- 1Tianjin International Joint Research & Development Center of Food Science and Engineering, Tianjin Key Laboratory of Food Biotechnology, College of Biotechnology and Food Science, Tianjin University of Commerce, Tianjin, China
- 2Department of Science, School of Science and Technology, Hong Kong Metropolitan University, Kowloon, Hong Kong SAR, China
- 3State Key Laboratory of Marine Pollution and Department of Chemistry, City University of Hong Kong, Kowloon, Hong Kong SAR, China
Due to close proximity to urban development, mangroves exposed to the contamination of polybrominated diphenyl ethers (PBDEs). Decabromodiphenyl ether (BDE-209) is one of the most predominant PBDE congener. The present study aimed to investigate the toxic effects of BDE-209 on the antioxidative and non-antioxidative responses of Kandelia obovata, a very common mangrove species, under different concentrations of BDE-209, 0.1, 1, 5 and 10 mg l-1. BDE-209 did not exhibit any negative effects on the growth of K. obovata seedlings. The stimulatory effects of BDE-209 on the enzymes including superoxide dismutase, peroxidase and catalase only occurred in weeks 1 and 4 and diminished in week 8. The concentrations of total polyphenols (TP) and extractable condensed tannins (ECT) were not affected by BDE-209. The production of O2·- was induced only at the very high level of BDE-209 (10 mg l-1). H2O2 was induced only in weeks 1 and 4 under BDE-209 treatment. BDE-209 was taken up by the roots of K. obovata, translocated to above-ground tissues, and accumulated in plant tissues with the concentrations declined in the order of root > propagule > stem > leaf. Although BDE-209 has higher molecular weight and higher log Kow than other PBDE congeners, the K. obovata seedlings could absorb, translocate and accumulate BDE-209. These findings suggested that mangrove plants could take up, accumulate PBDEs, and BDE-209 are less toxic than other congeners but more difficult to be removed by mangrove systems.
Introduction
Polybrominated diphenyl ethers (PBDEs) are manufactured as additive brominated flame retardants and widely distributed in the environment (Alaee et al., 2003; Portet-Koltalo et al., 2021). Mangroves often receive anthropogenic contamination as they are usually convenient discharge sites for waste and wastewater (Qiu et al., 2010; Ouyang and Guo, 2016), and PBDEs have been recorded in mangrove sediments (Binelli et al., 2007; Qiu et al., 2010). The problems of PBDE contamination in mangroves and their harmful effects on our environment cannot be ignored.
PBDEs pose oxidative stresses to organisms by over-production of reactive oxygen species (ROS), which might lead to poor growth or even death (Zezza et al., 2019). However, few studies reported the effects of PBDEs on plants and their antioxidative responses. Huang et al. (2013) found that PBDEs could increase the activities of nitro-reductase and glutathione-transferase in roots of ryegrass, pumpkin and maize. Our previous study (Wang et al., 2014a) showed BDE-47 posed significantly effects of the activities of three antioxidative enzymes, superoxide dismutase (SOD), peroxidase (POD) and catalase (CAT) of two mangrove plant species, Kandelia obovata (K. obovata) and Avicennia marina (A. marina). Knowledge on the role of antioxidative enzymes in mangrove plants to remove excess ROS produced by PBDE contamination is limited. Not only enzymatic antioxidants, non-enzymatic antioxidants in mangrove plants, such as tannins were commonly found and related to their tolerance (Huang et al., 2010a). Whether the non-enzymatic antioxidative defense system of mangrove plants could be induced to combat the oxidative stress posed by PBDE contamination has rarely been reported. Only Zhou et al. (2019) in our group reported the implication of tannin changes in two mangrove plants, K. obovata and Bruguiera gymnorhiza under PBDE contamination.
It is also important to understand the fate of PBDEs in the environment, particularly, bioaccumulation, transformation and elimination, as these are useful information in determining how the burdens and associated health risks of PBDEs change over time (Mueller et al., 2006). The predominant congeners of PBDEs in environmental samples, such as air, sewage sludge, soil and sediment, were BDE-209 and BDE-47 (Ramu et al., 2005; Li et al., 2009; Jin et al., 2010). The most dominant congener in many sediment samples was BDE-209, followed by BDE-47, BDE-99 and BDE-100 (Mai et al., 2005; Luo et al., 2007; Wang et al., 2007; Chen et al., 2009; Sun et al., 2013a). The accumulation of BDE-209 and BDE-47 in sediment could be due to the more extensive uses and the global BFRs consumption pattern of these commercial PBDE products (Wang et al., 2007). The former is the main component of a commercial product deca-BDEs, while the latter is the main component of another commercial product penta-BDEs, according to the file of US Environmental Protection Agency (EPA).
Therefore, attention should be paid to the toxic effects of BDE-209 and -47, and we reported the effects of BDE-47 on two mangrove plant species, K. obovata and A. marina (Wang and Tam, 2013; Wang et al., 2014a). Here we aimed to study the effects of BDE-209 on the growth of the seedlings of K. obovata during an eight-week hydroponic culture, and compare with BDE-47 (Wang et al., 2014a). In the present study, the hydroponic was used instead of soil culture, since the latter might reduce the toxicity and bioavailability of PBDEs (Mueller et al., 2006). It is also common to use a hydroponic culture to examine the direct toxicity of persistent organic pollutants (Liu and Schnoor, 2008; Wang et al., 2012). K. obovata, belonging to Rhizophoraceae family, is a very common mangrove species distributed along the coastlines of East-Pacific Archipelagos and Asia. It also can be found in all mangrove associations in south China. Therefore, K. obovata is chosen as a model mangrove plant in the present study.
Materials and methods
Chemicals
Spiked BDE-209 was purchased from Dr. Ehrenstorfer (Germany) (purity > 99%). The analytical reagent (AR) grade Tween 20 and butanol were obtained from Sigma. Enzyme kits for SOD, POD and CAT measurements were purchased from Nanjing Jiancheng Bioengineering Institute, China. All chemicals and reagents were of analytical reagent grades. Deionized water was used throughout the experiment.
Experiment setup and sampling
One year old K. obovata seedlings were collected (the details have been listed in SI 2.1) and treated with four contamination levels of BDE-209 were 0.1 (low, L), 1 (medium, M), 5 (high, H) and 10 (very high, VH) mg l-1. Preliminary experiments showed that 0.5% of Tween 20 were sufficient to dissolve the highest contamination level used in this experiment for BDE-209, and the recovery percentages of 10 mg l-1 BDE-209 was 78.1%. Positive control with nutrient solution and solvent control with nutrient solution plus 0.5% Tween 20 for BDE-209 were prepared in the same way as the treatments but without BDE-209. The sampling days were at the end of weeks 1, 4 and 8.
Plant growth
The dry weight and Chlorophyll a (Chl a) and chlorophyll b (Chl b) were measured, and the details have been listed in SI 2.2.
Determination of antioxidative enzymes
The fresh samples of roots and leaves were used to assay the activities of different antioxidative enzymes (SOD, POD and CAT), and the details have been listed in SI 2.3.
Determination of malondialdehyde (MDA)
Malondialdehyde (MDA) is a final decomposition product of lipid peroxidation and has been used as an index for the status of lipid peroxidation. The details of MDA measurement have been listed in SI 2.4.
Determination of ROS production (O2·- and H2O2)
The fresh samples of roots and leaves were used to determine ROS production, that is, superoxide radical (O2·-) and hydrogen peroxide (H2O2). They were measured, and the details have been listed in SI 2.5.
Determination of tannins and related polyphenols
Total polyphenols (TP) and extractable condensed tannins (ECT) were measured, and the details have been listed in SI 2.6.
Determination of uptake, translocation and debromination of BDE-209
Extraction of BDE-209 in plant samples was performed by accelerated solvent extraction (ASE) purchased from Dionex (USA) according to the method described by our previous work Zhu et al. (2014a); Zhu et al. (2014b) with some modifications. In brief, 0.1 g freeze-dried plant sample was spiked with 10 µl PCB-209 in acetone (10 µg ml-1) as a surrogate standard. The sample mixture was then loaded into the ASE cell fitted with an ASE filter. Two cycles of plant sample extraction were performed using n-hexane as the solvent at a pressure of 1500 psi and temperature of 100 °C, each with a static time of five minutes. The extract was reduced to 1 ml by slow rotary evaporation. All the concentrated extracts were cleaned on a 10 mm i.d. florisil column (5 g, pre-washed by actone), eluted with 20 ml of n-hexane and concentrated to 1 ml prior to gas chromatography-mass spectrometry (GC-MS) analysis. The BDE-209 remained in the hydroponic culture solution was extracted three times by liquid-liquid extraction, using 10 ml of hexane as the solvent and 10 μl of PCB-209 (10 µg ml-1) as the surrogate standard, each at 15 minutes. The extract was concentrated by rotary evaporation to an accurate 1 ml for GC-MS analysis.
GC-MS 6890N/5975 of Agilent Technologies (Palo Alto, CA, USA) equipped with an Agilent 7638B series auto-injector Agilent Technologies and a capillary column (DB-5HT, crosslined 5% phenyl methyl siloxane, 15 m × 0.25 mm, 0.1 μm film thickness) was used to determine the concentration of BDE-209 in the extract. Helium and methane (Hong Kong Oxygen & Acetylene Co., Ltd) with a purity of 99.999% were used as carrier gases with a flow rate of 1.5 ml min-1. GC conditions were as follows: The column was coupled directly to the mass, and the flow rate for the helium carrier gas was 1.5 ml min-1. The injector temperature was 240°C. 2 μl of sample was injected in the splitless mode. The purge flow rate was 50 ml min-1. The temperature was programmed from 150°C (held isothermal for two minutes) to 300°C at a rate of 6°C/min, held isothermal for three minutes. MS condition: The Negative Chemical Ionization (NCI) source was used, and the temperature of both ion source and interface was 250°C. The mass selective detector (MSD) was operated in a selected ion monitoring (SIM) mode. The quantitative ions m/z 79, 81, 487 and 489 were selected for the detection of BDE-209, while ions m/z 464, 498 and 500 were used to monitor PCB-209. Solvent delay time was three minutes. The standard curves were made every time before and after the samples were run.
The limits of detection (LOD) of BDE-209, defined as a signal of three times the noise level, in roots, propagules, stems and leaves of K. obovata, were 0.680, 0.701, 0.683 and 0.695 ng g-1. In hydroponic culture solution, the LOD values of BDE-209 were 0.820 µg l-1. For the quality control, a matrix-spike recovery test was used to determine the recovery of the method. In brief, 0.1 grams of the freeze-dried mangrove plant sample cultured in the green house without PBDE spike was spiked with the mixed standard of 1 µg ml-1 BDE-209 and 0.1 µg ml-1 PCB-209. The same plant sample, but without PBDE spike, was used as the blank. The hydroponic culture solution was also spiked with the same concentration of BDE-209 and PCB-209 as mentioned above, while the solution without PBDE spike was used as the blank. The recoveries (n = 6) of BDE-209 and PCB-209 in plant tissues and hydroponic culture solution were in the range of 70.1 to 89.3 and 94.3 to 102.0%, respectively. Most of the recovery percentages were close to 90%, so the reported concentrations were not corrected by the surrogate recovery rate obtained from the matrix spiked test.
Statistical analyses
Mean and standard deviation of the three replicates for each treatment or control at each sampling time were calculated. A parametric two-way analysis of variance (ANOVA) followed by a Tukey’s multiple comparison test were performed to test any significant differences in the dependent variables (i.e., biomass, Chl a, Chl b, SOD, POD, CAT, MDA, O2·-, H2O2, TP and ECT) with BDE-209 treatments and sampling times as the two fixed factors. If interactions between these two factors were significant at p ≤ 0.05, the differences among BDE-209 treatments at the same sampling time were compared by a parametric one-way ANOVA. Data were tested for the normality and homogeneity of variance assumption of the parametric ANOVA test, and no transformation was needed. All statistical analyses were done by a Statistical Package for Social Science (SPSS Version 16.0), USA.
Results
Effects of BDE-209 on K. obovata
Growth
All treatment groups with different concentrations of BDE-209 in this experiment had no significant differences were observed for all growth parameters of K. obovata during the 8-week experiment, indicating that the growth of K. obovata seedlings may not be affected by BDE-209 contamination. The dry weight of root, propagule, stem and leaf of all treatments and the controls were comparable, ranging from 0.92-1.03, 6.41-6.99, 0.67-0.85 and 0.89-1.13 g, respectively. Similarly, the leaf number, ranging from 4-6, Chl a, b, a+b and a/b of the leaves in the ranges of 0.85-1.23, 0.30-0.52, 1.22-1.80 mg g-1 and 2.41-3.29, respectively, were not significantly different among all samples (Table 1).
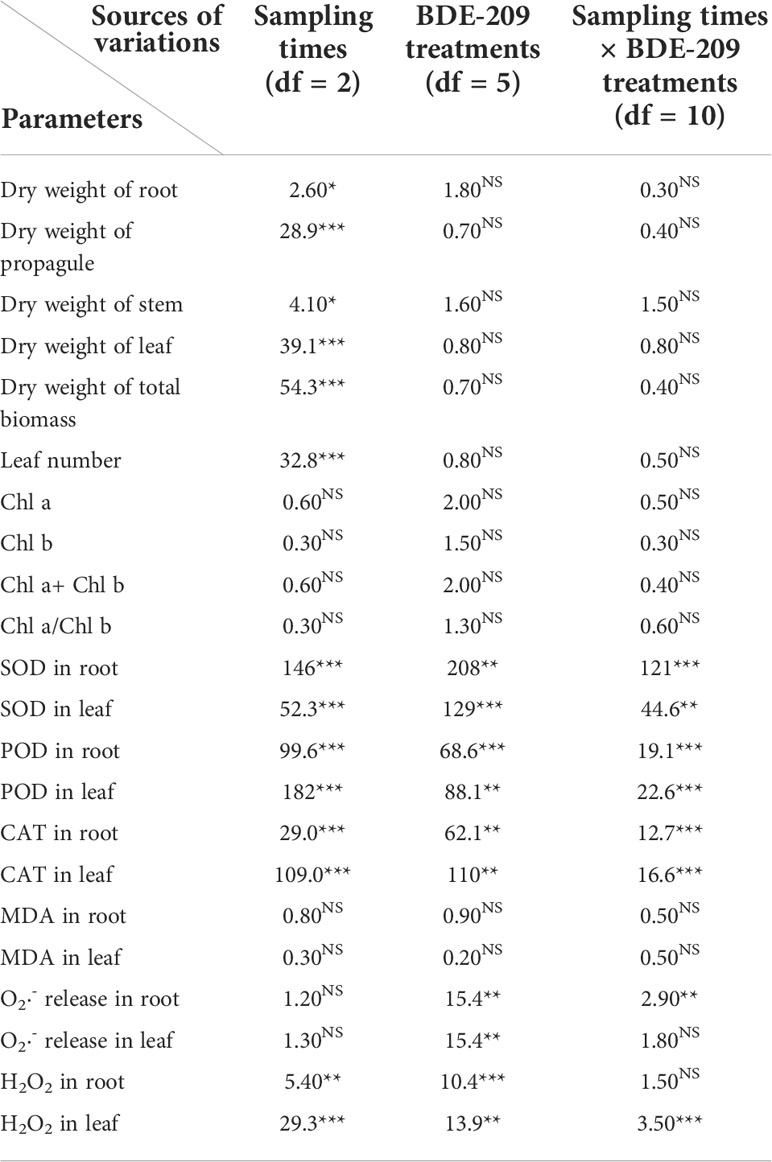
Table 1 F-values of two-way ANOVA tests showing the effects of sampling times and BDE-209 treatments on different parameters in roots and leaves of K. obovata (*: p ≤ 0.05; **: p ≤ 0.01; ***: p ≤ 0.001; NS: Not significant; df: Degree of freedom).
Antioxidative enzymes
The effects of BDE-209 on SOD were only observed under 10 mg l-1 BDE-209 in the root and leaf samples at weeks 1 and 4 (Figure S1). POD activities in both root and leaf samples at weeks 1 and 4 were significantly enhanced by BDE-209 contamination, even at 0.1 mg l-1 BDE-209 (Figure S1), but no effects were found on POD in week 8 (Figure S1). Similarly, CAT activity increased according to the levels of BDE-209 in weeks 1 and 4 but BDE-209 had no effects on CAT in week 8 (Figure S1). The two-way ANOVA statistical analyses showed that the effects of BDE-209 treatments, sampling times and interaction on SOD, POD and CAT activities were all significant at p ≤ 0.001, suggesting that the enzymatic responses were both time- and BDE-209 level-specific (Table 1). The enzymatic responses of roots to BDE-209 were comparable to that of leaves.
MDA
MDA contents in roots and leaves showed little changes during the experiment and were not affected by the contamination levels of BDE-209. The MDA content in roots and leaves in all samples ranged from 10.7-11.8 and 5.2-5.5 nmol g-1 FW, respectively.
ROS production
The responses of the O2·- release and H2O2 content of roots to BDE-209 treatments were comparable to those of leaves (Figure S2). Only the very high level of BDE-209 (10 mg l-1) caused significant increases in O2·- release in roots and leaves in weeks 1 and 4, but dropped to the same level as the control in week 8 (Figure S2). This pattern was similar to that of SOD activity (Figure S1). The content of H2O2 in both roots and leaves of K. obovata seedlings increased with the contamination levels of BDE-209 during the first four weeks, but BDE-209 did not have any significant effects in week 8 (Figure S2). Such trend was more or less the same as that of POD and CAT activities (Figure S1). BDE-209 treatments could stimulate the production of ROS in both leaves and roots of K. obovata seedlings, but the seedlings could recover from the oxidative stress of BDE-209 with the ROS levels returned to the control level in week 8. This implied that the excess ROS induced by BDE-209 might have been scavenged by the antioxidative defense system of K. obovata or the seedlings might have adapted to BDE-209 as the experiment progressed. Such short-term effect of BDE-209 on the production of ROS in K. obovata seedlings was different from that of BDE-47 (Wang et al., 2014a), which enhanced the production of ROS throughout the entire study period.
The correlation coefficients among antioxidative enzymes (SOD, POD and CAT), MDA and ROS production (O2·- release and H2O2) in the roots and leaves of K. obovata under BDE-209 exposure are summarized in Table S1. All three antioxidative enzymes were significantly correlated with each other, suggesting that they functioned together to counter-balance the harmful effects caused by ROS under BDE-209 treatments. The correlation coefficient values were comparable between the roots and leaves in K. obovata under BDE-209 exposure, implying that the two tissues had similar antioxidative enzyme responses to BDE-209.
Tannins and related polyphenols
Figure S3 showed that BDE-209 at 10 mg l-1 did not have any significant effects on TP and ECT contents during the 8-week experiment.
BDE-209 remained in hydroponic culture solution and accumulated in tissues of K. obovata
The residual concentrations of BDE-209 in the hydroponic culture solution did not have any significant changes with sampling time (Figure 1 and Table S2). This trend was different from that of BDE-47, which decreased significantly with sampling time under the two highest contamination levels, 5 and 10 mg l-1 BDE-47 (Wang et al., 2014b). The concentrations of BDE-209 remained in the hydroponic culture solution at the end of the experiment were significantly higher than that of BDE-47. Compared with the tissue concentrations of BDE-47, the concentrations of BDE-209 accumulated in the plant tissues of K. obovata were significantly lower (Wang et al., 2014b), even though the plant concentrations of BDE-209 increased significantly with time and contamination levels according to one-way ANOVA at p ≤ 0.05 (Table S2). Among plant tissues, the concentration of BDE-209 in root was much higher than that in propagule, followed by stem, and leaf had the least uptake (Figure 2).
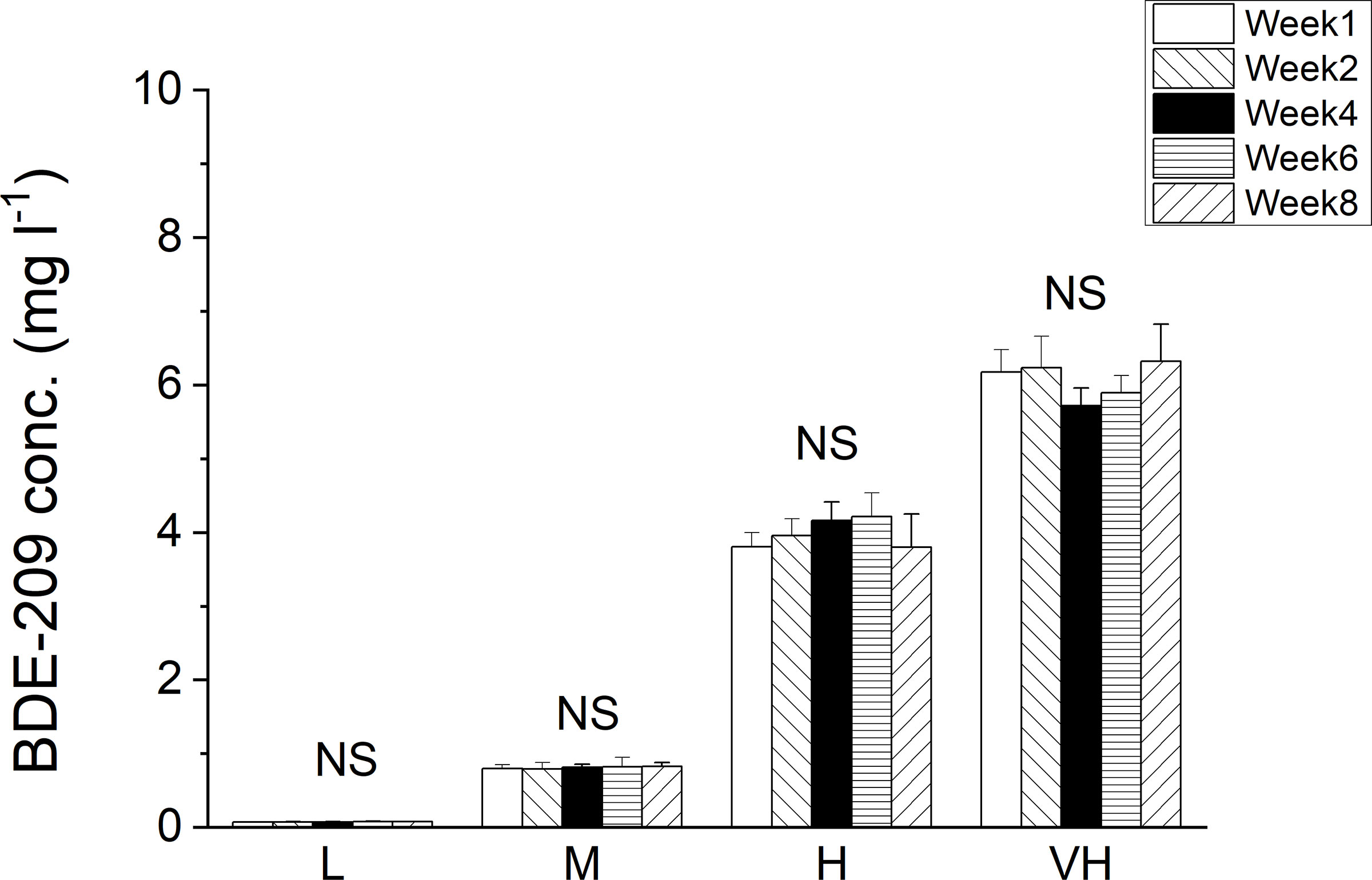
Figure 1 Concentrations of BDE-209 (mg l-1) remained in hydroponic culture solution under different contamination levels of BDE-209 (L: 0.1 mg l-1 BDE-209, M: 1 mg l-1 BDE-209, H: 5 mg l-1 BDE-209, VH: 10 mg l-1 BDE-209; NS: No significant difference among sampling times under the same treatment at p > 0.05 according to one-way ANOVA; Mean and standard deviation of three replicates are shown).
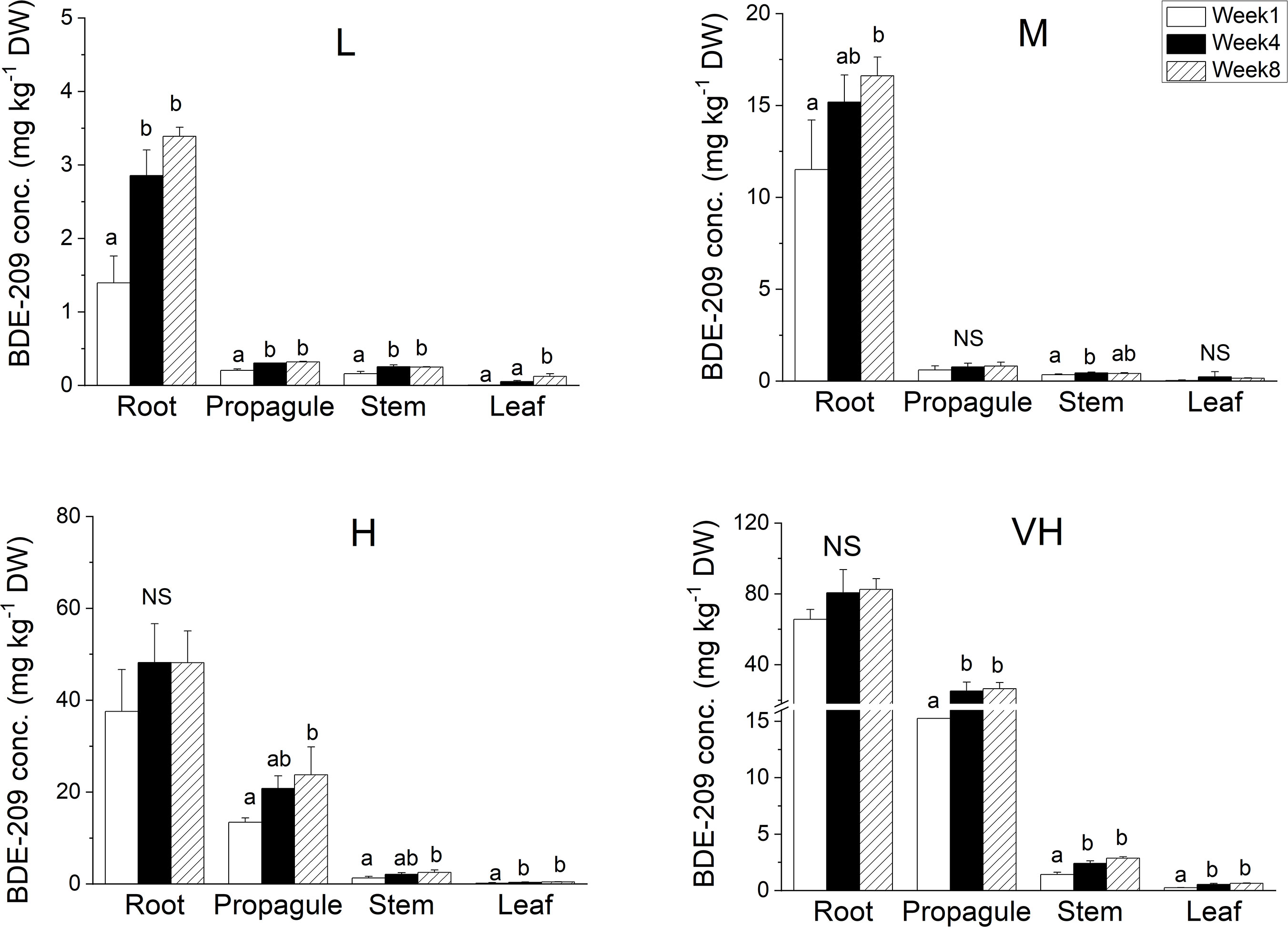
Figure 2 Concentrations of BDE-209 (mg kg-1 DW) in different tissues of K. obovata seedlings under different contamination levels of BDE-209 (L: 0.1 mg l-1 BDE-209, M: 1 mg l-1 BDE-209, H: 5 mg l-1 BDE-209, VH: 10 mg l-1 BDE-209; In the same tissue under the same treatment, bars with different letters show significant differences among sampling times at p ≤ 0.05 according to one-way ANOVA followed by Tukey’s tests; NS: Not significant; Mean and standard deviation of three replicates are shown).
The mass balance calculation of BDE-209 during the 8-week experiment showed that the residual BDE-209 remained in the hydroponic culture solution varied from 81.1 to 91.3% of the total inputs (Table 2), which were significantly higher than those of BDE-47 (11.5 to 27.7%) (Wang et al., 2014b). The amounts of BDE-209 remained in the hydroponic culture solution continuously increased from weeks 1 to 8 according to one-way ANOVA at p ≤ 0.05. The amounts of BDE-209 accumulated in plants increased significantly with contamination levels, and also from weeks 1 to 4, but no further increase thereafter according to one-way ANOVA at p ≤ 0.05 (Table 2). These results suggested that the plant uptake of BDE-209 might become slower as the experiment progressed. The total uptake of BDE-209 in plant tissues of K. obovata ranging from 2.70 to 14.9% (Table 2), as well as the net losses of BDE-209 (0.40 - 11.3%) were significantly lower than those of BDE-47 (Wang et al., 2014b) according to Student t-test at p ≤ 0.05 level. These findings indicated that the uptake and transformation of BDE-209 by K. obovata seedlings were less than that of BDE-47 under hydroponic culture. The losses of BDE-209 increased with time like those of BDE-47 but became relatively stable from week 4 onwards. Same as BDE-47, no new peaks with less bromine atoms were found in different tissues of K. obovata and in the hydroponic culture solution according to the GC-MS total ion chromatograms of BDE-209, suggesting that debromination did not occur in the present study lasted only eight weeks.
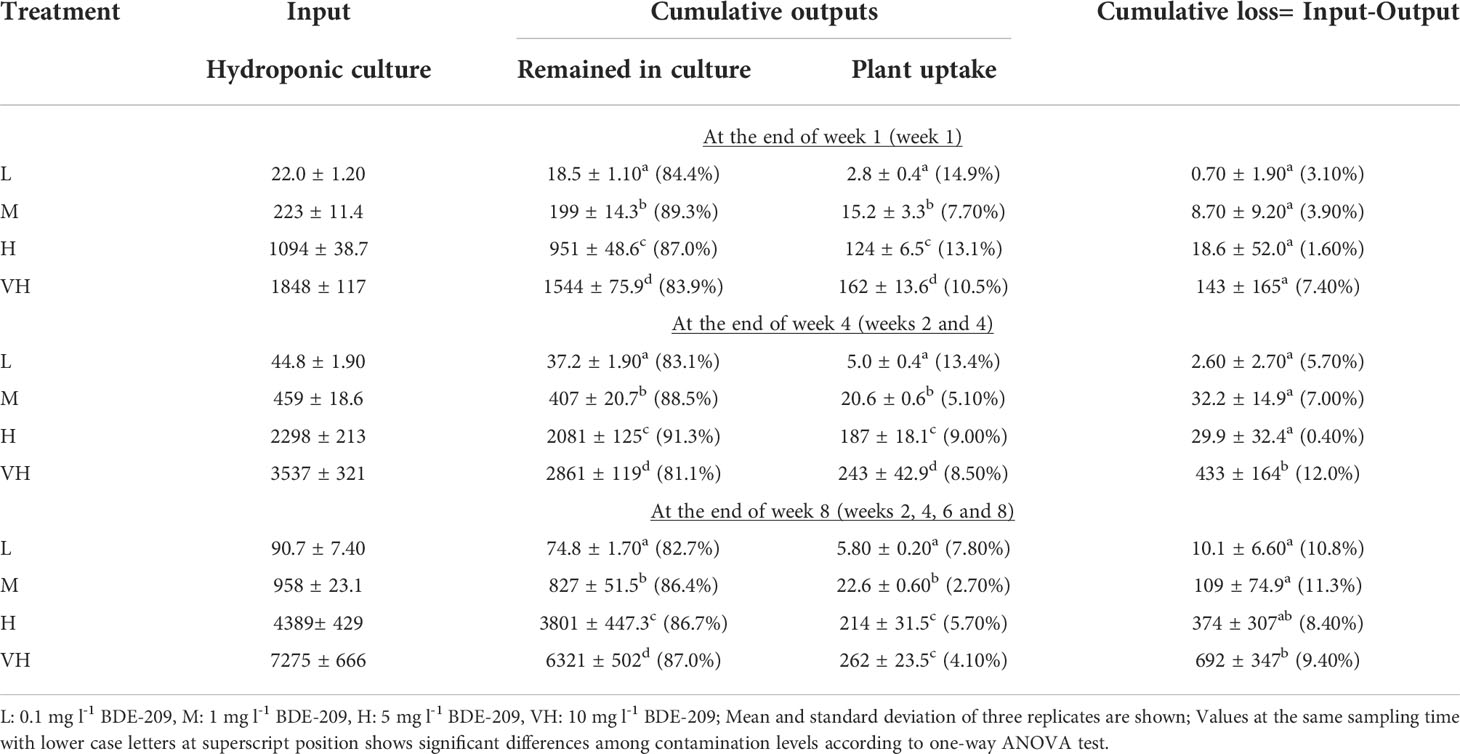
Table 2 Amounts of BDE-209 (µg) and percentages to total inputs (in brackets) remained in hydroponic culture solution, uptake in K. obovata seedlings and loss during an 8-week hydroponic experiment.
Discussion
Toxic effects of BDE-209
BDE-209 did not observe significant differences in growth and non-enzymatic antioxidants and may not have any effect on it, while the effects of BDE-47 on the growth, enzymatic and non-enzymatic antioxidants of K. obovata were concentration-dependent and lasted throughout the 8-week experiment (Wang et al., 2014a). The stimulatory effects of BDE-209 on SOD, POD and CAT in both leaves and roots of K. obovata were only significant in weeks 1 and 4. After 8-week experiment, the antioxidative enzymes of K. obovata exposed to BDE-209, even at the very high level (10 mg l-1) returned to the same values as the control. The more toxic and longer-lasting effects of BDE-47 than BDE-209 might be explained by two reasons: First, the uptake of BDE-47 by K. obovata would be higher than BDE-209, due to its lower log Kow value and higher solubility as reported by previous studies (Rahman et al., 2001; Teclechiel et al., 2009). Zhang et al. (2013) also suggested that the lower toxicity of BDE-209 was likely due to its high molecular weight or size, resulting small uptake by organisms. Second, BDE-47 and BDE-209 had different chemical structures leading to different stabilities between BDE-209 and BDE-47. Br and O are atoms with high electronegativity, while H has low electronegativity. As all the carbons on BDE-209 are connected with Br or O, the electrons are well spreaded, thus lowering the electrostatic potential and the reactivity, BDE-209 is thus the most stable PBDE congener (Huang et al., 2011). Higher stability of BDE-209 might also lead to lower bioavailability to the living organisms than BDE-47. Previous studies reported that BDE-47 was more toxic and bioaccumulative than BDE-209 (Gandhi et al., 2011).
Uptake of toxic pollutants by plants
Toxic contaminants such as persistent organic pollutants (POPs) and heavy metals in soil could be taken up by plants (Prasad, 2004; Yan and Tam, 2011). A wide range of terrestrial plant species, such as radish, zucchini, tobacco, nightshade, ryegrass, alfalfa, pumpkin, summer squash, maize, etc., could take up diverse groups of toxic pollutants, including PBDEs but with different abilities (Mueller et al., 2006; Huang et al., 2010b; Sun et al., 2013b). Especially, as wetland plants, many mangrove plant species were reported to uptake and bioaccumulate POPs. The root of B. gymnorrhiza accumulated more pyrene than that of K. candel grown in pyrene bottom-contaminated sediments (Ke et al., 2003). Pi et al. (2017) reported that two mangrove species, Excoecaria agallocha L. and K. obovata could uptake of mixed polycyclic aromatic hydrocarbons (PAHs) and PBDEs in wastewater. Chen et al. (2015) reported that nitrogen addition affected the uptake of PBDEs by a mangrove plant, Aegiceras corniculatum. All these indicated that plant uptake of POPs was species-specific. In the present study, K. obovata, the two commonest mangrove species in Hong Kong SAR, also took up BDE-209 from hydroponic culture. The mechanisms controlling the uptake of PBDEs in plant species are still unresolved, and one possible mechanism is that Fe plaque formed on root surfaces of mangroves could effectively immobilize organic pollutants (Pi et al., 2017). More research is needed to understand what causes the species-specificity of plant uptake.
Following uptake, toxic chemicals are translocated and accumulated in plants but at different amounts among tissues. The accumulation of PBDEs in stems and leaves may result from a combination of uptake through the root uptake from the soil pathway and foliar uptake from the air (Huang et al., 2011). In the control (without any spiking of PBDEs), no BDE-209 were detected in any plant tissue, indicating that the route of foliar uptake from the air to the aboveground accumulation could be neglected. The highest BDE-209 concentrations were in root, followed by propagule, stem, and the lowest in leaf. Similar declining trends of accumulation from root to leaf were recorded in the uptake of PCBs, DDTs and PBDEs in plants grown directly in contaminated soils (Lunney et al., 2004; Huang et al., 2011; Zhao et al., 2012). These declining trends further supported that the accumulation of PBDEs in plants was mainly due to the root uptake, followed by gradual transfer, to propagules, stems, and then to the leaves.
The total uptake and accumulation of BDE-209 in plant tissues of K. obovata increased with the initial contamination levels in the hydroponic culture solution. Similarly, Prasad (2004) also reported that the metal uptake increased with increasing metal concentrations in external medium. The uptake of PCB in the genus of Luzula exhibited a great increase relative to increasing soil PCB concentrations (Dawn Pier et al., 2002). The availability of the chemical and the factors restricted its bioavailability such as the lipid content and morphology of roots, the acquisition and/or translocation mechanisms of the plants, etc., may also affect the plant uptake.
Biotransformation of toxic pollutants in plants
Volatilization, degradation and metabolism are important processes determining the fate of pollutants following the uptake, translocation and accumulation of toxic pollutants in plants. It has been demonstrated that PBDEs could be degraded/debrominated into more toxic lower brominated congeners (de-PBDE products) in plants (Wang et al., 2011). The debrominated products of BDE-28, -47 and -99 were recorded in maize (Zhao et al., 2012), and a debromination product (BDE-28) was also identified in the young whole pumpkin plant after exposure to BDE-47 (Sun et al., 2013b). These studies showed that the debromination of PBDEs occurred but its rates and pathways varied from plant species to species.
In the present study, BDE-209 was not debrominated and no newly-borne lower brominated congeners were found in plant tissues and in the hydroponic culture. Previous research showed that the debromination of PBDEs took long time and was more preferably under anaerobic condition (Robrock et al., 2008; Zhu et al., 2014b). As the studies on the degradation, metabolism and fate of PBDEs are still limited to terrestrial plants with very few studies in aquatic ecosystems (Huang et al., 2010b; Wang et al., 2011; Wang et al., 2012; Sun et al., 2013b), the debromination of PBDEs in mangrove plants which are saline, aquatic and ligneous deserves more attention and future study.
The toxic effects of only one PBDE congener were examined in the present study. However, natural environments are often contaminated by a mixture of PBDE congeners. The behavior of PBDE mixture in mangrove soil and the interactions between different PBDE congeners should be further studied. Additionally, the present mangrove microcosm work only investigated the responses of mangrove seedlings to a single addition of BDE-209 to the hydroponic culture at the beginning of the study. However, in natural environments, PBDE contamination from waste dumping or wastewater discharge may occur from time to time. The effects of intermittent and/or continuous discharges of PBDEs on mangrove plants merit further research.
Conclusions
When compared with BDE-47, BDE-209 did not observe any significant negative effects on the growth of K. obovata seedlings. Our results suggested that BDE-209 was less toxic to K. obovata than BDE-47.
The K. obovata seedlings could also absorb, translocate and accumulate BDE-209 from the culture solution. However, most of the spiked BDE-209 was still remained in the hydroponic culture solution. These results suggested that BDE-209, due to its higher molecular weight and higher log Kow, was more difficult to be removed by mangrove system than BDE-47.
This was the first comprehensive study revealing the effects of PBDEs on the growth, enzymatic and non-enzymatic antioxidative responses of mangrove seedlings, as well as their tolerance to PBDE stresses. The abilities of mangrove species to remove, uptake and accumulate PBDEs were reported. The work provided important information on the fate of PBDEs, which could be used for selecting the appropriate species for phytoremediation.
Data availability statement
The original contributions presented in the study are included in the article/Supplementary Material. Further inquiries can be directed to the corresponding authors.
Author contributions
YW, conceptualization, methodology, software, writing - original draft, and visualization. Y-TD, software, validation, and investigation. NT, writing - review and editing. All authors contributed to the article and approved the submitted version.
Funding
This work was supported by the Natural Science Foundation of Tianjin (grant numbers 17JCQNJC02400 and 18JCYBJC96000), the National Training Programs for Innovation and Entrepreneurship of Undergraduates (grant number 202110069002 and 202210069014).
Conflict of interest
The authors declare that the research was conducted in the absence of any commercial or financial relationships that could be construed as a potential conflict of interest.
Publisher’s note
All claims expressed in this article are solely those of the authors and do not necessarily represent those of their affiliated organizations, or those of the publisher, the editors and the reviewers. Any product that may be evaluated in this article, or claim that may be made by its manufacturer, is not guaranteed or endorsed by the publisher.
Acknowledgments
We wish to thank the editors and the reviewers for their valuable comments and suggestions on this paper.
Supplementary material
The Supplementary Material for this article can be found online at: https://www.frontiersin.org/articles/10.3389/fmars.2022.955770/full#supplementary-material
References
Alaee M., Arias P., Sjodin A., Bergman A. (2003). An overview of commercially used brominated flame retardants, their applications, their use patterns in different countries/regions and possible modes of release. Environ. Int. 29, 683–689. doi: 10.1016/S0160-4120(03)00121-1
Binelli A., Sarkar S. K., Chatterjee M., Riva C., Parolini M., Bhattacharya B. D. (2007). Concentration of polybrominated diphenyl ethers (PBDEs) in sediment cores of sundarban mangrove wetland, northeastern part of bay of Bengal (India). Mar. Pollut. Bull. 54, 1220–1229. doi: 10.1016/j.marpolbul.2007.03.021
Chen J., Zhou H. C., Wang C., Zhu C. Q., Tam N. F.Y. (2015). Short-term enhancement effect of nitrogen addition on microbial degradation and plant uptake of polybrominated diphenyl ethers (PBDEs) in contaminated mangrove soil. J. Hazardous Materials 300, 84–92. doi: 10.1016/j.jhazmat.2015.06.053
Chen L. G., Huang Y. M., Peng X. C., Xu Z. C., Zhang S. K., Ren M. Z. (2009). PBDEs in sediments of the beijiang river, China: Levels, distribution, and influence of total organic carbon. Chemosphere 76, 226–231. doi: 10.1016/j.chemosphere.2009.03.033
Dawn Pier M., Zeeb B. A., Reimer K. J. (2002). Patterns of contamination among vascular plants exposed to local sources of polychlorinated biphenyls in the Canadian Arctic and subarctic. Sci. Total Environ. 297, 215–227. doi: 10.1016/S0048-9697(02)00134-1
Gandhi N., Bhavsar S. P., Gewurtz S. B., Tomy G. T. (2011). Can biotransformation of BDE-209 in lake trout cause bioaccumulation of more toxic, lower-brominated PBDEs (BDE-47, -99) over the long term? Environ. Int. 37, 170–177. doi: 10.1016/j.envint.2010.08.013
Huang G. Y., Wang Y. S., Sun C. C., Dong J. D., Sun Z. X. (2010a). The effect of multiple heavy metals on ascorbate, glutathione and related enzymes in two mangrove plant seedlings (Kandelia candel and Bruguiera gymnorrhiza). Oceanol Hydrobiol Stud. 39, 11–25. doi: 10.2478/v10009-010-0010-z
Huang H. L., Zhang S. Z., Christie P. (2011). Plant uptake and dissipation of PBDEs in the soils of electronic waste recycling sites. Environ. pollut. 159, 238–243. doi: 10.1016/j.envpol.2010.08.034
Huang H. L., Zhang S. Z., Christie P., Wang S., Xie M. (2010b). Behavior of decabromodiphenyl ether (BDE-209) in the soil-plant system: Uptake, translocation, and metabolism in plants and dissipation in soil. Environ. Sci. Technol. 44, 663–667. doi: 10.1021/es901860r
Huang H. L., Zhang S. Z., Wang S., Lv J. T. (2013). In vitro biotransformation of PBDEs by root crude enzyme extracts: Potential role of nitrate reductase (NaR) and glutathione S-transferase (GST) in their debromination. Chemosphere 90, 1885–18922. doi: 10.1016/j.chemosphere.2012.10.013
Jin S. W., Yang F. X., Hui Y., Xu Y., Lu Y. A., Liu J. K. (2010). Cytotoxicity and apoptosis induction on RTG-2 cells of 2,2',4,4'-tetrabromodiphenyl ether (BDE-47) and decabrominated diphenyl ether (BDE-209). Toxicol. Vitro 24, 1190–1196. doi: 10.1016/j.tiv.2010.02.012
Ke L., Wang W. Q., Wong T. W. Y., Wong Y. S., Tam N. F. Y. (2003). Removal of pyrene from contaminated sediments by mangrove microcosms. Chemosphere 51, 25–34. doi: 10.1016/S0045-6535(02)00811-1
Li J., Liu X., Yu L. L., Zhang G., Li X. D., Lee C. S. L., et al. (2009). Comparing polybrominated diphenyl ethers (PBDEs) in airborne particles in guangzhou and Hong Kong: Sources, seasonal variations and inland outflow. J. Environ. Monit. 11, 1185–1191. doi: 10.1039/B900525K
Liu J., Schnoor J. L. (2008). Uptake and translocation of lesser-chlorinated polychlorinated biphenyls (PCBs) in whole hybrid poplar plants after hydroponic exposure. Chemosphere 73, 1608–1616. doi: 10.1016/j.chemosphere.2008.08.009
Lunney A. I., Zeeb B. A., Reimer K. J. (2004). Uptake of weathered DDT in vascular plants: Potential for phytoremediation. Environ. Sci. Technol. 38, 6147–6154. doi: 10.1021/es030705b
Luo Q., Cai Z. W., Wong M. H. (2007). Polybrominated diphenyl ethers in fish and sediment from river polluted by electronic waste. Sci. Total Environ. 383, 115–127. doi: 10.1016/j.scitotenv.2007.05.009
Mai B. X., Chen S. J., Luo X. J., Chen L. G., Yang Q. S., Sheng G. Y. (2005). Distribution of polybrominated diphenyl ethers in sediments of the pearl river delta and adjacent south China Sea. Environ. Sci. Technol. 39, 3521–3527. doi: 10.1021/es048083x
Mueller K. E., Mueller-Spitz S. R., Henry H. F., Vonderheide A. P., Soman R. S., Kinkle B. K., et al. (2006). Fate of pentabrominated diphenyl ethers in soil: Abiotic sorption, plant uptake, and the impact of interspecific plant interactions. Environ. Sci. Technol. 40, 6662–6667. doi: 10.1021/es060776l
Ouyang X., Guo F. (2016). Paradigms of mangroves in treatment of anthropogenic wastewater pollution. Sci. Total Environ. 544, 971–979. doi: 10.1016/j.scitotenv.2015.12.013
Pi N., Wu Y., Zhu H. W., Wong Y. S., Tam N. F.Y. (2017). The uptake of mixed PAHs and PBDEs in wastewater by mangrove plants under different tidal flushing regimes. Environment. Pollut. 231, 104–114. doi: 10.1016/j.envpol.2017.07.085
Portet-Koltalo F., Guibert N., Morin C., de Mengin-Fondragon F., Frouard A. (2021). Evaluation of polybrominated diphenyl ether (PBDE) flame retardants from various materials in professional seating furnishing wastes from French flows. Waste Manage. 131, 108–116. doi: 10.1016/j.wasman.2021.05.038
Prasad M. N. V. (2004). Heavy metal stress in plants: From biomolecules to ecosystems. 2nd Ed (Heidelberg: Springer-verlag), 462–465.
Qiu Y. W., Zhang G., Guo L. L., Zheng G. J., Cai S. Q. (2010). Bioaccumulation and historical deposition of polybrominated diphenyl ethers (PBDEs) in deep bay, south China. Mar. Environ. Res. 70, 219–226. doi: 10.1016/j.marenvres.2010.05.004
Rahman F., Langford K. H., Scrimshaw M. D., Lester J. N. (2001). Polybrominated diphenyl ether (PBDE) flame retardants. Sci. Total Environ. 275, 1–17. doi: 10.1016/S0048-9697(01)00852-X
Ramu K., Kajiwara N., Tanabe S., Lam P. K. S., Jefferson T. A. (2005). Polybrominated diphenyl ethers (PBDEs) and organochlorines in small cetaceans from Hong Kong waters: Levels, profiles and distribution. Mar. Pollut. Bull. 51, 669–676. doi: 10.1016/j.marpolbul.2005.02.041
Robrock K. R., Korytar P., Alvarez-Cohen L. (2008). Pathways for the anaerobic microbial debromination of polybrominated diphenyl ethers. Environ. Sci. Technol. 42, 2845–2852. doi: 10.1021/es0720917
Sun J. L., Chen Z. X., Ni H. G., Zeng H. (2013a). PBDEs as indicator chemicals of urbanization along an urban/rural gradient in south China. Chemosphere 92, 471–476. doi: 10.1016/j.chemosphere.2013.01.036
Sun J. T., Liu J. Y., Yu M., Wang C., Sun Y. Z., Zhang A. Q., et al. (2013b). In vivo metabolism of 2,2',4,4'-tetrabromodiphenyl ether (BDE-47) in young whole pumpkin plant. Environ. Sci. Technol. 47, 3701–3707. doi: 10.1021/es4003263
Teclechiel D., Sundstrom M., Marsh G. (2009). Synthesis of polybrominated diphenyl ethers via symmetrical tetra- and hexabrominated diphenyliodonium salts. Chemosphere 74, 421–427. doi: 10.1016/j.chemosphere.2008.09.046
Wang Y. W., Jiang G. B., Lam P. K. S., Li A. (2007). Polybrominated diphenyl ether in the East Asian environment: A critical review. Environ. Int. 33, 963–973. doi: 10.1016/j.envint.2007.03.016
Wang Y., Tam N. F. Y. (2013). “Effects of a congener of polybrominated diphenyl ethers (BDE-47) on the growth of two mangrove plant species, their uptake, translocation and biotransformation,” in In 13th International Conference on Environmental Science and Technology (CEST 2013). Athens, Greece.
Wang S., Zhang S. Z., Huang H. L., Lu A. X., Ping H. (2012). Debrominated, hydroxylated and methoxylated metabolism in maize (Zea mays l.) exposed to lesser polybrominated diphenyl ethers (PBDEs). Chemosphere 89, 1295–1301. doi: 10.1016/j.chemosphere.2012.05.026
Wang S., Zhang S. Z., Huang H. L., Zhao M. M., Lv J. T. (2011). Uptake, translocation and metabolism of polybrominated diphenyl ethers (PBDEs) and polychlorinated biphenyls (PCBs) in maize (Zea mays l.). Chemosphere 85, 379–385. doi: 10.1016/j.chemosphere.2011.07.002
Wang Y., Zhu H., Tam N. F. Y. (2014a). Effect of a polybrominated diphenyl ether congener (BDE-47) on growth and antioxidative enzymes of two mangrove plant species, Kandelia obovata and Avicennia marina, in south China. Mar. pollut. Bull. 85 (2), 376–384. doi: 10.1016/j.marpolbul.2014.02.012
Wang Y., Zhu H., Tam N. F. Y. (2014b). Polyphenols, tannins and antioxidant activities of eight true mangrove plant species in south China. Plant Soil 374 (1), 549–563. doi: 10.1007/s11104-013-1912-9
Yan Z. Z., Tam N. F. Y. (2011). Temporal changes of polyphenols and enzyme activities in seedlings of Kandelia obovata under lead and manganese stresses. Mar. pollut. Bull. 63, 438–444. doi: 10.1016/j.marpolbul.2011.04.027
Zezza D., Tait S., Della Salda L., Amorena M., Merola C., Perugini M. (2019). Toxicological, gene expression and histopathological evaluations of environmentally realistic concentrations of polybrominated diphenyl ethers PBDE-47, PBDE-99 and PBDE-209 on zebrafish embryos. Ecotoxicol Environ. Saf. 183, 109566. doi: 10.1016/j.ecoenv.2019.109566
Zhang X. X., Tang X. X., Zhou B., Wang Y. (2013). Effects of decabromodiphenyl ether (BDE-209) on inter-specific competition between two species of marine bloom-forming microalgae. PloS One 8, e56084. doi: 10.1371/journal.pone.0056084
Zhao M. M., Zhang S. Z., Wang S., Huang H. L. (2012). Uptake, translocation, and debromination of polybrominated diphenyl ethers in maize. J. Environ. Sci. 24, 402–409. doi: 10.1016/S1001-0742(11)60748-1
Zhou H. C., Tam F. Y. N., Cheung S. G. (2019). “Implication of tannin changes in two mangrove plants, Kandelia obovata and Bruguiera gymnorhiza under PBDE contamination,” in In 9th International Conference on Marine Pollution and Ecotoxicology (ICMPE-9). O–37. University of Hong Kong, Hong Kong
Zhu H. W., Wang Y., Tam N. F. Y. (2014a). Microcosm study on fate of polybrominated diphenyl ethers (PBDEs) in contaminated mangrove sediment. J. Hazardous Mater 265, 61–68. doi: 10.1016/j.jhazmat.2013.11.046
Keywords: Mangroves, Kandelia obovata, BDE-209, ecotoxicology, uptake, accumulate
Citation: Wang Y, Du Y-T and Tam NFY (2022) Effects of decabromodiphenyl ether (BDE-209) on a mangrove plant, Kandelia obovata and the uptake, translocation and accumulation of BDE-209. Front. Mar. Sci. 9:955770. doi: 10.3389/fmars.2022.955770
Received: 29 May 2022; Accepted: 01 July 2022;
Published: 22 July 2022.
Edited by:
Meilin Wu, South China Sea Institute of Oceanology (CAS), ChinaReviewed by:
Kit Yue Kwan, Beibu Gulf University, ChinaYouji Wang, Shanghai Ocean University, China
Xiaoshou Liu, Ocean University of China, China
Copyright © 2022 Wang, Du and Tam. This is an open-access article distributed under the terms of the Creative Commons Attribution License (CC BY). The use, distribution or reproduction in other forums is permitted, provided the original author(s) and the copyright owner(s) are credited and that the original publication in this journal is cited, in accordance with accepted academic practice. No use, distribution or reproduction is permitted which does not comply with these terms.
*Correspondence: Ying Wang, eWluZ3dhbmdAdGpjdS5lZHUuY24=; Nora Fung-Yee Tam, Znl0YW1AaGttdS5lZHUuaGs=