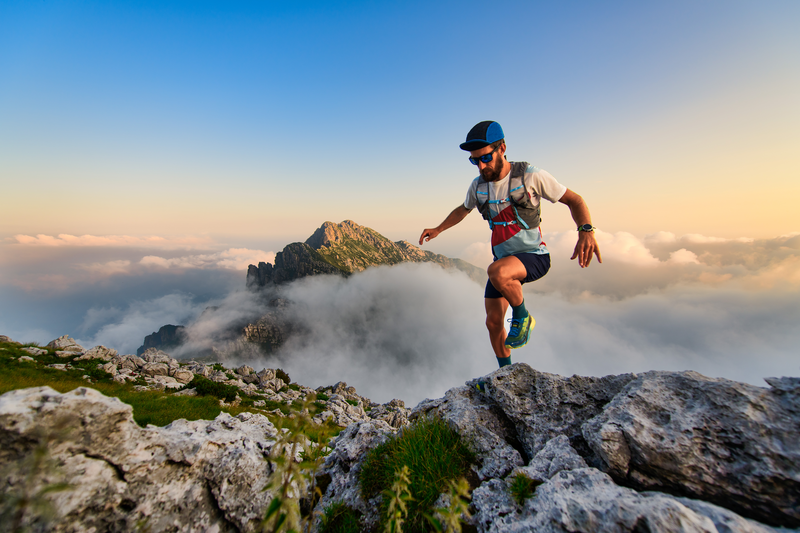
94% of researchers rate our articles as excellent or good
Learn more about the work of our research integrity team to safeguard the quality of each article we publish.
Find out more
ORIGINAL RESEARCH article
Front. Mar. Sci. , 15 September 2022
Sec. Marine Biology
Volume 9 - 2022 | https://doi.org/10.3389/fmars.2022.950880
This article is part of the Research Topic Comprehensive Utilization and Evaluation of Low-Value Marine Organisms and Seafood Processing Waste/By-products View all 5 articles
The rate of breast cancer is rapidly increasing and discovering medications with therapeutic effects play a significant role in women’s health. Drugs derived from marine sponges have recently received FDA approval for the treatment of malignant tumors, including metastatic breast cancer. Spirastrella pachyspira (marine sponge) is mainly obtained from the western coastal region of India, and its anticancer potential has not been explored. Hence, the present study aimed to evaluate the anticancer potential of Spirastrella pachyspira extracts and its bioactive molecule sphingosine. The extracts were prepared using hexane, chloroform, ethyl acetate, and ethanol. The cytotoxic potential of the extracts were determined by an in-vitro MTT assay using SK-BR-3 cancer cell line. Subsequently, acute toxicity investigation was conducted in Swiss albino mice. Then, the anticancer effects of the extract was investigated in a xenograft model of SK-BR-3 caused breast cancer. DAPI staining was used to assess the extract’s ability to induce apoptosis. In addition, in-silico study was conducted on sphingosine with extracellular site of HER2. The ethyl acetate extract of Spirastrella pachyspira (IC50: 0.04 µg/ml) showed comparable anticancer effects with standard doxorubicin (IC50: 0.054 µg/ml). The LD50 of the extracts in acute toxicity testing was fund to be 2000 mg/kg b.wt. The survival index of mice in ethanol extract was 83.33%, whereas that of standard doxirubicin was 100%, indicating that ethyl acetate extract Spirastrella pachyspira has good antiproliferative/cytotoxic properties. The results were well comparable with standard doxorubicin. Further, the docking studies of sphingosine against HER2 demonstrated that the bioactive molecule engage with the extracellular region of HER2 and block the protein as also shown by standard trastuzumab. The findings of this research suggest that Spirastrella pachyspira and sphingosine may be potential candidate for the treatments of breast cancer, particularly for HER2 positive cells. Overall, the present results demonstrate that sphingosine looks like a promising molecule for the development of new drugs for the treatment of cancer. However, in order to carefully define the sphingosine risk-benefit ratio, future research should focus on evaluating in-vivo and clinical anticancer studies. This will involve balancing both their broad-spectrum effectiveness and their toxicity.
Breast cancer is the most common type of cancer worldwide and one of the leading causes of deaths in women (Yap et al., 2021). Breast cancer can be divided into various biological subgroups based on immunohistochemistry and gene expression analysis. Using a gene expression profile-based classification system, tumors are primarily divided into five major molecular subtypes: 1) the estrogen receptor (ER)-positive, HER2-negative, progesterone receptor (PR)-positive (luminal A); 2) the estrogen receptor (ER)-positive, HER2-negative, PR-negative (luminal B); 3) the human epidermal growth factor receptor 2 (HER2)-enriched with ER-negative, HER2-positive, PR-negative; 4) normal-like breast cancer or 5) the basal-like or triple-negative or TNBCs presented with ER-negative, PR-negative and HER2-negative which considered the most aggressive than others (Schnitt, 2010; Eliyatkın et al., 2015). There is also genetic profiling on the other molecular subtypes, such as luminal B-like breast cancer (ER-positive, HER2-positive, PR-negative or PR-positive) and luminal C however it is less well defined (Jaaskelainen et al., 2020). Clinical oncology and the scientific community have shown considerable interest in breast cancer subtypes that overexpress HER2, which has led to the investigation of first-generation anti-HER2 agents such as trastuzumab (Eiger et al., 2021). Trastuzumab, an approved drug, is a monoclonal antibody that binds to the HER2 receptor directly to treat this kind of breast cancer. Despite trastuzumab’s efficacy, the fundamental problem with the therapy continues to be the occurrence of both innate and acquired resistance. Therefore, it’s essential to research alternate second-line therapies. When HER ligands bind to the extracellular region of the receptor, the HER family is homo- or heterodimerized, and downstream signaling pathways like the PI3K/Akt pathway and the mitogen-activated protein kinase (MAPK) pathway, which is linked to cell proliferation, are activated (associated with cell survival). Deregulation of these pathways as a result of abnormal expression or activation of HER-family members can result in cancer’s hallmarks of increased proliferation, decreased apoptosis, angiogenesis, and invasiveness. As a result, members of the HER family have gained popularity as therapeutic targets (Roskoski, 2014; Stanley et al., 2017). In addition to cell lines such as SUM190PT, HCC202, SUM225CWN, and UACC893, SK-BR3 cell lines are widely recognized as ER-negative HER2 breast cancer models as well (Dai et al., 2017).
Nature has revealed its source of novel anticancer drugs over the past twenty-five years with an increasing number of new compounds sourced from the marine environment. The chemical and biological diversities of the marine environment are immeasurable, and form an extraordinary resource for the discovery of new anticancer drugs. Despite the technological challenges in characterizing and scaling up production of bioactive compounds several anticancer compounds have entered preclinical and/clinical trials (Kalimuthu and Se Kwon, 2013). To date, marine organisms specifically sponges, sponge microbe symbiotic association, gorgonian, actinomycetes and soft coral have been explored for potential anticancer agents. Among them, marine sponges, Phylum porifera are the most primitive of the multicellullar organisms comprising approximately 15,000 sponge species. Marine sponges are considered to be the simplest and oldest living multicellular organisms existing for more than 600 million years. Sponges are primarily filter feeders since most rely on the water that constantly circulates through their porous bodies from which they filter out microorganisms and organic particles as their food source. Moreover, they produce a plethora of secondary metabolites to repel and deter predators and to compete for space with other sessile marine organisms (Essack et al., 2011). Secondary metabolites found in marine sponges have intriguing chemopreventive and chemotherapeutic effects on tumors. The anticancer drug vidarabine was initially isolated in the 1950s by Bergmann and Feeney from a peculiar nucleoside found in the sponge Tethya crypta (Sagar et al., 2010). Numerous nucleosides, sterols, alkaloids, peroxides, terpenes, fatty acids, derivatives of amino acids, and cyclic peptides have been identified as active substances thus far, and at least around 50 of them have the potential to be chemopreventive and/or anticancer agents. In this regard, marine sponge-derived bioactive metabolites continue to be one of the most promising sources of new drug leads as investigated in this study.
Spirastrella pachyspira, a marine sponge belonging to the family Spirastrellidae and genus Spirastrella is widely distributed in red sea and Indian Ocean and usually grows on the corals at 4 to 8 feet deep. When alive, the color is violet usually, though pink is noted in some parts and the interior is pale yellow in color. The modified spirasters form a sort of plate by the interlocking of their spines and this plate is distinctly seen in boiled out preparations. The tylostyles, deeply buried in the tissue emerges in groups from the upper part of the plates. These spicules are plumosely arranged. In some places, the tylostyles emerging from the adjacent brushes converge and usually such an arrangement is noted in surface ridges (Kumar and Shah, 2014). It was reported that it has broad spectrum antimicrobial properties (Mohan Kumar and selvi, 2012; Kumar and Jogan, 2014). Various bioactive compounds such as spongistatins 4 and 5 (Pettit et al., 1993), lyso-Platelet activating factors (Alam et al., 2001), sphingosine sulfates (Alam et al., 2002), spirastrelloide A (Williams et al., 2003), chlorine and bromine of helinane (Martin et al., 2005), mollenyne A (Morinaka and Molinski, 2011), collagen and lysophosphatidyl glycerol (Jang et al., 2012) were isolated from various sponges of the genus Spirastrella. However, Spirastrella pachyspira has not been investigated to identify its bioactive compounds and to evaluate any other biological properties apart from antimicrobial according to the literature and our knowledge. In contrast, numerous other Spirastrella species have had their bioactive compounds identified and their potential anticancer properties investigated (Pettit et al., 1993; Alam et al., 2001; Alam et al., 2002; Williams et al., 2003; Martin et al., 2005; Morinaka and Molinski, 2011; Jang et al., 2012; Mohan Kumar and selvi, 2012; Kumar and Jogan, 2014). Additionally, according to one of our earlier laboratory findings, sphingosine is a major bioactive compound of Spirastrella pachyspira, and sphingosine sulfates are isolated previously from Spirastrella abata (Jang et al., 2012). Sphingosine sulfate demonstrated a significant anticancer activity that was even more potent than standard cisplatin and doxorubicin against human solid tumor cell lines (Alam et al., 2002; Jang et al., 2012). So, in addition to its antimicrobial properties, we anticipated that Spirastrella pachyspira and its bioactive compound sphingosine would also have potential anticancer activity against HER2-positive cancer cells (Figure 1). Therefore, the objective of the current study was to investigate in-vitro and in-vivo anticancer activity of Spirastrella pachyspira and to conduct in-silico molecular docking approach on sphingosine.
Figure 1 Schematic representation of the impact of anticancer effect of Spirastrella pachyspira against HER2-positive cancer cells along with its antimicrobial effect.
Spirastrella pachyspira (marine sponge) was collected from the coastal around Mandapam in the Palk Bay zone, Ramanathapuram, Tamilnadu, INDIA, which were then cleaned to remove contaminants and kept at 4°C until further use. The sponge was then authenticated by the registered scientist (Dr. G. Sivaleela, Scientist-B, Zoological survey of India, Chennai, INDIA) and the voucher specimen (registration number S-261) was deposited in Marine Biology Regional Centre/Zoological Survey of India, INDIA. Prior to experimentation, the sponges were thawed at room temperature and were cut into small pieces before being soaked in solvent of increasing polarities like n-hexane, chloroform, ethyl acetate and methanol. For each solvent, the successive extraction was carried out using the cold-maceration method for 72 hours and then filtered. Each extract was then concentrated using a rotary evaporator under reduced pressure and a controlled temperature. Until further use, the dried extracts were stored at 4°C.
SK-BR-3 cells were obtained from the National Centre for Cell Science (NCCS), Pune, INDIA. The cells were maintained in Dulbecco’s Modified Eagle Medium (DMEM) and supplemented with 10% Fetal Bovine Serum (FBS), with penicillin (100 units/ml) and streptomycin (100 μg/ml). The cells were cultured in 75 cm2 culture flask and were incubated at humidified atmosphere with 5% carbon dioxide (CO2) at 37°C. Cell respiration as an indicator of cell viability and proliferation was determined using a mitochondrial dependent reduction of 3-(4,5-dimethyl thiazol-2-yl)-2,5-diphenyl tetrazolium bromide (MTT) to formazan. SK-BR-3 cells were seeded in 96-well plates at a density of 8,000 cells/200 μl/well. Cells were treated with different concentrations of all the four extracts of Spirastrella pachyspira (ranging between 1X100 and 1X107 ng) after 48 hours following plating. They were incubated at 37°C for 48 hours. At the 44th hour following drug exposure, the cells were incubated at 37°C with 0.5 mg/ml MTT for four hours. At the end of the experiment, the medium was removed and the insoluble formazan product was dissolved in 200 μl of dimethyl sulfoxide (DMSO) and kept it for 15 minutes in dark. The intensity of purple blue developed was measured at 570 and 630 nm using a UV-Visible spectrophotometer. Finally, the percentage growth inhibitory rate of the extracts was calculated using the following formula (Mosmann, 1983)
SK-BR-3 cells were seeded in 6 well plates at a density of 1X105cells/well and were allowed to grow for a period of 24 hours. The ethyl acetate extract was administered at two different concentrations (10 and 30 µg/ml) as follows.
Group 1 [SK-BR-3 cells + Vehicle]
Group 2 [SK-BR-3 cells + Ethyl acetate extract of Spirastrella pachyspira (10 µg/ml)]
Group 3 [SK-BR-3 cells + Ethyl acetate extract of Spirastrella pachyspiras (30 µg/ml)]
At the end of incubation, cell morphology was monitored under an inverted light microscope. The cells were fixed with 4% paraformaldehyde for 20 minutes, washed with phosphate buffered saline (PBS) and incubated in DAPI (1 µg/ml) for 10 minutes. After washing with PBS, the cells were observed using fluorescent microscope with a peak excitation wavelength of 340 nm (Chang et al., 2006).
Acute oral toxicity study was performed according to the OECD test guideline 423: Acute toxic class method (OECD, 2002). Briefly, young healthy adult female swiss albino mice (n = 6) weighing between 18-22 g b.wt. were divided into two groups of three animals in each group. The animals were individually housed in a well-ventilated polypropylene cage. A 12-hours light/12-hours dark artificial photoperiod was maintained. Room temperature 22°C (± 3°C) and relative humidity 40-65% were maintained in the room. The animals had free access to pelleted feed (M/s. Provimi Animal Nutrition Pvt Ltd, Bengaluru, INDIA) and reverse osmosis (Rios, USA) purified water ad libitum. The animals were acclimatized for seven days to the laboratory conditions. Guidelines of “guide for the care and use of laboratory animals” (Institute of Laboratory Animal Resources, National Academic Press 1996; NIH publication number #85-23, revised 1996) were strictly followed throughout the study. Institutional animal ethics committee (IAEC), Sri Ramachandra University, Chennai, INDIA, approved the study (IAEC/XLIII/SRU/422/15). The experiment was conducted in a stepwise manner (Steps I & II). The extract was administered once orally via gastric intubation at 2000 mg/kg b.wt.
Group I (Step-I): Ethyl acetate extract of Spirastrella pachyspira (2000 mg/kg b.wt.)
Group II (Step-II): Ethyl acetate extract of Spirastrella pachyspira (2000 mg/kg b.wt.)
The lethality and abnormal clinical signs were observed on the dosing day at 30 minutes, 1st hour, 2nd hour and 4th hour and thereafter (for 13 days). The body weight was recorded before dosing and thereafter once in a week until the completion of the experiment. Gross pathological changes were also observed at the end of experiment.
Female athymic mice (18-22 g b.wt.) obtained from Vivo-Biotech, INDIA was used for the study. The same process outlined in section 4.4 was used for animal maintenance. Following acclimatization, the animals were divided into four groups of six animals each.
Group I: SK-BR-3-induced + Vehicle
Group II: SK-BR-3-induced + Doxorubicin (1 mg/kg b.wt., i.p.)
Group III: SK-BR-3-induced + Ethyl acetate extract of Spirastrella pachyspira (100 mg/kg b.wt., p.o)
Group IV: SK-BR-3-induced + Ethyl acetate extract of Spirastrella pachyspira (200 mg/kg b.wt., p.o)
SK-BR-3 cells 5 X 106 were mixed with matrigel in the ratio 1:1 before subcutaneous injection into both sides of the animals’ ventral flank region using a 26-gauge needle. Subsequently, the growth of the xenograft was observed for a period of 14 days. Once the tumor reaches 100 mm3 (day 0), the ethyl acetate extract and the reference drug doxorubicin (1 mg/kg body weight) were administered to the respective groups for 15 days while the tumor volume was measured once in every three days. The tumor size was measured with calipers and the growth rates were recorded and calculated using the following equation: Tumor volume (V; mm3) =L*B*W where L = tumor length, B = tumor breadth, W = tumor width. The survival rate was also recorded. At the end of the experiment, the animals were sacrificed by cervical dislocation. The tumor tissue was collected, fixed in buffered formaldehyde and was cut into sections for further histopathological study (Holiday and Speirs, 2011).
Sphingosine, a bioactive molecule from the sponge, was retrieved from the pubchem database and was prepared using the ligprep tool. Based on the penalty scores, the best prepared ligand was picked. The RCSB repository was used to obtain the HER2 protein (1N8Z), and the missing chains, atoms, as well as hydrogen were added. The protein was then optimized and minimized for docking (Cho et al., 2003). The receptor grid was mapped and evaluated using the SIE predictor tool. Molecular docking was performed with a standard precision against the active site of the HER2 protein, with a maximum of 20 poses per ligand.
All data obtained from the experiments were expressed as mean ± SEM. Statistical analysis was performed by a one-way ANOVA, followed by Tukey’s test using GraphPad Prism 5.0. A p-value of <0.05 was considered as statistically significant.
Spirastrella pachyspira extracts were evaluated for their in-vitro cytotoxic activity against SK-BR-3 cell line (a HER2 positive cancer cell) in 48 hours assay where the cells were exposed to different concentrations of all four extracts. The extracts were investigated in quadruplicate at each concentration and the cumulative variation were maintained at less than 20% between the data points. Finally, it was inferred from the graph that the appearance of cytotoxic changes following exposure to the extract was in a concentration-dependent manner. It is important to note that at higher concentrations, there was a significant reduction in cell density. The American National Cancer Institute guidelines (NCI) set the limit of activity for crude extracts at 50% inhibition (IC50) of proliferation of less than 30 mg/ml following a 24 hours exposure (Ren et al., 2014). The IC50 values were below the stated point for all four extracts, with the lowest (0.04 µg/ml) seen in the ethyl acetate extract followed by 1.29 µg/ml (methanolic extract), 1.88 µg/ml (chloroform extract) and 2.51 µg/ml (hexane extract) (Figure 2). In fact, the ethyl acetate extract exhibited well comparable cytotoxicity as that of standard doxorubicin (0.054 µg/ml). In the present study, the cytotoxic effect of Spirastrella pachyspira extracts were in a concentration-dependent manner. The IC50 of the four extracts was in the range of 0.04-2.50 µg/ml with the ethyl acetate extract exhibiting an IC50 value of 0.04 µg/ml. Therefore, the ethyl acetate extract of Spirastrella pachyspira was selected for further investigations in this research.
Apoptosis is a common mode of action of chemotherapeutic agents including in products derived from natural sources. The apoptosis inducing potential of ethyl acetate extract of Spirastrella pachyspira was determined using a DAPI staining. After treatment with the extract, the increase in the apoptotic cells including the characteristics of apoptotic cells and evident as seen in the DNA fragmentations which form important hallmarks of apoptosis. On the other hand, the control group showed no increase of apoptotic cells with no evidence of DNA fragments. This phenomenon is indicative of ethyl acetate extract specifically inducing apoptosis of cancer cells (Figure 3).
Figure 3 Apoptotic morphological evaluation of SK-BR-3 cells by DAPI staining (inverted fluorescence microscopy, 200x). (A) Control group, Healthy SK-BR-3 cancerous cells without blue fluorescence, (B) Treatment with ethyl acetate extract of Spirastrella pachyspira (10 μg/ml) and (C) Treatment with ethyl acetate extract of Spirastrella pachyspira (30 μg/ml), showed condensed and fragmented apoptotic bodies with blue fluorescence and nuclear or chromatin condensation.
The tabulated results indicates that there were no treatment related deaths, abnormal clinical signs or remarkable body weight changes (Tables 1, 2) observed. There was also no gross pathological changes seen in all animals. Based on the above results, the LD50 of the investigated drug was higher than 2000 mg/kg b.wt. indicating that the test drug is in the “category-5 or unclassified” in accordance to the globally harmonized system of classification of chemicals.
The survival index of the animals was 66.67% indicating that the decrease in the survival index seen is contributed by the tumor burden of the mice. In comparison, the index was 83.33% in treated mice when compared to the standard doxorubicin (100%) which revealed a decrease in the tumor burden of the treated mice (Table 3). To date, literature review indicated that the in-vivo antitumorigenic study of ethyl acetate extract of Spirastrella pachyspira has not been previously reported. In the present study the ethyl acetate extract suppressed HER2 positive tumor. At baseline, all four groups of athymic mice had a tumor volume in the range of 100-110 mm3. The representative images on the final day of treatment indicated the presence of a visible tumor in the control mice (tumor volume of 90.88 mm3). In comparison, visually the tumor had almost disappeared in doxorubicin-treated mice with a negligent tumor volume of 14.66 mm3. In the case of ethyl acetate-treated mice the tumor volumes were also lower (29.16 mm3 for 100 mg/kg b.w. and 22.30 mm3 for 200 mg/kg b.w.). On the 15th day of treatment, measurement of tumor volume at both doses of Spirastrella pachyspira ethyl acetate extract showed a significant reduction in tumor volume with a p<0.01 when compared to the control (Table 4 and Figure 4). Overall, the tumor reduction substantiated the antiproliferative/cytotoxic property of ethyl acetate extract of Spirastrella pachyspira with an IC50 value of 0.04 µg/ml.
Table 3 The effect of ethyl acetate extract of Spirastrella pachyspira on the survival index of athymic mice bearing s.c. SK-BR-3 tumor.
Table 4 The effect of ethyl acetate extract of Spirastrella pachyspira on tumor volume of athymic mice bearing s.c. SK-BR-3 tumor.
Figure 4 The effect of ethyl acetate extract of Spirastrella pachyspira on tumor volume of athymic mice bearing s.c. SK-BR-3 tumor.
Hematoxylin & Eosin (H & E) staining shows that tumors from untreated mice have fibrous stromal proliferation and infiltrating tumor cells that are crowded, enlarged, pleomorphic and with hyperchromatic nuclei (Figure 5). In doxorubicin-treated mice, the tumors have almost disappeared. Since the change in tumor size was very obvious, only the skin of the athymic mice was used for the H & E staining. The H & E staining of doxorubicin-treated mice indicated that the skin had normal epidermis, dermis, hair follicle, sebaceous gland, adipose tissues, connective tissues and muscles. However, the H & E staining of the mice treated with the ethyl acetate extract (100 mg/kg) revealed tumor cell nested in a circumscribed and nodular pattern within the breast stroma. The H & E staining of mice treated with a higher concentration of the ethyl acetate extract (200 mg/kg) indicated negligible tumor size and revealed skin with normal epidermis, dermis, hair follicle, sebaceous gland, adipose tissues, connective tissues and muscles indicating the potential anticancer activity of the extract.
Figure 5 Representative images of the control, ethyl acetate extract, doxorubicin-treated tumor and its histopathology findings in athymic mice. (A) Control mice with vehicle, (B) Control mice tumor (H & E, 400 X), (C) Doxorubicin-treated (1 mg/kg), (D) Doxorubicin-treated skin (H & E, 400X), (E) Ethyl acetate extract-treated (100 mg/kg b.wt.), (F) Ethyl acetate extract of Spirastrella pachyspira-treated (100 mg/kg b.wt.) (H & E, 400 X), (G) Ethyl acetate extract of Spirastrella pachyspira-treated (200 mg/kg b.wt.), (H) Ethyl acetate extract of Spirastrella pachyspira-treated (200 mg/kg b.wt.) (H & E, 400 X).
Sphingosine, a bioactive molecule, was selected to dock with the HER2 extracellular area. Since the binding site is only found in domain IV, the search was limited to the particular said domain. A binding site prediction tool was used to predict the binding site followed by evaluation of the maximum number of sites. The ligands were docked against the extracellular area with typical accuracy. Subsequently, the docked postures were examined. It was discovered that sphingosine interacted with ASP570, PRO571, and CYS601, i.e. the domain IV’s second and third loops with a docking score of -2.036 Kcal/mol. Additionally, Several orientations were also discovered to interact with the residues ASP560, GLU558 and PRO571. Since the second and third loops were demonstrated to generate conformational changes for inhibition, comparable sphingosine interactions were investigated. The binding position of sphingosine was compared to that of the standard drug trastuzumab used in the treatment of HER2 inhibition (Figure 6).
Figure 6 Binding site similarity of sphingosine and trastuzumab (A) interaction of sphingosine with extracellular site of HER2 (B) Binding pattern of sphingosine with extracellular pocket of HER2 (C) binding pattern of trastuzumab with extracellular pocket of HER2.
The leading cause of cancer death among women worldwide is advanced breast cancer. Recent advances in genomics-proteomics and drug delivery allow for a better understanding of the molecular pharmacology of cancer and have challenged researchers to design target-based treatments (Kumar and Adki, 2018; He et al., 2020). Nevertheless, the presence of the human epidermal growth factor receptor 2 (HER2) plays a role in detecting breast cancer tumors (Mitri et al., 2012). Elevated HER2 receptor is a key factor in HER2-positive breast cancer cell proliferation, viability, and invasion. The MAPK and PI3K/AKT pathways are two of the main signaling pathways that HER2 activates. HER2 has thus become a key focus for the therapy of breast cancer (Ren et al., 2014). HER2 is found abundantly in the plasma membrane of SKB-R3 cells, a well-known breast cancer cell line that overexpresses HER2 and is used in numerous in-vitro studies. Cancer cure and therapy is remain challenging despite huge investment made in research for novel discovery of anticancer drugs. The oncological field has looked at a variety of sources, including plants, animals, and minerals, in an effort to find new molecular medicines. Secondary metabolites found in abundance in marine sponges have intriguing chemopreventive and chemotherapeutic effects on tumors (Calcabrini et al., 2017).
There are few marine-derived drugs being used to treat cancer including cytarabine (Cytosar-U®) and trabectedin (Yodelis®), and more than 20 are undergoing phase II–III clinical trials (Jimenez et al., 2018). The present study with ethyl acetate extract of Spirastrella pachyspira affirmed the anticancer activity on SKB-R3 breast cancer cell line. The toxicity of ethyl acetate extract of Spirastrella pachyspira was found active against SKBR3, with cell viability decreasing with increasing drug concentration. These findings were consistent with those of other marine sponges reported in previous investigations (Malhão et al., 2019; Elissawy et al., 2021).
Since the permeability of the DAPI stain in the live cell is very limited, the control cells exhibit very little fluorescence, whereas in the apoptotic cells, the cell membrane was damaged allowing the DAPI stain to penetrate the cell easily to exhibit a bright fluorescence (Li et al., 2003; Ansil et al., 2014; Elissawy et al., 2021). The ethyl acetate extract of Spirastrella pachyspira treated cells showed apoptotic changes in a concentration-dependent manner like DNA fragmentation or nucleus/chromatin condensation. The DAPI assay of ethyl acetate extract of Spirastrella pachyspira is a confirmation of its apoptotic potential. The results are well comparable with previous studies carried out on natural products (Kapuscinski, 1995; Wang et al., 2018).
The findings from acute toxicity studies indicated that the LD50 value was greater than 2000 mg/kg falls in category -5 or “unclassified” in accordance to the Globally Harmonized System of Classification of Chemicals (Pratt, 2002). Further, the survival index (83.33%) and tumor volume on the 15th day in a xenograft model in athymic mice is a clear indication that the ethyl acetate extract of Spirastrella pachyspira has been potential candidate as an anticancer agent. The findings above were very comparable to existing literature (Meyer et al., 2015; Puchalapalli et al., 2016; Ruiz-Torres et al., 2017)
Additionally, in our earlier laboratory research, we have isolated and characterized sphingosine, a key bioactive compound of the ethyl acetate extract of Spirastrella pachyspira. According to earlier studies, sponge-derived phytosphingosine sulfates exhibited a range of biological properties. In particular, they showed significant cytotoxicity against a number of cancer cell lines that was on broadly well comparable with standard doxorubicin and cisplatin (Alam et al., 2002; Jang et al., 2012). In the current study, we postulated that inhibiting HER2 positive breast cancers may be achieved by targeting the HER2 protein using sphingosine. As approach is based on the pharmacophore properties provided by the known inhibitors, 3D-QSAR pharmacophore-based drug discovery has grown in popularity for identifying potential novel lead candidates. One monoclonal antibody, trastuzumab, is used as first-line therapy for HER2-overexpressed breast cancer. However, trastuzumab administration has some side effects and may result in cancer resistance (Ratnasari et al., 2018). Sphingosine demonstrated a potent HER2 inhibitory activity based on docking energies, and the results are highly comparable to standard trastuzumab and the existing study findings (Satyanarayanajois et al., 2009; Arannilewa et al., 2018). Nonetheless, several potential therapeutic ideas derived from sponges have stagnated in clinical trials due to instability, toxicity, less potency or pharmacokinetics issues (Ramanjooloo et al., 2021; Varijakzhan et al., 2021). In addition to the significant effect of this bioactive compound, further research into exploiting it into nanocarriers has been envisioned and initiated (Ramanjooloo et al., 2021). Nanoencapsulation or conjugation of bioactive substances in carriers such as liposomes may increase a number of properties, including targeted delivery to the active site, solubility, stability, and protection against degradation, hence boosting bioavailability. Examples based on prior research include the utilization of sponge Haliclona sp. spicules (SHS) and liposomes on a skin model (Zhang et al., 2019; Liang et al., 2020), water-insoluble chemicals, avarol generated from the marine sponge Dysidea avara (Nakarada et al., 2020), and others with notable results. The capacity of various diseases to attack different parts of body locations can increase the likelihood that specific treatments will be developed in the near future (Anjum et al., 2016). Overall, sphingosine appears as a potential candidates for further in-vitro and in-vivo studies for the evaluation of anticancer activity.
As far as we are aware, this is the first investigation into the anticancer properties of Spirastrella pachyspira and its main active molecule, sphingosine, in response to breast cancer. The results of the current study proved that ethyl acetate extract of Spirastrella pachyspira showed potential cytotoxic effect against the SK-BR3 breast cancer cell line. Our findings also showed that ethyl acetate extract of Spirastrella pachyspira had anti-cancer properties on SK-BR3 cells that are overexpressing the HER2 gene. The major molecule sphingosine has demonstrated through molecular docking that it may be a promising drug-like candidate for the treatment of breast cancer. In terms of binding energy, sphingosine demonstrated high affinity for HER2 in comparison to the standard drug Trastuzumab, indicating that this molecule may have comparable or even greater therapeutic efficacy to Trastuzumab. Overall, we believe that Spirastrella pachyspira (marine sponge) can be essentially studied further for the development of potent anti-breast cancer medicines, and to prove its activity is mainly due to the presence of sphingosine. More detailed studies are needed to establish the proper role of Spirastrella pachyspira and its major bioactive molecule sphingosine for their therapeutic application and aid in the ongoing battle against breast cancer.
Recent scientific advances in the use of liposomes against breast cancer have established the feasibility of a variety of targeting strategies, including peptide-based strategies. As for future perspectives, we believe that the use of small molecules such as peptides will enable a greater penetration of bioactive moieties into the cell. In addition, the use of liposomes to enhance drug delivery to the targeted site while increasing bioavailability is also important. These strategies may address issues associated with natural products, such as toxicity and rapid clearance from the systemic circulation, while also advancing the clinical relevance of these drug delivery systems and improving their anticancer potentials (Figure 7).
Figure 7 Future applications of liposomes functionalized with heptapeptide-loaded Spirastrella pachyspira extracts against breast cancer cells. The heptapeptide LTVSPWY has a high affinity for human mammary adenocarcinoma SK-BR3 cells; thus, it can be used to deliver bioactive compounds from Spirastrella pachyspira’s extract to cells for internalization, which may result in significant apoptosis when the effects are combined together.
The datasets presented in this study can be found in online repositories. The names of the repository/repositories and accession number(s) can be found in the article/Supplementary Material.
The animal study was reviewed and approved by Institutional Animal Ethics Committee (IAEC), Sri Ramachandra University, IAEC/XLIII/SRU/422/2015. Written informed consent was obtained from the owners for the participation of their animals in this study.
Writing-original draft: SRM, CK, SJ, LSW, and MS; Conceptualization: SRM, CK, SJ, LSW, and MS; Supervision: SRM, CK, SJ, LSW, and MS; Resources: SRM, CK, SJ, LSW, MS, KC, SHG, MYB, NNIMR, VS, SF, NKF, SZS, KVS, SS, and VKS; Data curation: SRM, CK, SJ, LSW, MS, KC, SHG, MYB, NNIMR, VS, SF, NKF, SZS, KVS, SS, and VKS; Writing-review and editing: SRM, CK, SJ, LSW, MS, KC, SHG, MYB, NNIMR, VS, SF, NKF, SZS, KVS, SS, and VKS. All authors have read and agreed to the published version of the manuscript.
All the authors of this manuscript are thankful to their respective Departments/Universities for successful completion of this study. The authors extend their appreciation to the Deanship of Scientific Research at King Khalid University for supporting this research through Large Groups (RGP.2/52/1443). The figures in this manuscript were created with the support of https://biorender.com under a paid subscription (ref: C08A1A0B-0002; 3 November 2021).
The authors declare that the research was conducted in the absence of any commercial or financial relationships that could be construed as a potential conflict of interest.
All claims expressed in this article are solely those of the authors and do not necessarily represent those of their affiliated organizations, or those of the publisher, the editors and the reviewers. Any product that may be evaluated in this article, or claim that may be made by its manufacturer, is not guaranteed or endorsed by the publisher.
Alam N., Bae B., Hong J., Lee C., Shin B., Im K., et al. (2001). Additional bioactive lyso-PAF congeners from the sponge Spirastrella abata. J. Nat. Prod. 64 (4), 533–535. doi: 10.1021/np0005210
Alam N., Wang W., Hong J., Lee C. O., Im K. S., Jung J. H. (2002). Cytotoxic sphingosine 4-sulfates from the sponge Spirastrella abata. J. Nat. Prod. 65 (6), 944–945. doi: 10.1021/np010312v
Anjum K., Abbas S. Q., Shah S. A., Akhter N., Batool S., Hassan S. S. (2016). Marine sponges as a drug treasure. Biomol. Ther. 24, 347–362. doi: 10.4062/biomolther.2016.067
Ansil P., Wills P., Varun R., Latha M. (2014). Cytotoxic and apoptotic activities of Amorphophallus campanulatus tuber extracts against human hepatoma cell line. Res. Pharm. Sci. 9 (4), 269–277. Available at: https://www.ncbi.nlm.nih.gov/pmc/articles/PMC4314875/
Arannilewa A. J., Suleiman Alakanse O., Adesola A. O., Israel Malachi O., Michael Obaidu I., Oluwafemi E. E., et al. (2018). Molecular docking analysis of cianidanol fromGinkgo biloba with HER2+ breast cancer target. Bioinformation 14 (9), 482–487. doi: 10.6026/97320630014482
Calcabrini C., Catanzaro E., Bishayee A., Turrini E., Fimognari C. (2017). Marine sponge natural products with anticancer potential: An updated review. Mar. Drugs 15 (10), 310. doi: 10.3390/md15100310
Chang H. K., Shin M. S., Yang H. Y., Lee J. W., Kim Y. S., Lee M. H., et al. (2006). Amygdalin induces apoptosis through regulation of bax and bcl-2 expressions in human DU145 and LNCaP prostate cancer cells. Biol. Pharm. Bull. 29 (8), 1597–1602. doi: 10.1248/bpb.29.1597
Cho H. S., Mason K., Ramyar K. X., Stanley A. M., Gabelli S. B., Denney D. W., et al. (2003). Structure of the extracellular region of HER2 alone and in complex with the herceptin fab. Nature 421, 756–760. doi: 10.1038/nature01392
Dai X., Cheng H., Bai Z., Li J. (2017). Breast cancer cell line classification and its relevance with breast tumor subtyping. J. Cancer 8, 3131–3141. doi: 10.7150/jca.18457
Eiger D., Agostinetto E., Saude-Conde R., De Azambuja E. (2021). The exciting new field of HER2-low breast cancer treatment. Cancers (Basel) 13 (5), 1015. doi: 10.3390/cancers13051015
Elissawy A. M., Soleiman Dehkordi E., Mehdinezhad N., Ashour M. L., Mohammadi Pour P. (2021). Cytotoxic alkaloids derived from marine sponges: A comprehensive review. Biomolecules 11 (2), 258. doi: 10.3390/biom11020258
Eliyatkın N., Yalçın E., Zengel B., Aktaş S., Vardar E. (2015). Molecular classification of breast carcinoma: From traditional, old-fashioned way to a new age, and a new way. J. Breast Health 11, 59–66. doi: 10.5152/tjbh.2015.1669
Essack M., Bajic V., Archer J. (2011). Recently confirmed apoptosis-inducing lead compounds isolated from marine sponge of potential relevance in cancer treatment. Mar. Drugs 9 (12), 1580–1606. doi: 10.3390/md9091580
He B., Sui X., Yu B., Wang S., Shen Y., Cong H. (2020). Recent advances in drug delivery systems for enhancing drug penetration into tumors. Drug Del. 27, 1474–1490. doi: 10.1080/10717544.2020.1831106
Holiday D., Speirs V. (2011). Choosing the right cell line for breast cancer research. Breast Cancer Res. 13 (4), 215. doi: 10.1186/bcr2889
Jaaskelainen A., Roininen N., Karihtala P., Jukkola A. (2020). High parity predicts poor outcomes in patients with luminal b-like (HER2 negative) early breast cancer: A prospective Finnish single-center study. Front. Oncol. 10, 1470. doi: 10.3389/fonc.2020.01470
Jang K. H., Lee Y., Sim C. J., Oh K. B., Shin J. (2012). Bioactive lipids from the sponge Spirastrella abata. Bioorg. Med. Chem. Lett. 22 (2), 1078–10 81. doi: 10.1016/j.bmcl.2011.11.105
Jimenez P. C., Wilke D. V., Costa-Lotufo L. V. (2018). Marine drugs for cancer: Surfacing biotechnological innovations from the oceans. Clinics (Sao Paulo) 73 (Suppl. 1), e482s. doi: 10.6061/clinics/2018/e482s
Kalimuthu S., Se Kwon K. (2013). Cell survival and apoptosis signaling as therapeutic target for cancer: Marine bioactive compounds. Int. J. Mol. Sci. 14 (2), 2334–2354. doi: 10.3390/ijms14022334
Kapuscinski J. (1995). DAPI: A DNA-specific fluorescent probe. Biotech. Histochem. 70 (5), 220–233. doi: 10.3109/10520299509108199
Kumar M. S., Adki K. M. (2018). Marine natural products for multi-targeted cancer treatment: A future insight. BioMed. Pharmacother. 105, 233–245. doi: 10.1016/j.biopha.2018.05.142
Kumar M., Jogan S. (2014). Primary characterization of sponge associated bacteria of marine sponges - halichondria glabrata, cliona lobata, Spirastrella pachyspira and their antimicrobial properties. J. Microbiol. Biotech. Food Sci. 4 (2), 117–121. doi: 10.15414/jmbfs.2014.4.2.117-121
Kumar M. S., Shah B. (2014). Comparative structural morphometry and elemental composition of three marine sponges from western coast of India. Microsc. Res. Tech. 77 (4), 296–304. doi: 10.1002/jemt.22343
Liang X., Zhang J., Ou H., Chen J., Mitragotri S., Chen M. (2020). Skin delivery of siRNA using sponge spicules in combination with cationic flexible liposomes. Mol. Ther. Nucleic Acids 20, 639–648. doi: 10.1016/j.omtn.2020.04.003
Li M., Wu R. S., Tsai J. S. (2003). DAPI derivative: A fluorescent DNA dye that can be covalently attached to biomolecules. Bioorg. Med. Chem. Lett. 13 (24), 4351–4354. doi: 10.1016/j.bmcl.2003.09.038
Malhão F., Ramos A. A., Buttachon S., Dethoup T., Kijjoa A., Rocha E. (2019). Cytotoxic and antiproliferative effects of preussin, a hydroxypyrrolidine derivative from the marine sponge-associated fungus Aspergillus candidus KUFA 0062, in a panel of breast cancer cell lines and using 2D and 3D cultures. Mar. Drugs 17 (8), 448. doi: 10.3390/md17080448
Martin M. J., Berrue F., Amade P., Fernández R., Francesch A., Reyes F., et al. (2005). Halogenated helinane derivatives from the marine sponge Spirastrella hartmani. J. Nat. Prod. 68 (10), 1554–1555. doi: 10.1021/np050247f
Meyer C. J., Krauth M., Wick M. J., Shay J. W., Gellert G., De Brabander J. K., et al. (2015). Peloruside a inhibits growth of human lung and breast tumor xenografts in an athymic nu/nu mouse model. Mol. Cancer Ther. 14 (8), 1816–1823. doi: 10.1158/1535-7163.MCT-15-0167
Mitri Z., Constantine T., O’regan R. (2012). The HER2 receptor in breast cancer: Pathophysiology, clinical use, and new advances in therapy. Chemother. Res. Pract. 2012, 743193. doi: 10.1155/2012/743193
Mohan Kumar A. S., selvi T. (2012). Prospective study on antimicrobial protein of Spirastrella inconstans against methicillin resistant Staphylococcus aureus (MRSA). Int. J. Biol. Chem. Sci. 6 (4), 1428–1436. doi: 10.4314/ijbcs.v6i4.3
Morinaka B. I., Molinski T. F. (2011). A long-chain chlorodibromohydrin amide from the sponge Spirastrella mollis. Org. Lett. 13 (24), 6338–6341. doi: 10.1021/ol2024778
Mosmann T. (1983). Rapid colorimetric assay for cellular growth and survival: application to proliferation and cytotoxicity assays. J. lmmunol. Methods 65 (1-2), 55–63. doi: 10.1016/0022-1759(83)90303-4
Nakarada D., Pejin B., Tommonaro G., Mojovic M. (2020). Liposomal integration method for assessing antioxidative activity of water insoluble compounds towards biologically relevant free radicals: example of avarol. J. Liposome Res. 30, 218–226. doi: 10.1080/08982104.2019.1625378
OECD (2002). “Guidelines for the testing of Chemicals/Section 4,” in Health effects test no. 423: Acute oral toxicity - acute toxic class method (Paris, France: Organization for Economic Cooperation and Development).
Pettit G., Herald C., Cichacz Z., Gao F., Schmidt J., Boyd M., et al. (1993). Isolation and structure of the powerful human cancer cell growth inhibitors spongistatins 4 and 5 from an African Spirastrella spinispirulifera (Porifera). J. Chem. Soc. Chem. Commun. 24), 1805–1807. doi: 10.1039/c39930001805
Pratt I. S. (2002). Global harmonisation of classification and labelling of hazardous chemicals. Toxicol. Lett. 128 (1-3), 5–15. doi: 10.1016/S0378-4274(01)00529-X
Puchalapalli M., Zeng X., Mu L., Anderson A., Hix Glickman L., Zhang M., et al. (2016). NSG mice provide a better spontaneous model of breast cancer metastasis than athymic (Nude) mice. PloS One 11 (9), e0163521. doi: 10.1371/journal.pone.0163521
Ramanjooloo A., Andersen R. J., Bhaw-Luximon A. (2021). Marine sponge-derived/inspired drugs and their applications in drug delivery systems. Fut Med. Chem. 13, 487–504. doi: 10.4155/fmc-2020-0123
Ratnasari T., Sri Wulandari R. A., Balqis and Indarto D. (2018). Identification of human epidermal growth factor receptor 2 antagonist in silico for breast cancer therapy, derived from indonesian phytochemicals. 2018 2nd International Conference on Biomedical Engineering (IBIOMED) 2018, 29–33. doi: 10.1109/IBIOMED.2018.8534924
Ren W., Liu Y., Wan S., Fei C., Wang W., Chen Y., et al. (2014). BMP9 inhibits proliferation and metastasis of HER2-positive SK-BR-3 breast cancer cells through ERK1/2 and PI3K/AKT pathways. PloS One 9 (5), e96816. doi: 10.1371/journal.pone.0096816
Roskoski R. (2014). ErbB/HER protein-tyrosine kinases: Structures and small molecule inhibitors. Pharmacol. Res. 87, 42–59. doi: 10.1016/j.phrs.2014.06.001
Ruiz-Torres V., Encinar J. A., Herranz-López M., Pérez-Sánchez A., Galiano V., Barrajón-Catalán E., et al. (2017). An updated review on marine anticancer compounds: The use of virtual screening for the discovery of small-molecule cancer drugs. Molecules 22 (7), 1037. doi: 10.3390/molecules22071037
Sagar S., Kaur M., Minneman K. P. (2010). Antiviral lead compounds from marine sponges. Mar. Drugs 8, 2619–2638. doi: 10.3390/md8102619
Satyanarayanajois S., Villalba S., Jianchao L., Lin G. M. (2009). Design, synthesis, and docking studies of peptidomimetics based on HER2-herceptin binding site with potential antiproliferative activity against breast cancer cell lines. Chem. Biol. Drug Des. 74 (3), 246–257. doi: 10.1111/j.1747-0285.2009.00855.x
Schnitt S. J. (2010). Classification and prognosis of invasive breast cancer: from morphology to molecular taxonomy. Modern Pathol. 23 Suppl 2, S60–S64. doi: 10.1038/modpathol.2010.33
Stanley A., Ashrafi G. H., Seddon A. M., Modjtahedi H. (2017). Synergistic effects of various her inhibitors in combination with IGF-1R, c-MET and src targeting agents in breast cancer cell lines. Sci. Rep. 7, 3964. doi: 10.1038/s41598-017-04301-8
Varijakzhan D., Loh J. Y., Yap W. S., Yusoff K., Seboussi R., Lim S. E., et al. (2021). Bioactive compounds from marine sponges: Fundamentals and applications. Mar. Drugs 19 (5), 246. doi: 10.3390/md19050246
Wang W., Chen T., Xu H., Ren B., Cheng X., Qi R., et al. (2018). Curcumin-loaded solid lipid nanoparticles enhanced anticancer efficiency in breast cancer. Molecules 23 (7), 1578. doi: 10.3390/molecules23071578
Williams D. E., Roberge M., Van Soest R., Andersen R. J. (2003). An antimitotic macrolide isolated from the Caribbean marine sponge Spirastrella coccinea. J. Am. Chem. Soc. 125 (18), 5296–5297. doi: 10.1021/ja0348602
Yap K. M., Sekar M., Seow L. J., Gan S. H., Bonam S. R., Mat Rani N. N. I., et al. (2021). Mangifera indica (Mango): A promising medicinal plant for breast cancer therapy and understanding its potential mechanisms of action. Breast Cancer 13, 471–503. doi: 10.2147/bctt.s316667
Keywords: breast cancer, Spirastrella pachyspira, SK-BR-3 cell lines, molecular docking, HER2, anticancer, sphingosine
Citation: Morais SR, K C, Jeyabalan S, Wong LS, Sekar M, Chidambaram K, Gan SH, Begum MY, Izzati Mat Rani NN, Subramaniyan V, Fuloria S, Fuloria NK, Safi SZ, Sathasivam KV, Selvaraj S and Sharma VK (2022) Anticancer potential of Spirastrella pachyspira (marine sponge) against SK-BR-3 human breast cancer cell line and in silico analysis of its bioactive molecule sphingosine. Front. Mar. Sci. 9:950880. doi: 10.3389/fmars.2022.950880
Received: 23 May 2022; Accepted: 23 August 2022;
Published: 15 September 2022.
Edited by:
Cataldo Pierri, University of Bari Aldo Moro, ItalyReviewed by:
Inese Cakstina-Dzerve, Riga Stradins University, LatviaCopyright © 2022 Morais, K, Jeyabalan, Wong, Sekar, Chidambaram, Gan, Begum, Izzati Mat Rani, Subramaniyan, Fuloria, Fuloria, Safi, Sathasivam, Selvaraj and Sharma. This is an open-access article distributed under the terms of the Creative Commons Attribution License (CC BY). The use, distribution or reproduction in other forums is permitted, provided the original author(s) and the copyright owner(s) are credited and that the original publication in this journal is cited, in accordance with accepted academic practice. No use, distribution or reproduction is permitted which does not comply with these terms.
*Correspondence: Chitra K, Y2hpdHJhLmtAc3JpcmFtYWNoYW5kcmEuZWR1Lmlu; Ling Shing Wong, bGluZ3NoaW5nLndvbmdAbmV3aW50aS5lZHUubXk=; Mahendran Sekar, bWFoZW5kcmFuc2VrYXJAdW5pa2wuZWR1Lm15
†These authors have contributed equally to this work
Disclaimer: All claims expressed in this article are solely those of the authors and do not necessarily represent those of their affiliated organizations, or those of the publisher, the editors and the reviewers. Any product that may be evaluated in this article or claim that may be made by its manufacturer is not guaranteed or endorsed by the publisher.
Research integrity at Frontiers
Learn more about the work of our research integrity team to safeguard the quality of each article we publish.