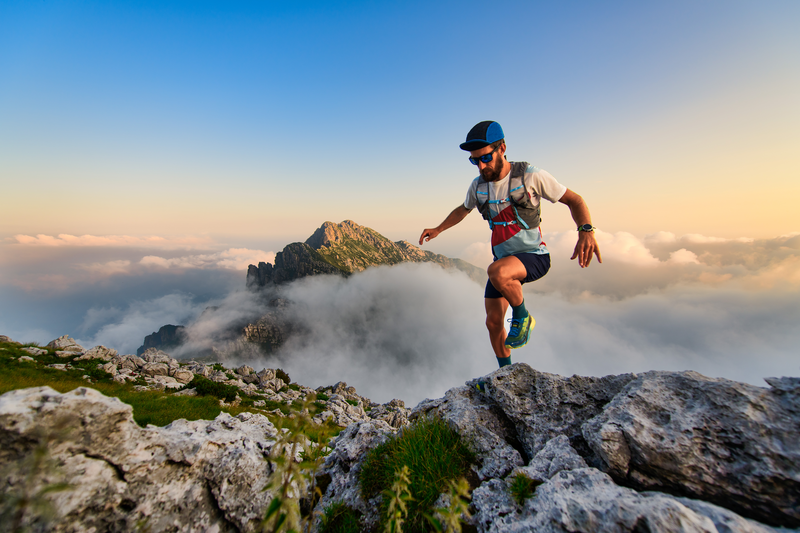
94% of researchers rate our articles as excellent or good
Learn more about the work of our research integrity team to safeguard the quality of each article we publish.
Find out more
DATA REPORT article
Front. Mar. Sci. , 02 August 2022
Sec. Marine Pollution
Volume 9 - 2022 | https://doi.org/10.3389/fmars.2022.954622
This article is part of the Research Topic Coastal and Marine Environmental Quality Assessments View all 28 articles
Chlorella is a green single-celled alga (Bito et al., 2020) that is easily cultivable, and it consists of 20% lipid content from dry biomass (Scarsella et al., 2010; Al-lwayzy et al., 2014). It is also the most popular microalga in the market and is often selected due to the higher level of carotenoids present and its ability to grow in a wide variety of climatic conditions (Gors et al., 2009). Chlorella has a high content of about 51%–58% protein, 12%–17% carbohydrate, and 14%–22% lipid (calculated as % of dry matter). Most importantly, its growth rate and biomass production largely depend on environmental factors (Spolaore et al., 2006).
Microalgae have received a lot of attention due to their potential benefits in many applications, and therefore, enhancing the biomass by regulating environmental factors responsible for the growth becomes necessary for feasible algal culture (Khan et al., 2018). The chemical composition of algae (cellular content) varies according to species and its physiological responses to biotic and abiotic factors such as temperature, illumination, pH, nutrients, and growth phase (Brown et al., 1993; Carvalho and Malcata, 2000). Apart from light intensity, other physicochemical parameters like temperature, pH, and salinity are also the vital environmental factors that influence the photosynthetic activity and behavior of microalgal growth rate (Blinová et al., 2015). Pulz and Gross (2004) stated that successful algal biotechnology mainly depends on selecting the right species with relevant properties for specific culture conditions and products.
Numerous studies have documented that environmental factors such as temperature, pH, salinity, and light have a substantial impact on the growth of microalgae (Mata et al., 2010; Zongo and Boussim, 2015; Chowdury et al., 2020). In general, studies on microalgae have shown that their growth was significantly enhanced when one or two optimum environmental factors were combined. The compensatory effects of numerous factors are observed to be responsible for this improvement (Cheng and He, 2014; Salama et al., 2018). The current study will explore how Chlorella vulgaris would respond to the ideal environmental parameters such as temperature, pH, salinity, and light intensity, both individually and in combination. The present study is an attempt to achieve high growth and biomass production with optimized environmental variables.
The NIOT vessel Sagar Paschimi was used for a scientific cruise to survey the microalgal diversity near the Puducherry coast. The study species C. vulgaris was isolated from the coastal waters (11° 54′ 27.54″ N; 79° 49′ 35.70″ E) and identified (JF894249, NCBI GenBank, USA) and deposited in a public repository at National Facility for Marine Cyanobacteria, Bharathidasan University, India (Accession number, BDU GD 003). The micro algae were cultured in 20-L polycarbonate carboys using F/2 medium (Guillard). The cell growth was monitored by cell count with the help of a Neubauer chamber (Santos and Mariano, 2014). The dry biomass was estimated by filtering 5 ml of the sample using 0.22-µm GFC filter paper and dried in a hot air oven for 24 h maintained at 70°C (Zhu and Lee, 1997).
The effects of physicochemical parameters like temperature, pH, and salinity were examined to identify the optimal growth conditions for C. vulgaris. Different temperatures like 20°C, 25°C, 30°C, 35°C, and 40°C were attempted in this experiment using separately controlled incubators (Scigenics Biotech, Orbitek) fitted with fluorescent lamps (42 µmol m−2 s−1). Similarly, studies were conducted at different pH, viz., 6, 7, 8, 9, and 10. The pH of the culture medium was measured using a pH meter (Metrohm), and the pH level was adjusted using NaOH and HCl to raise or lower the value. In another set of experiments, the effect of salinity on the growth rate was carried out at different salinities (0, 10, 20, 30, and 50 Practical Salinity Unit (PSU)). Salinity was determined using a refractometer (Atago, Japan), and the culture medium was adjusted with distilled water or NaCl to either reduce and/or increase the salinity. Experiments on different light intensities was carried out under phototrophic conditions using fluorescent lamp (Philips 23W) covered with different colored filters such as red (700–625 nm), orange (624–600 nm), blue (499–465 nm), violet (425–400 nm), green (569–500 nm), and yellow (599–670 nm), along with one positive control (+) (white light, 400–700 nm) and negative control (−) (dark).
The experiments were conducted over a 15-day period in triplicate. The biomass was estimated every 5 days, and the cell count was monitored daily. The values are expressed as mean ± S.E. The SPSS software (version 20.0) was used for the statistical analysis. To find out statistical differences, the data were subjected to a two-way analysis of variance (ANOVA) followed by Tukey’s multiple comparison post hoc test. Differences were statistically significant at p < 0.05 and p < 0.001.
In the present investigation, a higher growth rate was observed at 25°C in terms of cell count and biomass. The trend was then followed by 20°C and 30°C compared with 35°C and 40°C on the 15th day (Figure 1A). Higher biomass of around 1.0 g L−1 was achieved on the 10th day itself at 25°C (Supplementary Figure 1), and in some cases, maximum cell counts were observed from the 10th to 12th day of the experiment. In line with our observation, a report has documented that the optimum temperature for freshwater Chlorella is also 25°C (Singh and Singh, 2015). Another study has revealed that C. vulgaris cells were able to tolerate a higher temperature of even 40°C (Sharma et al., 2012). Our findings indicated that C. vulgaris can grow in a wide range of temperatures (20°C–35°C), but the best growth rate was noticed at 25°C, followed by 20°C. Importantly, the biomass tends to decrease at higher temperature.
Figure 1 (A) Growth of C. vulgaris culture at different temperature. (B) Growth of C. vulgaris culture at different pH. (C) Growth of C. vulgaris culture at different salinities. (D) Growth of C. vulgaris culture at different light intensities. (Experiments were conducted in triplicate and the values are expressed as mean ± S.E.).
Among the cultures maintained at different pH, pH 8.0 showed higher growth rate followed by pH 9.0 and 7.0 (Figure 1B). Cultures maintained at pH 6.0 and 10.0 are also observed with a considerable growth rate. A report has documented that C. vulgaris can grow in a wide range of pH, from 4 to 10 (Khalil et al., 2010). From the results of the present study, it was concluded that higher biomass production was obtained on 15th day, between pH 8 (0.959 g L−1) and 9 (0.924 g L−1) compared with other pH conditions (Supplementary Figure 2). Earlier reports have suggested that microalgal cultures grown at pH 8 to 9 favor high growth and biomass production (Difusa et al., 2015; Bartley et al., 2016; Qiu et al., 2017). Our study results indicated that all pH conditions tested (6–10) supported the growth of the algae, with the best growth rate being at pH 8, followed by pH 9.
The influence of different salinities (0, 10, 20, 30, and 50 PSU) was investigated in the present study on the growth rate of C. vulgaris. The better growth rate in terms of cell counts (Figure 1C) and biomass was observed at 30 PSU, followed by 20, 10, 0, and 50 PSU (Supplementary Figure 3). Previous studies have shown that the highest growth rate in terms of cell counts was observed at 30 PSU on the 15th day of the experiment (Jiang and Chen, 1999; Ranga Rao et al., 2007). In the present study, 30 PSU resulted in higher biomass (1.0 g L−1) on the 10th day compared with other salinity groups, and this observation is supported by a previous study by Fathi and Asem (2013). Interestingly, an important observation was noticed during the experiment, which highlighted that 0 PSU showed a better growth rate and biomass production than 50 PSU.
In the different light intensities experiments conducted under phototrophic conditions, blue light followed by red exhibited a significant increase in cell count (Figure 1D) on 15th day. Asuthkar et al. (2016) demonstrated that Chlorella pyrenoidosa cultured under blue light showed a higher growth rate than under red and white lights. In the present study, colored lights such as green and yellow showed fewer cell counts compared with control+ (white light). The results of the present study revealed that a higher biomass production was recorded on the 15th day in blue light–exposed cells (1.3 g L−1), followed by red (1.14 g L−1) and other light-exposed cells (Supplementary Figure 4).
On the basis of individual tests performed under various environmental conditions, the ideal environmental factors were combined and evaluated for the growth rate of C. vulgaris. The culture was kept at 25°C, pH 8.0, and 30 PSU with light covered with a blue filter. The results were fascinating in that the maximum production of cells and biomass (1.52 g L−1) was recorded under the blue light on the 10th day and was also the highest outcome in comparison with previous trials (Figures 2A, B). A study has also demonstrated that Pavlova lutheri grows at its maximum specific growth rate and has the highest lipid content when exposed to 30 to 40 PSU, pH 7.5 to 9.0, and 24 h of light (Shah et al., 2014). The findings of this study show that blue wavelengths, when combined with optimized environmental factors, favored the better growth rate of the algae and also enhanced the biomass production to a significant extent.
Figure 2 Combined experiment with optimized environmental variables: (A) cell growth and (B) biomass production. Experiments were conducted in triplicate and the values are expressed as mean ± S.E. The same letters (a, b, and c) indicate no significant difference between exposure groups at different exposure periods, whereas different letters indicate statistically significant differences (p < 0.05) between different exposure periods and groups.
It can be concluded that a temperature of 25°C, pH 8.0, salinity of 30 PSU, and blue light (499–465 nm) are the ideal parameters for the best growth of C. vulgaris. A combined experiment with optimized environmental factors showed enhanced growth rate and higher biomass production. Further, experiments are being explored to utilize the chlorella biomass to extract the most vital bioactive compounds and evaluate its pharmacological properties.
The original contributions presented in the study are included in the article/supplementary material. Further inquiries can be directed to the corresponding author.
AJ: Methodology validation, data processing, and writing—original draft. TK: Conceptualization, sampling design, sample collection, laboratory analysis, and data validation. BS: Laboratory analysis. SR: Manuscript corrections and suggestions. RK: Manuscript review. GD: Manuscript review, suggestion, and project administration. All authors contributed to the article and approved the submitted version.
The authors gratefully acknowledge the Ministry of Earth Sciences, Govt. of India, for funding this project. The authors are thankful to Dr. G. A. Ramadass, Director, National Institute of Ocean Technology, for his constant support and encouragement. The authors thank the Management of Meenakshi Academy of Higher Education and Research (MAHER) for the support. We would also like to thank Mr. Krupa Ratnam from National Institute of Ocean Technology for his untiring help in presenting the figures.
The authors declare that the research was conducted in the absence of any commercial or financial relationships that could be construed as a potential conflict of interest.
All claims expressed in this article are solely those of the authors and do not necessarily represent those of their affiliated organizations, or those of the publisher, the editors and the reviewers. Any product that may be evaluated in this article, or claim that may be made by its manufacturer, is not guaranteed or endorsed by the publisher.
The Supplementary Material for this article can be found online at: https://www.frontiersin.org/articles/10.3389/fmars.2022.954622/full#supplementary-material
Al-lwayzy S. H., Yusaf T., Al-Juboori R. A. (2014). Biofuels from the fresh water microalgae chlorella vulgaris (FWM-CV) for diesel engines. Energies. 7, 1829–1851. doi: 10.3390/en7031829
Asuthkar M., Gunti Y., Rao R., Rao C. S., Yadavalli R. (2016). Effect of different wavelengths of light on the growth of chlorella pyrenoidosa. Int. J. Pharma. Scien. Res. 7, 847–851. doi: 10.13040/IJPSR.0975-8232
Bartley M. L., Boeing W. J., Daniel D., Dungan B. N., Schaub T. (2016). Optimization of environmental parameters for nannochloropsis salina growth and lipid content using the response surface method and invading organisms. J. Appl. Phycol. 28, 15–24. doi: 10.1007/s10811-015-0567-8
Bito T., Okumura E., Fujishima M., Watanabe F. (2020). Potential of chlorella as a dietary supplement to promote human health. Nutrients. 12, 2524. doi: 10.3390/nu12092524
Blinová L., Bartošová A., Gerulová K. (2015). Cultivation of microalgae (Chlorella vulgaris) for biodiesel production. Res. Pap. Fac. Mater. Sci. Technol. Slovak. Univ. Technol. 23, 87–95. doi: 10.1515/rput-2015-0010
Brown M. R., Dunstan G. A., Jeffrey S. W., Volkman J. K., Barrett S. M., LeRoi J. M. (1993). The influence of irradiance on the biochemical composition of the prymnesiophyte isochrysis sp. J. Phycol 29, 601–612. doi: 10.1111/j.0022-3646.1993.00601.x
Carvalho A. P., Malcata F. X. (2000). Effect of culture media on production of polyunsaturated fatty acids by pavlova lutheri. Cryptogamie Algol. 21, 59–71. doi: 10.1016/S0181-1568(00)00101-X
Cheng D., He Q. (2014). Assessment of environmental stresses for enhanced micro algal biofuel production - an overview. Front. Energy Res. 2, 1–8. doi: 10.3389/fenrg.2014.00026
Chowdury K. H., Nahar N., Deb U. K. (2020). The growth factors involved in microalgae cultivation for biofuel production: A review. Comput. Water Energy Environ. Engin. 9, 185–215. doi: 10.4236/cweee.2020.94012
Difusa A., Talukdar J., Kalita M. C., Mohanty K., Goud V. V. (2015). Effect of light intensity and pH condition on the growth, biomass and lipid content of microalgae scenedesmus species. Biofuels 6, 37–44. doi: 10.1080/17597269.2015.1045274
Fathi M., Asem A. (2013). Investigating the impact of NaCl salinity on growth, β-carotene, and chlorophyll a in the content life of halophytes of algae chlorella sp. AACL Bioflux. 6, 241–245. http://www.bioflux.com.ro/docs/2013.20133143677
Gors M., Schumann R., Hepperle D., Karsten U. (2009). Quality analysis of commercial chlorella products used as dietary supplement in human nutrition. J. Appl. Phycol. 22, 265–276. doi: 10.1007/s10811-009-9455-4
Jiang Y., Chen F. (1999). Effects of salinity on cell growth and docosahexaenoic acid content of the heterotrophic marine microalga crypthecodinium cohnii. J. Industr. Microbiol. Biotechnol. 23, 508–513. doi: 10.1038/sj.jim.2900759
Khalil Z. I., Asker M. M., El-Sayed S., Kobbia I. A. (2010). Effect of pH on growth and biochemical responses of dunaliella bardawil and chlorella ellipsoidea. World J. Microbiol. Biotechnol. 26, 1225–1231. doi: 10.1007/s11274-009-0292-z
Khan M. I., Shin J. H., Kim J. D. (2018). The promising future of microalgae: current status, challenges, and optimization of a sustainable and renewable industry for biofuels, feed, and other products. Microb. Cell factor. 17, 36. doi: 10.1186/s12934-018-0879-x
Mata T. M., Martins A. A., Caetano N. S. (2010). Microalgae for biodiesel production and other applications: A review. Renew. Sust. Ener. Rev. 14, 217–232. doi: 10.1016/j.rser.2009.07.020
Pulz O., Gross W. (2004). Valuable products from biotechnology of microalgae. Appl. Microbiol. Biotechnol. 65, 635–648. doi: 10.1007/s00253-004-1647-x
Qiu R., Gao S., Lopez P. A., Ogden K. L. (2017). Effects of pH on cell growth, lipid production and CO2 addition of microalgae chlorella sorokiniana. Algal Res. 28, 192–199. doi: 10.1016/j.algal.2017.11.004
Ranga Rao A., Sarada R., Ravishankar G. A. (2007). Influence of CO2 on growth and hydrocarbon production in botryococcus braunii. J. Microbiol. Biotechnol. 17, 414–419.
Salama E. S., Hwang J. H., El-Dalatony M. M., Kurade M. B., Kabra A. N., Abou-Shanab R. A., et al. (2018). Enhancement of microalgal growth and biocomponent-based transformations for improved biofuel recovery: A review. Bioresource Technol. 258, 365–375. doi: 10.1016/j.biortech.2018.02.006
Santos K. R., Mariano A. B. (2014). Determination of optimal algae concentration for continuous growth of scenedesmus sp. in CHU and modified CHU media. Therm. Engin. 13, 52–58. doi: 10.5380/reterm.v13i2.62096
Scarsella M., Belotti G., De Filippis P., Bravi M. (2010). Study on the optimal growing conditions of chlorella vulgaris in bubble column photobioreactors. Chem. Engin. Trans. 20, 85–90. doi: 10.3303/CET1020015
Shah S. M.U., Che Radziah C., Ibrahim S., Latiff F., Othman M. F., Abdullah M. A.. (2014). Effects of photoperiod, salinity and pH on cell growth and lipid content of pavlova lutheri. Ann. Microbiol. 64, 157–164. doi: 10.1007/s13213-013-0645-6
Sharma R., Singh G. P., Sharma V. K. (2012). Effects of culture conditions on growth and biochemical profile of chlorella vulgaris. Plant Pathol. Microbiol. 3, 2–6. doi: 10.4172/2157-7471.1000131
Singh S. P., Singh P. (2015). Effect of temperature and light on the growth of algae species: A review. Renew. Sustain. Energy Rev. 50, 431–444. doi: 10.1016/j.rser.2015.05.024
Spolaore P., Joannis-Cassan C., Duran E., Isambert A. (2006). Commercial applications of microalgae. J. Biosci. Bioeng. 101, 87–96. doi: 10.1263/jbb.101.87
Zhu C. J., Lee Y. K. (1997). Determination of biomass dry weight of marine microalgae. J. Appl. Phycol. 9, 189–194. doi: 10.1023/A:1007914806640
Keywords: plankton, microalgae, Chlorella, marine environment, long term study
Citation: Josephine A, Kumar TS, Surendran B, Rajakumar S, Kirubagaran R and Dharani G (2022) Evaluating the effect of various environmental factors on the growth of the marine microalgae, Chlorella vulgaris. Front. Mar. Sci. 9:954622. doi: 10.3389/fmars.2022.954622
Received: 27 May 2022; Accepted: 28 June 2022;
Published: 02 August 2022.
Edited by:
Meilin Wu, South China Sea Institute of Oceanology (CAS), ChinaReviewed by:
Thilagam Harikrishnan, Pachaiyappa’s College for Men, IndiaCopyright © 2022 Josephine, Kumar, Surendran, Rajakumar, Kirubagaran and Dharani. This is an open-access article distributed under the terms of the Creative Commons Attribution License (CC BY). The use, distribution or reproduction in other forums is permitted, provided the original author(s) and the copyright owner(s) are credited and that the original publication in this journal is cited, in accordance with accepted academic practice. No use, distribution or reproduction is permitted which does not comply with these terms.
*Correspondence: Thalavai Shivasankarasubbiah Kumar, dHNrdW1hcm5pb3RAZ21haWwuY29t; Gopal Dharani, ZGhhcmFAbmlvdC5yZXMuaW4=; Anthony Josephine, YWpvc2VwaGluZWFudGhvbnlAZ21haWwuY29t
Disclaimer: All claims expressed in this article are solely those of the authors and do not necessarily represent those of their affiliated organizations, or those of the publisher, the editors and the reviewers. Any product that may be evaluated in this article or claim that may be made by its manufacturer is not guaranteed or endorsed by the publisher.
Research integrity at Frontiers
Learn more about the work of our research integrity team to safeguard the quality of each article we publish.