- 1Faculty of Fisheries and Food Science, Universiti Malaysia Terengganu, Kuala Nerus, Malaysia
- 2Institute of Food Security and Sustainable Agriculture, Universiti Malaysia Kelantan, Jeli, Malaysia
- 3Faculty of Agro-Based Industry, Universiti Malaysia Kelantan, Jeli Campus, Kelantan, Malaysia
- 4Faculty of Agriculture, Universiti Islam Sultan Sharif Ali (UNISSA), Bandar Seri Begawan, Brunei
- 5Higher Institution Centre of Excellence (HICoE), Institute of Tropical Aquaculture and Fisheries, University Malaysia Terengganu, Kuala Nerus, Malaysia
The spread of Vibrio spp. and the prevalence of vibriosis around the world are often correlated with global warming, especially an increase in sea surface temperature. However, many ecological and virulence factors also trigger the spread of Vibrio species, while vibriosis occurs at both low and high temperatures. Recent studies that focused on a single factor (e.g. temperature) and one species (e.g. V. vulnificus) also showed that single factors such as temperature were insufficient to explain the pathogenicity and virulence of Vibrio spp. In this review, we have compiled and discussed the existing literature on the effect of temperature on different growth and pathogenicity of Vibrio spp. A systematic literature search was conducted using Web of Science (WoS) and SCOPUS databases up to 1st January 2022. After applying the selection criteria, a final list of 111 articles was included in this review. We have found that only certain Vibrio spp. are positively impacted by the increase in temperature. The results showed that 47% of the articles reported an increase in growth with the increase in temperature, while 38% showed that the relationship is complex. Reduced growth (6%) and no effect (9%) were also reported. These results showed that most but not all Vibrio spp. were positively impacted by an increase in temperature. The results indicated that V. alginolyticus, V. cholerae, V. parahaemolyticus, and V. vulnificus are the biggest threats to human and animal wellbeing.
Introduction
The increased occurrence of vibrio diseases (vibriosis) and the spread of Vibrio spp. to new geographical areas were correlated with global warming, especially temperature. This could be due to Vibrio spp. ability to remain in a “viable but nonculturable” (VBNC) state, until favourable conditions such as a rise in temperature occur, in which it becomes culturable and grows (Hernández-Cabanyero et al., 2020; Montanchez and Kaberdin, 2020). However, many ecological and virulence factors are also correlated with the spread of Vibrio species and vibriosis. Moreover, despite the rich literature on vibriosis, these studies were not connected and were either too narrow or too qualitative (Froelich et al., 2017; Rahman et al., 2017; Hernández-Cabanyero et al., 2019; Montánchez et al., 2019). This became evident when studies that focused on one factor (e.g. temperature) and one species (e.g. V. vulnificus) found that single factors such as temperature were insufficient to explain the pathogenicity and virulence of Vibrio spp. in many cases (Kim et al., 2012; Froelich et al., 2017; Rahman et al., 2017; Hernández-Cabanyero et al., 2019; Montánchez et al., 2019). This was unlikely as even among Vibrio spp., their optimum growth parameters (temperature, salinity, and pH conditions) are significantly different. For example, V. vulnificus can remain viable and grow between 4-14°C, while its optimum growth is at a much higher temperature (25°C) and low salinity (1-2%). V. cholerae could grow in 10% salinity and 35°C but flourished at a slightly lower temperature (30°C) and higher salinity (15%) (Chowdhury et al., 2017). Besides, cold water vibriosis outbreaks associated with V. salmonicida were reported to occur at very low temperatures (3-10°C) both in nature and in laboratory settings (Colquhoun et al., 2002). Other arguments can also be observed in published literature including the minimum temperature that enables V. parahaemolyticus growth. For instance, some studies have reported that V. parahaemolyticus needs a minimum temperature of 13°C to grow in broth (Kim et al., 2012), while other studies argued that a temperature as low as 5°C is sufficient (Miles et al., 1997). The increased prevalence of pathogenic Vibrio species calls for global attention from researchers and clinicians. Therefore, a systematic review of temperature vs growth rate of vibrio is important to predict Vibriosis episodes. Identifying these risks will also help in formulating mitigation plans, especially for seafood illnesses in humans as well as for the mariculture industry. Hence, this systematic review aimed to compile the literature that addressed the effect of an increase in temperature on the growth of Vibrio spp. It is hypothesized that only certain thermostable Vibrio spp. are positively impacted by an increase in temperature. The review will also address the increased pathogenicity of Vibrio spp. under elevated temperatures to assess which Vibrio spp. pose the highest threat in this era of global warming.
Methodology
Review Question and Scope
Previous studies reported opposing arguments and findings on the correlation between global warming and the growth of Vibrio spp. were the main motivations behind this systematic review. Besides, Vibrio spp. have significantly different optimum growth temperatures and conditions. We addressed 1) Do all Vibrio spp. flourish and become virulent with the increase in temperature? and, 2) Which Vibrio spp. pose a significant threat to humans and animals in the era of global warming.’ During the write-up and whenever applicable, the articles were cited chronologically (year) to show the progress or direction of the research.
Search strategy
A systematic literature search was conducted using Web of Science (WoS) and SCOPUS databases up to 1st January 2022. The search was limited to these 2 databases due to the quality of these databases and the abundance of literature on the subject. The keywords used were “Vibrio AND temperature”. A total of 3063 and 3542 documents were retrieved from WoS and SCOPUS databases, respectively. The search was then limited to the article search keyword appearing in the title which yielded a search term of “TITLE (vibrio AND temperature)”. A total of 202 and 238 articles were retrieved from WOS and SCOPUS databases respectively which were then subjected to further screening. These 2 search results were combined (202 + 238) resulting in 440 articles which were then subjected to screening.
Screening and Eligibility Criteria
All the 202 articles in WOS were included in the 238 articles from SCOPUS and duplicates were removed resulting in 238 articles that were then subjected to inclusion/exclusion criteria. Articles (n=128) were excluded due to either no full paper, not in English, or no growth profiles. Examples of articles excluded due to lack of growth profiles were articles on the effect of temperature on non-growth aspects such as susceptibility to infection, fatty acid production, food applications, substrate utilization, etc. A final list of 111 articles was considered for this review (Figure 1).
Results and Discussion
Initial Screening
Initial screening of the selected publications (n=111) showed that 91% of the articles argued that temperature has a significant impact on Vibrio spp. growth, while no effect was observed over the tested range in 9% of the articles (10 out of 111). The increase in temperature positively correlated with growth in 47% of the articles (52 out of 111), while temperature negatively correlated with growth in 6% of the articles (7 out of 111) and. The relationship between the increase in temperature and growth of Vibrio spp. was found to be complex or multifactorial in 42/111 articles (38%). In the initial screening, WOS was expected to yield fewer articles as the database contains fewer journals. For example, in 2016, WoS contained 13,605 journals, while Scopus contained 20,346 journals (Mongeon and Paul-Hus, 2016). Initial classification justified the aim of this review as the relationship between Vibrio spp. growth and temperature was found to be complex in 38% of the studies, even though more articles (47%) supported the consensus that Vibrio spp. grow better at higher temperatures. Most recent articles were also in favour of the theory that the relationship is significant but might not be as linear. For instance, Vibrio cases in Canada with maximum sea surface temperature (SST) were studied over 25 years (1992 to 2017) (Galanis et al., 2020). The correlation was significant with the correlation coefficient (r2) of 0.46, which is considered not strong (Fischer et al., 2016; Ramakers et al., 2017). V. vulnificus virulence was also found recently to be multifactorial due to the complex interaction between temperature and other factors such as iron availability. The study showed that despite temperature increasing growth and general virulence factors (e.g. Motility), main virulence factors (e.g. hemolysin VvhA) were controlled by iron concentration (Hernández-Cabanyero et al., 2020). Hence, the discrepancies presented in the introduction were supported by this review.
Effect of Temperature On The Growth of Vibrio spp.
Vibrio spp. were able to grow in temperatures ranging from 0.5°C to 48°C which showed their unique adaptability to changes in temperature (Figure 2). First, this range covers Vibrio spp. that could be potentially pathogenic to animals (22°C), especially aquatic animals, and humans (37°C) (Tall et al., 2013). Secondly, several human and animal pathogens species, V. cholerae, V. parahaemolyticus, and V. vulnificus, were able to grow at ≤10°C and ≥35°C. This is concerning as V. cholera, V. parahaemolyticus, V. alginolyticus, and V. vulnificus are well known to be pathogenic to humans (Baker-Austin et al., 2018). Optical density (OD) data showed a slight positive correlation with temperature while log growth data did not show any pattern. The temperature range was then divided into 4 intervals to investigate if certain species are correlated with certain temperature ranges. The intervals selected were ≤10°C, 11-22°C, 23-35°C, and ≥36°C (Figure 2). A disproportionately high number of studies focused on V. parahaemolyticus. Hence, data were converted to a percentage and labelled as prevalence. V. alginolyticus, V. cholerae, V. costicola, V. parahaemolyticus, and V. vulnificus were found to grow in all 4 temperature ranges, while V. harveyi and V. Marinus were able to grow in 1 interval only (23-35°C). Growth ranges from reviewed articles were compiled and compared with the optimum temperature of each species (Table 1). The table also highlights the adaptability of Vibrio spp. to a broad range of temperatures outside their optimum temperature.
Vibrio spp. That Are Negatively Impacted by the Rise in Temperature
Initial screening of selected articles showed that an increase in temperature led to a decreased growth of Vibrio spp. in very few articles (n= 7/111). Vibrio spp. that were negatively affected by the increase in temperature was V. harveyi, V. Marinus, V. salmonicida, V. shiloi, V. tasmaniensis, and V. vulnificus. These species were either psychrophilic bacteria (cold-loving) or were cultured under extremely unfavourable conditions. V. harveyi was cultured in seawater at 30°C (optimum 26°C) and under carbon limitation and showed a reduction in culturable cells and cell size. The rod-shaped morphology also changed to a coccoid-like cell (Montánchez et al., 2019). The second species was V. Marinus which showed that its viability and protein synthesis was higher at 15 than 22 and 25°C (Cooper and Morita, 1972). At 20°C, the synthesis of DNA and RNA in V. Marinus was also inhibited, while the expression of enzymes such as malic dehydrogenase, which is part of a citric acid cycle, was deregulated (Morita and Albright, 1968). V. Marinus optimum growth was reported to be 15°C in a previous study with a very short generation time of just 1 hr at 15°C (Morita and Albright, 1968) or 18°C for ATCC 1538. Growth of V. salmonicida at 17 and 20°C was low, while growth was optimum at temperatures ≤15°C (on agar). However, for V. salmonicida, optimum growth occurred at 15°C (Colquhoun et al., 2002) and for ATCC 1538. VBNC state was induced in V. shiloi (optimum temperature 26-30°C) when cultured at 20°C, while in terms of morphology, cell lysis and curling were also observed (Kushmaro et al., 2001; Vattakaven et al., 2006). VBNC state was also induced in V. tasmaniensis (optimum temperature 28°C) when cultured at 20°C after 157 days due to starvation (artificial seawater media). The rod shape of cells also changed more rapidly to coccoid and became completely coccoid on day 147 (Thompson et al., 2003; Vattakaven et al., 2006). V. shiloi, optimum growth was reported to be 30°C in a previous study (Kushmaro et al., 2001) or 26°C for ATCC BAA-91, while V. tasmaniensis (optimum growth 28°C) (Thompson et al., 2003). These two species were negatively impacted by the culture media (artificial seawater) which was nutritionally deficient (Vattakaven et al., 2006). V. vulnificus was negatively impacted by temperature when 2.20 ×106 CFU was recorded at 5°C compared to 2.09 ×106 CFU when cultured at 20°C, which was due to low pH and salinity (Hijarrubia et al., 1996). V. vulnificus 4965 were not able to grow at temperatures below 13°C or above 22°C when cultured at 10 ppt salinity. Survival at 21°C was also lower than at 14°C at a broad range of salinity (5–25 ppt). A mixture of 3 V. vulnificus strains (4793, 4832, and ATCC 27562) could also not grow well at 5 and 35°C (Kaspar and Tamplin, 1993). V. vulnificus, optimum growth was reported to be 36°C in a previous study (Kim et al., 2012) or 30°C for ATCC 27562, however, it was negatively impacted due to low pH and salinity (Hijarrubia et al., 1996).
Vibrio spp. That Were Not Significantly Impacted by the Rise in Temperature
Initial screening showed that no significant effect of temperature was observed over the tested range in 10/111 (9%) articles. Vibrio spp. that were not significantly affected by the increase in temperature over tested ranges were V. alginolyticus, V. cholerae, V. fischeri, V. parahaemolyticus, V. splendidus, and V. vulnificus. Most of the aforementioned species were heat-stable or thermophilic, while few were marine vibrios and were impacted mainly by salinity. Five strains of V. alginolyticus from various ecological sources were cultured at various temperatures (5-42°C), salinity (0.5-6% NaCl), and pH (4.5-12). The results showed extraordinary flexibility as almost all strains were able to grow in almost all conditions if given enough time (> 3 days) (Farid and Larsen, 1981). V. alginolyticus was also able to survive a wide range of temperatures (22 to 42°C) (Gu et al., 2016). Growth, collagenase activity, and protease activity in V. alginolyticus at 30 and 37°C were affected by oxygen and not temperature (Hare et al., 1981). Another study on V. alginolyticus cultured at a wide temperature range of 26-42°C reported that swarming was only observed when the salinity was suitable, indicating the salinity is the main factor affecting its growth, while salinity as low as 0.6% was enough for growth at 20-28°C (Ulitzur, 1975). V. cholerae’s growth was studied at 4, 25, and 35°C and 0.5 and 10% salinity. The results showed that the total count of cells was similar in the range of 4-35°C, while the physiological state (growth phase) and inoculum size had a bigger effect than temperature and salinity (Montilla et al., 1996). V. fischeri was able to grow at a very broad temperature range (8-34°C), moreover, temperature stress at the extremes (8 and 34°C) led to the evolution and higher growth fitness (Cohen et al., 2019). The Most Probable Number (MPN) of V. parahaemolyticus was not significantly different in the temperature range of 20-25°C, while high salinity was considered as the main factor in reducing the MPN (Larsen et al., 2015). V. parahaemolyticus was extremely tolerant to temperature and grew at temperatures as low as 8.3°C and as high as 45.4°C, while water activity was considered as the main factor in slowed growth (Miles et al., 1997). Growth of 2 V. splendidus strains cultured in several media at 4, 10, or 22°C, and under varying salinities was studied. The results showed that temperature did not significantly affect the growth, while salinity and nutrient content were determined as the main factors (Armada et al., 2003). MPN of V. vulnificus was not significantly different in the temperature range of 20-25°C, while high salinity was considered the main factor in reducing the MPN (Larsen et al., 2015). V. vulnificus isolated over 2 years with temperatures ranging from 6.7 to 35.6°C showed that growth was affected by salinity (28 ppt and above) rather than temperature (Motes et al., 1998).
Temperature Upshift Enhances the Growth of Vibrio spp.
Even though 38% showed that the rise of vibriosis is multifactorial, most of the articles 47% (n= 52/111) showed that the abundance of Vibrio spp. was positively impacted by the rise in temperature agreed with the consensus that Vibrio species grow better at higher temperatures. This indicated that the frequent occurrence of vibriosis might be due to environmental conditions such as global warming and higher sea surface temperature favouring the growth of Vibrio spp. Vibrio spp. that were positively affected by the increase in temperature were V. alginolyticus, V. anguillarum, V. cholera, V. coralliilyticus, V. costicola, V. harveyi, V. parahaemolyticus, V. salmonicida, V. shiloi, and V. vulnificus. Most of the articles in this section studied Vibrio species that grow better in higher temperatures.
First, V. cholerae (optimum growth at 37°C) showed maximum growth at higher temperatures (23-30°C), while it often entered the VBNC state at lower temperatures (4°C). The culturability was successfully recovered by re-incubation at 20-23°C (Ravel et al., 1995; Johnston and Brown, 2002). Temperature upshift to 37°C was reported to recover the culturability of V. cholerae from VBNC state by upregulating various metabolic pathways such as carbohydrate and phosphate metabolism (Debnath et al., 2019). A more detailed study on growth phases showed that temperature shortened the lag time at higher temperatures (22-30°C) compared to 15°C where no growth was recorded (Wang and Gu, 2005). Another study also showed that V. cholerae grew exponentially with the increase of temperature from 15 to 37°C (Kirschner et al., 2008). When cultured at various temperatures ranging from 10 to 30°C, V. cholerae also showed that temperature highly and linearly correlated with growth (Fu et al., 2013). The positive effect of temperature on V. cholerae was also reported in prevalence studies where higher abundance was observed at temperature >19°C compared to <19°C (Louis et al., 2003). Higher temperatures also significantly increased the prevalence of V. cholerae in blue crabs (Sullivan and Neigel, 2018). Pathogenic strains of V. cholerae such as V. cholerae O1 EI Tor also showed that temperature increase from 15 to 25°C increased biofilm formation which is related to growth (Stauder et al., 2010). V. cholerae O1 cultured at 5 to 30°C also showed that growth increased with the increase of temperature (Huq et al., 1984). The O1 strains were also able to survive temperatures as high as 65°C (Castro-Rosas and Escartin, 2005). A recent study on several pathogenic and non-pathogenic strains (O395, C6706, and N16961) subjected to various temperatures (4, 22 and 37°C) showed a significant reduction in growth and initiation of VBNC state at 4°C, while optimum growth was recorded at 22°C (Wu et al., 2016).
The second major species was V. coralliilyticus (optimum growth 30°C) where its growth increased when cultured at temperatures ranging from 16 to 30°C (Ben-Haim et al., 2003; Boroujerdi et al., 2009). Temperature upshift from 25 to 30°C also improved the growth of V. coralliilyticus (Frydenborg et al., 2014). The increased growth was correlated with prolonged exponential and stationary phases (Ben-Haim et al., 2003; Kimes et al., 2012). An increase in temperature from 24 and 27°C also changed the metabolomic profiles significantly (Boroujerdi et al., 2009). Higher temperatures also significantly increased the prevalence of environmental Vibrio spp. such as V. tubiashii which is closely related to V. coralliilyticus is associated with Pocillopora damicornis coral bleaching (Tout et al., 2015).
The third major thermophilic species was V. parahaemolyticus (optimum growth at 37°C) and were still able to grow at a wider temperature range (15 to 36°C) (Beuchat, 1974). The increase in temperature was reported to have increased growth in numerous studies. There was a direct relationship between growth rate and temperature when cultured at 13 to 36°C (Kim et al., 2012), 5-20°C (Mudoh et al., 2014) and 4 to 37°C (Han et al., 2016). It also grew better at 20°C compared to 4°C (Johnston and Brown, 2002). The increase in growth at higher temperatures (30.4 and 37°C) was correlated with a shorter generation time of 12-14 min (Horie et al., 1966), shorter lag time (Kim et al., 2012) and a faster growth rate (Fernandez-Piquer et al., 2011). For instance, V. parahaemolyticus grown at 3.6 to 30.4°C showed that the growth rate at 30.4°C (0.135 log10 CFU/h) was significantly higher compared to 3.6°C (0.015 log10 CFU/h) (Fernandez-Piquer et al., 2011). Optimum growth rate, shortest lag time, and maximum population density were all observed at 36°C (Kim et al., 2012). Studies on pathogenic and non-pathogenic strains also agreed on the positive effect of temperature on V. parahaemolyticus’s growth. Growth and metabolic activities of pathogenic V. parahaemolyticus were higher at 15-44°C, while at 10-20°C growth was up to 3 folds slower mainly due to prolonged lag-phase (Boonyawantang et al., 2012). V. parahaemolyticus O3: K6 cultured at a temperature range of 15-25°C showed a positive correlation with growth, with maximum growth recorded at 25°C (Nishina et al., 2004). Pathogenic and non-pathogenic V. parahaemolyticus were grown at various temperatures (10 and 30°C) and media (broth and oyster slurry). Those results showed higher temperature led to higher growth rates and shorter lag times (Yoon et al., 2008). The standard growth rate of pathogenic and non-pathogenic V. parahaemolyticus cultured at 4, 10, and 24°C also showed that maximum growth rate was observed in the highest temperature of 24°C (Cowan et al., 2019). Besides growth, the abundance and prevalence were also increased with the increase of temperature. For example, at temperatures ≥25°C, abundance was as high as 94%, while at temperatures <10°C, abundance was as low as 15% (Cook et al., 2002). A meta-analysis showed that V. parahaemolyticus was significantly and positively correlated with temperature in both water and raw oysters (Young et al., 2015). Recent studies supported these earlier findings where higher temperatures significantly increased the prevalence of V. parahaemolyticus in blue crabs (Sullivan and Neigel, 2018).
The last major species were V. vulnificus (optimum growth at 30°C), which in laboratory cultures, grew at a range of 13-42°C with its optimum growth recorded at 37°C (Kelly, 1982). V. vulnificus cultured at a temperature range of 5-15°C showed maximum growth at 15°C, while at lower temperatures, the initial inoculum was able to remain viable but not grow (Nishina et al., 2004). Another study also showed that a low temperature of 4°C was detrimental to the growth of V. vulnificus and initiated VBNC state, while culturability was possible at 10°C (Wolf and Oliver, 1992). Temperature upshift from 5 to 25°C also recovered the culturability of improved the growth of V. vulnificus even in starvation conditions (Biosca et al., 1996). Other studies also showed that it grew at higher temperatures (20 and 25°C) compared to lower temperatures (4 and 7.2°C) (Johnston and Brown, 2002; Limthammahisorn et al., 2008). Simultaneous increases in temperature and reduction in salinity (25°C/2.5% to 37°C/0.9%) increased the growth of V. vulnificus (Kim and Shin, 2011). Recent studies showed a similar trend where V. vulnificus cultured at a temperature range of 25-37°C showed a consistent increase in growth (Kim et al., 2016). The positive effect of temperature increase on growth was correlated to a higher growth rate and short lag time at 22 and 30°C compared to 15°C (Wang and Gu, 2005). Direct positive relationships between growth rate and temperature (11 to 36°C) were also reported, while optimum growth rate, shortest lag time, and maximum population density were all observed at 36°C (Kim et al., 2012). Studies on various types of V. vulnificus showed that the bacteria always grew better at higher temperatures. For example, wild and mutant strains grew faster at 37°C compared to 24°C by speeding up entry into the log phase by a couple of hours (Elgaml et al., 2014). Environmental and clinical strains cultured at different temperatures (24-37°C), pH (5.5-8.5) and iron concentrations (18-200 μM) showed that most strains grew better at 30 and 37°C compared to 24°C (Çam and Brinkmeyer, 2020). The abundance and prevalence of V. vulnificus also increased with the increase in temperature. A study showed that V. vulnificus made up 85.7 and 210.9 CFU/mL out of 5.7 ×106 CFU/mL and 2.1 ×107 CFU/mL total bacteria, respectively (Randa et al., 2004). Higher temperatures also significantly increased the prevalence of V. vulnificus in blue crabs (Sullivan and Neigel, 2018). Several other Vibrio spp. that were positively impacted by a rise in temperature was also reported. V. alginolyticus (optimum growth at 37°C) cultured at 37°C showed a short generation time of 12-14 min (Horie et al., 1966), which was also its optimum temperature (ATCC strain). V. anguillarum’s (optimum growth at 26°C) was cultured at different temperatures (15°C and 25°C) and iron concentrations and the results showed that growth and survival were significantly higher at 25°C (Lages et al., 2019). V. costicola (optimum growth at 30°C) grew at temperatures between 4 to 37°C, however, optimum growth occurred at 37°C (Adams and Russell, 1992). V. harveyi (optimum growth at 26°C) cultured at the temperature range of 12.9-27.1°C and salinity of 0.6 to 3.4% showed that an increase in temperature made the bacteria grow faster at higher temperatures where at 12.9, 15, and 27.1 C °C it took 144, 120 and 28 h to reach maximum growth (Zhou et al., 2012). V. salmonicida (optimum growth at 15°C) grew better at higher temperatures (15°C) compared to a lower temperature (7.5°C) regardless of salinity (1 or 2.5% NaCl) (Karlsen et al., 2008). Another study also reported a higher growth rate and short lag time at 22 and 30°C compared to 15°C (Wang and Gu, 2005). Vibrio AK-1 (later identified as V. shiloi) (optimum growth at 26-30°C) was cultured at a temperature range of 15-35°C and showed a continuous increase in growth rate which plateaued at 35°C (Kushmaro et al., 1998). When grown in seawater at a temperature range of 16-28°C, the results showed that growth reached 9.5 ×108 at 16°C, while at 28°C the growth increased and reached 4.5 ×109 (Israely et al., 2001). Growth was also significantly improved by temperature upshift from 25 to 30°C (Frydenborg et al., 2014). The increased growth of V. shiloi was correlated to faster entry into the log phase and a higher survival rate by extending the exponential growth period to 30°C compared to 23°C (Murali et al., 2010). Two studies on an unidentified Vibrio spp. called Vibrio ANT-300 (optimum growth <13°C) showed that increasing the temperature from 0 to either 7, 13, or 16°C improved growth, protein synthesis and integrity, and polyketides synthesis (Araki, 1991; Araki, 1992).
Temperature-Controlled Virulence of Vibrio spp.
Temperature-related virulence and pathogenesis of Vibrio spp. indicated that global warming favours the growth of Vibrio spp., however, for the bacteria to cause diseases virulence is an important factor. Hence, the articles in this review were screened again to summarize how high temperature induces virulence/pathogenicity. For instance, in the control group (22°C), Oceanospirillales was the most abundant order associated with P. damicornis coral, while at higher a temperature (31°C) Vibrionaceae order became dominant (Tout et al., 2015). An increase in temperature was found to induce pathogenicity/virulence in numerous Vibrio spp., namely V. alginolyticus, V. anguillarum, V. coralliilyticus, V. harveyi, V. parahaemolyticus, V. salmonicida, V. shiloi, and V. vulnificus. An increase in culture temperatures of V. alginolyticus from 22 to 37°C led to an increase in the production of exotoxins and the expression of virulence factors, however, at up to 42°C, the expression of exotoxins and virulence factors were inhibited (Gu et al., 2016). V. anguillarum RV22 showed differential expression of virulence genes at different temperatures (15°C and 25°C). At high temperatures (25°C), chemotaxis, motility, iron uptake, vanchrobactin, hemolysins, and outer membrane components were upregulated (Lages et al., 2019).
V. cholerae O1 EI Tor showed that when a temperature increased by 15 to 25°C, the virulence increased due to higher biofilm formation, increased the expression of e N-acetylglucosamine-binding protein A (GbpA) and mannose-sensitive haemagglutinin (MSHA) which increased its ability to colonize new host (Stauder et al., 2010). During recovery from the VBNC state, proteomic analysis of V. cholerae cultured at 37°C showed increased expression of 13 out of 19 proteins compared to VBNC (Debnath et al., 2019). The increase in temperature significantly increases the expression of extracellular protease in V. coralliilyticus which leads to higher coral (P. damicornis) bleaching and lysing (Ben-Haim et al., 2003). Metabolomics analysis of V. coralliilyticus under increased temperature leads to changes in osmoregulation and energy production pathways, while in terms of metabolites, major changes were in betaine, succinate, and glutamate (Boroujerdi et al., 2009). V. coralliilyticus exposed to increased temperature (above 25°C) lead to increased expression of virulence factors related to motility (e.g. Flagellar proteins), host degradation (e.g. proteases), resistance (e.g. efflux pumps), and upregulation of transcriptional factors (e.g. quorum sensing) (Kimes et al., 2012). The ability of V. cholerae Serovar O1 maximum growth of and attachment to copepods at 25 and 30°C was significantly better than at 5 or 15°C (Huq et al., 1984). Virulence gene expression such as adherence factors was found to occur at 17°C causing moralities in Haliotis tuberculata abalone due to V. harveyi infection (Travers et al., 2009). V. harveyi cultured at 28°C (optimum 26°C) led to 100% mortality in abalone Haliotis diversicolor (Jiang et al., 2013). V. harveyi cultured in seawater at 30°C (optimum 26°C) and under carbon limitation showed increased expression of virulence factors such as lytic enzymes (Montánchez et al., 2019). Clinical and virulent strains of V. parahaemolyticus increased with the increase in temperature (Turner et al., 2014). V. parahaemolyticus were cultured at 4 to 37°C result in significantly stronger growth, biofilm formation, quorum sensing (autoinducer-2 production), and exoprotease activity at a temperature of ≥ 25°C (Han et al., 2016). Novel outer membrane protein (OMP) called VS-P2 was expressed in V. salmonicida when cultured at 20°C compared to its optimum temperature of 15°C (Colquhoun et al., 2002). V. salmonicida cultured at higher temperature (15°C) showed higher expression of flagellin gene expression in comparison to a lower temperature (7.5°C) (Karlsen et al., 2008).
Vibrio AK-1 (later identified as V. shiloi) showed that the pathogen did not infect the coral Oculina patagonica and caused bleaching at 16°C, while at 20-29°C continuous increase in bleaching was observed (Kushmaro et al., 1998). V. shiloi was able to infect and bleach coral O. patagonica at 28°C but not at 16°C (Israely et al., 2001). V. vulnificus was found to increase the expression of rpoD, rpoS, luxS, smcR, crp and vvpE and the production of V. vulnificus protease (VVP) at 26°C, while at 37°C it increases the expression of rpoN, vvhA, and luxT and production of V. vulnificus hemolysin (VVH) (Elgaml et al., 2014). An increase in culture temperature of V. vulnificus showed that the expression of virulence factor siderophore increased (Turner et al., 2014). V. vulnificus cultured at a temperature range of 25-37°C showed a consistent increase in growth as well as expression of virulence regulators such as cyclic AMP receptor protein and vulnibactin receptor (Kim et al., 2016). A combined increase in temperature and reduction in salinity (25°C/2.5% to 37 °C/0.9%) increased the growth and expression of Metalloprotease VvpE in V. vulnificus which caused haemorrhage, necrosis, and edema (Kim et al., 2012). V. shiloi grown at 23 and 30°C showed higher activity of a probable virulence factor (Mn-SOD) at 30°C (Murali et al., 2010).
The low temperature was also found to induce virulence/pathogenicity in Vibrio spp. as reported in a few studies. V. anguillarum RV22 showed high virulence at 15°C despite its optimal temperature for growth was 25°C, while expression of mediated catalase activity (oxyR) and catalase gene (katG) were upregulated at 4°C (Lages et al., 2019). V. Marinus (optimum temperature 15-18°C) was reported to have a very short generation time of just 1 hr at 15°C (Horie et al., 1966). Coldwater vibriosis outbreaks associated with V. salmonicida occur at 3-10°C. In the laboratory, Atlantic salmon’s smolts were challenged with 1 ×107 ml-1 and cultured at 10°C (optimum 15°C) leading to ≈85% mortality (Colquhoun et al., 2002). V. salmonicida’s growth, biofilm formation, iron sequestration, litR expression were higher at a lower temperature (8-12°C) compared to a higher temperature (14-16°C) (Hansen et al., 2014). V. splendidus cultured at 10°C (optimum 26°C) upregulated numerous genes associated with virulence, adhesion, and quorum sensing (Liu et al., 2016). V. vulnificus were cultured at 24°C and produced up to 3 times more biofilm than at 30 or 37°C (Çam and Brinkmeyer, 2020).
Multifactorial Aspects of Vibrio spp. Growth and Pathogenicity
In the initial screening, the relationship between the increase in temperature and the growth of Vibrio spp. was shown to be complex or multifactorial in 38% of the articles (Figure 3). Hence, these factors affected the generally direct and positive correlation between temperature and Vibrio spp. growth was compiled. The fact that 38% of the articles showed the relationship between Vibrio spp. growth and increase in temperature are not linear but rather multifactorial justified the review. In summary, factors besides temperatures that affected the growth and survival of Vibrio spp. were the compounding/interactions of various parameters (n=25 articles), genotype/phenotype (n=24 articles), ecological (n=14 articles), salinity/water activity (n=13 articles), pH (n=4 articles). This showed that the species or strain was the most influential single factor that affected the direct relationship between temperature and growth of Vibrio spp. Other studies (n=14 articles) reported several other parameters such as chemical oxygen demand, dissolved organic matter, geographical location, growth phase, inoculum size, iron availability, media, nutrient, and oxygen availability.
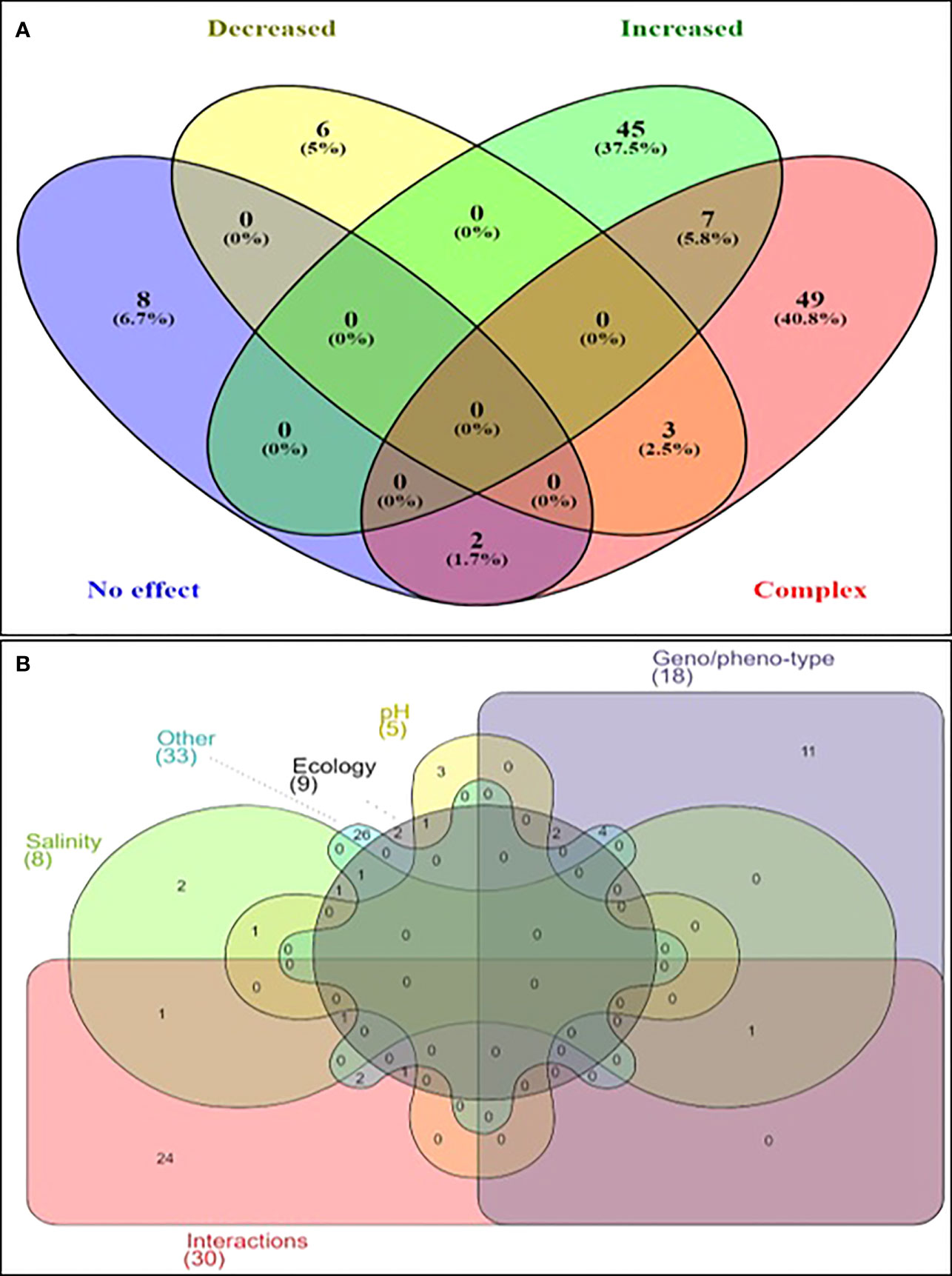
Figure 3 Venn Diagram illustrating the effect of temperature on Vibrio spp. (A) Effect of temperature on upshift on the growth of Vibrio spp. (B) Multifactorial parameters affecting the growth of Vibrio spp. under elevated temperatures.
The first factor was the compounding effects and interactions between various genetics and physicochemical parameters. Few studies reported that multiple factors affected the growth where a certain trend in interactions was not determined. For example, V. cholerae was significantly affected by several factors such as strain (O1 strain), presence of higher temperature, favourable ecological conditions (grow in oyster Crassostrea virginica), preferred lower salinity, distance from the river mouth, and enrichment method (DePaola and Hwang, 1995). V. cholerae abundance at higher temperatures (>19°C) was further compounded by the presence of plankton in the culture (Louis et al., 2003). The abundance of V. cholerae in oysters (C. virginica) was significantly affected by strain (O1 or non-O1), site, salinity, and season as well as their interactions (Chávez et al., 2005). Besides temperatures, the growth of V. cholerae was significantly affected by the biomass of zooplankton and water conductivity (Kirschner et al., 2008). Three V. vulnificus (serovar E) strains were cultured in different media (MSWYE and MSWYEA) and at various salinities (0.3 to 3.8% NaCl). Temperature, salinity, the interaction between temperature and salinity, and capsule all affected the growth significantly (Marco-Noales et al., 1999). Recent studies also supported these earlier findings where an abundance of Vibrio spp. generally and certain species (V. aestuarianus, V. tasmaniensis, and V. crassostreae) were affected by various factors such as ecology (free-living or plankton associated), species, strain (pathogenic or non-pathogenic), and season (Lopez-Joven et al., 2018b). V. vulnificus cultured at 20 to 37°C also showed growth and virulence were dependent on multiple biotic and abiotic factors including the host (Hernández-Cabanyero et al., 2020). Interactions between temperature and ecology were the most common interactions reported. The positive effect of temperature on the growth of V. cholerae O1 was compounded with the presence of copepods, where attachment to copepods significantly improved the survival of V. cholerae (Huq et al., 1984). The abundance of V. cholerae, V. parahaemolyticus, and V. vulnificus was also significantly affected by the combined effect of temperature and copepod abundance (Turner et al., 2014). V. shiloi associated with coral bleaching was not able to survive the toxicity of oxygen radicals produced by symbiotic zooxanthellae microalgae at a lower temperature (23°C) compared to at 30°C (Murali et al., 2010). Several ecological V. vulnificus strains were cultured at 7 to 45°C showed that individually, long-term survival (>2 days) was observed at temperatures ranging from 13 to 22°C. When the 3 strains were mixed, long-term growth was sustainable at 12 and 20°C and lower salinities (10 ppt) (Kaspar and Tamplin, 1993). V. fischeri and V. logei are symbiont to several Sepiola affinis, S. intermedia, S. ligulata, and S. robusta were cultured in Sepiola spp. and laboratory cultures at 18 to 26°C. V. fischeri was able to dominate V. logei when the temperature was 26°C, while at 18°C, V. logei dominated which showed a strain-dependent outcome (Nishiguchi, 2000). Interactions between temperature and species/strain were also often reported. V. parahaemolyticus serotype O3:K6 (tdh and orf8 positive) cultured in Salmon fish (Oncorhynchus spp.) at 0-30°C, showed that the effect of temperature on growth was less predictable at a lower temperature (16-20°C) compared to a higher temperature (25-35°C) (Yang et al., 2009). Culturable Vibrio population in water samples from various sites (industrial, recreational, and protected) were maintained at different temperatures (22 to 37°C). The results showed a significant effect of temperature where at 37°C, only 6 Vibrio spp. were detected while at 22°C, 20 species were detected, which showed strain dependence. At 37°C, only V. alginolyticus (85.9%), V. campbelli (2%), V. cholerae (<1%), V. communis (<1%), V. harveyi (10.7%) and V. parahaemolyticus (<1%) (Tall et al., 2013). The abundance of clinical and virulent strains of V. parahaemolyticus increased with the increase in temperature and copepod, however, normal strains did not correlate with temperature (Turner et al., 2014). Pathogenic (tdh positive) and non-pathogenic V. parahaemolyticus were cultured at 4-28°C in Manila clam (Ruditapes philippinarum) and the results showed that lower temperatures (4 and 15°C) slowed the non-pathogenic strains (Lopez-Joven et al., 2018a). Vibrio spp. related infections were well correlated with temperature from 1992 to 2016, however, the occurrence of Vibrio infections in 2015 was not explained by the date. The 2015 incidence was regarded as the probable effect of strain (subtype) and its compounding effect on temperature (Galanis et al., 2020). Interaction between temperature and salinity was also reported to affect the growth of V. parahaemolyticus and V. cholerae. The abundance of V. parahaemolyticus growth at 20 or 37°C was significantly affected by interactions between temperature and salinity (0.05-0.5 M), while a combination of low temperature and low salinity was the least favourable for the bacteria (Gray and Muir, 1977). V. parahaemolyticus prevalence was significantly different between sampling sites and exposure time to certain temperatures and salinity (Sullivan and Neigel, 2018). Clinical and environmental strains of V. cholerae cultured at various temperatures (10, 15, 20, and 25°C) and salinities (5, 15, 25, and 35%) also showed there were interactions between temperature and salinity affected the growth (Singleton et al., 1982). V. costicola grew at temperatures between 4 to 37°C, however, optimum growth occurred at different salinities throughout the temperature range (Adams and Russell, 1992). Interaction between species/strain and site was also reported to affect the growth of V. vulnificus. V. vulnificus in Shellshock Oysters (C. virginica) harvested from various sites with a temperature range of 27.8 to 31°C and held at temperatures ranging from 24 to 28.3°C showed that the site significantly affected MPN count which ranged from 300 to 5100 MPN/g despite the small temperature variations (Cook, 1994). V. vulnificus prevalence was also significantly different between sampling sites and exposure time to certain temperatures and salinity (Sullivan and Neigel, 2018). Other less common interactions also affected the growth of Vibrio spp. were also reviewed. For instance, V. alginolyticus cultured at various temperatures (5-42°C), salinity (0.5-6% NaCl), and pH (4.5-12) showed that compounding extreme conditions (e.g. low pH and low salinity) inhibited its growth (Farid and Larsen, 1981). Interaction between salinity and species/strain was reported to affect the growth of V. anguillarum. A total of 9 serovars cultured at various temperatures (20-37°C) and salinity (0.86-4% NaCl) showed that strain type and salinity affected maximum growth and tolerance to high temperature (Guérin-Faublée et al., 1995). The growth stage/phase also affected V. cholerae O1 cultured at various temperatures (6-30°C), salinities (8-32 ppt), and media. The results showed that the high temperatures were beneficial only in the early stage of growth, while long-term survival at 18°C was better than 30°C (McCarthy, 1996). V. parahaemolyticus abundance in mussels was also affected by the interaction of salinity and chlorophyll-a, while abundance in water samples was affected by the combined effect of temperature and turbidity (Julie et al., 2010). TnphoA transposon V. vulnificus strains (different morphotypes) was cultured in Oysters (C. virginica) and using different media and temperatures (0.5 to 24°C). The results showed that the highest survival was observed at 5°C, hence, the higher temperature didn’t cause a higher V. vulnificus count. The bacteria could resist a wider range of temperatures on agar but not in the oyster, while inoculum size also affected the growth (Murphy and Oliver, 1992). V. vulnificus cultured in media with varying amounts of bacterioplankton (mainly Nodularia spumigena) and temperature (12, 18, or 25°C) showed interactions and the concentration of dissolved organic matter significantly affected their growth more than temperature (Louis et al., 2003). Growth of Vibrio spp. cultured in various media compositions and temperatures (12, 18, or 25°C) showed that maximum growth occurred at 18°C, while dissolved organic matter maximized their growth (Eiler et al., 2007). Non-marine Vibrio sp. Teb5a1 was able to tolerate a wide range of temperature (4 to 49°C) and salinity (0 to 100 g/L). Survival and growth depended on salinity and the ability to express various virulence factors related to osmotic stress, motility, and antibacterial agents (Gallardo et al., 2016).
The second most common factor was the genotype and phenotype of tested strains such as species, strain, and pathogenicity. Affected Vibrio species were V. alginolyticus, V. anguillarum, V. cholerae, V. fischeri, V. marinus, V. parahaemolyticus, V. salmonicida, and V. vulnificus. For example, V. alginolyticus and V. parahaemolyticus cultured at 24°C showed 2 clusters in each species, one with a short generation time (12-14 hrs) and one with a long generation time (20-25 min) (Horie et al., 1966). A total of 9 V. anguillarum serovars were cultured at various temperatures (20-37°C) and salinity (0.86-4% NaCl). The results showed that strain type affected maximum growth and tolerance to high temperature (Guérin-Faublée et al., 1995). V. cholerae cultured at 35 and 42°C showed that non-Ol serogroup strains did not grow at 42°C (DePaola et al., 1987). The abundance of V. cholerae serovars such as O395, C6706, and N16961 was also significantly different among those tested (Lipp et al., 2003; Wu et al., 2016). Several V. fischeri strains grown at various temperatures (12 to 32°C) and salinities (24, 32, and 38 ppt) showed that optimum growth conditions were different among strains. V. fischeri ES114 preferred low salinity while V. fischeri ET401 preferred higher salinity, while environmental strains (ES114 & ET401) showed higher adaptability compared to V. fischeri ATCC 7744 (Soto et al., 2009). A total of 3 V. parahaemolyticus (T-3765-1, SB04-422, and ATCC 17802) strains were cultured at various temperatures (-18 to 48°C) and under various conditions (e.g. water-ethylene glycol bath) and maintained in different media (fish homogenate or TSB) were mainly affected by strain type (Covert and Woodburn, 1972). Non-pathogenic V. cholera and V. parahaemolyticus grew faster than pathogenic strains (Yoon et al., 2008; Fu et al., 2013). V. parahaemolyticus cultured at 10 to 30°C and in different media (broth or slurry) showed that strain (pathogenic and non-pathogenic) and media both impacted the growth. Pathogenic strains showed longer lag time and lower specific growth rates when exposed to low temperature (10°C), while broth showed higher growth than slurry (Yoon et al., 2008). Three strains of V. parahaemolyticus were grown at various temperatures, salinity, pH, and the salt type and the results showed that salinity had the most effective in determining the survival of tested strains. Mutant strains and the expression of toxR and rpoS (VP2553) increased the resistance to extreme environmental conditions (Whitaker et al., 2010). Pathogenic V. parahaemolyticus (JL 223) isolated from Korean raw oysters (Crassostrea gigas) grew faster than ATCC strain V. parahaemolyticus 33844 (Kim et al., 2012). Strain and genotype significantly affected V. parahaemolyticus ability to grow at various temperatures and salinities (Liu et al., 2016). Pathogenic V. parahaemolyticus associated with Jinjiang oyster (Crassostrea rivularis) were more susceptible to lower temperatures (5 and 15°C) compared to non-pathogenic strains, while their growth was maximized at 25 and 35°C (Wang et al., 2018). More than 20 strains of V. salmonicida (wild, type, and genetically modified) were cultured at various temperatures (4-16°C). The results showed colony morphology and ideal temperature for growth and biofilm formation were different across strains. Wild-type strains preferred 12°C, while litR mutant strains preferred 8°C (Hansen et al., 2014). Wild and luxO mutant V. vulnificus were grown at 26 and 37°C and the mutant grew faster than the wild-type strain at both temperatures (Elgaml et al., 2014). Clinical strains of V. vulnificus consistently produced more biofilm at 24°C than environmental strains, while iron concentration increased biofilm formation regardless of strain source (Çam and Brinkmeyer, 2020). Cell type (translucent or opaque), growth phase (stationary or logarithmic) and media (modified seawater yeast extract or Heart Infusion Agar) affected the culturability and survival of V. vulnificus (Biosca et al., 1996). Environmental and clinical strains of V. vulnificus were cultured at different temperatures (24-37°C), pH (5.5-8.5), and iron concentration (18-200 μM). The results showed that pH affected environmental strains only (Çam and Brinkmeyer, 2020). Other studies investigated the effect of unidentified or unspecified species/strains. For instance, a total of 69 Vibrio spp. were studied and clustered into 7 clusters based on genotypic similarity. However, in terms of virulence, only cluster 1 showed virulence, while all 22 strains in that cluster were V. marinus (Benediktsdottir et al., 1998). In another study of 118 Vibrio spp. Psychrotrophic strains, the results showed there were 2 distinct ecotype strains, one with an optimum temperature of 15 to 25°C and the other 20 to 30°C. These 2 ecological groups also had distinct adenylate concentrations and energy charge patterns (Morii and Sarukawa, 2000). Growth of 69 Vibrio spp. At various temperatures (4 to 37°C) and salinities (0 to 8%) showed that different strains preferred different growth conditions (Benediktsdottir et al., 1998).
The third factor was the ecology surrounding tested strains such as V. cholerae, V. parahaemolyticus, V. fischeri, V. shiloi, and V. vulnificus. V. cholerae O1 cultured at various temperatures (6-30°C), salinities (8-32 ppt), and media showed that ecological competitions reduced the long-term survival of V. cholerae O1 strains compared to monoculture (McCarthy, 1996). V. cholerae abundance was affected by environmental conditions such as the presence of phytoplankton, which was positively correlated to growth (Lipp et al., 2003). Different V. cholerae strains colonizing or attached to shrimp carapace survived longer than strains cultured in solution (Castro-Rosas and Escartin, 2005). The presence of plankton in the culture increased the growth of both Serovar O1 and non-O1/non-O139 V. cholerae (Alam et al., 2006). The ability of V. fischeri to colonize and infect symbiont organisms was affected by flagella-based motility (Karlsen et al., 2008). Growth of V. parahaemolyticus on oyster slurry was slower compared to growth in broth regardless of the temperature (Yoon et al., 2008). V. shiloi was able to remain viable at 16 and 28°C in laboratory culture conditions. However, in ecological conditions, it did not survive 16°C in the presence of O. patagonica coral. This indicated that the coral was able to produce metabolites to kill the bacteria at 16°C, while morphological differences (size and presence of flagellum) were also observed between laboratory and ecological settings (Israely et al., 2001). V. vulnificus also showed the importance of ecological factors as it did not grow at temperatures below 20°C in its habitat, while in laboratory cultures, it grew at temperatures ranging from 13 to 42°C (Kelly, 1982). V. vulnificus is endogenous to Oysters (C. virginica) and behaved differently compared to laboratory-grown V. vulnificus and it could not compete with the microflora in and colonize the oyster host (Kaspar and Tamplin, 1993).
The fourth most common factor was the physicochemical parameters such as salinity and pH which affected the growth of V. anguillarum, V. marinus, V. parahaemolyticus, V. salmonicida, and V. vulnificus. A total of 9 V. anguillarum serovars were cultured at various temperatures (20-37°C) and salinity (0.86-4% NaCl). The results showed that salinity affected maximum growth and tolerance to high temperatures (Guérin-Faublée et al., 1995). Salinity also reduced the V. marinus resistance to temperature (Morita and Albright, 1968). V. parahaemolyticus strains (T-3765-1, SB04-422, and ATCC 17802) grown at various conditions (temperatures, salinity, additives, etc) showed that at certain salinities almost all strains could not grow (Covert and Woodburn, 1972). V. salmonicida was flagellated, more motile, and showed higher flagellin (flaA, flaB, flaC, flaD, flaE, and flaF) gene expression at higher salinity (2.5%) compared to low salinity (7.5%) (Karlsen et al., 2008). V. vulnificus was grown at salinities ranging from 0.5 to 10.5% NaCl concentration and showed that it was able to grow at 0.5 to 6.5% NaCl, while its optimum salinity was 1-2% (Kelly, 1982). In another study, V. vulnificus could not survive salinities greater than 25 ppt (Kaspar and Tamplin, 1993). Water activity which is related to salinity was also reported to affect growth in several studies. Water activity and salinity also affected lag period, growth rate, and total cell number of V. parahaemolyticus (Beuchat, 1974). Four V. parahaemolyticus strains cultured at 5–50°C and water activity (0.936–0.995) showed that growth was significantly affected by strain type with 38.349 showing the highest growth. The bacteria were water activity-dependent and also could not grow in upper and lower limits of temperature (< 10 and > 48°C) (Miles et al., 1997).
The fifth factor (pH) was less common compared to salinity/water activity but it significantly affected the growth of V. anguillarum, V. cholerae, V. parahaemolyticus, V. salmonicida, and V. vulnificus. V. salmonicida and V. vulnificus flourished at a very small pH range ( ± 2-3), V. cholera while tolerated pH ranging from 5.2 to 9.2 (Wang and Gu, 2005). V. anguillarum cultured at various temperature (0-30°C), salinity (0.5-4% NaCl), and pH (5-9) showed that it was only able to grow in certain ranges (e.g. pH 5.5- 8.5) (Olafsen et al., 1981). V. anguillarum cultured at various pH (6-9) showed that the bacteria could not grow at pH 6 (Larsen, 1984). Different V. cholerae strains survived longer in 1.5 pH than 2.5 (Castro-Rosas and Escartin, 2005). A total of 6 V. parahaemolyticus strains (107914, 8700 04:K11, 284-72, O, T-3765-1 03:K7 and 4750 02:K3) at various temperature (5 to 30°C) salinities (3 or 7%) showed that almost all strains were able to grow at all condition but at different pH (Beuchat, 1973).
Several other parameters that directly or indirectly affected the growth were also reported in reviewed articles. Yeast extract, peptone concentration, NaCl concentration, and oxygen availability significantly affected the growth of V. alginolyticus (Horie et al., 1966). Iron sequestration was also shown to be a key virulence factor in V. anguillarum and V. vulnificus (Colquhoun and Sørum, 2001). V. anguillarum subjected to iron limitation led to upregulated production of LPS, hemolysins, lysozyme, siderophores, and other virulence factors (Lages et al., 2019). Growth of V. anguillarum and V. salmonicida was found to depend on the form of media where results obtained in broth were slightly different (<10°C) than agar (Colquhoun et al., 2002). The ability of V. anguillarum and V. cholerae to colonize and infect symbiont organisms was affected by flagella-based motility (Karlsen et al., 2008). The physiological state (growth phase) had a greater effect than temperature and salinity on V. cholerae conversion from non-O1 to O1 serogroup (Montilla et al., 1996). V. cholerae cultured in media with varying amounts of bacterioplankton (mainly N. spumigena) and temperature (12, 18, or 25°C) showed that only the concentration of dissolved organic matter significantly affected their growth (Louis et al., 2003). V. cholerae prevalence was significantly different between sampling sites, while exposure time, temperature, and salinity also had effects (Sullivan and Neigel, 2018). V. cholera AN59 growth varied by up to 100-fold depending on initial inoculum size (2 to 106 CFU/ml–1) as well as the media used (ASW or spent ASW) (Debnath et al., 2019). Inoculum size and iron concentration also significantly affected growth, cell density, and siderophore production in V. salmonicida 1193/87 (Colquhoun and Sørum, 2001). Chemical oxygen demand (COD) tested at 5 to 40 mg l-1 significantly and positively correlated to V. cholera growth by maximizing growth rate and cell density (Fu et al., 2013). Starvation was also shown to induce VBNC state in V. fischeri and V. harveyi at the temperature between 4°C and 22°C (Vattakaven et al., 2006). V. coralliilyticus infections depended on many factors such as the simultaneous expression of several virulence factors (e.g. flagellar, secretion, and quorum sensing) (Kimes et al., 2012). Total Vibrio and total V. parahaemolyticus abundance in water, shrimp, fish, and seagrass was significantly affected by the sampling site despite the site having similar temperatures (27 and 31°C) and salinity (28 to 34%) (Kaneko and Colwell, 1974). The prevalence of pathogenic (tdh+) V. parahaemolyticus was higher in enriched media compared to direct plating (Cook et al., 2002). Even though V. parahaemolyticus generally preferred higher temperature, the positive effect of temperature up-shift was eliminated by the growth phase where only Vibrio spp. in the stationary phase preferred the temperature up-shift (Nishino and Kogure, 2003). Environmental and clinical strains of V. vulnificus were cultured at different temperatures (24-37°C), pH (5.5-8.5), and iron concentration (18-200 μM) showed that iron availability and concentration were positively correlated to biofilm formation (Çam and Brinkmeyer, 2020).
Extremophiles Vibrio spp. (grow at ≤10°C or/and ≥35°C)
Several Vibrio species were found to be able to grow in high temperatures (≥35°C) and could be species of concern in this global warming era. Hence, these species were compiled for future reference and policymaking. These species were V. alginolyticus, V. cholerae, V. fischeri, V. marinus, V. parahaemolyticus, and V. vulnificus. Among these, V. cholerae, V. parahaemolyticus, and V. vulnificus were also able to grow ≤10°C. Other cold-resistant that could grow at ≤10°C species were V. anguillarum, V. fischeri, V. salmonicida, V. shiloi, V. splendidus, and V. tasmaniensis (Figure 3). One study reported that V. alginolyticus, V. anguillarum, V. damsela, V. fluvialis, V. harveyi, V. ordalii, V. parahaemolyticus, V. splendidus, and V. vulnificus were also able to grow at temperature ≥37°C, however, growth curves were not provided (Benediktsdottir et al., 1998) (Figure 4). The overall findings of this systematic literature review are summarized in Supplementary Figure 1.
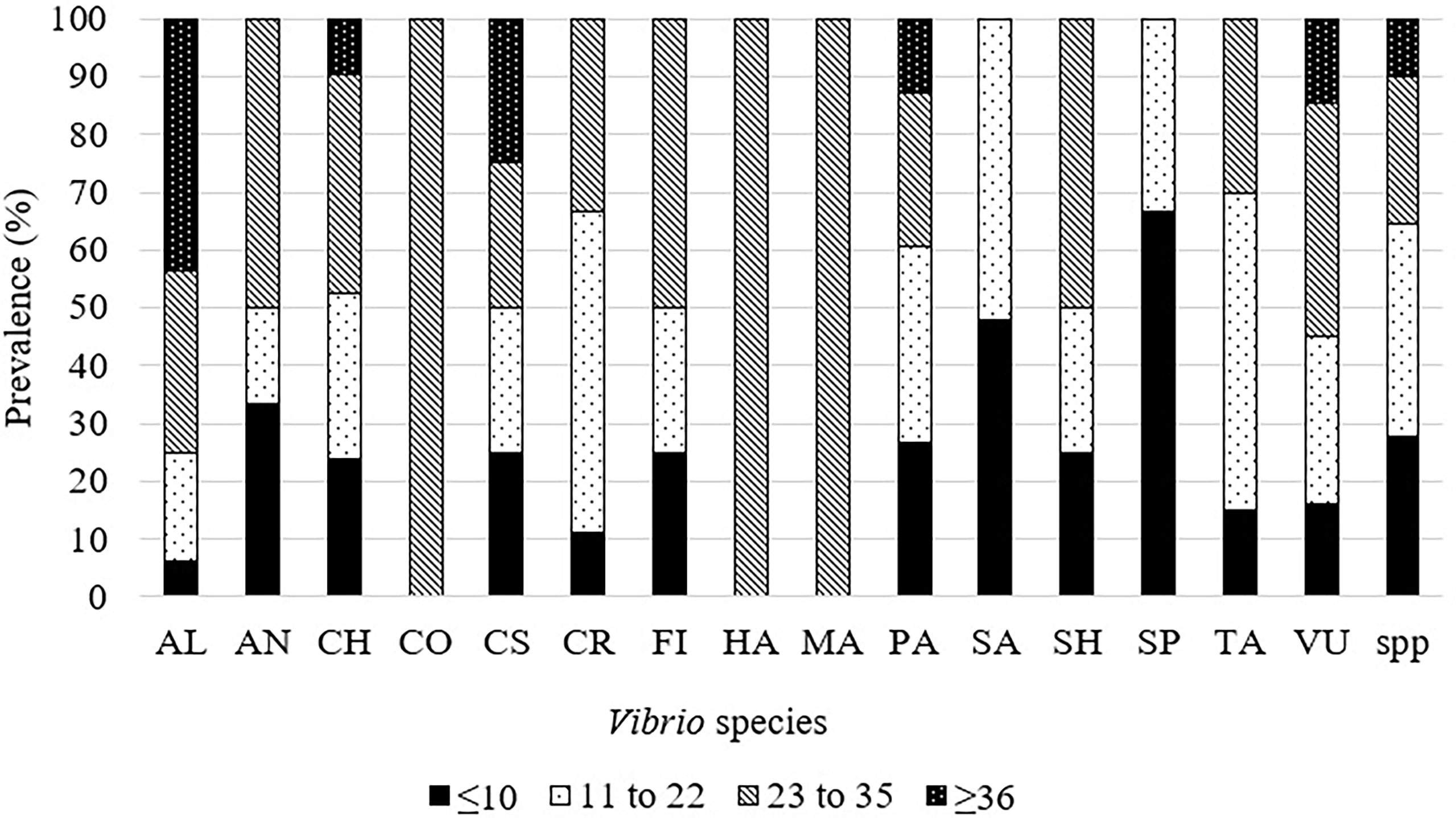
Figure 4 Prevalence of Vibrio spp. at various temperatures in reviewed articles. AL, V. alginolyticus; AN, V. anguillarum; CH, V. cholerae; CO, V. coralliilyticus; CS: V. costicola, CR: V. crassostreae, FI: V. fischeri, HA: V. harveyi, MA: V. marinus, PA: V. parahaemolyticus, SA: V. salmonicida, SH: V. shiloii, SP: V. splendidus, TA, V. tasmaniensis; VU, V. vulnificus; spp.:Unidentified Vibrio species.
Therapeutic Strategies for Vibriosis-Related Infections
Vibrio spp. have been the causative agents for vibriosis diseases such as cholera and food poisoning in the past. These infections are expected to be a major disease in the future as well. Hence, an overview of therapeutic strategies against Vibrio spp. is discussed below. There are several antimicrobial agents that can inhibit or kill Vibrio spp. such as antibiotics. In a 2015 survey, out of 8056 cases of cholera and other vibrio illness in humans, 5243 (~65%) were prescribed antibiotics. Quinolones accounted for 56% of these antibiotic prescriptions and were the only class that significantly reduced mortality. Other antibiotics prescribed included cephalosporins and tetracyclines, while penicillins were the least effective (Wong et al., 2015). A 10 years retrospective study on humans reported that cephalosporins and tetracyclines were used initially to treat the potentially fatal V. vulnificus, while additional antibiotics were prescribed based on antibiotic resistance patterns. Another study recommended doxycycline combined with 3rd generation cephalosporins (Cai et al., 2021). Bacteriocins are ribosomally synthesized peptides secreted by various bacterial strains to kill closely related species (Sheikh et al., 2022). Numerous bacteriocins and bacteriocins-like-inhibitory substances were found to inhibit Vibrio spp. while presenting a lower risk of resistance. Recently, bacteriocins isolated from Vibrio spp. (called vibriocin) showed effective and narrow-spectrum activity against numerous Vibrio spp. More critically, vibriocin was effective against pathogenic strains such as V. alginolyticus, V. harveyi, and V. parahaemolyticus (Sheikh et al., 2022). Bacteriocin isolated from tiger shrimp Penaeus monodon was also effective against pathogenic V. alginolyticus (Feliatra et al., 2018). Another antimicrobial peptide produced by Bacillus paralicheniformis inhibited V. harveyi (Choyam et al., 2021).
Phage therapy is another vibriosis control approach with a narrow spectrum activity that was successful, particularly for the aquaculture industry. Previous studies reported the efficacy of vibriophages against key vibrio pathogens such as V. parahaemolyticus, V. campbellii, and V. alginolyticus. Vibriophages have the ability to selectively recognize and inhibit Vibrio species or even certain strains (Dubey et al., 2021; Lomelí-Ortega et al., 2021). Vibriophage KVP40 was also found to infect numerous Vibrio spp. including V. anguillarum, V. parahaemolyticus, V. harveyi, and V. cholerae, while the mechanism of action was reported to via attacking the outer membrane protein K (OmpK) (Tan et al., 2015).Inhibition of quorum sensing (QS) which is critical in coordinating the expression of certain genes is another therapeutic approache that is not well explored. Inhibiting these cell-to-cell communications between bacteria was found to be key in controlling vibriosis. For example, furanones were proposed as a promising treatment for luminescent vibriosis in aquaculture (Huzmi et al., 2019).
The use of natural products in aquaculture as antimicrobial agents is also becoming increasingly popular because they are more eco-friendly than conventional antimicrobials which are a threat to animals, humans, and the ecosystem health (Schar et al., 2020). Natural products such as postbiotics (mainly Lactic acid) and essential oils (eugenol and thymol) have also been found to be effective against Vibrio spp. For example, eugenol and thymol inhibited the growth of V. parahaemolyticus strains (KCTC 2729, ATCC 17802, ATCC 27969 and ATCC 33844) and V. harveyi ATCC 1118 (Toushik et al., 2022).
Despite Vibrio spp. being a significant threat to animals and humans, there are numerous therapeutic strategies for vibriosis-related infections. However, more research is needed to optimize these treatments for humans and animals, as well as for each species.
Conclusion
Vibrio spp. were able to grow at a wide range of environmental conditions such as temperatures, salinities, and pH which could lead to its dominance in the environment. However, the review hypothesized that only certain thermostable Vibrio spp. are positively impacted by the increase in temperature. This hypothesis was validated by reviews as 47% of the articles reported that the increase in growth was due to an increase in temperature, while 38% reported that the increase in growth was multifactorial. Reduced growth (6%) and no effect (9%) were also reported. The temperature outside its optimum ranges also caused Vibrio spp. to evolve and change its metabolic activities, increase the expression of virulence genes and shorten generation time. These factors improved the ability in colonizing new hosts such as phytoplanktons, zooplanktons algae, crustaceans, and fish. Vibrios were also able to survive starvation by going into VBNC state for very long periods (>150 days) and then recovering their growth when favourable conditions such as high temperature occurred. In terms of research questions, the first research question in this review article was whether all Vibrio spp. flourish and become virulent at higher temperatures. Based on the 15 species in the reviewed articles, the answer is no. Several species were not able to grow at ≥ 37°C, namely, V. salmonicida, V. anguillarum, V. splendidus, V. coralliilyticus, V. crassostreae, V. fischeri, V. harveyi, V. marinus, V. shiloii and V. tasmaniensis. However, these species were found to be pathogenic at lower temperatures. The second research question aimed at identifying which Vibrio spp. pose a significant threat to humans and animals in the era of global warming. Vibrio spp. that grew well at ≥ 37°C were V. alginolyticus, V. cholerae, V. costicola, V. parahaemolyticus and VU: V. vulnificus. Based on the pathogenicity of these Vibrio spp., the species that pose the highest risk to humans and animals are V. alginolyticus, V. cholerae, V. parahaemolyticus and V. vulnificus. Efforts to combat global warming are needed and being carried out for long-term impact, however, novel antibiotics that can inhibit these species are urgently needed for short-term treatment of diseases caused by these pathogens, especially for humans and fish.
Author Contributions
HS: Data collection, conceptualization and original draft writing. MN: conceptualization and final draft writing. FA: Data collection. LA: original draft writing. NM: formal analysis. JK: final draft writing. KN: Funding acquisition. All authors contributed to the article and approved the submitted version.
Funding
This work was funded by the Ministry of Higher Education (MOHE), Malaysia under Higher Institution Centre of Excellence (HICoE), Institute of Tropical Aquaculture and Fisheries (AKUATROP) program [Vot. No. 63933, JPT.S(BPKI) 2000/016/018/015 Jld.3 (23) and Vot. No. 56050, UMT/PPPI/2-2/5 Jld.2 (24)]. All authors would like to dedicate special gratitude to whoever involves and participated in this research project.
Conflict of Interest
The authors declare that the research was conducted in the absence of any commercial or financial relationships that could be construed as a potential conflict of interest.
Publisher’s Note
All claims expressed in this article are solely those of the authors and do not necessarily represent those of their affiliated organizations, or those of the publisher, the editors and the reviewers. Any product that may be evaluated in this article, or claim that may be made by its manufacturer, is not guaranteed or endorsed by the publisher.
Acknowledgments
The authors would like to thank the Centre for Research and Innovation Management (CRIM) for their assistance in gathering the resources needed in writing this review.
Supplementary Material
The Supplementary Material for this article can be found online at: https://www.frontiersin.org/articles/10.3389/fmars.2022.959830/full#supplementary-material
References
Adams R. L., Russell N. J. (1992). Interactive Effects of Salt Concentration and Temperature on Growth and Lipid Composition in the Moderately Halophilic Bacterium Vibrio costicola. Can. J. Microbiol. 38, 823–827. doi: 10.1139/m92-134
Alam M., Sadique A., Nur-A-Hasan, Bhuiyan N. A., Nair G. B., Siddique A., et al. (2006). Effect of Transport at Ambient Temperature on Detection and Isolation of Vibrio cholerae From Environmental Samples. Appl. Environ. Microbiol. 72, 2185–2190. doi: 10.1128/AEM.72.3.2185-2190.2006
Araki T. (1991). The Effect of Temperature Shifts on Protein Synthesis by the Psychrophilic Bacterium Vibrio sp. strain. ANT-300. Microbiol. 137, 817–826. doi: 10.1099/00221287-137-4-817
Araki T. (1992). An Analysis of the Effect of Changes in Growth Temperature on Proteolysis In Vivo in the Psychrophilic Bacterium Vibrio sp. strain. ANT-300. Microbiol. 138, 2075–2082. doi: 10.1099/00221287-138-10-2075
Armada S. P., Farto R., Pérez M. J., Nieto T. P. (2003). Effect of Temperature, Salinity and Nutrient Content on the Survival Responses of Vibrio splendidus Biotype I. Microbiology 149, 369–375. doi: 10.1099/mic.0.25574-0
Baker-Austin C., Oliver J. D., Alam M., Ali A., Waldor M. K., Qadri F., et al. (2018). Vibrio spp. Infections. Nat. Rev. Dis. Primers 4, 1–19. doi: 10.1038/s41572-018-0005-8
Benediktsdottir E., Helgason S., Sigurjónsdóttir H. (1998). Vibrio spp.Isolated From Salmonids With Shallow Skin Lesions and Reared at Low Temperature. J. fish. Dis. 21, 19–28. doi: 10.1046/j.1365-2761.1998.00065.x
Ben-Haim Y., Zicherman-Keren M., Rosenberg E. (2003). Temperature-Regulated Bleaching and Lysis of the Coral Pocillopora Damicornis by the Novel Pathogen Vibrio coralliilyticus. Appl. Environ. Microbiol. 69, 4236–4242. doi: 10.1128/AEM.69.7.4236-4242.2003
Beuchat L. R. (1973). Interacting Effects of Ph, Temperature, and Salt Concentration on Growth and Survival of Vibrio parahaemolyticus. Appl. Microbiol. 25, 844–846. doi: 10.1128/am.25.5.844-846.1973
Beuchat L. (1974). Combined Effects of Water Activity, Solute, and Temperature on the Growth of Vibrio parahaemolyticus. Appl. Microbiol. 27, 1075–1080. doi: 10.1128/am.27.6.1075-1080.1974
Biosca E. G., Amaro C., Marco-Noales E., Oliver J. D. (1996). Effect of Low Temperature on Starvation-Survival of the Eel Pathogen Vibrio vulnificus Biotype 2. Appl. Environ. Microbiol. 62, 450–455. doi: 10.1128/aem.62.2.450-455.1996
Boonyawantang A., Mahakarnchanakul W., Rachtanapun C., Boonsupthip W. (2012). Behavior of Pathogenic Vibrio parahaemolyticus in Prawn in Response to Temperature in Laboratory and Factory. Food Control. 26, 479–485. doi: 10.1016/j.foodcont.2012.02.009
Boroujerdi A. F., Vizcaino M. I., Meyers A., Pollock E. C., Huynh S. L., Schock T. B., et al. (2009). NMR-Based Microbial Metabolomics and the Temperature-Dependent Coral Pathogen Vibrio coralliilyticus. Environ. Sci. Technol. 43, 7658–7664. doi: 10.1021/es901675w
Burks D. J., Norris S., Kauffman K. M., Joy A., Arevalo P., Azad R. K., Wildschutte H., & (2017). Environmental Vibrios Represent a Source of Antagonistic Compounds that Inhibit Pathogenic Vibrio cholerae and Vibrio parahaemolyticus strains. Microbiologyopen, 6(5), e00504. doi: 10.1002/mbo3.504
Cai R., Zhen M., Guan Z., Li M., Liao Q., Liu X., et al. (2021). New Atypical Manifestations and Prognostic Factors of Vibrio vulnificus Infection: A 10-Year Retrospective Study. Japanese J. Infect. Dis, 74(6), 549–553. JJID-2020. doi: 10.7883/yoken.JJID.2020.843s
Çam S., Brinkmeyer R. (2020). The Effects of Temperature, Ph, and Iron on Biofilm Formation by Clinical Versus Environmental Strains of Vibrio vulnificus. Folia microbiol. 65, 557–566. doi: 10.1007/s12223-019-00761-9
Castro-Rosas J., Escartin E. (2005). Increased Tolerance of Vibrio cholerae O1 to Temperature, Ph, or Drying Associated With Colonization of Shrimp Carapaces. Int. J. Food Microbiol. 102, 195–201. doi: 10.1016/j.ijfoodmicro.2004.12.015
Chávez M. D. R. C., Sedas V. P., Borunda E. O., Reynoso F. L. (2005). Influence of Water Temperature and Salinity on Seasonal Occurrences of Vibrio cholerae and Enteric Bacteria in Oyster-Producing Areas of Veracruz, México. Mar. pollut. Bull. 50, 1641–1648. doi: 10.1016/j.marpolbul.2005.06.036
Chowdhury F. R., Nur Z., Hassan N., Von Seidlein L., Dunachie S. (2017). Pandemics, Pathogenicity and Changing Molecular Epidemiology of Cholera in the Era of Global Warming. Ann. Clin. Microbiol. Antimicrob. 16, 1–6. doi: 10.1186/s12941-017-0185-1
Choyam S., Jain P. M., Kammara R. (2021). Characterization of a Potent New-Generation Antimicrobial Peptide of Bacillus. Front. Microbiol. 12, 710741. doi: 10.3389/fmicb.2021.710741
Cohen M. L., Mashanova E. V., Rosen N. M., Soto W. (2019). Adaptation to Temperature Stress by Vibrio fischeri Facilitates This Microbe’s Symbiosis With the Hawaiian Bobtail Squid (Euprymna Scolopes). Evolution 73, 1885–1897. doi: 10.1111/evo.13819
Colquhoun D., Alvheim K., Dommarsnes K., Syvertsen C., Sørum H. (2002). Relevance of Incubation Temperature for Vibrio salmonicida Vaccine Production. J. Appl. Microbiol. 92, 1087–1096. doi: 10.1046/j.1365-2672.2002.01643.x
Colquhoun D., Sørum H. (2001). Temperature Dependent Siderophore Production in Vibrio salmonicida. Microbial. pathogen. 31, 213–219. doi: 10.1006/mpat.2001.0464
Cook D. W. (1994). Effect of Time and Temperature on Multiplication of Vibrio vulnificus in Postharvest Gulf Coast Shellstock Oysters. Appl. Environ. Microbiol. 60, 3483–3484. doi: 10.1128/aem.60.9.3483-3484.1994
Cook D. W., Bowers J. C., Depaola A. (2002). Density of Total and Pathogenic (Tdh+) Vibrio parahaemolyticus in Atlantic and Gulf Coast Molluscan Shellfish at Harvest. J. Food Prot. 65, 1873–1880. doi: 10.4315/0362-028X-65.12.1873
Cooper M. F., Morita R. Y. (1972). Interaction of Salinity and Temperature on Net Protein Synthesis and Viability of Vibrio marinus 1, 2.Limnology and Oceanography 17, 556–565. doi: 10.4319/lo.1972.17.4.0556
Covert D., Woodburn M. (1972). Relationships of Temperature and Sodium Chloride Concentration to the Survival of Vibrio parahaemolyticus in Broth and Fish Homogenate. Appl. Microbiol. 23, 321–325. doi: 10.1128/am.23.2.321-325.1972
Cowan M., Finston T., Marshall R., de la Bastide P. Y. (2019). Post-Harvest Temperature Regimes for Pacific Oysters (Crassostrea Gigas) and Growth Response of Vibrio parahaemolyticus. Aquacult. Res. 50, 3471–3476. doi: 10.1111/are.14289
Debnath A., Mizuno T., Miyoshi S.-I. (2019). Comparative Proteomic Analysis to Characterize Temperature-Induced Viable But non-Culturable and Resuscitation States in Vibrio cholerae. Microbiology 165, 737–746. doi: 10.1099/mic.0.000798
de O Santos E., Alves N., Dias G. M., Mazotto A. M., Vermelho A., Vora G. J.& , Thompson F. L., , (2011). Genomic and proteomic analyses of the coral pathogen Vibrio coralliilyticus reveal a diverse virulence repertoire. The ISME journal, 5(9), 1471–1483. doi: 10.1038/ismej.2011.19
Depaola A., Hwang G.-C. (1995). Effect of Dilution, Incubation Time, and Temperature of Enrichment on Cultural and PCR Detection of Vibrio cholerae Obtained From the Oyster Crassostrea Virginica. Mol. Cell. Probes 9, 75–81. doi: 10.1016/S0890-8508(95)80031-X
DePaola A., Kaysner C., Mcphearson R. (1987). Elevated Temperature Method for Recovery of Vibrio cholerae From Oysters (Crassostrea Gigas). Appl. Environ. Microbiol. 53, 1181–1182. doi: 10.1128/aem.53.5.1181-1182.1987
Dubey S., Singh A., Kumar B. T., Singh N. K., Tyagi A. (2021). Isolation and Characterization of Bacteriophages From Inland Saline Aquaculture Environments to Control Vibrio parahaemolyticus Contamination in Shrimp. Indian J. Microbiol. 61 (2), 212–217. doi: 10.1007/s12088-021-00934-6
Eiler A., Gonzalez-Rey C., Allen S., Bertilsson S. (2007). Growth Response of Vibrio cholerae and Other Vibrio spp. To Cyanobacterial Dissolved Organic Matter and Temperature in Brackish Water. FEMS Microbiol. Ecol. 60, 411–418. doi: 10.1111/j.1574-6941.2007.00303.x
Elgaml A., Higaki K., Miyoshi S.-I. (2014). Effects of Temperature, Growth Phase and luxO-Disruption on Regulation Systems of Toxin Production in Vibrio vulnificus Strain L-180, a Human Clinical Isolate. World J. Microbiol. Biotechnol. 30, 681–691. doi: 10.1007/s11274-013-1501-3
Farid A., Larsen J. (1981). Growth of Vibrio alginolyticus: Interacting Effects on Ph, Temperature, Salt Concentration, and Incubation Time. Zentralblatt Für Bakteriologie Mikrobiologie Und Hygiene: I. Abt. Originale C: Allgemeine, Angewandte Und Ökologische Mikrobiologie2, 68–75. doi: 10.1016/S0721-9571(81)80019-1
Feliatra F., Muchlisin Z. A., Teruna H. Y., Utamy W. R., Nursyirwani N., Dahliaty A. (2018). Potential of Bacteriocins Produced by Probiotic Bacteria Isolated From Tiger Shrimp and Prawns as Antibacterial to Vibrio, Pseudomonas, and Aeromonas Species on Fish. F1000research 7, 415–415. doi: 10.12688/f1000research.13958.1
Fernandez-Piquer J., Bowman J. P., Ross T., Tamplin M. L. (2011). Predictive Models for the Effect of Storage Temperature on Vibrio parahaemolyticusViability and Counts of Total Viable Bacteria in Pacific Oysters (Crassostrea Gigas). Appl. Environ. Microbiol. 77, 8687–8695. doi: 10.1128/AEM.05568-11
Fischer K., Nijdam A., Holmström M., Petrini P., Ljung R., van der Schouw Y., et al. (2016). Evaluating Outcome of Prophylaxis in Haemophilia: Objective and Self-Reported Instruments Should be Combined. Haemophilia 22, e80–e86. doi: 10.1111/hae.12901
Froelich B., Phippen B., Fowler P., Noble R., Oliver J. (2017). Differences in Abundances of Total VibrioSpp., V. pulnificus and V. parahaemolyticus in Clams and Oysters in North Carolina. Appl. Environ. Microbiol. 83, e02265–e02216. doi: 10.1128/AEM.02265-16
Frydenborg B. R., Krediet C. J., Teplitski M., Ritchie K. B. (2014). Temperature-Dependent Inhibition of Opportunistic Vibrio pathogens by Native Coral Commensal Bacteria. Microbial. Ecol. 67, 392–401. doi: 10.1007/s00248-013-0334-9
Fu S., Shen J., Liu Y., Chen K. (2013). A Predictive Model of Vibrio choleraefor Combined Temperature and Organic Nutrient in Aquatic Environments. J. Appl. Microbiol. 114, 574–585. doi: 10.1111/jam.12058
Fu Y, Ho B. T, Mekalanos J. J. (2018). Tracking Vibrio cholerae cell-cell interactions during infection reveals bacterial population dynamics within intestinal microenvironments. Cell host and microbe, 23(2), 274–281. doi: 10.1016/j.chom.2017.12.006
Galanis E., Otterstatter M., Taylor M. (2020). Measuring the Impact of Sea Surface Temperature on the Human Incidence of Vibrio Spp. Infection in British Columbia, Canada 1992–2017. Environ. Health 19, 1–7. doi: 10.1186/s12940-020-00605-x
Gallardo K., Candia J. E., Remonsellez F., Escudero L. V., Demergasso C. S. (2016). The Ecological Coherence of Temperature and Salinity Tolerance Interaction and Pigmentation in a non-Marine Vibrio Isolated From Salar De Atacama. Front. Microbiol. 7, 1943. doi: 10.3389/fmicb.2016.01943
Gray R. J., Muir A. M. (1977). Salt Deprivation and Low Temperature Sensitivity of Vibrio parahaemolyticus. J. Food Sci. 42, 689–693. doi: 10.1111/j.1365-2621.1977.tb12580.x
Guérin-Faublée V., Rosso L., Vigneulle M., Flandrois J. P. (1995). The Effect of Incubation Temperature and Sodium Chloride Concentration on the Growth Kinetics of Vibrio anguillarum and Vibrio anguillarum-Related Organisms. J. Appl. bacteriol. 78, 621–629. doi: 10.1111/j.1365-2672.1995.tb03108.x
Gu D., Guo M., Yang M., Zhang Y., Zhou X., Wang Q. (2016). A ςe-Mediated Temperature Gauge Controls a Switch From LuxR-Mediated Virulence Gene Expression to Thermal Stress Adaptation in Vibrio alginolyticus. PloS Pathog. 12, e1005645. doi: 10.1371/journal.ppat.1005645
Han N., Mizan M. F. R., Jahid I. K., Ha S.-D. (2016). Biofilm Formation by Vibrio parahaemolyticus on Food and Food Contact Surfaces Increases With Rise in Temperature. Food Control. 70, 161–166. doi: 10.1016/j.foodcont.2016.05.054
Hansen H., Bjelland A. M., Ronessen M., Robertsen E., Willassen N. P. (2014). LitR is a Repressor of Syp Genes and has a Temperature-Sensitive Regulatory Effect on Biofilm Formation and Colony Morphology in Vibrio (Aliivibrio) Salmonicida. Appl. Environ. Microbiol. 80, 5530–5541. doi: 10.1128/AEM.01239-14
Hare P., Long S., Robb F., Woods D. R. (1981). Regulation of Exoprotease Production by Temperature and Oxygen in Vibrio alginolyticus. Arch. Microbiol. 130, 276–280. doi: 10.1007/BF00425940
Hernández-Cabanyero C., Lee C. T., Tolosa-Enguis V., Sanjuán E., Pajuelo D., Reyes-López F., et al. (2019). Adaptation to Host in Vibrio vulnificus, a Zoonotic Pathogen That Causes Septicemia in Fish and Humans. Environ. Microbiol. 21, 3118–3139. doi: 10.1111/1462-2920.14714
Hernández-Cabanyero C., Sanjuán E., Fouz B., Pajuelo D., Vallejos-Vidal E., Reyes-López F. E., et al. (2020). The Effect of the Environmental Temperature on the Adaptation to Host in the Zoonotic Pathogen Vibrio vulnificus. Front. Microbiol. 11, 489. doi: 10.3389/fmicb.2020.00489
Hijarrubia M., Lazaro B., Sunen E., Fernandez-Astorga A. (1996). Survival of Vibrio vulnificusunder Ph, Salinity and Temperature Combined Stress. Food Microbiol. 13, 193–199. doi: 10.1006/fmic.1996.0024
Horie S., Okuzumi M., Kato N., Saito K. (1966). Comparative Observations on the Range of Growth Temperature Among Three Biotypes of Vibrio parahaemolyticus. Bull. Jap. Soc Sci. Fish. 32, 424–426. doi: 10.2331/suisan.32.424
Huq A., West P. A., Small E. B., Huq M. I., Colwell R. R. (1984). Influence of Water Temperature, Salinity, and pH on Survival and Growth of Toxigenic Vibrio cholerae Serovar 01 Associated With Live Copepods in Laboratory Microcosms. Appl. Environ. Microbiol. 48, 420–424. doi: 10.1128/aem.48.2.420-424.1984
Huzmi H., Ina-Salwany M. Y., Natrah F. M. I., Syukri F., Karim M. (2019). Strategies of Controlling Vibriosis in Fish. Asian J. Appl. Sci. 7 (5), 513–521. doi: 10.24203/ajas.v7i5.5933
Israely T., Banin E., Rosenberg E. (2001). Growth, Differentiation and Death of Vibrio shiloi in Coral Tissue as a Function of Seawater Temperature. Aquat. Microbial. Ecol. 24, 1–8. doi: 10.3354/ame024001
Jiang Q., Shi L., Ke C., You W., Zhao J. (2013). Identification and Characterization of Vibrio harveyi Associated With Diseased Abalone Haliotis Diversicolor. Dis. Aquat. Organisms 103, 133–139. doi: 10.3354/dao02572
Johnston M., Brown M. (2002). An Investigation Into the Changed Physiological State of Vibrio bacteria as a Survival Mechanism in Response to Cold Temperatures and Studies on Their Sensitivity to Heating and Freezing. J. Appl. Microbiol. 92, 1066–1077. doi: 10.1046/j.1365-2672.2002.01657.x
Julie D., Solen L., Antoine V., Annick D., Dominique H. H. (2010). Ecology of Pathogenic and non-Pathogenic Vibrio parahaemolyticus on the French Atlantic Coast. Effects of Temperature, Salinity, Turbidity and Chlorophyll a. Environ. Microbiol. 12, 929–937. doi: 10.1111/j.1462-2920.2009.02136.x
Kaneko T., Colwell R. R. (1974). Distribution of Vibrio parahaemolyticus and Related Organisms in the Atlantic Ocean Off South Carolina and Georgia. Appl. Microbiol. 28, 1009–1017. doi: 10.1128/am.28.6.1009-1017.1974
Karlsen C., Paulsen S. M., Tunsjø H. S., Krinner S., Sørum H., Haugen P., et al. (2008). Motility and Flagellin Gene Expression in the Fish Pathogen Vibrio salmonicida: Effects of Salinity and Temperature. Microbial. pathogen. 45, 258–264. doi: 10.1016/j.micpath.2008.06.002
Kaspar C. A., Tamplin M. (1993). Effects of Temperature and Salinity on the Survival of Vibrio vulnificus in Seawater and Shellfish. Appl. Environ. Microbiol. 59, 2425–2429. doi: 10.1128/aem.59.8.2425-2429.1993
Kelly M. T. (1982). Effect of Temperature and Salinity on Vibrio (Beneckea) Vulnificus Occurrence in a Gulf Coast Environment. Appl. Environ. Microbiol. 44, 820–824. doi: 10.1128/aem.44.4.820-824.1982
Kim C.-M., Ahn Y.-J., Kim S.-J., Yoon D.-H., Shin S.-H. (2016). Temperature Change Induces the Expression of vuuA Encoding Vulnibactin Receptor and Crp Encoding Cyclic AMP Receptor Protein in Vibrio Vulnificus. Curr. Microbiol. 73, 54–64. doi: 10.1007/s00284-016-1026-8
Kimes N. E., Grim C. J., Johnson W. R., Hasan N. A., Tall B. D., Kothary M. H., et al. (2012). Temperature Regulation of Virulence Factors in the Pathogen Vibrio coralliilyticus. ISME J. 6, 835–846. doi: 10.1038/ismej.2011.154
Kim Y. W., Lee S. H., Hwang I. G., Yoon K. S. (2012). Effect of Temperature on Growth of Vibrio paraphemolyticus and Vibrio vulnificus in Flounder, Salmon Sashimi and Oyster Meat. Int. J. Environ. Res. Public Health 9, 4662–4675. doi: 10.3390/ijerph9124662
Kim C.-M., Shin S.-H. (2011). Change of Vibrio vulnificus Metalloprotease VvpE Production by Temperature and Salinity. J. Bacteriol. Virol. 41, 147–156. doi: 10.4167/jbv.2011.41.3.147
Kirschner A. K., Schlesinger J., Farnleitner A. H., Hornek R., Süß B., Golda B., et al. (2008). Rapid Growth of Planktonic Vibrio cholerae non-O1/non-O139 Strains in a Large Alkaline Lake in Austria: Dependence on Temperature and Dissolved Organic Carbon Quality. Appl. Environ. Microbiol. 74, 2004–2015. doi: 10.1128/AEM.01739-07
Kushmaro A., Banin E., Loya Y., Stackebrandt E., Rosenberg E. (2001). Vibrio shiloi Sp. Nov., the Causative Agent of Bleaching of the Coral Oculina Patagonica. Int. J. system. evolution. Microbiol. 51, 1383–1388. doi: 10.1099/00207713-51-4-1383
Kushmaro A., Rosenberg E., Fine M., Haim Y. B., Loya Y. (1998). Effect of Temperature on Bleaching of the Coral Oculina Patagonica by Vibrio AK-1. Mar. Ecol. Prog. Ser. 171, 131–137. doi: 10.3354/meps171131
Lages M. A., Balado M., Lemos M. L. (2019). The Expression of Virulence Factors in Vibrio anguillarum is Dually Regulated by Iron Levels and Temperature. Front. Microbiol.10(OCT), 2335. doi: 10.3389/fmicb.2019.02335
Larsen J. (1984). Vibrio anguillarum: Influence of Temperature, Ph, NaCl Concentration and Incubation Time on Growth. J. Appl. bacteriol. 57, 237–246. doi: 10.1111/j.1365-2672.1984.tb01388.x
Larsen A., Rikard F., Walton W., Arias C. (2015). Temperature Effect on High Salinity Depuration of Vibrio Vulnificus and V. parahaemolyticus From the Eastern Oyster (Crassostrea Virginica). Int. J. Food Microbiol. 192, 66–71. doi: 10.1016/j.ijfoodmicro.2014.09.025
Limthammahisorn S., Brady Y. J., Arias C. R. (2008). Gene Expression of Cold Shock and Other Stress-Related Genes in Vibrio Vulniflcus Grown in Pure Culture Under Shellstock Temperature Control Conditions. J. Food Prot. 71, 157–164. doi: 10.4315/0362-028X-71.1.157
Lipp E. K., Rivera I. N., Gil A. I., Espeland E. M., Choopun N., Louis V. R., et al. (2003). Direct Detection of Vibrio cholerae and ctxA in Peruvian Coastal Water and Plankton by PCR. Appl. Environ. Microbiol. 69, 3676–3680. doi: 10.1128/AEM.69.6.3676-3680.2003
Liu B., Liu H., Pan Y., Xie J., Zhao Y. (2016). Comparison of the Effects of Environmental Parameters on the Growth Variability of Vibrio parahaemolyticus Coupled With Strain Sources and Genotypes Analyses. Front. Microbiol. 7, 994. doi: 10.3389/fmicb.2016.00994
Lomelí-Ortega C. O., Martínez-Sández A. J., Barajas-Sandoval D. R., Reyes A. G., Magallón-Barajas F., Veyrand-Quíros B., et al. (2021). Isolation and Characterization of Vibriophage Vb_Vc_SrVc9: An Effective Agent in Preventing Vibrio Campbellii Infections in Brine Shrimp Nauplii (Artemia Franciscana). J. Appl. Microbiol. 131 (1), 36–49. doi: 10.1111/jam.14937
Lopez-Joven C., De Blas I., Roque A. (2018a). Temperature Effects on the Growth and Survival of Tdh Positive Vibrio parahaemolyticus in Tissues of Postharvest Manila Clam (Ruditapes Philippinarum). Food Microbiol. 75, 61–64. doi: 10.1016/j.fm.2017.10.016
Lopez-Joven C., Rolland J.-L., Haffner P., Caro A., Roques C., Carré C., et al. (2018b). Oyster Farming, Temperature, and Plankton Influence the Dynamics of Pathogenic Vibrios in the Thau Lagoon. Front. Microbiol.9(OCT), 2530. doi: 10.3389/fmicb.2018.02530
Louis V. R., Russek-Cohen E., Choopun N., Rivera I. N., Gangle B., Jiang S. C., et al. (2003). Predictability of Vibrio cholerae in Chesapeake Bay. Appl. Environ. Microbiol. 69, 2773–2785. doi: 10.1128/AEM.69.5.2773-2785.2003
Marco-Noales E., Biosca E. G., Amaro C. (1999). Effects of Salinity and Temperature on Long-Term Survival of the Eel Pathogen Vibrio vulnificus Biotype 2 (Serovar E). Appl. Environ. Microbiol. 65, 1117–1126. doi: 10.1128/AEM.65.3.1117-1126.1999
Mccarthy S. (1996). Effects of Temperature and Salinity on Survival of Toxigenic Vibrio cholerae O1 in Seawater. Microbial. Ecol. 31, 167–175. doi: 10.1007/BF00167862
Miles D. W., Ross T., Olley J., Mcmeekin T. A. (1997). Development and Evaluation of a Predictive Model for the Effect of Temperature and Water Activity on the Growth Rate of Vibrio parahaemolyticus. Int. J. Food Microbiol. 38, 133–142. doi: 10.1016/S0168-1605(97)00100-1
Mongeon P., Paul-Hus A. (2016). The Journal Coverage of Web of Science and Scopus: A Comparative Analysis. Scientometrics 106, 213–228. doi: 10.1007/s11192-015-1765-5
Montanchez I., Kaberdin V. R. (2020). Vibrio harveyi: A Brief Survey of General Characteristics and Recent Epidemiological Traits Associated With Climate Change. Mar. Environ. Res. 154, 104850. doi: 10.1016/j.marenvres.2019.104850
Montánchez I., Ogayar E., Plágaro A. H., Esteve-Codina A., Gómez-Garrido J., Orruño M., et al. (2019). Analysis of Vibrio harveyi Adaptation in Sea Water Microcosms at Elevated Temperature Provides Insights Into the Putative Mechanisms of its Persistence and Spread in the Time of Global Warming. Sci. Rep. 9, 1–12. doi: 10.1038/s41598-018-36483-0
Montilla R., Chowdhury M., Huq A., Xu B., Colwell R. R. (1996). Serogroup Conversion of Vibrio cholerae non-O1 to Vibrio cholerae O1: Effect of Growth State of Cells, Temperature, and Salinity. Can. J. Microbiol. 42, 87–93. doi: 10.1139/m96-014
Morii H., Sarukawa H. (2000). Growth Pattern and Adenosine Phosphates in Psychrotrophic Vibrio Spp. At Different Growth Temperatures. Fish. Sci. 66, 826–833. doi: 10.1046/j.1444-2906.2000.00134.x
Morita R., Albright L. (1968). Moderate Temperature Effects on Protein, Ribonucleic Acid and Deoxyribonucleic Acid Syntheses by Vibrio marinus, an Obligately Psychrophilic Marine Bacterium. Z. für allgemeine Mikrobiologie 8, 269–273. doi: 10.1002/jobm.19680080404
Motes M., Depaola A., Cook D., Veazey J., Hunsucker J., Garthright W., et al. (1998). Influence of Water Temperature and Salinity on Vibrio Vulnificus in Northern Gulf and Atlantic Coast Oysters (Crassostrea Virginica). Appl. Environ. Microbiol. 64, 1459–1465. doi: 10.1128/AEM.64.4.1459-1465.1998
Mudoh M., Parveen S., Schwarz J., Rippen T., Chaudhuri A. (2014). The Effects of Storage Temperature on the Growth of Vibrio parahaemolyticus and Organoleptic Properties in Oysters. Front. Public Health 2, 45. doi: 10.3389/fpubh.2014.00045
Murali M. R., Raja S. B., Devaraj S. N. (2010). Neutralization of Radical Toxicity by Temperature-Dependent Modulation of Extracellular SOD Activity in Coral Bleaching Pathogen Vibrio shiloi and its Role as a Virulence Factor. Arch. Microbiol. 192, 619–623. doi: 10.1007/s00203-010-0588-2
Murphy S. K., Oliver J. D. (1992). Effects of Temperature Abuse on Survival of Vibrio vulnificus in Oysters. Appl. Environ. Microbiol. 58, 2771–2775. doi: 10.1128/aem.58.9.2771-2775.1992
Nishiguchi M. K. (2000). Temperature Affects Species Distribution in Symbiotic Populations of Vibrio Spp. Appl. Environ. Microbiol. 66, 3550–3555. doi: 10.1128/AEM.66.8.3550-3555.2000
Nishina T., Wada M., Ozawa H., Hara-Kudo Y., Konuma H., Hasegawa J., et al. (2004). Growth Kinetics of Vibrio parahaemolyticus O3 : K6 Under Varying Conditions of Ph, NaCl Concentration and Temperature. Food Hygiene Saf. Sci. (Shokuhin Eiseigaku Zasshi) 45, 35–37. doi: 10.3358/shokueishi.45.35
Nishino T., Kogure K. (2003). Transient Temperature Up-Shift Suppresses the Loss of Culturability of Vibrio parahaemolyticus Stressed by Low Temperature and Nutrient Deficiency. Microbes Environments 18, 227–230. doi: 10.1264/jsme2.18.227
Olafsen J. A., Christie M., Raa J. (1981). Biochemical Ecology of Psychrotrophic Strains of Vibrio anguillarum Isolated From Outbreaks of Vibriosis at Low Temperature. Zentralblatt Für Bakteriologie Mikrobiologie Und Hygiene: I. Abt. Originale C: Allgemeine, Angewandte Und Ökologische Mikrobiologie2, 339–348. doi: 10.1016/S0721-9571(81)80027-0
Rahman M. S., Carraro R., Cardazzo B., Carraro L., Meneguolo D. B., Martino M. E., et al. (2017). Molecular Typing of Vibrio parahaemolyticus Strains Isolated From Mollusks in the North Adriatic Sea. Foodborne Pathog. Dis. 14, 454–464. doi: 10.1089/fpd.2016.2263
Ramakers G. G., Smulders Y. E., Van Zon A., Van Zanten G. A., Grolman W., Stegeman I. (2017). Correlation Between Subjective and Objective Hearing Tests After Unilateral and Bilateral Cochlear Implantation. BMC Ear Nose Throat Disord. 17, 1–8. doi: 10.1186/s12901-017-0043-y
Randa M. A., Polz M. F., Lim E. (2004). Effects of Temperature and Salinity on Vibrio vulnificus Population Dynamics as Assessed by Quantitative PCR. Appl. Environ. Microbiol. 70, 5469–5476. doi: 10.1128/AEM.70.9.5469-5476.2004
Ravel J., Knight I. T., Monahan C. E., Hill R. T., Colwell R. R. (1995). Temperature-Induced Recovery of Vibrio cholerae From the Viable But Nonculturable State: Growth or Resuscitation? Microbiology 141, 377–383. doi: 10.1099/13500872-141-2-377
Schar D., Klein E. Y., Laxminarayan R., Gilbert M., Van Boeckel T. P. (2020). Global Trends in Antimicrobial Use in Aquaculture. Sci. Rep. 10 (1), 1–9. doi: 10.1038/s41598-020-78849-3
Sheikh H., John A., Musa N., Alfatama M., Fadhlina A. (2022). Vibrio Spp. And Their Vibriocin as a Vibriosis Control Measure in Aquaculture. Appl. Biochem. Biotechnol., 1–15. doi: 10.1007/s12010-022-03919-3
Singleton F., Attwell R., Jangi S., Colwell R. (1982). Effects of Temperature and Salinity on Vibrio cholerae Growth. Appl. Environ. Microbiol. 44, 1047–1058. doi: 10.1128/aem.44.5.1047-1058.1982
Soto W., Gutierrez J., Remmenga M., Nishiguchi M. K. (2009). Salinity and Temperature Effects on Physiological Responses of Vibrio fischeri From Diverse Ecological Niches. Microbial. Ecol. 57, 140–150. doi: 10.1007/s00248-008-9412-9
Stauder M., Vezzulli L., Pezzati E., Repetto B., Pruzzo C. (2010). Temperature Affects Vibrio cholerae O1 El Tor Persistence in the Aquatic Environment via an Enhanced Expression of GbpA and MSHA Adhesins. Environ. Microbiol. Rep. 2, 140–144. doi: 10.1111/j.1758-2229.2009.00121.x
Sullivan T. J., Neigel J. E. (2018). Effects of Temperature and Salinity on Prevalence and Intensity of Infection of Blue Crabs, Callinectes Sapidus, by Vibrio cholerae, V. Parahaemolyticus and V. Vulnificus in Louisiana. J. invertebrate Pathol. 151, 82–90. doi: 10.1016/j.jip.2017.11.004
Tall A., Hervio-Heath D., Teillon A., Boisset-Helbert C., Delesmont R., Bodilis J., et al. (2013). Diversity of Vibrio Spp. Isolated at Ambient Environmental Temperature in the Eastern English Channel as Determined by Pyr H Sequencing. J. Appl. Microbiol. 114, 1713–1724. doi: 10.1111/jam.12181
Tan D., Svenningsen S. L., Middelboe M. (2015). Quorum Sensing Determines the Choice of Antiphage Defense Strategy in Vibrio anguillarum. MBio 6 (3), e00627–e00615. doi: 10.1128/mBio.00627-15
Thompson F. L., Thompson C., Swings J. (2003). Vibrio tasmaniensis Sp. Nov., Isolated From Atlantic Salmon (Salmo Salar L.). System. Appl. Microbiol. 26, 65–69. doi: 10.1078/072320203322337326
Toushik S. H., Park J. H., Kim K., Ashrafudoulla M., Ulrich M. S. I., Mizan M. F. R., et al. (2022). Antibiofilm Efficacy of Leuconostoc Mesenteroides J. 27-Derived Postbiotic and Food-Grade Essential Oils Against Vibrio parahaemolyticus, Pseudomonas Aeruginosa, and Escherichia Coli Alone and in Combination, and Their Application as a Green Preservative in the Seafood Industry. Food Res. Int. 156, 111163. doi: 10.1016/j.foodres.2022.111163
Tout J., Siboni N., Messer L. F., Garren M., Stocker R., Webster N. S., et al. (2015). Increased Seawater Temperature Increases the Abundance and Alters the Structure of Natural VibrioPopulations Associated With the Coral Pocillopora Damicornis. Front. Microbiol. 6, 432. doi: 10.3389/fmicb.2015.00432
Travers M. A., Basuyaux O., Le Goïc N., Huchette S., Nicholas J. L., Koken M., et al. (2009). Influence of Temperature and Spawning Effort on Haliotis Tuberculata Mortalities Caused by Vibrio Harveyi: An Example of Emerging Vibriosis Linked to Global Warming. Global Change Biol. 15, 1365–1376. doi: 10.1111/j.1365-2486.2008.01764.x
Turner J. W., Malayil L., Guadagnoli D., Cole D., Lipp E. K. (2014). Detection of Vibrio parahaemolyticus, Vibrio vulnificus and Vibrio Cholerae With Respect to Seasonal Fluctuations in Temperature and Plankton Abundance. Environ. Microbiol. 16, 1019–1028. doi: 10.1111/1462-2920.12246
Ulitzur S. (1975). Effect of Temperature, Salts, pH and Other Factors on the Development of Peritrichous Flagella in Vibrio Alginolyticus. Arch. Microbiol. 104, 285–288. doi: 10.1007/BF00447338
Vattakaven T., Bond P., Bradley G., Munn C. B. (2006). Differential Effects of Temperature and Starvation on Induction of the Viable-But-Nonculturable State in the Coral Pathogens Vibrio Shiloi and Vibrio Tasmaniensis. Appl. Environ. Microbiol. 72, 6508–6513. doi: 10.1128/AEM.00798-06
Wang Y., Gu J. (2005). Influence of Temperature, Salinity and pH on the Growth of Environmental Aeromonas and Vibrio Species Isolated From Mai Po and the Inner Deep Bay Nature Reserve Ramsar Site of Hong Kong. J. Basic Microbiol.: Int. J. Biochem. Physiol. Genetics Morphol. Ecol. Microorganisms 45, 83–93. doi: 10.1002/jobm.200410446
Wang Y., Zhang H.-Y., Fodjo E. K., Kong C., Gu R.-R., Han F., et al. (2018). Temperature Effect Study on Growth and Survival of Pathogenic Vibrio parahaemolyticus in Jinjiang Oyster (Crassostrea Rivularis) With Rapid Count Method. J. Food Qual2018, 2060915. doi: 10.1155/2018/2060915
Whitaker W. B., Parent M. A., Naughton L. M., Richards G. P., Blumerman S. L., Boyd E. F. (2010). Modulation of Responses of Vibrio Parahaemolyticus O3: K6 to pH and Temperature Stresses by Growth at Different Salt Concentrations. Appl. Envi ron. Microbiol. 76, 4720–4729. doi: 10.1128/AEM.00474-10
Wolf P. W., Oliver J. D. (1992). Temperature Effects on the Viable But non-Culturable State of Vibrio vulnificus. FEMS Microbiol. Lett. 101, 33–39. doi: 10.1111/j.1574-6941.1992.tb01646.x
Wong K. C., Brown A. M., Luscombe G. M., Wong S. J., Mendis K. (2015). Antibiotic Use for Vibrio Infections: Important Insights From Surveillance Data. BMC Infect. Dis. 15 (1), 1–9. doi: 10.1186/s12879-015-0959-z
Wu B., Liang W., Kan B. (2016). Growth Phase, Oxygen, Temperature, and Starvation Affect the Development of Viable But non-Culturable State of Vibrio cholerae. Front. Microbiol. 7, 404. doi: 10.3389/fmicb.2016.00404
Xie J., Bu L., Jin S., Wang X., Zhao Q., Zhou S., Xu Y. (2020). Outbreak of vibriosis caused by Vibrio harveyi and Vibrio alginolyticus in farmed seahorse Hippocampus kuda in China. Aquaculture, 523, 735168. doi: 10.1002/mbo3.504
Yang Z.-Q., Jiao X.-A., Li P., Pan Z.-M., Huang J.-L., Gu R.-X., et al. (2009). Predictive Model of Vibrio parahaemolyticus Growth and Survival on Salmon Meat as a Function of Temperature. Food Microbiol. 26, 606–614. doi: 10.1016/j.fm.2009.04.004
Yoon K., Min K., Jung Y., Kwon K., Lee J., Oh S. (2008). A Model of the Effect of Temperature on the Growth of Pathogenic and Nonpathogenic Vibrio parahaemolyticus Isolated From Oysters in Korea. Food Microbiol. 25, 635–641. doi: 10.1016/j.fm.2008.04.007
Young I., Gropp K., Fazil A., Smith B. A. (2015). Knowledge Synthesis to Support Risk Assessment of Climate Change Impacts on Food and Water Safety: A Case Study of the Effects of Water Temperature and Salinity on Vibrio parahaemolyticus in Raw Oysters and Harvest Waters. Food Res. Int. 68, 86–93. doi: 10.1016/j.foodres.2014.06.035
Keywords: vibrio, growth, global warming, pathogenicity, SLR, treatment
Citation: Sheikh HI, Najiah M, Fadhlina A, Laith AA, Nor MM, Jalal KCA and Kasan NA (2022) Temperature Upshift Mostly but not Always Enhances the Growth of Vibrio Species: A Systematic Review. Front. Mar. Sci. 9:959830. doi: 10.3389/fmars.2022.959830
Received: 02 June 2022; Accepted: 24 June 2022;
Published: 29 July 2022.
Edited by:
Siddhartha Pati, NatNov Bioscience Pvt Ltd., IndiaReviewed by:
Ipsita Mohanty, Children’s Hospital of Philadelphia, United StatesArabinda Mahanty, National Rice Research Institute Indian Council of Agricultural Research, India
Copyright © 2022 Sheikh, Najiah, Fadhlina, Laith, Nor, Jalal and Kasan. This is an open-access article distributed under the terms of the Creative Commons Attribution License (CC BY). The use, distribution or reproduction in other forums is permitted, provided the original author(s) and the copyright owner(s) are credited and that the original publication in this journal is cited, in accordance with accepted academic practice. No use, distribution or reproduction is permitted which does not comply with these terms.
*Correspondence: Nor Azman Kasan, bm9yYXptYW5AdW10LmVkdS5teQ==