- 1State Key Laboratory of Water Environment Simulation, School of Environment, Beijing Normal University, Beijing, China
- 2Environmental Development Center, Ministry of Ecology and Environment, Beijing, China
The relationship between Cd and soil phosphatase activity has been given some concerns due to serious soil Cd contamination. However, the effects of high-risk Cd pollution on the soil phosphorus mineralization process are still kept unclear in reclaimed coastal wetlands. Here, we investigated the impacts of Cd additions at three levels on phosphorus mineralization and phosphatase activities in reclaimed coastal wetland soils with different reclamation ages (e.g., 100-year, 40-year, and 10-year) in the Pearl River Estuary by a 40-day laboratory incubation experiment. The results showed that lower soil phosphatase activity was observed in the reclaimed wetlands with longer reclamation age, which led to the lower increase in cumulative net phosphorus mineralization and Occluded P. High Cd exposure facilitated the phosphatase activities and phosphorus mineralization through the promotion of Occluded P and Al/Fe-P contents in the 100-year and 10-year reclaimed wetland soils, respectively. The addition of the low-dose of Cd to the soil inhibited phosphorus mineralization in the middle of incubation in 40-year reclaimed wetland soils, while the high-dose Cd addition had little effect. The findings of this work indicate that the older reclaimed wetlands might have lower phosphorus mineralization potentials, while higher Cd pollution might lead to soil phosphorus loss by improving phosphorus mineralization in reclaimed coastal wetlands.
Introduction
Phosphorus (P) is one of the most essential elements for plants (Bünemann, 2015). The organic P (Po) mineralization is a fundamental mechanism for regulating P release in soil/sediment and provides a potential mineral P supply for plant growth (Spohn and Kuzyakov, 2013; Bai et al., 2019). In wetland ecosystems, an in-depth understanding of the soil P mineralization process is critical to uncovering the relationships between P cycles and primary productivity, plant uptake, and potential P export to surface water (Fellman and D'Amore, 2007). Over the past few decades, coastal wetlands have been affected by the sea-level rise in the context of global climate change and human activities such as sewage discharge and reclamation (Sun et al., 2015), which will greatly affect the biogeochemical processes of P and further influence coastal wetland ecosystem health.
Over the past thousand years, coastal reclamation has been one of the important ways to replenish coastal arable lands and more than 10 million hectares of tidal flats have been reclaimed along the coast of China (Li et al., 2012). Coastal wetlands have been enclosed by thousands of kilometers of new “Great Wall” (Ma et al., 2014). Many previous studies have illustrated that coastal wetland reclamation could lead to significant changes in soil nutrients and heavy metals (Yin et al., 2017; Zhang et al., 2019; Lu et al., 2020). Yin et al. (2017) observed a significant decrease in P contained within primary minerals (DeP) and an increase in authigenic P contents after the reclamation. Moreover, the reclaimed wetlands always have a higher risk of heavy metal pollution due to intensified human activities such as leaching desalination and excessive agrochemical applications (Reddy and DeLaune, 2008). Cao et al. (2020) presented that the coastal reclamation led to a moderately polluted state of heavy metals compared to geochemical background values from the 1980s based on the multi-metal Nemerow pollution index. Especially, higher Cadmium (Cd) pollution risk has been widely reported in farmlands and coastal wetlands in China (Yuan et al., 2021; Li et al., 2022). Severe soil Cd pollution in coastal reclaimed wetlands has also been observed and attracted widespread attention (Lu et al., 2020; Chen et al., 2022). Generally, in coastal areas of eastern China, salt marsh reclamation increased Cd contents in soils that were 6.9 times higher than the background value, with highest pollution risk among all heavy metals studied (Chen et al., 2022). However, the effects of high-risk Cd pollution in reclaimed coastal wetland soils, on the soil phosphorus mineralization process are still kept unclear.
The soil P mineralization-immobilization dynamics are dominated by microbial activities and phosphatase secreted by microorganisms and plants (Oehl et al., 2001; Margalef et al., 2021). At present, there is no consistent conclusion about the effects of Cd on phosphatase (Cui et al., 2021). Quiquampoix and Mousain (2005) and Yang et al. (2016) reported Cd stimulates phosphatase activity, whereas some researchers observed the inhibiting effect of Cd (Pan and Yu, 2011; Xian et al., 2015). For microbial cells, Cd is capable of producing toxic effects (Aponte et al., 2020), while the mechanisms of Cd toxicity to microorganisms related to P cycling in reclaimed coastal wetlands are not clearly understood. However, little information is available on the effects of Cd on phosphatase activity and the relationship between the P mineralization process and phosphatase activity in coastal reclaimed wetland soils. Therefore, it is necessary to investigate the effects of Cd on the soil P mineralization process in coastal reclaimed wetlands in order to fill the knowledge gap.
Here, we are presenting a study about the effects of Cd with different levels on the P mineralization process and phosphatase activity in coastal reclaimed wetland soils along a 100-year reclamation chronosequence in the Pearl River Estuary (PRE) of China. Due to economic and social development demands, reclamation activities have a long history of over 100 years in this region (Bai et al., 2015; Han et al., 2019), which led to the reclaimed tidal flat soils in the PRE being heavily contaminated by heavy metals (Li et al., 2012). An investigation in the PRE by Gu et al. (2014) found that Cd contents in 97% of soil samples exceeded the class II criteria and 31% of samples above the class III criteria according to the soil quality standards in China (GB 156181995), and Cd pollution appeared to be the most serious. The primary objectives of this work were (1) to compare the impacts of different Cd additions on soil cumulative net mineralization of P, net P mineralization rate, inorganic P (Pi) fractions, alkaline phosphatase (ALP) activity, and acid phosphatase (ACP) activity in reclaimed wetlands with different reclamation ages in the PRE, (2) to explore how reclamation age regulates the impacts of Cd on soil P mineralization process, and (3) to identify the relationships among the reclamation age, phosphatase activity and soil P-related indicators in reclaimed coastal wetlands.
Materials and methods
Study area and soil sampling
The PRE has a subtropical marine monsoon climate, with the annual average temperature and precipitation of 21.4 ~ 22.4°C and 1600 ~ 2300 mm, respectively (Han et al., 2019). There is a complex river network with an average annual runoff of 336 × 109 m3 in the PRE (Liu et al., 2017). According to the Soil Taxonomy (Iuss Working Group, 2006), the dominant soil types in the PRE are Mollic Fluvisol, Eutric Geysol, and Irragric Anthrosols (Han et al., 2019). Large-scale reclamation activities have happened in the Nansha District and approximately 554 km2 of tidal flats have been reclaimed as croplands (Xiao et al., 2015), which were mainly planted bananas, sugarcane, longan, pawpaw, etc. (Han et al., 2019). The reclaimed wetlands with different reclamation ages were selected as study areas in the Nansha District of Guangzhou, South China (Figure 1A).
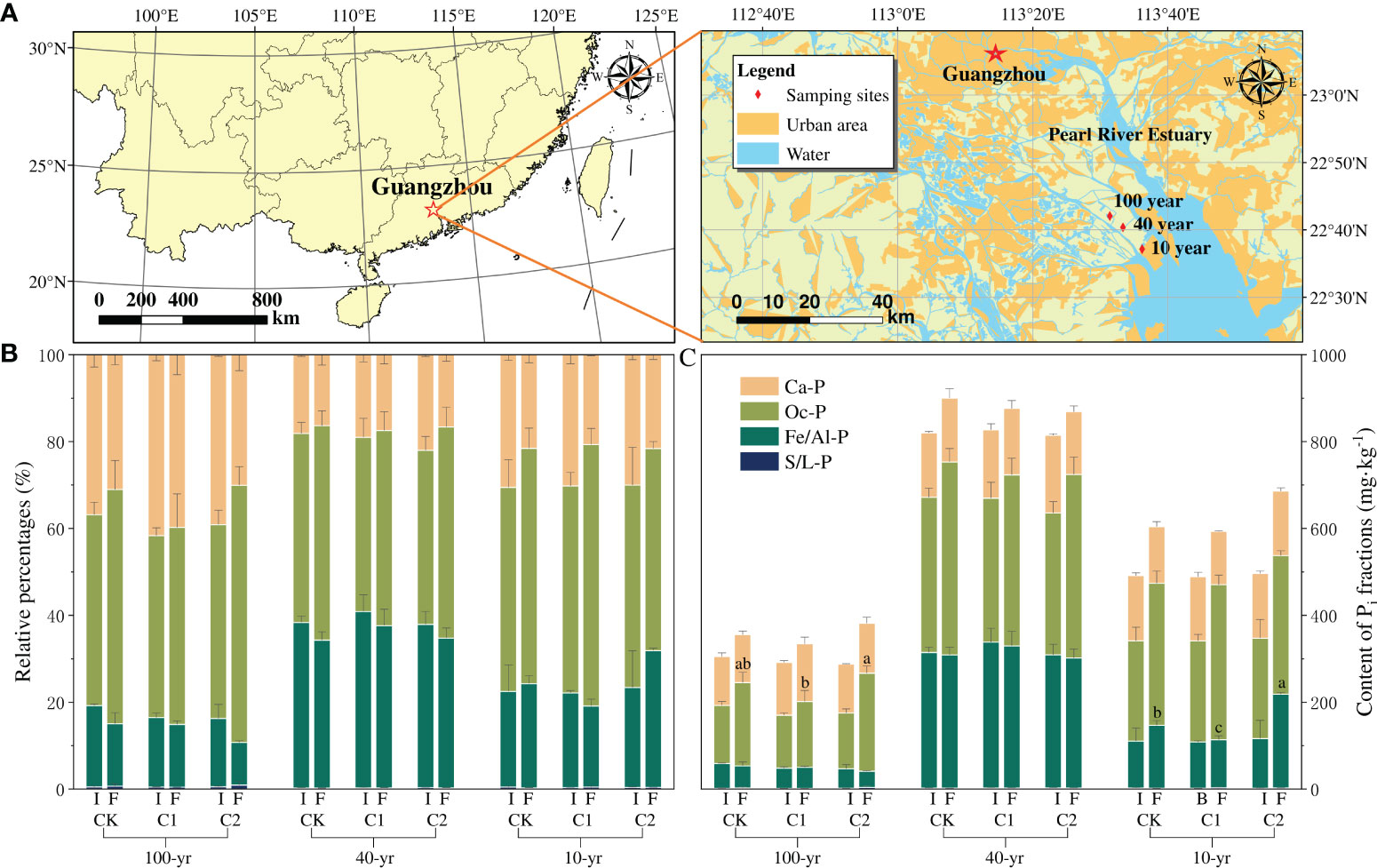
Figure 1 (A) Location map of sampling sites in the Pearl River Estuary. The percentages (B) and contents (C) of Pi fractions in 10-year, 40-year, and 100-year reclaimed soils under different Cd addition treatments. Data represent the mean ± SE (n = 3). ‘I’ and ‘F’ represent initial incubation on day 0 and final incubation on day 40, respectively. Different lowercase letters indicate significant differences among different Cd addition treatments (P < 0.05). Groups that are not marked with a letter mean no significant difference (P > 0.05).
According to the 100-year chronosequence of reclamation and the method of “space-for-time substitutions” (Walker et al., 2010), three study areas with the reclamation ages of 100 years (100-yr), 40 years (40-yr), and 10 years (10-yr) were selected (Figure 1A), with sugarcane, longan, and banana planted, respectively (Bai et al., 2015; Han et al., 2019). At each study area, approximately 20 kg of surface soils (0-10 cm) with three replicates were collected using a plastic shovel after the plant materials and organic residues were removed. Soil samples were brought back to the laboratory for chemical analysis and incubation experiments. The physicochemical properties of soil samples are described in Supplementary Table 1.
Experimental design
Fresh soil samples for incubation were passed through a 5mm sieve to remove coarse plant debris and stones, and then thoroughly mixed to make a homogenous mixture. Microcosm experiments were set up in 500 ml opening jars, with approximately 100 g (fresh weight) of soil sample pressed down into the bottom of each incubation jar. The jars were put in the incubator at 25°C in the dark, pre-incubating for 3 days. We set three treatments for each type of soil (CK: control; C1: low dose of Cd addition, 1.5 mg·kg-1; C2: high dose of Cd addition, 10 mg·kg-1) with three replicates. Exposure concentrations are based on the ISQV-low value and ISQV-high value in the interim sediment quality values (ISQVs) of Hong Kong (Chapman et al., 1999). After the pre-incubation, Cd was added evenly to the microcosms by adding salt solutions (CdCl2), and the CK group added an equal amount of deionized water. The change in soil moisture due to the addition of salt solutions is the same and can be negligible. Jars were put back in the incubator with ventilated cling film covered and incubated for 40 days. The soil samples were collected on days 0, 3, 6, 9, 15, 25, and 40. The destructive sampling strategy was used in our study, which included 189 jars for incubation. Each soil sample was divided into two parts, one part was stored at 4°C to measure the enzyme activity, and the remained was air-dried, ground, and passed through a 100-mesh sieve for measuring P-related chemical indicators. Soil moisture was maintained by weighing the jars continuously and replenishing the lost water.
Sample processing and analyzing
Soil pH was measured by a pH meter (Hach Company, Loveland, CO, USA) in a 1:5 fresh soil to deionized water slurry. The soil moisture content was obtained by drying soils at 105°C for 48 h in an oven. Soil organic matter (SOM) was determined using the dichromate oxidation-colorimetric method (Nelson and Sommers, 1983). Total carbon (TC) contents were analyzed on an elemental analyzer (CHOS, Vario EL, Germany). Total P (Pt) and Cd were measured by acid digestion and the Inductively Coupled Plasma-Atomic Emission Spectrometry (ICP-AES; Zhang et al., 2017). Blanks and certified reference materials (GBW07401) were run periodically to assess the precision and accuracy of this method. The recovery rates of the spiked samples of P and Cd ranged from 97.5%-106.2% and 95%-105%, and the detection limit of the methods is 6.07 mg/kg and 0.02 mg/kg, respectively. Po was determined by the burning method (500°C for 1 h) and extracted with 1.0 mol/L H2SO4 solution (Aspila et al., 1976), while the Pi content of each sample was estimated by the difference between Pt and Po. The Pi fractions were measured by a modified sequential extraction method (Pierzynski, 2000; Bai et al., 2017). Soluble and loosely bound P (S/L-P), Al and Fe-bound P (Fe/Al-P), occluded P (Oc-P), and Ca-bound or apatite P (Ca-P) were extracted by different extractants in sequence. The acid phosphatase and alkaline phosphatase activities were measured through p-nitrophenyl phosphate assays according to the method described by (Dick et al., 2000).
Cumulative net P mineralization and net P mineralization rate
The specific calculation formulas of cumulative net P mineralization (CNPM, mg·kg-1) and net P mineralization rate (RNPM, mg·kg-1·d-1) are given as follows (Achat et al., 2010; Bai et al., 2019):
where i refers to the sampling batch, Pi and Pi-1 refer to the inorganic P content in soil at the ith day and (i-1)th day, respectively; P0 refers to the initial inorganic P content in soil. ti and ti-1 are the incubation of i days and i-1 days, respectively. P mineralization and immobilization is a dynamic process (Arenberg and Arai, 2019; Arenberg and Arai, 2021). If the RNPM is positive and the CNPM increases after a period of incubation, which indicates that the P mineralization is greater than the immobilization, otherwise it’s the opposite.
Statistical analysis
Data processing and statistical analysis were carried out using Excel 2019 and SPSS 20.0 software packages. One-way ANOVA analysis was performed to identify the differences in RNPM, ACP, and ALP activities among different treatments of Cd addition and sampling time. The statistical significance (P < 0.05) of the mean differences among different treatments ;and sampling times were identified by using the Fisher LSD test. Spearman correlation analysis was carried out to reveal the relationship among Cd concentration, reclamation age, phosphatase activity, RNPM, and CNPM. All figures were created using the Origin 2021 and ArcGIS 10.2 software packages.
Results
Initial and final Pi fractions in three reclaimed soils under different Cd addition treatments
At the initial stage of incubation, Oc-P showed the highest percentage, with no significant difference in all treatment groups (P > 0.05), while S/L-P content was the lowest (Figure 1B). The relative percentage of Al/Fe-P in Pi followed the order 40-yr reclaimed soils > 10-yr reclaimed soils > 100-yr reclaimed soils, while Ca-P was the opposite. Moreover, higher percentages of Al/Fe-P were observed in the reclaimed soils with lower pH values, which is opposite to Ca-P (Supplementary Table 1).
At the end of the incubation, the contents of Ca-P and S/L-P had no significant difference in all treatment groups (Figure 1C; P > 0.05). Compared with the initial level, the contents of Al/Fe-P at the end of incubation did not change significantly (P > 0.05) except for the C2 group in 10-yr reclaimed soils (P < 0.05). The contents and relative percentages of Oc-P in all treatment groups were increased, leading to an increase in Pi, but no statistically significant difference was observed in some groups (P > 0.05). And in the CK treatments without Cd addition, Oc-P increased less in reclaimed soils with longer reclamation age. In 100-yr reclaimed soils, Oc-P contents showed a significant increase in high-dose Cd addition treatments compared to low-dose Cd addition (P < 0.05) and took the dominant responsibility for the increase in Pi. Comparatively, in 40-yr reclaimed soils, no significant changes were observed between initial and final levels of P fractions under different treatments. Unlike 100-yr and 40-yr reclaimed soils, Al/Fe-P content in 10-yr reclaimed soils was increased by 46.2% (P < 0.05) under high-dose Cd addition treatments, resulting in an increase in soil Pi.
Changes of phosphatase activity in three reclaimed soils under different Cd addition treatments
The differences in ALP and ACP activities at different incubation times are shown in Figures 2A, B. Over the whole incubation period, ACP and ALP activities in three reclaimed soils had the same trend for all treatment groups, showing a fluctuation from 0 to 15 days and a more stable tendency from 15 to 40 days. ALP activity peaked on day 6 in almost all treatment groups and decreased to a minimum on day 9, except for 10-yr reclaimed soils in the high-dose Cd treatment. While the ACP activity exhibited a relatively smaller fluctuation and peaked on day 9. Notably, ACP and ALP activities in the 10-yr reclaimed soils were significantly higher (P < 0.05) in the C2 treatment group than those in C1 and CK treatments on day 9.
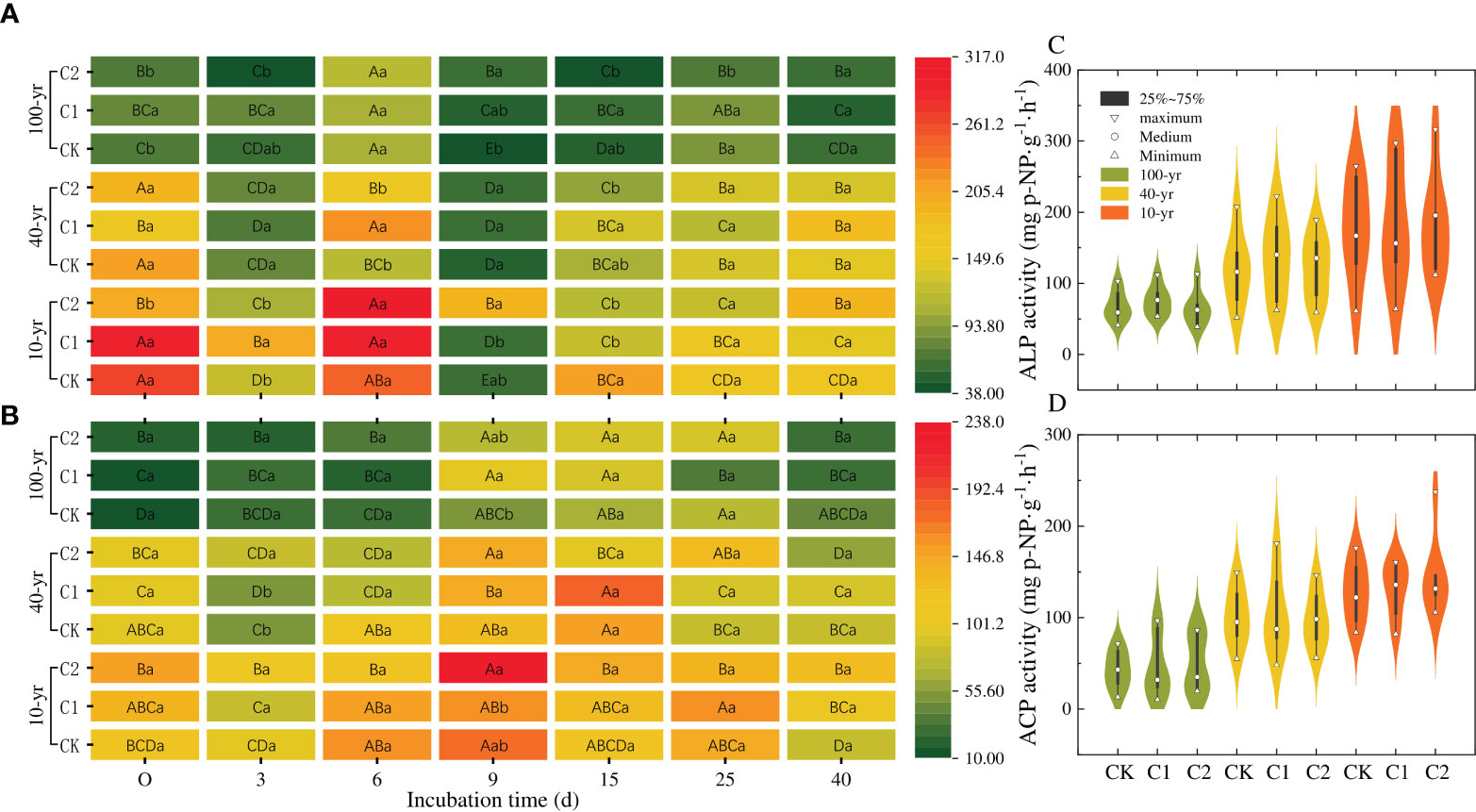
Figure 2 Heatmaps indicate differences in ALP (A) and ACP (B) activities in 10-year, 40-year, and 100-year reclaimed soils under different Cd addition treatments during 40 days of incubation. Violin plots show ALP (C) and ACP (D) activities in three reclaimed soils under different Cd addition treatments. Different capital letters and lowercase letters indicate the significant differences among sampling time and Cd addition treatments, respectively (P < 0.05).
Figures 2C, D showed no significant difference in ACP and ALP activities in three reclaimed soils between CK, low-dose, and high-dose Cd addition treatments (P > 0.05). The 10-yr reclaimed soils exhibited an increase in the average ACP and ALP activities in high-dose Cd addition treatments compared to CK, but there was no statistical significance.
Changes of CNPM and RNPM in three reclaimed soils under different Cd addition treatments
The CNPM values in all reclaimed wetland soils showed similar overall trends under different treatments in a 40-day incubation period (Figure 3A), displaying a fluctuation from 0 to 15 days and a stable increase after 15 days of incubation. The CNPM values in these reclaimed soils rapidly reached a peak after the incubation of 3-9 days of incubation, then dropped sharply to a minimum on day 15, and then gradually increased with the increasing incubation time. The CNPM values in 100-yr reclaimed wetland soils kept a steady change under the Cd addition treatments followed the order C2>C1>CK, while low CNPM values were observed over the incubation period.
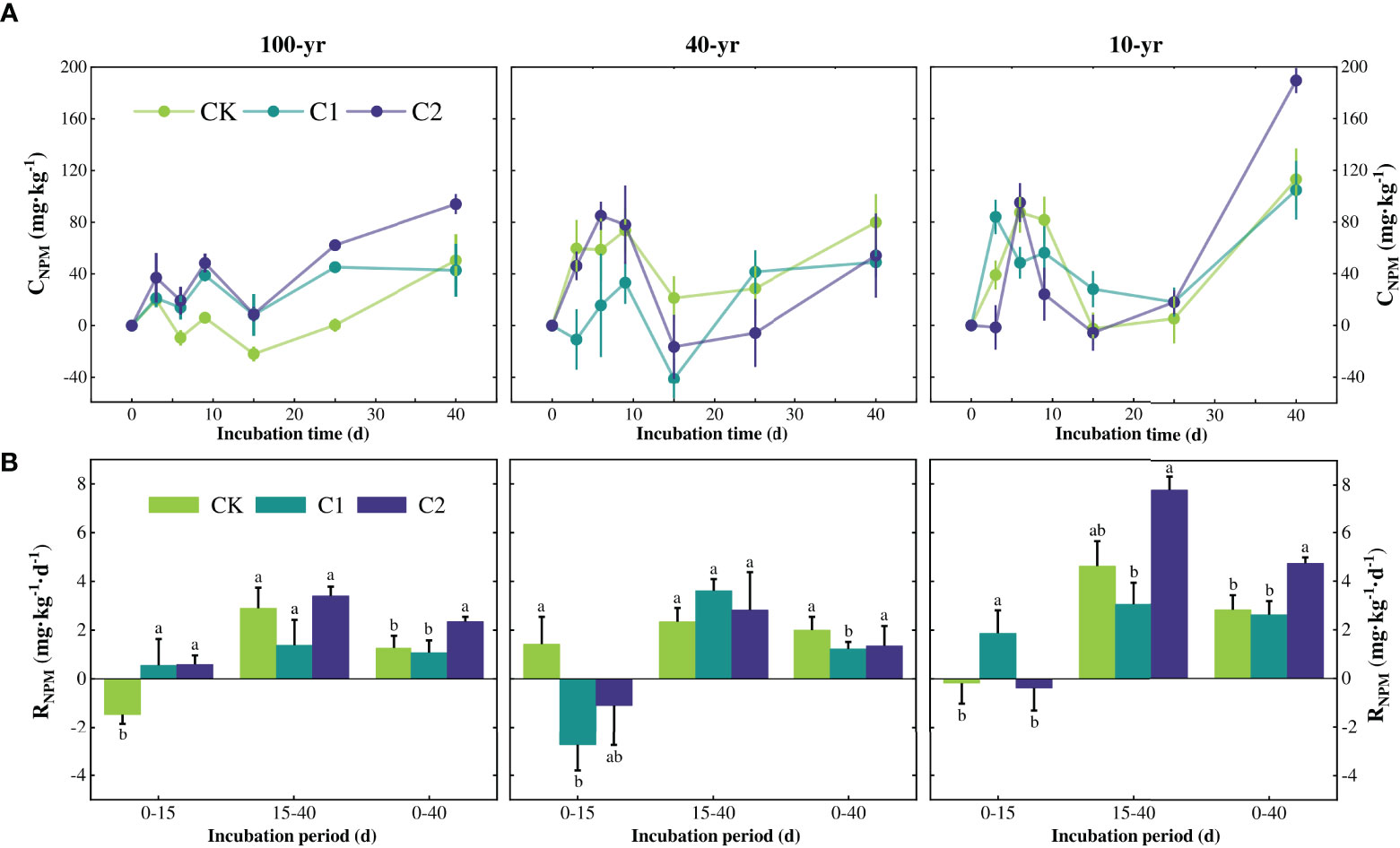
Figure 3 CNPM (A) and RNPM (B) in 100-year, 40-year, and 10-year reclaimed soils under the CK, low-dose Cd, and high-dose Cd treatments during 40 days of incubation. Lowercase letters indicate the significant differences among treatments of different Cd addition (P < 0.05). Data are means ± standard errors (mean ± SE).
RNPM values in three reclaimed soils showed larger variation over the first 15 days of incubation under different Cd addition treatments (P < 0.05), while no significant difference (P > 0.05) was observed during 15-40 days except for 10-yr reclaimed soils (Figure 3B). Comparatively, some negative RNPM values were observed in some reclaimed soils in different Cd addition treatments during the period of 0-15 days, while the RNPM all exhibited positive values in three reclaimed soils during the period of 15-40 and 0-40 days. In the CK treatments without Cd addition, longer reclamation history caused a lower average RNPM value over 40 days of incubation, and the RNPM values were 1.26 ± 0.51 mg·kg-1·d-1 in 100-yr reclaimed soils, 2.00 ± 0.55 mg·kg-1·d-1 in 40-yr reclaimed soils, and 2.83 ± 0.60 mg·kg-1·d-1 in 10-yr reclaimed soils, respectively. The high-dose Cd addition in 100-yr and 10-yr reclaimed soils had significantly higher RNPM values compared with the CK and C1 groups (P < 0.05), whereas no significant effects were observed in the treatment of low-dose Cd addition.
Correlation analysis
Spearman correlation analysis results showed that soil phosphatase activity was tightly associated with the reclamation age (Figure 4). The longer reclamation age improved a significant decrease in ACP activity (Spearman’s rho = -0.73, P < 0.001) and ALP activity (Spearman’s rho = -0.69, P < 0.001). CNPM values also exhibited a poor negative correlation with reclamation ages (Spearman’s rho = -0.23, P > 0.05). As for Cd addition dose, no significant correlation was observed with phosphatase activity, RNPM, and CNPM. ALP and ACP activities had a significant positive correlation (Spearman’s rho = 0.38, P < 0.01), while both did not show significant correlation with RNPM (Spearman’s rho = -0.25, P > 0.05).
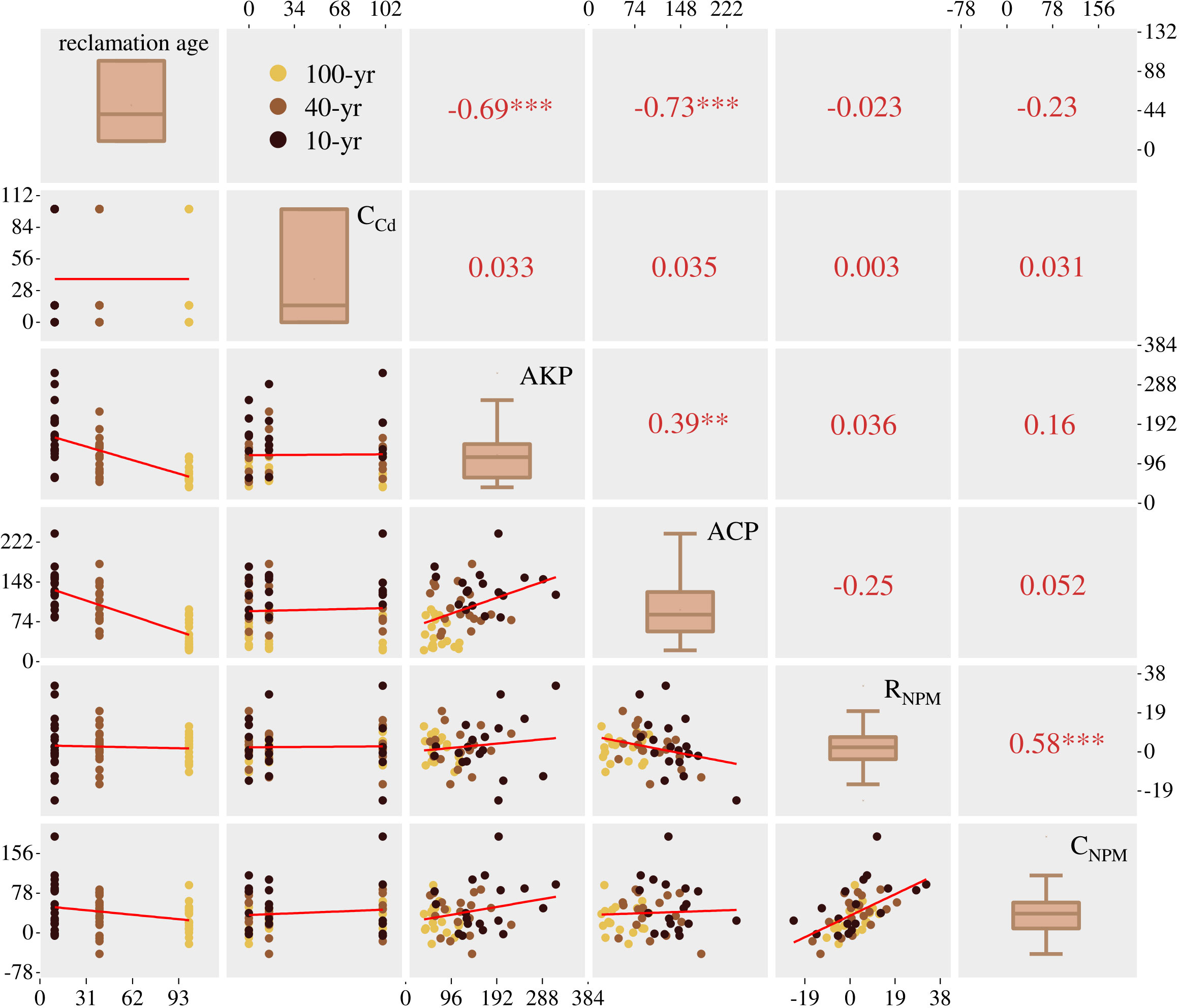
Figure 4 Spearman correlation analysis among Cd concentration, reclamation ages, ACP activity, ALP activity, CNPM and RNPM. Notes: Ccd, Cd addition dose. ***Significant correlation at P < 0.001 level. **Significant correlation at P < 0.01 level.
Discussion
The relative percentages of Pi fractions in 10-year, 40-year, and 100-year reclaimed soils were different. It is widely established that Pi reacts with dissolved metals to form Al/Fe phosphate precipitates in acidic soil conditions and Ca phosphates in alkaline (Arenberg and Arai, 2019), which resulted in changes in the relative proportions of Ca-P and Al/Fe-P in three reclaimed soils. The reason why the relative percentage of Oc-P was the highest is that Oc-P will gradually increase and dominate eventually during the soil development (Yang and Post, 2011). The contribution of S/L-P content to the Pt pool is typically low (≈ 0.1%; Jones and Oburger, 2011), but it is vital as the pool for the P uptake by plants and microorganisms (Kruse et al., 2015). Po is mineralized to form soluble P, then soluble P is strongly absorbed onto Al/Fe (hydr)oxides or forms Al/Fe phosphate precipitates by reacting with dissolved metals, i.e. transformed into loosely bound P and Al and Fe-bound P. Over time precipitated P can become occluded progressively to resistant to further transformation through soil aggregation (Yang and Post, 2011). It is suspected that the increase in Al/Fe-P caused by the addition of high-dose of Cd in the 10-year reclaimed soils (Figure 1B) was due to the mineralization occurring after 25 days of incubation (Figure 3A), and Al/Fe-P does not have enough time to be fully converted to Oc-P.
This study mainly focused on the short-term P mineralization process. It is speculated that if the incubation is continued after 40 days of incubation, RNPM and phosphatase activity will decrease owing to the gradual decrease in carbon, which is similar to those of the results (Achat et al., 2010). Generally, three reclaimed soils had more P mineralized than P immobilized under all treatment groups and exhibited an overall increase in CNPM and Oc-P (Figure 3A and Figure 1C). Generally, the reclaimed soils with a longer reclamation history had lower soil phosphatase activity, which led to the lower rise in CNPM and Oc-P to some extent. This was not consistent with the prevailing view that phosphatase activity raised with the increasing reclamation age (Li et al., 2014), which might be associated with the different soil moisture contents in three reclaimed soils (Table S1). This may be due to the soil moisture is considered to be the dominant factor leading to the different phosphatase activity levels and is positively correlated with phosphatase activity (Arenberg and Arai, 2019).
Over the incubation, RNPM, CNPM, and phosphatase activity in three reclaimed soils exhibited a similar trend in all treatment groups. They showed fluctuations in the early stage of incubation (0-15 days) and a stable and increasing tendency in the later stage of incubation (15-40 days), which is similar to the results in floodplain soils by Arenberg and Arai (2021). However, there was no significant correlation between soil RNPM and ACP or ALP activities (Figure 4). The possible explanation was that the measured phosphatase activity represents potential activity rather than true activity, not directly equivalent to RNPM (Bünemann, 2015), and RNPM represents the average net mineralization rate over the period prior to sampling. The main P-related processes in the microcosms include microbially-mediated enzymatic (biochemical) P mineralization, the weaker microbial (biological) P remineralization, and microbial P immobilization (Achat et al., 2010; Jones and Oburger, 2011). Phosphatases as the agents responsible for P mineralization in the microcosms are mainly secreted by microorganisms, and ALP is mainly excreted from bacteria while ACP is released from fungi (Nannipieri et al., 2011; Arenberg and Arai, 2021). In the early stage of incubation, microorganisms might be disturbed, and rapidly multiply accompanied with fast P mineralization and immobilization under the conditions of sufficient substrates, causing fluctuations in phosphatase activity and RNPM. After the microorganisms gradually adapted to the environment in the later stage of incubation, the phosphatase activity tended to stabilize. However, microbial P immobilization and remineralization gradually weakened due to reduced substrate levels (Bünemann et al., 2004). Therefore, a slightly increasing trend of P mineralization can be observed in the later stage of incubation.
High Cd exposure facilitated the P mineralization process through improving Oc-P and Al/Fe-P contents in 100-year and 10-year reclaimed soils, respectively (Figure 3A). High-dose Cd exposure stimulated ALP and ACP activities in the 10-year reclaimed soils, which supports the result by Yang et al. (2016) who reported that phosphatase is activated by Cd. This is because divalent cations (e.g. Ca, Mg, Zn, and Cd) have been found to act as phosphatase activators (Quiquampoix and Mousain, 2005). In the later period of incubation under the high-dose Cd addition treatments, a significant increase in RNPM owing to the increase in ACP and ALP activities on day 9 with a short-term time lag in 10-year reclaimed soils. And P immobilization might be significantly weakened with the emergence of microbial toxicity after day 15 (Aponte et al., 2020). A previous study has reported that extracellular stabilized enzyme activity was not affected by the composition, abundance, and activity of the soil microbiota and was more stable (Nannipieri et al., 2003). So, Cd might indirectly contribute to a significant increase in RNPM in the late stages of incubation of 10-year reclaimed soils (Figure 3B). In 100-year reclaimed soils, the increase in CNPM promoted by Cd addition was in the early stage of incubation, which is mainly associated with the increase in phosphatase activity and inhibition of microbial colonization and P immobilization. The advance in time compared to 10-year reclaimed soils may be due to the lower phosphatase activity and weaker microbial resilience in response to stresses in 100-year reclaimed soils (Huang et al., 2020). In addition, it is notable that the inhibition of the low dose of Cd on P mineralization was found in 40-year reclaimed soils, which may be related to the higher Pi contents and SOM, and lower soil pH values promoting microbial P immobilization (Seshadri et al., 2016; Wan et al., 2020). Nannipieri et al. (2011) mentioned that short-term laboratory incubations could not reflect the toxic effects in long-term heavy metal polluted soils. It is hypothesized that if the incubation time is prolonged, the toxicity of Cd will become apparent and RNPM and phosphatase activity will decrease in the presence of Cd addition.
Conclusion
This study highlights the effect of Cd pollution on the soil P mineralization process in coastal reclaimed wetlands along a 100-year reclamation chronosequence in the PRE. The reclamation age affected soil P fractions and phosphatase activities, which in turn led to different P mineralization dynamics. High Cd exposure promoted phosphatase activity and P mineralization at the end of the incubation in the 100-year and 10-year coastal reclaimed wetland soils, whereas low Cd addition had no significant effect. The older reclaimed coastal wetlands might have lower phosphorus mineralization potentials and higher Cd pollution might lead to P loss by contributing to soil P mineralization. These effects may be caused by the Cd stress on soil microorganisms, and thus the in-depth studies on microbial community structure and function, and functional genes related to P mineralization are needed to reveal the mechanisms of Cd affecting P mineralization. Though the findings of this study were related to the soil samples in the PRE, they can help to better understand the P transformation in reclaimed coastal wetland soils under Cd pollution stress and provide a scientific basis for soil P dynamics prediction and soil fertility management.
Data availability statement
The raw data supporting the conclusions of this article will be made available by the authors, without undue reservation.
Author contributions
JB and ZG designed the research. ZL analyzed the data, and wrote the manuscript with contributions from JB, GC, and RT. ZG and GZ performed all field work and all biochemical analyses. All authors contributed to the article and approved the submitted version.
Conflict of interest
The authors declare that the research was conducted in the absence of any commercial or financial relationships that could be construed as a potential conflict of interest.
Publisher’s note
All claims expressed in this article are solely those of the authors and do not necessarily represent those of their affiliated organizations, or those of the publisher, the editors and the reviewers. Any product that may be evaluated in this article, or claim that may be made by its manufacturer, is not guaranteed or endorsed by the publisher.
Acknowledgments
The authors express their appreciation to the Key Project of National Natural Science Foundation of China (U1901212) for financial support of this study.
Supplementary material
The Supplementary Material for this article can be found online at: https://www.frontiersin.org/articles/10.3389/fmars.2022.965197/full#supplementary-material
References
Achat D. L., Bakker M. R., Zeller B., Pellerin S., Bienaime S., Morel C. (2010). Long-term organic phosphorus mineralization in spodosols under forests and its relation to carbon and nitrogen mineralization. Soil Biol. Biochem. 42 (9), 1479–1490. doi: 10.1016/j.soilbio.2010.05.020
Aponte H., Medina J., Butler B., Meier S., Cornejo P., Kuzyakov Y. (2020). Soil quality indices for metal(loid) contamination: An enzymatic perspective. Land. Degrad. Dev. 31 (17), 2700–2719. doi: 10.1002/ldr.3630
Arenberg M. R., Arai Y. (2019). Uncertainties in soil physicochemical factors controlling phosphorus mineralization and immobilization processes. Adv. Agronom. 154, 153–200. doi: 10.1016/bs.agron.2018.11.005
Arenberg M. R., Arai Y. (2021). Effects of native leaf litter amendments on phosphorus mineralization in temperate floodplain soils. Chemosphere 266, 129210. doi: 10.1016/j.chemosphere.2020.129210
Aspila K. I., Agemian H., Chau A. S. (1976). A semi-automated method for the determination of inorganic, organic and total phosphate in sediments. Analyst 101 (1200), 187–197. doi: 10.1039/an9760100187
Bai J., Lu Q., Zhao Q., Wang J., Gao Z., Zhang G. (2015). Organochlorine pesticides (OCPs) in wetland soils under different land uses along a 100-year chronosequence of reclamation in a Chinese estuary. Sci. Rep. 5, 17624. doi: 10.1038/srep17624
Bai J., Ye X., Jia J., Zhang G., Zhao Q., Cui B., et al. (2017). Phosphorus sorption-desorption and effects of temperature, pH and salinity on phosphorus sorption in marsh soils from coastal wetlands with different flooding conditions. Chemosphere 188, 677–688. doi: 10.1016/j.chemosphere.2017.08.117
Bai J., Yu Z., Yu L., Wang D., Guan Y., Liu X., et al. (2019). In-situ organic phosphorus mineralization in sediments in coastal wetlands with different flooding periods in the yellow river delta, China. Sci. Total. Environ. 682, 417–425. doi: 10.1016/j.scitotenv.2019.05.176
Bünemann E. K. (2015). Assessment of gross and net mineralization rates of soil organic phosphorus - A review. Soil Biol. Biochem. 89, 82–98. doi: 10.1016/j.soilbio.2015.06.026
Bünemann E. K., Bossio D. A., Smithson P., Frossard E., Oberson A. (2004). Microbial community composition and substrate use in a highly weathered soil as affected by crop rotation and p fertilization. Soil Biol. Biochem. 36 (6), 889–901. doi: 10.1016/j.soilbio.2004.02.002
Cao Z., Wang L., Yang L., Yu J., Lv J., Meng M., et al. (2020). Heavy metal pollution and the risk from tidal flat reclamation in coastal areas of jiangsu, China. Mar. Pollut. Bull. 158, 111427. doi: 10.1016/j.marpolbul.2020.111427
Chapman P. M., Allard P. J., Vigers G. A. (1999). Development of sediment quality values for Hong Kong special administrative region: a possible model for other jurisdictions. Mar. Pollut. Bull. 38 (3), 161–169. doi: 10.1016/S0025-326X(98)00162-3
Chen J., Liu J., Hong H., Liang S., Zhao W., Jia H., et al. (2022). Coastal reclamation mediates heavy metal fractions and ecological risk in saltmarsh sediments of northern jiangsu province, China. Sci. Total. Environ. 825, 154028. doi: 10.1016/j.scitotenv.2022.154028
Cui Y., Wang X., Wang X., Zhang X., Fang L. (2021). Evaluation methods of heavy metal pollution in soils based on enzyme activities: A review. Soil Ecol. Lett. 3 (3), 169–177. doi: 10.1007/s42832-021-0096-0
Dick W. A., Cheng L., Wang P. (2000). Soil acid and alkaline phosphatase activity as pH adjustment indicators. Soil Biol. Biochem. 32 (13), 1915–1919. doi: 10.1016/S0038-0717(00)00166-8
Fellman J. B., D'Amore D. V. (2007). Nitrogen and phosphorus mineralization in three wetland types in southeast Alaska, USA. Wetlands 27 (1), 44–53. doi: 10.1672/0277-5212(2007)27[44:Napmit]2.0.Co;2
Gu Y., Li Q., Fang J., He B., Fu H., Tong Z. (2014). Identification of heavy metal sources in the reclaimed farmland soils of the pearl river estuary in China using a multivariate geostatistical approach. Ecotoxicol. Environ. Saf. 105, 7–12. doi: 10.1016/j.ecoenv.2014.04.003
Han L., Bai J., Gao Z., Wang W., Wang D., Cui B., et al. (2019). Polycyclic aromatic hydrocarbons (PAHs) in surface soils from reclaimed and ditch wetlands along a 100-year chronosequence of reclamation in a Chinese estuary: Occurrence, sources, and risk assessment. Agric. Ecosyst. Environ. 286. doi: 10.1016/j.agee.2019.106648
Huang L., Bai J., Wen X., Zhang G., Zhang C., Cui B., et al. (2020). Microbial resistance and resilience in response to environmental changes under the higher intensity of human activities than global average level. Global Change Biol. (26), 2377–2389. doi: 10.1111/gcb.14995
Iuss Working Group W. R. B. (2006). World reference base for soil resources 2006 : A framework for international classification, correlation and communication (Rome: FAO).
Jones D. L., Oburger E. (2011). “Solubilization of phosphorus by soil microorganisms,” in Phosphorus in action (Berlin, German:Springer), 169–198.
Kruse J., Abraham M., Amelung W., Baum C., Bol R., Kuhn O., et al. (2015). Innovative methods in soil phosphorus research: A review. J. Plant Nutr. Soil Sci. 178 (1), 43–88. doi: 10.1002/jpln.201400327
Li Q., Chen Y., Fu H., Cui Z., Shi L., Wang L., et al. (2012). Health risk of heavy metals in food crops grown on reclaimed tidal flat soil in the pearl river estuary, China. J. Hazard. Mater. 227-228, 148–154. doi: 10.1016/j.jhazmat.2012.05.023
Li J., Pu L., Zhu M., Zhang J., Li P., Dai X., et al. (2014). Evolution of soil properties following reclamation in coastal areas: A review. Geoderma 226, 130–139. doi: 10.1016/j.geoderma.2014.02.003
Liu F., Niu L., Chen H., Li P., Tian F., Yang Q. (2017). Seasonal changes of polycyclic aromatic hydrocarbons in response to hydrology and anthropogenic activities in the pearl river estuary, China. Mar. Pollut. Bull. 117 (1-2), 255–263. doi: 10.1016/j.marpolbul.2017.01.061
Li C., Wang H., Liao X., Xiao R., Liu K., Bai J., et al. (2022). Heavy metal pollution in coastal wetlands: A systematic review of studies globally over the past three decades. J. Hazard. Mater. 424, 127312. doi: 10.1016/j.jhazmat.2021.127312
Lu Q., Bai J., Zhang G., Wu J. (2020). Effects of coastal reclamation history on heavy metals in different types of wetland soils in the pearl river delta: Levels, sources and ecological risks. J. Clean. Prod. 272:122668. doi: 10.1016/j.jclepro.2020.122668
Ma Z., Melville D. S., Liu J., Chen Y., Yang H., Ren W., et al. (2014). Rethinking china's new great wall. Science 346 (6212), 912–914. doi: 10.1126/science.1257258
Margalef O., Sardans J., Maspons J., Molowny-Horas R., Fernandez-Martinez M., Janssens I. A., et al. (2021). The effect of global change on soil phosphatase activity. Glob. Chang. Biol. 27 (22), 5989–6003. doi: 10.1111/gcb.15832
Nannipieri P., Ascher J., Ceccherini M. T., Landi L., Pietramellara G., Renella G. (2003). Microbial diversity and soil functions. Eur. J. Soil Sci. 54 (4), 655–670. doi: 10.1046/j.1351-0754.2003.0556.x Berlin, German: Springer.
Nannipieri P., Giagnoni L., Landi L., Renella G. (2011). “Role of phosphatase enzymes in soil,” in Phosphorus in action (Berlin, German:Springer), 215–243.
Nelson D. A., Sommers L. E. (1983). “Total carbon, organic carbon, and organic matter,” in Methods of soil analysis, (SSSA Book Series), New Jersey, United States: Wiley. 9, 539–579.
Oehl F., Oberson A., Sinaj S., Frossard E. (2001). Organic phosphorus mineralization studies using isotopic dilution techniques. Soil Sci. Soc Am. J. 65 (3), 780–787. doi: 10.2136/sssaj2001.653780x
Pan J., Yu L. (2011). Effects of cd or/and Pb on soil enzyme activities and microbial community structure. Ecol. Eng. 37 (11), 1889–1894. doi: 10.1016/j.ecoleng.2011.07.002
Pierzynski G. M. (2000). Methods of phosphorus analysis for soils, sediments, residuals, and waters (North Carolina, United States:North Carolina State University Raleigh).
Quiquampoix H., Mousain D. (2005). “Enzymatic hydrolysis of organic phosphorus,” in Organic phosphorus in the environment (Bonn, Germany:CAB eBooks), 89–112.
Reddy K. R., DeLaune R. D. (2008). Biogeochemistry of wetlands: Science and applications (Florida, United States: CRC press).
Seshadri B., Bolan N. S., Wijesekara H., Kunhikrishnan A., Thangarajan R., Qi F., et al. (2016). Phosphorus-cadmium interactions in paddy soils. Geoderma 270, 43–59. doi: 10.1016/j.geoderma.2015.11.029
Spohn M., Kuzyakov Y. (2013). Phosphorus mineralization can be driven by microbial need for carbon. Soil Biol. Biochem. 61, 69–75. doi: 10.1016/j.soilbio.2013.02.013
Sun Z., Sun W., Tong C., Zeng C., Yu X., Mou X. (2015). China's coastal wetlands: conservation history, implementation efforts, existing issues and strategies for future improvement. Environ. Int. 79, 25–41. doi: 10.1016/j.envint.2015.02.017
Walker L. R., Wardle D. A., Bardgett R. D., Clarkson B. D. (2010). The use of chronosequences in studies of ecological succession and soil development. J. Ecol. 98 (4), 725–736. doi: 10.1111/j.1365-2745.2010.01664.x
Wan W., Hao X., Xing Y., Liu S., Zhang X., Li X., et al. (2020). Spatial differences in soil microbial diversity caused by pH-driven organic phosphorus mineralization. Land. Degrad. Dev. 32 (2), 766–776. doi: 10.1002/ldr.3734
Xian Y., Wang M., Chen W. (2015). Quantitative assessment on soil enzyme activities of heavy metal contaminated soils with various soil properties. Chemosphere 139, 604–608. doi: 10.1016/j.chemosphere.2014.12.060
Xiao R., Bai J., Lu Q., Zhao Q., Gao Z., Wen X., et al. (2015). Fractionation, transfer, and ecological risks of heavy metals in riparian and ditch wetlands across a 100-year chronosequence of reclamation in an estuary of China. Sci. Total. Environ. 517, 66–75. doi: 10.1016/j.scitotenv.2015.02.052
Yang X., Post W. M. (2011). Phosphorus transformations as a function of pedogenesis: A synthesis of soil phosphorus data using hedley fractionation method. Biogeosciences 8 (10), 2907–2916. doi: 10.5194/bg-8-2907-2011
Yang J., Yang F., Yang Y., Xing G., Deng C., Shen Y., et al. (2016). A proposal of "core enzyme" bioindicator in long-term Pb-zn ore pollution areas based on topsoil property analysis. Environ. pollut. 213, 760–769. doi: 10.1016/j.envpol.2016.03.030
Yin A., Gao C., Zhang M., Wu P., Yang X. (2017). Rapid changes in phosphorus species in soils developed on reclaimed tidal flat sediments. Geoderma 307, 46–53. doi: 10.1016/j.geoderma.2017.07.034
Yuan X., Xue N., Han Z. (2021). A meta-analysis of heavy metals pollution in farmland and urban soils in China over the past 20 years. J. Environ. Sci. (China). 101, 217–226. doi: 10.1016/j.jes.2020.08.013
Zhang G., Bai J., Xiao R., Zhao Q., Jia J., Cui B., et al. (2017). Heavy metal fractions and ecological risk assessment in sediments from urban, rural and reclamation-affected rivers of the pearl river estuary, China. Chemosphere 184, 278–288. doi: 10.1016/j.chemosphere.2017.05.155
Keywords: net phosphorus mineralization rate, Cd pollution, phosphatase activity, reclamation history, wetland soils
Citation: Liu Z, Gao Z, Bai J, Zhang G, Tang R and Chen G (2022) Effects of Cd addition on soil phosphorus mineralization in reclaimed coastal wetlands along a 100-year reclamation chronosequence in the Pearl River Estuary (China). Front. Mar. Sci. 9:965197. doi: 10.3389/fmars.2022.965197
Received: 09 June 2022; Accepted: 04 July 2022;
Published: 22 July 2022.
Edited by:
Zeng Zhou, Hohai University, ChinaCopyright © 2022 Liu, Gao, Bai, Zhang, Tang and Chen. This is an open-access article distributed under the terms of the Creative Commons Attribution License (CC BY). The use, distribution or reproduction in other forums is permitted, provided the original author(s) and the copyright owner(s) are credited and that the original publication in this journal is cited, in accordance with accepted academic practice. No use, distribution or reproduction is permitted which does not comply with these terms.
*Correspondence: Junhong Bai, anVuaG9uZ2JhaUAxNjMuY29t