial exact 95% CI 0.2-38.5%: n = 12) on nearby Stewart Island (Michael et al., 2016)– both islands having a feral cat population (Roberts et al., 2021). In comparison, anti-T. gondii antibodies were not detected in animals from the remote and felid-free Enderby Island in the subantarctic (one-sided 97.5% CI 0.0-12.3%: n = 28) (Michael et al., 2016) – despite the presence of a feral cat population on the nearby main island of the Auckland Islands (Roberts et al., 2021). The latter finding is consistent with the negative results (one-sided 97.5% CI 0.0-3.5%: n = 104) of the 2007-2008 survey of the Australian fur seal colony (adult females only) on Kanowna Island (-39.1547, 146.3108) in northern Bass Strait (Lynch et al., 2011), and the low 5.3% (binomial exact 95% CI 0.1-26.0%: n = 19) seroprevalence in a 2014 survey of the southern (South American) sea lion colony (Otaria flavescens) (adults of both sexes) inhabiting Big Shag Island (-51.39493, -58.31644) on the north coast of the East Falkland Island (Lindsay and Gray, unpublished data). Both surveys used the same MAT methodology as the present study (with a higher 1:64 cutoff in the fur seal survey).
Differences in prevalence by location, particularly for island colonies, likely reflect varying degrees of seal exposure to T. gondii through prey – a function of parasite prevalence in prey and the quantity of prey consumed. The latter should result in greater cumulative exposure risk with increasing age, which has been documented in multiple pinnipeds (Lambourn et al., 2001; Carlson-Bremer et al., 2015). The absence of an age correlation with seropositivity in the present study is likely due to the small sample size and relatively narrow age range (median age 9.5 y, range 5.5-14.5 y). The parasite prevalence in prey is likely to vary with the species of prey. The Australian sea lion is principally a benthic (ocean floor) forager of Australian continental shelf waters, its temporally and spatially variable diet including many species of cephalopods (e.g., octopus, cuttlefish and squid), benthic or demersal (near the ocean floor) cartilaginous (e.g., sharks and rays) and ray-finned fish (e.g., leatherjackets, salmon and eels), and crustaceans (e.g., rock lobster and swimming crabs) (Peters et al., 2015; Berry et al., 2017). Toxoplasma gondii DNA has been detected in demersal and benthopelagic fish species elsewhere in the world, a Mediterranean basin study finding it in both piscivorous (diet predominantly fish) and omnivorous (diet predominantly small benthic invertebrates or zooplankton) species (Marino et al., 2019). The detection of T. gondii DNA in intestinal and gill samples supports ingestion (gut transit) and filter-feeding, respectively, as mechanisms for acquisition and carriage of the parasite. Increased exposure of benthic fish species to the parasite may result from the concentration of oocysts in the benthos, suggested to be facilitated by marine invertebrates (Aguirre et al., 2019). The benthic foraging behavior of the Australian sea lion is therefore one explanation for its level of exposure to T. gondii.
Variation in T. gondii prevalence in prey is also likely impacted by other factors determining their exposure to the parasite – for instance, regional cat density (reflecting the degree of urbanization for domestic cats and the population density of feral cats), proximity to terrestrial water run-off (e.g., river delta or estuary), factors determining the volume of terrestrial water run-off (e.g., seasonal rainfall and storm events) and ocean currents (given the organism’s prolonged persistence in the marine environment). Unlike males, the foraging range for adult female Australian sea lions is focused on the waters surrounding breeding colonies (Lowther et al., 2011), with inshore and offshore foraging ecotypes identified (Lowther and Goldsworthy, 2011). The waters around Dangerous Reef are impacted by The South Australian current, the easterly flowing current sweeping past some of Australia’s less populous and arid southern coastline (Australian Government Bureau of Meteorology, 2022), and by tidal flows exiting the Spencer Gulf and potentially the smaller adjacent and more populous Gulf St Vincent (Australia’s Integrated Marine Observing System (IMOS), 2021). A high rate of domestic cat ownership among a predominantly coastally distributed population is therefore a possible source of oocyst contamination of the marine environment around the felid-free island of Dangerous Reef.
Some of Australia’s other coastal islands, such as Kangaroo Island, (Taggart et al., 2019a; Algar et al., 2020) have a high feral cat population with a high seroprevalence of T. gondii (87-89%) (O'Callaghan et al., 2005). Evidence for these animals substantially contributing to biological environmental contamination comes from the high prevalence of cat-borne parasitic diseases (toxoplasmosis and sarcocystosis) in the island’s domestic sheep flocks (Taggart et al., 2019b; Lanyon and O'Handley, 2020). Consequently, a serosurvey of pups from the Seal Bay colony on Kangaroo Island was included in the present study to better understand any direct health implications for the colony’s pup population, and as a comparator to the serosurvey results from the two felid-free islands. The absence of exposure evidence for the pup population (and the positive serosurvey of adults from a regional felid-free island) suggest survey of the juvenile and adult cohorts (ideally including mother-pup pairings) is necessary to better understand the age-related level of T. gondii exposure in this colony. This could guide risk assessment for epizootic disease outbreak should other factors like comorbidity alter the existing host-parasite balance and inform the scope and effectiveness of the feral cat eradication program recently implemented on the island (Commonwealth of Australia, 2015; Landscape South Australia, 2022). Furthermore, comparison of T. gondii genotype from clinical toxoplasmosis cases in seals with that in feral (and domestic) cats may add further to our understanding of disease epidemiology.
While acknowledging the potential for selection and survival bias, an absence of pup seropositivity in the present study excludes detection of early postnatal infection in pre-weaned pups yet to commence prey ingestion, the transplacental vertical transmission of infection from mother to pup, and the transplacental or colostrum transfer of maternal IgG antibodies (or their lack of persistence to sampling age). For seal pups exposed to T. gondii oocysts in the postnatal period, seroconversion with MAT-detectable antibodies occurs within three weeks (Gajadhar et al., 2004). The seropositivity of approximately 8-12 m old California sea lion pups (weaning between 6-11 m of age) was attributed to commencement of foraging trips and their initial ingestion of prey (Carlson-Bremer et al., 2015). The transplacental vertical transmission of T. gondii to an early or late-stage fetus is less commonly reported but has been detected in marine mammals such as Risso’s dolphin (Resendes et al., 2002) and the California sea lion (Carlson-Bremer et al., 2015).
The adaptation of neonates to the extrauterine environment and their protection from infectious agents is variably reliant on the passive transfer of maternal antibodies across the placenta in utero, or in colostrum soon after birth (reviewed in Chucri et al., 2010). Pinnipeds have an endotheliochorial placenta (Sinha and Erickson, 1974), and typically only 5-10% of maternal IgG antibodies undergo transfer to the fetus in placentas of this type (Tizard, 2018). Acquisition of passive immunity in pinniped pups is likely to be highly dependent on adequate ingestion and absorption of colostrum. The exact duration of persistence of maternal antibodies in pinniped pups is not known, however it is likely to be short, perhaps a few weeks (Harder et al., 1993; Puryear et al., 2021). Toxoplasma gondii seropositivity has been detected in a 14 d old grey seal (Halichoerus grypus) pup along with seronegativity in two 18-19 d old pups, all three belonging to seropositive females (Measures et al., 2004). Failure to detect maternal antibodies in pups in the present study may therefore reflect limited sampling of pups in the first two weeks of life – partly attributed to protective maternal (and aggressive male mate-guard) attendance for the first 9-10 days after birth. The importance of sampling age is also supported by a seroprevalence study of bearded seals in Norway, which included 65 pre-weaned pups, all of whom tested negative, while two-thirds (n = 15) of adults tested positive (Jensen et al., 2009). This included six seropositive females, all nursing seronegative pups of unspecified age. Recommendations for subsequent T. gondii serosurveys of Australian sea lion pups include sampling of stillbirths and perinatal deaths less than two weeks old, and histologic and immunohistochemical examination of placental tissues followed by PCR confirmation of the presence of T. gondii DNA. Detection of recent births and collection of well-preserved placental tissues is only feasible at a closely monitored colony such as Seal Bay. The number of observed stillborn animals at this colony is historically low, while perinatal death within the first two weeks is a more common occurrence, often the result of conspecific trauma, endemic hookworm infection or starvation (R. Gray, pers comm).
Storage time of the adult sera is a potential limitation of the present study, although results are unlikely to have been impacted given their storage at -80C. Reliable serological measurement of anti-toxoplasma antibodies in human sera has been observed for at least six years in samples stored at -20° C (Dard et al., 2017). A lack of validation in pinniped species of the MAT test is an additional study limitation, although it has been the most used method for serological surveys in these species over the past decade (Dubey et al., 2020), at cut-off values ranging from 1:25 to 1:65. The cut-off value of 1:40 for the present study was selected to best align with previous investigations in these species. MAT has not been validated in other marine mammals either, although in northern sea otters (Enhydra lutris kenyoni) for instance, 95% (n = 20) of T. gondii isolates in a study of that species were from animals testing seropositive by MAT (Verma et al., 2018). This included one animal seropositive at 1:100 dilution. Comparatively, all seropositive animals in the present study had high titers – positive at >1:4,000 dilution. The MAT methodology has been suggested to overestimate seroprevalence in the presence of sample hemolysis or a high serum lipid content, reducing specificity (Blanchet et al., 2014; van de Velde et al., 2016). Of note was the absence of any false positive results for the pup population, in which grossly detectable lipemia is frequently observed following recent feeding.
The endangered Australian sea lion population has failed to recover to 18th century pre-sealing abundance, despite its protected status. Given the contribution of T. gondii to limiting population recovery in other marine mammals and the role of high order predators as sentinels for marine contamination (Bossart, 2011), ongoing serological surveillance is recommended with expansion to include other infectious diseases likely to compromise population resilience.
Data availability statement
The raw data supporting the conclusions of this article will be made available by the authors, without undue reservation.
Ethics statement
The animal studies were reviewed and approved by The University of Sydney (Pups - Protocol Number 2014/726) and La Trobe University Animal Ethics Committees (Adults - Permit AEC00/42(L)).
Author contributions
SL and RG designed (with additional input from JS) and executed the study and collected pup samples. SL performed the laboratory testing (with additional input from RO), curated the data, and wrote the initial manuscript draft. SL and CC analyzed the data. All authors contributed to the manuscript and approved the submitted version.
Funding
This study was supported in part by The Hermon Slade Foundation (HSF 16-03), and by the FS Quiney and BR Richards bequests (Sydney School of Veterinary Science, The University of Sydney: 2016).
Acknowledgments
The authors greatly appreciate the provision of adult Australian sea lion serum samples and morphometric data by Rebecca McIntosh (Phillip Island Nature Parks, Cowes, Victoria, Australia) and Simon Goldsworthy (South Australian Research and Development Institute, West Beach, South Australia, Australia). We also appreciate the provision of adult southern sea lion serum samples by Alastair Baylis (South Atlantic Environmental Research Institute, Stanley, Falkland Islands). We thank Mariel Fulham, Shannon Taylor, and Matthew Gray for their assistance with sample collection from Australian sea lion pups. We also thank the staff of the South Australian Department for Environment and Water (DEW), Seal Bay, Kangaroo Island, for their logistical support and fieldwork assistance.
Conflict of interest
The authors declare that the research was conducted in the absence of any commercial or financial relationships that could be construed as a potential conflict of interest.
Publisher’s note
All claims expressed in this article are solely those of the authors and do not necessarily represent those of their affiliated organizations, or those of the publisher, the editors and the reviewers. Any product that may be evaluated in this article, or claim that may be made by its manufacturer, is not guaranteed or endorsed by the publisher.
Supplementary material
The Supplementary Material for this article can be found online at: https://www.frontiersin.org/articles/10.3389/fmars.2022.965865/full#supplementary-material
References
Aguirre A. A., Longcore T., Barbieri M., Dabritz H., Hill D., Klein P. N., et al. (2019). The one health approach to toxoplasmosis: epidemiology, control, and prevention strategies. Ecohealth 16, 378–390. doi: 10.1007/s10393-019-01405-7
Ahmadpour E., Rahimi M. T., Ghojoghi A., Rezaei F., Hatam-Nahavandi K., Oliveira S. M. R., et al. (2022). Toxoplasma gondii infection in marine animal species, as a potential source of food contamination: a systematic review and meta-analysis. Acta Parasitol 67, 592–605. doi: 10.1007/s11686-021-00507-z
Algar D., Johnston M., Tiller C., Onus M., Fletcher J., Desmond G., et al. (2020). Feral cat eradication on Dirk Hartog island, Western Australia. Biol. Invasions 22, 1037–1054. doi: 10.1007/s10530-019-02154-y
Australian Government Bureau of Meteorology (2022) Forecast help. Available at: http://www.bom.gov.au/oceanography/forecasts/forecast-help.shtml (Accessed 23 March 2022). Australian Government.
Australia’s Integrated Marine Observing System (IMOS) (2021) Ocean current: tides. Available at: http://oceancurrent.imos.org.au/tides/SA_spd/2021/2021122100.html (Accessed 24 March 2022).
Berry T. E., Osterrieder S. K., Murray D. C., Coghlan M. L., Richardson A. J., Grealy A. K., et al. (2017). DNA Metabarcoding for diet analysis and biodiversity: A case study using the endangered Australian sea lion (Neophoca cinerea). Ecol. Evol. 7 (14), 5435–5453. doi: 10.1002/ece3.3123
Bigot-Clivot A., La Carbona S., Cazeaux C., Durand L., Géba E., Le Foll F., et al. (2022). Blue mussel (Mytilus edulis) - a bioindicator of marine water contamination by protozoa: laboratory and in situ approaches. J. Appl. Microbiol. 132, 736–746. doi: 10.1111/jam.15185
Bilgmann K., Armansin N., Ferchaud A. L., Normandeau E., Bernatchez L., Harcourt R., et al. (2021). Low effective population size in the genetically bottlenecked Australian sea lion is insufficient to maintain genetic variation. Anim. Conserv. 24, 847–861. doi: 10.1111/acv.12688
Blanchet M. A., Godfroid J., Breines E. M., Heide-Jorgensen M. P., Nielsen N. H., Hasselmeier I., et al. (2014). West Greenland Harbour porpoises assayed for antibodies against Toxoplasma gondii: false positives with the direct agglutination method. Dis. Aquat. Organ 108, 181–186. doi: 10.3354/dao02715
Bossart G. D. (2011). Marine mammals as sentinel species for oceans and human health. Vet. Pathol. 488, 676–690. doi: 10.1177/0300985810388525
Campbell R. A., Gales N. J., Lento G. M., Baker C. S. (2008). Islands in the sea: extreme female natal site fidelity in the Australian sea lion, Neophoca cinerea. Biol. Lett. 4, 139–142. doi: 10.1098/rsbl.2007.0487
Campbell K., Paparini A., Gomez A. B., Cannell B., Stephens N. (2022). Fatal toxoplasmosis in little penguins (Eudyptula minor) from penguin island, Western Australia. Int. J. Parasitol. Parasites Wildl. 17, 211–217. doi: 10.1016/j.ijppaw.2022.02.006
Carlson-Bremer D., Colegrove K. M., Gulland F. M., Conrad P. A., Mazet J. A., Johnson C. K. (2015). Epidemiology and pathology of Toxoplasma gondii in free-ranging California sea lions (Zalophus californianus). J. Wildl. Dis. 51, 362–373. doi: 10.7589/2014-08-205
Chucri T. M., Monteiro J. M., Lima A. R., Salvadori M. L., Kfoury J. R. Jr., Miglino M. A. (2010). A review of immune transfer by the placenta. J. Reprod. Immunol. 87 (1-2), 14–20. doi: 10.1016/j.jri.2010.08.062
Commonwealth of Australia (2015) Threat abatement plan for predation by feral cats, commonwealth of Australia. Available at: https://www.dcceew.gov.au/environment/biodiversity/threatened/publications/tap/threat-abatement-plan-feral-cats (Accessed 21 April 2022).
Coupe A., Howe L., Burrows E., Sine A., Pita A., Velathanthiri N., et al. (2018). First report of Toxoplasma gondii sporulated oocysts and Giardia duodenalis in commercial green-lipped mussels (Perna canaliculus) in new Zealand. Parasitol. Res. 117, 1453–1463. doi: 10.1007/s00436-018-5832-8
Dard C., Bailly S., Drouet T., Fricker-Hidalgo H., Brenier-Pinchart M. P., Pelloux H. (2017). Long-term sera storage does not significantly modify the interpretation of toxoplasmosis serologies. J. Microbiol. Methods 134, 38–45. doi: 10.1016/j.mimet.2017.01.003
Donahoe S. L., Rose K., Slapeta J. (2014). Multisystemic toxoplasmosis associated with a type II-like Toxoplasma gondii strain in a New Zealand fur seal (Arctocephalus forsteri) from New South Wales, Australia. Vet. Parasitol. 205, 347–353. doi: 10.1016/j.vetpar.2014.07.022
Dubey J. P., Murata F. H. A., Cerqueira-Cézar C. K., Kwok O. C. H., Grigg M. E. (2020). Recent epidemiologic and clinical importance of Toxoplasma gondii infections in marine mammals: 2009–2020. Veterinary Parasitol. 288, 109296. doi: 10.1016/j.vetpar.2020.109296
Fayer R., Dubey J. P., Lindsay D. S. (2004). Zoonotic protozoa: from land to sea. Trends Parasitol. 20, 531–536. doi: 10.1016/j.pt.2004.08.008
Gajadhar A. A., Measures L., Forbes L. B., Kapel C., Dubey J. P. (2004). Experimental Toxoplasma gondii infection in grey seals (Halichoerus grypus). J. Parasitol. 90, 255–259. doi: 10.1645/GE-144R
Gales N. J., Shaughnessy P. D., Dennis T. E. (1994). Distribution, abundance and breeding cycle of the Australian sea lion Neophoca cinerea (Mammalia: Pinnipedia). J. Zoology 234, 353–370. doi: 10.1111/j.1469-7998.1994.tb04853.x
Goldsworthy S. D., Hodgson J., Holman D. (2020). Australian Sea lion investigations: 2018-19 (Adelaide: South Australian Research and Development Institute (Aquatic Sciences). SARDI Publication No. F2020/000052-1. SARDI Research Report Series No. 1051.
Goldsworthy S. D., Shaughnessy P. D., Smart J., Mackay A., Bailleul F., Reinhold S.-L., et al. (2019). Monitoring of Seal Bay and other populations on Kangaroo Island: 2017/2018 (Adelaide: Report to the Department for Environment and Water. South Australian Research and Development Institute (Aquatic Sciences), 59pp. SARDI Publication No. F2014000322-5. SARDI Research Report Series No. 1018.
Harder T. C., Stede M., Willhaus T., Schwarz J., Heidemann G., Liess B. (1993). Morbillivirus antibodies of maternal origin in harbour seal pups (Phoca vitulina). Vet. Rec. 132, 632–633. doi: 10.1136/vr.132.25.632
Jensen S. K., Aars J., Lydersen C., Kovacs K. M., Åsbakk K. (2009). The prevalence of Toxoplasma gondii in polar bears and their marine mammal prey: evidence for a marine transmission pathway? Polar. Biol. 33, 599–606. doi: 10.1007/s00300-009-0735-x
Jensen S. K., Nymo I. H., Forcada J., Godfroid J., Hall A. (2012). Prevalence of Toxoplasma gondii antibodies in pinnipeds from Antarctica. Vet. Rec. 171, 249. doi: 10.1136/vr.100848
Kabay M. J. (1996). Toxoplasmosis in a sea lion (Neophoca cinerea). Available at: https://aapspextranet.animalhealthaustralia.com.au/wp-content/uploads/2019/04/43_march1996.pdf [Accessed 25 August 2022]. Australian Society for Veterinary Pathology.
Kreuder C., Miller M. A., Jessup D. A., Lowenstine L. J., Harris M. D., Ames J. A., et al. (2003). Patterns of mortality in southern sea otters (Enhydra lutris nereis) from 1998-2001. J. Wildl. Dis. 39, 495–509. doi: 10.7589/0090-3558-39.3.495
Lambourn D. M., Jeffries S. J., Dubey J. P. (2001). Seroprevalence of Toxoplasma gondii in harbor seals (Phoca vitulina) in southern Puget Sound, Washington. J. Parasitol. 87, 1196–1197. doi: 10.1645/0022-3395(2001)087[1196:Sotgih]2.0.Co;2
Landscape South Australia (2022) Kangaroo Island feral cat eradication program. Available at: https://www.landscape.sa.gov.au/ki/pest-management/kangaroo-island-feral-cat-eradication-program (Accessed 9 March 2022).
Lanyon S. R., O'Handley R. M. (2020). Relationship between Toxoplasma gondii seroprevalence and lamb marking in South Australian sheep flocks. Aust. Vet. J. 98, 525–528. doi: 10.1111/avj.13004
Legge S., Murphy B. P., McGregor H., Woinarski J. C. Z., Augusteyn J., Ballard G., et al. (2017). Enumerating a continental-scale threat: how many feral cats are in Australia? Biol. Conserv. 206, 293–303. doi: 10.1016/j.biocon.2016.11.032
Lindsay S. A., Caraguel C. G. B., Gray R. (2021). Topical ivermectin is a highly effective seal 'spot-on': A randomised trial of hookworm and lice treatment in the endangered Australian sea lion (Neophoca cinerea). Int. J. Parasitol. Parasites Wildl. 16, 275–284. doi: 10.1016/j.ijppaw.2021.11.002
Lindsay D. S., Collins M. V., Mitchell S. M., Cole R. A., Flick G. J., Wetch C. N., et al. (2003). Sporulation and survival of Toxoplasma gondii oocysts in seawater. J Eukaryot Microbiol 50 Suppl, 687–688. doi: 10.1111/j.1550-7408.2003.tb00688.x
Lindsay D. S., Dubey J. P. (2009). Long-term survival of Toxoplasma gondii sporulated oocysts in seawater. J. Parasitol. 95 (4), 1019–1020. doi: 10.1645/GE-1919.1
Lowther A. D., Goldsworthy S. D. (2011). Detecting alternate foraging ecotypes in Australian sea lion (Neophoca cinerea) colonies using stable isotope analysis. Mar. Mammal. Sci. 27, 567–586. doi: 10.1111/j.1748-7692.2010.00425.x
Lowther A., Goldsworthy S. (2012). Head start: Australian sea lion pups gain experience of adult foraging grounds before weaning. Mar. Biol. 159, 2687–2696. doi: 10.1007/s00227-012-2026-2
Lowther A. D., Harcourt R. G., Hamer D. J., Goldsworthy S. D. (2011). Creatures of habit: foraging habitat fidelity of adult female Australian sea lions. Mar. Ecol. Prog. Ser. 443, 249–263. doi: 10.3354/meps09392
Lynch M., Nielsen O., Duignan P. J., Kirkwood R., Hoskins A., Arnould J. P. (2011). Serologic survey for potential pathogens and assessment of disease risk in Australian fur seals. J. Wildl. Dis. 47, 555–565. doi: 10.7589/0090-3558-47.3.555
Marino A. M. F., Giunta R. P., Salvaggio A., Castello A., Alfonzetti T., Barbagallo A., et al. (2019). Toxoplasma gondii in edible fishes captured in the Mediterranean basin. Zoonoses Public Health 66, 826–834. doi: 10.1111/zph.12630
Mazzariol S., Centelleghe C., Petrella A., Marcer F., Beverelli M., Di Francesco C. E., et al. (2021). Atypical toxoplasmosis in a Mediterranean monk seal (Monachus monachus) pup. J. Comp. Pathol. 184, 65–71. doi: 10.1016/j.jcpa.2021.02.005
McIntosh R. R. (2007). Life history and population demographics of the Australian sea lion (Melbourne (Vic: La Trobe University).
Measures L. N., Dubey J. P., Labelle P., Martineau D. (2004). Seroprevalence of Toxoplasma gondii in Canadian pinnipeds. J. Wildl. Dis. 40, 294–300. doi: 10.7589/0090-3558-40.2.294
Michael S. A., Howe L., Chilvers B. L., Morel P., Roe W. D. (2016). Seroprevalence of Toxoplasma gondii in mainland and sub-Antarctic New Zealand sea lion (Phocarctos hookeri) populations. N Z Vet. J. 64, 293–297. doi: 10.1080/00480169.2016.1191974
Miller M. A., Gardner I. A., Kreuder C., Paradies D. M., Worcester K. R., Jessup D. A., et al. (2002). Coastal freshwater runoff is a risk factor for Toxoplasma gondii infection of southern sea otters (Enhydra lutris nereis). Int. J. Parasitol. 32(8), 997–1006. doi: 10.1016/s0020-7519(02)00069-3
Miller M. A., Moriarty M. E., Henkel L., Tinker M. T., Burgess T. L., Batac F. I., et al. (2020). Predators, disease, and environmental change in the nearshore ecosystem: mortality in southern sea otters (Enhydra lutris nereis) from 1998–2012. Front. Mar. Sci. 7. doi: 10.3389/fmars.2020.00582
O'Callaghan M., Reddin J., Lehmann D. (2005). Helminth and protozoan parasites of feral cats from Kangaroo Island. Trans. R. Soc. South Australia Incorporated 129, 81–83.
Poulle M.-L., Le Corre M., Bastien M., Gedda E., Feare C., Jaeger A, et al. (2021). Exposure of pelagic seabirds to Toxoplasma gondii in the Western Indian ocean points to an open sea dispersal of this terrestrial parasite. PloS One 16 (8), e0255664. doi: 10.1371/journal.pone.0255664
Peters K. J., Ophelkeller K., Bott N. J., Deagle B. E., Jarman S. N., Goldsworthy S. D. (2015). Fine-scale diet of the Australian sea lion (Neophoca cinerea) using DNA-based analysis of faeces. Mar. Ecol. 36, 347–367. doi: 10.1111/maec.12145
Puryear W., Sawatzki K., Bogomolni A., Hill N., Foss A., Stokholm I., et al. (2021). Longitudinal analysis of pinnipeds in the northwest Atlantic provides insights on endemic circulation of phocine distemper virus. Proc. Biol. Sci. 288, 20211841. doi: 10.1098/rspb.2021.1841
Reisfeld L., Sacristán C., Ferreira Machado E., Sánchez-Sarmiento A. M., Costa-Silva S., Ewbank A. C., et al. (2019). Toxoplasmosis and sarcocystis spp. infection in wild pinnipeds of the Brazilian coast. Dis. Aquat. Organ 136, 235–241. doi: 10.3354/dao03410
Resendes A. R., Almería S., Dubey J. P., Obón E., Juan-Sallés C., Degollada E., et al. (2002). Disseminated toxoplasmosis in a Mediterranean pregnant Risso's dolphin (Grampus griseus) with transplacental fetal infection. J. Parasitol. 88, 1029–1032. doi: 10.1645/0022-3395(2002)088[1029:Dtiamp]2.0.Co;2
Roberts J. O., Jones H. F. E., Roe W. D. (2021). The effects of Toxoplasma gondii on New Zealand wildlife: implications for conservation and management. Pac. Conserv. Biol. 27, 208–220. doi: 10.1071/pc20051
Roe W. D., Howe L., Baker E. J., Burrows L., Hunter S. A. (2013). An atypical genotype of Toxoplasma gondii as a cause of mortality in Hector's dolphins (Cephalorhynchus hectori). Vet. Parasitol. 192, 67–74. doi: 10.1016/j.vetpar.2012.11.001
Roe W. D., Michael S., Fyfe J., Burrows E., Hunter S. A., Howe L. (2017). First report of systemic toxoplasmosis in a New Zealand sea lion (Phocarctos hookeri). N Z Vet. J. 65, 46–50. doi: 10.1080/00480169.2016.1230526
Sepulveda M. A., Seguel M., Alvarado-Rybak M., Verdugo C., Munoz-Zanzi C., Tamayo R. (2015). Postmortem findings in four South American sea lions (Otaria byronia) from an urban colony in Valdivia, Chile. J. Wildl. Dis. 51 (1), 279–282. doi: 10.7589/2013-07-161
Sergeant E. S. G. (2018a) Epitools epidemiological calculators: population or cluster level sensitivity. Available at: https://epitools.ausvet.com.au/populationse (Accessed 8 April 2022). Ausvet.
Sergeant E. S. G. (2018b) Epitools epidemiological calculators: confidence of freedom following negative surveillance. Available at: https://epitools.ausvet.com.au/pfree (Accessed 8 April 2022). Ausvet.
Shapiro K., Krusor C., Mazzillo F. F., Conrad P. A., Largier J. L., Mazet J. A., et al. (2014). Aquatic polymers can drive pathogen transmission in coastal ecosystems. Proc. Biol. Sci. 281 (1795), 20141287. doi: 10.1098/rspb.2014.1287
Shapiro K., Miller M. A., Packham A. E., Aguilar B., Conrad P. A., Vanwormer E., et al. (2016). Dual congenital transmission of Toxoplasma gondii and Sarcocystis neurona in a late-term aborted pup from a chronically infected southern sea otter (Enhydra lutris nereis). Parasitology 143, 276–288. doi: 10.1017/S0031182015001377
Sinha A. A., Erickson A. W. (1974). Ultrastructure of the placenta of Antarctic seals during the first third of pregnancy. Am. J. Anat. 141, 263–279. doi: 10.1002/aja.1001410208
Taggart P. L., Caraguel C. G. B., McAllister M. M. (2020). Fractional seroprevalence rates in common prey species can cause more than half of feral cats to be exposed to Toxoplasma gondii annually. Vet. Parasitol. 288, 109306. doi: 10.1016/j.vetpar.2020.109306
Taggart P., Fancourt B., Bengsen A., Peacock D., Hodgens P., Read J., et al. (2019a). Evidence of significantly higher island feral cat abundance compared with the adjacent mainland. Wildl. Res. 46, 378–385. doi: 10.1071/WR18118
Taggart P. L., Stevenson M. A., Firestone S. M., McAllister M. M., Caraguel C. G. B. (2019b). Spatial analysis of a cat-borne disease reveals that soil pH and clay content are risk factors for sarcocystosis in sheep. Front. Vet. Sci. 6. doi: 10.3389/fvets.2019.00127
Threatened Species Scientific Committee (2020) Conservation advice. Neophoca cinerea, Australian sea lion. Department of Agriculture, Water and the Environment, Canberra. Available at: www.environment.gov.au/biodiversity/threatened/species/pubs/22-conservation-advice-23122020.pdf (Accessed 29 August 2021).
Tizard I. R. (2018). "Immunity in the fetus and newborn," in Veterinary Immunology. tenth edition (St. Louis, Missouri: Elsevier), 247–260.
van de Velde N., Devleesschauwer B., Leopold M., Begeman L., Ijsseldijk L., Hiemstra S., et al. (2016). Toxoplasma gondii in stranded marine mammals from the North Sea and Eastern Atlantic Ocean: findings and diagnostic difficulties. Veterinary Parasitol. 230, 25–32. doi: 10.1016/j.vetpar.2016.10.021
Keywords: antibody, Australian sea lion, Neophoca cinerea, seal, pinniped, serology, toxoplasma gondii
Citation: Lindsay SA, Caraguel CGB, O’Handley R, Šlapeta J and Gray R (2022) Toxoplasma gondii seroprevalence in the endangered Australian sea lion (Neophoca cinerea). Front. Mar. Sci. 9:965865. doi: 10.3389/fmars.2022.965865
Received: 10 June 2022; Accepted: 16 August 2022;
Published: 05 September 2022.
Edited by:
Eric N. Villegas, United States Environmental Protection Agency, United StatesReviewed by:
Raquel Xavier, Centro de Investigacao em Biodiversidade e Recursos Geneticos (CIBIO-InBIO), PortugalJitender Dubey, Agricultural Research Service (USDA), United States
Copyright © 2022 Lindsay, Caraguel, O’Handley, Šlapeta and Gray. This is an open-access article distributed under the terms of the Creative Commons Attribution License (CC BY). The use, distribution or reproduction in other forums is permitted, provided the original author(s) and the copyright owner(s) are credited and that the original publication in this journal is cited, in accordance with accepted academic practice. No use, distribution or reproduction is permitted which does not comply with these terms.
*Correspondence: Rachael Gray, cmFjaGFlbC5ncmF5QHN5ZG5leS5lZHUuYXU=