- 1Department of Environmental Oceanography, Chonnam National University, Yeosu, South Korea
- 2Big Data Fishery Resource Management Interdisciplinary Program, Chonnam National University, Yeosu, South Korea
- 3Department of Ocean Integrated Science, Chonnam National University, Yeosu, South Korea
In temperate estuaries, rainfall causes environmental fluctuations, such as salinity and suspended particulate matter (SPM), and can affect zooplankton distribution patterns. This study focused on the effect of temporary freshwater inflow on the composition of major zooplankton species and changes in their ecological status in the Seomjin River estuary before (June) and after (August) rainfall in 2018. Environmental data were collected from 14 and 15 stations before and after rainfall, respectively. All factors except for chlorophyll-a (Chl-a) concentration differed significantly before and after rainfall (p<0.05), and a salinity gradient extended to Yeosu Bay from Gwangyang Bay. Zooplankton abundance decreased significantly after rainfall. There was a high correlation between indicator species abundance and environmental factors after rainfall (correlation coefficient: 0.7521); however, the indicator species and environmental factors did not exhibit a significant correlation with salinity before rainfall. In terms of feeding habit composition, the carnivore proportion showed a significant decrease after rainfall compared to before rainfall (p<0.001), while the particle feeder proportion showed a significant increase after rainfall compared to before (p<0.001). In particular, Corycaeus spp. contributed significantly to the decrease in carnivore abundance after rainfall. Among the particle feeders, Copepodites significantly increased in abundance after rainfall. Carnivore abundance was negatively correlated with salinity, and particle feeder abundance was positively correlated with potential prey sources (SPM and Chl-a concentration), suggesting that particle feeders respond to the food-rich environment after rainfall.
Introduction
Estuaries are gradual transition zones between rivers and coasts (Elliott and McLusky, 2002). Estuarine biogeochemical processes, including water mixing, transportation, and salinity gradients, affect the abundance and composition of organisms (Primo et al., 2009; Jones et al., 2016; Gutierrez et al., 2018; Ciszewski et al., 2022). As multiple interactions (e.g., biotic and abiotic, interspecific, and intraspecific) occur simultaneously in an estuarine ecosystem, analyzing the effects of various environmental parameters is challenging (David et al., 2005). In addition, a temporary influx of freshwater can drastically alter the ecosystem in temperate regions during summer (D’Avanzo et al., 1996; Murrell et al., 2018; Shen et al., 2022).
In estuarine environments, zooplankton species diversity and abundance can be affected by many physicochemical conditions and food availability (Froneman, 2004; Modéran et al., 2010; Nandy and Mandal, 2020; Shropshire et al., 2020; Telesh, 2004). In particular, the impact of suspended particulate matter (SPM) on plankton food availability in estuarine–coastal environments may be slightly controversial. Although many studies have shown that SPM has a negative effect on zooplankton in estuarine ecosystems (Alcaraz et al., 1980; White and Dagg, 1989; Arendt et al., 2011; Kang, 2012), several studies have suggested that increases in SPM can have a positive effect on the population maintenance of zooplankton because of the possible nutritional benefits from organic or potential food matter adhered to the SPM (Poulet, 1978; Arruda et al., 1983; Kwon et al., 2002; Cloern et al., 2017). Therefore, SPM can be used as an alternative food source (Poulet, 1978; Roman, 1984; David et al., 2005; David et al., 2006; Lee et al., 2019; Menéndez et al., 2019). Zooplankton have also been recognized as an indicator because they can sensitively respond to environmental changes (Dufrêne and Legendre, 1997; Beaugrand, 2004; Bonnet and Frid, 2004). Copepods, in particular, constitute approximately 70%–90% of the zooplankton abundance in the ocean; thus, it is necessary to understand how they respond to rainfall events and contribute to biogeochemical fluctuations (Kiørboe, 1997; Madhupratap, 1999; Thompson et al., 2013).
In the study area, many studies were focused on zooplankton distribution by the salinity gradient or their seasonal variation (Park et al., 2002; Jang et al., 2004; Lee et al., 2017). However, few studies have evaluated the increase in SPM as a potential food source after rainfall, as well as the changes in the feeding habits of zooplankton caused by the increase in SPM. In this study, we aimed to find and evaluate the factors influenced by a rainfall event in temperate estuarine–coastal environments on changes in the zooplankton community structure and feeding habit composition. To achieve these purposes, we analyzed the following items: (1) the relationship between the indicator species and various environmental variables and (2) changes in the feeding habit composition of the dominant species.
Materials and methods
Study region
Yeosu Bay is located in the south-central region of the Korean Peninsula (Figure 1) and is connected to Gwangyang Bay through the Yeosu Channel. Gwangyang Bay is generally shallow, with a water depth of 2.4–8.0 m compared with the water depth of 30 m in Yeosu Bay (Kim et al., 2014; Choo, 2020). The Seomjin River and Gwangyang Bay are major introducers of nutrients and SPM to the study area (Kwon et al., 2001). However, Kang et al. (2019; 2020a; 2020b) reported that the main component of SPM is a phytoplankton-based autochthonic source, although SPM is gradually increasing, owing to artificial activities such as dredging and reclamation in Gwangyang Bay. The southern part of Yeosu Bay is connected to the South Sea of Korea and is seasonally affected by the Tsushima Warm Current. The tidal cycle is semidiurnal, with a maximum tidal range of 3.40 m during spring tide and 1.10 m during neap tide (Shaha and Cho, 2009). In summer, 300–400 m3 s-1 of the Seomjin River water is continuously discharged into Gwangyang Bay and the Yeosu Channel, with 81.5% outflowing to the outside of Yeosu Bay through the Yeosu Channel (Kim et al., 2014). In this study, 15 stations were chosen in Gwangyang Bay in the Seomjin River Estuary (Stations 1–4) and Yeosu Bay (Stations 5–15).
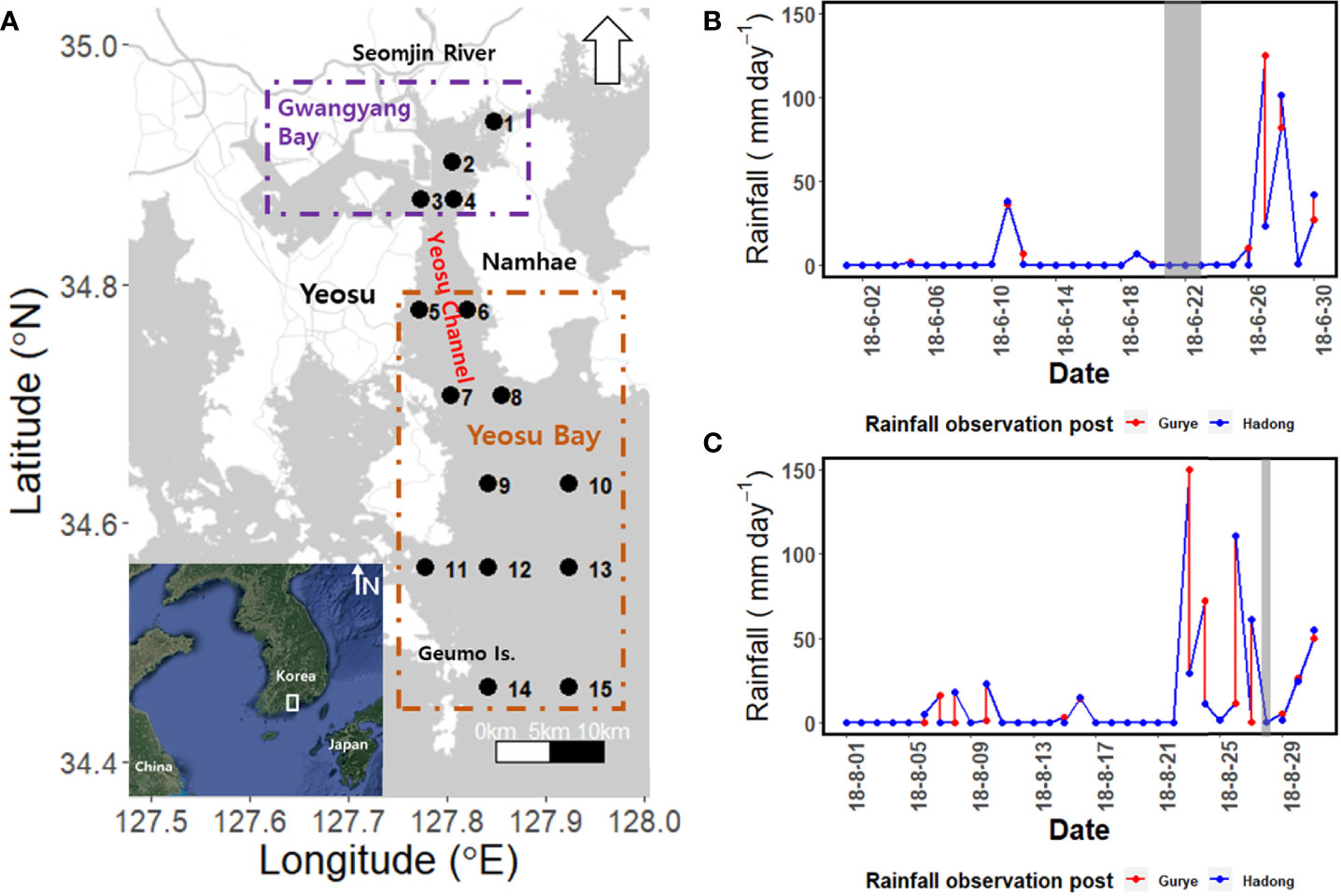
Figure 1 (A) Map of the study region, including the Yeosu Channel. Rainfall data for Seomjin River (B) upstream (Gurye) and (C) downstream (Hadong). Shaded dates indicate the survey period.
Environmental factors
Data were collected in June and August 2018, before and after rainfall. We considered the salinity gradient from the downstream entrance of the Seomjin River to the southern end of Geumo Island using the shipping vessel R/V Cheong-Gyeong-Ho from the Chonnam National University (Figure 1). Sea surface temperature (SST) and salinity data were collected using a multiparameter water quality measurement device (Water quality multi-parameter Pro DSS; YSI, Ohio, USA) in June, and a conductivity, temperature, and depth profiler (CTD) (SBE 19plus V2; Sea-bird Electronic, Washington, USA) in August. Although salinity measurements are unitless as indicated by recent studies (Williams et al., 2015; Gustafson et al., 2022; Wang et al., 2022), we used “psu” for distinguishing from classical unit (‰). Chlorophyll-a (Chl-a) concentration was obtained at each station by collecting 500 ml of surface seawater using a plastic bucket and filtering it through Whatman glass fiber filters {GF/F 47 mm; Whatman, Birmingham, UK). The filtered glass fibers were frozen until measurement. After adding 90% acetone to the frozen glass fiber filter, the pigment was extracted for 24 h.
Chl-a concentrations were measured using a spectrophotometer (Mega-800; Scinco Co., Seoul, Korea), according to the method described by Parsons et al. (1984). For SPM measurements, a glass fiber filter {GF/F 47 mm; Whatman, Birmingham, UK) was washed with distilled water in the laboratory and predried at 60°C for 24 h in a drying oven. The dried filter paper was weighed using an analytical balance (PR124/E; OHAUS Corp., New Jersey, USA) prior to sampling. In situ, 500 ml of surface seawater was collected in a plastic bucket, filtered through GF/F, frozen at –20°C, and transported to the laboratory. The dry weight of SPM was measured by drying the transported filter at 60°C for 24 h in a drying oven and weighing it (Korea’s Ministry of Environment, 2018). Owing to the possible effects of rainfall in the upper (Gurye) and lower (Hadong) reaches of the Seomjin River on the zooplankton community in Yeosu Bay, relevant data from these two locations were extracted from the Water Resources Management Information System (Figures 1B, C).
Zooplankton sample collection
Owing to the large variation in zooplankton abundance, we performed sampling when the sea level was the highest during the spring tide period. Zooplankton were collected vertically using a conical net (net mouse diameter: 45 cm; mesh size: 200 µm). To calculate the filtered seawater volume, a flowmeter (Model 488115; Hydro-Bios Co., Altenholz, Germany) was attached to the entrance of the net. The collected zooplankton were immediately fixed in the field with a sodium tetraborate–buffered formaldehyde solution at a final concentration of 5%. Zooplankton samples were subsampled using a Folsom-type splitter of 1/8–1/128. Species identification of the zooplankton samples was performed where possible using a stereo microscope (SMZ645; Nikon, Tokyo, Japan), and the microstructure of appendages was observed using a high-magnification optical microscope (Eclipse E200; Nikon, Tokyo, Japan). Zooplankton samples were converted into individuals per cubic meter (inds. m-3). Species were identified according to Chihara and Murano (1997); Soh (2010); Soh et al. (2013), and Soh and Moon (2014), and taxonomic systematics followed the WoRMS Editorial Board (2022).
Data and statistical analyses
Data converted to the log (x+1) index were used to normalize the total zooplankton abundance. For environmental factors (water temperature, salinity, Chl-a concentration, and SPM), Z-score standardization was performed to remove the units. Hierarchical cluster analysis was performed according to the Ward link method based on the Bray–Curtis dissimilarity index to measure similarities in zooplankton communities between stations (Legendre and Legendre, 1998). In addition, cluster analysis was performed using the nMDS (non-metric multidimensional scaling) ordination method (Clarke, 1993).
Using the cluster analysis results, an indicator value analysis was performed to identify the indicator species that affected each classified group (Dufrêne and Legendre, 1997). Subsequently, canonical correspondence analysis (CCA) and correlation analysis were performed by converting the indicator species abundance data to log (x+1) + 1 because of the exclusive species abundance by the assemblage group.
Bio-ENV analysis was performed to detect significant correlations between indicator species abundance and environmental variables (de Carvalho et al., 2015). A t-test was performed to identify the differences in environmental factors between the non-rainy period (June) and immediately after rainfall (August), and then, the differences in total zooplankton abundance were confirmed using the Mann–Whitney U test. In addition, the feeding habits of the zooplankton observed in Yeosu Bay in June and August were obtained from previous studies (Table 1). A chi-square test was performed to confirm the differences in the zooplankton feeding habit composition according to period. All data analyses were performed using the R program (version 4.1.0, R Core Team, 2020), whereas another graphing was performed using the “ggplot2” package; indicator species analysis using the “indicspecies” package; and CCA, correlation analysis, and Bio-ENV analysis using the “vegan” package.
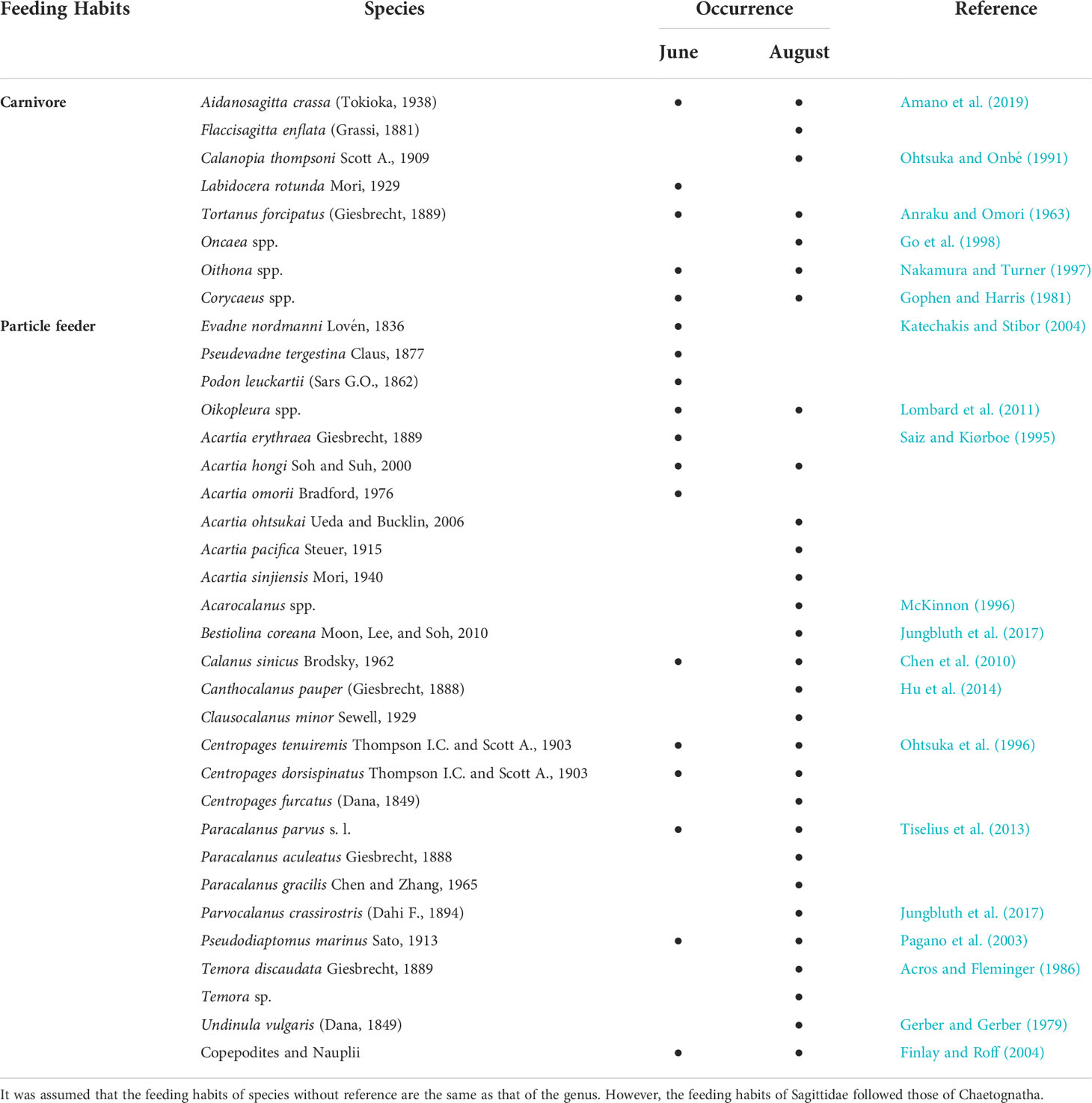
Table 1 Observed zooplankton occurrence in Yeosu Bay in June and August according to the feeding habits.
Results
Environmental factors
The SST ranged from 22.5°C to 26.0°C before the rainfall and from 26.3°C to 28.0°C after rainfall (Table 2). Both before and after rainfall, the mean SST was higher in Gwangyang Bay than in Yeosu Bay. In Yeosu Bay, the SST was relatively low in the southwest regions (Figures 2A, B). Surface salinity ranged from 28.3 to 30.7 psu before the rainfall and from 20.8 to 32.8 psu after rainfall (Table 2). There were few differences in the salinity gradient between Gwangyang Bay and Yeosu Bay before the rainfall, but after rainfall, the salinity gradient extended to Yeosu Bay (Figures 2C, D).
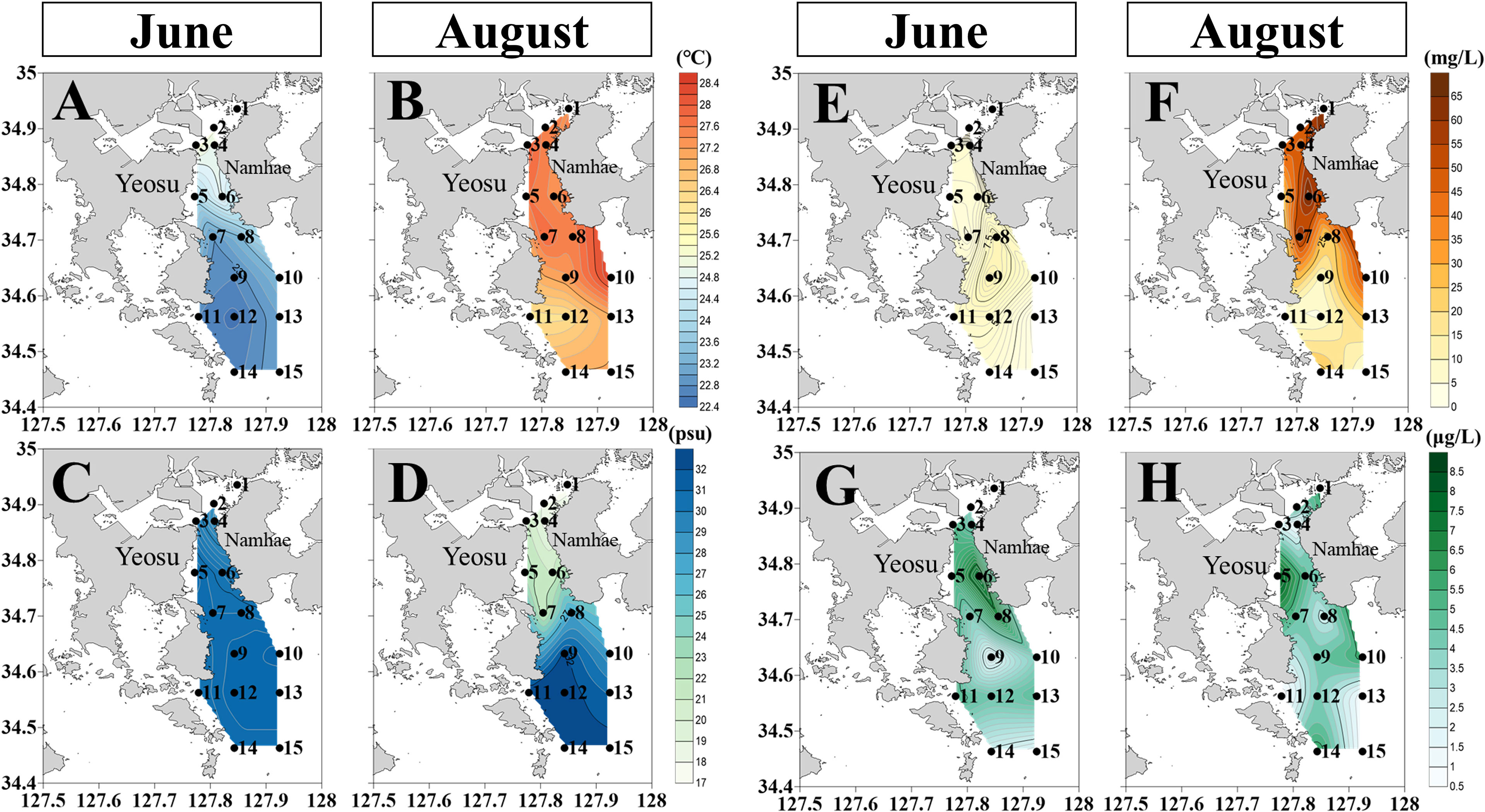
Figure 2 Horizontal distribution of environmental variables in June and August 2018. Sea surface temperature during June (A) and August (B). Surface salinity during June (C) and August (D). SPM during June (E) and August (F). Chlorophyll–a concentration during June (G) and August (H).
SPM ranged from 0.4 to 18.1 mg L-1 before the rainfall and from 1.6 to 68.8 mg L-1 after rainfall (Table 2). There were no significant spatial differences, except in Station 2, before the rainfall. After rainfall, the SPM gradually decreased with increasing distance from the entrance of the Seomjin River estuary, and its tendency was similar to the distribution of the surface salinity gradient (Figures 2E, F). The Chl-a concentration ranged from 1.7 to 7.4 μg L-1 before the rainfall and from 0.5 to 8.5 μg L-1 after rainfall (Table 2). The Chl-a concentration was higher in the northeast of Yeosu Bay and lower in the center of Yeosu Bay before the rainfall, whereas it was higher in the central part of Yeosu Bay and was uniformly distributed after rainfall (Figures 2G, H).
The t-test analysis revealed that the average salinity before rainfall was significantly higher than that after rainfall (p<0.001). Water temperatures and the SPM were higher after rainfall than before rainfall (p<0.05 and p<0.001, respectively). The Chl-a concentration was not significantly different before and after rainfall (p>0.05). The t-test analysis revealed that the average salinity before rainfall was significantly higher than that after rainfall (p<0.001). SST and SPM were higher after rainfall than before rainfall (p<0.05 and p<0.001, respectively).
Zooplankton abundance pattern before and after rainfall
Four taxonomic groups (Appendicularians, Branchiopods, Copepods, Chaetognaths) were present before rainfall, and among these, only branchiopods were absent after rainfall (Figure 3). Appendicularian abundance ranged from 2.6% to 17.1%, except for Station 7 (no occurrence), before the rainfall, and in some transition zones (Stations 6–9) ranged from 0.4% to 2.8% after rainfall (Figure 3B). The proportion of appendicularians was higher in Yeosu Bay than in Gwangyang Bay before rainfall (Figure 3B). Branchiopod abundance ranged from 0.3% to 15.9% before rainfall and did not occur after rainfall (Figure 3). Branchiopods occupy a higher proportion in Yeosu Bay than in Gwangyang Bay. Copepod abundance ranged from 58.7% to 96.6% before rainfall and from 79.7% to 98.0 % after rainfall (Figure 3). Copepods were the most dominant among the zooplankton taxa, accounting for more than 58% of the total zooplankton abundance both before and after rainfall. Chaetognath abundance ranged from 0.3% to 29.8% before the rainfall and from 2.0% to 20.3% after rainfall (Figure 3). The proportion of chaetognaths before rainfall was higher in Yeosu Bay than in Gwangyang Bay, whereas it tended to be higher in Gwangyang Bay than in Yeosu Bay (Figure 3B).
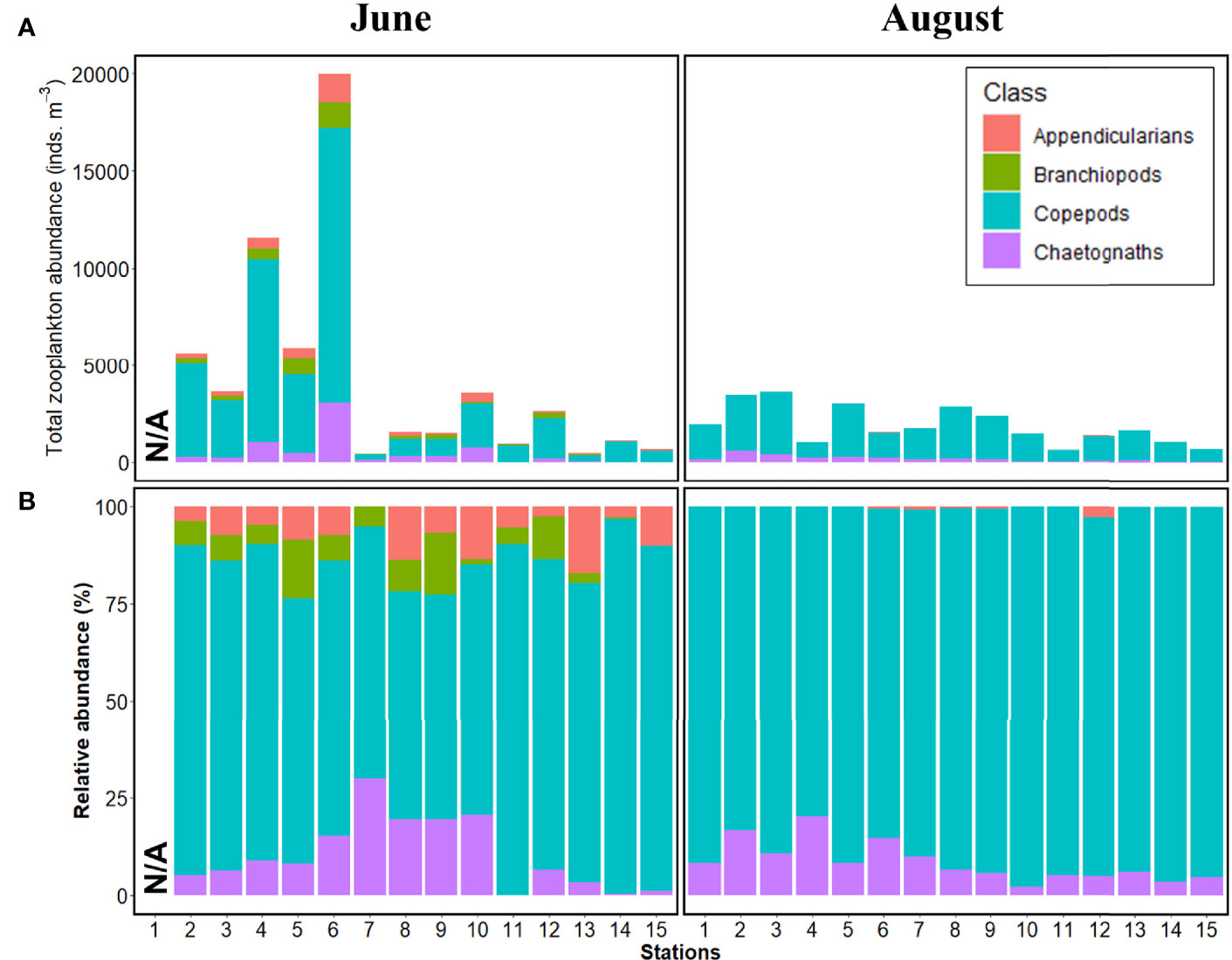
Figure 3 Zooplankton abundance patterns in Yeosu Bay in June (left) and August (right). (A) Total zooplankton abundance (inds. m-3). (B) Stacked percentage (%) of zooplankton abundance. N/A represents non-sampled stations.
The zooplankton abundance ranged from 416 to 19,975 inds. m-3 before rainfall, and 617–3,582 inds. m-3 after rainfall (Figure 3A). A significant decrease was observed in the total zooplankton abundance after rainfall (mean: 405±833 inds. m-3) than that before rainfall (mean: 141±331 inds. m-3) (Figure 3A, p<0.001, Mann–Whitney U test). Copepod abundance significantly contributed to the decrease in the total zooplankton abundance.
Feeding habit composition of the dominant species before and after rainfall
Acartia omorii, Paracalanus parvus s. l., Corycaeus spp., and copepodites were predominant before rainfall, and Bestiolina coreana, Centropages dorsispinatus, P. parvus s. l., and copepodites after rainfall (Figure 4). Copepodites were the most dominant taxa both before and after rainfall. The proportions of A. omorii and P. parvus s. l. tended to decrease from Gwangyang Bay to Yeosu Bay before rainfall, whereas the proportions of copepodites and P. parvus s. l. tended to increase from Gwangyang Bay to Yeosu Bay after rainfall (Figure 4). Zooplankton were distinguished by their two feeding habits (particle feeders, PFs; carnivores) before and after rainfall (Figure 4B). Before the rainfall, PFs ranged from 59.4% to 87.0%, and the carnivore proportion ranged from 13.0% to 40.6% (Figure 4B). After rainfall, the proportion of PFs increased by 70.3–94.9% and that of carnivores decreased by 5.1–29.7% (Figure 4B). PF accounted for more than 50% of the total zooplankton abundance both before and after rainfall. In addition, a significant increase in the PF proportion (Table 3, p<0.001) and a significant decrease in the carnivore proportion before and after rainfall were confirmed (Table 3, p<0.001).
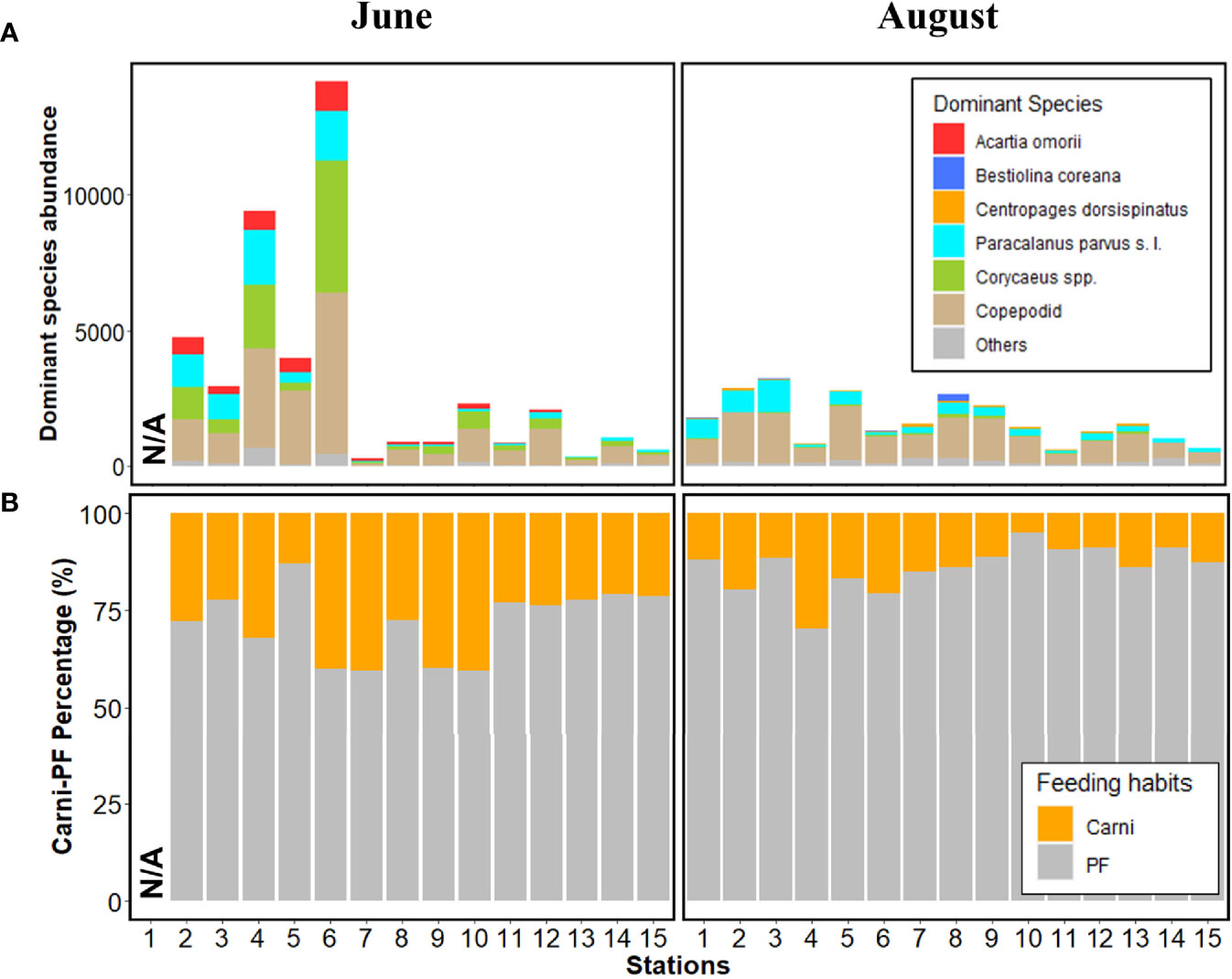
Figure 4 (A) Copepod abundance (inds. m-3) in June (left) and August (right). (B) Carnivore-particle feeder percentage (%). N/A represents non-sampled stations. Carni, carnivores; PF, particle feeder.
Before rainfall, the carnivore abundance was positively correlated with SST, salinity, SPM, and Chl-a concentration. PF abundance also showed a positive correlation with all environmental variables, and the relationship with SPM was highly significant (Table 4, p<0.001). After rainfall, the carnivore abundance was positively correlated with SST, SPM, and Chl-a concentration but negatively correlated with salinity. However, water temperature and Chl-a concentrations were the only significant factors (Table 4; p<0.01 and p<0.05, respectively). Except for the relationship with salinity, which was not statistically significant (Table 4, p>0.05), the other factors exhibited a positive correlation (p<0.05). This correlation result suggests that salinity caused a reduction in the carnivore abundance, and prey factors (SPM and Chl-a concentration) were affected by an increase in PF abundance.
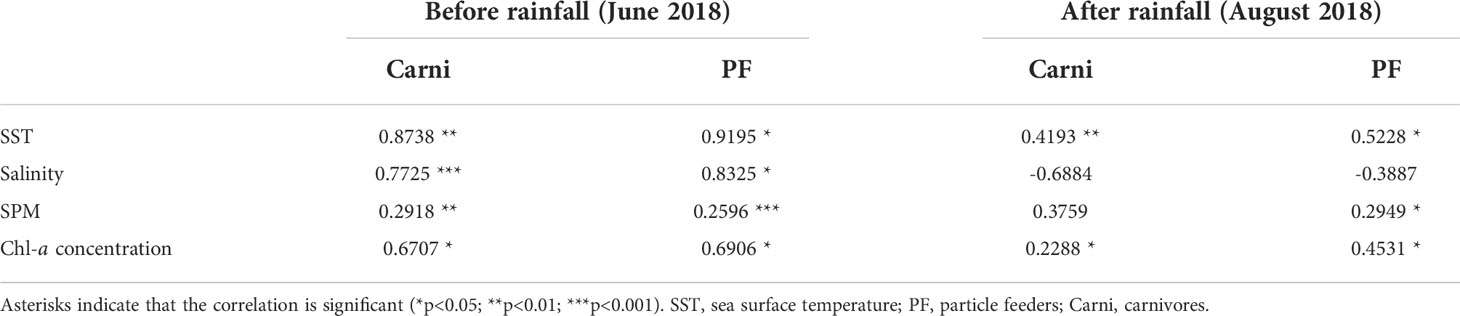
Table 4 Pearson correlation between the abundance of feeding habit groups on zooplankton and environment factors.
Factors controlling variations in indicator species before and after rainfall
Based on Bray–Curtis dissimilarity–based cluster analysis, the study area was divided into three groups by zooplankton assemblages before rainfall (A, B, and C) and two groups after rainfall (A and B). IndVal analysis was performed only in Groups A and C before rainfall and Groups A and B after rainfall (Figure 5; Table 5). Although Group B included several stations before rainfall event, there were no species with an indicator power index greater than 25. Neritic species (A. erythraea, A. hongi, Evadne nordmanni, Labidocera rotunda, and Tortanus forcipatus) were extracted from both Groups A and C before rainfall, and they were statistically insignificant (Table 5). After rainfall, Group A comprised neritic and brackish species (A. erythraea, A. ohtuskai, A. sinjiensis, B. coreana, Parvocalanus crassirostris, and Pseudodiaptomus marinus), whereas Group B mainly comprised offshore warm-current species (A. pacifica, Canthocalanus pauper, C. furcatus, and Temora discaudata) in Yeosu Bay (Table 5).
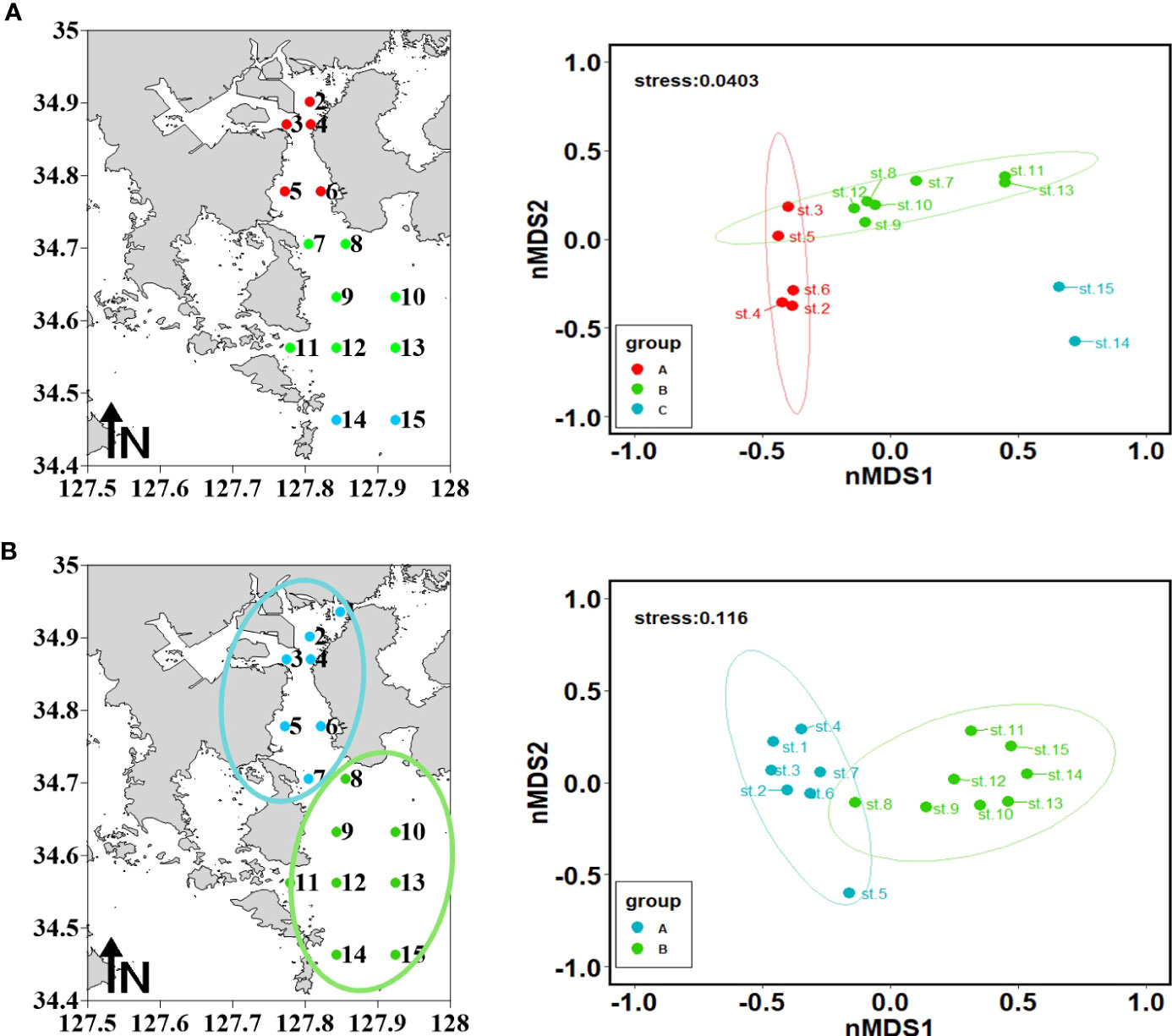
Figure 5 Results of Bray–Curtis dissimilarities–based cluster analysis and non-metric multidimensional scaling (nMDS) ordination plots for June (A) and August (B).
The accumulated contribution of the two axes dividing Groups A and C was 83.86% before rainfall. The factors that had a significant correlation with neritic indicator species (E. nordmanni, A. hongi, A. erythraea, and T. forcipatus) before rainfall were surface water temperature and surface salinity (p<0.05) (Figures 6A, C). However, they did not significantly correlate with other environmental factors (Figures 6A, C). Indicator species showed a positive correlation with water temperature and a negative correlation with salinity (p<0.05) (Figure 6C). After rainfall, the accumulated contribution of the two axes, dividing groups A and B, was 86.62% (Figure 6B). Indicator species were consisted of A. erythraea, B. coreana, A. ohtsukai, Parvocalanus crassirostris, Pseudocalanus marinus, and A. sinjiensis for Group A and Calanus sinicus, Canthocalanus pauper, Temora discaudata, C. furcatus, and A. pacifica for Group B. The indicator species of Group A showed a positive correlation with SST and SPM and a negative correlation with salinity (p<0.05) (Figure 6D). The indicator species of Group B showed a positive correlation with salinity and a negative correlation with SST, Chl-a concentration, and SPM (p<0.05) (Figure 6D). Moreover, after rainfall, there was a high correlation between the indicator species and the environmental factors (Figure 6D, p<0.05).
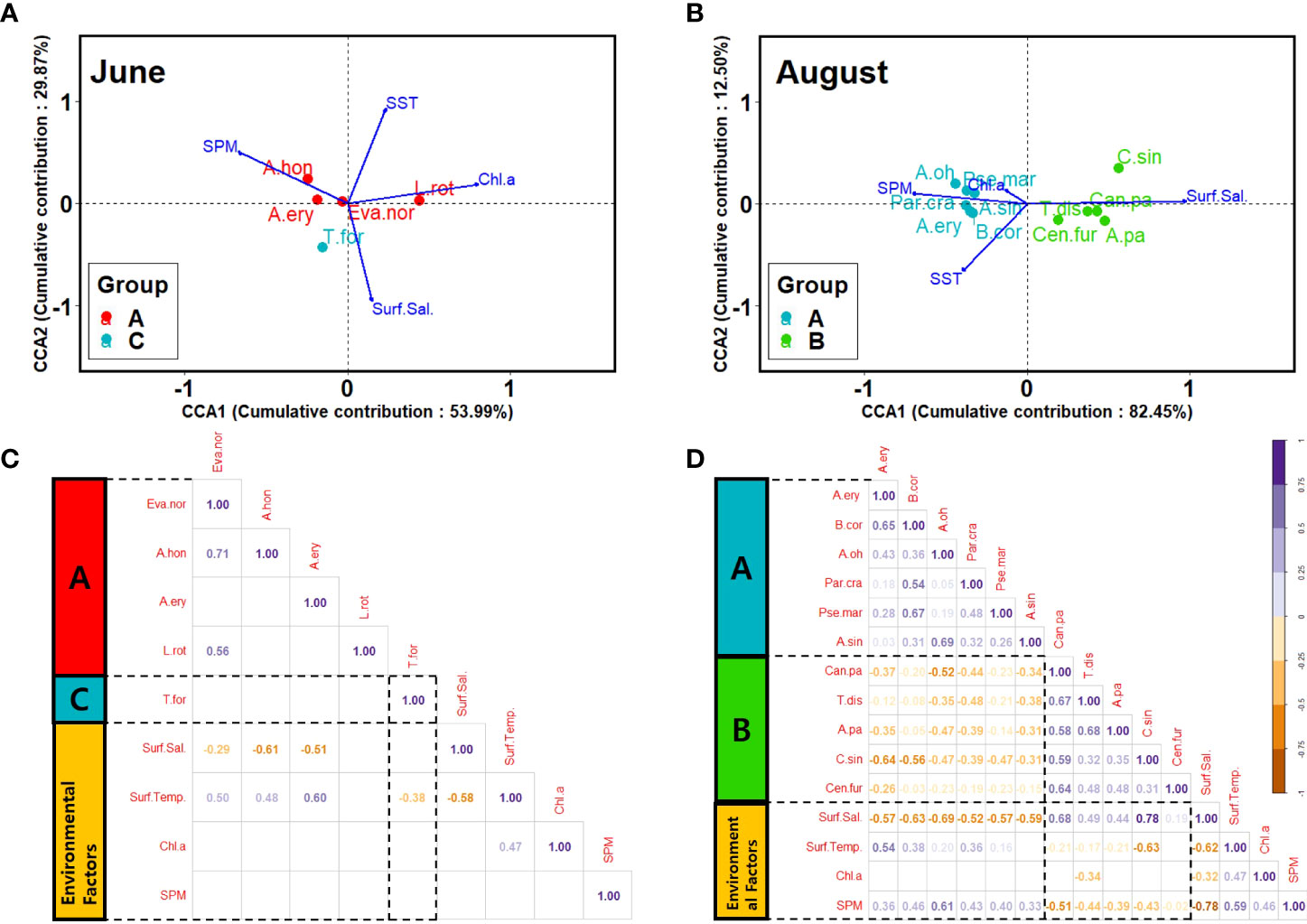
Figure 6 Results of canonical correspondence analysis (CCA) and correlation analysis. The correlation analysis between indicator species and environmental factors in this study (p<0.05). (A, C) June; (B, D) August. SST, Sea surface temperature; Eva. nor, Evadne nordmanni; A. hon, Acartia hongi; A. ery, A. erythraea; L. rot, Labidocera rotunda; T. for, Tortanus forcipatus; A. pa, A. pacifica; A. oh, A. ohtsukai; C. sin, Calanus sinicus; Can. pa, Canthocalanus pauper; B. cor, Bestiolina coreana; Par. cra, Parvocalanus crassirostris; T. dis, Temora discaudata.
Bio-ENV was used to analyze the combination of environmental factors that exerted the greatest effects on indicator species abundance, and the results indicated the highest correlation between surface water temperature and surface salinity before rainfall (correlation coefficient: 0.7576, Table 6). However, the highest correlation was observed for a single combination of surface salinity after rainfall (correlation coefficient: 0.7521, Table 6).
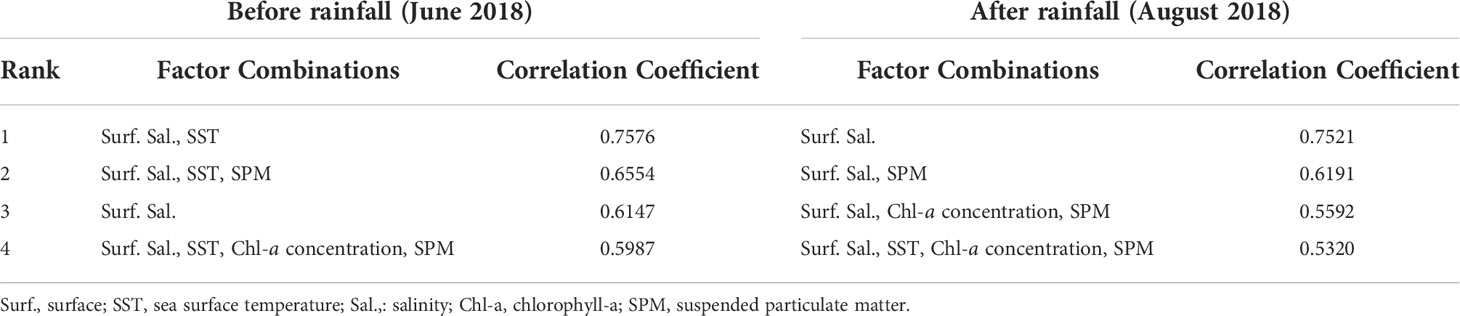
Table 6 Correlation between environmental factors and log (x+1) + 1-transformed zooplankton abundance data using Bio-ENV analysis (Spearman rank correlation).
Discussion
SST increased overall, owing to the time difference before and after rainfall (Figures 2A, B). Salinity gradually increased with increasing distance from the Seomjin River after rainfall, whereas there was no spatial difference before rainfall (Figures 2C, D). Although salinity has been recognized as an important factor in the distribution of zooplankton in the Seomjin River Estuary, the salinity gradient can vary greatly depending on rainfall conditions, even within the same season (Figures 2C, D). Large amounts of freshwater are introduced from the surrounding areas into the temperate estuaries during summer (Jha et al., 2013; Parab et al., 2013; Sahu et al., 2013). The inflow of freshwater can transport nutrients to increase the phytoplankton biomass (Zhao and Guo, 2011). However, Chl-a concentration was not significantly different before and after rainfall in this study (p>0.05, t-test), indicating that there was no difference in the phytoplankton biomass used as a food source for zooplankton before and after rainfall (Figures 2G, H). In contrast, SPM increased significantly after rainfall compared to before rainfall (p<0.05, t-test). It has been found that SPM in the study area is mainly dead or aggregated phytoplankton rather than inorganic matter (Kang et al., 2019; Kang et al., 2020a). SPM inflow from the Seomjin River can be recognized as an increase in the nutritional opportunities for PFs. The PF group increased in abundance, and the carnivore group abundance significantly decreased after rainfall (Table 3). SPM can play a pivotal role as a food source for zooplankton (e.g., copepods and cladocerans) if organic matter-based or nutrient substances are attached to inorganic substances (Poulet, 1978; Arruda et al., 1983). Therefore, these results and the origin of SPM in the study area show that SPM acts as a potential food source for PFs. To understand the fluctuating characteristics of estuaries, it is necessary to periodically monitor the zooplankton population and distribution according to their feeding habits.
According to the zooplankton monitoring data of over 21 years in the study area, PFs were the dominant group in most studies (Table 7). Since the study region is constantly affected by the Seomjin River water, the continuous inflow of SPM seems to consist of an environment in which PF, in particular immature copepods (copepodites), can prosper. However, in previous studies, copepodites have been significantly underestimated in the proportion of PF in feeding habit composition. Therefore, we suggest that the feeding habit composition and the ratio of copepodites can be used as an index that can well express the variability of the environmental condition after rainfall.
Feeding habits after rainfall compared to before rainfall indicated a significant decrease in carnivore proportion and an increase in PF proportion (p<0.001, Table 3). The reduction in the carnivore proportion after rainfall was largely attributed to Corycaeus spp. (Figure 4). Copepodites contributed greatly to the increase in PF proportion after rainfall, which can be explained as follows: first, copepodites and P. parvus s. l., which appeared dominant both before and after rainfall in the study region, would have survived rapid salinity changes because most of them have a euryhaline habitat range (Suh et al., 1991; Moon et al., 2012); second, copepodites and P. parvus s. l. would have increased in environments rich in phytoplankton-based particulate prey transported from the Seomjin River (Kwon et al., 2002; Kang et al., 2019; Kang et al., 2020a). This was supported by the correlation analysis results between zooplankton abundance, feeding habits, and environmental factors. In addition, although the particulate organic matter content was not measured separately in this study, previous studies have confirmed that SPM can be used as a food source by copepods (Poulet, 1978; Roman, 1984; David et al., 2005; Lee et al., 2019; Menéndez et al., 2019).
The abundance of branchiopods and appendicularians, a representative taxonomic group belonging to PF, decreased significantly after rainfall (Figure 3). They had population maintenance strategies such as parthenogenesis or with short generation times under environmental conditions suitable for reproduction and growth (Paffenhöfer, 1973; Hopcroft and Roff, 1995; Hopcroft et al., 1998; Korhola and Rautio, 2001). On the other hand, their populations are reduced by various factors, and many studies have been revealed to be determined by biological and chemical factors rather than physical factors (Brett, 1989; Marmorek and Korman, 1993; Tomita et al., 2003). The inflow of freshwater owing to rainfall can cause rapid changes in the estuarine environment and affect their distribution.
Most copepods are salinity-resistant species, such as neritic or brackish species. In particular, P. parvus s. l. suitably dominate in summer when environmental fluctuations are severe because of their fast reproduction cycle and short egg hatching time (Ianora, 1998). A. omorii occurred only before rainfall, while the species that occurred only after rainfall were B. coreana and C. dorsispinatus (Figure 4A). A. omorii is known to favor water temperatures less than 25°C and is the dominant species in coastal environments (Shim and Yun, 1990; Soh and Suh, 1993). After rainfall, B. coreana and C. dorsispinatus inhabit brackish waters and coastal waters, mainly in the summer in Gwangyang Bay (Jang et al., 2004; Moon et al., 2011). Our results included most of the species that have been studied in Gwangyang and Yeosu Bay (Table 7). In particular, the increase of particle feeders such as B. coreana and C. dorsispinatus seems to be related with the increase of SPM after rainfall. Meanwhile, after rainfall, indicator species analysis clearly distinguished Group A, which was affected by the Seomjin River, from Group B, which was affected offshore, and no statistically significant species were observed in Yeosu Bay, regardless of the sufficiently high indicator power index in the IndVal analysis (Table 5). However, before rainfall, the study area was composed of a single ecological community because of the low correlation with physical factors, such as water temperature and salinity.
Conclusions
This study confirmed that the salinity gradient owing to rainfall is extended to the coast with a large difference, even within the same season. SPM, which rapidly increases with rainfall, can provide nutritional benefits to PFs as a potential food source in estuarine–coastal environments. This suggests that it could contribute to restore the decreased zooplankton populations after rainfall in estuarine–coastal environments. Contrary to previous studies, our study is valuable for evaluating SPM as a potential source of zooplankton and supports its importance as a potential source in temporary, highly turbid estuarine–coastal environments. In future studies, periodic monitoring of the process of restoring zooplankton populations will be required through short-term sampling after rainfall.
Data availability statement
The raw data supporting the conclusions of this article will be made available by the authors, without undue reservation.
Author contributions
YSJ contributed to manuscript writing, data analysis and interpretation, discussion, and statistical analysis; authored and reviewed drafts of the manuscript; and approved the final draft. SC contributed to field sampling, zooplankton identification, manuscript writing, data analysis and interpretation, discussion, and statistical analysis; authored and reviewed the drafts of the manuscript; and approved the final draft. HYS contributed to funding acquisition, conceptualization, data interpretation, and discussion; authored and reviewed drafts of the manuscript; and approved the final draft. All authors have contributed to the manuscript and approved the submitted version.
Funding
This research was a part of the project titled “Research center for fishery resource management based on the information and communication technology” (2022, grant number 20180384) and supported by Korea Institute of Marine Science & Technology Promotion (KIMST, 20220558) funded funded by the Ministry of Oceans and Fisheries.
Acknowledgments
We are grateful to the crew of the R/V Cheong-Gyeong-Ho, and Zooplankton Species Diversity Laboratory members at the Chonnam National University for their support in the field. We would also like to thank Editage (www.editage.co.kr) for the English language editing.
Conflict of interest
The authors declare that the research was conducted in the absence of any commercial or financial relationships that could be construed as a potential conflict of interest.
Publisher’s note
All claims expressed in this article are solely those of the authors and do not necessarily represent those of their affiliated organizations, or those of the publisher, the editors and the reviewers. Any product that may be evaluated in this article, or claim that may be made by its manufacturer, is not guaranteed or endorsed by the publisher.
References
Acros F., Fleminger M. (1986). Distribution of filter-feeding calanoid copepods in the eastern equatorial pacific, CalCOFI rep., vol. 27 (California:California cooperative oceanic fisheries).
Alcaraz M. T., Paffenhoffer G., Strickler R. (1980). 22. catching the algae: a first account of visual observations on filter–feeding calanoids. Evol. Ecol. 3, 241–248.
Amano K., Abe Y., Matsuno K., Yamaguchi A. (2019). Yearly comparison of the planktonic chaetognath community in the chukchi Sea in the summers of 1991 and 2007. Polar. Sci. 19, 112–119. doi: 10.1016/j.polar.2018.11.011
Anraku M., Omori M. (1963). Preliminary survey of the relationship between the feeding habit and the structure of the mouth-parts of marine copepods 1. Limnol. Oceanogr. 8 (1), 116–126. doi: 10.4319/lo.1963.8.1.0116
Arendt K. E., Dutz J., Jónasdóttir S. H., Jung–Madsen S., Mortensen J., Møller E. F., et al. (2011). Effects of suspended sediments on copepods feeding in a glacial influenced sub–Arctic fjord. J. Plankton. Res. 33 (10), 1526–1537. doi: 10.1093/plankt/fbr054
Arruda J. A., Marzolf G. R., Faulk R. T. (1983). The role of suspended sediments in the nutrition of zooplankton in turbid reservoirs. Ecology 64 (5), 1225–1235. doi: 10.2307/1937831
Beaugrand G. (2004). The north Sea regime shift: evidence, causes, mechanisms and consequences. Prog. Oceanogr. 60 (2–4), 245–262. doi: 10.1016/j.pocean.2004.02.018
Bonnet D., Frid C. (2004). Seven copepod species considered as indicators of water-mass influence and changes: results from a Northumberland coastal station. ICES J. Mar. Sci. 61 (4), 485–491. doi: 10.1016/j.icesjms.2004.03.005
Brett M. T. (1989). Zooplankton communities and acidification processes (a review). Wat. Air Soil pollut. 44, 387–414. doi: 10.1007/BF00279267
Chen M. R., Kâ K., Hwang J. S. (2010). Diet of the copepod calanus sinicus brodsky 1962 (Copepoda, calanoida, calanidae) in northern coastal waters of Taiwan during the northeast monsoon period. Crustaceana, 83(7):851–864. doi: 10.1163/001121610x504360
Chihara M., Murano M. (1997). An illustrated guide to marine plankton in Japan (Tokyo, Japan: Tokai University Press), 1–260.
Choo H. S. (2020). Temporal and spatial fluctuation characteristics of sea surface temperature in yeosu bay, Korea. J. Korean Soc Fish. Ocean. Technol. 56 (4), 322–339. doi: 10.3796/KSFOT.2020.56.4.322
Ciszewski K., Wawrzyniak W., Czerniejewski P. (2022). Effect of artificial regime shifts and biotic factors on the intensity of foraging of planktivorous fish. Animals 12 (1), 17. doi: 10.3390/ani12010017
Clarke K. R. (1993). Non-parametric multivariate analyses of changes in community structure. Austral. Ecol. 18 (1), 117–143. doi: 10.1111/j.1442–9993.1993.tb00438.x
Cloern J. E., Jassby A. D., Schraga T. S., Nejad E., Martin C. (2017). Ecosystem variability along the estuarine salinity gradient: Examples from long-term study of San Francisco bay. Limnol. Oceanogr. 62 (S1), S272–S291. doi: 10.1002/lno.10537
D’Avanzo C., Kremer J. N., Wainright S. C. (1996). Ecosystem production and respiration in response to eutrophication in shallow temperate estuaries. Mar. Ecol. Prog. Ser. 141, 263–274. doi: 10.3354/meps141263
David V., Sautour B., Chardy P., Leconte M. (2005). Long-term changes of the zooplankton variability in a turbid environment: the gironde estuary (France). Estuar. Coast. Shelf. Sci. 64 (2-3), 171–184. doi: 10.1016/j.ecss.2005.01.014
David V., Sautour B., Galois R., Chardy P. (2006). The paradox high zooplankton biomass–low vegetal particulate organic matter in high turbidity zones: what way for energy transfer? J. Exp. Mar. Biol. Ecol. 333 (2), 202–218. doi: 10.1016/j.jembe.2005.12.045
de Carvalho G. C., de Sá–Neto R. J., Barros F. (2015). Thirty years of use of multivariate quantitative methods in benthic community ecology of marine and coastal habitats: looking to the past to planning the future. Scientometrics 105 (1), 593–610. doi: 10.1007/s11192–015–1667–6
Dufrêne M., Legendre P. (1997). Species assemblages and indicator species: the need for a flexible asymmetrical approach. Ecol. Monogr. 67 (3), 345–366. doi: 10.1890/0012–9615(1997)067[0345:SAAIST]2.0.CO;2
Elliott M., McLusky D. S. (2002). The need for definitions in understanding estuaries. Estuar. Coast. Shelf. Sci. 55 (6), 815–827. doi: 10.1006/ecss.2002.1031
Finlay K., Roff J. C. (2004). Radiotracer determination of the diet of calanoid copepod nauplii and copepodites in a temperate estuary. ICES. J. Mar. Sci. 61 (4), 552–562. doi: 10.1016/j.icesjms.2004.03.010
Froneman P. W. (2004). Zooplankton community structure and biomass in a southern African temporarily open/closed estuary. Estuar. Coast. Shelf. Sci. 60 (1), 125–132. doi: 10.1016/j.ecss.2003.12.002
Gerber R. P., Gerber M. B. (1979). Ingestion of natural particulate organic matter and subsequent assimilation, respiration and growth by tropical lagoon zooplankton. Mar. Biol. 52 (1), 33–43. doi: 10.1007/bf00386855
Go Y. B., Oh B. C., Terazaki M. (1998). Feeding behavior of the poecilostomatoid copepods oncaea spp. on chaetognaths. J. Mar. Syst. 15 (1–4), 475–482. doi: 10.1016/S0924–7963(97)00038–9
Gophen M., Harris R. P. (1981). Visual predation by a marine cyclopoid copepod, corycaeus anglicus. J. Mar. Biol. Assoc. UK. 61 (2), 391–399. doi: 10.1017/S0025315400047020
Gustafson C. D., Key K., Siegfried M. R., Winberry J. P., Fricker H. A., Venturelli R. A., et al. (2022). A dynamic saline groundwater system mapped beneath an Antarctic ice stream. Science 376 (6593), 640–644. doi: 10.1126/science.abm3301
Gutierrez M. F., Tavşanoğlu Ü.N., Vidal N., Yu J., Teixeira–de Mello F., Çakiroglu A. I., et al. (2018). Salinity shapes zooplankton communities and functional diversity and has complex effects on size structure in lakes. Hydrobiologia 813 (1), 237–255. doi: 10.1007/s10750–018–3529–8
Hopcroft R. R., Roff J. C. (1995). Zooplankton growth rates: extraordinary production by the larvacean oikopleura dioica in tropical waters. J. Plankton Res. 17 (2), 205–220. doi: 10.1093/plankt/17.2.205
Hopcroft R. R., Roff J. C., Bouman H. A. (1998). Zooplankton growth rates: the larvaceans appendicularia, fritillaria and oikopleura in tropical waters. J. Plankton Res. 20 (3), 539–555. doi: 10.1093/plankt/20.3.539
Hu S., Guo Z., Li T., Carpenter E. J., Liu S., Lin S. (2014). Detecting in situ copepod diet diversity using molecular technique: development of a copepod/symbiotic ciliate–excluding eukaryote–inclusive PCR protocol. PloS One 9 (7), e103528. doi: 10.1371/journal.pone.0103528
Ianora A. (1998). Copepod life history traits in subtemperate regions. J. Mar. Syst. 15 (1-4), 337–349. doi: 10.1016/S0924-7963(97)00085-7Acros
Jang M. C., Jang P. G., Shin K. S., Park D. W., Jang M. (2004). Seasonal variation of zooplankton community in gwangyang bay. Korean J. Environ. Biol. 22, 11–29.
Jha D. K., Prashanthi Devi M., Vinithkumar N. V., Das A. K., Dheenan P. S., Venkateshwaran P., et al. (2013). Comparative investigation of water quality parameters of aerial & rangat bay, Andaman islands using in-situ measurements and spatial modelling techniques. Water Qual. Expo. Health 5 (2), 57–67. doi: 10.1007/s12403-013-0086-2
Jones S., Perissinotto R., Carrasco N. K., Vosloo A. (2016). Impact of a flood event on the zooplankton of an estuarine lake. Mar. Biol. Res. 12 (2), 158–167. doi: 10.1080/17451000.2015.1104417
Jungbluth M. J., Selph K. E., Lenz P. H., Goetze E. (2017). Species–specific grazing and significant trophic impacts by two species of copepod nauplii, parvocalanus crassirostris and bestiolina similis. Mar. Ecol. Prog. Ser. 572, 57–76. doi: 10.3354/meps12139
Kang H. K. (2012). Effects of suspended sediments on reproductive responses of paracalanus sp. (Copepoda: Calanoida) in the laboratory. J. Plankton. Res. 34 (7), 626–635. doi: 10.1093/plankt/fbs033
Kang Y., Kang Y. H., Kim J. K., Kang H. Y., Kang C. K. (2020b). Year–to–year variation in phytoplankton biomass in an anthropogenically polluted and complex estuary: A novel paradigm for river discharge influence. Mar. Pollut. Bull. 161, 111756. doi: 10.1016/j.marpolbul.2020.111756
Kang S., Kim J. H., Hwang J. H., Bong Y. S., Ryu J. S., Shin K. H. (2020a). Seasonal contrast of particulate organic carbon (POC) characteristics in the geum and seomjin estuary systems (South Korea) revealed by carbon isotope (δ13C and Δ14C) analyses. Water Res. 187, 116442. doi: 10.1016/j.watres.2020.116442
Kang S., Kim J. H., Kim D., Song H., Ryu J. S., Ock G., et al. (2019). Temporal variation in riverine organic carbon concentrations and fluxes in two contrasting estuary systems: Geum and seomjin, south Korea. Environ. Int. 133, 105126. doi: 10.1016/j.envint.2019.105126
Katechakis A., Stibor H. (2004). Feeding selectivities of the marine cladocerans penilia avirostris, podon intermedius and evadne nordmanni. Mar. Biol. 145 (3), 529–539. doi: 10.1007/s00227–004–1347–1
Kiørboe T. (1997). Population regulation and role of mesozooplankton in shaping marine pelagic food webs. Hydrobiologia 363 (1), 13–27. doi: 10.1023/A:1003173721751
Kim B. J., Ro Y. J., Jung K. Y., Park K. S. (2014). Numerical modeling of circulation characteristics in the kwangyang estuarine system. J. Korean. Soc Coast. Ocean. Eng. 26 (4), 253–266. doi: 10.9765/KSCOE.2014.26.4.253
Korea’s Ministry of Environment (2018). Standard methods for the examination of seawater. 46–49pp. (Korea: NIBR and Ministry of Environment).
Korhola A., Rautio M. (2001). “Cladocera and other branchiopod crustaceans,” in Tracking environmental change using lake sediments (Dordrecht: Springer), 5–41. doi: 10.1007/0-306-47671-1_2
Kwon K. Y., Moon C. H., Kang C. K., Kim Y. N. (2002). Distribution of particulate organic matters along the salinity gradients in the seomjin river estuary. Korean. J. Fish. Aquat. Sci. 35 (1), 86–96. doi: 10.5657/kfas.2002.35.1.086
Kwon K. Y., Moon C. H., Yang H. S. (2001). Behavior of nutrients along the salinity gradients in the seomjin river estuary. Korean. J. Fish. Aquat. Sci. 34 (3), 199–206.
Lee S. J. (2012). “Long term variation of zooplankton community in the northern channel of Gwangyang bay. M. S. Thesis,” (Yeosu, Korea: Chonnam National Univ.).
Lee S. W., Park G., Choi K. H. (2019). Biomass of plankton and macrobenthos and benthic species diversity in relation to environmental gradients in a nationwide coastal survey. Reg. Stud. Mar. Sci. 26, 100502. doi: 10.1016/j.rsma.2019.100502
Lee E. H., Seo M. H., Yoon Y. H., Choi S. D., Soh H. Y. (2017). Environmental factors affecting zooplankton community in gwangyang bay. Korean J. Environ. Biol. 35 (4), 631–639. doi: 10.11626/kjeb.2017.35.4.631
Legendre P., Legendre L. (1998). “Developments in environmental modelling,” in Numerical ecology (Kidlington, UK: Elsevier).
Lombard F., Selander E., Kiørboe T. (2011). Active prey rejection in the filter-feeding appendicularian oikopleura dioica. Limnol. Oceanogr. 56 (4), 1504–1512. doi: 10.4319/lo.2011.56.4.1504
Madhupratap M. (1999). Free-living copepods of the Arabian Sea: Distributions and research perspectives. Indian. J. Mar. Sci. 28, 146–149.
Marmorek D. R., Korman J. (1993). The use of zooplankton in a biomonitoring program to detect lake acidification and recovery. Wat. Air Soil pollut. 69, 223–241. doi: 10.1007/BF00478160
McKinnon A. D. (1996). Growth and development in the subtropical copepod acrocalanus gibber. Limnol. Oceanogr. 41 (7), 1438–1447. doi: 10.4319/lo.1996.41.7.1438
Menéndez M. C., Baleani C. A., Amodeo M. R., Acha E. M., Piccolo M. C. (2019). Assessment of surf zone zooplankton dynamics in a southwestern Atlantic sandy beach: Seasonal cycle and tidal height influence. Estuar. Coast. Shelf. Sci. 227, 106307. doi: 10.1016/j.ecss.2019.106307
Modéran J., Bouvais P., David V., Le Noc S., Simon–Bouhet B., Niquil N., et al. (2010). Zooplankton community structure in a highly turbid environment (Charente estuary, france): Spatio–temporal patterns and environmental control. Estuar. Coast. Shelf. Sci. 88 (2), 219–232. doi: 10.1016/j.ecss.2010.04.002
Moon S. Y., Seo M. H., Shin Y. S., Soh H. Y. (2012). Seasonal variation of mesozooplankton communities in the semi-enclosed muan bay, Korea. Ocean. Polar. Res. 34 (1), 1–18. doi: 10.4217/OPR.2012.34.1.001
Moon S. Y., Seo M. H., Soh H. Y. (2011). Distribution characteristics of two dominant paracalanids on temperature and salinity in the brackish and coastal waters from the west and south coasts of Korea. Korean J. Environ. Biol. 29 (1), 11–16.
Murrell M. C., Caffrey J. M., Marcovich D. T., Beck M. W., Jarvis B. M., Hagy J. D. (2018). Seasonal oxygen dynamics in a warm temperate estuary: effects of hydrologic variability on measurements of primary production, respiration, and net metabolism. Estuaries Coasts 41 (3), 690–707. doi: 10.1007/s12237-017-0328-9
Nakamura Y., Turner J. T. (1997). Predation and respiration by the small cyclopoid copepod oithona similis: How important is feeding on ciliates and heterotrophic flagellates? J. Plankton. Res. 19 (9), 1275–1288. doi: 10.1093/plankt/19.9.1275
Nandy T., Mandal S. (2020). Unravelling the spatio-temporal variation of zooplankton community from the river matla in the sundarbans estuarine system, India. Oceanologia 62 (3), 326–346. doi: 10.1016/j.oceano.2020.03.005
Ohtsuka S., Onbé T. (1991). Relationship between mouthpart structures and in situ feeding habits of species of the family pontellidae (Copepoda: Calanoida). Mar. Biol. 111 (2), 213–225. doi: 10.1007/BF01319703
Ohtsuka S., Shimozu M., Tranmura A., Fukuchi M., Hattori H., Sasaki H., et al. (1996). Relationships between mouthpart structures and in situ feeding habits of five neritic calanoid copepods in the chukchi and northern Bering seas in October 1988 Proceedings of the NIPR Symposium on Polar Biology, (17th symposium on polar biology) (9), 153–168. doi: 10.15094/00005312
Paffenhöfer G. A. (1973). The cultivation of an appendicularian through numerous generations. Mar. Biol. 22 (2), 183–185. doi: 10.1007/BF00391782
Pagano M., Kouassi E., Saint–Jean L., Arfi R., Bouvy M. (2003). Feeding of acartia clausi and pseudodiaptomus hessei (Copepoda: Calanoida) on natural particles in a tropical lagoon (Ebrié, côte d'Ivoire). Estuar. Coast. Shelf. Sci. 56 (3–4), 433–445. doi: 10.1016/S0272–7714(02)00193–2
Parab S. G., Matondkar S. P., Gomes H. D. R., Goes J. I. (2013). Effect of freshwater influx on phytoplankton in the mandovi estuary (Goa, India) during monsoon season: Chemotaxonomy. J. Water Resour. Prot. 5, 349–361. doi: 10.4236/jwarp.2013.53A036
Park C., Lee P. G., Yang S. R. (2002). Variation of zooplankton distribution in the seomjin river estuary with respect to season and salinity gradients. Sea. 7 (2), 51–59.
Parsons T. R., Maita Y., Lalli C. M. (1984). A manual of chemical and biological methods for seawater analysis (Oxford: Pergamon Press), 173.
Poulet S. A. (1978). Comparison between five coexisting species of marine copepods feeding on naturally occurring particulate matter. Limnol. Oceanogr. 23 (6), 1126–1143. doi: 10.4319/lo.1978.23.6.1126
Primo A. L., Azeiteiro U. M., Marques S. C., Martinho F., Pardal M.Â. (2009). Changes in zooplankton diversity and distribution pattern under varying precipitation regimes in a southern temperate estuary. Estuar. Coast. Shelf. Sci. 82 (2), 341–347. doi: 10.1016/j.ecss.2009.01.019
R Core Team (2020). R: A language and environment for statistical computing (Vienna, Austria: R Foundation for Statistical Computing). Available at: https://www.R-project.org/.
Roman M. R. (1984). Utilization of detritus by the copepod, acartia tonsa 1. Limnol. Oceanogr. 29 (5), 949–959. doi: 10.4319/lo.1984.29.5.0949
Sahu B. K., Begum M., Khadanga M. K., Jha D. K., Vinithkumar N. V., Kirubagaran R. (2013). Evaluation of significant sources influencing the variation of physico-chemical parameters in port Blair bay, south Andaman, India by using multivariate statistics. Mar. Pollut. Bull. 66 (1-2), 246–251. doi: 10.1016/j.marpolbul.2012.09.021
Saiz E., Kiørboe T. (1995). Predatory and suspension feeding of the copepod acartia tonsa in turbulent environments. Mar. Ecol. Prog. Ser. 122, 147–158. doi: 10.3354/meps122147
Shaha D. C., Cho Y. K. (2009). Comparison of empirical models with intensively observed data for prediction of salt intrusion in the Sumjin River estuary, Korea. Hydrol. Earth Syst. Sci., 13 (6), 923–933. doi: 10.5194/hess-13-923-2009
Shen X., Cai Y., Su M., Wan H., Shen Y., Yang Z. (2022). High discharge intensified low net ecosystem productivity, hypoxia, and acidification at three outlets of the pearl river estuary, China. Water Res. 214, 118171. doi: 10.1016/j.watres.2022.118171
Shim J. H., Yun K. H. (1990). Seasonal variation and production of zooplankton in chonsu bay, Korea. Korea. J. Oceanol. Soc 25 (4), 229–239.
Shropshire T. A., Morey S. L., Chassignet E. P., Bozec A., Coles V. J., Landry M. R., et al. (2020). Quantifying spatiotemporal variability in zooplankton dynamics in the gulf of Mexico with a physical–biogeochemical model. Biogeosciences 17 (13), 3385–3407. doi: 10.5194/bg-17-3385-2020
Soh H. Y. (2010). Invertebrate fauna of Korea: Volume 21, number 3, marine planktonic copepods (Korea: National Institute of Biological Resources), 1–197 pp.
Soh H. Y., Moon S. Y. (2014). Invertebrate fauna of Korea: Volume 34, number 28, marine planktonic copepods (Korea: National Institute of Biological Resources), 1–138 pp.
Soh H. Y., Moon S. Y., Wi J. H. (2013). Invertebrate fauna of Korea: Volume 21, number 28, marine planktonic copepods (Korea: National Institute of Biological Resources), 1–157 pp.
Soh H. Y., Suh H. L. (1993). Seasonal fluctuation of the abundance of the planktonic copepods in kwangyang bay. Korean J. Environ. Biol. 11 (1), 26–34.
Suh H. L., Soh H. Y., Cha S. S. (1991). Salinity and distribution of zooplankton in the estuarine system of mankyong river and dongjin river. Korean J. Environ. Biol. 26, 181–192.
Telesh I. V. (2004). Plankton of the Baltic estuarine ecosystems with emphasis on Neva estuary: a review of present knowledge and research perspectives. Mar. pollut. Bull. 49 (3), 206–219. doi: 10.1016/j.marpolbul.2004.02.009
Thompson G. A., Dinofrio E. O., Alder V. A. (2013). Structure, abundance and biomass size spectra of copepods and other zooplankton communities in upper waters of the southwestern Atlantic ocean during summer. J. Plankton. Res. 35 (3), 610–629. doi: 10.1093/plankt/fbt014
Tiselius P., Saiz E., Kiørboe T. (2013). Sensory capabilities and food capture of two small copepods, paracalanus parvus and pseudocalanus sp. Limnol. Oceanogr. 58 (5), 1657–1666. doi: 10.4319/lo.2013.58.5.1657
Tomita M., Shiga N., Ikeda T. (2003). Seasonal occurrence and vertical distribution of appendicularians in toyama bay, southern Japan Sea. J. Plankton Res. 25 (6), 579–589. doi: 10.1093/plankt/25.6.579
Wang W., Yu Z., Song X., Chi L., Zhou P., Wu Z., et al. (2022). Hypoxia formation in the East China Sea by decomposed organic matter in the kuroshio subsurface water. Mar. Pollut. Bull. 177, 113486. doi: 10.1016/j.marpolbul.2022.113486
White J. R., Dagg M. J. (1989). Effects of suspended sediments on egg production of the calanoid copepod acartia tonsa. Mar. Biol. 102 (3), 315–319. doi: 10.1007/BF00428483
Williams A. K., McInnes A. S., Rooker J. R., Quigg A. (2015). Changes in microbial plankton assemblages induced by mesoscale oceanographic features in the northern gulf of Mexico. PloS One 10 (9), e0138230. doi: 10.1371/journal.pone.0138230
WoRMS Editorial Board (2022) World register of marine species (VLIZ). Available at: https://www.marinespecies.org (Accessed 2022-03-30).
Keywords: environmental factor, suspended particulate matter, statistical analysis, zooplankton community, feeding habit structure, Yeosu Bay
Citation: Jeong YS, Choo S and Soh HY (2022) Influence of rainfall events on zooplankton community characteristics and feeding habits in estuarine–coastal environments. Front. Mar. Sci. 9:950695. doi: 10.3389/fmars.2022.950695
Received: 23 May 2022; Accepted: 27 June 2022;
Published: 28 July 2022.
Edited by:
Ganesh Thiruchitrambalam, Pondicherry University, Port Blair Campus, IndiaReviewed by:
Gopalakrishnan Thilagam, Pachaiyappa's College for MenRajamanickam Krishnamurthy, Arignar Anna Government Arts and Science College Chennai, India
Vikas Pandey, National Institute of Ocean Technology, India
Copyright © 2022 Jeong, Choo and Soh. This is an open-access article distributed under the terms of the Creative Commons Attribution License (CC BY). The use, distribution or reproduction in other forums is permitted, provided the original author(s) and the copyright owner(s) are credited and that the original publication in this journal is cited, in accordance with accepted academic practice. No use, distribution or reproduction is permitted which does not comply with these terms.
*Correspondence: Ho Young Soh, hysoh@chonnam.ac.kr
†These authors have contributed equally to this work and share first authorship