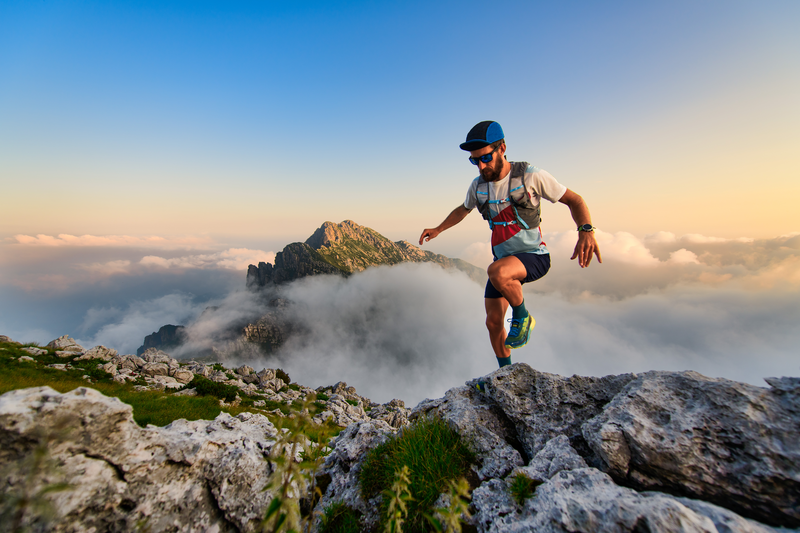
95% of researchers rate our articles as excellent or good
Learn more about the work of our research integrity team to safeguard the quality of each article we publish.
Find out more
ORIGINAL RESEARCH article
Front. Mar. Sci. , 26 July 2022
Sec. Marine Pollution
Volume 9 - 2022 | https://doi.org/10.3389/fmars.2022.956474
This article is part of the Research Topic Coastal Environmental and Ecological Data Analysis View all 30 articles
Eutrophication, mainly caused by the oversupply of inorganic nitrogen and phosphate, has increased and become a serious environmental problem in the coastal bays of Beibu Gulf, a newly developing industry and port in South China. However, the sources of nitrate are poorly understood in the gulf. In this study, nitrate dual isotopes (δ15N-NO3- and δ18O-NO3-) and ammonium isotopes (δ15N-NH4+) were measured during the rainy season to identify the nitrate sources and elucidate their biogeochemical processes in Xi Bay, a semi-enclosed bay that is strongly affected by human activities in the Beibu Gulf. The results showed that a high dissolved inorganic nitrogen (DIN, 10.24-99.09 µmol L-1) was observed in Xi Bay, particularly in the bay mouth. The concentrations of DIN in the bay were 1.5 times higher than that in Qinzhou Bay and 1.7 times than that in Tieshangang Bay, which mainly influenced by the intensive human activities (i.e., industrial and port activities). In addition, lower values of δ15N-NO3- and δ18O-NO3- and higher values of δ15N-NH4+ were observed in the upper bay, suggesting that microbial nitrification occurs in the upper bay, which was the dominant nitrate source in the upper bay (39%). In addition to nitrification, external sources, including sewage and manure (33%), soil N (15%) and fertilizer (11%), contributed to the higher nutrients in the upper bay. In the lower bay, severe nitrogen pollution led to a weaker impact of biological processes on isotopic fractionation, although a high Chl a level (average of 7.47 µg L-1) was found in this region. The heavy nitrate pollution in the lower bay mainly originated from sewage and manure (54%), followed by soil N (26%) and fertilizer (17%). The contribution of the nitrate source from atmospheric deposition was relatively low in the bay (<3%). This study suggests that biogeochemical processes have little impact on nitrate dual isotopes under heavy nitrogen pollution, and isotopes are an ideal proxy for tracing nitrogen sources.
Coastal bays receive terrestrial and riverine nutrient inputs, which have increased considerably in recent decades (Jickells, 1998; Gruber and Galloway, 2008; Savage et al., 2010; Yan et al., 2017). The increase in anthropogenic inputs has resulted in a host of environmental problems, such as eutrophication (Malone and Newton, 2020; Zhou et al., 2020), seasonal hypoxia (Rabalais et al., 2002; Howarth et al., 2011; Yu et al., 2021) and harmful algal blooms (Li et al., 2014; Glibert et al., 2014), in coastal bays, which greatly affect biogeochemical cycles and coastal ecosystems. Nitrogen (N) plays a key role in regulating marine primary productivity (Falkowski et al., 1998; Moore et al., 2013), and thus, its availability influences the sequestration of anthropogenic CO2 in coastal seas and climate changes. However, increasing N loads to coastal bays, associated with industrial, agricultural, urban and domestic waste, have aroused widespread public concern (Yang et al., 2018). Therefore, a better understanding of N sources and their recycling processes is beneficial for designing effective management practices to protect and improve aquatic ecosystems.
The stable N isotopes (δ15N) of various pools of N, combined with the oxygen isotope of nitrate (δ18O-NO3-), have been proven to be useful in identifying nitrogen sources and their biogeochemical processes (Kendall, 1998; Ye et al., 2016; Yan et al., 2017; Yang et al., 2018; Lao et al., 2019a; Chen et al., 2020). For example, sewage and manure have higher δ15N values (4-25‰) than soil N (-0.1-8.3‰), fertilizer (-1.9-3.0‰) and atmospheric deposition (-1.8-4.1‰) (Chen et al., 2019a; Kendall, 1998; Xue et al., 2009; Zhang et al., 2018) because the volatilization of δ15N-depleted ammonia is produced from human and animal waste and thus results in the enrichment of δ15N in the residual N pools (Xue et al., 2009; Yang et al., 2018). In addition, the applicability of this technique can also reflect biogeochemical transformations associated with N cycles. Nitrification first oxidizes ammonia to nitrite and then further oxidizes it to nitrate (Casciotti et al., 2011). A nitrogen isotope effect of 14-42‰ has been found for the former step (Casciotti et al., 2003 and Casciotti et al., 2010), whereas an inverse kinetic isotope effect occurs for the latter step (Casciotti, 2009); thus, δ15N-NO3- values are close to 0‰ during nitrification (Sigman et al., 2005 and Sigman et al., 2009; Smart et al., 2015). In addition, oxygen (O) is sourced from water (H2O) and dioxygen (O2) during nitrification. Typically, one-third of O in the newly formed nitrate is considered to originate from O2, with two-thirds originating from H2O, and δ18O-NO3- values are 7.8‰ during nitrification (Kendall, 1998; Wankel et al., 2006; Chen et al., 2009). Moreover, assimilation and denitrification cause an increase in δ15N-NO3- and δ18O-NO3- values in the residual nitrate pool, as lighter isotopes (14N and 16O) are preferentially utilized by phytoplankton and microorganisms, with an increase in the δ15N-NO3-:δ18O-NO3- ratio of 1 (Granger et al., 2004; Sigman et al., 2005; Granger et al., 2008). Thus, stable isotopes can serve as a useful tool in advancing our understanding of nitrogen dynamics in coastal bays.
Beibu Gulf is a semi-enclosed gulf and a newly developing industry and port area in the northwestern South China Sea (SCS). There are several rivers around the northern Beibu Gulf, and the influence of runoff input by the rivers and tidal influx from the outer gulf constitutes a well estuary-bay multi-ecosystem and has become an important mariculture area in China (Lao et al., 2020 and Liu et al., 2020; Xu et al., 2020: Xu et al., 2021; Lao et al., 2021a; Lao et al., 2022a and 2022b). However, with the rapid development of local urbanization and industrialization, the coastal bays in Beibu Gulf have been subjected to a high loading of contaminants from anthropogenic inputs, such as domestic, industrial, port and agricultural waste (Lao et al., 2019b; Xu et al., 2020: Lao et al., 2021a; Lao et al., 2021b; Lao et al., 2021c; Lao et al., 2021d: Xu et al., 2021 and Lao et al., 2022a). The nutrients discharged into coastal bays from runoff input have increased over the past 20 years, and the increase in phosphorus input has decreased the N/P ratios in several coastal bays in the Beibu Gulf (Lao et al., 2020 and Lao et al., 2021c). Moreover, the eutrophication of some coastal bays in the Beibu Gulf has increased in recent decades due to the increase in terrestrial nutrient input, particularly the nitrogen input (Lai et al., 2014; Guo et al., 2020; Lao et al., 2021a and Lao et al., 2021c; Pan et al., 2021). In addition, sewage is the dominant nitrate source in most bays/areas in the Beibu Gulf, which is identified by the dual nitrate isotopes (Pan et al., 2021; Lao et al., 2021b; Chen et al., 2022). This has resulted in an increase in the frequency of harmful algal blooms in the Beibu Gulf over the past decades, particularly in the past ten years (Xu et al., 2019).
Xi Bay is a semi-enclosed bay located on the coast of Fangchenggang city, which is an important industrial and port area in the northwestern Beibu Gulf. In addition to being affected by industrial activities, Xi Bay is also affected by a large amount of terrestrial contaminant input from the Fangcheng River in the upper bay (Lao et al., 2020). It is reported that a higher contaminant level was found in the coastal bay, including high nutrient loads, particularly in the rainy seasons (Lao et al., 2019b; Lao et al., 2021a). This is mainly affected by the heavy land-source discharge during the rainy seasons (Lao et al., 2021a). However, in such bays that are seriously affected by intensive human activities (i.e., industrial and port activities), the sources of nitrate are poorly understood. In this study, nitrate dual isotopes (δ15N-NO3- and δ18O-NO3-) and ammonium isotopes (δ15N-NH4+) were measured during the rainy season (July 2021) to determine the nitrate sources and their biogeochemical processes in Xi Bay.
Xi Bay is a semi-enclosed bay in the northern Beibu Gulf, located on the coast of Fangchenggang city, South China. The climate of the bay is influenced by the East Asian monsoon, and the southwest monsoon prevails during summer and the northeast monsoon prevails during winter. The annual rainfall in the bay is 2441 mm; most rainfall occurs in the rainy season (April to October, accounting for 91% of the annual rainfall), and the highest rainfall occurs in July (accounting for 23% of the annual rainfall) (China Meteorological Data Sharing Service System, http://data.cma.cn/site/index.html). The monthly mean air temperature ranges from 14.3 to 28.6 °C, with an annual average of 22.7 °C. There is the Fangcheng River in the upper bay (Figure 1). The Fangcheng River is approximately 98 km long with a drainage area of 843 km2. The annual runoff of the Fangcheng River is 542.25 × 108 m3, with the highest runoff in July (127.41 × 108 m3), and flows into the northern end of Xi Bay (Lao et al., 2020; Lao et al., 2022b). The eastern part of Xi Bay is the urban center of Fangchenggang city. The eastern bay mouth of Xi Bay is the industrial and port area. Thus, Xi Bay is a coastal bay greatly affected by human activities in the Beibu Gulf.
In this study, a cruise was conducted in Xi Bay in July (rainy season) 2021. A total of 16 seawater stations were collected in the bay, including a station (X1) in the Fangcheng River estuary and a station (X16) in the outer bay, using a rosette sampler fitted with 5-L Niskin bottles. The pH was measured in-suit with a pH meter (PHS-3C, Shanghai, China), and salinity was measured with a salinometer (SYA2-2). Dissolved oxygen (DO) was immediately determined using the Winkler titration method, with a precision of 0.07mg L-1. COD samples were determined by the potassium permanganate oxidation method with a precision of 0.15 mg L-1. The seawater samples for the dual nitrate isotopes (δ15N-NO3- and δ18O-NO3-) and ammonium isotope (δ15N-NH4+) were filtered by precombustion (450°C, 4 h) GF/F (glass fiber filters, 47-mm diameter, Whatman). The filtrate was transferred into a 250-ml precleaned (acid clean) polyethylene bottle and stored at -20°C until further analysis. Seawater was filtered by GF/F for the chlorophyll a (Chl a) sample, and the filtered GF/F was stored at -20°C until further analysis.
DO samples were measured on site by using the Winkler titration method. The Chl a samples were extracted by acetone (9:1) and measured by a spectrophotometer (Lorenzen, 1967). Nutrients, including PO43-, NO2-, NO3- and NH4+, were determined using a San++ continuous flow analyzer (Skalar, Netherlands). The detection limit of PO43- was 0.02 µg L-1, and the detection limits of NO2-, NO3- and NH4+ were 0.1 µg L-1. The δ15N-NO3- and δ18O-NO3-values were measured by the cadmium-azide method (McIlvin and Altabet, 2005) using a GasBench II-MAT 253 (253 Plus, Thermo Scientific, United States). Briefly, the NO2- in the seawater was removed by sulfamic acid (Granger et al., 2008). NO3- was reduced to NO2- by adding Cd and then further reduced to N2O by adding sodium azide buffer. For the analysis of δ15N-NH4+, BrO- (pH 12) was added to the seawater to oxidize NH4+ to NO2-, and then NaAsO2 was added to remove excess NaAsO2. The yield was verified via colorimetric nitrite determination. Then, sodium azide buffered with acetic acid was added to reduce NO2- to N2O (Zhang et al., 2007). The N2O was purified and separated by TraceGas (Isoprime), and the compositions of δ15N-NO3-, δ18O-NO3- and δ15N-NH4+ were determined by GasBench II-MAT 253. The IAEA-N3 international standard was used to calibrate the values of δ15N-NO3- and δ18O-NO3-, and the international standards of USGS 25, USGS26 and IAEA-N1 were used to calibrate the values of δ15N-NH4+. The reproducibility of duplicate analysis of δ15N-NO3-, δ18O-NO3- and δ15N-NH4+ was<0.3‰ (mean ± 0.1‰),<0.6‰ (mean ± 0.3‰) and<0.3‰ (mean ± 0.1‰), respectively.
Isotopic values and nutrient concentrations from simple physical mixing can be calculated by the salinity-based conservative mixing model (Fry, 2002; Ye et al., 2016; Lao et al., 2019a). The equation is as follows:
where q is the proportional contributions of the two endmembers. The terms S, N and δ are the salinity, nutrient and isotopic values of the two endmembers, and Smix, Nmix and δmix are the theoretical values of mixing of the two endmembers. According to equations (1) to (4):
Thus, salinity-based nutrient mixing exhibits linear conservative mixing (6), whereas isotopic mixing exhibits curvilinear mixing. The distributions of isotopic values and nutrient levels are expected to fall on the mixing line between two endmembers. The deviation from the mixing line suggests external sources or the presence of transformation processes (Wankel et al., 2006; Yang et al., 2018; Lao et al., 2019a; Lao et al., 2021b; Chen et al., 2022).
The proportional contributions of potential nitrate sources can be quantified by a Bayesian mixing model, which was created and run in the SIAR (stable isotope analysis in R 4.1.2) package. This model has been widely used to quantify the potential nitrate sources in marine systems (Xue et al., 2009; Korth et al., 2014; Davis et al., 2015; Zhang et al., 2018: Lao et al., 2019a; Lao et al., 2021b and Chen et al., 2022). The framework can be found in Xue et al. (2009) and Moore and Semmens (2008) as follows:
where Xij represents the isotopic value j of the mixed water i (i =1, 2, 3,…, N and j =1, 2, 3,…, j), Sjk represents the source value k (k =1, 2, 3,…, k) on isotope j and represents the normal distribution with an average of μ and a standard deviation of ω, Pk represents the proportional contribution of source k, cjk represents the fractionation factor and represents a normal distribution with an average of λ and a standard deviation of τ, and ϵjk represents the residual error of the additional unquantified variation between individual mixed samples and represents a normal distribution with an average of 0 and a standard deviation of σ.
Statistical analyses were conducted by the software SPSS 19.0 for Windows. The linear regression in this study was conducted by SigmaPlot 11.0.
The distributions of physicochemical parameters are presented in Figure 2. The surface seawater temperature ranged from 31.90 to 32.40 °C (an average of 32.13 °C). The salinity ranged from 8.97 to 24.22 (an average of 18.60), with a decreasing trend from the upper bay to the outer bay and the lowest salinity in the Fangcheng River estuary and the highest salinity in the outer bay. This suggests that the seawater in Xi Bay is greatly affected by freshwater input from the Fangcheng River. The pH values ranged from 7.81 to 8.04, with an average of 7.95. The DO level ranged from 5.73 mg L-1 to 7.00 mg L-1 (an average of 6.41 mg L-1), with a lower DO level in the upper bay (< 6 mg L-1). The Chl a level ranged from 1.60 to 12.30 µg L-1 (average of 5.41 µg L-1), and a higher Chl a level was found in the bay mouth. The COD concentrations ranged from 1.09 to 2.18 mg L-1, with an average of 1.52 mg L-1.
Figure 2 Spatial distributions of physicochemical parameters, including temperature (A), salinity (B), pH (C), DO (D), Chl a (E) and COD (F), in Xi Bay during the rainy season.
The distributions of nutrients are presented in Figure 3. The concentrations of PO43- ranged from 0.36 to 1.17 µmol L-1 (an average of 0.67 µmol L-1). A higher PO43- concentration was found in the estuary and the upper bay and exhibited a decreasing trend from the upper bay to the outer bay (Figure 3A). This result suggests that the PO43- in Xi Bay is mainly influenced by the runoff input. High DIN (dissolved inorganic nitrogen, the sum of NO3-, NO2- and NH4+) concentrations were found in Xi Bay. The concentration of NO3- ranged from 8.21 to 89.93 µmol L-1, with an average of 31.05 µmol L-1. Although the NO3- concentration exhibited a decreasing trend from the upper bay to near the bay mouth, a significantly high NO3- concentration was found in the bay mouth (stations X13, X14 and X15, over 3 times than that in the Fangcheng River estuary), which is located in the industrial and port areas (Figures 3B, C). Similar distribution patterns were also found in the concentrations of NO2- and NH4+ (Figure 3). The concentrations of NO2- and NH4+ ranged from 0.61 to 2.54 µmol L-1 (an average of 1.11 µmol L-1) and 1.26 to 7.36 µmol L-1 (an average of 3.01 µmol L-1), and high concentrations were all found in the bay mouth. Notably, the concentrations of DIN in the bay mouth (an average of 98.28 µmol L-1) were significantly higher than those in the Fangcheng River (an average of 58.64 µmol L-1) during a similar rainy month (Lao et al., 2020). This result suggests that the discharge of DIN caused by intensive human activities (i.e., industrial and port activities) in the bay mouth is much higher than the runoff input in Xi Bay. In addition, the concentrations of DIN in Xi Bay (an average of 35.17 µmol L-1) during a similar rainy month (August 2018) were 1.5 times those in Qinzhou Bay (22.99 µmol L-1) and 1.7 times those in Tieshangang Bay (20.51 µmol L-1) (Lao et al., 2021a), which are semi-enclosed bays that are greatly affected by intensive human activities in the northern Beibu Gulf (Chen et al., 2022). Thus, it can be concluded that Xi Bay is a bay seriously affected by artificial inorganic nitrogen input in the Beibu Gulf.
Figure 3 Spatial distributions of nutrients in Xi Bay during the rainy season. (A),PO43- (B), NO3-(C), NO2- (D), NH4+.
The distribution characteristics of δ15N-NO3-, δ18O-NO3- and δ15N-NH4+ in Xi Bay during the rainy season are presented in Figure 4. The values of δ15N-NO3-, δ18O-NO3- and δ15N-NH4+ ranged from 4.6 to 13.7‰, 3.1 to 15.3‰ and -3.0 to 26.3‰, with averages of 7.5‰, 7.1‰ and 13.5‰, respectively. Generally, the values of δ15N-NO3- and δ18O-NO3- were lower in the upper bay and exhibited an increasing trend to the bay mouth (Figure 4). Corresponding to the DIN, the highest values of δ15N-NO3- and δ18O-NO3- were all observed in the bay mouth. In contrast, higher values of δ15N-NH4+ were observed in the upper bay (Figure 4A). The difference in isotopic values in the upper bay and bay mouth suggests that different biogeochemical processes or sources of nitrogen could have occurred in the two regions.
Figure 4 Spatial distributions of nutrients in Xi Bay during the rainy season. (A), δ15N-NO3- (B), δ18O-NO3-(C), δ15N-NH4+
In this study, the lowest salinity at station X1 was selected as the freshwater endmember, and the highest salinity at the outer bay (station X16) was selected as the marine endmember. The hydrological characteristics, nutrient concentrations and isotopic values of freshwater and marine endmembers are presented in Table 1.
Table 1 The hydrological characteristics, nutrient concentrations and isotopic values of freshwater and marine endmembers of Xi Bay.
Higher nutrient concentrations were also observed in the other coastal bays of Beibu Gulf during the rainy seasons (Lai et al., 2014; Lao et al., 2021a and Lao et al., 2021c), which were mainly related to the heavy land-source discharge induced by the increasing runoff around the coastal Beibu Gulf (Lao et al., 2020; Lao et al., 2021a). Particularly in Qinzhou Bay, because there are several rivers, such as the Qin River, Maoling River and Dafeng River, that directly input a large amount of terrestrial contaminants into the bay (Chen et al., 2019a; Zhang et al., 2019; Lao et al., 2020; Lao et al., 2021c; Lao et al., 2022b; Lyu et al., 2022). However, heavier DIN pollution was found in Xi Bay. Similarly, there is a Fangcheng River that directly inputs into Xi Bay, and 626,669 t of nitrogen and 4,987 t of phosphorus are discharged directly into the bay every year, including 69% of nitrogen and 60% of phosphorus in the rainy season (Lao et al., 2020). In addition, a significant correlation between PO43- concentrations and salinity was found in Xi Bay (Figure 5A), suggesting that the PO43- in the bay was mainly affected by terrestrial input. There was no relationship between DIN concentration and salinity (Figure 5B). This was mainly because the high DIN concentration occurred in the bay mouth. However, if the stations in the bay mouth (X13, X14 and X15) were excluded, a significant correlation between DIN concentration and salinity was found in Xi Bay (Figure 5C). This result suggests that the DIN in the bay is also affected by terrestrial input. An extremely high DIN level was observed in the bay mouth, suggesting that the DIN in Xi Bay was also greatly influenced by anthropogenic discharge. Fangchenggang is a major industrial and port city in the Beibu Gulf, and industrial and port transportation activities may cause a great load of environmental pollution in the coastal area (Lai et al., 2014; Chen et al., 2019a; Lao et al., 2021a and Xu et al., 2019; Lao et al., 2021d). Thus, except for the runoff input, industrial and port transportation also contributed greatly to the DIN load in Xi Bay, which could be responsible for the high DIN pollution compared to the other coastal bays in the Beibu Gulf. In addition, the N/P ratios were high in Xi Bay (ranging from 21 to 272, with an average of 69), particularly in the bay mouth (an average of 241), which was significantly higher than the Redfield ratio of 16 (the ratio at which nutrients are utilized by phytoplankton). This result is similar to previous studies in coastal Fangchenggang city (Lai et al., 2014; Lao et al., 2021a). The high DIN discharge could be responsible for the high N/P ratios in the coastal bay. Moreover, Fangchenggang city is an important production area of phosphate fertilizer around the Beibu Gulf urban agglomeration, and the PO43- concentration in the coastal area is relatively higher than that in other coastal bays in the Beibu Gulf, which is mainly influenced by the waste produced from the phosphate fertilizer plant in the city (Lai et al., 2014; Lao et al., 2021a). In this study, higher PO43- concentrations (with a minimum [PO43-] of >0.4 µmol L-1) suggest that neither P nor N acted as a limiting nutrient in this ecosystem despite higher N/P ratios found in the bay and that environmental conditions were favorable for phytoplankton blooms. In addition, a decreasing N/P ratio was found in the coastal bays of Beibu Gulf due to increasing P input (Lao et al., 2021a and Lao et al., 2021c; Chen et al., 2022), which has resulted in harmful algal blooms increasing in frequency in recent years, especially in the past ten years (Xu et al., 2019; Kang et al., 2020; Guan et al., 2022). This could be responsible for the high Chl a level in the bay, particularly in the bay mouth (Figure 2). A similar situation has been documented in Xiangshan Bay of the East China Sea (Yang et al., 2018).
Figure 5 The linear relationship between PO43- and salinity all stations of Xi Bay (A); the linear relationship between DIN and salinity all stations of Xi Bay(B); the linear relationship between DIN and salinity in Xi bay that exclude the stations in the bay mouth (C).
Lower values of dual nitrate isotopes (δ15N-NO3- and δ18O-NO3-) were observed, whereas higher δ15N-NH4+ values were observed in the upper bay (stations X1 to X7). In addition, the nutrients in the bay are slightly above the mixing lines, particularly in the bay mouth (except for PO43-) (Figure 6). These results suggest that decomposition/nitrification and/or external sources may occur in the bay. During the nitrification process, light N (δ14N) in the NH4+ pool is preferentially utilized by microorganisms, resulting in the enrichment of δ15N-NH4+ while adding depleted δ14N to the residual nitrate pool (Sigman et al., 2005; Chen et al., 2009; Ye et al., 2016; Lao et al., 2021b). If nitrification is the dominant process in the bay, isotopic fractionation during the process can be estimated by open-system Rayleigh fractionation (Altabet, 2006; Ye et al., 2016; Chen et al., 2022) as follows:
Figure 6 Distribution of nutrients and isotopic values against salinity in Xi Bay. The black dots are the samples excluding the stations in the bay mouth of Xi Bay; the blue dots are the samples in the bay mouth. The pink lines indicate the mixing line. The inner bay includes the upper bay and lower bay (stations X1 to X12); the bay mouth includes stations X13 to X15. (A), PO43- (B), NO2- (C) NO3- (D) NH4+ (E), δ15N-NO3- (F), δ18O-NO3- (G), δ15N-NH4+
where δm is the measured δ15N-NH4+ value; δi is the initial δ15N-NH4+ value; ϵ is the fractionation factor; and fNH4 is the fraction of measured NH4+ concentration relative to the initial NH4+ concentration ([NH4+]m/[NH4+]i). The slope of the δ15N-NH4+- fNH4 linear regression line corresponds to the isotopic effects of NH4+ oxidation in seawater (Ye et al., 2016). In our results, a weak but still significant correlation was found between δ15N-NH4+ values and fNH4 in the upper bay (Figure 7). In addition, the overall estimated ϵ (-25.21‰) falls well within the reported values for N fractionation factors during nitrification in field studies (-14 to -38‰) (Casciotti et al., 2003; Ye et al., 2016). This further suggests that microbial nitrification occurs in the upper bay. Microbial decomposition/nitrification could consume oxygen and release carbon dioxide in the water column, which may be responsible for the lower DO level (an average of 6.20) and pH value (an average of 7.88) in the upper bay (Figure 2).
However, if nitrification was the dominant process in the upper bay, the δ15N-NO3- values should be close to 0‰ (Sigman et al., 2005 and Sigman et al., 2009; Smart et al., 2015). Higher δ15N-NO3- (ranging from 4.9 to 6.1‰) and δ18O-NO3- (ranging from 3.1 to 8.4‰) values were found in the upper bay. This indicates that other processes or external sources may also occur in the bay. Both the processes of assimilation and denitrification can enrich the dual nitrate isotopes in the residual water column, with coupled δ15N-NO3- and δ18O-NO3- fractionation effects of ~1 (Granger et al., 2004; Sigman et al., 2005; Granger et al., 2011). However, both assimilation and denitrification consume nutrients, which is consistent with the distribution of nutrients above the mixing lines (Figure 6). In addition, there is no relationship between δ15N-NO3- and δ18O-NO3- values (R2 = 0.13, p>0.05). This further confirms that the processes of assimilation and denitrification are not responsible for the slight enrichment of dual nitrate isotopes in the upper bay, which can be related to external sources. Sewage and manure are usually characterized by relatively high δ15N-NO3- values (4‰ to 25‰) because of the volatilization of δ15N-depleted ammonia produced from human and animal waste (Xue et al., 2009). Soil N is another source that is characterized by relatively higher δ15N-NO3- values (0‰ to 8‰) (Xue et al., 2009). Previous studies suggested that a higher proportional contribution of soil N (30%) was found in strong hydrodynamic conditions with several river inputs in Qinzhou Bay, whereas a lower contribution of soil N (20%) was found in weak hydrodynamic conditions with river inputs in Tieshanggang Bay (Chen et al., 2022). Particularly in the rainy seasons, heavy rainfall could carry more land-source nutrients into the coastal Beibu Gulf (Lao et al., 2021a). In addition, manure and sewage were the dominant nitrate sources in the coastal bays of Beibu Gulf, which were mainly influenced by intensive human activities, such as mariculture, industrial and shipping activities (Lao et al., 2021b; Chen et al., 2022).
In the lower bay (stations X8 to X12) and the bay mouth (stations X13 to X15), the increased δ15N-NO3- and δ18O-NO3- values may be influenced by the characteristics of high isotopic nitrate sources (i.e., sewage and manure) and/or the processes of denitrification and assimilation. However, denitrification in water column can be first ruled out due to the high DO levels (> 6 mg L-1) in the lower bay and the bay mouth. The high Chl a level indicates that assimilation may occur in the lower bay and the bay mouth. In addition, the PO43- concentrations were below the mixing line (Figure 6), which may be related to consumption by phytoplankton. However, there is no correlation between δ15N-NO3- and δ18O-NO3- values (R2 = 0.07, p>0.05). In addition, according to the open-system Rayleigh fractionation (equation 12), the fractionation during the process of assimilation can be calculated. There is no relationship between δ15N-NO3- and fNO3 (R2 = 0.25, p>0.05) in the lower bay and the bay mouth, and the overall estimated ϵ (0.7‰) significantly deviates from the range of reported values (-3 to -9‰) during assimilation (York et al., 2007; Ye et al., 2016; Chen et al., 2022). This suggests that assimilation is unlikely to be the dominant process causing the increase in δ15N-NO3- and δ18O-NO3- values in the lower bay and the bay mouth. Moreover, a high NH4+ concentration (>6.30 µmol L-1) was found in the bay mouth, indicating that NH4+ is sufficient, and phytoplankton preferentially assimilate NH4+ (Glibert et al., 2016). However, if the assimilated NH4+ by phytoplankton was the dominant process in the lower bay and the bay mouth, increasing δ15N-NH4+ values can be expected. In contrast, lower δ15N-NH4+ values were found in the lower bay and the bay mouth (Figure 4). Thus, the external DIN sources could be responsible for the high DIN level in the bay mouth. The coast of the bay mouth is an important port and industrial area in Fangchenggang city. Intensive human activities and industrial emissions may be responsible for the high DIN concentration in the bay mouth (Lao et al., 2021a). In addition, heavy rainfall during the rainy seasons could also scour masses of pollutants into the bay, particularly in heavily polluted wharves and industrial areas (Lao et al., 2021a).
Because of the lower influence of isotopic fractionation caused by biological processes, such as denitrification and assimilation, isotopic characteristics may provide a signal on the various nitrate sources in Xi Bay, and the Bayesian mixing model can be used to quantify the potential nitrate sources (Lao et al., 2019a). In previous studies, potential nitrate sources, including sewage and manure, soil N, fertilizer and atmospheric deposition, have been reported in the coastal Beibu Gulf (Lao et al., 2021b; Chen et al., 2022). In Beibu Gulf, sewage and manure are considered as the most important nitrate sources in the coastal areas (Pan et al., 2021; Lao et al., 2021b; Chen et al., 2022). In addition, due to the input of many rivers into the Beibu Gulf, the rivers can input a large amount of agricultural nitrogen fertilizer and soil N into the coastal waters (Pan et al., 2021; Chen et al., 2022). Therefore, based on previous literature, we carried out a Bayesian mixed model calculation for four potential sources that may affect nitrate content in Xi Bay. In this study, the potential nitrate source from sewage and manure was selected from the measured values in the Beibu Gulf (Pan et al., 2021), and nitrate sources from soil N and fertilizer were selected from the coastal areas in the gulf (Jin et al., 2020), and the source from precipitation was selected near the Beibu Gulf in Zhanjiang city (Chen et al., 2019c). The δ15N-NO3- and δ18O-NO3- values of these four potential sources are presented in Table 2. As discussed above, nitrification is another important nitrate source in the upper bay, and thus, the isotopic values of δ15N-NO3- (0‰) and δ18O-NO3- (7.8‰) (Chen et al., 2009 and Chen et al., 2019b) are considered a nitrate source endmember in the upper bay. The results of the quantification of potential nitrate sources in Xi Bay are presented in Figure 8. In the upper bay, the nitrate is mainly formed by nitrification (accounted for 39%). Except for the nitrate formed by nitrification, the nitrate from sewage and manure was another dominant external source in the upper bay (ranging from 28% to 38%, average of 33%), followed by soil N (ranging from 0% to 31%, average of 15%) and fertilizer (ranging from 0% to 29%, average of 11%), while the source from atmospheric deposition was the lowest (2%). In the lower bay and the bay mouth, due to the influence of intensive human activities, heavy nitrate pollution mainly originated from sewage and manure (ranging from 44% to 64%, average of 54%), followed by soil N (ranging from 4% to 45%, average of 26%) and fertilizer (ranging from 1% to 33%, average of 17%), and atmospheric deposition accounted for only 3%. According to the calculated results, the high proportion of sewage and manure was responsible for the high DIN pollution in Xi Bay, particularly in the bay moth. Similar results were also reported in the other coastal bays in the Beibu Gulf, which suggests that sewage and manure were the dominant nitrate sources (Lao et al., 2021b; Pan et al., 2021; Chen et al., 2022). With the rapid development of urbanization and industrialization in the Beibu Gulf, the population density of coastal cities has gradually increased, and land-based wastewater discharge has increased (Lao et al., 2019a; Lao et al., 2020; Liu et al., 2020; Lao et al., 2021d), resulting in an increase in eutrophication in the coastal seawater of Beibu Gulf in recent decades (Lao et al., 2021a and Lao et al., 2021c). In addition, soil N was another important source that contributed to the heavy nitrogen pollution in the bay. With the increase in rainfall in the rainy season, a large amount of soil N on the land can be washed away and enter the coastal bay with the river (Lao et al., 2022b). Previous studies suggested that the nitrate source from the soil N in a coastal bay with riverine input was significantly higher than that in a bay without riverine input during the same period (Chen et al., 2022; Lao et al., 2022b). Moreover, nitrogen fertilizer (almost in the reduced form) is heavily applied in catchment agriculture in the Beibu Gulf during the rainy seasons (Xia et al., 2011; Kaiser et al., 2014; Lao et al., 2021a: Lao et al., 2021b), which could leak and contribute to the bay in this rainy season.
Figure 8 Proportion of potential nitrate sources in bay A during rainy season. (A) Proportion of potential nitrate sources in Upper bay, (B) Proportion of potential nitrate sources in Low bay and bay mouth. NN: nitrate from nitrification.
Nitrate and aammonium isotopes (δ15N-NO3-, δ18O-NO3- and δ15N-NH4+) were measured to determine the nitrate sources and the biogeochemical processes associated with nitrogen cycling in Xi Bay in Beibu Gulf, a semi-enclosed bay strongly affected by human activities. A high DIN concentration was observed in Xi Bay, particularly in the bay mouth. In addition, the DIN concentration was significantly higher than that in the other coastal bays in Beibu Gulf (the concentration in Xi Bay is 1.5 times that in Qinzhou Bay and 1.7 times that in Tieshangang Bay), suggesting high nitrogen pollution in the bay due to intensive human activities (i.e., industrial and port activities). Lower values of δ15N-NO3- and δ18O-NO3- and higher δ15N-NH4+ values were observed in the upper bay, suggesting that microbial nitrification occurs in the upper bay, and the nitrate formed by nitrification accounted for 39%, which is the dominant nitrate source in the upper bay. Except for nitrification, external sources, including sewage and manure (accounting for 33%), soil N (accounting for 15%) and fertilizer (accounting for 11%), contributed to the higher nutrients in the upper bay. In the lower bay and the bay mouth, severe nitrogen pollution resulted in less impact of biological processes on isotopic fractionation. The heavy nitrate pollution in the lower bay and the bay mouth mainly originated from sewage and manure (accounting for 54%), followed by soil N (accounting for 26%) and fertilizer (accounting for 17%), and atmospheric deposition accounted for only 3%. Overall, biogeochemical processes have little impact on nitrate dual isotopes under heavy nitrogen pollution, and isotopes are an ideal proxy for tracing nitrogen sources.
The original contributions presented in the study are included in the article/supplementary material. Further inquiries can be directed to the corresponding author.
GJ was responsible for the conceptualization. SC and QL prepared and wrote the original draft. SC, QL and GJ wrote, reviewed, and edited the manuscript. QL were responsible for the data curation. CC, QZ and XL were responsible for the experimental operation. SC, QL and XL were responsible for field sampling. QL and GJ funded the acquisition. All authors contributed to the article and approved the submitted version.
We would like to thank Marine Environmental Monitoring Centre of Beihai, State Oceanic Administration, for their support in collecting water samples. This study was supported by the Guangdong Provincial College Innovation Team Project (2019KCXTF021), the Guangxi Natural Science Foundation of China (2020GXNSFBA297065) and First-class Discipline Plan of Guangdong Province (080503032101, 231420003).
The authors declare that the research was conducted in the absence of any commercial or financial relationships that could be construed as a potential conflict of interest.
All claims expressed in this article are solely those of the authors and do not necessarily represent those of their affiliated organizations, or those of the publisher, the editors and the reviewers. Any product that may be evaluated in this article, or claim that may be made by its manufacturer, is not guaranteed or endorsed by the publisher.
Casciotti K. L. (2009). Inverse kinetic isotope fractionation during bacterial nitrite oxidation. Geochimica Cosmochimica Acta 73 (7), 2061–2076. doi: 10.1016/j.gca.2008.12.022
Casciotti K. L., Buchwald C., Santoro A. E., Frame C. (2011). Assessment of nitrogen and oxygen isotopic fractionation during nitrification and its expression in the marine environment. Methods enzygogy 486, 253–280. doi: 10.1016/B978-0-12-381294-0.00011-0
Casciotti K. L., McIlvin M., Buchwald C. (2010). Oxygen isotopic exchange and fractionation during bacterial ammonia oxidation. Limnology Oceanography 55 (2), 753–762. doi: 10.4319/lo.2010.55.2.0753
Casciotti K. L., Sigman D. M., Ward B. B. (2003). Linking diversity and stable isotope fractionation in ammonia-oxidizing bacteria. Geomicrobiology J. 20 (4), 335–353. doi: 10.1080/01490450303895
Chen B., Dong D., Qin X., Xu M., Chen X., Shi M. (2019a). Relationship between the occurrence of red tides and the dynamic response mechanisms in the northern beibu gulf. J. Coast. Res. 93 (SI), 185–193. doi: 10.2112/SI93-026.1
Chen F., Jia G., Chen J. (2009). Nitrate sources and watershed denitrification inferred from nitrate dual isotopes in the beijiang river, south China. Biogeochemistry 94 (2), 163–174. doi: 10.1007/s10533-009-9316-x
Chen F., Lao Q., Jia G., Chen C., Zhu Q., Zhou X. (2019c). Seasonal variations of nitrate dual isotopes in wet deposition in a tropical city in China. Atmospheric Environ. 196, 1–9. doi: 10.1016/j.atmosenv.2018.09.061
Chen C., Lao Q., Shen Y., Jin G., Chen F., Su Q., et al. (2022). Comparative study of nitrogen cycling between a bay with riverine input and a bay without riverine input, inferred from stable isotopes. Front. Mar. Sci. 9, 885037. doi: 10.3389/fmars.2022.885037
Chen F., Lao Q., Zhang S., Bian P., Jin G., Zhu Q., et al. (2020). Nitrate sources and biogeochemical processes identified using nitrogen and oxygen isotopes on the eastern coast of hainan island. Continental Shelf Res. 207, 104209. doi: 10.1016/j.csr.2020.104209
Chen F., Zhou X., Lao Q., Wang S., Jin G., Chen C., et al. (2019b). Dual isotopic evidence for nitrate sources and active biological transformation in the northern south China Sea in summer. PloS One 14 (1), e0209287. doi: 10.1371/journal.pone.0209287
Davis P., Syme J., Heikoop J., Fessenden-Rahn J., Perkins G., Newman B., et al. (2015). Quantifying uncertainty in stable isotope mixing models. J. Geophysical Research: Biogeosciences 120 (5), 903–923. doi: 10.1002/2014JG002839
Falkowski P. G., Barber R. T., Smetacek V. (1998). Biogeochemical controls and feedbacks on ocean primary production. science 281 (5374), 200–206. doi: 10.1126/science.281.5374.200
Fry B. (2002). Conservative mixing of stable isotopes across estuarine salinity gradients: A conceptual framework for monitoring watershed influences on downstream fisheries production. Estuaries 25, 264–271. doi: 10.1007/BF02691313
Glibert P. M., Icarus Allen J., Artioli Y., Beusen A., Bouwman L., Harle J., et al. (2014). Vulnerability of coastal ecosystems to changes in harmful algal bloom distribution in response to climate change: projections based on model analysis. Global Change Biol. 20 (12), 3845–3858. doi: 10.1111/gcb.12662
Glibert P. M., Wilkerson F. P., Dugdale R. C., Raven J. A., Dupont C. L., Leavitt P. R., et al. (2016). Pluses and minuses of ammonium and nitrate uptake and assimilation by phytoplankton and implications for productivity and community composition, with emphasis on nitrogen-enriched conditions. Limnology Oceanography 61 (1), 165–197. doi: 10.1002/lno.10203
Granger J., Prokopenko M. G., Sigman D. M., Mordy C. W., Morse Z. M., Morales L. V., et al. (2011). Coupled nitrification-denitrification in sediment of the eastern Bering Sea shelf leads to 15N enrichment of fixed n in shelf waters. J. Geophysical Research: Oceans 116 (C11). doi: 10.1029/2010jc006751
Granger J., Sigman D. M., Lehmann M. F., Tortell P. D. (2008). Nitrogen and oxygen isotope fractionation during dissimilatory nitrate reduction by denitrifying bacteria. Limnology Oceanography 53 (6), 2533–2545. doi: 10.4319/lo.2008.53.6.2533
Granger J., Sigman D. M., Needoba J. A., Harrison P. J. (2004). Coupled nitrogen and oxygen isotope fractionation of nitrate during assimilation by cultures of marine phytoplankton. Limnology Oceanography 49 (5), 1763–1773. doi: 10.4319/lo.2004.49.5.1763
Gruber N., Galloway J. N. (2008). An earth-system perspective of the global nitrogen cycle. Nature 451, 293–296. doi: 10.1038/nature06592
Guan W., Bao M., Lou X., Zhou Z., Yin K. (2022). Monitoring, modeling and projection of harmful algal blooms in China. Harmful Algae 111, 102164. doi: 10.1016/j.hal.2021.102164
Guo J., Wang Y., Lai J., Pan C., Wang S., Fu H., et al. (2020). Spatiotemporal distribution of nitrogen biogeochemical processes in the coastal regions of northern beibu gulf, south China sea. Chemosphere 239, 124803. doi: 10.1016/j.chemosphere.2019.124803
Howarth R., Chan F., Conley D. J., Garnier J., Doney S. C., Marino R., et al. (2011). Coupled biogeochemical cycles: eutrophication and hypoxia in temperate estuaries and coastal marine ecosystems. Front. Ecol. Environ. 9 (1), 18–26. doi: 10.1890/100008
Jickells T. D. (1998). Nutrient biogeochemistry of the coastal zone. Science 281 (5374), 217–222. doi: 10.1126/science.281.5374.217
Jin Z., Wang J., Chen J., Zhang R., Li Y., Yaoting L., et al. (2020). Identifying the sources of nitrate in a small watershed using δ15N-δ18O isotopes of nitrate in the kelan reservoir, guangxi, China. Agric. Ecosyst. Environ. 297, 106936. doi: 10.1016/j.agee.2020.106936
Kaiser D., Unger D., Qiu G. (2014). Particulate organic matter dynamics in coastal systems of the northern beibu gulf. Continental Shelf Res. 82, 99–118. doi: 10.1016/j.csr.2014.04.006
Kendall C. (1998). “Tracing nitrogen sources and cycling in catchments,” in Isotope tracers in catchment hydrology. Eds. Kendall C., McDonnel J. J. (New York: Elsevier), 519–576.
Korth F., Deutsch B., Frey C., Moros C., Voss M. (2014). Nitrate source identification in the Baltic Sea using its isotopic ratios in combination with a Bayesian isotope mixing model. Biogeosciences 11 (17), 4913–4924. doi: 10.5194/bg-11-4913-2014
Lai J., Jiang F., Ke K., Xu M., Lei F., Chen B. (2014). Nutrients distribution and trophic status assessment in the northern beibu gulf, China. Chin. J. oceanology limnology 32 (5), 1128–1144. doi: 10.1007/s00343-014-3199-y
Lao Q., Cai S., Huang P., Chen F., Su Q., Lei X., et al. (2022a). Contaminant characteristics and influencing factors of heavy metals in seawater and sediments in a typical mariculture bay in south China. Front. Mar. Sci. 9. doi: 10.3389/fmars.2022.923494
Lao Q., Zhang S., Li Z., Chen F., Zhou X., Jin G., et al. (2022b). Quantification of the seasonal intrusion of water masses and their impact on nutrients in the Beibu Gulf using dual water isotopes. J. Geophys. Res. Oceans 127, e2021JC018065. doi: 10.1029/2021JC018065
Lao Q., Chen F., Liu G., Chen C., Jin G., Zhu Q., et al. (2019a). Isotopic evidence for the shift of nitrate sources and active biological transformation on the western coast of guangdong province, south China. Mar. pollut. Bull. 142, 603–612. doi: 10.1016/j.marpolbul.2019.04.026
Lao Q., Liu G., Gao J., Shen Y., Guo Z., Qing S., et al. (2021c). Study on the characteristics and eutrophication of nutrients in the mariculture farms of qinzhou bay, south China. Mar. Environ. Sci. 40 (3), 407–416. doi: 10.13634/j.cnki.mes.2021.03.011
Lao Q., Liu G., Gao J., Shen Y., Su Q., Chen C., et al. (2021b). Seasonal sources and cycling of nitrogen revealed by stable isotopes in the northeastern beibu gulf, China. J. Mar. Sci. Eng. 9 (10), 1123. doi: 10.3390/jmse9101123
Lao Q., Liu G., Shen Y., Su Q., Gao J., Chen F. (2020). Distribution characteristics and fluxes of nutrients in the rivers of the beibu gulf. Haiyang Xuebao 42 (12), 93–100. doi: 10.3969/j.issn.0253−4193.2020.12.010
Lao Q., Liu G., Shen Y., Su Q., Lei X. (2021a). Biogeochemical processes and eutrophication status of nutrients in the northern beibu gulf, south China. J. Earth System Sci. 130 (4), 1–14. doi: 10.1007/s12040-021-01706-y
Lao Q., Liu G., Zhou X., Chen F., Zhang S. (2021d). Sources of polychlorinated biphenyls (PCBs) and dichlorodiphenyltrichloroethanes (DDTs) found in surface sediment from coastal areas of beibu gulf: A reflection on shipping activities and coastal industries. Mar. pollut. Bull. 167, 112318. doi: 10.1016/j.marpolbul.2021.112318
Lao Q., Su Q., Liu G., Shen Y., Chen F., Lei X., et al. (2019b). Spatial distribution of and historical changes in heavy metals in the surface seawater and sediments of the beibu gulf, China. Mar. pollut. Bull. 146, 427–434. doi: 10.1016/j.marpolbul.2019.06.080
Li H. M., Tang H. J., Shi X. Y., Zhang C. S., Wang X. L. (2014). Increased nutrient loads from the changjiang (Yangtze) river have led to increased harmful algal blooms. Harmful Algae 39, 92–101. doi: 10.1016/j.hal.2014.07.002
Liu G., Lao Q., Su Q., Shen Y., Chen F., Qing S., et al. (2020). Spatial and seasonal characteristics of dissolved heavy metals in the aquaculture areas of beibu gulf, south China. Hum. Ecol. Risk Assessment: Int. J. 26 (7), 1957–1969. doi: 10.1080/10807039.2019.1629273
Lorenzen C. J. (1967). Determination of chlorophyll and pheopigments: spectrophotometric equations. Limnol Oceanogr 12, 343–346. doi: 10.4319/lo.1967.12.2.0343
Lyu H., Song D., Zhang S., Wu W., Bao X. (2022). Compound effect of land reclamation and land-based pollutant input on water quality in qinzhou bay, China. Sci. Total Environ. 826, 154183. doi: 10.1016/j.scitotenv.2022.154183
Malone T. C., Newton A. (2020). The globalization of cultural eutrophication in the coastal ocean: causes and consequences. Front. Mar. Sci. 7, 670. doi: 10.3389/fmars.2020.00670
McIlvin M. R., Altabet M. A. (2005). Chemical conversion of nitrate and nitrite to nitrous oxide for nitrogen and oxygen isotopic analysis in freshwater and seawater. Analytical Chem. 77 (17), 5589–5595. doi: 10.1021/ac050528s
Moore C. M., Mills M. M., Arrigo K. R., Berman-Frank I., Bopp L., Boyd P. W., et al. (2013). Processes and patterns of oceanic nutrient limitation. Nat. Geosci. 6 (9), 701–710. doi: 10.1038/ngeo1765
Moore J. W., Semmens B. X. (2008). Incoporating uncertainty and prior information into stable isotope mixing models. Ecol. Lett. 11, 470–480. doi: 10.1111/j.1461-0248.2008.01163.x
Pan K., Zheng X., Liu X., Jiang H. (2021). Nitrogen cycling in a tropical coral reef ecosystem under severe anthropogenic disturbance in summer: Insights from isotopic compositions. Water Res. 207, 117824. doi: 10.1016/j.watres.2021.117824
Rabalais N. N., Turner R. E., Wiseman W. J. Jr. (2002). Gulf of Mexico hypoxia, aka “The dead zone”. Annu. Rev. Ecol. Systematics 33 (1), 235–263. doi: 10.1146/annurev.ecolsys.33.010802.150513
Savage C., Leavitt P. R., Elmgren R. (2010). Effects of land use, urbanization, and climate variability on coastal eutrophication in the Baltic Sea. Limnology Oceanography 55 (3), 1033–1046. doi: 10.4319/lo.2010.55.3.1033
Sigman D. M., DiFiore P. J., Hain M. P., Deutsch C., Wang Y., Karl D. M., et al. (2009). The dual isotopes of deep nitrate as a constraint on the cycle and budget of oceanic fixed nitrogen. Deep Sea Res. Part I: Oceanographic Res. Papers 56 (9), 1419–1439. doi: 10.1016/j.dsr.2009.04.007
Sigman D. M., Granger J., DiFiore P. J., Lehmann M. M., Ho R., Cane G., et al. (2005). Coupled nitrogen and oxygen isotope measurements of nitrate along the eastern north pacific margin. Global Biogeochemical Cycles 19 (4), GB4022. doi: 10.1029/2005GB002458
Smart S. M., Fawcett S. E., Thomalla S. J., Weigand M. A., Reason C. J., Sigman D. M. (2015). Isotopic evidence for nitrification in the Antarctic winter mixed layer. Global Biogeochemical Cycles 29 (4), 427–445. doi: 10.1002/2014GB005013
Wankel S. D., Kendall C., Francis C. A., Paytan A. (2006). Nitrogen sources and cycling in the San Francisco bay estuary: A nitrate dual isotopic composition approach. Limnology Oceanography 51 (4), 1654–1664. doi: 10.4319/lo.2006.51.4.1654
Xia P., Meng X., Yin P., Cao Z., Wang X. (2011). Eighty-year sedimentary record of heavy metal inputs in the intertidal sediments from the nanliu river estuary, beibu gulf of south China Sea. Environ. pollut. 159 (1), 92–99. doi: 10.1016/j.envpol.2010.09.014
Xu C., Dan S. F., Yang B., Lu D., Kang Z., Huang H., et al. (2021). Biogeochemistry of dissolved and particulate phosphorus speciation in the maowei Sea, northern beibu gulf. J. Hydrology 593, 125822. doi: 10.1016/j.jhydrol.2020.125822
Xue D., Botte J., De Baets B., Accoe F., Nestler A., Taylor P., et al. (2009). Present limitations and future prospects of stable isotope methods for nitrate source identification in surface-and groundwater. Water Res. 43 (5), 1159–1170. doi: 10.1016/j.watres.2008.12.048
Xu C., Yang B., Dan S. F., Zhang D., Liao R., Lu D., et al. (2020). Spatiotemporal variations of biogenic elements and sources of sedimentary organic matter in the largest oyster mariculture bay (Maowei Sea), southwest China. Sci. Total Environ. 730, 139056. doi: 10.1016/j.scitotenv.2020.139056
Xu Y., Zhang T., Zhou J. (2019). Historical occurrence of algal blooms in the northern beibu gulf of China and implications for future trends. Front. Microbiol. 10, 451. doi: 10.3389/fmicb.2019.00451
Yang Z., Chen J., Li H., Jin H., Gao S., Ji Z., et al. (2018). Sources of nitrate in xiangshan bay (China), as identified using nitrogen and oxygen isotopes. Estuarine Coast. Shelf Sci. 207, 109–118. doi: 10.1016/j.ecss.2018.02.019
Yan X., Xu M. N., Wan X. S., Yang J. Y. T., Trull T. W., Dai M., et al. (2017). Dual isotope measurements reveal zoning of nitrate processing in the summer changjiang (Yangtze) river plume. Geophysical Res. Lett. 44 (24), 12–289. doi: 10.1002/2017GL075951
Ye F., Jia G., Xie L., Wei G., Xu J. (2016). Isotope constraints on seasonal dynamics of dissolved and particulate n in the pearl river estuary, south China. J. Geophysical Research: Oceans 121 (12), 8689–8705. doi: 10.1002/2016JC012066
York J. K., Tomasky G., Valiela I., Repeta D. J. (2007). Stable isotopic detection of ammonium and nitrate assimilation by phytoplankton in the waquoit bay estuarine system. Limnology Oceanography 52, 144–155. doi: 10.4319/lo.2007.52.1.0144
Yu L., Gan J., Dai M., Hui C. R., Lu Z., Li D. (2021). Modeling the role of riverine organic matter in hypoxia formation within the coastal transition zone off the pearl river estuary. Limnology Oceanography 66 (2), 452–468. doi: 10.1002/lno.11616
Zhang L., Altabet M. A., Wu T., Hadas O. (2007). Sensitive measurement of NH4+ 15N/14N (δ15NH4+) at natural abundance levels in fresh and saltwaters. Analytical Chem. 79 (14), 5297–5303. doi: 10.1021/ac070106d
Zhang D., Lu D., Yang B., Zhang J., Ning Z., Yu K. (2019). Influence of natural and anthropogenic factors on spatial-temporal hydrochemistry and the susceptibility to nutrient enrichment in a subtropical estuary. Mar. pollut. Bull. 146, 945–954. doi: 10.1016/j.marpolbul.2019.07.056
Zhang M., Zhi Y., Shi J., Wu L. (2018). Apportionment and uncertainty analysis of nitrate sources based on the dual isotope approach and a Bayesian isotope mixing model at the watershed scale. Sci. Total Environ. 639, 1175–1187. doi: 10.1016/j.scitotenv.2018.05.239
Keywords: nitrate dual isotopes, ammonium isotope, nitrogen pollution, source, Beibu Gulf
Citation: Cai S, Lao Q, Jin G, Chen C, Zhou X, Zhu Q and Lu X (2022) Sources of nitrate in a heavily nitrogen pollution bay in Beibu Gulf, as identified using stable isotopes. Front. Mar. Sci. 9:956474. doi: 10.3389/fmars.2022.956474
Received: 30 May 2022; Accepted: 28 June 2022;
Published: 26 July 2022.
Edited by:
Meilin Wu, South China Sea Institute of Oceanology, Chinese Academy of Sciences, ChinaReviewed by:
Jia Renming, Ministry of Natural Resources, ChinaCopyright © 2022 Cai, Lao, Jin, Chen, Zhou, Zhu and Lu. This is an open-access article distributed under the terms of the Creative Commons Attribution License (CC BY). The use, distribution or reproduction in other forums is permitted, provided the original author(s) and the copyright owner(s) are credited and that the original publication in this journal is cited, in accordance with accepted academic practice. No use, distribution or reproduction is permitted which does not comply with these terms.
*Correspondence: Guangzhe Jin, amluZ3Vhbmd6aGVAbGl2ZS5jbg==; amluZ3pAZ2RvdS5lZHUuY24=
Disclaimer: All claims expressed in this article are solely those of the authors and do not necessarily represent those of their affiliated organizations, or those of the publisher, the editors and the reviewers. Any product that may be evaluated in this article or claim that may be made by its manufacturer is not guaranteed or endorsed by the publisher.
Research integrity at Frontiers
Learn more about the work of our research integrity team to safeguard the quality of each article we publish.