- 1Zhejiang Marine Fisheries Research Institute, Zhoushan, China
- 2Key Laboratory of Sustainable Utilization of Technology Research for Fishery Resource of Zhejiang Province, Zhoushan, China
- 3Zhoushan Natural Resource Surveying and Mapping Design Center, Zhoushan, China
- 4Zhoushan Fishery Environments and Aquatic Products Quality Monitoring Center of Ministry of Agriculture China, Zhoushan, China
- 5School of Life Science, Jiangsu Normal University, Xuzhou, China
Bacterial community plays a vital role in the open-oceanic aquaculture ecosystem, and its stability is crucial for maintaining the health of mariculture fish. However, there are no reported studies on microbial communities in the culture environment of marine fish cages. In this study, bacterial community composition and diversity of seawater and sediment habitats from 5 large yellow croaker cage farms were first investigated by 16S rRNA-based high-throughput sequencing. The composition of bacterial communities was visualized at the phylum and genus levels. Meanwhile, biological and ecological functions were also predicted. The bacterial diversity observed at 3 inshore sampling sites was higher in seawater groups than that in sediment groups. The alpha diversity indices were influenced by the geographic location, especially the inshore site Yueqing (YQ), showing higher values than other sampling sites. The pathogenic bacteria were prevalent in the aquaculture environments, and probiotics showed a relatively low proportion by comparing the potential pathogens. Our study provides baseline data on bacterial communities and diversity in the open-oceanic environments of cage-culture large yellow croaker.
Introduction
Large yellow croaker (Pseudosciaena crocea) is an important mariculture fish in China. During the past few decades, its cage culture has rapidly been developing in the southeast coastal areas (Chen et al., 2018a; Wang P. et al., 2019; Chen et al., 2020). Based on the China Fisheries Yearbook in 2020, the gross yield of marine cage-culture large yellow croaker is more than 225,000 tons, which generates enormous commercial value (Li C. H. et al., 2020; Wang et al., 2020). However, various bacterial disease outbreaks of cage-culture large yellow croaker, such as visceral white nodules disease (VWND) (Li C. et al., 2020), nocardiosis (Wang G. L. et al., 2005), and vibriosis (Liu et al., 2016), cause severe economic losses annually (Zhang et al., 2014). Nearshore environmental pollution and the intensive aquaculture of high-density feed have become the inducement of epidemic diseases (Liu and De Mitcheson, 2008). Developing the offshore cage culture is a better way to increase fish production.
Environmental microorganisms, the important colonizers of the aquatic ecosystem, play crucial roles in nutrient cycling, foreign compounds metabolizing, water quality and the health of cultured fish (Blancheton et al., 2013; Li T. et al., 2017). Sediment accumulating massive organic and inorganic matters is a robust habitat for microorganisms and is the most biodiverse ecological environment in the ocean (Jorgensen et al., 2012; Zhang J. et al., 2019). The microbial community in sediment is closely related to the substantial shifts in the basic abiotic and biological qualities of the surrounding ecosystem. The changes probably result in disease outbreaks in aquaculture animals (Fan et al., 2019; Zeng et al., 2021). Sediment microbial communities are thereby considered as potential indicators for the environmental quality of aquaculture ecosystems (Cornall et al., 2016).
The water microbiota is important for the stability of the aquatic ecosystem and the healthy aquaculture since reared animals are directly exposed to water (Chen et al., 2019; Fan et al., 2019). In recent years, studies have focused on the bacterial community compositions of farmed seawater because of the occurrence of many potential pathogens in cultural seawater. For example, Vibrio harveyi, Vibrio alginolyticus, Vibrio parahaemolyticus and Pseudomonas plecoglossicida have been frequently linked to disease occurrence in large yellow croaker (Shan et al., 2005; Liu et al., 2016; Li C. H. et al., 2020). Conversely, probiotics in cultural water have a positive influence on controlling pathogenic bacteria and improving water quality, thereby maintaining the aquaculture ecosystem stability (Olmos et al., 2011; Giri et al., 2013; Del'Duca et al., 2013).
Although microbial communities in the marine sediment and seawater are closely related to the health of aquaculture animals, community structure, abundance, and function are significantly different between sediment and seawater (Ul-Hasan et al., 2019). Exploring the composition, diversity, and relationship of bacterial communities in the mariculture environments (sediment and seawater) contributes to the control of fish disease in aquaculture systems. For the aquaculture ecosystem of cage-culture large yellow croaker, systematic research comparing the bacterial community structure and diversity in seawater and sediment is lacking, limiting the disease management for healthy and sustainable aquaculture of large yellow croaker. In this study, high-throughput sequencing was used to investigate the sediment and seawater microbial communities and their relationships in the offshore and inshore cages of large yellow croaker from 5 farms located in Zhejiang Province, China. Our study provided valuable information for understanding the potential functions of the microbial communities in the marine cage farm environment and also highlighted the main pathogenic bacteria causing potential threats to the large yellow croaker industry in the aquaculture environments of Zhejiang coast, China.
Materials and methods
Sample collection and preparation
A total of 27 samples, including 15 seawater and 12 sediment samples, were collected in July and August 2021 from 5 commercial large yellow croaker farms located in Zhejiang Province, China (Figure 1). Of which, two stations, i.e., Zhoushan (ZS) and Taizhou (TZ), are offshore sampling sites (far from the inland living area), and other three stations, i.e., Yueqing (YQ), Zhujiajian (ZJJ) and Xiangshan (XS), are inshore sampling site (close to the inland living area). Samples were named using the convention “sample station + W(S)”, and the last number W(S) represents the seawater (sediment) sample. For each farm, 3 parallel seawater samples or sediment samples from three different aquaculture net cages were divided into a group. Sediment samples were collected at depths from the surface down to 20 cm using a stainless-steel core sampler and then placed into individual plastic sterile bags (Su et al., 2018). However, the sediment sample could not be collected at the sampling site ZS due to its sandy property. Each seawater sample was collected from 1 m above the sediment surface (Yu et al., 2018). Temperature, pH, salinity, and dissolved oxygen (DO) of seawater samples were measured using a portable water quality analyzer (YSI ProPlus, USA) (Table S1). All collected samples were transported to the laboratory in a portable ice box immediately within 12 h.
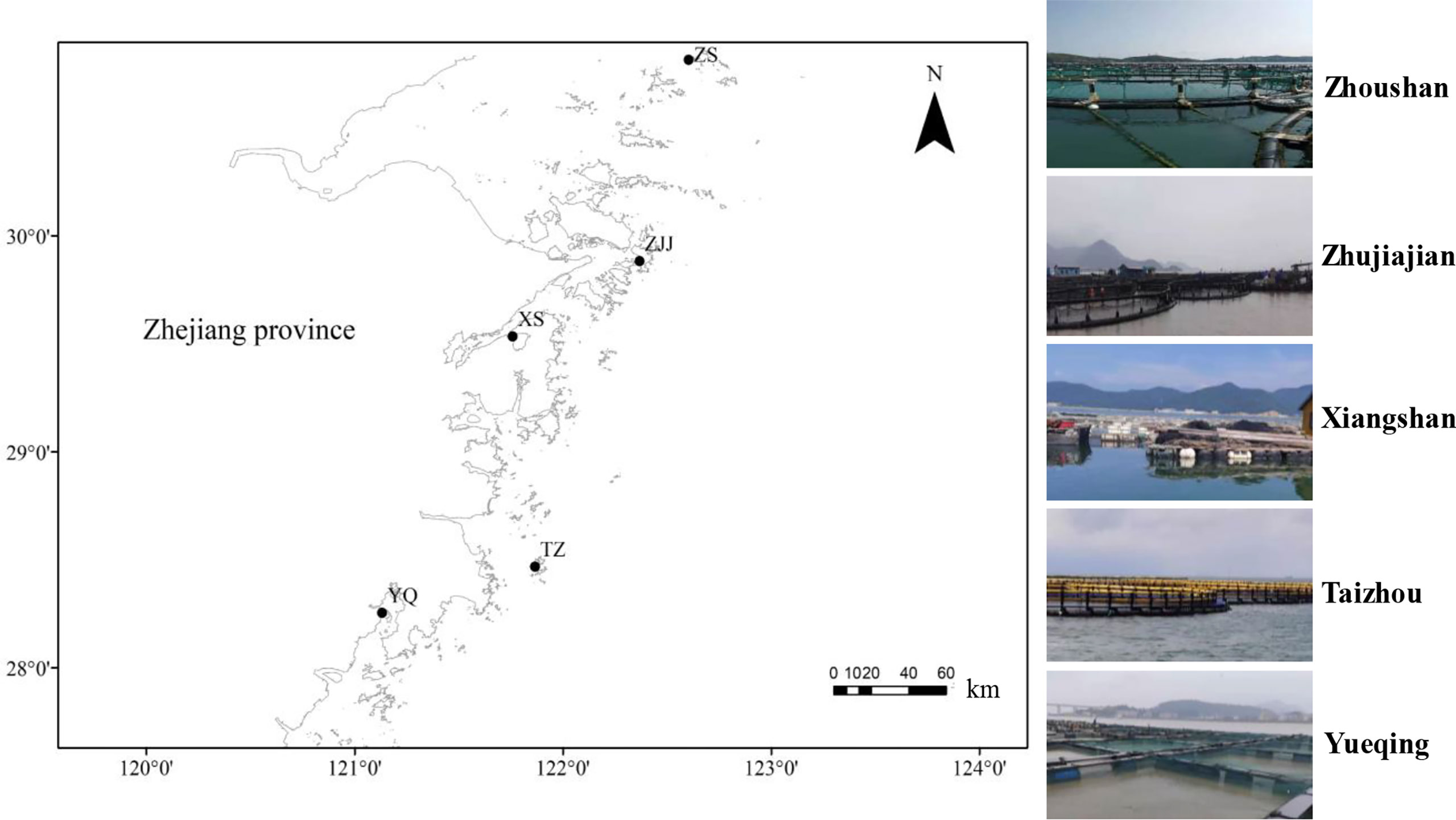
Figure 1 Geographic location of sampling sites from five large yellow croaker farms in Zhejiang province, China. Three parallel samples for seawater and sediment (excluding the sampling site ZS) were collected from three different cages in each surveyed site.
One L of each seawater sample was filtered with 0.22 μm Durapore® membrane filters (Millipore, MA, USA), which were collected into a 50 mL sterile centrifuge tube. The 20.0 g of each sediment sample was put into a 50 mL sterile centrifugal tube. Finally, all samples were frozen in liquid nitrogen and then stored at -80°C for further DNA extraction.
DNA extraction, PCR amplification and sequencing
The total genomic DNA (gDNA) of the filtered membranes and sediment samples were extracted by the HiPure Soil DNA Kits (Magen, Guangzhou, China) according to the manufacturer’s instructions. The integrality of DNA was assessed via 1% agarose gel electrophoresis, and the concentration and purity were determined by Nanodrop 2000 micro-spectrophotometer (Thermo Fisher Scientific, Waltham, MA, USA). The V3-V4 hypervariable regions of bacterial 16S rRNA genes were amplified using the following primers pair: 341F (5′-CCTACGGGNGGCWGCAG-3′) and 806R (5′-GGACTACHVGGGTATCTAAT-3′) with barcodes (Zhao et al., 2020). The polymerase chain reaction (PCR) assay was conducted using a 50 μL reaction mixture containing 10 μL of 5× reaction buffer, 10 μL of 5× high GC enhancer, 1.5 μL of 2.5 mM dNTPs, 1.5 μL of 10 μM each primer, 0.2 μL of DNA polymerase, and 50 ng of template DNA. The PCR program was set as follows: initial denaturation at 95°C for 5 min, followed by 30 cycles at denaturation 95°C for 1 min, annealing at 60°C for 1 min, and elongation 72°C for 1 min, with a final extension at 72°C for 7 min.
After electrophoresis on a 2% agarose gel, PCR products were purified using the AxyPrep DNA Gel Extraction Kit (Axygen Biosciences, Union City, CA, USA), quantified with ABI StepOnePlus Real-Time PCR System (Life Technologies, Carlsbad, CA, USA), and pooled in equimolar concentrations. Purified amplicons were paired-end sequenced (PE250) on an Illumina Novaseq 6000 platform at Genedenovo Biotechnology Co. Ltd., Guangzhou, China. Raw reads were deposited into the National Center for Biotechnology Information (NCBI) Sequence Read Archive (SRA) database (Accession Number: PRJNA839924).
Bioinformatics and statistical analysis
Raw reads of high-throughput sequencing were initially filtered by FASTP (version 0.18.0) (Chen et al., 2018b). FLASH software (version 1.2.11) (Magoč and Salzberg, 2011) was used to merge paired-end clean reads by following the criteria of an overlap > 10 bp and mismatch ratio > 2%. Spliced sequences of raw tags were quality-filtered via QIIME software (version 1.7.0) (Bokulich et al., 2013). Clean tags with ≥97% similarity were classified into one operational taxonomic unit (OTU) using UPARSE (version 9.2.64) (Edgar, 2013). Chimeric tags were checked and removed using the UCHIME algorithm to obtain the effective tags (Edgar et al., 2011). Each representative OTU sequence with the highest abundance was screened for further taxonomic analysis. Species annotation at each taxonomic level was performed using the RDP classifier (version 2.2) (Wang et al., 2007) via the SILVA database at an 80% confidence threshold (Pruesse et al., 2007). The stacked bar plot of community composition was conducted by using the “ggplot2” package in the R platform (version 2.2.1) (Wickham, 2011). The heatmap of species abundance was plotted using the R project pheatmap package (version 1.0.12) (Kolde and Kolde, 2015).
Chao1, ACE, Shannon, Simpson, and Good’s Coverage were considered to evaluate the Alpha diversity using QIIME (version 1.9.1) (Caporaso et al., 2010). Beta diversity, based on a weighted Unifrac distances at OTU levels, was calculated by GuniFrac package (version 1.0) in R project (Lozupone and Knight, 2005). The hierarchical clustering tree was calculated using the Unweighted Pair-group Method with Arithmetic Mean (UPGMA) method based on Bray-Curtis distances by QIIME software (version 1.9.1) (Caporaso et al., 2010). The functional profiles of the bacterial communities were predicted based on the Kyoto Encyclopedia for genes and genomes (KEGG) pathway using PICRUSt software (version 2.1.4) (Langille et al., 2013). Based on the annotation with the Greengenes database (OTUs with a 97% identity threshold), relative abundances of “potentially pathogenic” phenotype were analyzed using the BugBase web server. (Ward et al., 2017). Prediction of bacterial ecological functional profiles was performed using the Functional Annotation of Prokaryotic Taxa (FAPROTAX) database and associated software (version 1.0) (Louca et al., 2016). All statistical analyses, including Welch’s t-test, Wilcoxon rank test, Tukey’s HSD test, Kruskal-Wallis H test, Adonis and Anosim test, were conducted using R package software. Test for differences between the groups was performed using permutational multivariate analysis of variance (PERMANOVA). The statistical significance was set at P < 0.05.
Results
Sequencing analysis
After quality and chimera filtration of the raw reads, a total of 2,827,332 effective tags were obtained from the 27 samples, with an average of 102,182 and 106,743 effective sequences in the sediment and seawater samples, respectively. These sequences were clustered into 60,444 OTUs at a similarity level of 97%, which shared a total of 26,703 OTUs in 12 sediment samples and 33,741 OTUs in 15 seawater samples. In a further investigation of the OTUs distribution in all nine groups (each group = 3 samples), venn diagrams revealed that 418 and 333 core OTUs were observed in 4 sediment groups and 5 seawater groups (Figures 2A, B), respectively. A total of 168 core OTUs were shared in all 9 groups (Figure 2C). Total numbers of OTUs differed among the sediment groups, and the highest number of OTUs (3,920) was observed in the YQS group, while the lowest number of OTUs (1,949) was found in the XSS group. Similarly, the highest (4,156) and lowest (1,563) numbers of OTUs were detected in the YQW and TZW groups, respectively, showing differences among the seawater groups. Besides, the YQS and YQW groups had the highest number of unique OTUs (2,567 and 2,398) in their respective habitat groups.
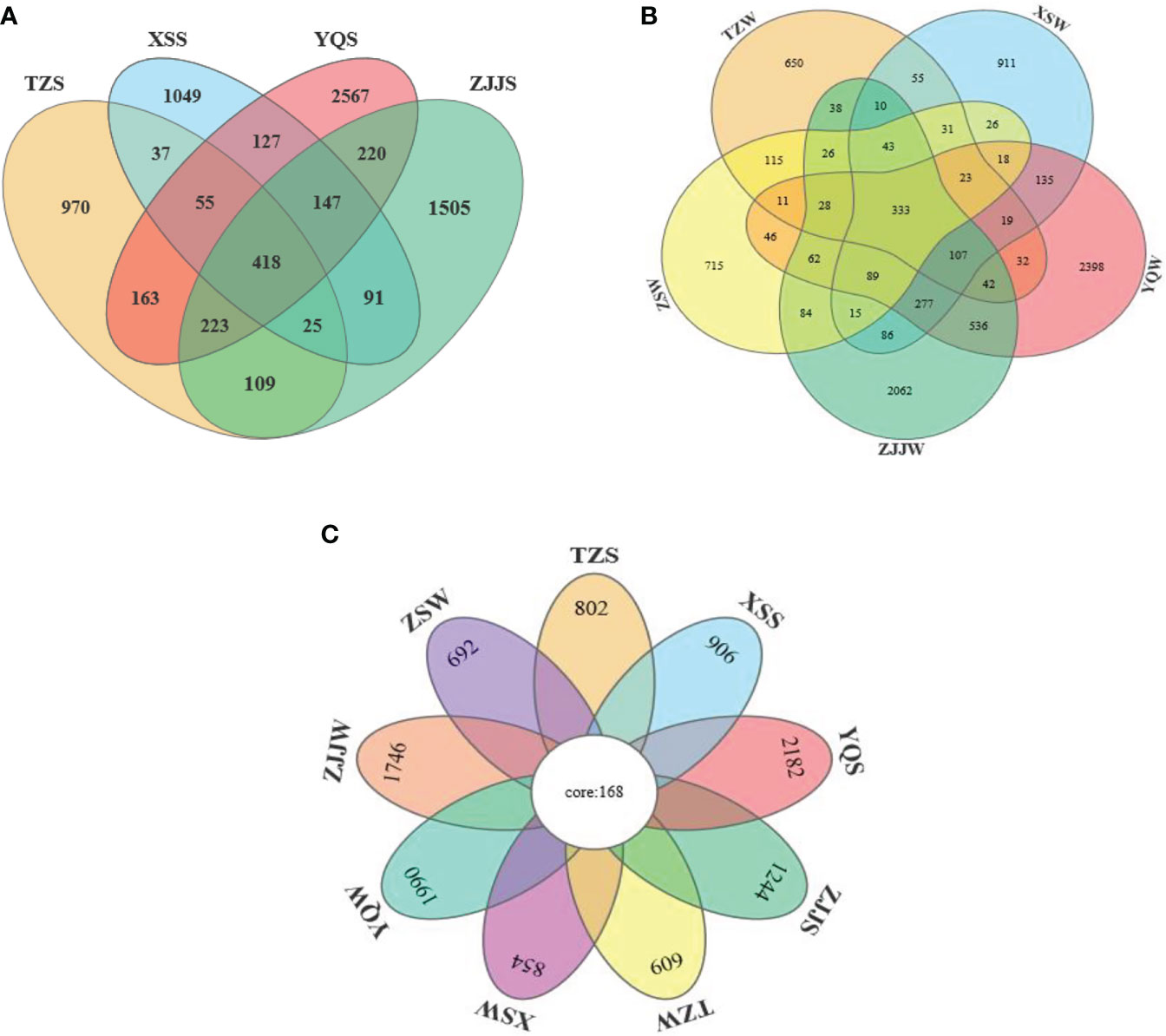
Figure 2 Venn diagrams of the OTUs distribution denoting the unique and core OTUs (A) in the sediment groups, (B) in the seawater groups and (C) in all nine groups.
Taxonomic composition of bacterial communities
The bacterial compositions for all seawater and sediment groups at the phylum level are shown in Figure 3A. In total, 55 identified phyla were observed, and the dominant phyla (relative abundance more than 10% at least in one group) were Proteobacteria, Bacteroidetes, Firmicutes, Epsilonbacteraeota, Actinobacteria, and Cyanobacteria (Table S2). Seawater and sediment groups at each sampling site, having a similar bacterial community composition for the dominant phyla, showed an abundance difference. Proteobacteria was the most abundant phylum in the TZS (42.1%), YQS (36.1%), and all seawater groups (30.4-77.3%). The phylum Bacteroidetes was the most dominant members in the XSS and ZJJS groups, with proportions of 57.1% and 52.6%, respectively. The highest relative abundances of other dominant phyla, including Firmicutes (27.3%), Epsilonbacteraeota (23.7%), Actinobacteria (22.4%), and Cyanobacteria (17.1%), were observed in the ZSW, YQS, ZSW and XSW groups, respectively. Furthermore, other phyla (Planctomycetes, Verrucomicrobia, Patescibacteria, and Chloroflexi) in the top 10 presented low abundance values (< 5%) for all groups.
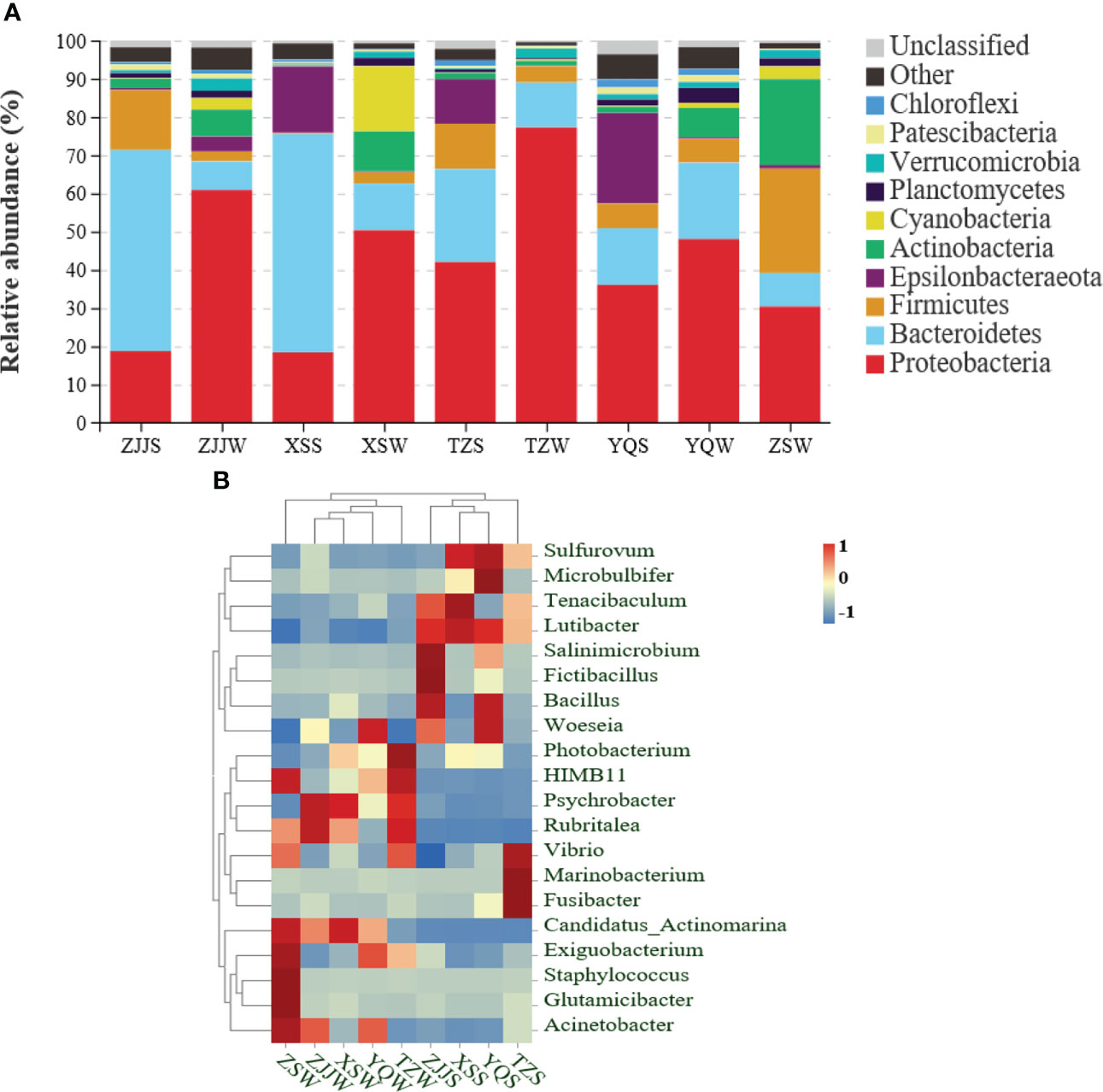
Figure 3 Relative abundance of dominant bacterial composition in each group. (A) Stacked bar plot analysis of bacterial abundance at the phylum level. The “Other” represents all other phyla outside the 10 listed phyla. (B) Heatmap analysis of the relative abundance of the top 20 genera. The relative abundance of each bacterial genus is depicted by the colour intensity ranged from 1 to -1.
At the genus level, a total of 205 bacterial genera were detected in all groups, and the top 20 genera with the higher relative abundance were used to generate the heatmap (Figure 3B). Results showed that seawater and sediment groups were divided into 2 separate clusters, suggesting a different bacterial composition of dominant genera between seawater and sediment. In addition, three inshore sites (YQ, ZJJ, and XS) exhibited a closer distance evolutionary relationship for each cluster. The dominant genera (relative abundance more than 10% at least in one group) were Psychrobacter (33.2% in ZJJW, 26.1% in XSW, and 23.6% in TZW), Tenacibaculum (45.7% in XSS, 22.4% in ZJJS, and 15.2% in TZS), Sulfurovum (23.6% in YQS and 17.3% in XSS) and Photobacterium (17.2% in TZW) (Table S3). The Tenacibaculum genus, with an average relative abundance of 21.1%, was the first dominant bacteria in 4 sediment groups, and the next most abundant bacterial genus was Sulfurovum (average abundance of 12.5%). For 5 seawater groups, the Psychrobacter genus had the highest average relative abundance (18.6%), followed by Photobacterium (average abundance of 5.8%) and Candidatus Actinomarina (average abundance of 4.9%). However, the genus Microbulbifer was not found in the TZW and ZSW groups.
Richness and diversity analysis
Shannon and Simpson indices were used to evaluate the species diversity (Table 1). For all 9 groups, the Shannon index ranged from to 4.54052 to 8.334583, and the Simpson index ranged from 0.836143 to 0.988079, which showed that the bacterial community diversity of the YQW group with maximum values of both indices was highest. The species richness was assessed by Chao and ACE indices (Table 1). The Chao1 index in 5 seawater and 4 sediment groups varied from 1690.59158 to 3551.65627, while the ACE index varied from 1780.89803 to 3670.66871. The highest Chao and ACE indices were also found in the YQW group. These results indicated that the YQW group had the most complex bacterial communities. Furthermore, the mean Good’s coverage of each group was more than 98.8% (ranging from 98.83% to 99.51%), suggesting that the sequencing depth was adequate to represent the bacterial community.
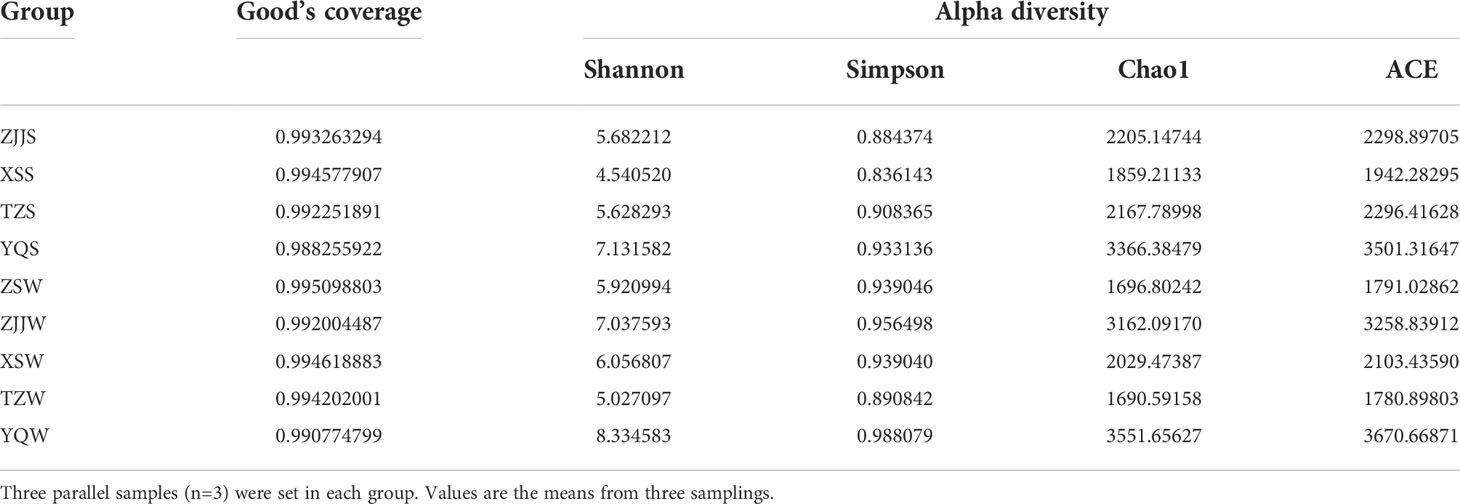
Table 1 Richness and diversity indexes of species in all nine groups at the similarity threshold of 97%.
The distribution boxplot (Figure 4) showed that the overall diversity (Shannon and Simpson indices) and richness (Chao1 and ACE indices) of the bacterial community had significant differences (P < 0.05) between the two habitat environments. The average Shannon and Simpson indices of YQW and XSW seawater groups were significantly higher (P < 0.05) compared to the YQS and XSS sediment groups, respectively, suggesting that the bacterial diversity of the seawater group was higher than that of the sediment group in these 2 sites. However, no significant difference was observed in their Chao1 and ACE indices (P > 0.05). In addition, the richness and diversity of the community did not display statistically significant differences (P > 0.05) between seawater and sediment groups from other sites, indicating that the bacterial diversity presented similar features in these seawater and sediment.
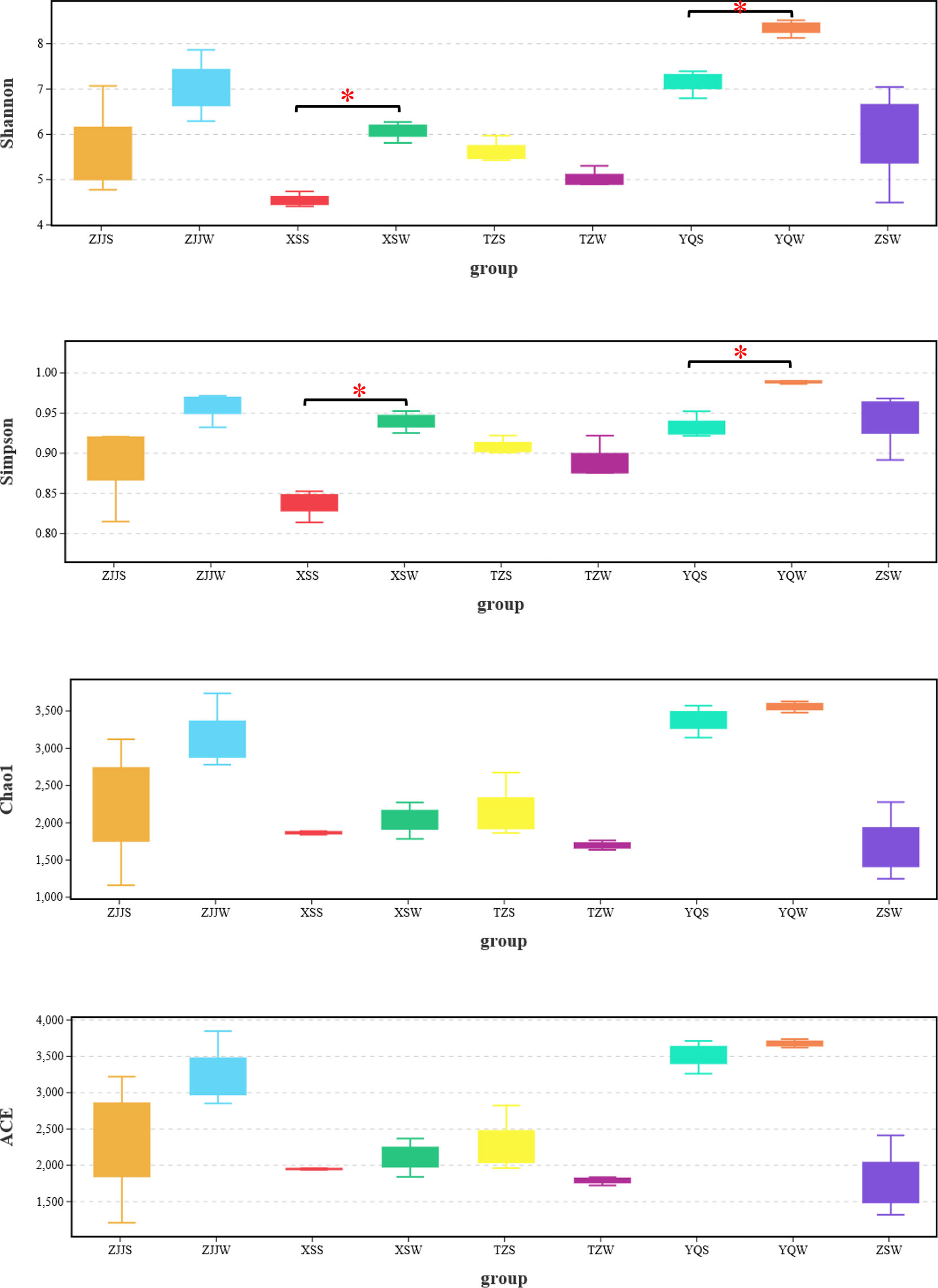
Figure 4 The alpha diversity comparison among all seawater and sediment groups via Shannon, Simpson, Chao1 and ACE indices. The “ * “ represents significant difference (P < 0.05) between the two groups.
The differences in bacterial community compositions among the seawater and sediment groups were further investigated at the OTU level through Principal Coordinate Analysis (PCoA) based on a weighted UniFrac distance and UPGMA with the bray-curtis distance. The PCoA results (Figure 5A) revealed that the bacterial communities of seawater and sediment groups were clustered separately and explained 33.91% (PCo1) and 15.36% (PCo2) of the variation, although there were some overlaps in ordination space between individual TZS and YQW samples. Distinct differences in the community compositions of seawater and sediment groups were also observed by UPGMA analysis (Figure 5B), generating 2 major independent clusters in the dendrogram except for the ZJJS2 sample.
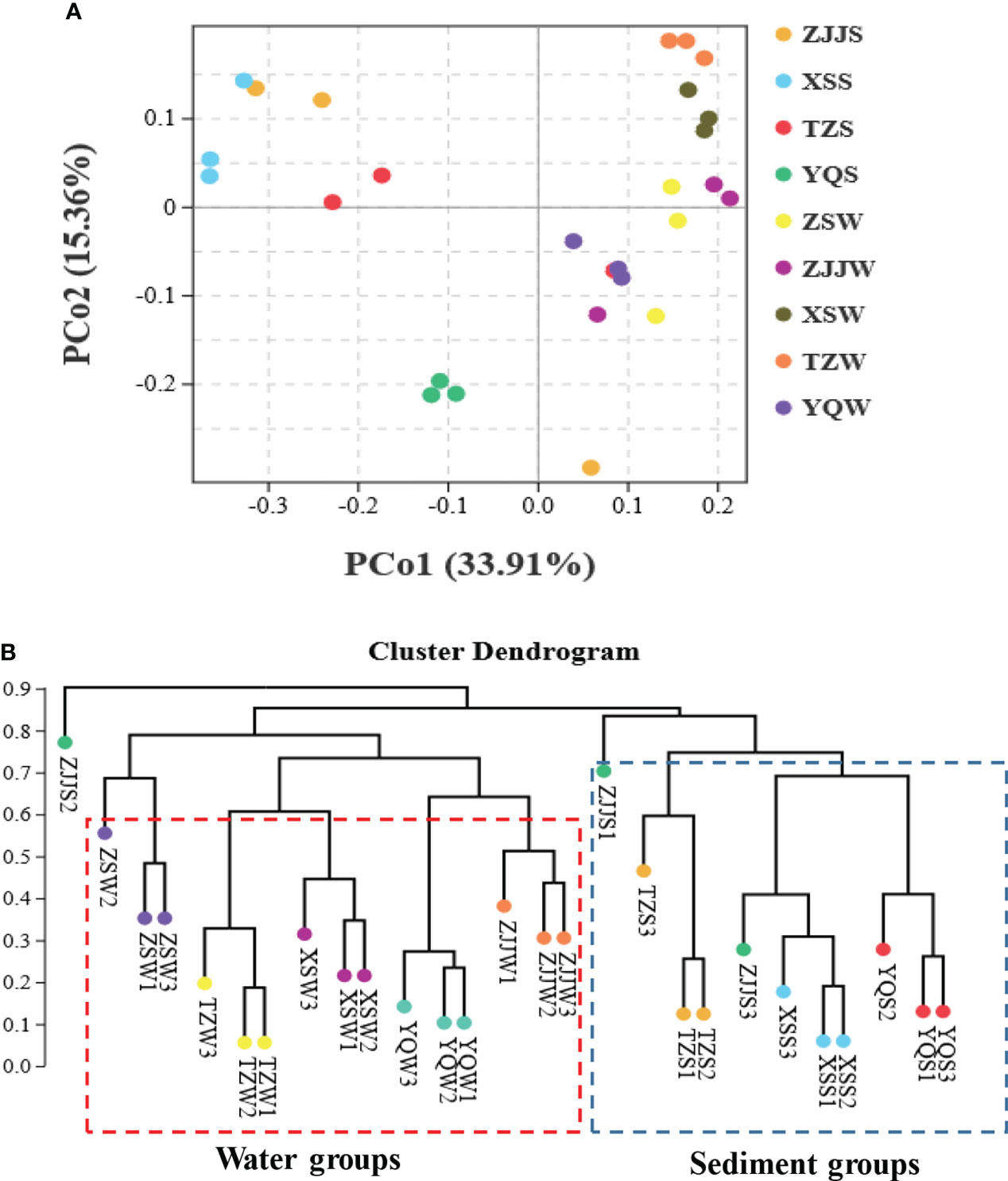
Figure 5 The bacterial community structural differences among the seawater and sediment groups. (A) Principal component analysis (PCoA) based on a weighted UniFrac distance; (B) UPGMA analysis based on a bray-curtis distance.
Functional prediction of the bacterial communities
The results of PICRUSt functional prediction (Figures 6A, B) showed that a total of 33 functional subcategories (level 2) were identified in both seawater and sediment groups, affiliating to the categories (level 1) of Metabolism, Genetic information processing, Cellular processes, Environmental information processing, Organismal systems and Human diseases. However, the subcategories of the Nervous system and Cancers were only found in the seawater groups. Besides, 183 KEGG Orthologies (KO) (level 3) were found across all samples. The most dominant KEGG pathways among all 9 groups have been presented in the heatmaps (Figure 6C), of which the top 10 abundant functional pathways were Biosynthesis of ansamycins (ko01051), Valine, leucine and isoleucine biosynthesis (ko00290), C5-Branched dibasic acid metabolism (ko00660), Fatty acid biosynthesis (ko00061), Biosynthesis of vancomycin group antibiotics (ko01055), Synthesis and degradation of ketone bodies (ko00072), D-Glutamine and D-glutamate metabolism (ko00471), Pantothenate and CoA biosynthesis (ko00770), Lipoic acid metabolism (ko00785), and Peptidoglycan biosynthesis (ko00550). Functional cluster analysis suggested that 9 groups were divided into 2 relatively independent clusters (Figure 6C). Three sediment groups, ZJJS, XSS, and YQS, from inshore sites were clustered together. However, another sediment group TZS from the offshore site, was clustered together with 5 seawater groups, indicating that the bacterial taxa of TZS and seawater maintained similar biological functions.
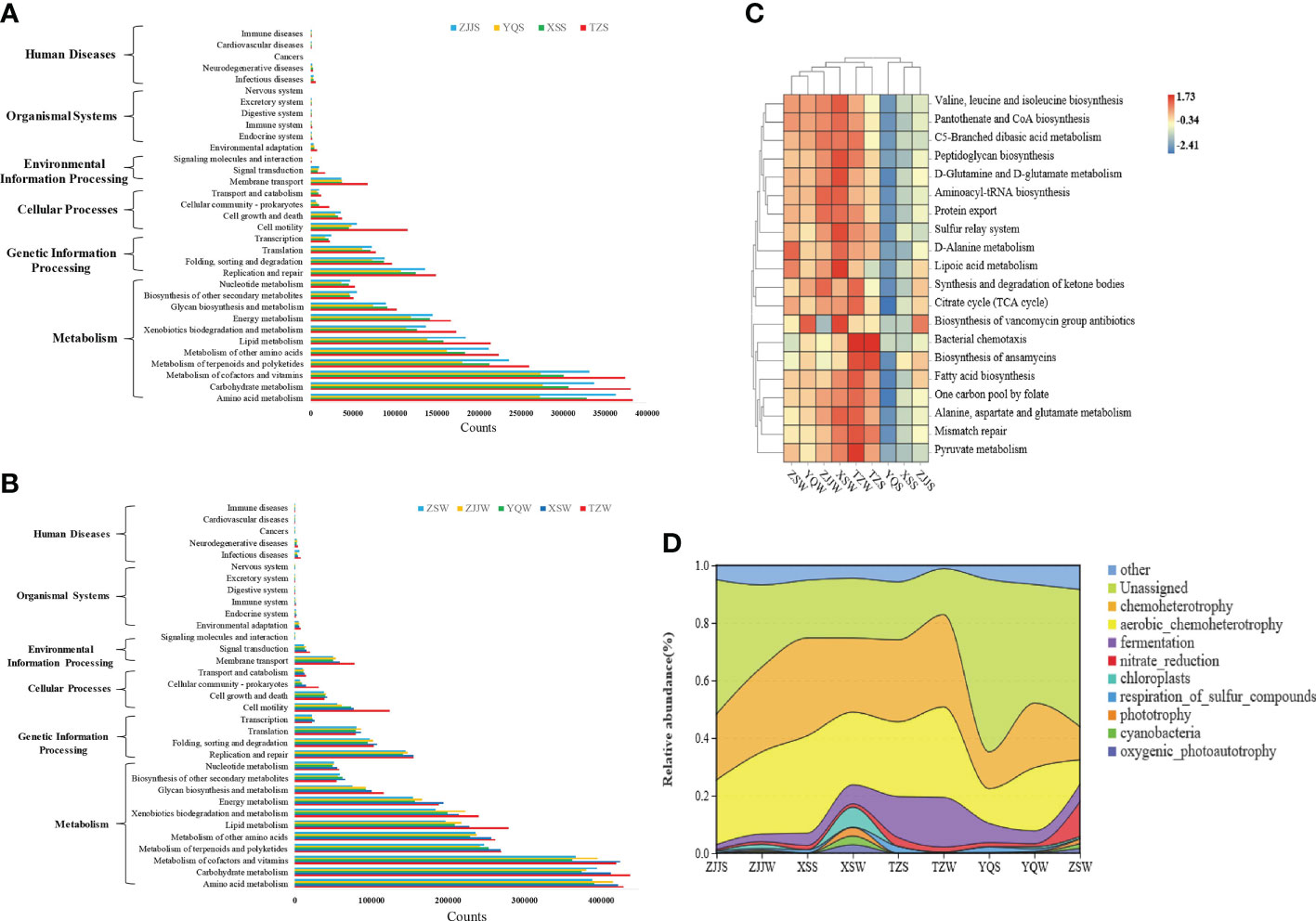
Figure 6 Predicted functional profiles for bacterial community by PICRUSt and FAPROTAX. (A, B) Histograms show the predicted functional categories (level 1) and subcategories (level 2) in the seawater and sediment groups. (C) Heatmap shows the top 20 abundant KEGG pathways (level 3) in all groups. (D) Rivermap shows the top 10 abundant ecological functional classes in all groups.
FAPROTAX was used to further analyze the ecological function of bacterial communities, especially the biogeochemical cycles of carbon, nitrogen, and sulfur. As shown in Figure 6D, a total of 73 putative ecological functional classes were identified for all seawater and sediment groups, and the most abundant functions were unassigned, chemoheterotrophy, aerobic chemoheterotrophy and fermentation, which (excluding “unassigned”) contributed to C cycle. Bacterial functions for N/S cycles mainly consisted of nitrate reduction and respiration of sulfur compounds, respectively. Overall, bacterial ecological functional profiles were not significantly different between the seawater and sediment groups, which is consistent with the results of the PICRUSt prediction.
Discussion
Microbial communities play a pivotal role in energy and matter cycles in the sea, and the stability of the micro-ecological environment is a prerequisite for the aquaculture fish health (Olafsen, 2001; Vadstein et al., 2018). Previous studies mainly focused on the bacterial community compositions of aquaculture environments in pond ecosystems (Zhao et al., 2020; Zhang et al., 2021; Zeng et al., 2021). However, microbial communities in the open-oceanic mariculture ecosystems are understudied compared to the pond environment microorganisms. Until now, the bacterial community structure and diversity regarding types of marine fish cage culture have never been reported. In the present study, the bacterial structure of seawater and sediments from 5 offshore and inshore large yellow croaker farms was investigated by high-throughput sequencing.
Bacterial community composition of large yellow croaker aquaculture environments
For the 5 seawater groups, Proteobacteria and Bacteroidetes were the 2 most dominant phyla, which is consistent with some previous results of the bacterial communities in seawater (Suh et al., 2015; Nurul et al., 2019; Nimnoi and Pongsilp, 2020). A similar study has also reported in the pond aquaculture water of sea cucumber (Apostichopus japonicas) (Zhao et al., 2020), in the recirculating aquaculture water of marine fish (Martins et al., 2013) and in the carriage water of ornamental fish (Gerzova et al., 2014). Bacteria in these phyla play a key role in the marine biogeochemical processes. Members of the phylum Proteobacteria are considered to have a specialized role in both aerobic and anaerobic biodegradation (Táncsics et al., 2012; Nho et al., 2018), whereas the Bacteroides phylum shows to be functional in organic pollutants removal (Mayer et al., 2016; Wang F. et al., 2021). Other major phyla Actinobacteria, Firmicutes and Cyanobacteria mentioned in the seawater groups, were also abundant. Actinobacteria was involved in degrading polycyclic aromatic hydrocarbons and polysaccharides and participating in the carbon recycling process (Chen et al., 2016). However, Actinobacteria and Firmicutes in the ZSW group were significantly more abundant than in other seawater groups, and Cyanobacteria in the XSW group was significantly higher. These results suggested that geographical positions influence the microflora abundance in the open-oceanic mariculture environments of large yellow croaker.
The composition analysis of bacterial community structures showed that the 2 most dominant phyla in 4 sediment groups were Bacteroidetes and Proteobacteria, accounting for a large proportion of the identified bacteria. Other reports on bacterial compositions in the marine sediments (Duncan et al., 2014; Choi et al., 2016; Ye et al., 2016) have also presented similar results, which agreed with the bacterial community in pond sediments related to some reared organisms (Fan et al., 2017; Liu et al., 2020). We found that Epsilonbacteraeota and Firmicutes were the 2 other major phyla in the sediment groups. Previous studies indicated that the phylum Firmicutes as a major bacterial community was relatively stable in the pond sediments (Fan et al., 2017). The phylum Epsilonbacteraeota is generally considered be one of the minor bacterial taxa in marine environments. However, Epsilonbacteraeota was identified as a major phylum in our study, which was also observed in the sediment of A. japonicus culture ponds in Dalian, China (Zhao et al., 2020). The relative abundance of the dominant phyla in the sediment groups responded to site differences. For example, the relative abundance of Epsilonbacteraeota in the ZJJS group was significantly lower than that in other sediment groups, whereas the relative abundance of Firmicutes was significantly lower in the XSS group.
Geographical positions affect bacterial community diversity
Bacterial species diversity analysis was performed to further explore whether the bacterial communities in the marine cage system are influenced by site-specific conditions. Variations in the bacterial community diversity were significant among different groups. Especially, the sampling site YQ possessed the highest Simpson, Shannon, Chao1, and ACE indices in both seawater and sediment groups, implying that the bacterial diversity in this site was higher than in other stations. As shown in Figure 1, the inshore site YQ located in the bay area is closer to the inland and easily influenced by humans because of agricultural, industrial and sanitary sewage discharge activities. Our previous study (Jin et al., 2017) also reported that seawater in the Yueqing (YQ) bay had a high level of faecal coliform and total bacterial count, associated with the impacts of river confluences and human beings. Thus, it could explain why the seawater and sediment from YQ exhibited higher bacterial diversity.
Comparing 5 seawater groups showed that bacterial richness and diversity presented higher values in three inshore sampling sites YQ, ZJJ and XS (Table 1). In these 3 sites, all indices were higher in seawater groups than in sediment groups, which is inconsistent with many previous findings in various environments, including marine (Wang et al., 2012), rivers (Abia et al., 2018), and aquaculture ponds (Guan et al., 2020). Bacterial community structure and diversity are affected by environmental factors, including temperature, pH, DO, salinity, nutrients, and heavy metals (Wang C. et al., 2021). Notably, pH, DO, and salinity levels were much lower in 3 inshore stations than in the other offshore stations (Table S1), which may be caused by the river and sewage input (Li et al., 2014). A large number of nutrient substances and pollutants are released to the inshore zone, altering the coastal aquaculture environments and influencing bacterial diversity (Ouyang et al., 2020). Relative to the sediment with niche stability, continuous flowing seawater is a dynamic liquid and is more susceptible to environmental changes (Ul-Hasan et al., 2019), which is the possible reason that the higher bacterial diversity was observed in the seawater groups than in sediment groups in these inshore sites. In addition, latitude that can change the distribution of temperature is considered be the other factor impacting the bacterial community in this study, which can be supported by previous studies (Wang S. et al., 2019). Thus, the variation of the above environmental conditions in the inshore and offshore sampling sites explained the difference in bacterial community diversity.
Potential pathogens and probiotics related to fish health
The phyla Proteobacteria, Firmicutes, and Bacteroides have been found to be predominated in large yellow croaker gut (Wei et al., 2018; Zhang C. et al., 2019). Compared with aquaculture environments in the present study, their compositions of dominant bacterial phyla were similar, which provides an important clue on the relationship of the bacterial communities in the large yellow croaker gut and the surrounding environments. Potential pathogens enrichment in an aquaculture environment can induce stress to fish guts and lead to bacterial infections in the fish digestive systems. To further assess or early forecast the risk of fish disease emergence by potentially pathogenic bacteria in the aquaculture environments, a “potentially pathogenic” phenotype prediction was performed using BugBase in this study. The contribution to “potentially pathogenic” phenotype at the phylum level showed that the abundance of potential pathogens was significantly varied between the seawater and sediment groups, and higher values were observed in seawater groups at the same sampling site (Figure S1). Proteobacteria, Bacteroidetes, and Crenarchaeota were the main pathogenic phyla in 4 sediment groups, while 5 dominant phyla Proteobacteria, Actinobacteria, Bacteroidetes, Cyanobacteria, and Firmicutes were found to be the main pathogens in all seawater groups, consistent with the bacterial community results. Among the pathogenic phyla identified, Proteobacteria had the highest abundance, accounting for 10.3%-35.0% of total phyla in each groups, which indicates that Proteobacteria as a main pathogenic contributing phylum can pose a significant health threat to cause diseases in the large yellow croaker intestines.
At the genus level, the abundance of potential pathogens in all 9 groups varied from 5.4% to 35.9% of total genera and obviously was higher in the seawater groups than that in sediment groups except for YQ (Figure 7). The top 5 pathogenic genera were Psychrobacter, Vibrio, Staphylococcus, Photobacterium and Exiguobacterium in seawater groups, while Vibrio, Microbulbifer, GOUTA19, Photobacterium and Psychrobacter were the main pathogenic genera in sediment groups (Table S4). The dominant pathogenic bacteria identified in this study included some of the most common fish pathogens, including the genera Vibrio, Photobacterium, and Staphylococcus in both the seawater and sediment groups. Vibrios as the traditional opportunistic pathogens are generally related to fish disease outbreaks (Gomez-Gil et al., 2014), and especially several species (e.g., V. alginolyticus, V. harveyi, V. parahaemolyticus, and V. anguillarum) have been reported to be pathogenic for the cultured large yellow croaker (Wang R. X. et al., 2005; Shan et al., 2005; Liu et al., 2016). Bacteria from the Photobacterium genera, for example, Ph. damselae subsp. piscicida, can cause severe fish pasteurellosis with disease symptoms of white nodules in the spleen and kidney (Toranzo et al., 2005; Jurelevicius et al., 2021). Staphylococcus aureus colonizes the skin and mucous membrane, leading to eye disease and ulcerations in marine fish when any break in the skin or compromise in the immune systems occurs (Pal et al., 2020; Jurelevicius et al., 2021). The occurrence of these pathogenic genera (Vibrio, Photobacterium, and Staphylococcus) in higher percentages indicate that they may pose a prospective threat to the large yellow croaker health.
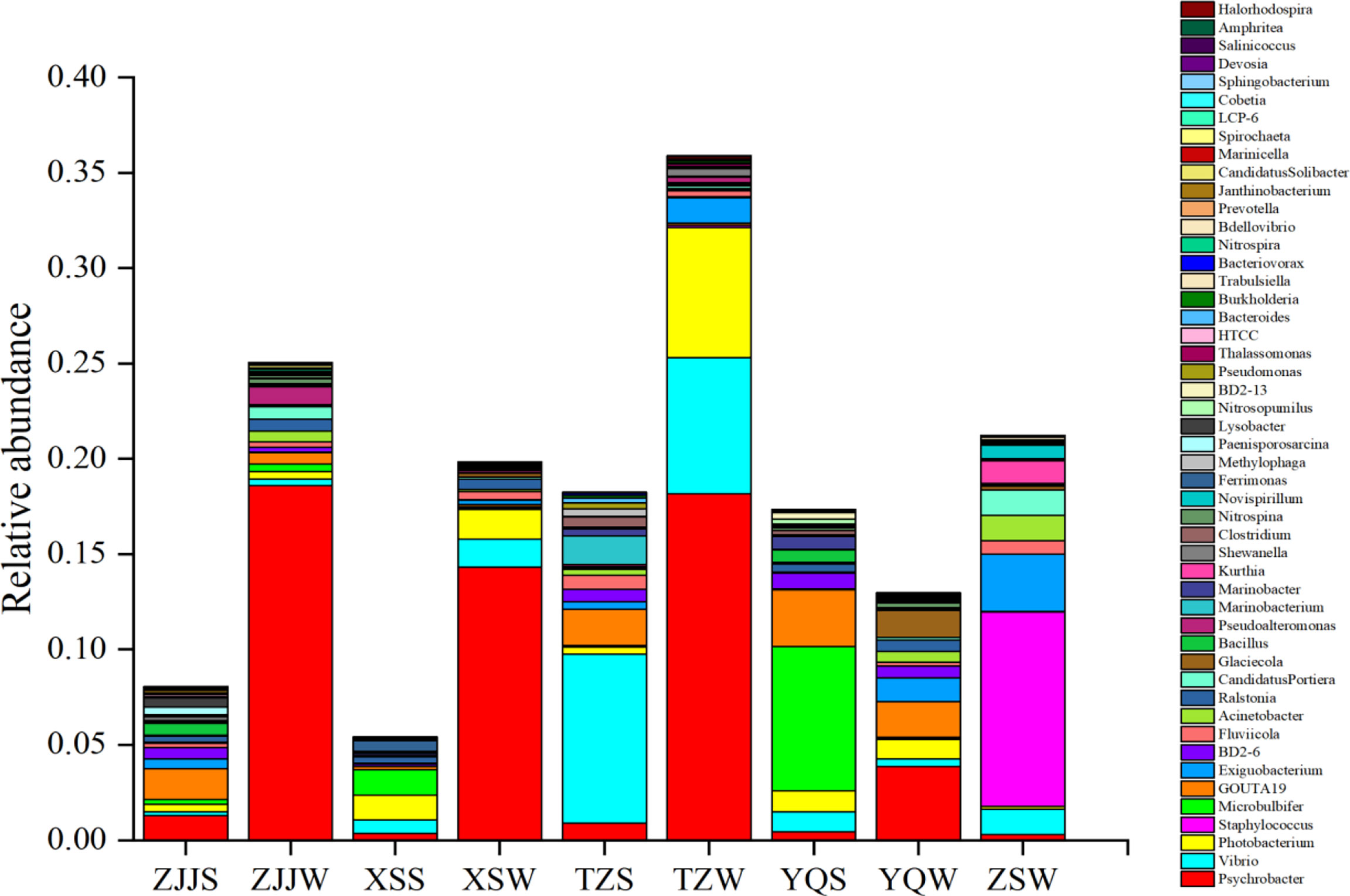
Figure 7 The species and relative abundance of potential pathogenic bacteria in the top 50 genera using the BugBase prediction.
Other fish pathogens that occupied a certain proportion in the top 50 genera also included Acinetobacter, Clostridium, Pseudomonas, and Burkholderia (Table S4). The presence of the Pseudomonas genus is a great concern, as is its species P. plecoglossicida associated with VWND outbreaks in farmed large yellow croaker (Shen et al., 2008; Li C. et al., 2020). The genus Acinetobacter was regarded as an emerging fish pathogen. It can cause severe skin diseases, which displays symptoms of shedding scales, tail-rot and gentle ulceration on the entire body (Li J. et al., 2017). Some species (e.g., A. pittii, A. calcoaceticus, A. baumannii and A. nosocomialis) are grave human clinical pathogens, which can cause various infections, such as secondary meningitis, bacteremia, and ventilator-associated pneumonia. Due to its prevalence across all seawater and sediment environments, it was worth showing more concern for a high risk of Acinetobacter infection to aquaculture fish and humans in this study. Generally, the genus Tenacibaculum, a widespread member of the marine microbiota, has been associated with skin lesions and fin rottenness in marine fish, including T. maritimum, T. discolor, T. gallaicum, T. ovolyticum, T. soleae, T. dicentrarchi, etc (Avendaño-Herrera et al., 2006; Pineiro-Vidal et al., 2008; Olsen et al., 2017). Heatmap analysis of bacterial community at the genus level (Figure 3B) showed high relative abundance values for Tenacibaculum in all 9 groups. However, the genus Tenacibaculum was detected with a low relative abundance for the contribution to “potential pathogenic” phenotype, which indicated that the pathogenic Tenacibaculum sp. appeared to occupy an extremely low proportion of the identified Tenacibaculum species. Notably, Tenacibaculum members in the present study could not pose a potential threat to the health of cage-culture large yellow croaker.
The probiotic bacteria are effective in improving water quality, inhibiting potential pathogens and enhancing host immunity in aquaculture. Some common probiotic species, such as Bacillus subtilis (Ai et al., 2011) and Clostridium butyricum (Yin et al., 2021), have been confirmed to be positive in improving immune response and promoting intestinal development and disease resistance for large yellow croaker. In the present study, probiotic bacterial genera Bacillus, Pseudoalteromonas, Shewanella, Kocuria, Bacteroides, Enterococcus, and Nitrobacter were detected in the aquaculture environment, and both the composition and abundance of probiotic bacterial genera were similar between seawater and sediment groups. However, the proportion of probiotics was relatively low in contrast to the pathogens, which corresponds with the result of Zhang et al. (2016) who reported that probiotics and pathogenic bacterial genera showed a negative correlation for the abundance in the aquaculture environments of white shrimp (Litopenaeus vannamei). The abundance of probiotics and pathogens was correlated with the environmental factors (pH, WT, TP, TN, and COD, etc.) (Zhu et al., 2013). Thus, further studies to assess the functional relationship between environmental factors and bacterial communities in the aquaculture environment of large yellow croaker are needed, which can facilitate microbiota management to control bacterial disease in the marine fish-cage aquaculture.
Conclusion
The present study provides baseline data on bacterial communities and diversity in the open-oceanic environments of cage-culture large yellow croaker along the Zhejiang coast in China. A total of 2,827,332 effective tags were achieved from 27 samples. Meanwhile, 418 and 333 core OTUs were observed in 4 sediment groups and 5 seawater groups, respectively. The most dominant phyla were Proteobacteria, Bacteroidetes, Firmicutes, Epsilonbacteraeota, Actinobacteria, and Cyanobacteria in all groups. At the genus level, Psychrobacter, Tenacibaculum, Sulfurovum, and Photobacterium were the dominant bacteria shared by the sediment and seawater groups. The inshore site YQ showed a higher bacterial diversity and richness than other sampling sites. The bacterial diversity observed at 3 inshore sampling sites (YQ, ZJJ, and XS) was higher in seawater groups than that in sediment groups. A total of 6 functional categories, 33 subcategories, and 183 KOs were found in both seawater and sediment groups, and 73 putative ecological functional classes were identified. Furthermore, the “potentially pathogenic” phenotype prediction revealed Proteobacteria was the main pathogenic contributing phylum. The 5 dominant genera Psychrobacter, Vibrio, Staphylococcus, Photobacterium, and Exiguobacterium, were the dominant pathogens in seawater groups, while the top 5 genera contributed to potential pathogenicity in sediment groups were as follows: Vibrio, Microbulbifer, GOUTA19, Photobacterium, and Psychrobacter. Other common fish pathogens had a certain percentage in the top 50 genera, including Acinetobacter, Clostridium, Pseudomonas, and Burkholderia. Probiotic bacterial genera Bacillus, Pseudoalteromonas, Shewanella, Kocuria, Bacteroides, Enterococcus, and Nitrobacter constituted low proportions in all groups.
Data availability statement
The datasets presented in this study can be found in online repositories. The name of the repository and accession numbers can be found in the article.
Author contributions
TL contributed to the design of the experiment and performed data analysis. DG conducted the experiments and drafed the manuscript. TL and DG revised the manuscript, and contributed equally to this article. YS prepared materials and assisted in samples collecting. JB participated in the data collection and analysis. LJ was the principal investigator who designed the study and finalized the article. All authors contributed to the article and approved the submitted version.
Funding
This research was funded by the Priority Academic Project of Zhejiang Marine Fisheries Research Institute (HYS-ZX-202103) and the Postgraduate Research & Practice Innovation Program of Jiangsu Province (KYCX21_2607).
Conflict of interest
The authors declare that the research was conducted in the absence of any commercial or financial relationships that could be construed as a potential conflict of interest.
Publisher’s note
All claims expressed in this article are solely those of the authors and do not necessarily represent those of their affiliated organizations, or those of the publisher, the editors and the reviewers. Any product that may be evaluated in this article, or claim that may be made by its manufacturer, is not guaranteed or endorsed by the publisher.
Supplementary material
The Supplementary Material for this article can be found online at: https://www.frontiersin.org/articles/10.3389/fmars.2022.963242/full#supplementary-material
References
Abia A. L. K., Alisoltani A., Keshri J., Ubomba-Jaswa E. (2018). Metagenomic analysis of the bacterial communities and their functional profiles in water and sediments of the apies river, south Africa, as a function of land use. Sci. Total. Environ. 616, 326–334. doi: 10.1016/j.scitotenv.2017.10.322
Ai Q., Xu H., Mai K., Xu W., Wang J., Zhang W. (2011). Effects of dietary supplementation of bacillus subtilis and fructooligosaccharide on growth performance, survival, non-specific immune response and disease resistance of juvenile large yellow croaker, Larimichthys crocea. Aquaculture 317 (1-4), 155–161. doi: 10.1016/j.aquaculture.2011.04.036
Avendaño-Herrera R., Toranzo A. E., Magariños B. (2006). Tenacibaculosis infection in marine fish caused by Tenacibaculum maritimum: a review. Dis. Aquat. Organ. 71 (3), 255–266. doi: 10.3354/dao071255
Blancheton J. P., Attramadal K. J. K., Michaud L., d’Orbcastel E. R., Vadstein O. (2013). Insight into bacterial population in aquaculture systems and its implication. Aquacult. Eng. 53, 30–39. doi: 10.1016/j.aquaeng.2012.11.009
Bokulich N. A., Subramanian S., Faith J. J., Gevers D., Gordon J. I., Knight R., et al. (2013). Quality-filtering vastly improves diversity estimates from illumina amplicon sequencing. Nat. Methods 10 (1), 57–59. doi: 10.1038/nmeth.2276
Caporaso J. G., Kuczynski J., Stombaugh J., Bittinger K., Bushman F. D., Costello E. K., et al. (2010). QIIME allows analysis of high-throughput community sequencing data. Nat. Methods 7 (5), 335–336. doi: 10.1038/nmeth.f.303
Chen Z., Chang Z., Zhang L., Jiang Y., Ge H., Song X., et al. (2019). Effects of water recirculation rate on the microbial community and water quality in relation to the growth and survival of white shrimp (Litopenaeus vannamei). BMC Microbiol. 19 (1), 1–15. doi: 10.1186/s12866-019-1564-x
Chen Y., Huang W., Shan X., Chen J., Weng H., Yang T., et al. (2020). Growth characteristics of cage-cultured large yellow croaker Larimichthys crocea. Aquacult. Rep. 16, 100242. doi: 10.1016/j.aqrep.2019.100242
Chen S., Su Y., Hong W. (2018a). Aquaculture of the large yellow croaker. Aquacult. China. 10, 297–308. doi: 10.1002/9781119120759.ch3_10
Chen P., Zhang L., Guo X., Dai X., Liu L., Xi L., et al. (2016). Diversity, biogeography, and biodegradation potential of actinobacteria in the deep-sea sediments along the southwest Indian ridge. Front. Microbiol. 7, 1340. doi: 10.3389/fmicb.2016.01340
Chen S., Zhou Y., Chen Y., Gu J. (2018b). Fastp: an ultra-fast all-in-one FASTQ preprocessor. Bioinformatics 34 (17), i884–i890. doi: 10.1093/bioinformatics/bty560
Choi H., Koh H. W., Kim H., Chae J. C., Park S. J. (2016). Microbial community composition in the marine sediments of jeju island: next-generation sequencing surveys. J. Microbiol. Biotechn. 26 (5), 883–890. doi: 10.4014/jmb.1512.12036
Cornall A., Rose A., Streten C., McGuinness K., Parry D., Gibb K. (2016). Molecular screening of microbial communities for candidate indicators of multiple metal impacts in marine sediments from northern Australia. Environ. Toxicol. Chem. 35 (2), 468–484. doi: 10.1002/etc.3205
Del'Duca A., Cesar D. E., Diniz C. G., Abreu P. C. (2013). Evaluation of the presence and efficiency of potential probiotic bacteria in the gut of tilapia (Oreochromis niloticus) using the fluorescent in situ hybridization technique. Aquaculture 388, 115–121. doi: 10.1016/j.aquaculture.2013.01.019
Duncan K., Haltli B., Gill K. A., Kerr R. G. (2014). Bioprospecting from marine sediments of new Brunswick, Canada: exploring the relationship between total bacterial diversity and actinobacteria diversity. Mar. Drugs 12 (2), 899–925. doi: 10.3390/md12020899
Edgar R. C. (2013). UPARSE: highly accurate OTU sequences from microbial amplicon reads. Nat. Methods 10 (10), 996–998. doi: 10.1038/nmeth.2604
Edgar R. C., Haas B. J., Clemente J. C., Quince C., Knight R. (2011). UCHIME improves sensitivity and speed of chimera detection. Bioinformatics 27 (16), 2194–2200. doi: 10.1093/bioinformatics/btr381
Fan L., Barry K., Hu G., Meng S., Song C., Qiu L., et al. (2017). Characterizing bacterial communities in tilapia pond surface sediment and their responses to pond differences and temporal variations. World J. Microbiol. Biotechnol. 33 (1), 1–12. doi: 10.1007/s11274-016-2144-y
Fan L., Wang Z., Chen M., Qu Y., Li J., Zhou A., et al. (2019). Microbiota comparison of pacific white shrimp intestine and sediment at freshwater and marine cultured environment. Sci. Total. Environ. 657, 1194–1204. doi: 10.1016/j.scitotenv.2018.12.069
Gerzova L., Videnska P., Faldynova M., Sedlar K., Provaznik I., Cizek A., et al. (2014). Characterization of microbiota composition and presence of selected antibiotic resistance genes in carriage water of ornamental fish. PloS One 9 (8), e103865. doi: 10.1371/journal.pone.0103865
Giri S. S., Sukumaran V., Oviya M. (2013). Potential probiotic Lactobacillus plantarum VSG3 improves the growth, immunity, and disease resistance of tropical freshwater fish, Labeo rohita. Fish. Shellfish. Immun. 34 (2), 660–666. doi: 10.1016/j.fsi.2012.12.008
Gomez-Gil B., Thompson C. C., Matsumura Y., Sawabe T., Iida T., Christen R., et al. (2014). “The famlily vibrionaceae,” in The prokaryotes: Gammaproteobacteria (Berlin Heidelberg: Springer), pp.660–713.
Guan X., Wang B., Duan P., Tian J., Dong Y., Jiang J., et al. (2020). The dynamics of bacterial community in a polyculture aquaculture system of Penaeus chinensis, Rhopilema esculenta and Sinonovacula constricta. Aquac. Res. 51 (5), 1789–1800. doi: 10.1111/are.14528
Jin L., Liu H., Xu D., Gu B. (2017). Parabolic stationing method application to intertidal shellfish (Sinonovacula constricta) and growing waters microbiological monitoring in yueqing bay, China. Mar. pollut. Bull. 116 (1-2), 64–70. doi: 10.1016/j.marpolbul.2016.12.061
Jorgensen S. L., Hannisdal B., Lanzén A., Baumberger T., Flesland K., Fonseca R., et al. (2012). Correlating microbial community profiles with geochemical data in highly stratified sediments from the Arctic mid-ocean ridge. P. Natl. Acad. Sci. U.S.A. 109 (42), E2846–E2855. doi: 10.1073/pnas.1207574109
Jurelevicius D., Cotta S. R., Montezzi L. F., Dias A. C., Mason O. U., Picão R. C., et al. (2021). Enrichment of potential pathogens in marine microbiomes with different degrees of anthropogenic activity. Environ. pollut. 268, 115757. doi: 10.1016/j.envpol.2020.115757
Kolde R., Kolde M. R. (2015). Package ‘pheatmap’. r package, Vol. 1. 790. Available online: https://cran.r-project.org/web/packages/pheatmap/ (accessed on 1 November 2019).
Langille M. G., Zaneveld J., Caporaso J. G., McDonald D., Knights D., Reyes J. A., et al. (2013). Predictive functional profiling of microbial communities using 16S rRNA marker gene sequences. Nat. Biotechnol. 31 (9), 814–821. doi: 10.1038/nbt.2676
Li J., Cao J., Wang X., Liu N., Wang W., Luo Y. (2017). Acinetobacter pittii, an emerging new multi-drug resistant fish pathogen isolated from diseased blunt snout bream (Megalobrama amblycephala yih) in China. Appl. Microbiol. Biot. 101 (16), 6459–6471. doi: 10.1007/s00253-017-8392-4
Li T., Li H., Gatesoupe F. J., She R., Lin Q., Yan X., et al. (2017). Bacterial signatures of “Red-operculum” disease in the gut of crucian carp (Carassius auratus). Microb. Ecol. 74 (3), 510–521. doi: 10.1007/s00248-017-0967-1
Li J., Wang H., Liu Y., Lin M., Liu X., Hu X. (2014). Distribution and diversity of coliform bacteria in estuary of jiahe river, China. Int. J. Environ. Res. 8 (2), 501–508. doi: 10.3967/bes2014.045
Li C., Wang S., Ren Q., He T., Chen X. (2020). An outbreak of visceral white nodules disease caused by Pseudomonas plecoglossicida at a water temperature of 12 °C in cultured large yellow croaker (Larimichthys crocea) in China. J. Fish. Dis. 43 (11), 1353–1361. doi: 10.1111/jfd.13206
Li C. H., Xiong J. B., Ding F. F., Chen J. (2020). Immune and gut bacterial successions of large yellow croaker (Larimichthys crocea) during Pseudomonas plecoglossicida infection. Fish. Shellfish. Immun. 99, 176–183. doi: 10.1016/j.fsi.2020.01.063
Liu M., De Mitcheson Y. S. (2008). Profile of a fishery collapse: why mariculture failed to save the large yellow croaker. Fish. Fish. 9 (3), 219–242. doi: 10.1111/j.1467-2979.2008.00278.x
Liu L., Ge M., Zheng X., Tao Z., Zhou S., Wang G. (2016). Investigation of Vibrio alginolyticus, V. harveyi, and V. parahaemolyticus in large yellow croaker, Pseudosciaena crocea (Richardson) reared in xiangshan bay, China. Aquacult. Rep. 3, 220–224. doi: 10.1016/j.aqrep.2016.04.004
Liu Z., Iqbal M., Zeng Z., Lian Y., Zheng A., Zhao M., et al. (2020). Comparative analysis of microbial community structure in the ponds with different aquaculture model and fish by high-throughput sequencing. Microb. Pathogene. 142, 104101. doi: 10.1016/j.micpath.2020.104101
Louca S., Parfrey L. W., Doebeli M. (2016). Decoupling function and taxonomy in the global ocean microbiome. Science 353 (6305), 1272–1277. 10.1126/science.aaf4507
Lozupone C., Knight R. (2005). UniFrac: a new phylogenetic method for comparing microbial communities. Appl. Environ. Microb. 71 (12), 8228–8235. doi: 10.1128/AEM.71.12.8228-8235.2005
Magoč T., Salzberg S. L. (2011). FLASH: fast length adjustment of short reads to improve genome assemblies. Bioinformatics 27 (21), 2957–2963. doi: 10.1093/bioinformatics/btr507
Martins P., Cleary D. F., Pires A. C., Rodrigues A. M., Quintino V., Calado R., et al. (2013). Molecular analysis of bacterial communities and detection of potential pathogens in a recirculating aquaculture system for Scophthalmus maximus and Solea senegalensis. PloS One 8 (11), e80847. doi: 10.1371/journal.pone.0080847
Mayer R. E., Bofill-Mas S., Egle L., Reischer G. H., Schade M., Fernandez-Cassi X., et al. (2016). Occurrence of human-associated bacteroidetes genetic source tracking markers in raw and treated wastewater of municipal and domestic origin and comparison to standard and alternative indicators of faecal pollution. Water Res. 90, 265–276. doi: 10.1016/j.watres.2015.12.031
Nho S. W., Abdelhamed H., Paul D., Park S., Mauel M. J., Karsi A., et al. (2018). Taxonomic and functional metagenomic profile of sediment from a commercial catfish pond in Mississippi. Front. Microbiol. 9, 2855. doi: 10.3389/fmicb.2018.02855
Nimnoi P., Pongsilp N. (2020). Marine bacterial communities in the upper gulf of Thailand assessed by illumina next-generation sequencing platform. BMC Microbiol. 20 (1), 1–11. 10.1186/s12866-020-1701-6
Nurul A. N. A., Muhammad D. D., Okomoda V. T., Nur A. A. B. (2019). 16S rRNA-based metagenomic analysis of microbial communities associated with wild Labroides dimidiatus from karah island, terengganu, Malaysia. Biotechnol. Rep. 21, e00303. doi: 10.1016/j.btre.2019.e00303
Olafsen J. A. (2001). Interactions between fish larvae and bacteria in marine aquaculture. Aquaculture 200 (1-2), 223–247. doi: 10.1016/s0044-8486(01)00702-5
Olmos J., Ochoa L., Paniagua-Michel J., Contreras R. (2011). Functional feed assessment on Litopenaeus vannamei using 100% fish meal replacement by soybean meal, high levels of complex carbohydrates and Bacillus probiotic strains. Mar. Drugs 9 (6), 1119–1132. doi: 10.3390/md9061119
Olsen A. B., Gulla S., Steinum T., Colquhoun D. J., Nilsen H. K., Duchaud E. (2017). Multilocus sequence analysis reveals extensive genetic variety within Tenacibaculum spp. associated with ulcers in sea-farmed fish in Norway. Vet. Microbiol. 205, 39–45. doi: 10.1016/j.vetmic.2017.04.028
Ouyang L., Chen H., Liu X., Wong M. H., Xu F., Yang X., et al. (2020). Characteristics of spatial and seasonal bacterial community structures in a river under anthropogenic disturbances. Environ. pollut. 264, 114818. doi: 10.1016/j.envpol.2020.114818
Pal M., Kerorsa G. B., Marami L. M., Kandi V. (2020). Epidemiology, pathogenicity, animal infections, antibiotic resistance, public health significance, and economic impact of staphylococcus aureus: a comprehensive review. Am. J. Public Health Res. 8 (1), 14–21. doi: 10.12691/ajphr-8-1-3
Pineiro-Vidal M., Riaza A., Santos Y. (2008). Tenacibaculum discolor sp. nov. and Tenacibaculum gallaicum sp. nov., isolated from sole (Solea senegalensis) and turbot (Psetta maxima) culture systems. Int. J. Syst. Evol. Micr. 58 (1), 21–25. doi: 10.1099/ijs.0.65397-0
Pruesse E., Quast C., Knittel K., Fuchs B. M., Ludwig W., Peplies J., et al. (2007). SILVA: a comprehensive online resource for quality checked and aligned ribosomal RNA sequence data compatible with ARB. Nucleic Acids Res. 35 (21), 7188–7196. doi: 10.1093/nar/gkm864
Shan J., Guoliang W., Qingsong Z., Tianlun Z., Yinger C. (2005). Epidemiology of Vibriosis in large yellow croaker Pseudosciaena crocea (Richardson) in marine cage culture. Fish. Sci. 24 (1), 17–19. doi: 10.16378/j.cnki.1003-1111.2005.01.005
Shen J. Y., Yu X. P., Pan X. Y., Xu W. J., Cao Z. (2008). Isolation and identification of pseudomonassis pathogen from cultured Pseudosciaena crocea. Mar. Fish. Res. 29 (1), 1–6. 10.1007/s11676-008-0012-9
Su Z., Dai T., Tang Y., Tao Y., Huang B., Mu Q., et al. (2018). Sediment bacterial community structures and their predicted functions implied the impacts from natural processes and anthropogenic activities in coastal area. Mar. pollut. Bull. 131, 481–495. doi: 10.1016/j.marpolbul.2018.04.052
Suh S. S., Park M., Hwang J., Kil E. J., Jung S. W., Lee S., et al. (2015). Seasonal dynamics of marine microbial community in the south Sea of Korea. PloS One 10 (6), e0131633. doi: 10.1371/journal.pone.0131633
Táncsics A., Szoboszlay S., Szabó I., Farkas M., Kovács B., Kukolya J., et al. (2012). Quantification of subfamily i. 2. c catechol 2, 3-dioxygenase mRNA transcripts in groundwater samples of an oxygen-limited BTEX-contaminated site. Environ. Sci. Technol. 46 (1), 232–240. doi: 10.1021/es201842h
Toranzo A. E., Magariños B., Romalde J. L. (2005). A review of the main bacterial fish diseases in mariculture systems. Aquaculture 246 (1-4), 37–61. doi: 10.1016/j.aquaculture.2005.01.002
Ul-Hasan S., Bowers R. M., Figueroa-Montiel A., Licea-Navarro A. F., Beman J. M., Woyke T., et al. (2019). Community ecology across bacteria, archaea and microbial eukaryotes in the sediment and seawater of coastal Puerto nuevo, Baja California. PloS One 14 (2), e0212355. doi: 10.1371/journal.pone.0212355
Vadstein O., Attramadal K. J., Bakke I., Forberg T., Olsen Y., Verdegem M., et al. (2018). Managing the microbial community of marine fish larvae: a holistic perspective for larviculture. Front. Microbiol. 9, 1820. doi: 10.3389/fmicb.2018.01820
Wang F., Dong W., Zhao Z., Wang H., Li W., Chen G., et al. (2021). Heavy metal pollution in urban river sediment of different urban functional areas and its influence on microbial community structure. Sci. Total. Environ. 778, 146383. doi: 10.1016/j.scitotenv.2021.146383
Wang Q., Garrity G. M., Tiedje J. M., Cole J. R. (2007). Naive Bayesian classifier for rapid assignment of rRNA sequences into the new bacterial taxonomy. Appl. Environ. Microb. 73 (16), 5261–5267. doi: 10.1128/AEM.00062-07
Wang X., Hu M., Gu H., Zhang L., Shang Y., Wang T., et al. (2020). Short-term exposure to norfloxacin induces oxidative stress, neurotoxicity and microbiota alteration in juvenile large yellow croaker Pseudosciaena crocea. Environ. pollut. 267, 115397. doi: 10.1016/j.envpol.2020.115397
Wang R. X., Li-Wen X. U., Feng J. (2005). A review of the pathogenic bacteria, diagnosis and vaccine of principal bacterial diseases of mariculture fish. South China Fish. Sci. 1 (6), 72–79. doi: 10.3969/j.issn.2095-0780.2005.06.012
Wang Y., Sheng H. F., He Y., Wu J. Y., Jiang Y. X., Tam N. F. Y., et al. (2012). Comparison of the levels of bacterial diversity in freshwater, intertidal wetland, and marine sediments by using millions of illumina tags. Appl. Environ. Microb. 78 (23), 8264–8271. doi: 10.1128/AEM.01821-12
Wang C., Wang Y., Liu P., Sun Y., Song Z., Hu X. (2021). Characteristics of bacterial community structure and function associated with nutrients and heavy metals in coastal aquaculture area. Environ. pollut. 275, 116639. doi: 10.1016/j.envpol.2021.116639
Wang G. L., Yuan S. P., Jin S. (2005). Nocardiosis in large yellow croaker, Larimichthys crocea (Richardson). J. Fish. Dis. 28 (6), 339–345. doi: 10.1111/j.1365-2761.2005.00637.x
Wang S., Zheng X., Xia H., Shi D., Fan J., Wang P., et al. (2019). Archaeal community variation in the qinhuangdao coastal aquaculture zone revealed by high-throughput sequencing. PloS One 14 (6), e0218611. doi: 10.1371/journal.pone.0218611
Wang P., Zhou Q., Feng J., He J., Lou Y., Zhu J. (2019). Effect of dietary fermented soybean meal on growth, intestinal morphology and microbiota in juvenile large yellow croaker, Larimichthys crocea. Aquac. Res. 50 (3), 748–757. doi: 10.1111/are.13929
Ward T., Larson J., Meulemans J., Hillmann B., Lynch J., Sidiropoulos D., et al. (2017). BugBase predicts organism-level microbiome phenotypes. BioRxiv, 133462. doi: 10.1101/133462
Wei N., Wang C., Xiao S., Huang W., Lin M., Yan Q., et al. (2018). Intestinal microbiota in large yellow croaker, Larimichthys crocea, at different ages. J. World Aquacult. Soc 49 (1), 256–267. doi: 10.1111/jwas.12463
Wickham H. (2011). ggplot2. Wiley interdiscip. Rev. Comput. Stat. 3 (2), 180–185. doi: 10.1002/wics.147
Ye Q., Wu Y., Zhu Z., Wang X., Li Z., Zhang J. (2016). Bacterial diversity in the surface sediments of the hypoxic zone near the changjiang estuary and in the East China Sea. Microbiologyopen 5 (2), 323–339. doi: 10.1002/mbo3.330
Yin Z., Liu Q., Liu Y., Gao S., He Y., Yao C., et al. (2021). Early life intervention using probiotic Clostridium butyricum improves intestinal development, immune response, and gut microbiota in large yellow croaker (Larimichthys crocea) larvae. Front. Immunol. 12, 215. doi: 10.3389/fimmu.2021.640767
Yu S. X., Pang Y. L., Wang Y. C., Li J. L., Qin S. (2018). Distribution of bacterial communities along the spatial and environmental gradients from bohai Sea to northern yellow Sea. PeerJ 6, e4272. doi: 10.7717/peerj.4272
Zeng S., Wei D., Hou D., Wang H., Liu J., Weng S., et al. (2021). Sediment microbiota in polyculture of shrimp and fish pattern is distinctive from those in monoculture intensive shrimp or fish ponds. Sci. Total. Environ. 787, 147594. doi: 10.1016/j.scitotenv.2021.147594
Zhang J., Chen M., Huang J., Guo X., Zhang Y., Liu D., et al. (2019). Diversity of the microbial community and cultivable protease-producing bacteria in the sediments of the bohai sea, yellow sea and south China Sea. PloS One 14 (4), e0215328. doi: 10.1371/journal.pone.0215328
Zhang Z., Deng Q., Wan L., Cao X., Zhou Y., Song C. (2021). Bacterial communities and enzymatic activities in sediments of long-term fish and crab aquaculture ponds. Microorganisms 9 (3), 501. doi: 10.3390/microorganisms9030501
Zhang H., Sun Z., Liu B., Xuan Y., Jiang M., Pan Y., et al. (2016). Dynamic changes of microbial communities in Litopenaeus vannamei cultures and the effects of environmental factors. Aquaculture 455, 97–108. doi: 10.1016/j.aquaculture.2016.01.011
Zhang C., Zheng X., Ren X., Li Y., Wang Y. (2019). Bacterial diversity in gut of large yellow croaker Larimichthys crocea and black sea bream sparus macrocephalus reared in an inshore net pen. Fish. Sci. 85 (6), 1027–1036. doi: 10.1007/s12562-019-01349-5
Zhang J. T., Zhou S. M., An S. W., Chen L., Wang G. L. (2014). Visceral granulomas in farmed large yellow croaker, Larimichthys crocea (Richardson), caused by a bacterial pathogen, Pseudomonas plecoglossicida. J. Fish. Dis. 37 (2), 113–121. doi: 10.1111/jfd.12075
Zhao Z., Jiang J., Pan Y., Dong Y., Chen Z., Zhang G., et al. (2020). Temporal dynamics of bacterial communities in the water and sediments of sea cucumber (Apostichopus japonicus) culture ponds. Aquaculture 528, 735498. doi: 10.1016/j.aquaculture.2020.735498
Keywords: Bacterial communities, high-throughput sequencing, marine cage-culture large yellow croaker, sediment, seawater, pathogen.
Citation: Li T, Guo D, Shen Y, Bao J and Jin L (2022) Comparative analysis of bacterial communities in the sediment and seawater environments from marine large yellow croaker cages (Zhejiang coast, China). Front. Mar. Sci. 9:963242. doi: 10.3389/fmars.2022.963242
Received: 07 June 2022; Accepted: 01 August 2022;
Published: 16 August 2022.
Edited by:
Jin Zhou, Tsinghua University, ChinaReviewed by:
Zhaofang Han, Xiamen University, ChinaSi Tang, Tsinghua University, China
Ming Fang, Jimei University, China
Copyright © 2022 Li, Guo, Shen, Bao and Jin. This is an open-access article distributed under the terms of the Creative Commons Attribution License (CC BY). The use, distribution or reproduction in other forums is permitted, provided the original author(s) and the copyright owner(s) are credited and that the original publication in this journal is cited, in accordance with accepted academic practice. No use, distribution or reproduction is permitted which does not comply with these terms.
*Correspondence: Lei Jin, amlubGVpMjM4OEAxMjYuY29t
†These authors have contributed equally to this work