- 1National Research Council of Italy, Institute for the Study of Anthropic Impacts and Sustainability in the Marine Environment, (CNR-IAS), Palermo, Italy
- 2National Research Council of Italy, Institute for Studies on the Mediterranean, (CNR-ISMed), Palermo, Italy
Interannual fluctuations in the structure and the composition of ichthyoplankton assemblages in the pelagic waters of the Strait of Sicily (SoS, Central Mediterranean) were investigated, trying to relate them to the observed variability in oceanographic conditions. Plankton data used in this study were from 16 summer surveys carried out in the SoS every year from 2001 to 2016, using oblique bongo plankton net (0–100 m) tows. Out of more than 12,000 fish larvae collected in the sampling stations included in the analysis, 9,519 of them were identified and regularly classified in 15 orders and 49 families. Ichthyoplankton assemblages, defined at the family level due to the uniform availability of this information along the time series, showed a decreasing trend over time in total larval abundance, along with taxonomic (family) richness and Shannon index (α diversity), more pronounced in the shelf area and in the slope area, respectively. Conversely, the relatively high levels of yearly compositional changes observed in the larval assemblage from both shelf and slope areas, as estimated by the Jaccard dissimilarity index (β diversity), did not show any significant linear trend. In addition, a biodiversity hotspot (both in terms of family richness and Shannon index) was evidenced in the frontal structure characterizing the southeastern part of the study area. Generalized additive models were used to evaluate the effect of oceanographic conditions on the temporal and spatial patterns of ichthyoplankton biodiversity. Results evidenced the role of salinity, surface temperature, and surface currents in modulating biodiversity indices, especially in the shelf area. Finally, the relevance of local frontal oceanographic structures in sustaining high biodiversity levels is postulated.
Introduction
Biodiversity is generally considered one of the key factors of ecosystem resilience in response to anthropogenic and natural pressures. Its positive impact on ecosystem functions and services, including fisheries production, is well established (Loreau et al., 2001; Cardinale et al., 2002; Bakun, 2006; Worm et al., 2006; Duffy, 2009; Naeem et al., 2009; Cardinale et al., 2012; Hooper et al., 2012; Tilman et al., 2012; Tilman et al., 2014), also in contrast to regime shifts (Gamfeldt et al., 2014; Ponti et al., 2014; Rocha et al., 2015).
The biodiversity in marine ecosystems is the result of the complex interconnection among all co-occurring organisms at different trophic levels, and the loss of just one ecological level may have severe effects on the functioning of the entire ecosystem community. Within this complex set of interspecific relationships, fish assemblage structure can be affected by several natural and anthropogenic processes, such as overfishing, pollution, habitat loss, and climate change (Jennings and Kaiser, 1998; Gray et al., 2005; Poulard and Blanchard, 2005; Lehodey et al., 2006; Rijnsdorp et al., 2009; Rochet et al., 2010). Therefore, a deeper understanding of the functioning of ecosystem-level processes is necessary to mitigate potential unsustainable exploitation and biodiversity losses and to support management approaches (Pikitch et al., 2004; Mittermeier et al., 2011).
In this framework, biodiversity studies based on fish early life stages can also provide information on spawning grounds and nursery areas, with implications on stock biomass fluctuations induced by recruitment variability (Houde, 2002; Ospina-Álvarez et al., 2013; Ospina-Álvarez et al., 2015; Torri et al., 2018; Patti et al., 2020). In particular, as survival and growth processes of many fish species are controlled by the impact of the marine environment on early life stages, further research on the temporal and spatial trends in the distribution and abundance of fish larvae in relation to changes in abiotic factors is highly relevant (Nonaka et al., 2000). In addition, changes in the structure and in the distribution of larval fish assemblages may be the effect of oceanographic factors, which, in turn, are also often characterized by high dynamic conditions in space and time (Moyano et al., 2009; Vilchis et al., 2009; Hernandez et al., 2010; Moyano and Hernández-León, 2011; Katsuragawa et al., 2014; Moyano et al., 2014).
In the Mediterranean Sea, previous studies on larval fish assemblages are quite limited for many taxa and are generally based on relatively short time series (Sabatés, 1990; Cuttitta et al., 2004; Isari et al., 2008; López-Sanz et al., 2009; Álvarez et al., 2012; Zarrad et al., 2013; Álvarez et al., 2015; Cuttitta et al., 2016a; Cuttitta et al., 2016b; Cuttitta et al., 2018; Malavolti et al., 2018; Zarrad et al., 2020; Zorica et al., 2020).
In particular, as far as concerns the study area of the present paper [i.e., the Strait of Sicily (SoS)], previous investigations were limited to the analysis of the structure of larval fish assemblages in relation to local physical forcings (Cuttitta et al., 2016a; Cuttitta et al., 2016b; Cuttitta et al., 2018). However, in the same area, no studies on the changes in larval biodiversity, both over time and/or among sub-regions, are still available. To our knowledge, the present study does represent the first attempt of evaluating trends in biodiversity of summer fish larval assemblages in the SoS, also using the longest reported time series (16 consecutive years) for the Mediterranean Sea.
As a model system, the SoS is a relatively small area that can be considered an optimal natural laboratory for evaluating trends in the biodiversity and structure of larval fish assemblages. This region is generally considered oligotrophic; however, the southern coast of Sicily is an upwelling area with relatively high productivity levels (Piccioni et al., 1988; Patti et al., 2010). In addition, local surface circulation and oceanographic features such as gyres and fronts (Robinson et al., 1991; Lermusiaux, 1999; Lermusiaux and Robinson, 2001; Bonanno et al., 2014; Placenti et al., 2022) were demonstrated to have an important role in concentration and retention processes for early stages of many taxa, including important pelagic fish species (García Lafuente et al., 2002; Falcini et al., 2015; Cuttitta et al., 2016a; Cuttitta et al., 2018; Torri et al., 2018; Falcini et al., 2020; Patti et al., 2020; Russo et al., 2021; Torri et al., 2021). Actually, in the SoS, the surface circulation is dominated by the motion of Atlantic waters, which is known as AIS (Atlantic Ionian Stream; Robinson et al., 1999) off the southern coast of Sicily. This along-channel meandering surface current transports fish larvae downstream (Patti et al., 2018; Falcini et al., 2020), and, in meeting the Ionian Sea water masses, originates a frontal structure where planktonic organisms are eventually concentrated and retained (García Lafuente et al., 2002). The path of AIS and its year-to-year variability may have consequences for the other predominant hydrological phenomena occurring in the region, such as the extension of upwelling, the formation of frontal features, and the strength in retention processes. In particular, the latter has been demonstrated to be the main mechanism able to modulate the interannual changes in stock biomass of local anchovy (Engraulis encrasicolus; Linnaeus, 1758) population (Patti et al., 2020).
In this context, although, with the aims of data analysis, the available yearly ichthyoplankton information was assembled at the family level only, the present paper intended to investigate, for the first time for the SoS, on the interannual changes in the size and the structure (α diversity) and in composition (β diversity) of larval fish assemblages, in a study area where plankton surveys were carried out during the summer season on a regular yearly basis over the period 2001–2016. In addition, the role of spatial oceanographic patterns in modulating larval biodiversity is also explored. In providing the analysis of the longest available time series of larval fish assemblages in the Central Mediterranean, this study is meant to pave the way to the evaluation of the relative importance of oceanographic conditions on the summer biodiversity patterns of planktonic fish stages in the SoS, with special emphasis on the role that surface current strength and local mesoscale features may have in supporting biodiversity hotspots.
Material and methods
Ichthyoplankton data
The study area is located in the SoS, off the southern Sicilian coast (Figure 1), covering a surface of about 25,000 km2.
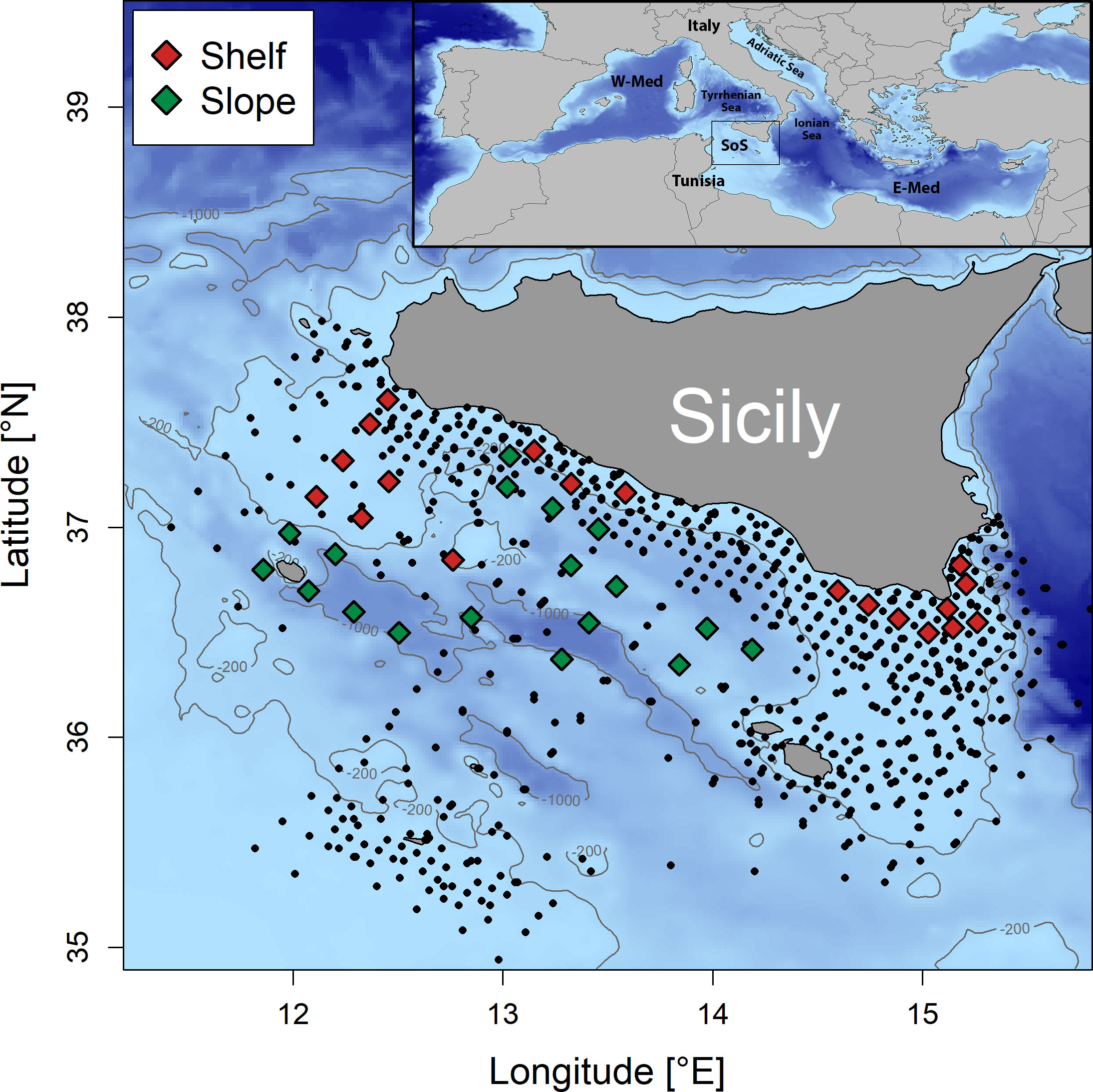
Figure 1 Map of locations of the sampling stations. Data collected from sites marked with squares, available for each one of 16 summer surveys carried out over the period 2001–2016, were used in the present study. Different colors refer to the bottom depth, red for stations with bottom depth ≤ 200 m (“Shelf”) and green for stations with bottom depth > 200 m (“Slope”). The sites of all stations sampled in the summer surveys 2001–2016 and listed in Table 1 are also displayed with black circles for the sake of comparison (see Figures S1, S2 and related discussion).
Oceanographic data and ichthyoplanktonic samples were collected in 16 annual oceanographic surveys carried out during the summer period on board of the R/V “Urania” (2001–2014) and R/V “Minerva Uno” (2015–2016). Table 1 shows the list of surveys, including information on the survey periods and on the total number of sampled stations by year (range: 119–229). Average duration time of the surveys is about 18 days, and the central day of the surveys is 14 July.
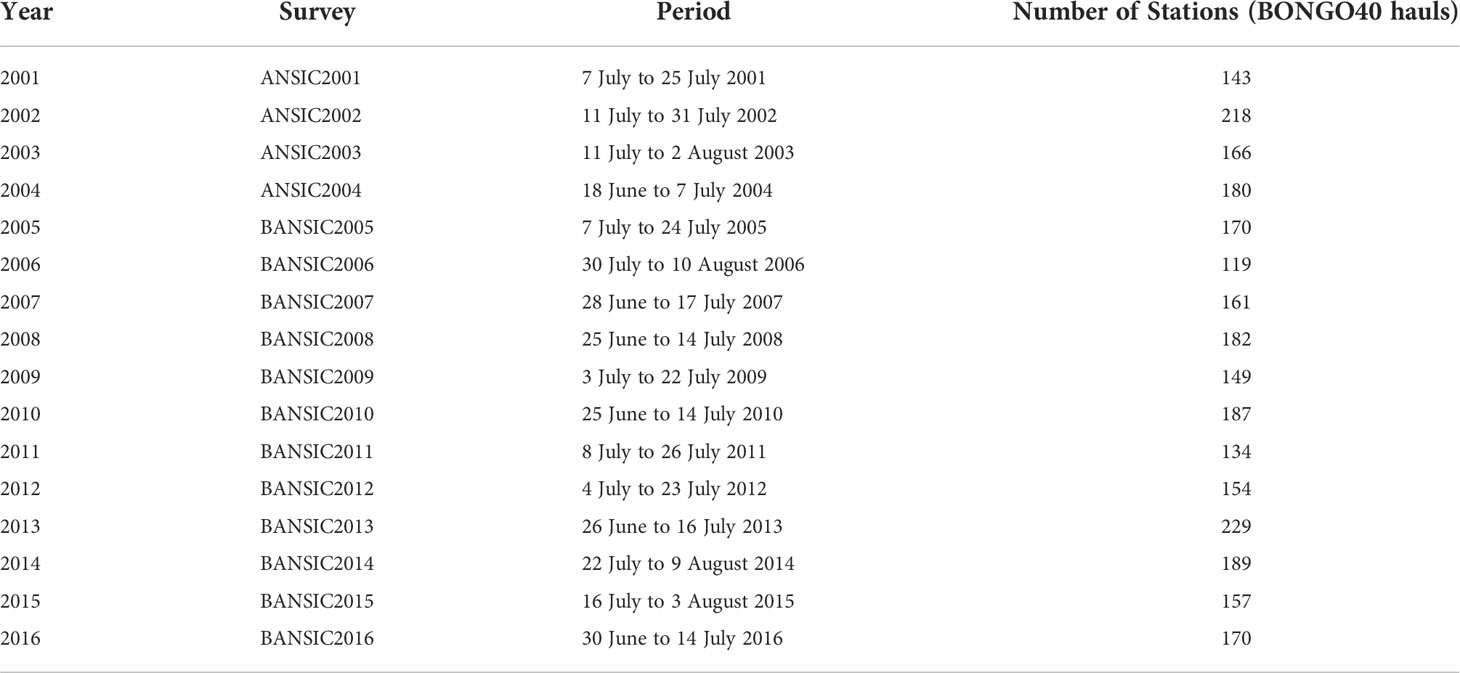
Table 1 List of ichthyoplankton summer surveys carried out in the study area during the period 2001–2016.
The sampling was based on a systematic station grid of 6 × 6 nautical miles on the continental shelf (bottom depth < 200 m) and on a grid of 12 × 12 nautical miles for the offshore areas with bottom depth greater than 200 m. However, to the sake of the present study, the analyses used only data from 37 sampling sites that were sampled in each one of the annual summer surveys that were regularly carried out over the period 2001–2016, 19 of them located on the continental shelf of the study area (“shelf” sub-region) and 18 over bottom depths greater than 200 m (“slope” sub-region) (Figure 1). In total, data from n. 592 sampling hauls (i.e., 37 sites multiplied by 16 years) were used for this study (about 22% of the stations listed in Table 1). This choice was driven by the need of ensuring comparability across years, to get unbiased estimates of diversity indices, not affected by the variable sampling effort over time both in terms of number of stations and extension of covered area.
Plankton samples were collected using a bongo net (Bongo40, with 40-cm opening) towed obliquely from the surface to 100-m depth or to 5 m from the bottom in shallower stations and equipped with a 200-μm mesh size net. Stations were sampled 24 h a day, to minimize bias in the catch of fish species that, during the larval stage, typically may show relatively large diel vertical migration patterns (Olivar et al., 2001; Sabatés et al., 2008; Olivar et al., 2014). All the samples used were from the same side and cod-end collector of the Bongo. Samples were immediately fixed after collection and preserved in a 10% buffered-formaldehyde (and/or 70% alcohol) and sea-water solution for further sorting in laboratory by stereomicroscopy.
Fish larvae were sorted from the rest of the plankton and identified, as far as possible, to the species taxonomic level. Taxonomic identification was based on Bertolini et al. (1956); Moser and Ahlstrom (1996); Costa (1999), and Tortonese (1970). However, because of heterogeneity in the capabilities of resolving the species (and/or genus) taxonomic level in sorted larvae across years (2001–2016), with the aims of the present paper, detected taxa were grouped at the family level (for a total of 49 different families identified in the selected sampling stations).
For the evaluation of rank abundance, raw counts of larval specimens were firstly standardized to numbers per square meter. Mean larval densities estimates were derived for each family and by year from the counts based on estimates of the volume of seawater filtered by mechanical flowmeters (General Oceanics Inc., FL, USA) and the tow depth for each sample.
Oceanographic data
A set of environmental parameters was selected and used for investigating on their influence on the temporal and spatial changes in biodiversity of local ichthyoplankton assemblages.
Continuous vertical profiles of temperature data were acquired in all Bongo40 plankton stations to characterize the physical properties of the water column. The collected downcast data were obtained by a multiparametric SBE 11 plus CTD probe and were quality-checked and processed according to the Mediterranean and Ocean Data Base instructions (Brankart, 1994), using the Seasoft-Win32 software. From the set of available environmental information collected in CTD casts, the parameters used in this study were bottom depth, temperature, and salinity.
As most of the larvae in the Mediterranean Sea during the summer are mainly concentrated in the mixed layer (Palomera, 1991; Mazzola et al., 2000), in this study, the 10-m depth was used as reference for temperature and salinity conditions in the selected stations. Specifically, the average values of the available measurements from the first 10 m of the water column were used, which can also be considered as representative of the surface conditions, as during the summer, the mixed layer depth (MLD; in m) is typically deeper than 10 m. The MLD (in m) itself is an additional parameter considered in the data analysis, and its value has been derived from each CTD profile using the algorithm described in Kara et al. (2000), as it is known to be an important driver for the definition of the potential spawning habitat (Planque et al., 2007).
The impact of mesoscale oceanographic features such as upwelling, cold filaments, and fronts on the ichthyoplankton spatial distribution (Torri et al., 2018; Patti et al., 2020) was also taken into account. Actually, oceanographic structures can influence the distribution of chemical and physical properties of the water column with a potential effect on larval survival and development (Cuttitta et al., 2004; Cuttitta et al., 2016a; Cuttitta et al., 2016b; Cuttitta et al., 2018). Therefore, the surface circulation features were evaluated by using data about the absolute dynamic topography (ADT; in cm) (daily data; spatial resolution: 0.125 × 0.125 degrees) and the derived u and v components of geostrophic currents, produced by Copernicus Marine Environment Monitoring Service (CMEMS; http://marine.copernicus.eu/). ADT data are representative of important oceanographic features such as mesoscale eddies and meanders (Pujol and Larnicol, 2005), which are able to affect primary production and can act as physical barriers for the larval distribution or be responsible for their dispersal offshore. In addition, the absolute geostrophic current speed (GCS; in cm s−1) was derived from the zonal (u) and meridional (v) components of surface current and used as an additional potential predictor in the subsequent modeling approach.
Finally, information about the sea surface chlorophyll-a concentration (Chl-a; in mg m−3) was also applied, as Chl-a is a good proxy of primary productivity (Joint and Groom, 2000) and indirectly can be helpful in depicting the presence of favorable feeding conditions for larvae. High-resolution (1 × 1 km) satellite daily data available for download from CMEMS were used for this purpose.
For all the satellite-based information, the available data were extracted for each plankton station included in the analysis, based on the spatial and temporal position of the associated sampling hauls.
Data analysis
The analysis of temporal and spatial changes in biodiversity of larval fish assemblages can be addressed using measures of α diversity, which evaluates the change over time in the size and in the structure, and of β diversity, which aims at assessing the compositional change within the assemblage (Whittaker, 1960; Whittaker, 1972).
For measuring changes in α diversity over the 16 year long period considered in this study, we firstly applied Family richness (Fr), by evaluating the number of taxonomic groups at the family level by station. In addition, for each sampling station, the Shannon diversity index (H’, at the family level) was calculated. When needed, the two indices were averaged by year and by sub-area (“shelf” and “slope” sub-regions) and were also used to evaluate potential spatial patterns in the study area in relation to the environmental conditions. Calculations were done using the function diversity in the R package vegan (Oksanen et al., 2020; R Core Team, 2022).
Compositional shifts in the assemblages (β diversity) were evaluated by the Jaccard pair-wise dissimilarity index (Jaccard, 1908; Magurran, 2004; Magurran et al., 2019), based on presence/absence (0/1) data at station level. In particular, temporal trends were evaluated using plots in which the first year of the time series (2001) was compared with each one of the successive years (Dornelas et al., 2014). Spatial patterns were also investigated as well, calculating the index by sub-area (“shelf” and “slope”).
The significance of linear trends in yearly larval standardized abundance and family richness was tested by applying a Bonferroni adjustment, to account for multiple testing and decreasing chances for a type I error (Miller, 1981).
Generalized additive models (GAMs; Hastie and Tibshirani, 1990) were used to examine the influence of environmental conditions on α and β diversity over time.
GAM is a semi-parametric extension of GLM, where the only underlying assumption made is that the functions are additive and the linear predictor is the sum of smoothing functions. This makes GAM very flexible, and they can fit very complex functions. Dependent variables were Family richness (Fr) and Shannon index (H’) for α diversity and Jaccard index for β diversity. Family richness has been modeled considering Poisson as discrete probability distribution and “log” as the link function between the stochastic and systematic parts of the model, whereas Gaussian distribution and the “identity” link have been implemented for Shannon and Jaccard indices. Explanatory variables used in GAMs were bottom depth, temperature, and salinity (at 10 m), surface Chl-a, MLD, ADT, GCS, and year. The potential non-linear relationships between covariates and the dependent variables were investigated by cubic regression splines. In addition, because one of the objectives of the analysis was to evaluate the role of the frontal feature characterizing the Eastern part of the study area as a retention mesoscale structure potentially able to favor biodiversity hotspots, latitude and longitude of sampling stations were also included among the independent variables.
Model estimations were based on a backward stepwise selection, starting from the entire set of explanatory variables that were chosen for the present study.
GAMs were implemented using the R package “mgcv” (Wood, 2011).
Results
In the selected group of 592 sampling hauls (37 sampling sites × 16 annual surveys) considered in this study, out of a total of 12,713 larvae sorted from the samples, 9,519 of these were identified (classified in 15 orders, 49 families, 64 genera, and 68 species), distributed in 6,046 larvae from stations located in the shelf area and 3,473 larvae from stations in the slope area. Four main ecological groups were singled out, i.e., meso-bathypelagic (M/B), demersal (D), pelagic/epipelagic (P/EP), and small pelagic (SP). The latter was the most abundant one, with 3,386 identified fish larvae from just two species (European anchovy, Engraulis encrasicolus, and Round sardinella, Sardinella aurita), which jointly represented more than 35% of the total number of identified larvae in the whole study area and which were mainly found in the continental shelf stations (about 75% of their total abundance was concentrated in that area for both the two species). There were also 2,916 demersal fish larvae (shelf, 2,127; slope, 789) grouped into 33 demersal families with 34 genera and 443 pelagic/epipelagic fish larvae (shelf, 304; slope, 139) grouped into five families with seven genera. Meso-bathypelagic fish larvae were 2,707 in number (shelf, 1,038; slope, 1,669) and grouped into nine families with 18 genera. They jointly represented about 29.1% of total abundance (49.0% in the slope area).
Larval density by unit surface (10 m2) and relative abundance aggregated at the family level and distributed by sub-regions (“shelf” and “slope”) are given in Table 2.
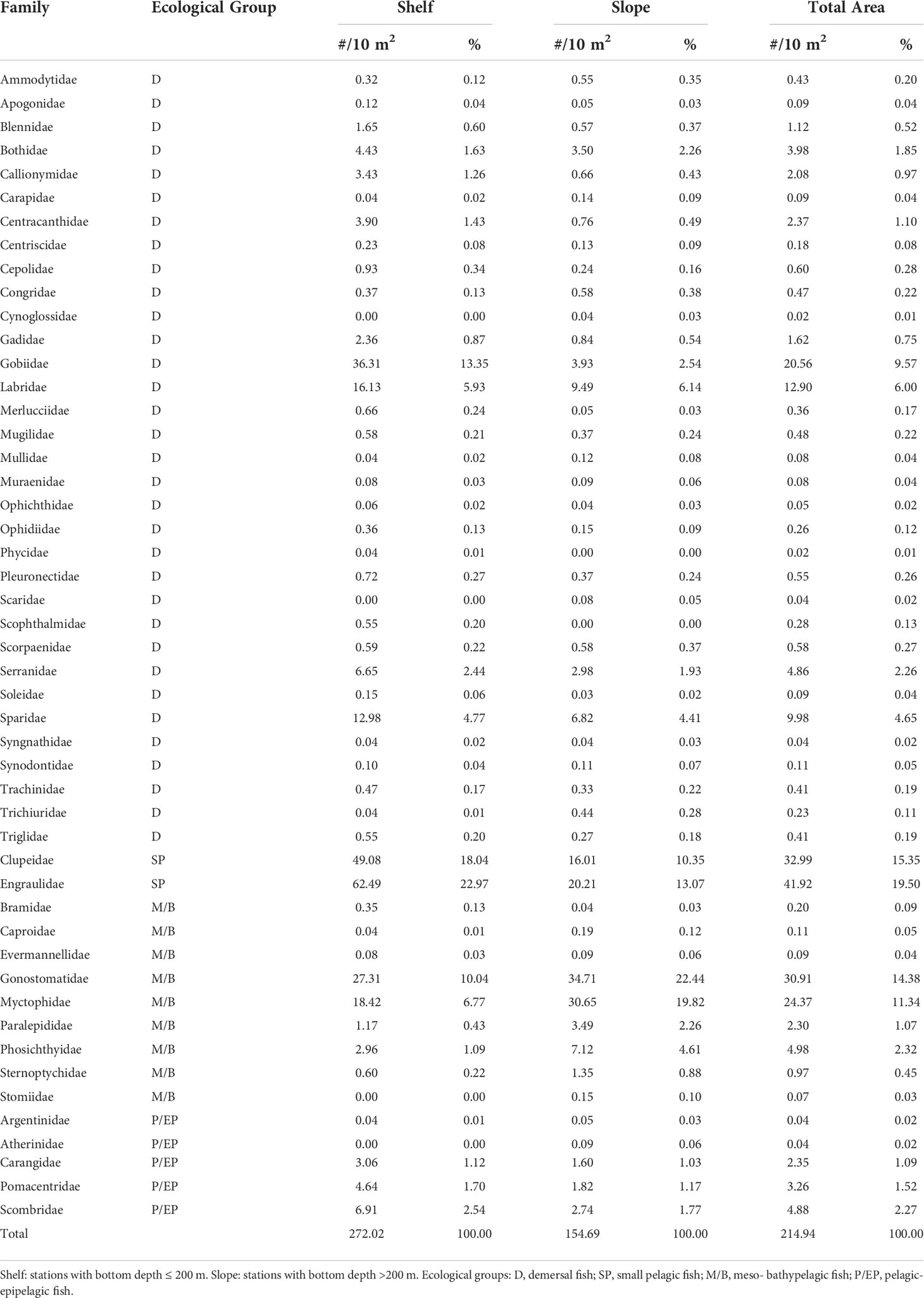
Table 2 Average density (#/m2) and relative abundance (%, percentage) of larval fish families identified in the 37 selected stations over the summer surveys 2001–2016, by sub-areas.
The total number of fish larvae sampled in the 37 stations included in this study across the 16 summer surveys over the period 2001–2016 fluctuated from a maximum of 860 in 2004 to a minimum of 382 in 2015, with a slight but significant (p < 0.05) decreasing trend that is due to the shelf sub-region, characterized by generally higher abundances compared to the slope sub-region (not shown). The same patterns are evident in Figure 2A, where yearly average larval density standardized by unit surface is shown instead. Again, the overall significant (p < 0.05) decreasing pattern is due to shelf stations, as larval density in slope stations does not indicate any significant trend in time.
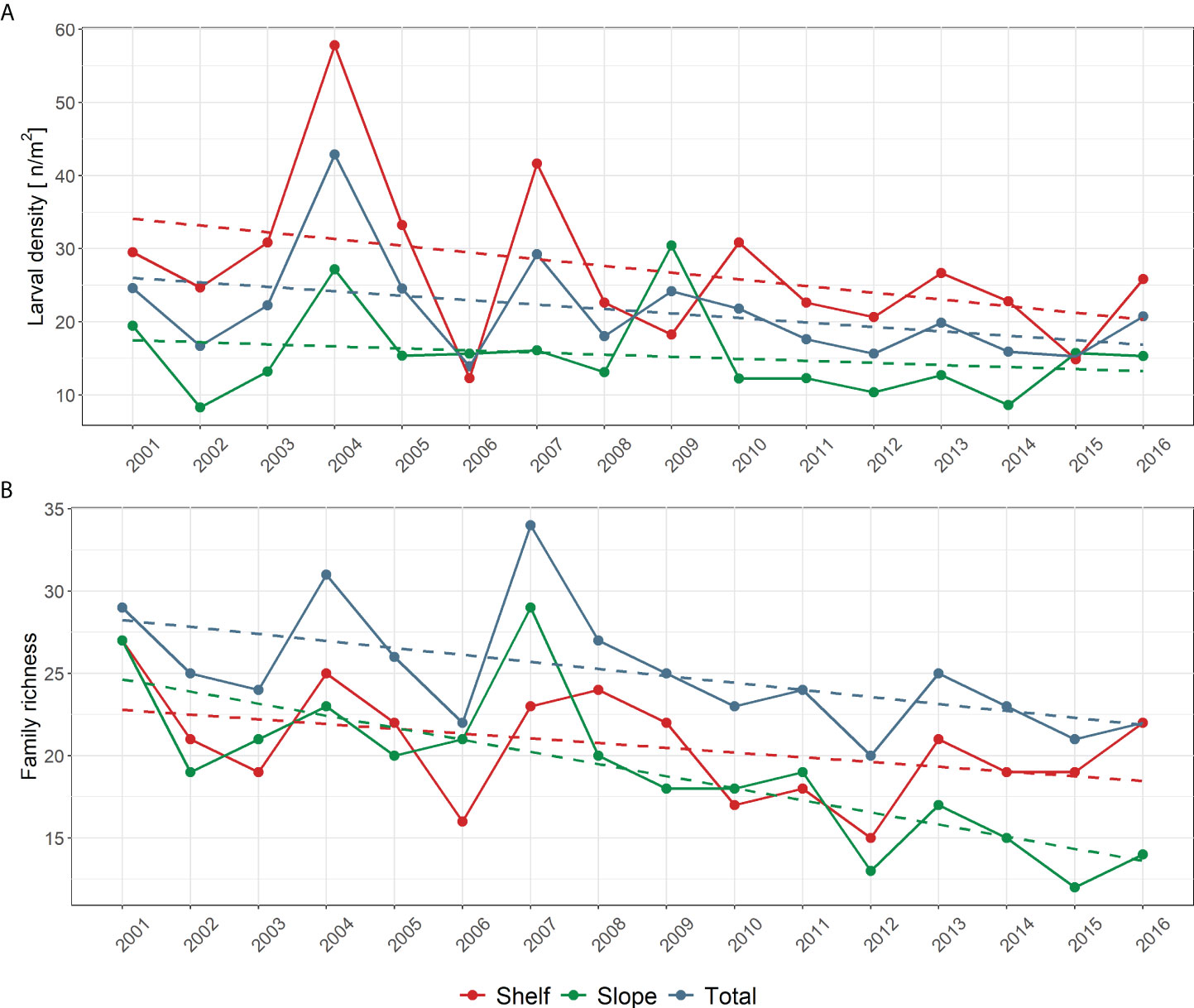
Figure 2 (A) Average total larval surface density (# m−2) and (B) trends in Family richness (Fr) in summer surveys (from 2001 to 2016). The general trend in the entire study area (37 selected stations) is shown, as well the trends in the continental shelf area (19 stations) and in the slope area (18 stations) (see Figure 1).
Figure 2B shows the trend in Family richness (Fr) over the considered period in the 37 selected stations. The total number of families exhibits a significant decreasing trend (p < 0.01), from about 28 families at the early 2000 to 22 families at mid 2010. However, the overall decreasing trend is mainly due to stations located at bottom depths greater than 200 m (“slope” sub-area; p < 0.001). It is worth noting that, when using information from all the sampling stations listed in Table 1, family richness exhibited a very similar decreasing trend (p < 0.05) (Figure S1, Supplementary Material). The same applies to larval density (Figure S2, Supplementary Material). In general, the results of the comparisons show that the trends in biodiversity observed in the 37 selected stations are representative of the patterns characterizing all the sampling stations listed in Table 1.
The average number of families in the 37 selected stations over the period 2001–2016 is Fr = 25.1 (Fr = 20.6 in the 19 stations of shelf area; Fr = 19.1 in the 18 stations of slope area). Out of the total number of 49 identified families, just 11 taxa were always present in the time series, with 22 taxa found in at least half of the years. The taxa accumulation curve over the whole study area shows the asymptotic value (Fr = 49 families) after the first 10 years of the time series (Figure S3).
Temporal trends of Shannon index (H’) and Family richness (Fr) are very similar. Namely, H’ is significantly decreasing (p < 0.05) over the considered periods (2001–2016), and as already observed for Fr, the overall pattern based on all the selected stations is mainly driven by stations in the slope area (Figure S4).
The spatial pattern of average total larval density (sum of specimens from all the identified families by unit surface) and of average Fr by sampling station over the entire period 2001–2016 are shown in Figure 3. Higher concentrations of larval stages are found in the southeastern part of the study area (Figure 3, left panel). The same region appears to represent a biodiversity hotspot (Figure 3, right panel).
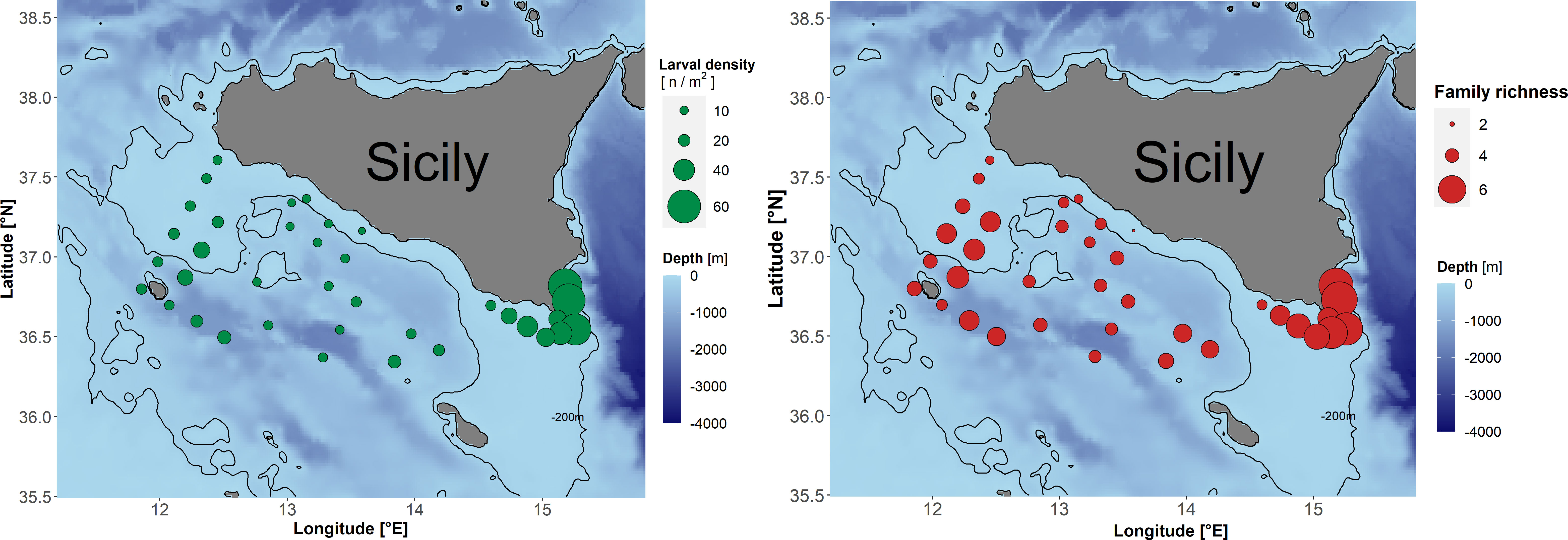
Figure 3 Left panel: Average larval density (# m−2). Right panel: Average Family richness (Fr) by sampling station over the period 2001–2016.
Finally, the average yearly Jaccard dissimilarity is shown in Figure S5. The rate of compositional changes (sensu; Magurran et al., 2019) appears to be quite of high throughout for the whole investigated period in both shelf and slope areas, but it does not indicate any significant linear trend through time, even when information is separated by sub-area (Figure S5). However, a marked decreasing drift is apparent in the last year of the time series (2016).
Rank abundances (and proportions), reported in Figure S6, evidenced that larval densities in shelf area are about two times higher than in the slope region and are dominated by Engraulidae (one species, European anchovy), Clupeidae (one species, Round sardinella), and Gobiidae, representing more than 50% of collected larvae. Engraulidae and Clupeidae are quite abundant even in the slope area (jointly accounting for about 20% of that sub-region), where the leading families are Gonostomatidae (mostly represented by just one identified species, Cyclothone braueri) and Myctophidae (assembling 15 species), together accounting for more than 40% of larval abundances.
Polar rank plots of Figure S7 show for the shelf area an alternation over the length of time series in the dominance of the two most abundant species in the larval fish assemblages, i.e., European anchovy (Engraulidae) and Round sardinella (Clupeidae). Conversely, in the slope area, the abundances of the two above small pelagic species appear to be mostly synchronous and are generally lower compared to the shelf area, with greater interannual fluctuations. The most abundant family in the slope area is Gonostomatidae, followed by Myctophidae. However, the occurrence of the latter two families is quite high in the shelf area as well, although with a lower stability in rank abundance. In addition, comparing Figure 2A with Figure S7, it is worth noting how the peaks in total larval density of the slope area detected in years 2001, 2004, 2009, and 2015-2016, as well as its “lows” in 2002, 2008, 2010, and 2014, are also linked to the impact of neritic families (i.e., Clupeidae, Engraulidae, and Gobiidae).
GAM output
Results from GAM runs on the whole dataset (shelf + slope subareas) and by sub-area are presented in Figures 4, 5 for the two indices of α diversity.
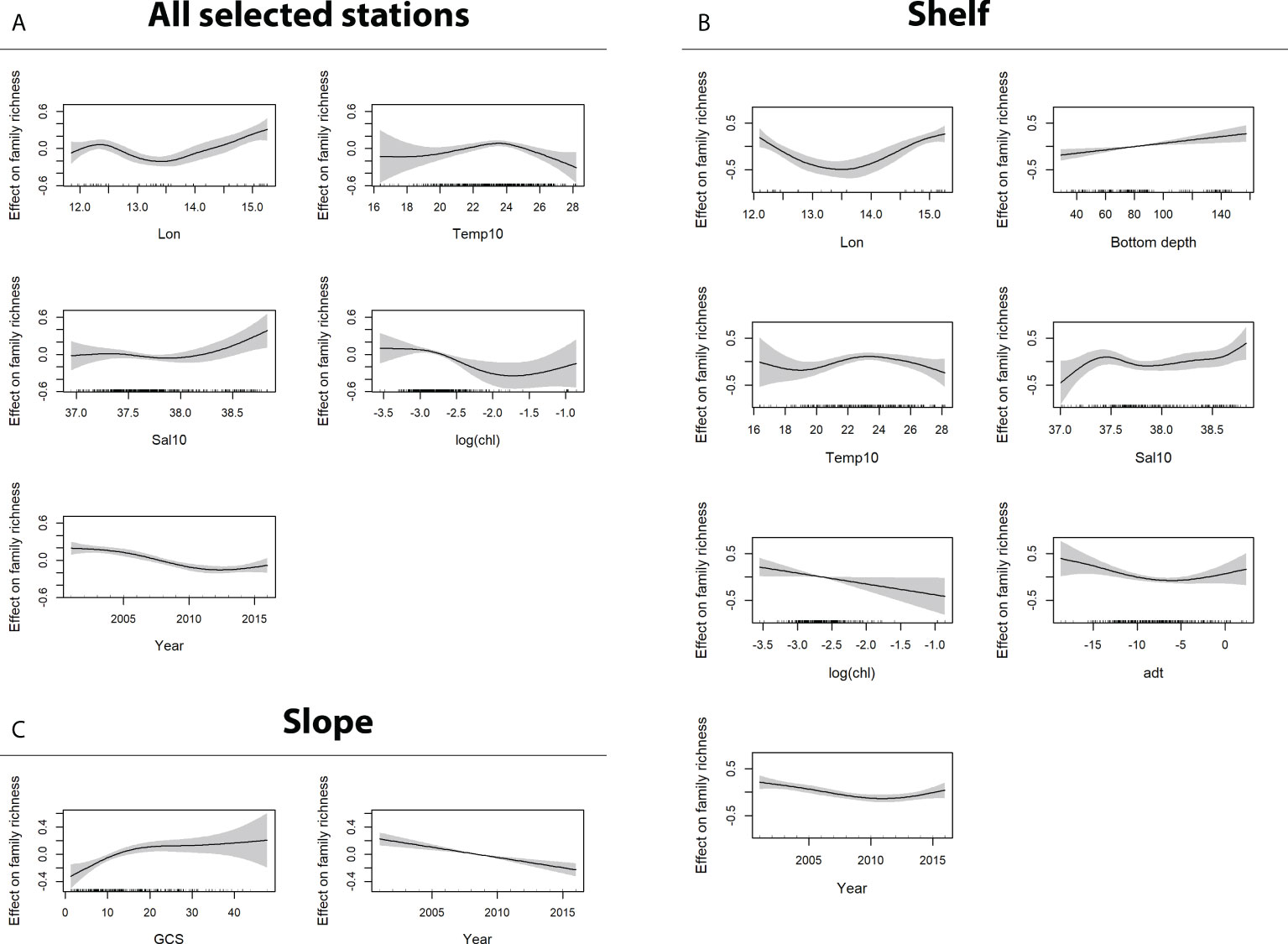
Figure 4 GAM output for the dependent variable Fr (Family richness). Sub-panels refer to model output obtained using (A) all data (shelf + slope), (B) shelf data only (bottom depth ≤ 200 m), and (C) slope data only (bottom depth > 200 m). Only significant factors are shown.
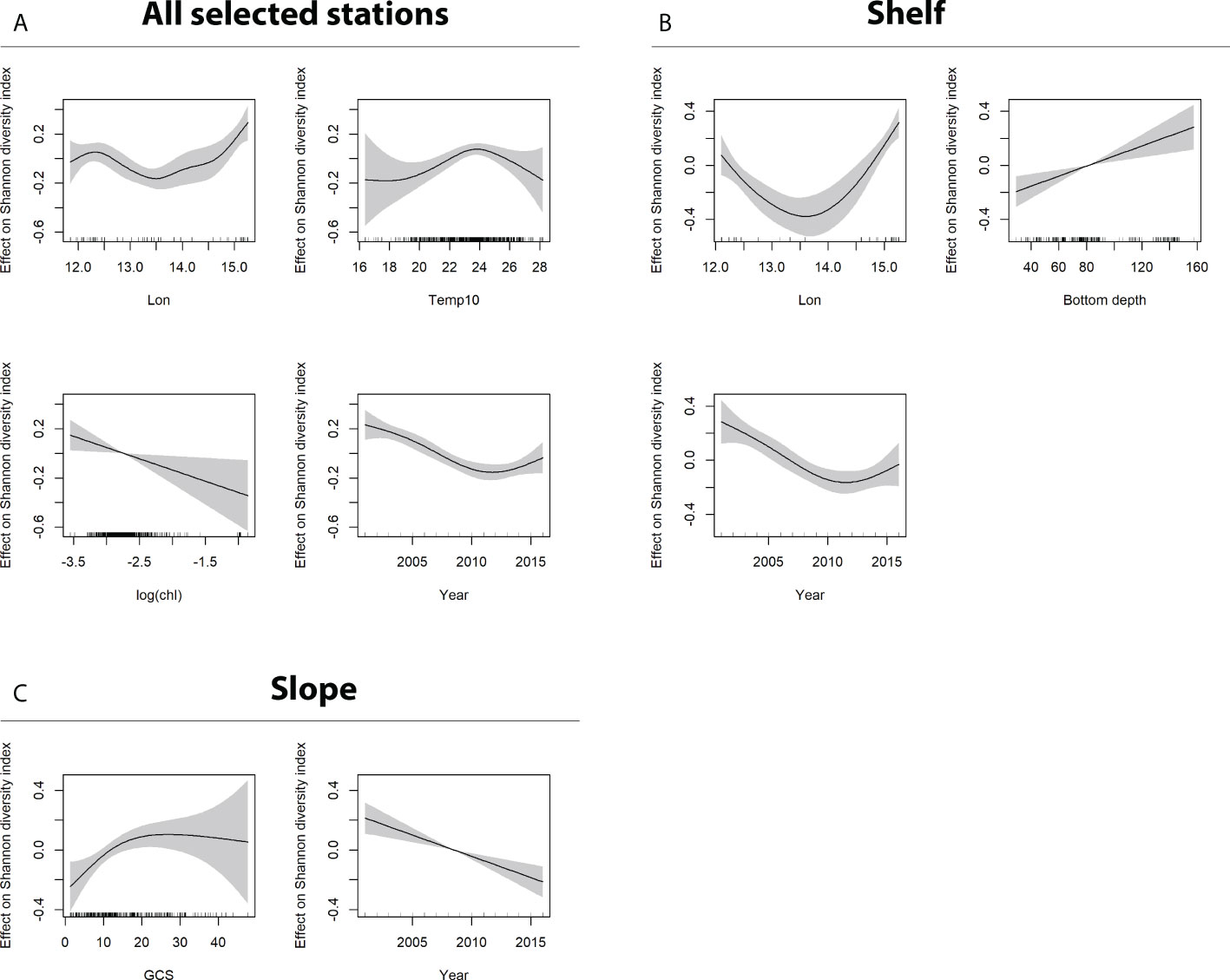
Figure 5 GAM output for the dependent variable H’ (Shannon diversity index). Sub-panels refer to model output obtained using (A) all data (shelf + slope), (B) shelf data only (bottom depth ≤ 200 m), and (C) slope data only (bottom depth > 200 m). Only significant factors are shown.
Specifically, the output of GAMs for the dependent variables Fr (Figure 4A) and H’ (Figure 5A) applied on all selected stations were almost coincident. The analysis of both metrics agreed in confirming the already observed general decreasing trend in biodiversity over time, at least up to 2012 (see also Figures S1, S4) and the west–east increasing diversity gradient already evidenced by Figure 3 (positive effect of greater than average values of longitude), and in showing an optimal temperature value at about 23°C–24°C and a negative effect of relatively large Chl-a values (concentration greater than 0.1 mg m−3). The only difference is the significant positive impact on family richness of “greater than average” salinity values, whereas salinity was not significant in the best model estimated for Shannon diversity index.
More detailed information was obtained by applying the same models to the shelf and slope sub-regions separately.
In the shelf area, the decreasing diversity over time and the effect of longitude are confirmed for both Fr (Figure 4B) and H’ (Figure 5B), and, in addition, the positive impact on biodiversity at increasing bottom depth is evidenced. The GAM model for Fr also indicated a positive response at relatively lower ADT values, which locally are generally associated to coastal upwelling conditions.
In the slope area (bottom depth >200m), the decreasing diversity over time is even more pronounced and the only additional significant factor is GCS, for both Fr (Figure 4C) and H’ (Figure 5C). Specifically, a negative impact on family richness for low current speed (values < 10 m s−1) is evident, as well a positive effect on Shannon index for current speed higher than 14.5 m s−1.
Finally, the GAM outputs for the dependent variable “Jaccard dissimilarity” are shown in Figure 6. Specifically, plots in Figure 6A refer to all available data (shelf + slope), Figure 6B to shelf data only (bottom depth ≤ 200 m), and Figure 6C to slope data (bottom depth > 200 m).
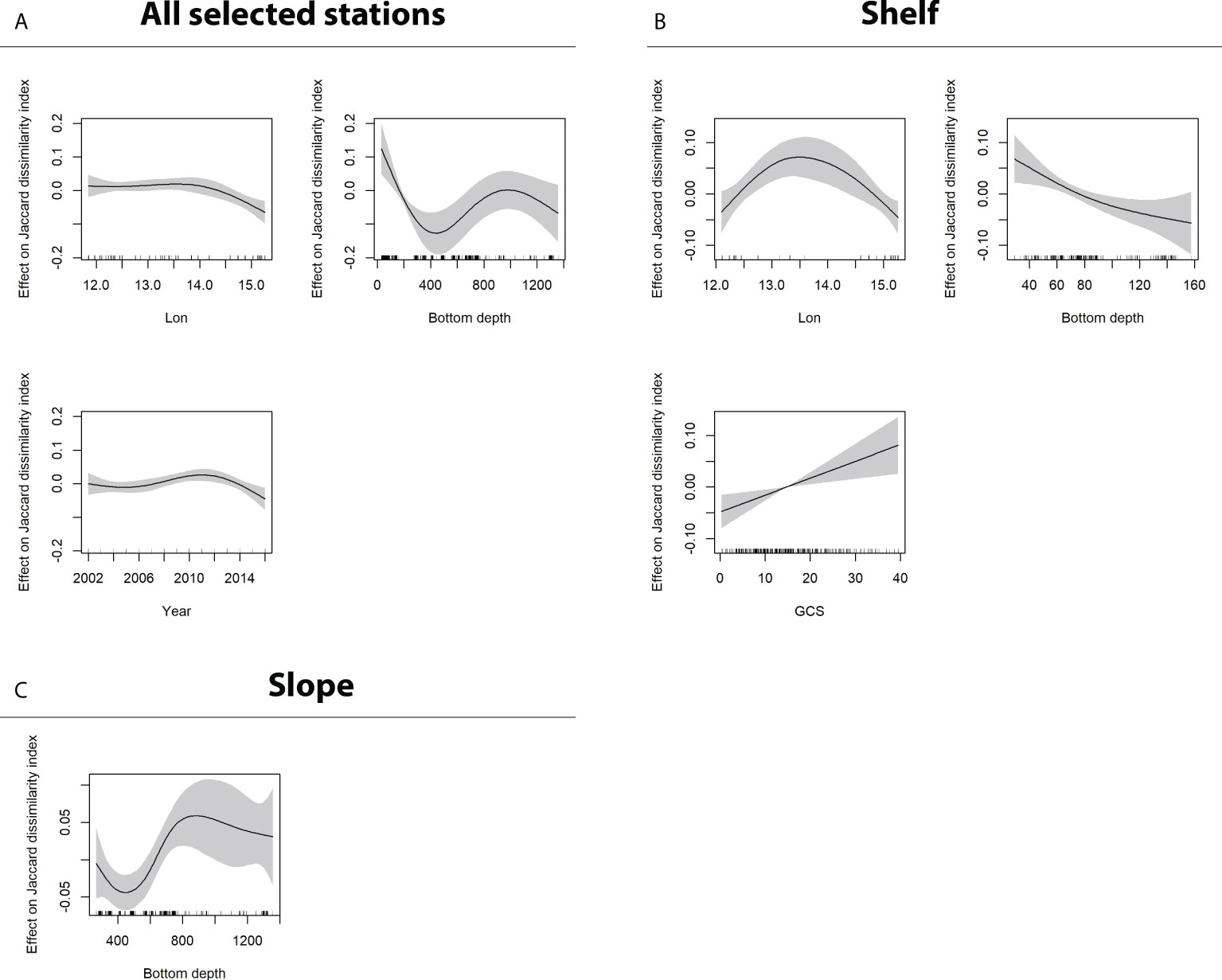
Figure 6 GAM output for the dependent variable Jaccard dissimilarity index. Sub-panels refer to model output obtained using (A) all data (shelf + slope), (B) shelf data (bottom depth ≤ 200 m), and (C) slope data (bottom depth > 200 m). Only significant factors are reported.
Results suggest a general significant decreasing compositional change (lower dissimilarity values), which is related to the last years of the time series (from 2012 onward). The observed temporal response mimics the one related to the longitudinal gradient. In addition, Jaccard dissimilarity shows a decreasing trend up to the bottom depth of about 400 m, which turns to be increasing for higher values. Finally, higher compositional changes in the shelf area (Figure 6B) are positively correlated with surface current speed and with intermediate longitude values, whereas in the slope sub-region (Figure 6C), the only significant factor is bottom depth, evidencing higher compositional changes at greater depths (> 600 m).
Discussion
Larval fish assemblage refers to ichthyoplankton data collected in continental shelf and slope areas of the SoS over 16 consecutive years (summer surveys carried out from 2001 to 2016). In particular, the sampling covered the depth layer of 0–100 m of the water column, identifying demersal, pelagic/epipelagic, meso/bathypelagic, and small pelagic species from 49 different fish families. Because of the limitations in species/genus identification that were related to the variable sorting skills of dedicated personnel over the relatively long period included in the analysis, the present study was based on larval information assembled at the family level. However, the role of the single families in affecting the detected patterns in terms of biodiversity indices was specified, also investigating whenever possible on the species involved in the changes of biodiversity indices.
More than one-third of the total number of collected larvae were from two small pelagic species, anchovy (family Engraulidae) and round sardinella (family: Clupeidae), a proportion that over the continental shelf area increased up to 42.0% (Table 2). However, the presence of meso/bathypelagic taxa was important and ubiquitous as well in both the shelf and slope sub-areas. After Clupeidae and Engraulidae, the most abundant families were Myctophidae (assembling 15 species) and Gonostomatidae (mostly one single species, Cyclothone braueri), jointly accounting for about 25% of the total in the whole study area (41.2% in the slope sub-region) (Table 2).
In terms of larval densities (Figure S6), Engraulidae (one species, Engraulis encrasicolus), Clupeidae (one species, Sardinella aurita), and Gobiidae alone accounted for more than 50% of the total taxa in shelf sub-area, where the spawning habitats of fish species from these families are located. Here, the highest average yearly larval concentration (up to 100 m−2) was found for anchovy, whose preferring spawning grounds in the study area occur at bottom depths shallower than 100 m (Quinci et al., 2022). In the slope area, the maximum average density (about 55 m−2) was observed for Gonostomatidae. The prevalence of Gonostomatidae in the offshore stations is in agreement with the spawning behavior of the dominant species (Cyclothone braueri) belonging to this family (Yoon et al., 2007) and with the spatial distribution of its larval stages (Torri et al., 2021). However, it is worth noting how Engraulidae and Clupeidae are quite abundant even in the slope area (jointly accounting for about 20% of the total) and that even other typically coastal species belonging to Labridae, Sparidae, and Gobidae are frequently found offshore.
In general, the observed patterns in the distribution of dominant species in both shelf and slope sub-areas are consistent with data reported for the Western and Central Mediterranean in previous studies (Torres et al., 2011; Cuttitta et al., 2016a; Cuttitta et al., 2016b; Cuttitta et al., 2018; Torri et al., 2018; Torri et al., 2021; Quinci et al., 2022), although further insights on the potential impact on the composition of larval assemblages by advection phenomena from/to the two sub-areas, as induced by local mesoscale oceanographic variability, are given herein. In particular, our analysis evidenced the impact of key hydrodynamic features on the ichthyoplankton biodiversity characterizing the SoS. The selection in the GAM analysis of surface current among the significant environmental factors able to affect biodiversity levels reflects this effect. However, it is interesting to note how the long-term data used in this study further support the dominance of larval stages of Clupeidae and Engraulidae in Sicilian and Tunisian waters of the SoS already found in previous investigations using much more time-limited datasets (Cuttitta et al., 2018). This is in contrast with the dominance of mesopelagic species observed in western Mediterranean (Torres et al., 2011), in the southern Tyrrhenian Sea (Cuttitta et al., 2016a), and in the Gulf of Sirte (Cuttitta et al., 2018). In addition, when considering all the stations over the period 2001–2016 listed in Table 1, the calculated yearly average taxa (family) richness (Fr = 33.4) is comparable with data (Fr = 34) reported in Cuttitta et al. (2016a), which refer to one survey carried out in the same area during the summer of 2009.
In general, the observed high abundance of myctophids and gonostomatids in the upper 100 m of the water column, even in the shelf area, confirms the epipelagic zone as an important environment for the development of larval stages of mesopelagic fishes, despite the typical depth distribution of the adult fractions of the corresponding fish populations (Watanabe et al., 2002; D’Elia et al., 2016).
The time series analysis of family richness by sub-areas evidenced greater biodiversity losses especially in the slope area during the last years of available data, from 2011 onward. This trend is probably linked the reduced advection of neritic taxa from the continental shelf and/or oceanic taxa to coastal areas. This was firstly suggested by the detected significance of surface current speed in GAMs applied to slope stations for both Tr and H’. Actually, although mesopelagic larvae dominate in the slope regions, their presence is quite common even in continental shelf probably in relation to the impact of horizontal advections linked to semi-permanent oceanographic structures that typically characterize this region during the summer season. Specifically, in agreement to Torri et al. (2021), the meandering path of the AIS can shape the distribution of mesopelagic species, transporting planktonic stages on the Adventure Bank (northwestern shelf) and on the Sicilian-Maltese Bank (southeastern shelf). Here, the occurrence of both neritic and oceanic species typically leads to higher biodiversity levels. Conversely, the presence of larval stages of coastal species in the slope region is only due to temporary advection by local oceanographic structures affected by the atmospheric events (Bignami et al., 2008; Torri et al., 2018). As a result, a reduced advection from coastal to the offshore zone may limit the contribution of neritic species to the larval assemblage in the slope area, leading to lower overall diversity in that region.
The potential role of the reduced advection of neritic taxa on the decreasing trend in biodiversity during the last years of the time series appears to be further confirmed by the lower average current speed regime characterizing the southeastern part of the study area (Figure S8). Actually, larval data show that, over the period 2010–2016, not only the abundance of the main neritic taxa was lower in the slope area (Figure S7), but that, in addition, other less frequent coastal/neritic families such as Blennidae, Callionymidae, Centracanthidae, Pomacentridae, Scorpaenidae, and Triglidae were often not found at all in our plankton samples, so reducing the level of Tr and H’ indexes (Table S1).
However, GAMs also indicated a moderate but significant decreasing temporal trend of α diversity metrics even in the shelf sub-area, where important drivers of spatial variability in biodiversity are the two factors Temp10 and ADT. In particular, high Fr values were found at low ADT and intermediate water temperature (23°C–24°C). Low ADT values over the shelf area are locally associated to coastal upwelling conditions, which are able to pump nutrient-rich waters, so supporting higher primary productivity (Patti et al., 2010). The detected optimal temperature level suggests a detrimental effect on biodiversity induced by extremely low- or high-temperature regimes. In addition, the lower surface current speed regime that characterized even the shelf area during the last years of the time series also appears to be beneficial in reducing compositional changes in the larval assemblage, as also indicated by the significance of current speed in the GAM model applied to the Jaccard dissimilarity index. However, the most important driver of turnover in the larval assemblage appears to be the bottom depth, suggesting an enhanced stability of the community in the coastal waters of neritic zone, where more important is the contribution to biodiversity by coastal demersal species. In this framework, it is worth noting that the decreasing trend in α biodiversity in shelf area, at least up to year 2012 was found to be linked the reduced occurrence of oceanic taxa such as Ophidiidae, Sternoptychidae, and Triglidae (Table S2), supporting the role of the advection of fish larvae from meso-bathypelagic and mostly deep demersal species from the slope area toward the coastal area in modulating biodiversity levels.
The differential trends between shelf and slope sub-areas also suggested to better investigate on other more specific spatial biodiversity patterns. Actually, Figure 3B evidenced the presence of a biodiversity hotspot in the frontal area characterizing the southeastern part of the study area, off the southernmost tip of Sicily (Cape Passero), as already reported by (Cuttitta et al. 2016a, Cuttitta et al. 2018). The observed pattern is further supported by the similarity between the response plots of the two explanatory factors Longitude and Salinity in Figures 4A, B, as it known that surface salinity locally presents a west–east increasing gradient that is induced by the motion of Atlantic Modified Water toward the Eastern Mediterranean basin (Robinson et al., 1999; García Lafuente et al., 2002). Therefore, the importance of concentration and retention processes locally induced by oceanographic features is confirmed by the present study (Figure 3A), not only in support of larval growth and survival, and related enhanced probability of recruitment success for many fish species as already shown by previous studies in the SoS (Torri et al., 2018; Patti et al., 2020; Russo et al., 2021; Torri et al., 2021; Russo et al., 2022; Cuttitta et al., 2022), but also more in general for sustaining high biodiversity levels (Figure 3B). Actually, frontal structures are generally responsible for plankton aggregation, so supporting the increase in feeding opportunities for fish larvae and inducing favorable conditions for their survival, as already observed in many other marine environments including the Mediterranean Sea (Bakun, 2006; Alemany et al., 2010; Avendaño-Ibarra et al., 2013; Erisman et al., 2017; Acha et al., 2018; Sato et al., 2018; Torri et al., 2018; Patti et al., 2020; Russo et al., 2021; Torri et al., 2021). In turn, this may account for the observed increased diversity in larval fish assemblages associated to spatial and temporal patterns of mesoscale oceanographic structures (Richardson et al., 2010; Rooker et al., 2013; Meinert et al., 2020). In the study area, the surface density front South of Cape Passero is dominated by temperature and is enhanced during the summer (Lermusiaux and Robinson, 2001; García Lafuente et al., 2005). Here, we speculate about the impact on larval biodiversity of the strength of density gradient across the front, which could be modulated by the alternating cyclonic and anti-cyclonic modes charactering the North Ionian Gyre (NIG) (Borzelli et al., 2009; Gačić et al., 2010; Gačić et al., 2013), whose effects on Sicilian intermediate waters have been recently investigated by Placenti et al. (2022). In particular, what we observed with data used in this study is higher biodiversity levels (and lower compositional changes as well) in the continental shelf stations of the frontal zone when the NIG is characterized by cyclonic circulation (years 2001–2005 and 2011–2016), a mode that is associated to enhanced advection of Atlantic Water (AW) to southern Levantine basin and a reduced deflection toward the Adriatic. Actually, in the same periods, the oceanographic conditions in the frontal zone were characterized by lower surface current speed (Figure S8) and higher temperature and salinity levels, testifying of a reduced inflow of AW in this area. However, the in-depth analysis of the relation between the quasi-decadal reversal of the NIG and larval biodiversity is out of the scope of the present paper and would deserve further insights.
Conclusion
In analyzing the temporal and spatial biodiversity patterns of larval fish assemblages in the SoS, this study may serve as a reference point for evaluating the role of changing environmental conditions on the community structure and ecosystem stability. In particular, the frontal feature characterizing during the summer the southeastern part of the study area, in favoring retention and concentration processes supporting growth and survival of early life stages, as already demonstrated in previous study on pelagic fish species, emerged as an important driver able to enhance biodiversity as well. Moreover, it has been shown that hydrological characteristics of the surface water affect ichthyoplankton biodiversity in coastal and offshore area, shading light on possible links with the quasi-decadal oceanographic variability patterns, such as the oscillation between anti-cyclonic and cyclonic phases characterizing the surface circulation of the Ionian Sea area, and encouraging further studies on possible future effects related to the climate change.
Data availability statement
The raw data supporting the conclusions of this article will be made available by the authors, without undue reservation.
Ethics statement
Ethical review and approval were not required for the animal study because this work is focused on planktonic stages in natural marine field.
Author contributions
BP analyzed the data and wrote the manuscript. MT contributed to data analyses and to the writing of the manuscript. AC contributed in sorting the plankton samples and in writing of the manuscript. All the authors contributed to develop the overall concept of the paper, interpreting the results and developing the discussion and the conclusions.
Funding
This study was mainly supported by the Italian National Research Council (CNR) through USPO office and by the FAO Regional Project MedSudMed “Assessment and Monitoring of the Fishery Resources and the Ecosystems in the Straits of Sicily”, funded by the Italian Ministry MIPAAF and co-funded by the Directorate General for Maritime Affairs and Fisheries of the European Commission (DG MARE). The study was also supported by several other research projects, including the EU projects “Distribution Biology and Biomass Estimates of the Sicilian Channel Anchovy” (MED96-052) and “The Sicilian Channel Anchovy Fishery and the underlying Oceanographic and Biological Processes conditioning their Interannual Fluctuations” (MED98-070), the Proreplus-Alif Project, funded by the Regione Siciliana Government (POR 2000–2006), and the Flagship Project RITMARE—The Italian Research for the Sea—coordinated by the Italian National Research Council and funded by the Italian Ministry of Education, University and Research within the National Research Program 2011–2013. Publication fees were covered by the ERA-NET Cofund on Blue Bioeconomy - Unlocking the potential of aquatic bioresources (BlueBio) — Grant Agreement number: 817992.
Acknowledgments
Mr. Emanuele Gentile, Master of the R/V Urania, and all his crew are thanked for their work in support to the plankton sampling during the oceanographic cruises. All the participating institutes and scientists who were on-board are gratefully acknowledged for their involvement in the work carried out. Special thanks go to Gaspare Buffa, Carmelo Buscaino, Luigi Giaramita, Carlo Patti, Francesco Placenti, and all researchers–technicians and scientific collaborators (including graduate students, PhD students, and postdoctoral researchers) for their support to the oceanographic cruises, sorting of ichthyoplankton samples and data processing, and to Salvatore Mazzola†, whose scientific efforts allowed the start of the regular sampling surveys that are at the base of the our study. Last but not the least, the first author wants to send special thanks to Dino Levi†, for inspiring the study thought his research work.
Conflict of interest
The authors declare that the research was conducted in the absence of any commercial or financial relationships that could be construed as a potential conflict of interest.
Publisher’s note
All claims expressed in this article are solely those of the authors and do not necessarily represent those of their affiliated organizations, or those of the publisher, the editors and the reviewers. Any product that may be evaluated in this article, or claim that may be made by its manufacturer, is not guaranteed or endorsed by the publisher.
Supplementary material
The Supplementary Material for this article can be found online at: https://www.frontiersin.org/articles/10.3389/fmars.2022.960929/full#supplementary-material
References
Acha E. M., Ehrlich M. D., Muelbert J. H., Pájaro M., Bruno D., Machinandiarena L., et al (2018). “Ichthyoplankton associated to the frontal regions of the southwestern atlantic,” in Plankton ecology of the southwestern Atlantic: From the subtropical to the subantarctic realm. Eds. Hoffmeyer M. S., Sabatini M. E., Brandini F. P., Calliari D. L., Santinelli N. H. (Berlin: Springer International Publishing), 219–246. doi: 10.1007/978-3-319-77869-3_11
Alemany F., Quintanilla L., Velez-Belchi P., Garcìa A., Cortés D., Rodríguez J. M., et al (2010). Characterization of the spawning habitat of Atlantic bluefin tuna and related species in the Balearic Sea (western Mediterranean). Prog. Oceanogr. 86, 21–38. doi: 10.1016/j.pocean.2010.04.014
Álvarez I., Catalán I. A., Jordi A., Palmer M., Sabatés A., Basterretxea G. (2012). Drivers of larval fish assemblage shift during the spring-summer transition in the coastal Mediterranean. Estuar. Coast. Shelf S. 97, 127–135. doi: 10.1016/j.ecss.2011.11.029
Álvarez I., Rodríguez J. M., Catalán I. A., Hidalgo M., Álvarez-Berastegui D., Balbín R., et al (2015). Larval fish assemblage structure in the surface layer of the northwestern Mediterranean under contrasting oceanographic scenarios. J. Plankton Res. 37 (4), 834–850. doi: 10.1093/plankt/fbv055
Avendaño-Ibarra R., Godínez-Domínguez E., Aceves-Medina G., González-Rodríguez E., Trasviña A. (2013). Fish larve response to biophysical changes in the gulf of California, Mexico (Winter-summer). J. Mar. Sci. 2013, 1–17. doi: 10.1155/2013/176760
Bakun A. (2006). Fronts and eddies as key structures in the habitat of marine fish larvae: Opportunity, adaptive response and competitive advantage. Sci. Mar. 70 (Suppl. 2), 105–122. doi: 10.3989/scimar.2006.70s2105
Bertolini F., D’Ancona U., Padoa Montalenti E., Ranzi S., Sanzo L., Sparta A., et al (1956). Uova, larve e stadi giovanili di teleostei. Fauna Flora Golfo Napoli Monogr. 38, 1–1064.
Bignami F., Böhm E., D’Acunzo E., D’Archino R., Salusti E. (2008). On the dynamics of surface cold filaments in the Mediterranean Sea. J. Mar. Syst. 74 (1-2), 429–442. doi: 10.1016/j.jmarsys.2008.03.002
Bonanno A., Placenti F., Basilone G., Mifsud R., Genovese S., Patti B., et al (2014). Variability of water mass properties in the strait of Sicily in summer period of 1998–2013. Ocean Sci. 10, 759–770. doi: 10.5194/os-10-759-2014
Borzelli G. L. E., Gačić M., Cardin V., Civitarese G. (2009). Eastern Mediterranean Transient and reversal of the Ionian Sea circulation. Geophys. Res. Lett. 36, L15108. doi: 10.1029/2009GL039261
Cardinale B. J., Duffy J., Gonzalez A., Hooper D. U., Perrings C., Venail P., et al (2012). Biodiversity loss and its impact on humanity. Nature 486, 59–67. doi: 10.1038/nature11148
Cardinale B. J., Palmer M. A., Collins S. L. (2002). Species diversity enhances ecosystem functioning through interspecific facilitation. Nature 415, 426–429. doi: 10.1038/415426a
Costa F. (1999). I pesci del Mediterraneo. Stadi larvali e giovanili. Messina: Grafo-Editor. Grafo E.
Cuttitta A., Arigò A., Basilone G., Bonanno A., Buscaino G., Rollandi L., et al (2004). Mesopelagic fish larvae species in the strait of Sicily and their relationships to main oceanographic events. Hydrobiologia 527 (1), 177–182. doi: 10.1023/B:HYDR.0000043299.65829.2f
Cuttitta A., Bonomo S., Zgozi S., Bonanno A., Patti B., Quinci E. M., et al (2016b). The influence of physical and biological processes on the ichthyoplankton communities in the gulf of sirte (Southern Mediterranean Sea). Mar. Ecol. 37 (4), 831–844. doi: 10.1111/maec.12362
Cuttitta A., Quinci E. M., Patti B., Bonomo S., Bonanno A., Musco M., et al (2016a). Different key roles of mesoscale oceanographic structures and ocean bathymetry in shaping larval fish distribution pattern: A case study in Sicilian waters in summer 2009. J. Sea Res. 115, 6–17. doi: 10.1016/j.seares.2016.04.005
Cuttitta A., Torri M., Zarrad R., Zgozi S., Jarboui O., Quinci E. M., et al (2018). Linking surface hydrodynamics to planktonic ecosystem: The case study of the ichthyoplanktonic assemblages in the central Mediterranean Sea. Hydrobiologia 821 (1), 191–214. doi: 10.1007/s10750-017-3483-x
Cuttitta A., Patti B., Musco M., Masullo T., Placenti F., Quinci E. M., et al. (2022). Inferring population structure from early life stage: The case of the european anchovy in the sicilian and maltese shelves. Water 14, 1427. doi: 10.3390/w14091427
D’Elia M., Warren J. D., Rodriguez-Pinto I., Sutton T. T., Cook A., Boswell K. M. (2016). Diel variation in the vertical distribution of deep-water scattering layers in the gulf of Mexico. Deep Sea Res. Part I Oceanogr. Res. Pap. 115, 91–102. doi: 10.1016/j.dsr.2016.05.014
Dornelas M., Gotelli N. J., McGill B. J., Shimadzu H., Moyes F., Sievers C., et al (2014). Assemblage time series reveal biodiversity change but not systematic loss. Science 344, 296–299. doi: 10.1126/science.1248484
Duffy J. E. (2009). Why biodiversity is important to the functioning of real-world ecosystems. Front. Ecol. Environ. 7, 437–444. doi: 10.1890/070195
Erisman B., Heyman W., Kobara S., Ezer T., Pittman S., Aburto-Oropeza O., et al (2017). Fish spawning aggregations: where well-placed management actions can yield big benefits for fisheries and conservation. Fish Fish. 18, 128–144. doi: 10.1111/faf.12132
Falcini F., Corrado R., Torri M., Mangano M. C., Zarrad R., Di Cintio A., et al (2020). Seascape connectivity of European anchovy in the central Mediterranean Sea revealed by weighted Lagrangian backtracking and bio-energetic modelling. Sci. Rep. 10 (1), 1–13. doi: 10.1038/s41598-020-75680-8
Falcini F., Palatella L., Cuttitta A., Buongiorno Nardelli B., Lacorata G., Lanotte A. S., et al (2015). The role of hydrodynamic processes on anchovy eggs and larvae distribution in the Sicily channel (Mediterranean sea): A case study for the 2004 data set. PLoS One 10 (4), e0123213. doi: 10.1371/journal.pone.0123213
Gačić M., Borzelli G. L. E., Civitarese G., Cardin V., Yari S. (2010). Can internal processes sustain reversals of the ocean upper circulation? the Ionian Sea example. Geophys. Res. Lett. 37, L09608. doi: 10.1029/2010GL043216
Gačić M., Schroeder K., Civitarese G., Cosoli S., Vetrano A., Borzelli G. L. E. (2013). Salinity in the Sicily channel corroborates the role of the Adriatic-Ionian bimodal oscillating system (BiOS) in shaping the decadal variability of the Mediterranean overturning circulation. Ocean Sci. 9, 83–90. doi: 10.5194/os-9-83-2013
Gamfeldt L., Lefcheck J. S., Byrnes J. E., Cardinale B. J., Duffy J. E., Griffin J. N. (2014). Marine biodiversity and ecosystem functioning: What’s known and what’s next? Oikos 124, 252–265. doi: 10.1111/oik.01549
García Lafuente J., García A., Mazzola S., Quintanilla L., Delgado J., Cuttitta A., et al (2002). Hydrographic phenomena influencing early life stages of the Sicilian channel anchovy. Fish. Oceanogr. 11, 31–44. doi: 10.1046/j.1365-2419.2002.00186.x
García Lafuente J., Vargas J. M., Criado F., García A., Delgado J., Mazzola S. (2005). Assessing the variability of hydrographic processes influencing the life cycle of the Sicilian channel anchovy, engraulis encrasicolus, by satellite imagery. Fish. Oceanogr. 14, 32–46. doi: 10.1111/j.1365-2419.2004.00304.x
Gray C. A., Johnson D. D., Broadhurst M. K., Young D. J. (2005). Seasonal, spatial and gear-related influences on relationships between retained and discarded catches in a multi-species gillnet fishery. Fish. Res. 75, 56–72. doi: 10.1016/j.fishres.2005.04.014
Hernandez F. J. Jr., Powers S. P., Graham W. M. (2010). Seasonal variability in ichthyoplankton abundance and assemblage composition in the northern gulf of Mexico off Alabama. Fish. Bull. 108, 193–207.
Hooper D. U., Adair E. C., Cardinale B., Byrnes J. E. K., Hungate B. A., Matulich K. L., et al (2012). A global synthesis reveals biodiversity loss as a major driver of ecosystem change. Nature 486, 105–108. doi: 10.1038/nature11118
Houde E. (2002). “Mortality,” in Fishery science, the unique contributions of early life stages. Eds. Fuiman L. A., Werner R. G. (Oxford: Blackwell Science), 64–87.
Isari S., Fragopoulu N., Somarakis S. (2008). Interranual variability in horizontal patterns of larval fish assemblages in the Northeastern Aegean Sea (Eastern Mediterranean) during early summer. Estuar. Coast. Shelf Sci. 79 (4), 607–619. doi: 10.1016/j.ecss.2008.06.001
Jaccard P. (1908). Nouvelles recerches sur la distribution florale. Bull. Soc Vaud. Sci. Nat. 44, 223–270.
Jennings S., Kaiser M. J. (1998). The effects of fishing on marine ecosystems. Adv. Mar. Biol. 34, 201–351. doi: 10.1016/S0065-2881(08)60212-6
Joint I., Groom S. (2000). Estimation of phytoplankton production from space: Current status and future potential of satellite re-mote sensing. J. Exp. Mar. Biol. Ecol. 250 (1-2), 233–255. doi: 10.1016/S0022-0981(00)00199-4
Kara A. B., Rochford P. A., Hurlburt H. E. (2000). An optimal definition for ocean mixed layer depth. J. Geophys. Res. 105 (C7), 16803–16821. doi: 10.1029/2000JC900072
Katsuragawa M., Dias J. F., Harari C., Namiki C., Zani-Teixeira M. L. (2014). Patterns in larval fish assemblages under the influence of the Brazil current. Cont. Shelf Res. 89, 103–117. doi: 10.1016/j.csr.2014.04.024
Lehodey P., Alheit J., Barange M., Baumgartner T., Beaugrand G., Drinkwater K., et al (2006). Climate variability, fish, and fisheries. J. Clim. 19, 5009–5030. doi: 10.1175/JCLI3898.1
Lermusiaux P. F. J. (1999). Estimation and study of mesoscale variability in the strait of Sicily. Dyn. Atm. Ocean. 29, 255–303. doi: 10.1016/S0377-0265(99)00008-1
Lermusiaux P. F. J., Robinson A. R. (2001). Features of dominant mesoscale variability, circulation patterns and dynamics in the strait of Sicily. Deep Sea Res. I 48, 1953–1997. doi: 10.1016/S0967-0637(00)00114-X
López-Sanz À., Vert N., Zabala M., Sabatés A. (2009). Small-scale distribution of fish larvae around the medes islands marine protected area (NW Mediterranean). J. Plankton Res. 31 (7), 763–775. doi: 10.1093/plankt/fbp024
Loreau M., Naeem S., Inchausti P., Bengtsson J., Grime J. P., Hector A. (2001). Biodiversity and ecosystem functioning: current knowledge and future challenges. Science 294, 804–808. doi: 10.1126/science.1064088
Magurran A. E., Dornelas M., Moyes F., Henderson P. A. (2019). Temporal β diversity–a macroecological perspective. Global Ecol. Biogeogr. 28, 1949–1960. doi: 10.1111/geb.130
Malavolti S., De Felice A., Costantini I., BIAGOTTI I., Canduci G., Grilli F., et al (2018). Distribution of engraulis encrasicolus eggs and larvae in relation to coastal oceanographic conditions (the South-Western Adriatic Sea case study). Mediterr. Mar. Sci. 19 (1), 180–192. doi: 10.12681/mms.14402
Mazzola S., García A., García Lafuente J. (2000). “Distribution, Biology and Biomass estimates of the Sicilian Channel anchovy. DG XIV, MED 96/052, Final Report”, in Many-species populations. An introduction to Mathematical Ecology, ed. Pielou E. C. (New York: Wiley-Interscience), 203–272.
Meinert C. R., Clausen-Sparks K., Cornic M., Sutton T. T., Rooker J. R. (2020). Taxonomic richness and diversity of larval fish assemblages in the oceanic gulf of Mexico: Links to oceanographic conditions. Front. Mar. Sci. 7. doi: 10.3389/fmars.2020.00579
Mittermeier R. A., Turner W. R., Larsen F. W., Brooks T. M., Gascon C. (2011). “Global biodiversity conservation: the critical role of hotspots,” in Biodiversity hotspots. Eds. Zachos F., Habel J. (Berlin: Springer). doi: 10.1016/j.gecco.2014.12.008
Moser H. G., Ahlstrom E. H. (1996). “Myctophidae: lanternfishes,” in The early stages on fishes in the California current region, California cooperative oceanic fisheries investigations (CalCOFI), atlas n°33. Ed. Moser H. G., 1505 (387–475). Lawrence, Kansas: Allen Press, Inc.
Moyano M., Hernández-León S. (2011). Intra- and interannual variability in the larval fish assemblage off gran canaria (Canary islands) over 2005-2007. Mar. Biol. 158 (2), 257–273. doi: 10.1007/s00227-010-1556-8
Moyano M., Rodríguez J. M., Benítez-Barrios V. M., Hernández-León S. (2014). Larval fish distribution and retention in the canary current system during the weak upwelling season. Fish. Oceanogr. 23 (3), 191–209. doi: 10.1111/fog.12055
Moyano M., Rodríguez J. M., Hernández-León S. (2009). Larval fish abundance and distribution during the late winter bloom off Gran Canaria Island, canary islands. Fish. Oceanogr. 18 (1), 51–61. doi: 10.1111/j.1365-2419.2008.00496.x
Naeem S., Bunker D. E., Hector A., Loreau M., Perrings C. (2009). Biodiversity, ecosystem-functioning, and human wellbeing. New York: Oxford University Press.
Nonaka R. H., Matsuura Y., Suzuki K. (2000). Seasonal variation in larval fish assemblages in relation to oceanographic conditions in the abrolhos bank region off eastern Brazil. Fish. Bull. 98, 767–784.
Oksanen J., Blanchet F. G., Friendly M., Kindt R., Legendre P., McGlinn D., et al (2020). Vegan: Community ecology package (R package version 2), 5–7. Available at: https://CRAN.R-project.org/package=vegan.
Olivar M. P., Sabatés A., Alemany F., Balbín R., Fernández de Puelles M. L., Torres A. P. (2014). Diel-depth distributions of fish larvae off the Balearic islands (western Mediterranean) under two environmental scenarios. J. Mar. Syst. 138, 127–138. doi: 10.1016/j.jmarsys.2013.10.009
Olivar M. P., Salat J., Palomera I. (2001). Comparative study of spatial distribution patterns of the early stages of anchovy and pilchard in the NW Mediterranean Sea. Mar. Ecol. Prog. Ser. 217, 111–120. doi: 10.3354/meps217111
Ospina-Álvarez A., Bernal M., Catalan A., Roos D., Bigot J.-L., Palomera I. (2013). Modeling fish egg production and spatial distribution from acoustic data: A step forward into the analysis of recruitment. PLoS One 8, e73687. doi: 10.1371/journal.pone.0073687
Ospina-Álvarez A., Catalán I. A., Bernal M., Roos D., Palomera I. (2015). From egg production to recruits: Connectivity and interannual variability in the recruitment patterns of European anchovy in the northwestern Mediterranean. Prog. Oceanogr. 138, 431–447. doi: 10.1016/j.pocean.2015.01.011
Palomera I. (1991). Vertical distribution of eggs and larvae of engraulis encrasicolus in stratified waters of the Western Mediterra-nean. Mar. Biol. 111 (1), 37–44. doi: 10.1007/BF01986343
Patti B., Guisande C., Bonanno A., Basilone G., Cuttitta A., Mazzola S. (2010). Role of physical forcings and nutrient availability on the control of satellite-based chlorophyll a concentration in the coastal upwelling area of the Sicilian channel. Sci. Mar. 74, 577–588. doi: 10.3989/scimar.2010.74n3577
Patti B., Torri M., Cuttitta A. (2020). General surface circulation controls the interannual fluctuations of anchovy stock biomass in the central Mediterranean Sea. Sci. Rep. 10, 1554. doi: 10.1038/s41598-020-58028-0
Patti B., Zarrad R., Jarboui O., Cuttitta A., Basilone G., Aronica S., et al (2018). Anchovy (Engraulis encrasicolus) early life stages in the central Mediterranean Sea: connectivity issues emerging among adjacent sub-areas across the strait of Sicily. Hydrobiologia 821 (1), 25–40. doi: 10.1007/s10750-017-3253-9
Piccioni A., Gabrielle M., Salusti E., Zambianchi E. (1988). Wind-induced upwellings off the southern coast of Sicily. Oceanol. Acta 11, 309–314.
Pikitch E. K., Santora C., Babcock E. A., Bakun A., Bonfil R., Conover D. O., et al (2004). Ecology. ecosystem-based fishery management. Science 305, 346–347. doi: 10.1126/science.1098222
Placenti F., Torri M., Pessini F., Patti B., Tancredi V., Cuttitta A., et al (2022). Hydrological and biogeochemical patterns in the Sicily channel: New insights from the last decade (2010- 2020). Front. Mar. Sci. 9, 733540. doi: 10.3389/fmars.2022.733540
Planque B., Bellier E., Lazure P. (2007). Modelling potential spawning habitat of sardine (Sardina pilchardus) and anchovy (Engraulis encrasicolus) in the bay of Biscay. Fish. Oceanogr. 16 (1), 16–30. doi: 10.1111/j.1365-2419.2006.00411.x
Ponti M., Perlini R. A., Ventra V., Grech D., Abbiati M., Cerrano C. (2014). Ecological shifts in Mediterranean coralligenous assemblages related to gorgonian forest loss. PLoS One 9 (7), e102782. doi: 10.1371/journal.pone.0102782
Poulard J. C., Blanchard F. (2005). The impact of climate change on the fish community structure of the eastern continental shelf of the bay of Biscay. ICES J. Mar. Sci. 62, 1436–1443. doi: 10.1016/j.icesjms.2005.04.017
Pujol I., Larnicol G. (2005). Mediterranean Sea eddy kinetic energy variability from 11 years of altimetric data. J. Mar. Sys. 58 (3), 121–142. doi: 10.1016/j.jmarsys.2005.07.005
Quinci E. M., Torri M., Cuttitta A., Patti B. (2022). Predicting potential spawning habitat by ensemble species distribution models: The case study of European anchovy (Engraulis encrasicolus) in the strait of Sicily. Water 14, 1400. doi: 10.3390/w14091400
R Core Team (2022). R: A language and environment for statistical computing (Vienna, Austria: R Foundation for Statistical Computing). Available at: https://www.R-project.org/.
Rocha J., Yletyinen J., Biggs R., Blenckner T., Peterson G. (2015). Marine regime shifts: drivers and impacts on ecosystems services. Philos. Trans. R. Soc. B Biol. Sci. 370, 20130273.
Richardson D. E., Llopiz J. K., Guigand C. M., Cowen R. K. (2010). Larval assemblages of large and medium-sized pelagic species in the straits of Florida. Prog. Oceanogr. 86, 8–20. doi: 10.1016/j.pocean.2010.04.005
Rijnsdorp A. D., Peck M. A., Engelhard G. H., Mollmann C., Pinnegar J. K. (2009). Resolving the effect of climate change on fish populations. ICES J. Mar. Sci. 66, 1570–1583. doi: 10.1093/icesjms/fsp056
Robinson A. R., Golnaraghi M., Leslie W. G., Artegiani A., Hetch A., Lazzoni E., et al (1991). The eastern Mediterranean general circulation: features structure and variability. Dyn. Atm. Ocean. 15, 215–240. doi: 10.1016/0377-0265(91)90021-7
Robinson A. R., Sellschopp J., Warn-Varnas A., Leslie W. G., Lozano C. J., Haley P. J., et al (1999). The Atlantic Ionian stream. J. Mar. Sys. 20, 113–128. doi: 10.1016/S0924-7963(98)00079-7
Rochet M.-J., Trenkel V. M., Carpentier A., Coppin F., Gil de Sola L., Léauté J.-P., et al (2010). Do changes in environmental pressures impact marine communities? an empirical assessment. J. Appl. Ecol. 47, 741–750. doi: 10.1111/j.1365-2664.2010.01841.x
Rooker J. R., Kitchens L. L., Dance M. A., Wells R. J., Falterman B., Cornic M. (2013). Spatial, temporal, and habitat-related variation in abundance of pelagic fishes in the gulf of Mexico: Potential implications of the deepwater horizon oil spill. PLoS One 8, e76080. doi: 10.1371/journal.pone.0076080
Russo S., Torri M., Patti B., Musco M., Masullo T., Di Natale M. V., et al (2022). Environmental conditions along tuna larval dispersion: Insights on the spawning habitat and impact on their development stages. Water 14, 1568. doi: 10.3390/w14101568
Russo S., Torri M., Patti B., Reglero P., Álvarez-Berastegui D., Cuttitta A., et al (2021). Unveiling the relationship between Sea surface hydrographic patterns and tuna larval distribution in the central Mediterranean Sea. Front. Mar. Sci. 8. doi: 10.3389/fmars.2021.708775
Sabatés A. (1990). Distribution pattern of larval fish populations in the northwestern Mediterranean. Mar. Ecol. Prog. Ser. 59 (1), 75–82. doi: 10.3354/meps059075
Sabatés A., Zaragoza N., Grau C., Salat J. (2008). Vertical distribution of early developmental stages in two coexisting clupeoid species, sardinella aurita and engraulis encrasicolus. Mar. Ecol. Prog. Ser. 364, 169–180. doi: 10.3354/meps07461
Sato M., Barth J. A., Benoit-Bird K. J., Pierce S. D., Cowles T. J., Brodeur R. D., et al (2018). Coastal upwelling fronts as a boundary for planktivorous fish distributions. Mar. Ecol. Prog. Ser. 595, 171–186. doi: 10.3354/meps12553
Tilman D., Isbell F., Cowles J. M. (2014). Biodiversity and ecosystem functioning. Annu. Rev. Ecol. Evol. Syst. 45, 471–493. doi: 10.1146/annurev-ecolsys-120213-091917
Tilman D., Reich P. B., Isbell F. (2012). Biodiversity impacts ecosystem productivity as much as resources, disturbance, or herbivory. Proc. Natl. Acad. Sci. U.S.A. 109, 10394–10397. doi: 10.1073/pnas.1208240109
Torres A. P., Reglero P., Balbin R., Urtizberea A., Alemany F. (2011). Coexistence of larvae of tuna species and other fish in the surface mixed layer in the NWMediterranean. J. Plankton Res. 33, 1793–1812. doi: 10.1093/plankt/fbr078
Torri M., Corrado R., Falcini F., Cuttitta A., Palatella L., Lacorata G., et al (2018). Planktonic stages of small pelagic fishes (Sardinella aurita and engraulis encrasicolus) in the central Mediterranean Sea: The key role of physical forcings and implications for fisheries management. Prog. Oceanogr. 162, 25–39. doi: 10.1016/j.pocean.2018.02.009
Torri M., Pappalardo A. M., Ferrito V., Giannì S., Armeri G. M., Patti C., et al (2021). Signals from the deep-sea: Genetic structure, morphometric analysis, and ecological implications of cyclothone braueri (Pisces, gonostomatidae) early life stages in the central Mediterranean Sea. Mar. Environ. Res. 169, 105379. doi: 10.1016/j.marenvres.2021.105379
Tortonese E. (1970). Osteichthyes. pesci ossei. fauna d’Italia. Bologna, Italia: Edizioni Caldarini.
Vilchis L. I., Balance L. T., Watson W. (2009). Temporal variability of neustonic ichthyoplankton assemblages of the eastern pacific warm pool: Can community structure be linked to climate variability? Deep Sea Res. I 56, 125–140. doi: 10.1016/j.dsr.2008.08.004
Watanabe H., Kawaguchi K., Hayashi A. (2002). Feeding habits of juvenile surface-migratory myctophid fishes (family myctophidae) in the kuroshio region of the Western North Pacific. Mar. Ecol. Prog. Ser. 236, 263–272. doi: 10.3354/meps236263
Whittaker R. H. (1960). Vegetation of the siskiyou mountains, Oregon and California. Ecol. Monogr. 30, 279–338. doi: 10.2307/1943563
Whittaker R. H. (1972). Evolution and measurement of species diversity. Taxon 21, 213–251. doi: 10.2307/1218190
Wood S. N. (2011). Fast stable restricted maximum likelihood and marginal likelihood estimation of semiparametric generalized linear models. J. R. Stat. Soc Ser. B Methodol. 73 (1), 3–36. doi: 10.1111/j.1467-9868.2010.00749.x
Worm B., Barbier E. B., Beaumont N., Duffy J. E., Folke C., Halpern B. S., et al (2006). Impacts of biodiversity loss on ocean ecosystem services. Science 314 (5800), 787–790. doi: 10.1126/science.1132294
Yoon W. D., Nival P., Choe S. M., Picheral M., Gorsky G. (2007). Vertical distribution and nutritional behaviour of cyclothone braueri, nematoscelis megalops, meganyctiphanes norvegica and salpa fusiformis in the NW Mediterranean mesopelagic zone. ICES CM 2007/F:03, 1–28.
Zarrad R., Alemany F., Rodriguez J.-M., Jarboui O., Lopez-Jurado J.-L., Balbin R. (2013). Influence of summer conditions on the larval fish assemblage in the eastern coast of Tunisia (Ionian Sea, southern Mediterranean). J. Sea Res. 76, 114–125. doi: 10.1016/j.seares.2012.08.001
Zarrad R., Rodríguez J. M., Alemany F., Charef A., Jarboui O., Missaoui H. (2020). Larval fish community composition and distribution of the central-southern mediterranean under summer and winter conditions. Acta Ichthyol. Piscat. 50 (3), 313–324. doi: 10.3750/AIEP/02957
Keywords: biodiversity, fish larvae, mesopelagic, demersal, pelagic, epipelagic, Central Mediterranean, Strait of Sicily
Citation: Patti B, Torri M and Cuttitta A (2022) Interannual summer biodiversity changes in ichthyoplankton assemblages of the Strait of Sicily (Central Mediterranean) over the period 2001–2016. Front. Mar. Sci. 9:960929. doi: 10.3389/fmars.2022.960929
Received: 03 June 2022; Accepted: 18 July 2022;
Published: 11 August 2022.
Edited by:
Çetin Keskin, Istanbul University, TurkeyReviewed by:
M. Pilar Olivar, Institute of Marine Sciences, Spanish National Research Council (CSIC), SpainMilica Mandic, University of Montenegro, Montenegro
Copyright © 2022 Patti, Torri and Cuttitta. This is an open-access article distributed under the terms of the Creative Commons Attribution License (CC BY). The use, distribution or reproduction in other forums is permitted, provided the original author(s) and the copyright owner(s) are credited and that the original publication in this journal is cited, in accordance with accepted academic practice. No use, distribution or reproduction is permitted which does not comply with these terms.
*Correspondence: Marco Torri, bWFyY28udG9ycmlAY25yLml0