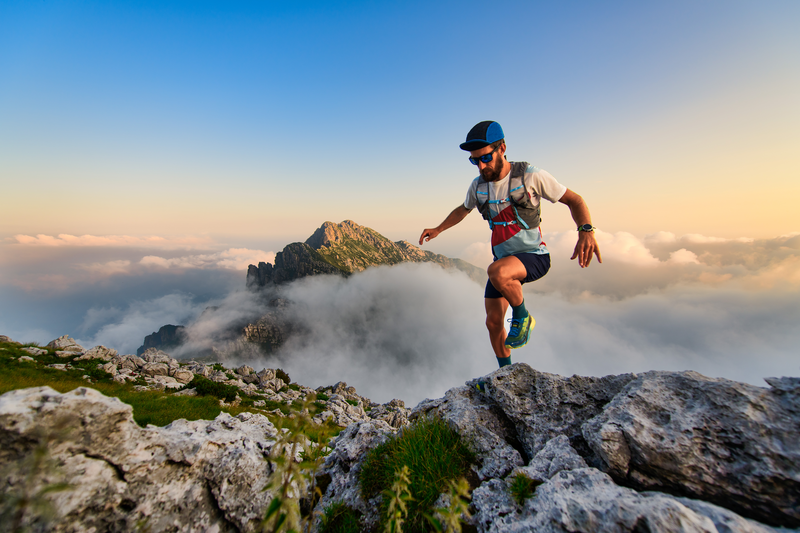
95% of researchers rate our articles as excellent or good
Learn more about the work of our research integrity team to safeguard the quality of each article we publish.
Find out more
ORIGINAL RESEARCH article
Front. Mar. Sci. , 09 August 2022
Sec. Marine Biology
Volume 9 - 2022 | https://doi.org/10.3389/fmars.2022.949233
The upside-down jellyfish Cassiopea increasingly occurs in many (sub-) tropical coastal habitats such as mangrove forests, seagrass meadows, and coral reefs. Its mixotrophic lifestyle and ecophysiological plasticity as well as a high regenerative capacity may be reasons for its success. While the regeneration of umbrella tissue and body structures (i.e. rhopalia and oral arms) was already demonstrated, it remains unclear whether a fully functioning medusa can regenerate from only umbrella tissue. In this study, we thus investigated the regeneration of umbrella fragments over time. We conducted a laboratory experiment for which we used 18 Cassiopea medusae of three different size classes that were cut into two pieces each, one fragment with oral arms and one without. Over a total observation period of 5 weeks, we regularly monitored survival, pulsation behavior, growth and the regeneration pattern of fragments. Findings revealed that 100% of the fragments with oral arms and 88% of the fragments without oral arms survived. Pulsation behavior occurred in all fragments and lasted until the end of the experiment in 94% of all fragments. The umbrella area of fragments without oral arms showed a significantly higher decrease in the first two weeks compared to fragments with oral arms. A complete regeneration of umbrella tissue was observed in all fragments, with and without oral arms alike, and 50% of all fragments even regenerated rhopalia or oral arms as body structures after 33 days. These results suggest an outstanding regenerative capacity of Cassiopea jellyfish after fragmentation. This may contribute to (i) explain the currently observed success of upside-down jellyfish and (ii) extend our knowledge about its regeneration process, which might even act as an asexual reproduction mode in Cassiopea.
The ability to replace lost body parts, which is generally defined as regeneration, is widespread in the animal kingdom (Bely and Nyberg, 2010). Among metazoans, the extent of regeneration is highly variable occurring at different life stages and levels of biological organisation (Alvarado, 2000; Bely and Nyberg, 2010). Animals with high regenerative capacities like planarians for example can regenerate their complete body out of small tissue pieces (Rink, 2012), while other animals like newts are only capable of regenerating specific body parts such as limbs (Kintner and Brockes, 1984).
In the phylum Cnidaria, regeneration occurs both during the polyp and medusa stage (Galliot and Schmid, 2002; Agata and Inoue, 2012; Fujita et al., 2021). The ability to reaggregate and regenerate from dissociated tissue fragments is present in all polyp species as well as in numerous medusa types (Galliot and Schmid, 2002). Especially in the Hydrozoa class, whole body regeneration and regeneration of multiple structures is common during both stages (Fujisawa, 2003; Fujita et al., 2019; Fujita et al., 2021). Scyphozoa polyps can regenerate their complete body after strobilation or after sustaining an injury (Steinberg, 1963; Werner, 1967; Neumann, 1977; Hofmann et al., 1996; Kroiher et al., 2000). Among the scyphozoan medusae, different regenerative capacities were reported (Abrams et al., 2015; Gamero-Mora et al., 2019).
An exceptionally high regenerative capacity was observed for the jellyfish Cassiopea (Zeleny, 1907; Stockard, 1910; Cary, 1916; Gamero-Mora et al., 2019). These jellyfish exhibit a unique benthic lifestyle (Bigelow, 1900), which gave them their colloquial name the “upside-down jellyfish”, and live in a close symbiotic relationship with photosynthetic dinoflagellates from the family Symbiodiniaceae (Thornhill et al., 2006; Lampert, 2016; LaJeunesse et al., 2018). They occur cosmopolitan in both tropical and subtropical coastal ecosystems (Morandini et al., 2017; Ohdera et al., 2018). The polyps of Cassiopea can regenerate their functional and radially symmetrical structures from fragments (Curtis and Cowden, 1972). Several studies demonstrated that also the umbrella tissue of medusae (Stockard, 1910; Cary, 1916), and even body structures such as oral arms and oral appendages (Zeleny, 1907; Gamero-Mora et al., 2019), had the potential to regenerate after injuries. The regeneration was accelerated with a greater extent of the injury (Zeleny, 1907) and the presence of sense organs (Cary, 1916). In addition, Stockard (1910) observed a decreasing body size during regeneration due to the increased energy demand. In contrast to Cassiopea, the moon jellyfish Aurelia aurita can rearrange its existing body structures to regain body symmetry instead of regenerating it (Abrams et al., 2015).
Despite several studies already reporting an exceptionally high regenerative capacity of Cassiopea (Zeleny, 1907; Stockard, 1910; Cary, 1916; Gamero-Mora et al., 2019), Gamero-Mora et al. (2019) and Fujita et al. (2021) even proposed that a fully functioning medusa could regenerate from just umbrella tissue. Our observations after a previous laboratory accident supported this proposal leading to our experiment where we wanted to assess (i) whether umbrella tissue could regenerate all body structures and thus enable whole body regeneration. Further, we aimed to examine (ii) the effect of medusa size on regeneration. We hypothesised that a whole-body regeneration may be possible, depending on the size of the umbrella tissue hosting the endosymbionts and the presence of oral arms enabling a mixotrophic feeding strategy to meet the increased energy demand (Stockard, 1910).
To address our hypotheses, 18 medusae of three different size classes were cut into two fragments each, one with oral arms and one without. We then monitored survival, pulsation behavior, growth and regeneration pattern in form of medusa development over a five-week period.
In total, 18 medusae were selected for fragmentation and 6 medusae were assigned to the control group. All 24 jellyfish were raised from the polyp stage under laboratory conditions. According to their umbrella diameter, all jellyfish were classified into three size classes and one control group: small (3.5 – 5.9 cm), medium (6.0 – 7.9 cm), large (8.0 – 11.1 cm) and the control group (2.4 – 8.1 cm), each containing six medusae. From each of the 18 medusae a triangular was cut out of the umbrella without damaging the oral arms using a scalpel thus generating a total of 36 fragments (Figure 1). There was no specific axis area selected for the cutting of the triangular shaped piece. Half of the triangular shaped pieces consisted only of umbrella tissue (UT-fragment), whereas the other half still had the oral region including oral arms attached (OA-fragment). Overall, seven groups were created that were differentiated by size and the presence or absence of oral arms (UT-small, UT-medium, UT-large, OA-small, OA-medium, OA-large, Control). All generated fragments and controls were distributed into six aquaria (Figure 1). Each aquarium contained OA- and UT-fragments of each size class as well as one control. All aquaria were kept at similar conditions (salinity: 37.0 ± 2.0‰, temperature: 26.0 ± 1.5°C, 12:12 h light/dark cycle with ~217 µmol m-2 s-1) during the experiment period of 33 days. The illuminance was measured in lux and converted to photosynthetically active radiation (PAR, μmol m-2 s-1) using a conversion factor of 52.0 (Tilstra et al., 2018). Twice a week all jellyfish with oral arms (OA-fragments and controls) were fed with freshly hatched Artemia nauplii. Each aquarium was sub-separated into three areas using perforated plexiglass to ensure equal water conditions in all parts and to help with the identification of the individual fragments. Weekly water exchanges of 10% ensured stable water parameters.
Figure 1 Experimental Design. Outline of the experimental design representing the distribution of the seven different groups (UT-small, UT-medium, UT-large, OA-small, OA-medium, OA-large, Control) within one aquarium. The fragment type is indicated by color (Oral Arm [OA] - fragment = red, Umbrella Tissue [UT] - fragment = blue, Control = white). Inside the aquarium two perforated plexiglass panels, indicated by black vertical lines, were used to better identify the fragments. In total, six aquaria were replicated using this design.
Overall survival was calculated in percentage for the different groups and the different fragment types. Fragments were classified as dead if no pulsation behavior was detectable and disintegration of the umbrella tissue became visible.
The average pulsation rate (in bpm) of every fragment, OA and UT likewise, was determined three times a week by counting the number of pulsations in one minute, which was repeated three times in a row and then averaged. A complete pulsation cycle (one contraction and the following relaxation of the umbrella tissue to the maximum extension) was only counted when the contraction was visible throughout the whole body. The weekly change in the average pulsation rate (CPR in % * d-1) was calculated for each fragment as follows:
where Pfinal is the average pulsation rate (in bpm) at the end and Pinitial the average pulsation rate at the beginning of the respective week and Δt is the interval in days.
The aboral umbrella area (in cm2) was determined from the weekly photographs using the polygon tracing function in ImageJ version 1.53e. For this, a photograph of each medusae with the largest expansion during one pulsation cycle was chosen. The weekly specific growth rates (SGR per day) were calculated using the following formula:
where Afinal and Ainitial are the final and initial average umbrella area (in cm2) of the respective week and Δt is the growth interval in days. SGR is expressed in cm2 of umbrella area per cm2 of umbrella area per day, which can be simplified as d-1 (Wijgerde et al., 2012; Tilstra et al., 2017).
To determine the development of the fragments, all fragments and controls were visually analyzed and a photo series from the aboral site was taken for a complete pulsation cycle once per week. Each fragment was then classified into a regeneration class according to the criteria in Table 1 and the associated calculation for the percentage of rebuild body structures in the Supplementary Material. Because the controls did not participate in the regeneration, a separate class was defined.
Table 1 Regeneration Classes. A total of 9 regeneration classes were defined depending on pulsation behavior, umbrella growth and development of body structures.
The statistical analyses were performed using R (v.4.0.5; R Development Core Team, 2021) with Rstudio (v.1.1.463; RStudio Team, 2016) and the car (v.3.0-10; Fox and Weisberg, 2019), the multcomp (v.1.4-16; Hothorn et al., 2008), the rstatix (v.0.7.0; Kassambara, 2021), the ggpubr (v.0.4.0; Kassambara, 2020) and the tidyverse (v. 1.3.0; Wickham et al., 2019) packages. To analyze differences in the initial and final regeneration classes for each group, a paired Wilcoxon signed rank test with continuity correction was applied. Dead fragments were excluded from this analysis beforehand. Weekly CPR were compared for each week between the seven groups using a single factorial variance analysis (ANOVA). A post hoc multiple comparison t-test with Bonferroni adjusted p-values was carried out afterwards. The required normal distribution for the ANOVA was checked by visual inspection of residual plots and variance homogeneity was checked with the Levene’s test (p > 0.05). Since these assumptions did not apply for the weekly SGR, a Kruskal-Wallis test was conducted to compare the SGR of the different groups in each week. A post hoc multiple comparison Wilcoxon rank sum test with adjusted p-values by the Benjamini and Hochberg method was carried out afterwards. The results were considered significant below a p-value of 0.05.
Two fragments from a total of 36 fragments died on the last day of the experiment (33 days after fragmentation) resulting in an overall fragment survival of 94.4%. The affected fragments belonged to the UT-medium and UT-large group. Thus, the survival in the UT-fragment group was 88.8% while the survival was 100.0% in the OA-fragment group.
Pulsation behavior occurred in all fragments and lasted until the end except for two fragments that died after 33 days of the experiment. The CPR were differentiated into groups and weeks (Figure 2). A significant effect of the factor “group” was only found in the first week (ANOVA, F = 4.66, p = 0.005). In this week, significantly higher CPR were found in the OA-small compared to the UT-small (multiple comparison t-test, p = 0.044), the UT-medium (multiple comparison t-test, p = 0.046) and the UT-large (multiple comparison t-test, p = 0.001) group.
Figure 2 Weekly change in pulsation rate (CPR) for the different groups (n = 6 for all groups). Control (CT), oral arm (OA) - and umbrella tissue (UT) - fragments are indicated by white, red and blue color, respectively. The data is presented as boxplots including the median (black line), the mean value (black cross) and outliers (black dots). Significant differences between groups according to a multiple comparison t-test with Bonferroni adjusted p-values are indicated by asterisks (*p< 0.05, **p< 0.01).
The SGR were analyzed weekly for each group (Figure 3). A significant effect of the factor “group” was found in the first (Kruskal-Wallis, X2 = 27.96, p< 0.001), the second (Kruskal-Wallis, X2 = 27.97,p < 0.001) and the third (Kruskal-Wallis, X2 = 13.51, p = 0.036) week. In the first week, significantly higher SGR were determined in the control and in the OA-small and OA-large group compared to all UT-groups (multiple comparison Wilcoxon rank sum test, p < 0.05). In the second week, significantly higher SGR were also detected in the control and the OA-small group compared to all UT-groups (multiple comparison Wilcoxon rank sum test, p < 0.05). Contrary to the results of the Kruskal-Wallis test for the third week, no significant differences between the groups were found according to the Wilcoxon rank sum post hoc test.
Figure 3 Weekly specific growth rate (SGR) for the different groups (n = 5 for the UT-medium and the UT-large group in week 5; n = 6 for the other groups). Control (CT), oral arm (OA) - and umbrella tissue (UT) - fragments are indicated by white, red and blue color, respectively. The data is presented as boxplots including the median (black line), the mean value (black cross) and outliers (black dots). Different letters indicate significant differences between the groups in the respective week according to a multiple comparison Wilcoxon rank sum test with adjusted p-values by the Benjamin and Hochberg method (p< 0.05).
All fragments were placed into regeneration class 2 after fragmentation because they remained actively pulsating and alive. Within 12 days after fragmentation the first signs of regeneration of umbrella tissue became visible. At the site of injury, new tissue which was brightly coloured developed. Furthermore, the umbrella shapes became more spherical in 31 fragments whilst the other 5 fragments were already spherical. After 19 days, 4 of the UT-fragments developed oral arms with oral funnels while the first UT-fragments regenerated rhopalia two weeks later. Compared to the UT-group, the regeneration of rhopalia was already observed after 19 days in 3 OA-fragments. At the end of the experiment, 18 fragments (10 OA- and 8 UT-fragments) had regenerated rhopalia and oral arms as new body structures and were therefore placed into regeneration classes 5 to 7, depending on the number of regenerated body structures. As an illustration of the regeneration process, a time series was created for a UT-fragment (Figure 4).
Figure 4 Development of an umbrella tissue (UT)-fragment. This timeseries shows the development of a fragment that only consisted of umbrella tissue. The documentation of the development continued after the official end of the experiment. The first picture (A) displayed the situation on the day of fragmentation. The following pictures were made (B) 5 days, (C) 12 days, (D) 19 days, (E) 26 days, (F) 33 days after fragmentation during the experiment and (G) 40 days, (H) 47 days, (I) 54 days, (J) 2 months, (K) 3 months and (L) 10 months after fragmentation outside the official experiment period. All pictures were taken on graph paper (1 mm x 1 mm).
Significant differences between the initial and final regeneration class were found in the OA-small (paired Wilcoxon, p = 0.036), OA-medium (paired Wilcoxon, p = 0.034), OA-large (paired Wilcoxon, p = 0.035) and UT-small (paired Wilcoxon, p = 0.034) group (Figures 5A–D). In contrast, no differences were found in the UT-medium (paired Wilcoxon, p = 0.169) and UT-large (paired Wilcoxon, p = 0.169) group (Figures 5E, F).
Figure 5 Regeneration classes during the experiment distributed into groups (A) oral arm (OA)-small, (B) OA-medium, (C) OA-large, (D) umbrella tissue (UT)-small, (E) UT-medium and (F) UT-large (n = 5 for the UT-medium and the UT-large group; n = 6 for the other groups). OA- and UT-fragments are indicated by red and blue colour, respectively. The control class is excluded since this group did not participate in regeneration. All data are shown as mean ± SE. Asterisks indicate significant differences between the initial and final regeneration class (*p< 0.05). The respective criteria for the different regeneration classes are shown in Table 1.
Several studies have already demonstrated a high regenerative capacity of the upside-down jellyfish Cassiopea (Zeleny, 1907; Stockard, 1910; Cary, 1916; Gamero-Mora et al., 2019). Linked to these previous findings, we could now demonstrate that a fully functioning medusa can regenerate from just umbrella tissue with no differences between size classes and fragment types.
During the experiment, all fragments maintained their pulsation behavior except for two fragments that died at the end of the experiment. Significantly different CPR between groups were only detected in the first week where a higher CPR was determined in the OA-large group compared to the different UT-groups. This could be related to the fact that the UT-groups had less rhopalia and umbrella tissue after fragmentation in which the pulsation was initiated, especially in comparison to the OA-large group (Mayer, 1908; Cary, 1916). In the following four weeks, no further differences in CPR were observed, presumably because the pulsation rate in the UT-groups only declined in the first week due to the fragmentation and remained the same afterwards. From the second week onwards, an average increase in the pulsation rate was observed in all groups. Nonetheless, no significant differences of the CPR were found between the control and all other groups (Figure 2). Therefore, this effect cannot be attributed to regeneration but was likely due to overall tank effects or an insufficient acclimatization phase that affected all groups. In the small tanks, the dissolved organic carbon (DOC) content was likely higher compared to larger tanks in which the jellyfish were previously kept which may lead to increased pulsation rates (Tilstra et al., 2022). Therefore, DOC measurements should be included in subsequent experiments.
Similar to the CPR, significant differences of the SGR between the groups were determined in the first and additionally the second week. In these weeks, a significant difference between the control and all UT-fragment groups was found (Figure 3) but not between control and OA-fragment groups. The UT-fragments were not fed during the experiment in comparison to the control and OA-fragment due to the absence of oral arms and oral funnels. Therefore, the UT-fragments were deprived from heterotrophic nutrition. Stockard (1910) could also demonstrate that unfed medusae decreased in size which was exacerbated in regenerating individuals. From the third week onwards, presumably no more differences were detected, as the UT-groups had reached a small size that could be sustained with only an autotrophic diet (Verde and McCloskey, 1998). Despite the overall regeneration of umbrella tissue which was visible in all groups, no significant growth was observed within the OA-fragment group. The high energy demand during regeneration may have caused old tissue to be consumed (Stockard, 1910) while new tissue was regenerated presumably resulting in no growth. In addition, all OA-fragments may have been underfed due to a low availability of heterotrophic food sources and therefore the increased energy demand may not have been fulfilled.
Overall, a very high survival of 100% for the OA-fragment and 88.8% for the UT-fragment group was reached during the experiment demonstrating a high tolerance of Cassiopea sp. medusae against physical damages. Because only two fragments died in total (one of which belonged to the UT-medium and UT-large group, respectively), no significant differences in the survival were found between the groups. Fragments from the UT-fragment group could have been more susceptible since only an autotrophic feeding mode was possible and these had to regenerate larger parts of their body compared to OA-fragments. Consistent with our findings, Stockard (1910) demonstrated that medusae which needed to regenerate larger parts decreased more in body size and became emaciated because energy for regeneration is drawn from the old body tissue. Eventually, the UT-fragments could have consumed themselves causing their own death.
Significant differences between the initial and final regeneration class were found in all OA-fragment groups (small, medium and large) and the UT-small group (Figure 5). In contrast, no significant differences were detected in the UT-large and UT-medium group although the dead fragments were excluded beforehand. This could be related to the aforementioned fact that these fragments had to regenerate larger parts and presumably became more emaciated than smaller fragments or fragments with oral arms (Stockard, 1910). As a result, the regeneration may have proceeded more slowly in these two groups.
Although no differences were detected in the UT-large and UT-medium group, regeneration of umbrella tissue was observed for all fragments and groups after 12 days. New umbrella tissue formed at the site of the injury and was brighter than the previously existing tissue. The brighter color is likely due to the absence of endosymbionts in the early development stage of the umbrella tissue (Oswald et al., 2007). Indeed, tissue became visually darker during the experiment, suggesting colonization with endosymbionts. This may be an important process for the fragment as its energy requirement is dependent on the close coupling of the host and endosymbiont (Freeman et al., 2016; Freeman et al., 2017). In general, the ability to regenerate umbrella tissue after fragmentation is well-known for medusae of Cassiopea (Stockard, 1910; Cary, 1916). Additionally, regeneration of body structures like rhopalia and oral arms was observed within all UT- and OA-groups. The regeneration of a new oral region containing oral arms from umbrella tissue was already described in previous studies after an injury at the margin of the umbrella (Gamero-Mora et al., 2019) and after removal of the oral region (Zeleny, 1907). Unlike these previous studies, we could now demonstrate that UT-fragments can combine these regenerative capacities to become spherical again by regenerating umbrella tissue and develop new body structures to become a fully functional medusa. This whole-body regeneration could be based on a trans-differentiation potential of striated muscle cells in the umbrella tissue already observed in the order Anthomedusae suggesting a complete regeneration of body structures (Schmid, 1992; Schmid & Reber-Müller, 1995). Nevertheless, it needs to be further investigated, whether the same process takes place in Cassiopea medusae. Furthermore, a sequence in the regeneration process was determined. As a first step the fragments became more spherical and afterwards regenerated new body structures. This sequence may potentially be correlated to the importance of radial symmetry for movement via propulsion (Abrams et al., 2015) and likely also for a complete and homogenous pulsation process. Therefore, regaining radial symmetry may be an important step in enabling further regeneration of body structures (Fujita et al., 2021).
Overall, this observed regenerative capacity of Cassiopea sp. is comparable to the regenerative capacity of the medusae of Clytia hemisphaerica and Campanularia johnstoni, both belonging to the Hydrozoa class (Schmid, 1974; Sinigaglia et al., 2020). In these species, it was demonstrated that a fully functioning medusa can regenerate from just umbrella tissue. Similar to our findings, it was further found that the fragments of C. hemisphaerica first become spherical before the regeneration of body structures occurs (Sinigaglia et al., 2020). Nevertheless, within the Scyphozoa class, to which Cassiopea belongs, no comparative regenerative capacity was yet observed. In the Scyphozoan jellyfish A. aurita only a rearrangement of existing body structures without regeneration was demonstrated (Abrams et al., 2015).
Our data suggest that whole body regeneration of Cassiopea sp.’ umbrella tissue could have a great ecological relevance. Together with the ecophysiological plasticity and very high resilience of Cassiopea medusae to changing environmental factors (Mayer, 1916; Aljbour et al., 2017; Banha et al., 2020; Mammone et al., 2021; Muffett et al., 2022; Tilstra et al., 2022), this high regenerative capacity could have further contributed to the recent invasive success of Cassiopea (Ohdera et al., 2018; Stampar et al., 2020). As injuries are very common in benthic invertebrates (Lindsay, 2010), the high regenerative capacity of Cassiopea could lead to the propagation of originally fragmented umbrella pieces. These injuries may be especially related to extreme environmental events such as storms or anthropogenic activities like bottom trawling (Lindsay, 2010). Nonetheless, it would require further investigation into the extent to which umbrella tissue is fragmented from Cassiopea medusae in their natural environment to identify the consequences.
Our findings also support the proposal of Cassiopea as a model organism to uncover mechanisms underlying tissue regeneration (Fujita et al., 2021). Overall, our results give rise to many new questions that should be addressed in further experiments. The most important of which is to assess the in-situ relevance of this regenerative capacity, e.g. can medusae of Cassiopea actively release umbrella tissue to reproduce asexually.
Altogether, we demonstrate that a fully functioning medusa of Cassiopea sp. can regenerate from only umbrella tissue with no significant differences between size classes and fragment types exceeding the current knowledge. We further show that Cassiopea sp. has a high tolerance against physical damage caused by fragmentation. In addition, our findings suggest that regeneration does not affect pulsation behavior but growth due to the increased energy demand. Fragments without oral arms may be especially affected because of their exclusive reliance on autotrophic nutrition. Fragments that need to regenerate larger body parts may even regenerate slower and become more emaciated due to the increased energy demand during regeneration.
In combination with its mixotrophic lifestyle and ecophysiological plasticity (Aljbour et al., 2017; Rädecker et al., 2017; Ohdera et al., 2018; Banha et al., 2020; Stampar et al., 2020; Mammone et al., 2021), this observed regenerative capacity could be an additional tool explaining the invasive success of Cassiopea. Since our results show that Cassiopea sp. has vast regenerative capacities in the umbrella tissue, it may further serve as a potent model organism in the quest to decipher cellular mechanisms that enable regeneration, as was also proposed by Fujita et al. (2021).
The original contributions presented in the study are included in the article/Supplementary Material. Further inquiries can be directed to the corresponding authors.
JP conceptualized the experiment. MO, JP, and JF designed and conducted the experiment. MO, JP, and JF analyzed the samples and the data. MO and JP wrote the manuscript. All authors contributed to the article and approved the submitted version.
A special thanks to Maxi Wenge, who helped us with the data collection. We would also like to thank the Ruhr University Bochum (Germany), Burgers’ Zoo (Arnhem, Netherlands), and the Leibniz Centre for Tropical Marine Research in Bremen (Germany) for providing us with Cassiopea sp. individuals. We also thank the reviewers for their comments which helped to improve the manuscript.
The authors declare that the research was conducted in the absence of any commercial or financial relationships that could be construed as a potential conflict of interest.
All claims expressed in this article are solely those of the authors and do not necessarily represent those of their affiliated organizations, or those of the publisher, the editors and the reviewers. Any product that may be evaluated in this article, or claim that may be made by its manufacturer, is not guaranteed or endorsed by the publisher.
The Supplementary Material for this article can be found online at: https://www.frontiersin.org/articles/10.3389/fmars.2022.949233/full#supplementary-material
Supplementary Table 1 | Calculation Regeneration Class.
Supplementary Table 2 | Regeneration Classes.
Supplementary Table 3 | Average Pulsation Rate.
Supplementary Table 4 | Umbrella Area.
Abrams M. J., Basinger T., Yuan W., Guo C. L., Goentoro L. (2015). Self-repairing symmetry in jellyfish through mechanically driven reorganization. PNAS. 112, E3365–E3373. doi: 10.1073/pnas.1502497112
Agata K., Inoue T. (2012). Survey of the differences between regenerative and non-regenerative animals. Dev. Growth Differ. 54, 143–152. doi: 10.1111/j.1440-169X.2011.01323.x
Aljbour S. M., Zimmer M., Kunzmann A. (2017). Cellular respiration, oxygen consumption, and trade-offs of the jellyfish Cassiopea sp. in response to temperature change. J. Sea Res. 128, 92–97. doi: 10.1016/j.seares.2017.08.006
Alvarado A. S. (2000). Regeneration in the metazoans: why does it happen? BioEssays. 22, 578–590. doi: 10.1002/(SICI)1521-1878(200006)22:6<578::AID-BIES11>3.0.CO;2-%23
Banha T., Mies M., Güth A. Z., Pomory C. M., Sumida P. (2020). Juvenile Cassiopea andromeda medusae are resistant to multiple thermal stress events. Mar. Biol. 167, 1–13. doi: 10.1007/s00227-020-03792-w
Bely A. E., Nyberg K. G. (2010). Evolution of animal regeneration: re-emergence of a field. Trends Ecol. Evolution. 25, 161–170. doi: 10.1016/j.tree.2009.08.005
Bigelow R. P. (1900). “The anatomy and development of Cassiopea xamachana,” in Memoirs of the Boston Society of Natural History 5 (Baltimore, MD:Johns Hopkins Press), 191–236.
Cary L. R. (1916). The influence of the marginal sense organs on metabolic activity in Cassiopea xamachana Bigelow. PNAS 2, 12, 709. doi: 10.1073/pnas.2.12.709
Curtis S. K., Cowden R. R. (1972). Regenerative capacities of the scyphistoma of Cassiopea (Phylum Coelenterata; Class Scyphozoa). Acta Embryol. Exp. Suppl, 429–454.
Freeman C. J., Stoner E. W., Easson C. G., Matterson K. O., Baker D. M. (2016). Symbiont carbon and nitrogen assimilation in the Cassiopea–Symbiodinium mutualism. Mar. Ecol. Prog. Ser. 544, 281–286. doi: 10.3354/meps11605
Freeman C. J., Stoner E. W., Easson C. G., Matterson K. O., Baker D. M. (2017). Variation in δ13C and δ15N values suggests a coupling of host and ymbiont metabolism in the Symbiodinium–Cassiopea mutualism. Mar. Ecol. Prog. Ser. 571, 245–251. doi: 10.3354/meps12138
Fujisawa T. (2003). Hydra regeneration and epitheliopeptides. Dev. Dyn. 226, 182–189. doi: 10.1002/dvdy.10221
Fujita S., Kuranaga E., Nakajima Y. (2019). Cell proliferation controls body size growth, tentacle morphogenesis, and regeneration in hydrozoan jellyfish Cladonema pacificum. 7 PeerJ, e7579. doi: 10.7717/peerj.7579
Fujita S., Kuranaga E., Nakajima Y. (2021). Regeneration potential of jellyfish: cellular mechanisms and molecular insights. Genes 12, 758. doi: 10.3390/genes12050758
Galliot B., Schmid V. (2002). Cnidarians as a model system for understanding evolution and regeneration. Int. J. Dev. Biol. 46, 39–48.
Gamero-Mora E., Halbauer R., Bartsch V., Stampar S. N., Morandini A. C. (2019). Regenerative capacity of the upside-down jellyfish Cassiopea xamachana. Zool. Stud. 58, e37. doi: 10.6620/ZS.2019.58-37
Hofmann D. K., Fitt W. K., Fleck J. (1996). Checkpoints in the life-cycle of Cassiopea spp.: control of metagenesis and metamorphosis in a tropical jellyfish. Int. J. Dev. Biol. 40, 331–338.
Hothorn T., Bretz F., Westfall P. (2008). Simultaneous inference in general parametric models. Biometrical J. 50, 346–363. doi: 10.1002/bimj.200810425
Kassambara A. (2021). Rstatix: pipe-friendly framework for basic statistical tests. r package version 0.7.0.
Kintner C. R., Brockes J. P. (1984). Monoclonal antibodies identify blastemal cells derived from dedifferentiating muscle in newt limb regeneration. Nature. 308, 67–69. doi: 10.1038/308067a0
Kroiher M., Siefker B., Berking S. (2000). Induction of segmentation in polyps of Aurelia aurita (Scyphozoa, Cnidaria) into medusae and formation of mirror-image medusa anlagen. Int. J. Dev. Biol. 44, 485–490.
LaJeunesse T. C., Parkinson J. E., Gabrielson P. W., Jeong H. J., Reimer J. D., Voolstra C. R., et al. (2018). Systematic revision of Symbiodiniaceae highlights the antiquity and diversity of coral endosymbionts. Curr. Biol. 28, 2570–2580. doi: 10.1016/j.cub.2018.07.008
Lampert K. P. (2016). “Cassiopea and its zooxanthellae”, in The cnidaria, past, present and future. Eds. Goffredo S., Dubinsky Z. (Cham: Springer), 415–423. doi: 10.1007/978-3-319-31305-4_26
Lindsay S. M. (2010). Frequency of injury and the ecology of regeneration in marine benthic invertebrates. Integr. Comp. Biol. 50, 479–493. doi: 10.1093/icb/icq099
Mammone M., Ferrier-Pagés C., Lavorano S., Rizzo L., Piraino S., Rossi S. (2021). High photosynthetic plasticity may reinforce invasiveness of upside-down zooxanthellate jellyfish in Mediterranean coastal waters. PloS One 16, e0248814i. doi: 10.1371/journal.pone.0248814
Mayer A. G. (1916). Nerve conduction, and other reactions in Cassiopea. Am. J. Physiol. 39, 375–393. doi: 10.1152/ajplegacy.1916.39.4.375
Morandini A., Stampar S., Maronna M., Da Silveria F. (2017). All non-indigenous species were introduced recently? The case study of Cassiopea (Cnidaria: Scyphozoa) in Brazilian waters. J. Mar. Biolog. Assoc. U.K. 97, 2, 321–2, 328. doi: 10.1017/S002531541600400
Muffett K. M., Aulgur J., Miglietta M. P. (2022). Impacts of light and food availability on early development of Cassiopea medusae. Front. Mar. Sci. 8. doi: 10.3389/fmars.2021.783876
Neumann R. (1977). Polyp morphogenesis in a scyphozoan: evidence for a head inhibitor from the presumptive foot end in vegetative buds of Cassiopeia andromeda. Wilhelm Roux. Arch. Entw. Mech. Org. 183, 79–83. doi: 10.1007/BF00849035
Ohdera A. H., Abrams M. J., Ames C. L., Baker D. M., Suescún-Bolívar L. P., Collins A. G., et al. (2018). Upside-down but headed in the right direction: review of the highly versatile Cassiopea xamachana system. Front. Ecol. Evol. 6. doi: 10.3389/fevo.2018.00035
Oswald F., Schmitt F., Leutenegger A., Ivanchenko S., D'Angelo C., Salih A., et al. (2007). Contributions of host and symbiont pigments to the coloration of reef corals. FEBS J. 274, 1102–1122. doi: 10.1111/j.1742-4658.2007.05661.x
Rädecker N., Pogoreutz C., Wild C., Voolstra C. R. (2017). Stimulated respiration and net photosynthesis in Cassiopeia sp. during glucose enrichment suggests in hospite CO2 limitation of algal endosymbionts. Front. Mar. Sci. 4. doi: 10.3389/fmars.2017.00267
R Development Core Team (2021). R: a language and environment for statistical computing (Vienna: R Foundation for Statistical Computing).
Rink J. C. (2012). Stem cell systems and regeneration in Planaria. Dev. Genes. Evol. 223, 67–84. doi: 10.1007/s00427-012-0426-4
Schmid V. (1974). Regeneration in medusa buds and medusae of Hydrozoa. Amer. Zool. 14, 773–781. doi: 10.1093/icb/14.2.773
Schmid V. (1992). Transdifferentiation in medusae. Int. Rev. Cytol. 142, 213–261. doi: 10.1016/S0074-7696(08)62077-X
Schmid V., Reber-Müller S. (1995). Transdifferentiation of isolated striated muscle of jellyfish in vitro: the initiation process. Semin. Cell. Biol. 6, 109–116. doi: 10.1006/scel.1995.0016
Sinigaglia C., Peron S., Eichelbrenner J., Chevalier S., Steger J., Barreau C., et al. (2020). Pattern regulation in a regenerating jellyfish. eLife. 9, e54868. doi: 10.7554/eLife.54868
Stampar S. N., Gamero-Mora E., Maronna M. M., Fritscher J. M., Oliveira B. S. P., Sampaio C. L. S., et al. (2020). The puzzling occurrence of the upside-down jellyfish Cassiopea along the Brazilian coast: a result of several invasion events? Zoologia. 37, 1–10. doi: 10.3897/zoologia.37.e50834
Steinberg S. N. (1963). The regeneration of whole polyps from ectodermal fragments of scyphistoma larvae of Aurelia aurita. Biol. Bull. 124, 337–343. doi: 10.2307/1539483
Stockard C. R. (1910). Studies of tissue growth. Arch. Entwick. Org. 29, 15–32. doi: 10.1007/BF02297062
Thornhill D. J., Daniel M. W., LaJeunesse T. C., Schmidt G. W., Fitt W. K. (2006). Natural infections of aposymbiotic Cassiopea xamachana scyphistomae from environmental pools of Symbiodinium. J. Exp. Mar. Biol. Ecol. 338, 50–56. doi: 10.1016/j.jembe.2006.06.032
Tilstra A., El-Khaled Y. C., Meier S., Wild C. (2022). Invasive upside-down jellyfish tolerate organic eutrophication and warming. Bull. Mar. Sci. 98 (0), 000–000. doi: 10.5343/bms.2021.0051
Tilstra A., van Hoytema N., Cardini U., Bednarz V. N., Rix L., Naumann M. S., et al. (2018). Effects of water column mixing and stratification on planktonic primary production and dinitrogen fixation on a northern Red Sea coral reef. Front. Microbiol. 9, 2351. doi: 10.3389/fmicb.2018.02351
Tilstra A., Wijgerde T., Dini-Andreote F., Eriksson B. K., Salles J. F., Pen I., et al. (2017). Light induced intraspecific variability in response to thermal stress in the hard coral Stylophora pistillata. PeerJ. 5, e3802. doi: 10.7717/peerj.3802
Verde E. A., McCloskey L. R. (1998). Production, respiration, and photophysiology of the mangrove jellyfish Cassiopea xamachana symbiotic with zooxanthellae: effect of jellyfish size and season. Mar. Ecol. Prog. Ser. 168, 147–162. doi: 10.3354/meps168147
Werner B. (1967). Stephanoscyphus allman (Scyphozoa Coronatae), ein rezenter Vertreter der Conulata? Palaüont. Z. 41, 137–153. doi: 10.1007/BF02988117
Wickham H., Averick M., Bryan J., Chang W., McGowan L. D., François R., et al. (2019). Welcome to the tidyverse. J. Open Source Software 4, 1686. doi: 10.21105/joss.01686
Wijgerde T., Henkemans P., Osinga R. (2012). Effects of irradiance and light spectrum on growth of the scleractinian coral Galaxea fascicularis — Applicability of LEP and LED lighting to coral aquaculture. Aquaculture. 344-349, 188–193. doi: 10.1016/j.aquaculture.2012.03.025
Keywords: Cassiopea sp., whole body regeneration, fragmentation, umbrella tissue, oral arms, survival, specific growth rate
Citation: Ostendarp M, Plewka J, Flathmann J, Tilstra A, El-Khaled YC and Wild C (2022) Complete and rapid regeneration of fragments from the upside-down jellyfish Cassiopea. Front. Mar. Sci. 9:949233. doi: 10.3389/fmars.2022.949233
Received: 20 May 2022; Accepted: 14 July 2022;
Published: 09 August 2022.
Edited by:
Stelios Katsanevakis, University of the Aegean, GreeceReviewed by:
Marta Mammone, University of Salento, ItalyCopyright © 2022 Ostendarp, Plewka, Flathmann, Tilstra, El-Khaled and Wild. This is an open-access article distributed under the terms of the Creative Commons Attribution License (CC BY). The use, distribution or reproduction in other forums is permitted, provided the original author(s) and the copyright owner(s) are credited and that the original publication in this journal is cited, in accordance with accepted academic practice. No use, distribution or reproduction is permitted which does not comply with these terms.
*Correspondence: Malte Ostendarp, bWFvc0B1bmktYnJlbWVuLmRl; Julia Plewka, anBsZXdrYUB1bmktYnJlbWVuLmRl
†These authors have contributed equally to this work and share first authorship
Disclaimer: All claims expressed in this article are solely those of the authors and do not necessarily represent those of their affiliated organizations, or those of the publisher, the editors and the reviewers. Any product that may be evaluated in this article or claim that may be made by its manufacturer is not guaranteed or endorsed by the publisher.
Research integrity at Frontiers
Learn more about the work of our research integrity team to safeguard the quality of each article we publish.