- Department of Ocean Studies and Marine Biology, Pondicherry University, Port Blair, India
An investigation was carried out to understand the effect of a cyclone Vardah on the functioning of macrobenthos. The assessment was accomplished by comparing before cyclone (BC) and after cyclone (AC) data of macrobenthos. Additionally, temporal changes in the faunal assemblages were evident through multivariate techniques. Five distinguished assemblages could be noticed through the Bray–Curtis similarity representing different phases of the cyclone. The cyclonic effect also resulted in the recruitment of some macrobenthic species and the loss of a few during the study period. Biological trait analysis identified subsurface deposit feeders, upward and downward conveyers, Ecological Group IV, mid-mobile macrobenthos and size class of 2–4 cm as the important groups that dominated the functioning of the macrobenthic community immediately after the cyclone. The resilience and recruitment of macrobenthos were explained using functional redundancy.
Introduction
A storm or a tropical cyclone is one of the phenomena that frequently occur in the tropical region. The Arabian Sea and the Bay of Bengal of the Indian subcontinent account for about 4.8% of total storms worldwide (Aguttes et al., 2000). For example, between 2011 and 2016, the Arabian Sea and Bay of Bengal encountered 20 mild and severe tropical cyclones (Vasudha, 2017). Storms have a big impact on the coastal areas, especially the sedimentary shoreline, and can alter coastal community structure temporally (Balica et al., 2012). Storms cause drastic shifting in sediment, salinity changes in tidal flats, and redistribution of the macrobenthic community, resulting in low species diversity and distribution and dominance of opportunistic species (Posey et al., 1996; Sukumaran et al., 2016). The impacts of storm disturbance on the macrobenthic community vary depending on the time of disturbances, including frequency and timing relative to recruitment events and life histories of resident fauna (Levin, 1984). The majority of the studies on the storm impact on coastal community structure are focused on the diversity, distribution, and assemblage patterns of benthic communities (Sukumaran et al., 2016). Although a few studies attempted to define the functional nature of the coastal community in response to storms (Posey et al., 1996; Sukumaran et al., 2016), there was a lack of explanation for the ecosystem functioning and the impact of disruption. Species diversity, composition, and abundance have long been utilized in traditional biodiversity research (Reizopoulou & Nicolaidou, 2007). Its findings were insufficient to describe the functioning of the entire ecosystem. Functional diversity (FD) (Petchey & Gaston, 2002; Villéger et al., 2008; Linden et al., 2012; Delfan et al., 2021) was introduced to describe the diversity of functional traits found among a group of species in a given ecosystem (Petchey & Gaston, 2002; Villéger et al., 2008; Linden et al., 2012; Mouillot et al., 2013; Delfan et al., 2021). Functional redundancy is mainly used to describe the resilience of lost species due to any perturbation.
Biological trait analysis (BTA) (Bremner et al., 2006) was used to characterize how the macrobenthic population responds to the storm. The trait-based approach establishes a more systematic connection between ecosystem services and species traits, as the species trait is the primary characteristic by which the organism influences ecosystem processes (Petchey & Gaston, 2002). Most of the studies were based on the feeding habitat trait approach (Posey et al., 1996; Jumars et al., 2016; Sukumaran et al., 2016). Apart from the trophic structure, the functioning of the benthic community is dependent on bioturbation, habitat, mobility, mortality rate, and body size (Donadi et al., 2015). There have been few studies to validate the ecosystem functioning in India’s marine benthic system (Vijapure et al., 2019; Bhowmik & Mandal, 2021; Sivadas et al., 2021). This study is the first attempt to investigate the functioning of the marine benthic community in the Andaman and Nicobar Islands. The objective of the present study is to access the effects of the cyclone Vardah on the assemblage pattern and the functioning of intertidal soft-bottom macrofauna. We hypothesized that extreme weather events like cyclones can disrupt the functioning of the macrobenthic community by creating a void in niche space and giving the opportunity to other species to fill the niche space.
Sampling and methodology
Study area
The Andaman and Nicobar group of islands is a union territory of India, and its geographical position borders are the Southeast Asian countries (i.e., Thailand, Indonesia, and Myanmar). The archipelago is oriented north–south, coordinates between 6°N and 14°N, and 92°E and 94°E and also covers an area of 8,249 km2. The archipelago consists of 572 islands and islets, out of which 38 are permanently inhabited islands. The Andaman and Nicobar Islands comprise both critical and pristine intertidal ecosystems. On 4th December 2016, a low-pressure area developed over the south Andaman sea, later it turned into a very severe cyclonic storm Vardah. At midnight of 7th December, it became a cyclonic storm Vardah. On 8th December, it passed the Port Blair coast with a wind speed of 50–60 kph (IMD, 2017). Sampling was conducted at Chatham (CH), Port Blair coast; it was a part of an ongoing investigation on the efficiency of sampling gear (Nosad et al., 2021) for macrobenthic fauna. Hence, only biological samples were collected for the analysis. CH (N11°41´08˝ and E092°43´23˝) was selected for the collection of macrobenthic faunal samples (Figure 1). It is located in the northeast part of the Port Blair coast, characterized by the presence of a vast exposed intertidal area, very fine sand sediment, and dissipative beach (Pandey & Thiruchitrambalam, 2019). The lush vegetation of seagrasses (e.g., Halodule uninervis and Thalassia hemprichii) covers the mid and low tide zone.
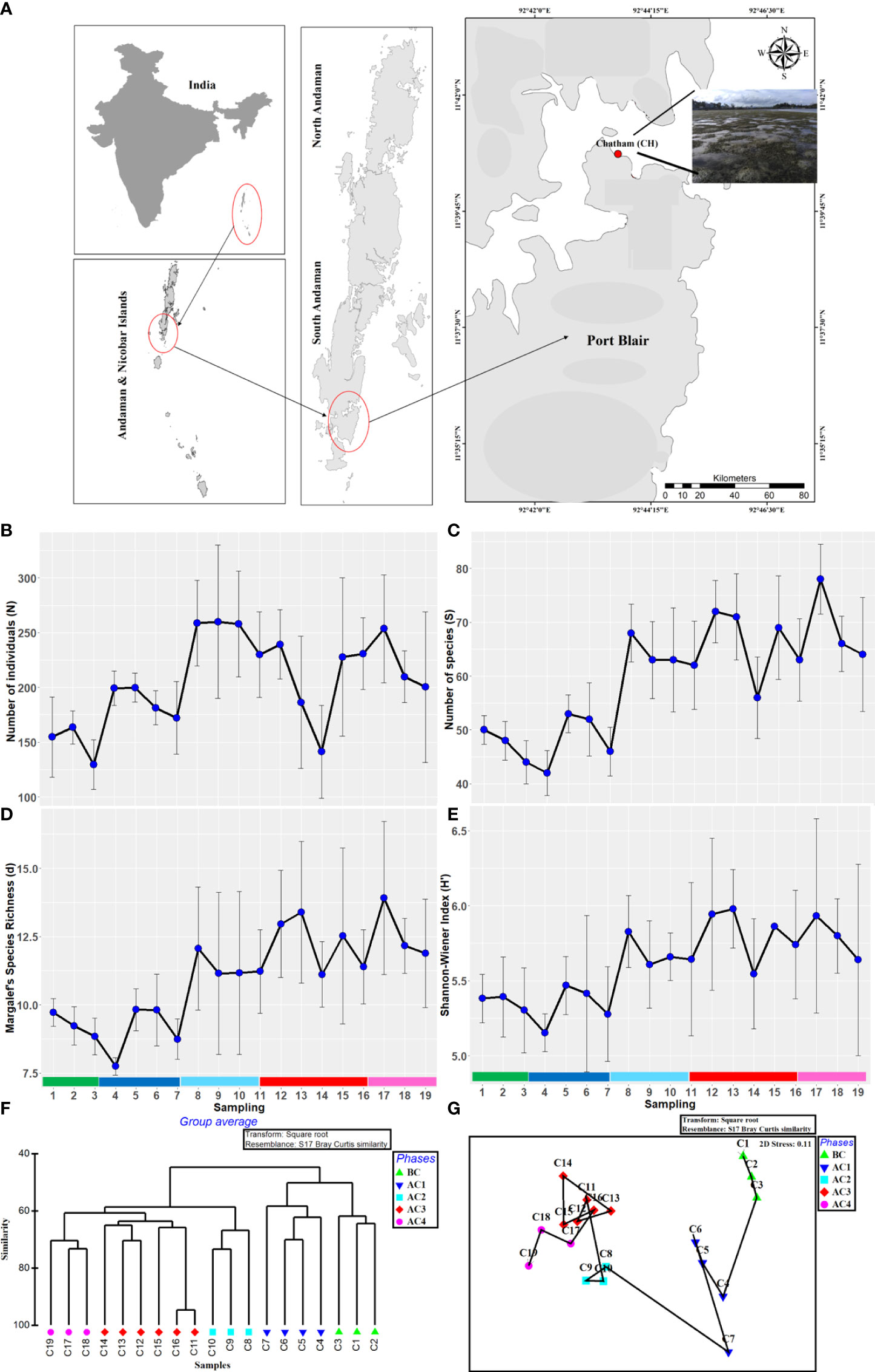
Figure 1 (A) Study area map. Macrobenthic community attributes: (B) number of individuals, (C) number of species, (D) Margalef’s index, (E) Shannon–Wiener index, (F) BC similarity dendrogram, and (G) nMDS plot. Sample size, n = 9 for panels (B–E). The color shades on the x-axis of line diagram depict cyclonic phases as shown in dendrogram and nMDS plot. BC, before cyclone; AC1, after cyclone phase 1; AC2, after cyclone phase 2; AC3, after cyclone phase 3; AC4, after cyclone phase 4.
Sampling methods
Initial sampling was conducted monthly for 7 months (September 2016–March 2017). Bimonthly sampling was conducted to assess the recruitment time for the macrobenthos for 2 years (April 2017–March 2019) because the recruitment time for the lost species due to perturbation may take several months to years. A total of 171 samples (1 station × 3 replicates × 3 waterlines × 19 samplings) were collected using a 25 × 25 cm quadrate during the study period. The collected sediment was screened through a test sieve with a mesh size of 0.5 mm, Magnesium chloride solution was added as a relaxant. Fixation was done with 5% buffered formalin mixed with rose bengal stain. Faunal groups were separated from the residue, enumerated, labeled, and stored in 70% ethanol for further examination. All taxa were identified up to a possible lower level of Linnaean classification (genus or species).
Data analysis
Univariate measures include the number of individuals (N ind.m−2), number of species (S), Margalef’s species richness (d), Shannon–Wiener diversity (H′ log2), and Evenness (j ′) and Simpson’s dominance index (1-Lambda). The collected raw data were pooled into time-series data of 19 samplings (mean of replicates per waterlines). The Bray–Curtis similarity resemblance matrix (after root transformed abundance data) was used to construct a dendrogram and non-metric multidimensional scaling (nMDS) plot. ANOSIM was used to determine the significant difference between the groups. All the analyses were performed using PRIMER v.6 (Clarke and Gorley, 2015). Six biological traits (i.e., bioturbation, ecological group, habitat, mobility, size, and feeding guild) were used for BTA and further subdivided into several modalities according to individual traits (Supplementary Table 1). The Fuzzy coding (Chevene et al., 1994) was implemented to process the abundance data for BTA. Trait data were collected from the online library and online software (e.g., AMBI; MarLIN BIOTIC; http://www.species-identification.org/) and literature (Queirós et al., 2013; Jumars et al., 2016; Ríos-Jara et al., 2009). Principal components analysis (PCA) bi-plot was constructed using CANOCO v4.5. FD was estimated using Rao’s quadratic entropy (RaoQ) (Rao, 1982). FD analysis was performed using “FD Package” (Laliberté et al., 2014) in R studio v 4.0.1. Functional redundancy was estimated by taking the ratio between FD and the Shannon–Wiener diversity index (FD/H`) (Linden et al., 2012). Linear regression was applied between FD and H` (Micheli & Halpern, 2005). All the line diagrams and linear regression were done using the “ggplot2” package in R studio v 4.0.1.
Results
Macrobenthic community structure
A total of 96 taxa belonging to 37 families under seven phyla were recorded during the study. Among the groups, Polychaeta contributed the highest number of taxa (73 taxa) followed by Amphipoda (8 taxa) and Gastropoda (6 taxa). A reduction in the species richness of total macrofauna from 45 into 42 taxa and an increase in the number of polychaete taxa from 29 to 35 taxa were noted immediately after the cyclone (AC1). The reduction in the number of taxa was observed immediately after the cyclone in Decapoda and Bivalvia. The number of taxa was increased from the fifth sampling after cyclone (AC) due to the recruitment of additional species in the area (Table 1). A total of 19,949 individuals were recorded during the study. Overall, the number of individuals ranged between 469 and 1,709 ind.m−2 (mean: 1050 ± 354 ind.m−2). Polychaetes were numerically important contributing 71% of the total population. The number of individuals increased up to 4% just after the cyclone occurred. Values of diversity indices were decreased after the cyclone; in contrast, the number of individuals increased (5.11%). H` was recorded lowest (5.13 ± 0.12) during the cyclone compared to other sampling periods. Margalef’s d values were recorded at all-time low during the cyclone. The number of species, Margalef’s d, and Shannon–Wiener diversity showed an increasing trend after AC1 (Figures 1B–D).
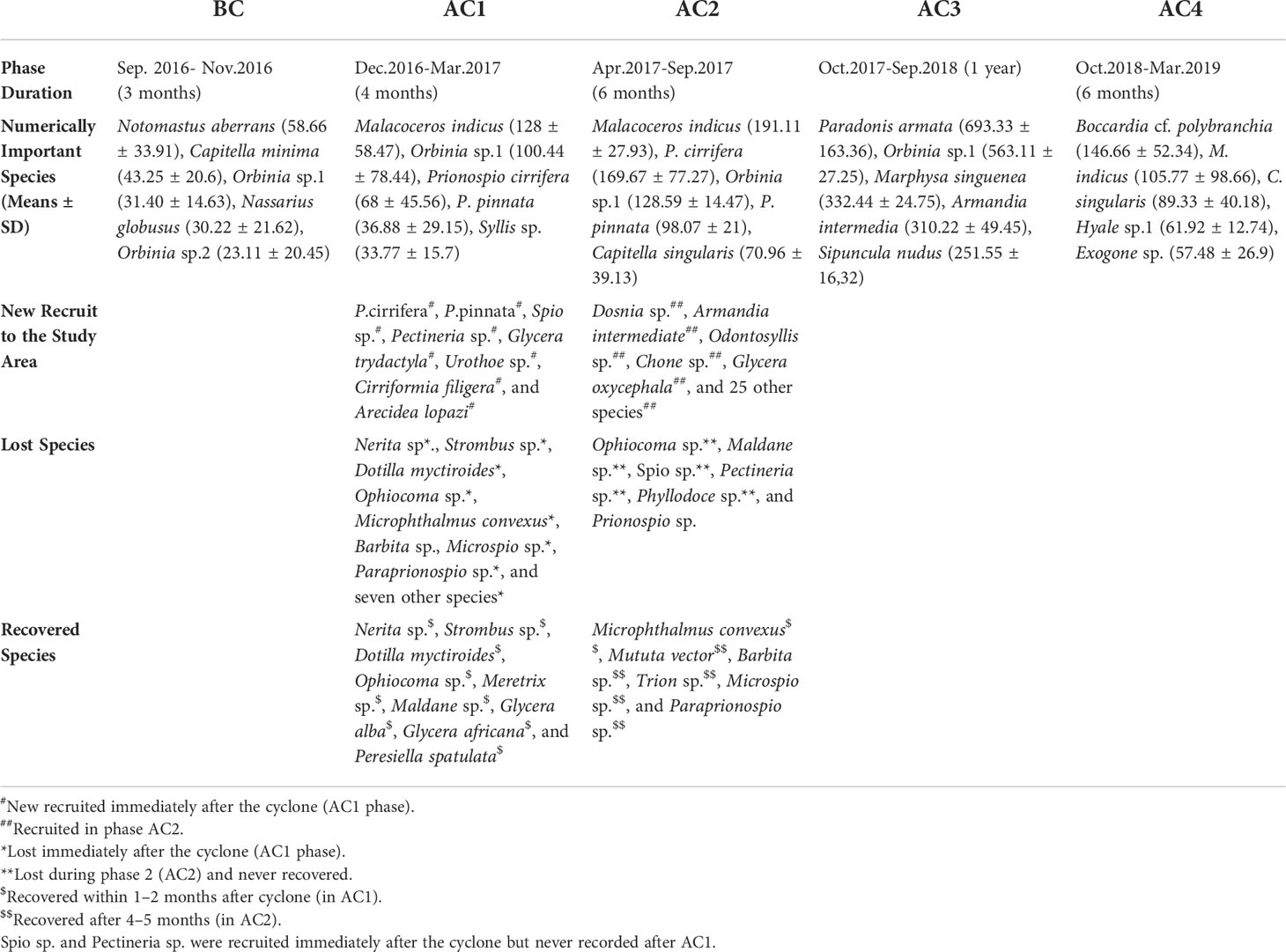
Table 1 Summary of numerically important species, species that lost due to cyclone and recovered, and new recruit to the station.
At 58% Bray–Curtis similarity, the resulting dendrogram showed five groups representing before the cyclone (BC), after the cyclone phase 1 (AC1), phase 2 (AC2), phase 3 (AC3), and phase 4 (AC4) (Figure 1F). ANOSIM showed a significant difference between these groups (Global R = 0.868, p < 0.001), implying distinct assemblages occurred during the timeline of the cyclone. On the nMDS plot, a trajectory was drawn from the first sampling to the last and showed a clear temporal pattern in the macrobenthic faunal assemblages (Figure 1G). The discriminating and characterizing species for each group were listed (Supplementary Table 2).
Biological trait analysis
The results of BTA showed that all traits exhibited more than 80% of the variation in the first two axes of PCA (Supplementary Table 3). Overall crawlers, bio-diffusors, surface deposit feeders, ecological group I, low mobile macrobenthos, and size class of <2cm were characteristics of BC, which were replaced by subsurface deposit feeders, upward and downward conveyers, ecological group IV, mid-mobile macrobenthos, and size class of 2–4 cm immediately after the cyclone (AC1). From the PCA analysis, it was observed that the feeding trait and ecological groups were the important traits for explaining the changes in BC and AC (Figures 2A, B). The most notable observation was that the predominant opportunistic Sub-surface deposit feeders (Ssdf) was represented by Capitellidae BC, which was replaced by Spionidae immediately AC. Similarly, the predominant bio-diffusors BC was replaced by UC/DC (Prionospio cirrifera, Prionospio pinnata, etc.) AC. The PCA results for all the traits showed a mixed pattern of trait distribution indicating that the traits in the area are contributing collectively to the functioning of macrobenthos community and are not affected by the temporal variations (Figures 2A–F). Although the trait distribution did not show any changes, variation in faunal assemblages was noticed, implying that the niche spaces of lost species were being filled by new recruits. For example, Sub-surface deposit feeders (Ssdf) was the dominant feeding modality in both the AC3 and AC4 phases where Sub-surface deposit feeders (Ssdf) was represented by Paradonis armata and Orbinia sp.1 in AC3 and were replaced by Boccardia cf. polybranchia and M.indicus in the AC4 phase. Sometimes, changes in assemblage patterns are reflected in the modalities for a few traits such as bioturbation. In the PCA, the assemblage of AC2 is dominated by UC/DC but replaced by surface depositors and BD in AC3 (Figure 2E).
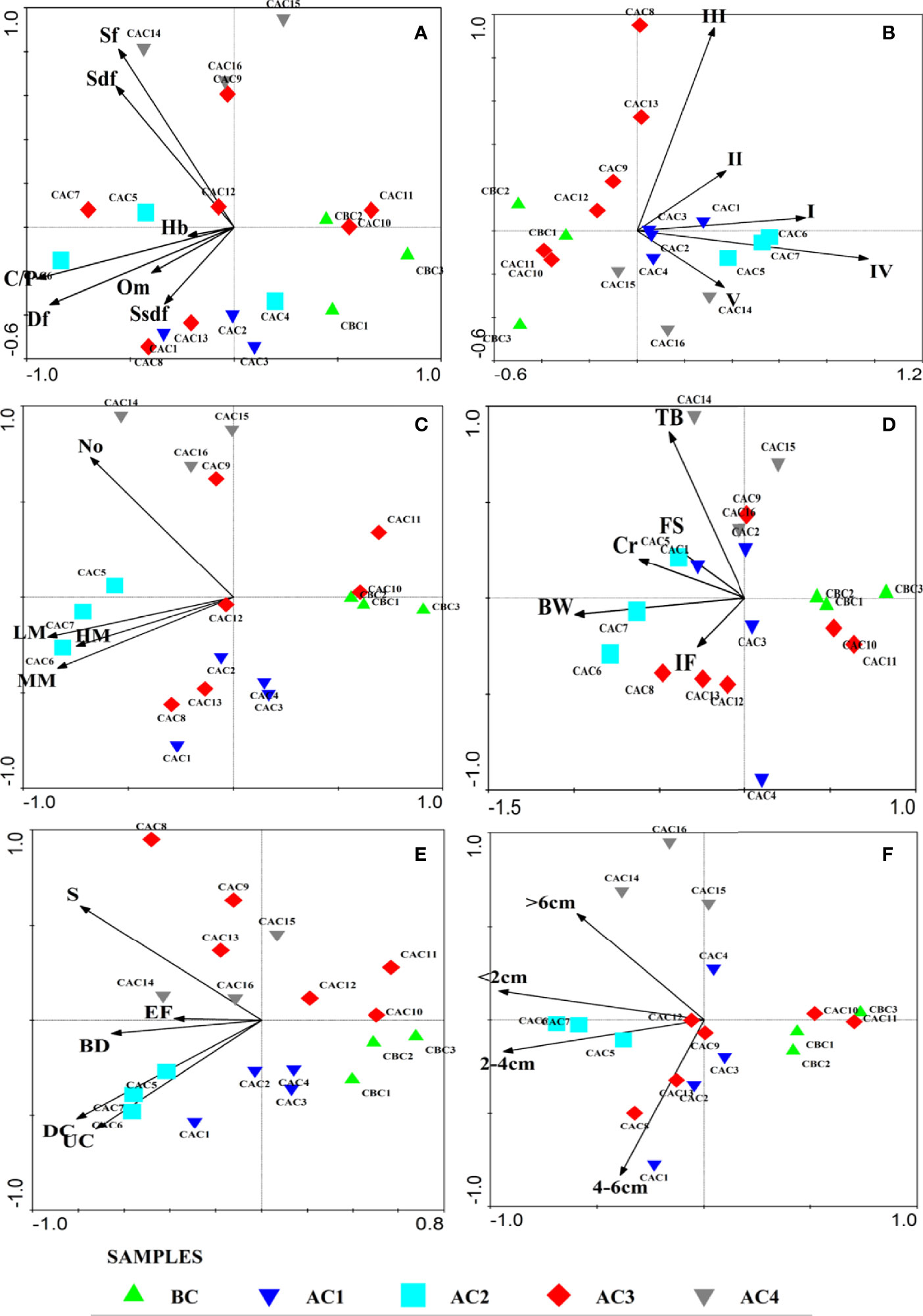
Figure 2 PCA bi-plot of different traits. (A) Feeding trait, (B) ecological groups, (C) locomotion, (D) habitat, (E) bioturbation, and (F) size. BC, before cyclone; AC1, after cyclone phase 1; AC2, after cyclone phase 2; AC3, after cyclone phase 3; AC4, after cyclone phase 4.
Functional diversity, functional redundancy, and recovery
The FD decreased immediately after the cyclone; moreover, the FD was showing a declining trend after the cyclone. However, the FD value was increased after the phase of AC2 (Supplementary Figure 1A). Similarly, the ratio between FD and H` showed a declining pattern immediately after the cyclone until the AC2 phase, it tends to increase afterward, and the highest value was recorded during AC4 (Supplementary Figure 1B). The low FD/H` value indicates high redundancy after the cyclone and the redundancy decreased during the AC4 phase. The linear regression model (Supplementary Figure 1C) showed a positive relationship between H` and FD (R Square: 0.387) with a low slope value of 0.057, indicating the high functional redundancy of macrobenthos during the study period. The high functional redundancy supports the resilience of species in an ecosystem, which was seen during the study. A total of 32 macrobenthos were recruited out of which three opportunistic species were recruited immediately and 29 were recruited in AC2. Seventeen macrobenthos species were lost when the cyclone encountered out of which 10 recovered immediately, whereas 7 species took 4–5 months for recovery. Two species Spio sp. and Prionospio sp. were encountered for a very short period (only in AC1) and lost throughout the study period (Table 1).
Discussion
An immediate effect of cyclone Vardah was observed in both the structure and the function of the macrobenthic community. The resilience of species was observed in the form of shared niches between species. Several studies have concluded that disturbances such as cyclones or tsunamis alter the sediment texture, grain size, and availability of organic matter and other environmental parameters, which are essential factors for stabilizing the benthic faunal community (Lomovasky et al., 2011; Sukumaran et al., 2016; Sugumaran et al., 2019). The physical disturbance may cause defaunation effects on a large area (Lu & Wu, 2000), which led to the dominance of opportunistic species. The present study found immediate recruitment of opportunistic species such as P. cirifera, P. pinnata, and Spio sp. After the cyclone, which leads to a reduction of species diversity, a similar increase in opportunistic species due to perturbation was recorded elsewhere (Lomovasky et al., 2011; Sukumaran et al., 2016).
Feeding traits and ecological groups were the characterizing functions working immediately AC. It is already established that feeding traits can explain the resource utilization post cyclone (Sukumaran et al., 2016); however, the use of more traits gives a better picture of ecosystem functioning (Bremner et al., 2006). In most of the studies, it was noticed that the dominance of opportunistic species often leads to a decrease in the number of feeding modalities (Peng et al., 2013). In the current study, feeding modalities were high immediately after the cyclone suggesting opportunistic species performing multiple functions in the ecosystem. Bioturbation is mainly influenced by the size of the organisms, density, and the mode of bioturbation of the residence macrofaunal group (Griffiths et al., 2017). An increase in a large size class, which was mostly predator glycerids, was observed immediately AC, which may be the reason for the increase in the bioturbation trait. All opportunistic species were Ssdf and SDF, and Karlson et al. (2010) have suggested an increase in Ssdf and SDF prevalence in the secondary producers. An increase in secondary producers lures the predators; hence, there was an increase in large size carnivorous group AC. The burrowing activity of organisms can disrupt the sediment and allow the oxygen and detritus penetration to go deep inside the sediment, which further helps in enriching the sediment with organic matter (Aller, 1983). The present study noted an increase in burrowing activities suggesting the reworking of sediment. Moreover, the burrowing activities support other organisms to settle which may be reflected in an increase in diversity in the AC2 phase.
FD is an essential tool to understand the ecosystem functioning (Degen et al., 2018); several studies have been carried out to understand the ecosystem functioning in response to any disturbance or habitat heterogeneity or environmental changes (Micheli & Halpern, 2005; Delfan et al., 2021). The dominance of opportunistic species with the same trait in the community and low species richness can cause a decrease in FD (Leung & Cheung, 2017). A similar reduction in FD was observed immediately AC due to an increase in the number of opportunistic species. Species diversity and FD are linearly correlated (Wong & Dowd, 2015; Leung & Cheung, 2017), so a decrease in species diversity can also cause a decrease in FD. The low FD can also define that the community relies on local retention rather than dispersal species from other areas (Boström et al., 2010). This can lead to the recovery of lost species due to the cyclone. Functional redundancy positively affects species resilience due to any perturbation (Biggs et al., 2020). The present study showed a high FD/H` value during AC4 and a low value up to the AC2 phase which means that the redundancy was highest immediately after the cyclone and gradually reduced in time. The value of slope obtained from the linear regression was close to zero, which denotes a high-redundancy value. High redundancy suggests that the availability of niche space is also high and multiple species have similar functions (Mason et al., 2005; Micheli & Halpern, 2005). The low FD and high redundancy during the cyclone imply an immediate effect of the cyclone on the macrobenthic community. The retention of local species made the platform for the resilience of lost species. Ecological communities with more redundant species are more likely to display resilience or gather stability than the community having low redundant species (Biggs et al., 2020). Although there are limited studies on the impact of the cyclone on the ecosystem functioning of the benthic organisms, we can still relate a part of our results to Sukumaran et al. (2016), where the cyclone Phyan affected the feeding trait of the polychaete community. The present study found similar results where feeding traits shifted due to the effect of cyclone Vardah. Gamito et al. (2012) suggested that a large-scale meteorological disturbance can cause recolonization by introducing larvae, juveniles, or adults from a faraway area to the location and can alter the species composition of the area. Similar results were observed during the study where six species were recruited newly to the location in the AC1 phase.
Conclusion
An immediate reduction in the diversity indices and shifting assemblage pattern of macrobenthos was observed, suggesting that cyclone has an immediate effect on macrobenthos rather than the long term. The effect resulted in the loss of species and a new recruit of species to the station. The dominant of opportunistic species demonstrated performing multiple functions aiming to occupy the niche space of lost species. Overall, the functional approach was more evident and explanatory in detecting the impact than general community attributes; hence, the use of the functional approach is recommended in similar studies. Finally, the recovery time for the lost species differs for different species as some recovered immediately, some took 4–5 months, and some species never recovered. The lack of environmental variables is a limitation of this study.
Data availability statement
The original contributions presented in the study are included in the article/supplementary material. Further inquiries can be directed to the corresponding author.
Author contributions
NS: Collection and analysis of samples, processing of data, writing-original draft, statistical analysis. RL: Analysis and taxonomic identification of macrobenthos. GT: Conceptualization, sampling design, data validation and reviewing and editing. All authors contributed to the article and approved the submitted version.
Acknowledgments
The authors are thankful to the authorities of Pondicherry University for providing the laboratory facilities and the University fellowship to (SN) for carrying out this research work. We thank the reviewers for their valuable suggestions. This work is dedicated to Late Prof. Akkur V. Raman, Andhra University, Visakhapatnam, an eminent marine biologist and a pioneer in the field of marine benthic ecology and coastal pollution research in India.
Conflict of interest
The authors declare that the research was conducted in the absence of any commercial or financial relationships that could be construed as a potential conflict of interest.
Publisher’s note
All claims expressed in this article are solely those of the authors and do not necessarily represent those of their affiliated organizations, or those of the publisher, the editors and the reviewers. Any product that may be evaluated in this article, or claim that may be made by its manufacturer, is not guaranteed or endorsed by the publisher.
Supplementary material
The Supplementary Material for this article can be found online at: https://www.frontiersin.org/articles/10.3389/fmars.2022.953985/full#supplementary-material
References
Aguttes J. P., Schrive J., Goldstein C., Rouze M., Raju G. (2000). MEGHA- TROPIQUES, a satellite for studying the water cycle and energy exchanges in the tropiques. Int. Geosci. Remote Sens. Symposium (IGARSS) 7, 3042–3044. doi: 10.1109/igarss.2000.860329
Aller R. C. (1983). The importance of the diffusive permeability of animal burrow linings in determining marine sediment chemistry. J. Mar. Res. 41 (2), 299–322. doi: 10.1357/002224083788520225
Balica S. F., Wright N. G., van der Meulen F. (2012). A flood vulnerability index for coastal cities and its use in assessing climate change impacts. (Natural Hazards), 64(1), 73–105. doi: 10.1007/s11069-012-0234-1
Bhowmik M., Mandal S. (2021). Do seasonal dynamics influence traits and composition of macrobenthic assemblages of sundarbans estuarine system, India? Oceanologia 63 (1), 80–98. doi: 10.1016/j.oceano.2020.10.002
Biggs C. R., Yeager L. A., Bolser D. G., Bonsell C., Dichiera A. M., Hou Z., et al. (2020). Does functional redundancy affect ecological stability and resilience? a review and meta-analysis. Ecosphere 11 (7), e03184. doi: 10.1002/ecs2.3184
Boström C., Törnroos A., Bonsdorff E. (2010). Invertebrate dispersal and habitat heterogeneity: expression of biological traits in a seagrass landscape. J. Exp. Mar. Biol. Ecol. 390 (2), 106–117. doi: 10.1016/j.jembe.2010.05.008
Bremner J., Rogers S. I., Frid C. L. J. (2006). Matching biological traits to environmental conditions in marine benthic ecosystems. J. Mar. Syst. 60, 302–316. doi: 10.1016/j.jmarsys.2006.02.004
Chevene F., Doleadec S., Chessel D. (1994). A fuzzy coding approach for the analysis of long-term ecological data. Freshw. Biol. 31 (3), 295–309. doi: 10.1111/j.1365-2427.1994.tb01742.x
Degen R., Aune M., Bluhm B. A., Cassidy C., Kędra M., Kraan C., et al. (2018). Trait-based approaches in rapidly changing ecosystems: A roadmap to the future polar oceans. Ecol. Indic. 91 (1), 722–736. doi: 10.1016/j.ecolind.2018.04.050
Delfan N., Ghodrati M., Naderloo R. (2021). Patterns of structural and functional diversity of macrofaunal communities in a subtropical mangrove ecosystem. Estuarine Coast. Shelf Sci. 252, 107288. doi: 10.1016/j.ecss.2021.107288
Donadi S., Klemens B., Alexander K., Dorothee L. (2015). The body-size structure of macrobenthos changes predictably along gradients of hydrodynamic stress and organic enrichment. Mar. Biol. 162, 675–685. doi: 10.1007/s00227-015-2614-z
Gamito S., Patrício J., Neto J. M., Teixeira H., Carlos J. (2012). Feeding diversity index as complementary information in the assessment of ecological quality status. Ecol. Indic. 19, 73–78. doi: 10.1016/j.ecolind.2011.08.003
Griffiths J. R., Kadin M., Nascimento F. J. A., Tamelander T., Törnroos A., Bonaglia S., et al. (2017). The importance of benthic–pelagic coupling for marine ecosystem functioning in a changing world. Global Change Biol. 23 (6), 2179–2196. doi: 10.1111/gcb.13642
IMD (2017) India Meteorological department, cyclone warning division, government of India new Delhi. Available at: https://rsmcnewdelhi.imd.gov.in/uploads/report/26/26_af079d_vardah.pdf.
Jumars P. A., Dorgan K. M., Lindsay S. M. (2016). Diet of worms emended: An update of polychaete feeding guilds diet of worms emended: An update of polychaete feeding guilds. Annu. Rev. Mar. Sci. 7, 497–520. doi: 10.1146/annurev-marine-010814-020007
Karlson A. M. L., Nascimento F. J. A., Näslund J., Elmgren R. (2010). Higher diversity of deposit-feeding macrofauna enhances phytodetritus processing. Ecology 91 (5), 1414–1423. doi: 10.1890/09-0660.1
Laliberté E., Legendre P., Shipley B. (2014). FD: measuring functional diversity from multiple traits, and other tools for functional ecology. r package version 1.0-12. Ecology 91, 299–305.
Leung J. Y. S., Cheung N. K. M. (2017). Can mangrove plantations enhance the functional diversity of macrobenthic community in polluted mangroves? Mar. pollut. Bull., 116 (1–2), 454–461. doi: 10.1016/j.marpolbul.2017.01.043
Levin L. A. (1984). Life history and dispersal patterns in a dense infaunal polychaete assemblage: Community structure and response to disturbance ecology, vol. 65 No. 4 (Aug. 1984) pp, 1185–1200.
Linden P., Patrício J., Marchini A., Cid N., Magalhães J., Carlos J. (2012). A biological trait approach to assess the functional composition of subtidal benthic communities in an estuarine ecosystem. Ecol. Indic. 20, 121–133. doi: 10.1016/j.ecolind.2012.02.004
Lomovasky B. J., Firstater F. N., Gamarra A., Mendo J., Iribarne O. O. (2011). Macro benthic community assemblage before and after the 2007 tsunami and earthquake at paracas bay, Peru. J. Sea Res. 65 (2), 205–212. doi: 10.1016/j.seares.2010.10.002
Lu L., Wu R. S. S. (2000). An experimental study on recolonization and succession of marine macrobenthos in defaunated sediment. Mar. Biol. 136 (2), 291–302. doi: 10.1007/s002270050687
Mason N. W. H., Mouillot D., Lee W. G., Wilson J. B. (2005). Functional richness, functional evenness and functional divergence: The primary components of functional diversity. Oikos 111 (1), 112–118. doi: 10.1111/j.0030-1299.2005.13886.x
Micheli F., Halpern B. S. (2005). Low functional redundancy in coastal marine assemblages. Ecol. Lett. 8 (4), 391–400. doi: 10.1111/j.1461-0248.2005.00731.x
Mouillot D., Graham N. A. J., Villéger S., Mason N. W. H., Bellwood D. R. (2013). A functional approach reveals community responses to disturbances. Trends Ecol. Evol. 28 (3), 167–177. doi: 10.1016/j.tree.2012.10.004
Nosad S., Ganesh T., Kiran L. R. (2021). Efficiency of sampling gears (Quadrate and core) and taxonomic resolution on the soft bottom intertidal macrobenthic community of port Blair coast. Res. J. Chem. Environ. 25 (11), 93–103. doi: 10.25303/2511rjce93103
Pandey V., Thiruchitrambalam G. (2019). Spatial and temporal variability of sandy intertidal macrobenthic communities and their relationship with environmental factors in a tropical island. Estuarine Coast. Shelf Sci. 224, 73–83. doi: 10.1016/j.ecss.2019.04.045
Peng S., Zhou R., Qin X., Shi H., Ding D. (2013). Application of macrobenthos functional groups to estimate the ecosystem health in a semi-enclosed bay. Mar. pollut. Bull. 74 (1), 302–310. doi: 10.1016/J.MARPOLBUL.2013.06.037
Petchey O. L., Gaston K. J. (2002). Functional diversity (FD), species richness and community composition. Ecol. Lett. 5 (3), 402–411. doi: 10.1046/j.1461-0248.2002.00339.x
Posey M., Lindberg W., Alphin T., Vose F. (1996). Influence of storm disturbance on an offshore benthic community. Bull. Mar. Science. 59 (3), 523–529.
Queirós A. M., Birchenough S. N. R., Bremner J., Godbold J. A., Parker R. E., Romero- Ramirez A., et al. (2013). A bioturbation classification of European marine infaunal invertebrates. Ecol. Evol. 3 (11), 3958–3985. doi: 10.1002/ece3.769
Rao C. R. (1982). Diversity and dissimilarity coefficients: A unified approach. Theor. Population Biol. 21 (1), 24–43. doi: 10.1016/0040-5809(82)90004-1
Reizopoulou S., Nicolaidou A. (2007). Index of size distribution (ISD): A method of quality assessment for coastal lagoons. Hydrobiologia 577 (1), 141–149. doi: 10.1007/s10750-006-0423-6
Ríos-Jara E., Navarro-Caravantes Ceciel-M., Galván-Villa Cristian-M., Lopez-Uriarte Ernesto, (2009). Bivalves and gastropods of the gulf of tehuantepec, mexico: A checklist of species with notes on their habitat and local distribution. J. Mar. Sci. vol. 2009, Article ID 176801, 12. doi: 10.1155/2009/176801
Sivadas S. K., Gupta G. V. M., Kumar S., Ingole B. S. (2021). Trait-based and taxonomic macrofauna community patterns in the upwelling ecosystem of the southeastern Arabian sea. Mar. Environ. Res. 170, 105431. doi: 10.1016/j.marenvres.2021.105431
Sugumaran J., Padmasai R., Altaff K. (2019). The effects of tropical cyclone gaja on sandy beach meiofauna of palk. Region. Stud. Mar. Sci. 31, 100747. doi: 10.1016/j.rsma.2019.100747
Sukumaran S., Vijapure T., Kubal P., Mulik J., Rokade M. A., Salvi S., et al. (2016). Polychaete community of a marine protected area along the West coast of India-prior and post the tropical cyclone, phyan. PLoS One 11 (8), 1–18. doi: 10.1371/journal.pone.0159368
Vasudha M. (2017). Observation of cyclone occurred in Arabian Sea and bay of Bengal from SAPHIR sensor data. Int. J. Appl. Eng. Res. 12 (22), 12821–12832.
Vijapure T., Sukumaran S., Neetu S., Chandel K. (2019). Macrobenthos at marine hotspots along the northwest Indian inner shelf: Patterns and drivers. Mar. Environ. Res. 144(August 2018), 111–124. doi: 10.1016/j.marenvres.2018.12.007
Villéger S., Mason N. W. H., Mouillot D. (2008). New multidimensional functional diversity indices for a multifaceted framework in functional ecology. Ecology 89 (8), 2290–2301. doi: 10.1890/07-1206.1
Keywords: biological trait analysis, cyclone effects, macrobenthic fauna, ecosystem functioning, functional redundancy
Citation: Sahu N, Lakra RK and Thiruchitrambalam G (2022) Effect of cyclonic storm Vardah on the community structure and ecosystem functioning of macrobenthic fauna in the intertidal region of South Andaman Islands. Front. Mar. Sci. 9:953985. doi: 10.3389/fmars.2022.953985
Received: 26 May 2022; Accepted: 27 June 2022;
Published: 28 July 2022.
Edited by:
Biraja Kumar Sahu, Council of Scientific and Industrial Research (CSIR), IndiaReviewed by:
Sadayan Paramasivam, Alagappa University, IndiaThadickal V. Joydas, King Fahd University of Petroleum and Minerals, India
Copyright © 2022 Sahu, Lakra and Thiruchitrambalam. This is an open-access article distributed under the terms of the Creative Commons Attribution License (CC BY). The use, distribution or reproduction in other forums is permitted, provided the original author(s) and the copyright owner(s) are credited and that the original publication in this journal is cited, in accordance with accepted academic practice. No use, distribution or reproduction is permitted which does not comply with these terms.
*Correspondence: Ganesh Thiruchitrambalam, Z2FuZXNodC5vbWJAcG9uZGl1bmkuZWR1Lmlu