- 1MARE – Marine and Environmental Sciences Centre, ARNET – Aquatic Research Network, ISPA – Instituto Universitário de Ciências Psicológicas, Sociais e da Vida, Lisboa, Portugal
- 2Portuguese Institute for the Sea and the Atmosphere – IPMA, Av. Doutor Alfredo Magalhães Ramalho, Algés, Portugal
- 3IEO - Instituto Español de Oceanografía, Subida a Radio Faro 50, Vigo, Spain
- 4Department of Coastal Systems (COS), NIOZ - Royal Netherlands Institute for Sea Research, Texel, Netherlands
Spawning habitats of cold-water, European small pelagic fishes have shifted poleward in the last three decades coincident with gradual ocean warming. We predicted present-day, season-specific habitat suitability for spawning by European sardine Sardina pichardus in the Atlantic Ocean and Mediterranean and Black Seas, and projected climate-driven changes in suitable areas from 2050-2099 under the IPCC – RCP 8.5 scenario. Sea surface temperature and distance to the coast had the greater influences in spawning habitats, reflecting the temperature- and coastal-dependent spawning of sardines. Chlorophyll-a was the third most important explanatory variable for spawning in winter to summer. Winds were predominantly important during autumn, whilst sea surface salinity was an important driver during spring and summer. Present-day, “hotspots” for spawning were identified in regions of highly productive, salty waters, where SST was between 6 and 18°C from autumn to spring or 16 and 25°C during summer and favourable winds occurred that would retain eggs and larvae closer to the coast (< 250 km). For future scenarios, forecasts indicate that environmental optima for spawning is projected to be in regions where SST varies between 11°C and 18°C from autumn to spring; and between 18°C and 24°C during summer. However, a negative relationship between phytoplankton productivity and habitat suitability induced by warming is likely to occur in the future. Projections suggest that suitable spawning habitats in all seasons will shift to higher latitudes, with a prominent range expansion along the coast of Norway during winter and autumn (> 83%). The total spawning area, however, was projected to contract in the future during spring (-10.5%) and autumn (-4.1%) due to losses of currently suitable areas along the Atlantic African Coast and Mediterranean Sea. Such regions currently support the greatest sardine stocks but climate-driven warming and decreased plankton productivity are projected to make these areas unsuitable for spawning and likely also for sardine fisheries in future.
Introduction
Marine fishes have evolved different successful life history strategies to spawn at specific times and in certain regions where oceanographic conditions help guarantee successful dispersal, survival and recruitment (Santos et al., 2004; Hinrichsen et al., 2016; Baltazar-Soares et al., 2018). Marine spawning habitats are acknowledged to not only accommodate early life stages of fishes, but also to guarantee fishery productivity (Gordó-Vilaseca et al., 2021). Seasonal shifts in ocean currents, winds, productivity, and water temperature are known to be major factors influencing species-specific spawning periods (Ganias, 2009; Mbaye et al., 2020; Torrado et al., 2021). Spawning is directly affected by adult population size and indirectly by environmental-driven changes affecting species distribution (Somarakis et al., 2019; Brosset et al., 2020; Peck et al., 2021). Disturbances in spawning habitats may alter recruitment patterns and, thus, affect fisheries (Ramzi et al., 2006). Therefore, the understanding of the environmental factors influencing the distribution of spawning habitats of fish species, both in the present-day and future conditions is pivotal to a better and adaptive management of fish stocks and the conservation of marine resources (Kallasvuo et al., 2017; Fernández-Corredor et al., 2021).
In the marine environment, organisms challenged by ocean warming, acidification and oxygen depletion by either tolerating local conditions with decrements in performance adapting to improve their physiological performance, or shifting their distribution to preserve climatic niche (Pörtner and Peck, 2010; Jutfelt (2020); Lima et al., 2022). In fish, such responses to new environmental conditions have been extensively studied for juvenile and adult phases, but little work has been conducted on early life stages such as eggs and larvae that are more vulnerable than juveniles and adults to suffer high rates of mortality through both bottom-up (temperature, prey) and top-down (predation) processes (Lima et al., 2019). For a number of reasons, therefore, eggs and larvae are expected to be among the most sensitive life stages to global change (Dahlke et al., 2020). Assessing the effects of climate change in spawning habitats is crucial to predict the future of fish stocks since connectivity among fish populations depends on the distribution patterns of early life stages (Petitgas et al., 2013).
Global warming is anticipated to have long and lasting impacts on ocean characteristics (Cavanaugh et al., 2015; Peck et al., 2018). An important process for some small pelagic fish will be the intensification (and changes in the zonal position) of alongshore winds and associated coastal upwelling and productivity (Xiu et al., 2018). These gradual, global-scale changes are expected to alter the trophodynamic processes such as predator-prey match-mismatch dynamics (Petitgas et al., 2013; Moyano et al., 2017). Moreover, changes in ocean circulation patterns will influence the dispersal of early life stages affecting habitat connectivity within and among marine fish populations and, consequently, successful life history strategies (Baltazar-Soares et al., 2018; Torrado et al., 2021). However, it is challenging to predict whether the spawning regions suitable now will remain so in the future. This challenge is underscored by the latitudinal shifts in productive spawning grounds of cold-water species of small pelagic fish (SPF) observed in Atlantic waters in the last three decades possibly as a response to ocean warming (Petitgas et al., 2012; Giannoulaki et al., 2014; Bruge et al., 2016).
The European sardine (Sardina pilchardus) represents one of the most important fishing resources in central Morocco, in the Mediterranean basin and off the Atlanto-Iberian upwelling ecosystem (ICES, 2006; Silva et al., 2015; DGRM, 2016). Fishing activities targeting sardines have also increased by 35% from the Bay of Biscay up to the North Sea since the 1990’s (Alheit et al., 2012), suggesting that this species has been migrating poleward. Rising temperatures in the North Sea have been suggested as the major driver for this shift (Sabatés et al., 2006; Palomera et al., 2007; Zeeberg et al., 2008) and sardine spawning habitats have also been reported to be shifting poleward (Beare et al., 2004; Fréon et al., 2009; Alheit et al., 2012). This northward expansion of adult sardines in the northeast Atlantic has been forecasted to include the entire Norwegian coast to the White Sea by 2100, under the worst case IPCC greenhouse gas scenario (RCP 8.5) (Schickele et al., 2020; Lima et al., 2022). Thus, to assess whether these new habitats are suitable as spawning areas is of major importance for the management of the species and its fisheries under future conditions.
Due to their relatively short life spans, small pelagic fish exhibit spatio-temporal variability in spawning linked to changes in a few, key environmental variables, such as water temperature, salinity, productivity, and wind direction and speed (Bernal et al., 2007; Maynou et al., 2020a). Both the Mediterranean and the Atlantic stocks of sardine exhibit protracted spawning seasons under a narrow range of temperatures (13 to 18°C) (Ganias et al., 2007; Stratoudakis et al., 2007). The spatial and temporal patterns of spawning are acknowledged to be highly variable throughout the year, but intensification of spawning activity is observed mainly during winter and spring months (Olivar et al., 2003; Coombs et al., 2006; Somarakis et al., 2006; Stratoudakis et al., 2007; Giannoulaki et al., 2014). At the present time, spawning in southern European areas appears to be triggered by decreasing water temperature in the autumn and completed as temperature increases again in the spring (Palomera et al., 2007; Fernández-Corredor et al., 2021). However, the phenology of sardine spawning under climate change may lead to seasonal mismatches and changes in recruitment (Fréon et al., 2009; Checkley et al., 2017).
Our objectives here were to test what are the best predictors for present-day sardine spawning and to provide projections of how climate change will impact the large-scale distributional range of seasonal spawning habitats potentially used by European sardine. We employed a multi-Habitat Suitability Model (HSM) approach based on the present-day distribution of spawning combined with IPCC worse-base (RCP8.5) projections of mid- (2050) and late- (2100) century ocean climate. By exploring the entire distributional range of this SPF, this study provide an important contribution to developing climate adaptation plans for the fisheries that have historically targeting this resource.
Materials and methods
Selection of occurrence data of eggs and larvae
Geo-referenced occurrence data (historical spatial points from 1905 to 2020) of European sardine eggs and larvae along the spawning areas were compiled from several databases available in several institutions. They include the Portuguese Institute for the Sea and the Atmosphere (IPMA), the Instituto Español de Oceanografía (IEO), the International Council for the Exploration of the Sea (ICES), the Ocean Biodiversity Information System (OBIS), the Marine and Environmental Sciences Centre (MARE) and the Centre of Marine Sciences of the University of Algarve (CCMAR) (Table S1). The spatial extent of the available dataset covers most of the known, present-day spawning habitats utilized by this species in the Northeast Atlantic Ocean and Mediterranean Sea (Figure S1). A total of 15,868 spatial points were then separated into four datasets representing each seasonal period: 2,248 for winter (Jan - Mar), 12,344 for spring (Apr - Jun), 691 for summer (Jul - Sep) and 585 for autumn (Oct - Dec) (Figure S1). The final dataset included occurrences from 1905 to 2019. As ocean warming and heat content increased with low rates between 1900 and 2015 when compared to the last decade (4.5 and 2 times higher, respectively), we consider that eggs and larvae captured 100 years ago likely faced the same ocean characteristics as present-day larvae (Garcia-Soto et al., 2021). Duplicated data among different databases and spatial points located outside the spawning range (i.e. 20°N-60°N; 35°W-40°E) were excluded from the datasets.
Selection of the environmental dataset for present-day spawning areas
We gathered 7 explanatory variables among biological, chemical, and physical remotely sensed data to statistically define the present-day, suitable spawning areas of the European sardine by season (Table S2). These variables are raster layers based on quarterly-averaged satellite images from daily measurements presented as grids under Geographic Information Systems representing the environmental characteristics around each sampling point. The variables were downloaded from NOAA’s WOA 2018 Data, Copernicus-Global Ocean Biogeochemistry Analysis and Forecast, Copernicus-Global Ocean Wind L4 and NASA’s Goddard Space Flight Center (Table S2). To reproduce the characteristics of the raster with the highest resolution, we downscaled all raster layers to a common spatial resolution of 0.01° by fitting kriging interpolations in the spatial analyst tools [ArcGis 10.4.1 (https://www.esri.com/)] or re-projections in the raster package [ (Hijmans et al., 2020) in R 3.6.2 (R Core Team, 2020] (Table S2).
Sea surface temperature (°C) (Locarnini et al., 2018), sea surface salinity (PSU) (Zweng et al., 2018), dissolved molecular oxygen (mol m-3) (Garcia et al., 2018) and chlorophyll a (mg m-3) (Perruche, 2018) are known to directly influence the spawning of the European sardine (Coombs et al., 2006; Fernández-Corredor et al., 2021). Near surface wind speed (m s-1) northward and eastward (Bentamy, 2020) was used as a proxy for casual factors such as passive dispersal or drifting to upwelling areas (Roy et al., 1992; Santos et al., 2005). Finally, the distance to the nearest coast (km) (NASA, 2009) was used as a proxy for the affinity of eggs and larvae to the neritic zone (Santos et al., 2006).
Data analysis, selection of predictors for the future scenario and spatial mapping
To describe the present-day suitability of spawning areas of the European sardine, sets of environmental predictors were constructed for each season. A Pearson’s correlation matrix was used to check the correlation among layers. Layers with correlation below 0.45 (P > 0.05) were included in the same set. Different modelling algorithms computed from the Biomod2 package (Thuiller et al., 2020) were applied in a presence/pseudo-absence approach to forecast the suitability of present-day spawning areas of the European sardine. As the response variable (yi), we used the presence/pseudo-absence of eggs and larvae of S. pilchardus. As explanatory variables (xi), we examined those variables selected for each seasonal set. The present-day suitability of spawning areas was obtained using an ensemble forecasting framework that combines models (i.e. mean distribution from all selected models) reproducing the most accurate spatial analyses (Pearson et al., 2006; Araújo and New, 2007; Buisson et al., 2010). Modelling algorithms included regression-based [(i) Generalized Linear Model (GLM), (ii) Generalized Additive Model (GAM)], machine learning methods [(iii) Artificial Neural Network (ANN), (iv) Flexible Discriminant Analysis (FDA), (v) Generalized Boosting Model (GBM), (vi) Random Forest (RF) and] and a classification method [(vii) Classification Tree Analysis (CTA)] (Table S3). We randomly selected pseudo-absences outside the realized niche and based on the optimization of Barbet-Massin, et al. (2012) using the same number of pseudo-absences as available presences for each season. Pseudo-absences were sampled in accessible areas that could be reached by dispersal.
Once the environmental sets, spatial points and algorithms were defined, we performed several model runs to define the most powerful variables and algorithms that could be included in the final ensemble forecast for each season through a backward selection approach. The respective explanatory power of the variables was calculated using the function “variable_importance” in the biomod2 package according to 1000 permutations (Thuiller et al., 2020). Simple, Pearson's correlation was computed five times between reference predictions and shuffled predictions and the return score is an average of 1 minus the correlation (1-cor). The highest the value, the more influence the variable has on the ensemble model. Variables whose explanatory power was below 0.10 were excluded from the analysis and new sets were built and re-analysed with all previously defined algorithms. The accuracy of each algorithm was based on scores > 0.85 for 5 metrics: Bias (BIAS), Probability of detection (POD), Relative Operating Characteristic (ROC), True Skill Statistic (TSS) and Success Ratio (SR) (Thuiller et al., 2009; Thuiller et al., 2016). Therefore, the final ensemble forecasts included only environmental variables and models above the defined thresholds.
To forecast the effects of climate change and to investigate potential changes in the suitability and range of spawning areas of the European sardine (i.e. present-day vs. future conditions), we used the average of all models available in the IPCC - RCP 8.5 greenhouse gas concentration levels simulated between 2050 and 2099 in the Coupled Model Intercomparison Project (CMIP5) (Moss et al., 2010; Scott et al., 2016). For the future ensemble models, we used the same environmental variables considered for each season of the present-day condition to allow comparisons among scenarios (Table 1 and Figure S2–S6). The only exception was the distance to the nearest coast as we considered this variable to be constant over time. Shoreline retreat will be less than 1 km over the next 78 years, being negligible in terms of habitat expansion when compared to present-day shoreline positions along the study area (Vousdoukas et al., 2017; Athanasiou et al., 2020).
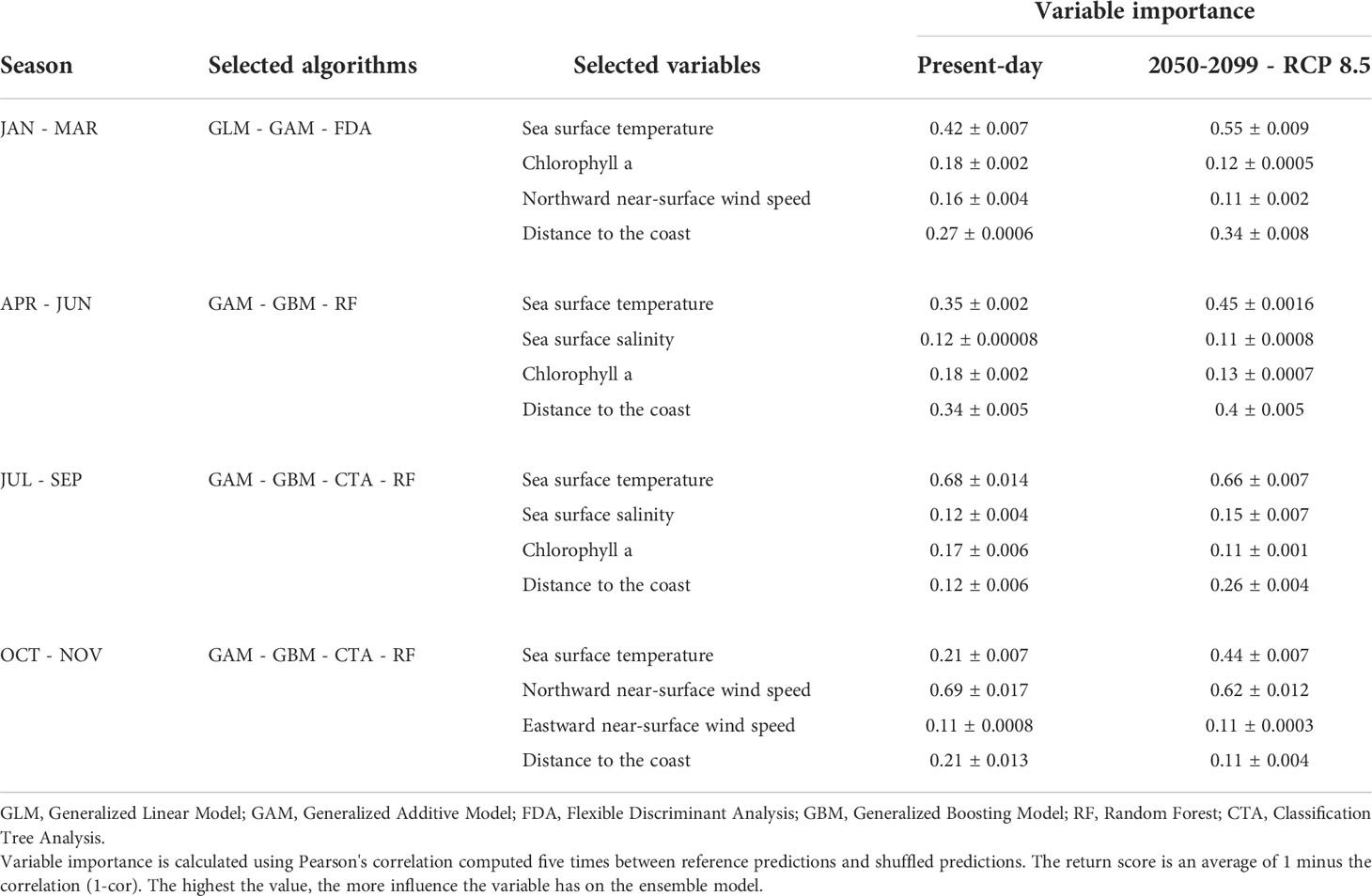
Table 1 Selected algorithms and variable importance of each season for the present-day and future scenarios.
The outputs of the final selected ensemble models are presented as plots of the best-fitting predictions for single variables shown as perspective. The mean distribution from all selected algorithms (or the ensemble forecast) was applied in a predictive mode to provide response estimates of suitability (0 to 1) for each season, over a wider grid of a largescale map at a GIS resolution of 0.01°.
The difference between suitability of future vs. present-day maps was investigated to evaluate the potential changes in spawning habitats (Figure 2). To detect range expansion and contraction in spawning habitats, binary HSMs were created to calculate the suitable areas in square kilometres for present-day and future maps using the spatial analyst tools in ArcGis 10.4.1 (https://www.esri.com/). The binary HSMs followed the threshold established by Ahmad et al. (2019). Suitability < 0.25 is considered as unsuitable habitats (= 0) and > 0.25 to 1 as potential spawning areas (= 1), namely “overall suitability”. Then, the comparison of suitable areas (km2) between present-day and future maps were converted to percentages of expansion or contraction in total and regional scales.
Results
Relative importance of environmental variables in structuring the suitability of sardine’s spawning habitats
The ensemble models for the winter season (January to March) included the algorithms GLM, GAM and FDA. Sea surface temperature (SST) was the most important predictor, accounting to 0.42 of explanatory power, followed by the distance to the nearest coast (DIST), chlorophyll a (Chl-a) and northward near-surface wind speed (NWS) (Table 1 and Figure S3). For the present-day winter, the environmental optimum for sardine spawning was predicted to be areas with winds predominantly blowing from the north with speeds from -6 to 0.3 m s-1 (predominant negative NWS), SST between 6 and 16°C, Chl-a > 0.25 mg m-3, and DIST < 250 km (Figure 1 and Table 2). For the future winter scenario, the explanatory power of environmental variables followed the same order as that for the present-day condition, but explanatory power of SST increased to 0.55 (Table 1). It was forecasted that environmental optima for spawning will occur in areas under the influence of winds blowing from both north and south, with speeds between -1.5 to 1.5 m s-1, where SST will warm up to 14-18°C, Chl-a will be above 0.07 mg m-3, and DIST will be also below 250 km (Figure 1 and Table 2).
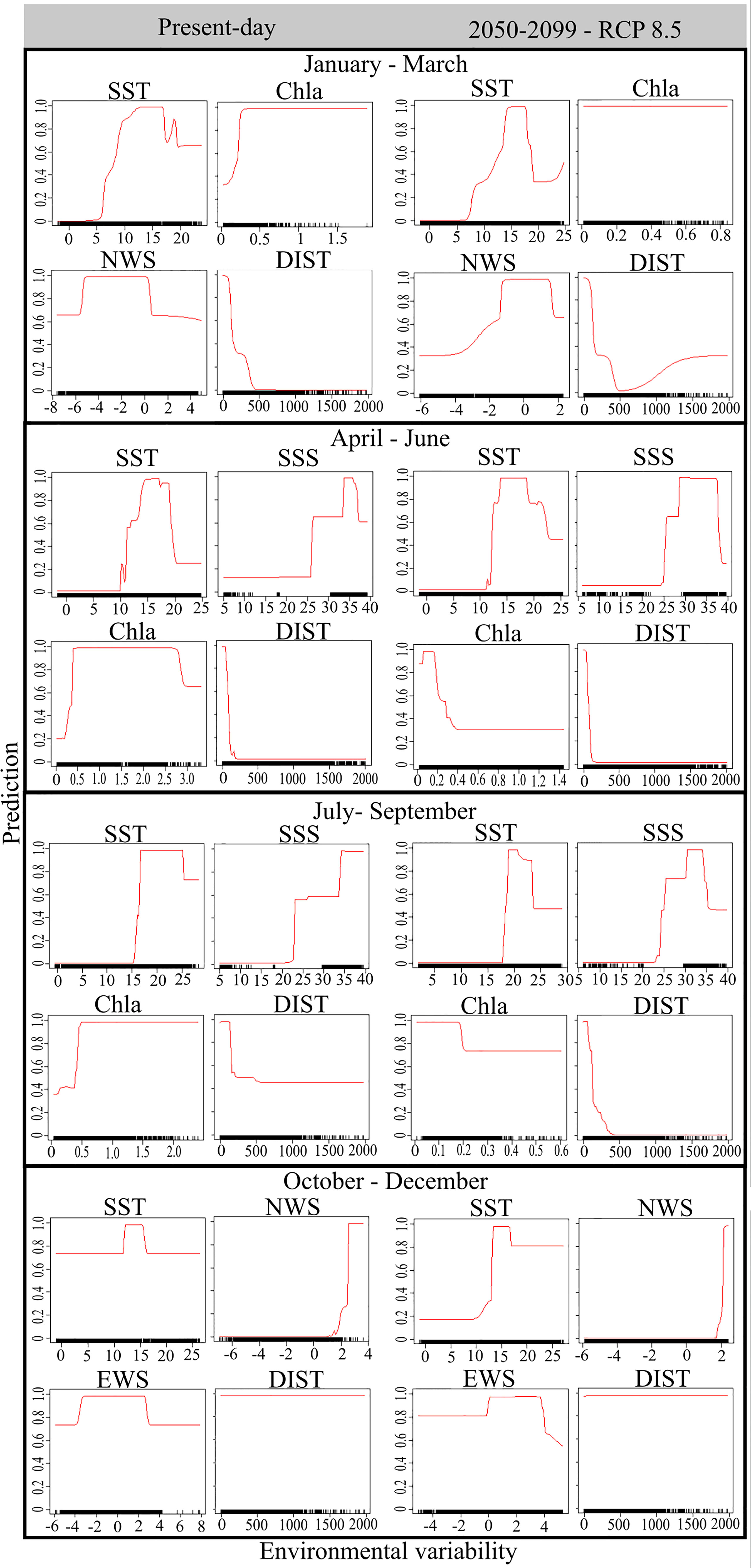
Figure 1 Relative importance of the selected environmental variables showing the plots of the effects of single variables for each seasonal period during the present-day and future scenarios. The y-axis reflects the suitability coefficients (solid red lines) of the ensemble model. The dashes on the x-axis, the so-called ‘rug’, indicates the density of points for the different suitability coefficients of each variable.
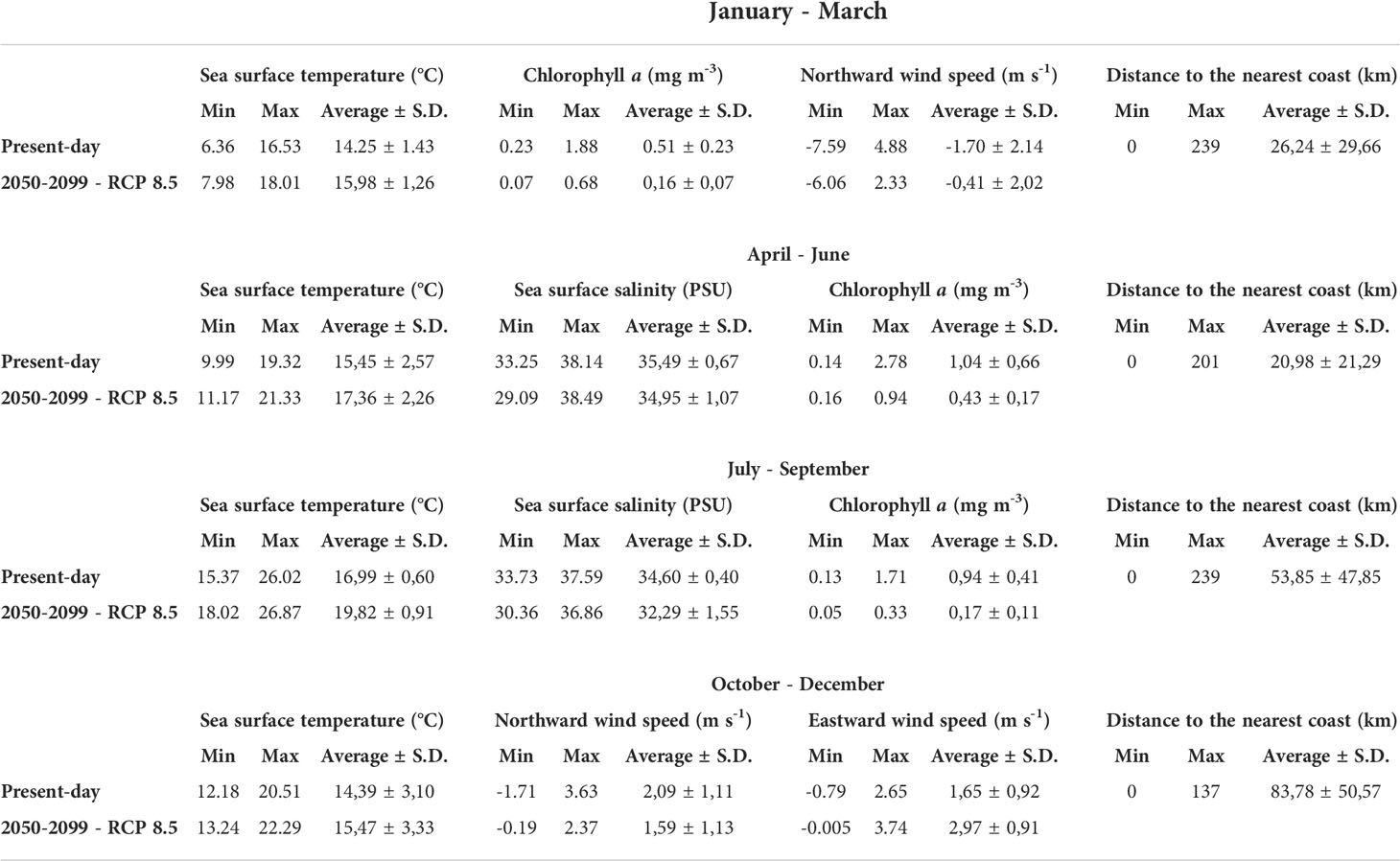
Table 2 Environmental conditions (averages from quarterly means) of present-day and future scenario for each season based on the occurrence dataset.
During spring (April to June), the ensemble model included the algorithms GAM, GBM and RF. The variable with the highest explanatory power was also SST (0.35), followed by DIST, Chl-a and sea surface salinity (SSS) (Table 1 and Figure S4). For the present-day spring, the suitable spawning areas were those with SST between 11 and 18°C, SSS between 26 and 38 PSU, Chl-a > 0.14 mg m-3 and DIST < 200 km (Figure 1 and Table 2). For the future spring scenario, the explanatory power of the environmental variables followed the same order of importance as that of the present-day condition, although SST importance increased to 0.45 (Table 1). Future habitat suitability will also follow the same optima observed for the present-day condition, except that low suitable spawning areas were forecasted in regions where Chl-a will be above 0.4 mg m-3 (Figure 1 and Table 2).
In the summer (July to September), the algorithms included in the ensemble model were GAM, GBM, CTA and RF. SST had the highest explanatory power (0.68) in the present-day condition, followed by Chl-a, DIST and SSS (Table 1 and Figure S5). Suitable areas for spawning were regions with SST between 16 to 25°C, SSS > 23.5 PSU, Chl-a > 0.4 mg m-3 and DIST < 200 km (Figure 1 and Table 2). For the future summer scenario, SST also had the highest explanatory power (0.66), followed by DIST, SSS and Chl-a (Table 1). Habitat suitability for spawning was forecasted to be in regions where SST will warm up to 18-24°C, SSS will be above 24 PSU, Chl-a will range between 0.05 and 0.35 mg m-3 and DIST will be less than 200 km.
The ensemble models for the autumn (October to December) included the algorithms GAM, GBM, CTA and RF. NWS was the most important predictor, accounting to 0.69 of explanatory power, followed by DIST, SST and eastward near-surface wind speed (EWS) (Table 1 and Figure S6). For the present-day autumn, the environmental optima for sardine spawning were predicted to be in areas under the influence of winds predominantly blowing from the south with speeds between 2 to 3 m s-1 (predominant positive NWS). These areas are concomitantly influenced by winds blowing from both west and east, with speeds between -4 to 3 m s-1 (from positive to negative EWS), where SST ranges between 12 and 15°C, regardless the DIST (Figure 1 and Table 2). For the future autumn scenario, the explanatory power of the environmental variables followed the same order of importance as that of the present-day condition, although SST importance increased to 0.44 (Table 1). Habitat suitability for spawning was forecasted to be also in areas under the influence of winds predominantly coming from the south with speeds above 2 m s-1 (predominant positive NWS). These areas will also be influenced by winds blowing from west with speeds between 0.1 to 4 m s-1 (predominant positive EWS), where SST will warm up to 13-17°C, regardless the DIST (Figure 1 and Table 2).
Seasonal suitability mapping and predictive shifts based on the IPCC future climatic scenario (2050-2099 - RCP 8.5)
According to our models, a wider range of spawning habitat with high suitability was predicted to occur during winter. From spring to summer suitability decreased within most of the distribution and increases again during autumn. This pattern is common to both present-day conditions and future scenarios (Figure 2). Overall, it was forecasted that habitat suitability for sardine spawning will increase northwards in the future, regardless the season.
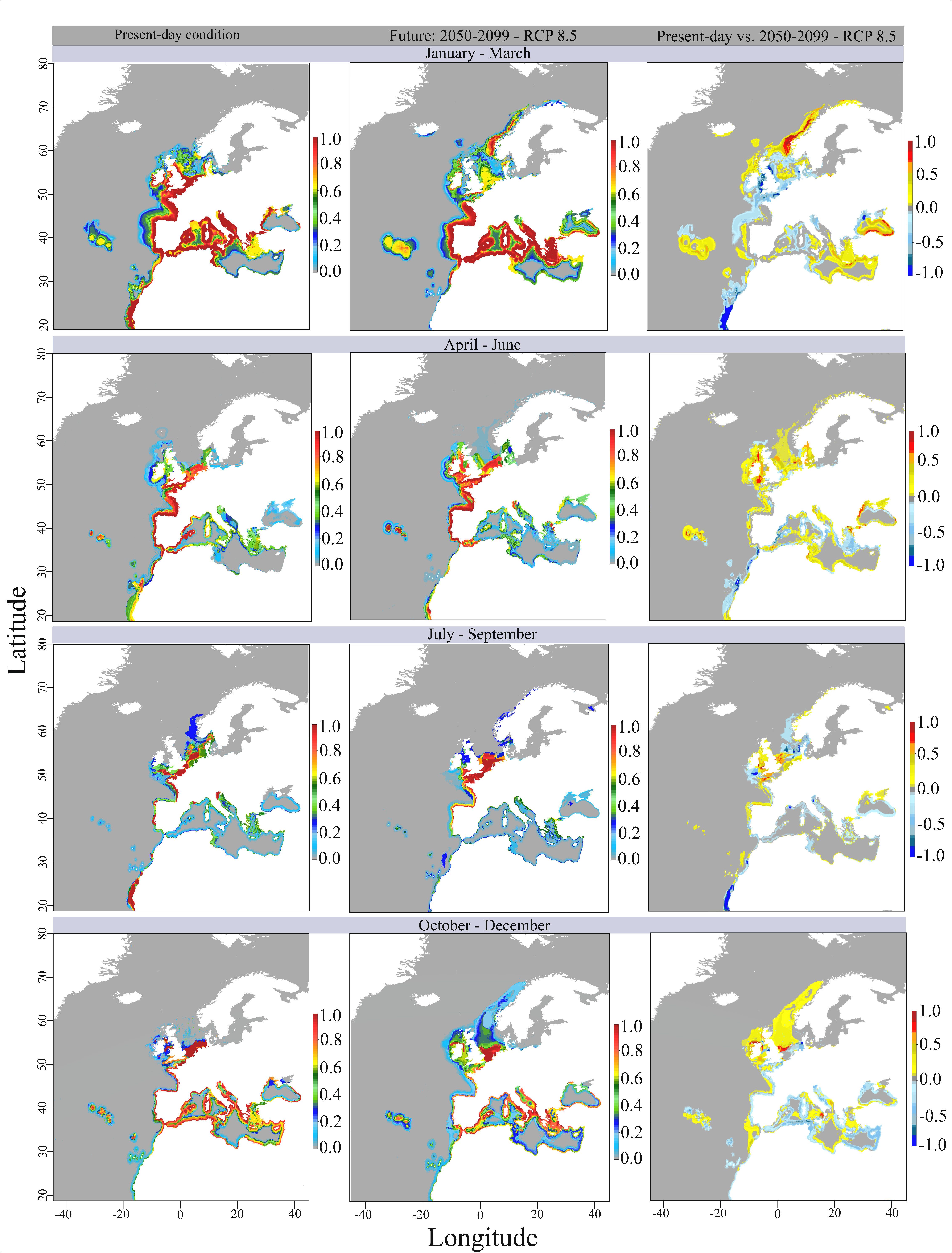
Figure 2 Maps of suitability indicating the variability in the probability of occurrence of eggs and larvae of Sardina pilchardus (from 0 to 1) as predicted by the seasonal ensemble models according to the present-day and future scenarios (first and second columns). Maps of shifts in suitability (third column) indicating gains and losses in the probability of occurrence of eggs and larvae as revealed by the differences between the present-day and 2050-2100 scenario under RCP 8.5. Geographic coordinate system: WGS 1984.
During the present-day winter, the highest suitability for spawning (> 0.5 to 1) is predicted to occur in the neritic zone along the coasts of Portugal, Bay of Biscay, around Ireland and UK, the southern North Sea, along the Atlantic African coast, as well as in the western Mediterranean, Adriatic and Aegean Seas, and western Black Sea. Suitability was also estimated to be high close to the Azores and Canary Islands. For the future winter scenario, suitability for spawning was projected to only be high closer to the coasts of Portugal, Bay of Biscay, the western Mediterranean, Adriatic and Aegean Seas (Figure 2).
It was projected that habitat suitability for spawning would decrease by -0.1 to -1 around Ireland and UK, in the southern North Sea, in the northern Black Sea, and in the Atlantic African Coast. Suitability losses from -0.1 to -0.6 were also projected off the Bay of Biscay and a bit far from the coasts of the western Mediterranean. Suitability gains of +0.1 to 1 will be observed around the Azores Islands, in the sea south Iceland, along the coast of Portugal, in the eastern Mediterranean and eastern Black Sea, as well as northward along the cost of Norway (Figure 2). Moreover, the total suitable area for spawning (>0.25) is ~4,706,000 km2 during winter for both present-day and future scenario. However, regionally, we forecasted that a severe spawning range contraction of 72.6% will be observed in the Atlantic African coast (Table 3). Contractions were also forecasted for the Iberian Peninsula, eastern Ireland, English Channel and North Sea and western Mediterranean. Spawning range expansions were forecasted to occur along the Norwegian coast (+83.25%) and in the Eastern Mediterranean (+26.51%).
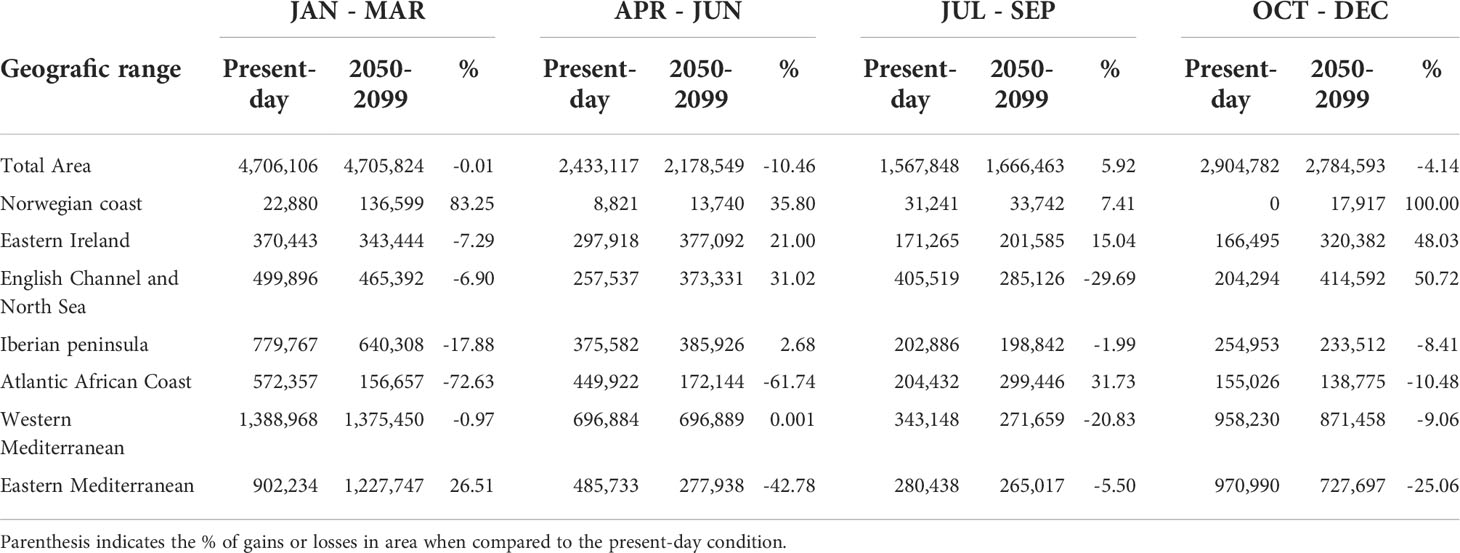
Table 3 Seasonal spawning areas calculated in square kilometres (Km2) showing suitable habitats under present-day conditions and future climatic scenario (2050-2099) considering overall suitability between >0.25 and 1.
In the present-day spring, the highest suitability for spawning (> 0.5 to 1) is predicted to occur in the neritic zone around the Iberian Peninsula, Bay of Biscay, English Channel, southern North Sea, along the Sahara and Moroccan coasts, as well as close to the Azores, Madeira and Canary Islands (Figure 2). For the future spring scenario, areas of high suitability for spawning will be predictably similar to those observed in the present-day condition, except by a higher suitability forecasted in the western UK and around Ireland (Figure 2).
Suitability gains of +0.1 to 1 are forecasted in most part of the northeast Atlantic coast, from Portugal to Norway; in the south Mediterranean, Adriatic and Black Seas, and in the Azores (Fig 3). Suitability losses from -0.1 to -1 were also forecasted along the Atlantic African coast and in the Aegean Sea. The total suitable area for spawning (>0.25) is forecasted to decrease by 10.46% during spring, especially due to spawning range contractions of 61.7% and 42.8% observed in the Atlantic African coast and eastern Mediterranean, respectively. Spawning range expansions were forecasted to occur along the Norwegian coast (+35.8%), English Channel and North Sea (+31%), eastern Ireland (+21%) and Iberian Peninsula (+2.68%) (Table 3).
For the present-day summer, restricted areas of high suitability for spawning (> 0.5 to 1) were estimated for near-coastal areas of the Atlantic coasts of Portugal, Spain and France, in the southern North Sea, in the Sahara coast, as well as closer to the coasts of the Adriatic and Aegean Seas (Figure 2). Most of the range in suitable habitats were characterized by occasional or rare spawning habitats with suitability below 0.4. In the future summer, areas of high suitability for spawning were projected be maintained except those in coastal waters off the Sahara(Figure 2).
Suitability gains of +0.1 to +1 were projected from Portugal up to the southern North Sea, around UK, in the northern Black Sea, in specific areas along the Norwegian coast and White Sea, as well as in the Azores, Canary and Madeira Islands (Fig 3). Suitability losses from -0.1 to -1 were projected along the Atlantic African coast, in the sea south Ireland, in the western North Sea and south Black Sea, and in most part of the Mediterranean coast (Figure 2). The total suitable area for spawning (>0.25) is forecasted to increase by 5.92% during summer, especially due to spawning range expansions of +31.7%, +15% and +7.41% observed in the Atlantic African coast, eastern Ireland and Norwegian coast, respectively. Spawning range contractions were forecasted to occur along the English Channel and North Sea (-29.7%), western Mediterranean (-20.8%), eastern Mediterranean (-5.5%) and Iberian Peninsula (-2%) (Table 3).
The highest suitability for spawning (> 0.5 to 1) in the present-day autumn was predicted to occur closer to the Atlantic coasts of Portugal, Spain and France, southern North Sea, closer to the coasts of northwest and southeast Black Sea, in most part of the Mediterranean coast, as well as close to the Azores and Madeira Islands (Figure 2). For the future autumn scenario, it was projected that overall suitability decreases in most spawning range. Areas of high suitability for spawning in the Atlantic will be similar to those observed for the present-day condition. However, high suitability is mainly forecasted to be maintained in the northern Mediterranean (Figure 2). Moreover, areas of low suitability (0.1 to 0.3) were projected along the Norwegian coast, characterizing this area as a rare spawning habitat in the RCP8.5 scenario.
It was projected that suitability will increase by +0.1 to +1 in the North Sea and around Ireland and by +0.1 to +0.5 along the Norwegian coast. Suitability losses from -0.1 to -0.8 were projected to occur closer to the Atlantic coasts of Portugal, Spain and France, in the southern North Sea, and in most part of the Mediterranean coast and Black Sea. The total suitable area for spawning (>0.25) was projected to decrease by 4.1% during autumn, especially due to spawning range contractions of 25%, 10,5%, 9% and 8.4% observed in the eastern Mediterranean, Atlantic African Coast, western Mediterranean and the Iberian Peninsula, respectively (Table 3). Expansions of the range of suitable spawning areas was projected to occur along the Norwegian coast (+100%), English Channel and North Sea (+50.72%), and eastern Ireland (+48.03%) (Table 3).
Discussion
The role of environmental drivers on the European sardine spawning habitats under a seasonal approach
Here we projected that areas of increased probability of spawning exhibited high variability among seasons and between present-day and future scenarios because current environmental characteristics changed relative to the future especially regarding warming, shifts in productivity and salinity, as well as in the intensity and directions of favourable winds. The environmental variables that presented the greater influences in spawning habitats were SST and DIS, as they were selected by all models. Therefore, we projected that potential “hot-spot” spawning areas for the European sardine are located along the neritic zone (<250 km from the nearest coast), comprising the entire spawning range of the species, where they take advantages of the year-round cold temperatures of the northeast Atlantic and of the warmer temperatures during the summer in the Mediterranean Sea.
Sardines are acknowledged to have a temperature-dependent spawning along their distribution range (Tugores et al., 2011; Abdelouahab et al., 2021; Gordó-Vilaseca et al., 2021). In addition, it is commonly reported in the literature that sardines take advantage of shelf upwelling systems with cold water and enhanced productivity, and under the influence of favourable winds that minimize offshore transport (Olivar et al., 2003; Santos et al., 2005; Stratoudakis et al., 2007; Giannoulaki et al., 2014; Abdelouahab et al., 2021).
According to our models, temperature ranges for spawning varied between 6°C and 25°C for the present-day condition, and between 11°C and 24°C for the future scenario (Table 2). The lowest SST values are associated with winter temperatures in the North Sea and the highest SST values are associated with summer temperatures in the Mediterranean Sea. The lowest limit of temperature for spawning purposes is not clear as there is no record of spawning during winter temperatures in the North Sea (the northern present-day habitat). However, our predicted SST optima that ranges from 13°C to 16°C from Autumn to Spring is in accordance with the range reported by Bernal et al (2007), associated with temperatures between 13.5 and 15°C for spawning in Atlantic waters. As well, our predicted summer SST optima, that ranges from 15°C to 25°C, is in accordance with the positive effects that summer temperatures (between 13°C to 26.3°C) have on sardine’s spawning in the Mediterranean Sea (Palomera et al., 2007; Schismenou et al., 2016; Gordó-Vilaseca et al., 2021). The projections emphasize that habitat suitability for sardine’s spawning, for both present-day and future scenarios, is higher and widely distributed during colder months, as observed during winter, when sardines spawn further from the coasts when compared to the other seasons, similarly as observed in regional studies (Coombs et al., 2006; Somarakis et al., 2006; Stratoudakis et al., 2007; Giannoulaki et al., 2014). Suitability starts to decrease from spring to summer, especially in the Mediterranean and Black Seas, where SST becomes higher and above the optima for spawning, and starts to increase again during autumn, as SST becomes lower in the Mediterranean, Black Sea and Eastern Atlantic (Figure 2).
Chlorophyll-a was the third most important environmental variable, not selected only by the autumn model (Table 1). Actually, sardine populations maximize egg production when primary productivity is enhanced under two hypotheses: adults start the phytoplankton feeding strategy to enhance energy flow to reproduction or adults spawn in favourable environmental conditions for proper development of early stages (Ganias, 2009; Fernández-Corredor et al., 2021). Here, we did not find a clear pattern concerning the influence of Chl-a in spawning neither in present-day or future scenario. Some studies have also acknowledged that sardine spawning is not closely tied to plankton production and such relationship is variable from area to area. In the Mediterranean Sea, for example, higher probabilities of sardine eggs presence in the Spanish coast were observed under moderate Chl-a values (Turgores et al., 2011), whilst negative correlations between Chl-a and egg/larvae abundance were found in the Gulf of Tunis (Zarrad et al., 2008). In Atlantic waters, from the Bay of Biscay northwards, suitable spawning temperatures coincide with increased seasonal plankton production (Coombs et al., 2006; Stratoudakis et al., 2007; Giannoulaki et al., 2014). On the other hand, in the English Channel, although temperatures are always within the spawning range, summer spawning is reduced due to the drop in plankton abundance (Coombs et al., 2006). During winter months off Portugal, plankton production is minimized, but spawning is enhanced as water column has maximum stability and minimum offshore transport (Coombs et al., 2006). Along the Atlantic African coast, spawning mostly occurs in times of upwelling providing lower temperatures and high plankton production (Coombs et al., 2006; Stratoudakis et al., 2007; Giannoulaki et al., 2014).
Our predictions for the present-day condition also emphasized that spawning suitable areas can be observed under different ranges of Chl-a optima and this range varies from season to season. The highest habitat suitability for spawning from winter to summer were observed in regions were Chl-a presented higher values (a positive relationship), such as around the Iberian Peninsula, Bay of Biscay, English Channel, southern North Sea, northern Mediterranean and Atlantic African coast (Figure 2). During the future scenario, these same regions were maintained highly suitable, however, Chl-a dropped to low and moderate values, evidencing a negative relationship between Chl-a and sardines in the future (Figure 2). Such negative relationship was not observed in the African coast. In fact, the present-day influence of northeasterly winds and the Ekman transport along the Atlantic African coast favour sardine spawning along the Moroccan coastal upwelling during the entire year, due to the presence of cold surface waters and high primary productivity (Abdelouahab et al., 2021). However, for this region, productivity has been projected to decrease in the future (Yool et al., 2015), coinciding with losses in habitat suitability across all seasons. Thus, higher SST and low productivity were predicted to decrease spawning probabilities along the African coast in the future regardless the season.
Winds were predominantly important during autumn (both northward and eastward winds) (Table 1). Wind-driven flow are acknowledged to have effects on the transport of sardine early life stages (Santos et al., 2004). The spawning areas during autumn are influenced by winds predominantly blowing from the south to the north concomitantly with the influence of winds blowing from west and from east. Thus, it is possible that the intensity and directions of these winds play a greater role in the retention of eggs and larvae in appropriate environments closer to the coasts of the Southern North Sea and Northern Mediterranean where SST range (10 to 15°C) is favourable for spawning when compared to other regions. Winds lose importance during the onset of winter (influenced only by northward winds). Winds predominantly blowing from the north to the south seems to retain eggs and larvae within upwelling systems with enhanced productivity. Although upwelling systems are predicted to be favourable areas for sardine spawning during winter, some studies off the Portuguese coast have suggested that egg and larval survival are negatively influenced by northerly winds and upwelling events (Borges et al., 2003, Santos et al., 2005) and positively influenced by prey abundance (Santos et al., 2005). On the other hand, along the Atlantic African coast, recruitment success increases with upwelling intensity and low to moderate wind speeds (Roy et al., 1992).
Sea surface salinity was detected in our model as an important environmental variable during spring and summer months (Table 1). The influence of salinity in sardine spawning habitats during these warmer months is possibly related to increased rainfall across Europe, which consequently increases river runoff towards coastal regions, lowering coastal salinities and creating patches of unsuitable regions. Although habitats of sardine’s early life stages are restricted to coastal areas with many estuaries, it is reported that eggs and larvae have lower tolerance to lower salinities, similarly as predicted by our models (Palomera et al., 2007; Ramos et al., 2009). Here, sardines were predicted to spawn predominantly in salty water during both present-day and future scenario. Sardines spawn occasionally or rarely in regions where SSS is below ~25 PSU and above ~36 PSU, such as in the Black Sea and in most Mediterranean Sea, respectively. High suitability for spawning was predicted in areas where salinity ranged from ~34 to ~36 PSU, such as observed in the northeast Atlantic coast (Figure 2). A positive relationship of sardine spawning areas (represented by egg abundance) and salinity were observed in the Atlantic coast of northwest Africa (Ettahiri et al., 2003; Abdelouahab et al., 2021), off Portugal (Angélico et al., 2004) and in the Bay of Biscay (Planque et al., 2007), where favourable conditions were reported to be between ~34 to 36.4 PSU.
Seasonality of sardine spawning and shifts on habitat suitability towards the future
To the best of our knowledge this is the first study forecasting the future spawning range of a small pelagic fish species, the European sardine, along their entire distribution range (across the Northeast Atlantic and Mediterranean Sea). Some limitations of the existing models to extrapolate the present-day results to future scenarios are discussed: (I) the uncertainty in the projections due to low occurrence records, and (II) the simplified nature of the projected models, which did not account to the full range of processes shaping spawning phenology, such as larval transport and retention.
According to our projections spawning probability is pronounced during winter months (January to March) in the northeast Atlantic, when a wider range of moderately to highly suitable habitats (>0.6) can be observed in both present-day and future scenario (Figure 2). Suitability for spawning is maintained higher during spring in the northeast Atlantic and lacks power from summer to autumn. Although we gathered a lower number of occurrence records for this period, our present-day projections are in close accordance with ichthyoplankton surveys datasets across the northeast Atlantic, what increases modelling certainties. Here, we considered the year split in seasons, which usually considers one month earlier than that proposed by our study. The seasons here followed predefined projections available in physical and biogeochemical climate models for the future scenario (Scott et al., 2016). In the English Channel, spawning intensifies from summer through autumn (Coombs et al., 2006); in the eastern Biscay and northern Spain from winter to spring (Giannoulaki et al., 2014); in Portugal from winter to summer, with pronounced intensity during winter (Stratoudakis et al., 2007); and along the Morocco coast from winter to spring (Coombs et al., 2006). The southern North Sea was projected to be an important, year-round spawning area for sardines in the present-day and projected future conditions. This region is always within the environmental optima for spawning regardless the seasonal shifts in SST and productivity across the Atlantic. The European sardine and other SPF, such as the European anchovy (Engraulis encrasicolus), have expanded their distribution towards the North Sea since the mid- 1990s, even using this region as spawning grounds (Beare et al., 2004; Kanstinger and Peck, 2009; Fréon et al., 2009; Alheit et al., 2012).
We forecasted that by 2050-2099 under RCP 8.5 the Norwegian coast will become suitable for spawning, especially during autumn and winter months (Figure 2). From autumn to winter, the pronounced habitat suitability gains (from +0.1 to +1) towards the Norwegian coast in the future is possibly related to the warming and the intensification of northward and eastward winds blowing especially from the south and from the west, respectively. On the other hand, the warming of winter temperatures, decreases the suitability for spawning off northern Portugal and Bay of Biscay, and around UK. During autumn, habitat suitability for these same regions increases possibly due to unpredicted change in SST towards the future and the intensification of favourable winds in these regions. From spring to autumn, the slight habitat suitability gains from southern Portugal to the North Sea and towards the Norwegian coast in the future may be attributed to a slight warming that does not overpass the optimal, as well as to the increased productivity and less salty water masses (< 36 PSU) in the eastern Atlantic.
During the winter in Mediterranean Sea, our projections ascertained that a wider range of moderately to highly suitable habitats (>0.6) can be observed in both present-day and future scenario (Figure 2). This is also in accordance with many studies reporting that spawning intensity in the Mediterranean is higher during winter due to lower suitable temperatures for spawning (Olivar et al., 2003; Somarakis et al., 2006; Ganias et al., 2007; Giannoulaki et al., 2014). Conversely, spawning intensity in the Mediterranean decreases from spring to summer and increases again during autumn. In the Mediterranean, autumn and winter SST do not change much between present-day and future scenario. The winter suitability gains observed in the eastern Mediterranean might be attributed to increased productivity and intensification of favourable winds, while most autumn suitability loss may be attributed to intensification of unfavourable winds.
In the Mediterranean, spring and summer SST increases towards the future. The spring suitability gains observed in the southern Mediterranean may be attributed to increased productivity and salinities between 34-36 PSU, while in the northern Mediterranean, suitability losses may be attributed to decreased productivity. In addition, most summer suitability losses observed in the Mediterranean may be attributed to warming and lower productivity. The spawning habitats for sardines, and other SPFs (e.g. the European anchovy E. encrasicolus and the round sardinella Sardinella aurita) are acknowledged to have been markedly reduced in the NW Mediterranean over the last decades due to rising temperatures and decreased primary productivity (Maynou et al., 2019; Salat et al., 2019; Pennino et al., 2020; Gordó-Vilaseca et al., 2021). In addition, projections for the end of the twenty-first century showed that spawning habitat suitability is likely to decrease slightly under RCP 8.5 for other SPFs, such as for the anchovy in the NW Mediterranean, due to changes in SST, SSS and Chl-a, possibly resulting in future spawning habitat contraction (Maynou et al., 2020b).
A global perspective on the role of climate change in spawning habitat expansion and contraction is still difficult to estimate as there are very few studies forecasting changes in spawning habitat suitability of small pelagic fishes along the northeast Atlantic and Mediterranean (Maynou et al., 2020b). For the European Sardine, future range expansions and contractions in spawning areas were highly variable among seasons and it is likely ruled by the variability in SST towards the future. Expansions were observed in regions where SST will increase enough to be within optimal values of each season. These expansive patterns are more pronounced in the eastern Mediterranean during winter, eastern Ireland, English Channel and North Sea during spring and autumn, African Atlantic coast during summer, as well as along the Norwegian coast during all seasons. This confirms that along the northeast Atlantic and during the winter in the Mediterranean, the combination of warmer annual patterns and winter temperatures may allow sardines to overcome thermal constraints during spawning and recruitment (Dahlke et al., 2020). Contractions were particularly observed in regions where SST will become warmer than the optima for each season. Contraction patterns were pronounced around the Iberian Peninsula during winter, eastern Mediterranean during spring and autumn, western Mediterranean, English Channel and North Sea during summer and the Atlantic African Coast during winter, spring and autumn. This confirms that if warming continues along these regions and time periods, sardines will probably be pushed to “climatic refuges” in the northern regions (Ben Rais Lasram et al., 2010), confirming the projections of our study.
Thus, it is clear in our projections that the total area for sardine spawning will suffer slight contractions of 10.46% and 4.14% during spring and autumn, respectively; will be maintained the same during winter; and will suffer a slight expansion of 5.92% during summer (Table 3). In addition, the Atlantic African coast will become less productive and warmer in the future, being out of the optima for sardine spawning from autumn to spring, resulting in spawning habitat contractions of 10.5% during autumn, 72.6% during winter and 62.7% during spring (Table 3). Therefore, we emphasize that although a northward shift (to higher latitudes) in the spawning range was projected by 2050-2099 under RCP 8.5, a contraction of the total area used for spawning from autumn to spring was projected in the future.
4.3. Match-mismatch of adult distribution and spawning habitats under future scenarios and implications for sardine stock management
To date, there is no evidence of mismatch between adult range distribution and spawning habitats under future scenarios. According to Lima et al. (2022), a poleward shift in the centre of the distribution range of adult sardines is projected by 2100 under RCP 8.5 along the entire coast of Norway and into the White Sea, as also confirmed by Schickele et al. (2021). A poleward shift in spawning habitats was also projected along the Norwegian coast, matching the future potential “hotspots” for sardine stocks. The shift in average temperature in the northern North Sea noticed by 1985 (SST increased from 6.5 °C to 8.5 °C on average) associated with the strengthening of the North Atlantic Oscillation, was accompanied by a gradual increase in sardine abundance at higher latitudes (Beare et al., 2004; Alheit et al., 2012). Actually, adult sardines are eurythermal clupeoids that can tolerate temperatures ranging from 8°C to 26°C (Coombs et al., 2006; Zwolinski et al., 2010; Garrido et al., 2016; Garrido et al., 2017), with an optimal range between >10°C and 22°C (Lima et al., 2022). Although Lima et al. (2022) did not consider seasonal approaches to create large-scale spatial projections, the predicted SST optima for adult distribution is close to the SST optima for spawning, which we forecasted to be between 11°C and 24°C in the future scenarios.
Some studies have reported that the shift of European sardine and anchovy (E. encrasicolus) populations to higher latitudes after mid-1990s is possibly due to loss of colder winters and warming, better supporting spawning and survival of early stages (Alheit et al., 2012; Petitgas et al., 2012; Giannoulaki et al., 2014). Temperate small pelagic fishes are also acknowledged to behaviourally track their optimal thermal conditions during long and lasting climatic shifts by migrating polewards (Silva et al., 2014; Han et al., 2020; Sebastian et al., 2020). These epipelagic species have heightened phenological plasticity as many of them are batch spawners that reproduce frequently with protracted spawning season. Also, they are exposed to larger changes in climate as they live in the upper water column, and their short life spans allows faster genetic adaptation to changing conditions (Peck et al., 2013; Asch, 2015). Genetic markers have revealed adaptive changes that favour key physiological factors shaping life history as SPF move to new areas. Baltazar-Soares et al. (2021), proposed that there is a temperature- and O2-dependent distribution of haplotype frequencies (mitochondrial genes directly involved in metabolic pathways), revealing signatures of selection of European sardines to cold temperatures and low-oxygen levels. The authors concluded that sardines may be able to follow environmental optima and shift their distribution northwards if SST warms up.
One of the key steps towards an ecosystem‐based approach to fisheries management is the monitoring of fish spawning habitats (Peck et al., 2009). Spawning is not only affected by changes in adult population size, but also by environmental-driven changes, which may indirectly alter recruitment and affect fisheries via bottom-up (e.g. trophodynamic processes impacting larval prey availability) and top-down controls (e.g. intensity of sardine larvae predation) (Costalago et al., 2015; Basilone et al., 2017; Peck et al., 2021). Therefore, knowledge on the spawning habitats of SPF, both in present-day and future scenarios, is crucial for understanding fish stock fluctuations, allowing them to be effectively managed and conserved. However, highly suitable habitat for spawning does not necessarily imply that eggs and larvae are highly abundant. This is because habitat suitability models have no ability to account for larval transport, larval abundance, and genetic adaptation or to predict how and whether sardine eggs and larvae would be viable in new locations where they will be influenced by the presence of other species and new physical and environmental conditions. For example, the gradual warming of the North Atlantic and the Norwegian currents towards the future, is likely to change tidal-mixing, river plumes, upwelling zones and shelf break fronts, altering productivity and food web across the region (Peck et al., 2021). Such new conditions will possibly affect the distribution and abundance of sardine early life stages through bottom-up and top-down controls. In this sense, the evaluation of historical datasets on the abundance of sardine eggs and larvae over the last decades is important to fulfil some knowledge gaps regarding the future of spawning habitats.
The phenology of SPF spawning and plankton production may evolve differently under climate change for many species, potentially leading to seasonal mismatches, affecting mortality and recruitment (Fréon et al., 2009; Asch, 2015; Checkley et al., 2017). In our study, we were not able to conclude whether the phenology of spawning will change towards the future through our projections, since no clear relationship between Chl-a and spawning habitat suitability was found; a similar pattern also observed for the relationship between plankton production and egg/larval abundance (Coombs et al., 2006; Stratoudakis et al., 2007; Giannoulaki et al., 2014). However, our forecasts evidenced that in most part of the distribution range, sardines will continue to spawn regardless food availability as SST will be maintained optimal for spawning during colder months, leading to the negative relationship between Chl-a and suitable habitats for spawning. An exception was projected along the Atlantic African coast, where plankton productivity strongly declined and SST was too will be above the optimal for spawning in addition to a strong decrease in plankton productivity. Thus, the positive relationship between Chl-a and suitability is possibly a reflection of the negative relationship between SST and suitability. This suggests that the temperature-dependent spawning of sardines will be maintained in the future and sardines will probably spawn in locations of optimal temperatures regardless food availability unless rapid evolution occurs to synchronize all these events. Therefore, if climate change increases the frequency of these mismatches between plankton availability and sardine early life stages, sardines’ life story strategy will need to withstand decreased foraging efficiency, slower growth, starvation, and lower survival rates, what is likely to lead to poor recruitment and declines in the abundance of adult stocks (Lima et al., 2022). Nevertheless, local adaptation may play a pivotal role in the match-mismatch processes between fish populations and local variation, with relevance in areas whose environmental conditions overcome fish ecophysiological limits.
This is the first study to provide climate change projections of the gains and losses in suitable areas for spawning by European sardine. This may provide a basis to define stronger measures to consistently monitor and to better manage areas where contractions of spawning habitats are predicted to occur, such as the Atlantic African coast and the Mediterranean Sea. Controversially, the largest sardine stocks with annual landings around 700,000 t are located in central Morocco (Africa), followed by the Mediterranean stocks, with annual landings around 80,000 t (Silva et al., 2015). Our projections suggest that these habitats will no longer be suitable for spawning by European sardine in the future (Table S4). Therefore, long-term monitoring of spawning activity, especially within these important sardine stocks, is crucial to propose better measures for the sustainability of sardine fisheries.
Conclusion
A poleward shift in the suitable habitats for the spawning of European sardine was projected to occur by 2050-2099 under RCP 8.5. This included a prominent expansion of suitable spawning habitats along the coast of Norway during winter and autumn but a contraction of the total spawning area from autumn to spring due to large losses of suitable habitat along the Atlantic African coast and Mediterranean Sea in the future. The environmental variables with highest explanatory power were sea surface temperature, distance to the nearest coast, chlorophyll-a and near-surface winds and sea surface salinity, with seasonal shifts in variables importance and contribution. Nevertheless, the environmental variables that presented the greater influence in spawning habitats were SST and DIST, being selected in all seasonal models and reflecting the temperature- and coastal-dependent spawning of sardines. Chlorophyll-a was the third most important driver, being selected from winter to summer. Winds were predominantly important during autumn, whilst sea surface salinity was an important environmental variable during spring and summer months.
In the present-day condition, “hotspots” for sardine spawning were estimated to occur in regions of high productivity (Chl-a > 0.14 mg m-3) and salty waters, where SST varies between 6°C and 18°C from autumn to spring; and between 16°C and 25°C during summer, under the influence of favourable winds that allow eggs and larvae to be retained within 250 km of the coast. In the future, environmental optima for spawning were projected to occur in regions where SST varies between 11°C and 18°C from autumn to spring; and between 18°C and 24°C during summer. However, a negative relationship is likely to occur between plankton productivity and habitat suitability, emphasizing that warming may impair spawning in regions of high food availability regardless the season. Therefore, losses in suitability and severe range contractions are forecasted to occur in the upwelling system of the Atlantic African coast and across the Mediterranean Sea, regions that in the present-day support the largest stocks of European sardine. So, will warming impair successful recruitment in these regions in the near future?
Due to the effects of potential overexploitation by fisheries, density-dependent processes and climatic variability (e.g. Atlantic Multidecadal Oscillation and North Atlantic Oscillation ), the spatiotemporal dynamics of spawning by sardine and other SPF tends to be highly variable which makes it challenging to make long-term projections of climate change impacts (Peck et al., 2013)., It is important, therefore, to maintain long-term time series of the occurrences of eggs and larvae of sardine and other species to better understand how extrinsic (environmental) and intrinsic (physiology, life history strategy) combine to constrain suitable spawning locations and times. These field data provide us the ability to estimate the extent to which spawning regions have shifted over the last decades and whether warming may induce putative changes in the physiology/behaviour of early life stages. Long-term field data will also enable us to look for signatures of selection to new conditions to understand, for example, the potential roles of acclimation and/or phenotypic or genetic adaptive responses by local populations as mechanisms to cope with historical and/or future climate change.
Data availability statement
The original contributions presented in the study are included in the article/Supplementary Material. Further inquiries can be directed to the corresponding author.
Author contributions
AL: Conceptualization, Methodology, Formal analysis, Data Curation, Writing - Original Draft, Investigation. SG: Project administration, Funding acquisition, Investigation, Validation, Data Curation. IR, DR and MA: Investigation, Validation, Data Curation. EG: Project administration, Funding acquisition, Investigation, Data Curation; MP: Writing - Review & Editing, Investigation, Validation, Visualization. GS: Project administration, Funding acquisition, Investigation, Supervision, Conceptualization, Writing - Original Draft. All authors contributed to the article and approved the submitted version.
Ackowledgments
The authors acknowledge the European Regional Development Fund (FEDER) through the Lisbon's Regional Operational Programme (LISBOA-01-0145-FEDER-032209) and the Portuguese Foundation for Science & Technology (FCT) through the project PTDC/BIA-BMA/32209/2017 (SARDITEMP), the project PTDC/MAR/115226/2009 (MATRIX), the strategic project MARE-UIDB/04292/2020 granted to MARE (Marine and Environmental Sciences Centre) and through the project LA/P/0069/2020 granted to the Associate Laboratory ARNET. We also thank the Project LIFE06NAT/P/192 (BIOMARES), funded by the European Union LIFE Program and co-funded by the cement company SECIL, Companhia de Cal e Cimentos S.A., Portugal. AL acknowledges the European Union's (EU's) Horizon 2020 (H2020) research and innovation programme under the Marie Skłodowska-Curie grant agreement No 101038057. IEO surveys have been co-funded by the EU through the European Maritime and Fisheries Fund within the National Program of collection, management, and use of data in the fisheries sector and support for scientific advice regarding the Common Fisheries Policy. This study is a contribution to the FutureMARES (EU H2020 No. 869300) project, the IMPRESS (RTI2018-099868-B-I00) project, ERDF, Ministry of Science, Innovation and Universities - State Research Agency and also of GAIN (Xunta de Galicia), GRC MERVEX (nº IN607-A 2018-4). We also thank members of the ICES-PICES Working Group on Small Pelagic Fish (WGSPF) for helpful discussions.
Conflict of interest
The authors declare that the research was conducted in the absence of any commercial or financial relationships that could be construed as a potential conflict of interest.
Publisher’s note
All claims expressed in this article are solely those of the authors and do not necessarily represent those of their affiliated organizations, or those of the publisher, the editors and the reviewers. Any product that may be evaluated in this article, or claim that may be made by its manufacturer, is not guaranteed or endorsed by the publisher.
Supplementary material
The Supplementary Material for this article can be found online at: https://www.frontiersin.org/articles/10.3389/fmars.2022.956654/full#supplementary-material
References
Abdelouahab H., Berraho A., Baibai T., Larissi J., Charib S., Agouzouk A., et al. (2021). Long term variation of sardine sardina pilchardus spawning along the Atlantic coast of northwest Africa (21–26°N): characterization and spatiotemporal variability in spawning habitat. Oceanologia 63, 1–11. doi: 10.1016/j.oceano.2020.08.006
Ahmad R., Khuroo A. A., Charles B., Hamid M., Rashid I., Aravind N. A. (2019). Global distribution modelling, invasion risk assessment and niche dynamics of leucanthemum vulgare (Ox-eye daisy) under climate change. Sci. Rep. 9, 1–15. doi: 10.1038/s41598-019-47859-1
Alheit J., Pohlmann T., Casini M., Greve W., Hinrichs R., Mathis M., et al. (2012). Climate variability drives anchovies and sardines into the north and Baltic seas. Prog. Oceanogr. 96, 128–139. doi: 10.1016/j.pocean.2011.11.015
Araújo M. B., New M. (2007). Ensemble forecasting of species distributions. Trends Ecol. Evol. 22, 42–47. doi: 10.1016/j.tree.2006.09.010
Asch R. G. (2015). Climate change and decadal shifts in the phenology of larval fishes in the California current ecosystem. Proc. Natl. Acad. Sci. U. S. A. 112, E4065–E4074. doi: 10.1073/pnas.1421946112
Athanasiou P., van Dongeren A., Giardino A., Vousdoukas M. I., Ranasinghe R., Kwadijk J. (2020). Uncertainties in projections of sandy beach erosion due to sea level rise: an analysis at the European scale. Sci. Rep. 10, 1–14. doi: 10.1038/s41598-020-68576-0
Baltazar-Soares M., Hinrichsen H.-H., Eizaguirre C. (2018). Integrating population genomics and biophysical models towards evolutionary-based fisheries management. ICES J. Mar. Sci. 75, 1214–1234. doi: 10.1093/icesjms/fsx244
Baltazar-Soares M., Lima A. R., de A., Silva G. (2021). Targeted sequencing of mitochondrial genes reveals signatures of molecular adaptation in a nearly panmictic small pelagic fish species. Genes (Basel). 12, 1–17. doi: 10.3390/genes12010091
Barbet-Massin M., Jiguet F., Albert C. H., Thuiller W. (2012). Selecting pseudo-absences for species distribution models: How, where and how many? Methods Ecol. Evol. 3, 327–338. doi: 10.1111/j.2041-210X.2011.00172.x
Basilone G., Mangano S., Pulizzi M., Fontana I., Giacalone G., Ferreri R., et al. (2017). European Anchovy (Engraulis encrasicolus) age structure and growth rate in two contrasted areas of the Mediterranean Sea: the paradox of faster growth in oligotrophic seas. Mediterr. Mar. Sci. 15, 739–752. doi: 10.12681/mms.2059
Beare D., Burns F., Jones E., Peach K., Portilla E., Greig T., et al. (2004). An increase in the abundance of anchovies and sardines in the north-western north Sea since 1995. Glob. Change Biol. 10, 1209–1213. doi: 10.1111/j.1529-8817.2003.00790.x
Ben Rais Lasram F., Guilhaumon F., Albouy C., Somot S., Thuiller W., Mouillot D. (2010). The Mediterranean Sea as a “cul-de-sac”. endemic fishes facing Climate ChangeGlob. Change Biol. 16, 3233–3245. doi: 10.1111/j.1365-2486.2010.02224.x
Bentamy A. (2020). Copernicus Marine service. Product user manual 2, 21. Wind_Glo_Phy_Climate_L4_Rep_012_003.
Bernal M., Stratoudakis Y., Coombs S., Angelico M. M., de Lanzós A. L., Porteiro C., et al. (2007). Sardine spawning off the European Atlantic coast: Characterization of and spatio-temporal variability in spawning habitat. Prog. Oceanogr. 74, 210–227. doi: 10.1016/j.pocean.2007.04.018
Borges M. F., Santos A. M. P., Crato N., Mendes H., Mota B. (2003). Sardine regime shifts off Portugal: A time series analysis of catches and wind conditions. Sci. Mar. 67, 235–244. doi: 10.3989/scimar.2003.67s1235
Brosset P., Smith A. D., Plourde S., Castonguay M., Lehoux C., Van Beveren E. (2020). A fine-scale multi-step approach to understand fish recruitment variability. Sci. Rep. 10, 16064. doi: 10.1038/s41598-020-73025-z
Bruge A., Alvarez P., Fontán A., Cotano U., Chust G. (2016). Thermal niche tracking and future distribution of Atlantic mackerel spawning in response to ocean warming. Front. Mar. Sci. 3. doi: 10.3389/fmars.2016.00086
Buisson L., Thuiller W., Casajus N., Lek S., Grenouillet G. (2010). Uncertainty in ensemble forecasting of species distribution. Glob. Change Biol. 16, 1145–1157. doi: 10.1111/j.1365-2486.2009.02000.x
Cavanaugh K. C., Parker J. D., Cook-Patton S. C., Feller I. C., Williams A. P., Kellner J. R. (2015). Integrating physiological threshold experiments with climate modeling to project mangrove species’ range expansion. Glob. Change Biol. 21, 1928–1938. doi: 10.1111/gcb.12843
Checkley D. M., Asch R. G., Rykaczewski R. R. (2017). Climate, anchovy, and sardine. Ann. Rev. Mar. Sci. 9, 469–493. doi: 10.1146/annurev-marine-122414-033819
Coombs S. H., Smyth T. J., Conway D. V. P., Halliday N. C., Bernal M., Stratoudakis Y., et al. (2006). Spawning season and temperature relationships for sardine (Sardina pilchardus) in the eastern north Atlantic. J. Mar. Biol. Assoc. United Kingdom 86, 1245–1252. doi: 10.1017/S0025315406014251
Costalago D., Garrido S., Palomera I. (2015). Comparison of the feeding apparatus and diet of European sardines sardina pilchardus of Atlantic and Mediterranean waters: Ecological implications. J. Fish Biol. 86, 1348–1362. doi: 10.1111/jfb.12645
Dahlke F. T., Wohlrab S., Butzin M., Pörtner H. O. (2020). Thermal bottlenecks in the life cycle define climate vulnerability of fish. Science 369, 65–70. doi: 10.1126/science.aaz3658
DGRM (2016). Directirate-gernal for natural resources, safety and maritime services (Portugal: 2016 Fleet Reports). Available at: https://www.dgrm.mm.gov.pt/documents/20143/46307/2016-RELAT%C3%93RIO_FROTA.pdf/eeffa787-4214-6e2c-dec3-a0d9f43e391d.
Ettahiri O., Berraho A., Vidy G., Ramandi M., Do Chi T. (2003). Observation on the spawning of sardina and sardinella off the south moroccan atlantic coast (21°–26°n). Fish. Res. 60, 207–222. doi: 10.1016/s0165-7836(02)00172-8
Fernández-Corredor E., Albo-Puigserver M., Pennino M. G., Bellido J. M., Coll M. (2021). Influence of environmental factors on different life stages of European anchovy (Engraulis encrasicolus) and European sardine (Sardina pilchardus) from the Mediterranean Sea: A literature review. Reg. Stud. Mar. Sci. 41, 101606. doi: 10.1016/j.rsma.2020.101606
Fréon P., Werner F., Chavez F. (2009). “Conjectures on future climate effects on marine ecosystems dominated by small pelagic fish,” in Climate change and small pelagic fish. Eds. Checkley D., Alheit J., Oozeki Y., Roy &C. (Cambridge: Cambridge University Press), 312–343. doi: 10.1017/CBO9780511596681.016
Ganias K. (2009). Linking sardine spawning dynamics to environmental variability. Estuar. Coast. Shelf Sci. 84, 402–408. doi: 10.1016/j.ecss.2009.07.004
Ganias K., Somarakis S., Koutsikopoulos C., Machias A. (2007). Factors affecting the spawning period of sardine in two highly oligotrophic seas. Mar. Biol. 151, 1559–1569. doi: 10.1007/s00227-006-0601-0
Garcia-Soto C., Cheng L., Caesar L., Schmidtko S., Jewett E. B., Cheripka A., et al. (2021). An overview of ocean climate change indicators: sea surface temperature, ocean heat content, ocean ph, dissolved oxygen concentration, arctic sea ice extent, thickness and volume, sea level and strength of the amoc (atlantic meridional overturning circulation). Front. Mar. Sci. 8. doi: 10.3389/fmars.2021.642372
Garcia H. E., Weathers K., Paver C. R., Smolyar I., Boyer T. P., Locarnini R. A., et al. (2018). World ocean atlas 2018, volume 3: Dissolved oxygen, apparent oxygen utilization, and oxygen saturation Vol. 83. Ed. Mishonov A. (Silver Spring, Maryland: NOAA Atlas NESDIS), 38pp.
Garrido S., Cristóvão A., Caldeira C., Ben-Hamadou R., Baylina N., Batista H., et al. (2016). Effect of temperature on the growth, survival, development and foraging behaviour of sardina pilchardus larvae. Mar. Ecol. Progr. Ser. 559, 131–145. doi: 10.3354/meps11881
Garrido S., Silva A., Marques V., Figueiredo I., Bryère P., Mangin A., et al. (2017). Temperature and food-mediated variability of European Atlantic sardine recruitment. Prog. Oceanogr. 159, 267–275. doi: 10.1016/j.pocean.2017.10.006
Giannoulaki M., Schismenou E., Pyrounaki E.-E., Tsagarakis K. (2014). “Habitat characterization and migrations,” in Biology and ecology of sardines and anchovies. Ed. Ganias K. (Boca Raton, FL: CRC Press), 190–241.
Gordó-Vilaseca C., Pennino M. G., Albo-Puigserver M., Wolff M., Coll M. (2021). Modelling the spatial distribution of Sardina pilchardus and Engraulis encrasicolus spawning habitat in the NW Mediterranean Sea. Mar. Environ. Res. 169, 105381 doi: 10.1016/j.marenvres.2021.105381
Han F., Jamsandekar M., Pettersson M. E., Su L., Fuentes-Pardo A. P., Davis B. W., et al. (2020). Ecological adaptation in atlantic herring is associated with large shifts in allele frequencies at hundreds of LOCI. Elife 9, 1–20. doi: 10.7554/ELIFE.61076
Hijmans R. J., van Etten J., Sumner M., Cheng J., Bevan A., Bivand R., et al. (2020). Package ‘raster’: Geographic data analysis and modeling (Repository CRAN). Available at: https://cran.r-project.org/web/packages/raster/raster.pdf.
Hinrichsen H. H., Lehmann A., Petereit C., Nissling A., Ustups D., Bergström U., et al. (2016). Spawning areas of eastern Baltic cod revisited: Using hydrodynamic modelling to reveal spawning habitat suitability, egg survival probability, and connectivity patterns. Prog. Oceanogr 143, 13–25. doi: 10.1016/j.pocean.2016.02.004
ICES (2006). Report of the working group on the assessment of mackerel, horse mackerel, sardine, and anchovy. ICES Document CM 36, 601.
Jutfelt F. (2020). Metabolic adaptation to warm water in fish. Funct. Ecol. 34, 1138–1141. doi: 10.1111/1365-2435.13558
Kallasvuo M., Vanhatalo J., Veneranta L. (2017). Modeling the spatial distribution of larval fish abundance provides essential information for management. Can. J. Fish. Aquat. Sci. 74(5), 636–649 doi: 10.1139/cjfas-2016-0008
Kanstinger P., Peck M. A. (2009). Co-Occurrence of European sardine (Sardina pilchardus), anchovy (Engraulis encrasicolus) and sprat (Sprattus sprattus) larvae in southern north Sea habitats: Abundance, distribution and biochemical-based condition. Scientia Marina 73S1, 141–152. doi: 10.3989/scimar.2009.73s1141
Lima A. R. A., Baltazar-Soares M., Garrido S., Riveiro I., Carrera P., Piecho-Santos A. M., et al. (2022). Forecasting shifts in habitat suitability across the distribution range of a temperate small pelagic fish under different scenarios of climate change. Sci. Total Environ. 804, 150167. doi: 10.1016/j.scitotenv.2021.150167
Lima A. R. A., Ferreira G. V. B., Barletta M. (2019). Estuarine ecocline function and essential habitats for fish larvae in tropical south Western Atlantic estuaries. Mar. Environ. Res. 151, 104786. doi: 10.1016/j.marenvres.2019.104786
Locarnini R. A., Mishonov A. V., Baranova O. K., Boyer T. P., Zweng M. M., Garcia H. E., et al. (2018). World ocean atlas 2018, volume 1: Temperature Vol. 81. Ed. Mishonov A. (Silver Spring, Maryland: NOAA Atlas NESDIS), 52pp.
Maynou F., Sabatés A., Ramirez-Romero E., Catalán I. A., Raya V. (2020b). Future distribution of early life stages of small pelagic fishes in the northwestern Mediterranean. Clim. Change 161, 567–589. doi: 10.1007/s10584-020-02723-4
Maynou F., Sabatés A., Raya V. (2020a). Changes in the spawning habitat of two small pelagic fish in the northwestern Mediterranean. Fish. Oceanogr. 29, 202–214. doi: 10.1111/fog.12464
Mbaye B., Doniol-Valcroze T., Brosset P., Castonguay M., Van Beveren E., Smith A., et al. (2020). Modelling Atlantic mackerel spawning habitat suitability and its future distribution in the north-west Atlantic. Fish. Oceanogr. 29, 84–99. doi: 10.1111/fog.12456
Moss R. H., Edmonds J. A., Hibbard K. A., Manning M. R., Rose S. K., Van Vuuren D. P., et al. (2010). The next generation of scenarios for climate change research and assessment. Nature 463, 747–756. doi: 10.1038/nature08823
Moyano M., Candebat C., Ruhbaum Y., Álvarez-Fernández S., Claireaux G., Zambonino-Infante J. L., et al. (2017). Effects of warming rate, acclimation temperature and ontogeny on the critical thermal maximum of temperate marine fish larvae. PloS One 12, e0179928. doi: 10.1371/journal.pone.0179928
NASA (2009). NASA Goddard Space Flight Center, Ocean Biology Processing Group. Distance to the nearest coast (Greenbelt MD, USA: Maintained by NASA Ocean Biology Distibuted Active Archive Center (OB.DAAC), Goddard Space Flight Center). Available at: https://oceancolor.gsfc.nasa.gov/docs/distfromcoast/.
Olivar M. P., Catalán I. A., Emelianov M., Fernández De Puelles M. L. (2003). Early stages of sardina pilchardus and environmental anomalies in the northwestern Mediterranean. Estuar. Coast. Shelf Sci. 56, 609–619. doi: 10.1016/S0272-7714(02)00210-X
Palomera I., Olivar M. P., Salat J., Sabatés A., Coll M., García A., et al. (2007). Small pelagic fish in the NW Mediterranean Sea: An ecological review. Prog. Oceanogr. 74, 377–396. doi: 10.1016/j.pocean.2007.04.012
Pearson R. G., Araújo M. B., Martinez-Meyer E., Brotons L., McClean C., Miles L., et al. (2006). Model-based uncertainty in species range prediction. J. Biogeogr. 33, 1704–1711. doi: 10.1111/j.1365-2699.2006.01460.x
Peck M. A., Alheit J., Bertrand A., Catalán I. A., Garrido S., Moyano M., et al. (2021). Small pelagic fish in the new millennium: A bottom-up view of global research effort. Prog. Oceanogr. 191, 102494. doi: 10.1016/j.pocean.2020.102494
Peck M. A., Arvanitidis C., Butenschön M., Canu D. M., Chatzinikolaou E., Cucco A., et al. (2018). Projecting changes in the distribution and productivity of living marine resources: A critical review of the suite of modelling approaches used in the large European project VECTORS. Estuar. Coast. Shelf Sci. 201, 40–55. doi: 10.1016/j.ecss.2016.05.019
Peck M. A., Kühn W., Hinrichsen H.-H., Pohlmann T. (2009). Inter-annual and inter-specific differences in the drift of fish eggs and yolksac larvae in the north Sea: A biophysical modeling approach. Sci. Mar. 73, 23–36. doi: 10.3989/scimar.2009.73s1023
Peck M. A., Reglero P., Takahashi M., Catalán I. A. (2013). Life cycle ecophysiology of small pelagic fish and climate-driven changes in populations. Prog. Oceanogr. 116, 220–245. doi: 10.1016/j.pocean.2013.05.012
Pennino M. G., Coll M., Albo-Puigserver M., Fernández-Corredor E., Steenbeek J., Giráldez A., et al. (2020). Current and future influence of environmental factors on small pelagic fish distributions in the northwestern mediterranean sea. Front. Mar. Sci. 7. doi: 10.3389/fmars.2020.00622
Perruche C. (2018). Product user manual for the Global Ocean Biogeochemistry Hindcast GLOBAL_REANALYSIS_BIO_001_029. Version 1. Copernicus Marine Environmental Monitoring Service, 17pp. doi: 10.25607/OBP-490
Petitgas P., Alheit J., Peck M. A., Raab K., Irigoien X., Huret M., et al. (2012). Anchovy population expansion in the north Sea. Mar. Ecol. Progr. Ser. 444, 1–13. doi: 10.3354/meps09451
Petitgas P., Rijnsdorp A. D., Dickey-Collas M., Engelhard G. H., Peck M. A., Pinnegar J. K., et al. (2013). Impacts of climate change on the complex life cycle of fishes. Fisher. Ocean. 22, 121–139. doi: 10.1111/fog.12010
Planque B., Bellier E., Lazure P. (2007). Modelling potential spawning habitat of sardine (Sardina pilchardus) and anchovy (Engraulis encrasicolus) in the bay of Biscay. Fish. Oceanogr. 16, 16–30. doi: 10.1111/j.1365-2419.2006.00411.x
Pörtner H. O., Peck M. A. (2010). Climate change effects on fishes and fisheries: towards a cause-and-effect understanding. J. Fish Bio. 77, 1745–1779. doi: 10.1111/j.1095-8649.2010.02783.x
Ramos S., Ré P., Bordalo A. A. (2009). New insights into the early life ecology of sardina pilchardus (Walbaum 1792) in the northern Iberian Atlantic (Walbaum 1792). Sci. Mar. 73 (3), 449–459. doi: 10.3989/scimar.2009.73n3449
Ramzi A., Hbid M. L., Ettahiri O. (2006). Larval dynamics and recruitment modelling of the Moroccan Atlantic coast sardine (Sardina pilchardus). Ecol. Modell. 197, 296–302. doi: 10.1016/j.ecolmodel.2006.03.036
R Core Team (2020). R: A language and environment for statistical computing (Vienna, Austria: R Foundation for Statistical Computing). Available at: http://www.r-project.org/index.html
Roy C., Cury P., Kifani S. (1992). Pelagic fish recruitment success and reproductive strategy in upwelling areas: Environmental compromises. South Afr. J. Mar. Sci. 12, 135–146. doi: 10.2989/02577619209504697
Sabatés A., Martín P., Lloret J., Raya V. (2006). Sea Warming and fish distribution: the case of the small pelagic fish, sardinella aurita, in the western Mediterranean. Glob. Change Biol. 12, 2209–2219. doi: 10.1111/j.1365-2486.2006.01246.x
Salat J., Pascual J., Flexas M., Chin T. M., Vazquez-Cuervo J. (2019). Forty-five years of oceanographic and meteorological observations at a coastal station in the NW Mediterranean: a ground truth for satellite observations. Ocean Dynamics 69 (9), 1067–1084. doi: 10.1007/s10236-019-01285-z
Santos A. J. P., Nogueira J., Martins H. (2005). Survival of sardine larvae off the Atlantic Portuguese coast: A preliminary numerical study. ICES J. Mar. Sci. 62, 634–644. doi: 10.1016/j.icesjms.2005.02.007
Santos A. M. P., Peliz A., Dubert J., Oliveira P. B., Angélico M. M., Ré P. (2004). Impact of a winter upwelling event on the distribution and transport of sardine (Sardina pilchardus) eggs and larvae off western Iberia: A retention mechanism. Cont. Shelf Res. 24, 149–165. doi: 10.1016/j.csr.2003.10.004
Santos A. M. P., Re P., Dos Santos A., Peliz Á. (2006). Vertical distribution of the European sardine (Sardina pilchardus) larvae and its implications for their survival. J. Plankton Res. 28, 523–532. doi: 10.1093/plankt/fbi137
Schickele A., Goberville E., Leroy B., Beaugrand G., Hattab T., Francour P., et al. (2021). European Small pelagic fish distribution under global change scenarios. Fish Fish. 22, 212–225. doi: 10.1111/faf.12515
Schickele A., Leroy B., Beaugrand G., Goberville E., Hattab T., Francour P., et al. (2020). Modelling European small pelagic fish distribution: Methodological insights. Ecol. Modell. 416, 108902. doi: 10.1016/j.ecolmodel.2019.108902
Schismenou E., Palmer M., Giannoulaki M., Alvarez I., Tsiaras K., Triantafyllou G., et al. (2016). Seasonal changes in otolith increment width trajectories and the effect of temperature on the daily growth rate of young sardines. Fish. Oceanogr. 25 (4), 362–372. doi: 10.1111/fog.12158
Scott J. D., Alexander M. A., Murray D. R., Swales D., Eischeid J. (2016). The climate change web portal: A system to access and display climate and earth system model output from the cmip5 archive. Bull. Am. Meteorological Society., 523–530. doi: 10.1175/BAMS-D-15-00035.1
Sebastian W., Sukumaran S., Zacharia P. U., Muraleedharan K. R., Dinesh Kumar P. K., Gopalakrishnan A. (2020). Signals of selection in the mitogenome provide insights into adaptation mechanisms in heterogeneous habitats in a widely distributed pelagic fish. Sci. Rep. 10, 1–14. doi: 10.1038/s41598-020-65905-1
Silva G., Lima F. P., Martel P., Castilho R. (2014). Thermal adaptation and clinal mitochondrial DNA variation of European anchovy. Proc. R. Soc B Biol. Sci. 281 (1792), 20141093. doi: 10.1098/rspb.2014.1093
Silva A., Moreno A., Riveiro I., Santos B., Pita C., Rodrigues J. G., et al. (2015). Sardine fisheries: resource assessment and social and economic situation. (Brussels:EU parliament. Directorate-General for internal policies. Policy Department B–structural and cohesion policy), 55p.
Somarakis S., Ganias K., Siapatis A., Koutsikopoulos C., MacHias A., Papaconstantinou C. (2006). Spawning habitat and daily egg production of sardine (Sardina pilchardus) in the eastern Mediterranean. Fish. Oceanogr. 15, 281–292. doi: 10.1111/j.1365-2419.2005.00387.x
Somarakis S., Tsoukali S., Giannoulaki M., Schismenou E., Nikolioudakis N. (2019). Spawning stock, egg production and larval survival in relation to small pelagic fish recruitment. Mar. Ecol. Prog. Ser. 617-618, 113–136. doi: 10.3354/meps12642
Stratoudakis Y., Coombs S., De Lanzós A. L., Halliday N., Costas G., Caneco B., et al. (2007). Sardine (Sardina pilchardus) spawning seasonality in European waters of the northeast Atlantic. Mar. Biol. 152, 201–212. doi: 10.1007/s00227-007-0674-4
Thuiller W., Georges D., Engler R., Breiner F. (2016). biomod2: ensemble platform for species distribution modeling (R package version 3), 3–7. Available at: https://cran.r-project.org/web/packages/biomod2/index.html.
Thuiller W., Georges D., Engler R., Breiner F. (2020). biomod2: ensemble platform for species distribution modeling (R package version 3.4.6). Available at: https://CRAN.R-project.org/package=biomod2.
Thuiller W., Lafourcade B., Engler R., Araújo M. B. (2009). BIOMOD – a platform for ensemble forecasting of species distributions. – Ecography 32, 369–373. doi: 10.1111/j.1600-0587.2008.05742.x
Torrado H., Mourre B., Raventos N., Carreras C., Tintoré J., Pascual M., et al. (2021). Impact of individual early life traits in larval dispersal: A multispecies approach using backtracking models. Prog. Oceanogr 192, 102518. doi: 10.1016/j.pocean.2021.102518
Tugores P., Giannoulaki M., Iglesias M., Bonanno A., Tičina V., Leonori I., et al. (2011). Habitat suitability modelling for sardine sardina pilchardus in a highly diverse ecosystem: The Mediterranean Sea. Mar. Ecol. Prog. Ser. 443, 181–205. doi: 10.3354/meps09366
Vousdoukas M. I., Mentaschi L., Voukouvalas E., Verlaan M., Feyen L. (2017). Extreme sea levels on the rise along europe’s coasts. Earth’s Futur. 5, 304–323. doi: 10.1002/2016EF000505
Xiu P., Chai F., Curchitser E. N., Castruccio F. S. (2018). Future changes in coastal upwelling ecosystems with global warming: The case of the California current system. Sci. Rep. 8, 1–9. doi: 10.1038/s41598-018-21247-7
Yool A., Popova E. E., Coward A. C. (2015). Future change in ocean productivity: Is the Arctic the new Atlantic. J. Geophys. Res. Ocean. 120, 7771–7790. doi: 10.1002/2015JC011167
Zarrad R., Missaoui H., Alemany F., Hamza A., Romdhane M. S., García A., et al. (2008). Distribution and abundance of early life stages of sardina pilchardus in the gulf of Tunis (Central Mediterranean Sea) in relation to environmental and biological factors. Sci. Mar. 72, 299–309. doi: 10.3989/scimar.2008.72n2299
Zeeberg J. J., Corten A., Tjoe-Awie P., Coca J., Hamady B. (2008). Climate modulates the effects of sardinella aurita fisheries off Northwest Africa. Fish. Res. 89 (1), 65–75. doi: 10.1016/j.fishres.2007.08.020
Zweng M. M., Reagan J. R., Seidov D., Boyer T. P., Locarnini R. A., Garcia H. E., et al. (2018). World ocean atlas 2018, volume 2: Salinity Vol. 82 Ed.(Mishonov A. (Silver Spring, Maryland:NOAA Atlas NESDIS), 50pp.
Keywords: ocean warming, global changes, species distribution models, sardina pilchardus, early life stages, ensemble forecast
Citation: Lima ARA, Garrido S, Riveiro I, Rodrigues D, Angélico MMP, Gonçalves EJ, Peck MA and Silva G (2022) Seasonal approach to forecast the suitability of spawning habitats of a temperate small pelagic fish under a high-emission climate change scenario. Front. Mar. Sci. 9:956654. doi: 10.3389/fmars.2022.956654
Received: 30 May 2022; Accepted: 25 July 2022;
Published: 19 August 2022.
Edited by:
Pengfei Lin, Chinese Academy of Sciences (CAS), ChinaReviewed by:
Chenying Guo, Chinese Academy of Sciences, ChinaPablo Brosset, Institut Français de Recherche pour l'Exploitation de la Mer (IFREMER), France
Hao Wei, Tianjin University, China
Copyright © 2022 Lima, Garrido, Riveiro, Rodrigues, Angélico, Gonçalves, Peck and Silva. This is an open-access article distributed under the terms of the Creative Commons Attribution License (CC BY). The use, distribution or reproduction in other forums is permitted, provided the original author(s) and the copyright owner(s) are credited and that the original publication in this journal is cited, in accordance with accepted academic practice. No use, distribution or reproduction is permitted which does not comply with these terms.
*Correspondence: André R. A. Lima, andre.ricardoaraujolima@gmail.com