- 1Department of Integrative Marine Ecology, Zoological Station “Anton Dohrn”, Naples, Italy
- 2National Interuniversity Consortium for Marine Sciences (CoNISMa), Rome, Italy
- 3Department of Biology, University of Bari Aldo Moro, Bari, Italy
- 4Department of Biology, University of Rome “Tor Vergata”, Rome, Italy
- 5Department of Biological and Environmental Sciences and Technologies, University of Salento, Lecce, Italy
- 6Department of Earth and Geoenvironmental Sciences, University of Bari Aldo Moro, Bari, Italy
- 7Environmental Surveys S.r.l. (ENSU), Taranto, Italy
A widespread and diversified mesophotic system of carbonate bioconstructions along the Southern Adriatic and Ionian Italian coasts is described, providing new data on the distribution, structure and associated megabenthic assemblages of mesophotic Mediterranean bioconstructions. The bioconstructions were detected at six different sites off the coasts of Apulia, in presence of marked morphological escarpments, developing on a basal substrate consisting of meso-Cenozoic carbonate rocks. Two biogenic structure types were observed, one mainly built by the nonsymbiotic scleractinians Phyllangia americana mouchezii and Polycyathus muellerae, at depths between approximately 35 and 55 m, and the other by the oyster Neopycnodonte cochlear, at depths from approximately 40 to 70 m. A total of 52 taxa of megabenthic invertebrates, belonging to 6 phyla, were found on the surface of the mesophotic bioconstructions, thus confirming the role of biodiversity hotspots of these carbonate structures. Megabenthic assemblages showed a remarkable heterogeneity both in pattern of species and abundance, probably depending on both the morphological differences of the seabed and the life traits of the single species. Primary bioconstructors seemed to influence the associated community pattern. This peculiar system deserves sound conservation measures in the light of the holistic ecosystem approach for the management of coastal marine areas.
Introduction
Marine bioconstructions are the result of building activities of benthic engineer species which, through the multigenerational accumulation of carbonate skeletons, can construct a three-dimensional biogenic framework that is topographically distinct with respect to the surrounding marine landscape. Bioconstructions are usually long-term formations that can be present in the seascape for millennia. At the same time, they are subjected to highly dynamic processes as a result of the interaction among biological processes, growth, and erosive phases and in turn are influenced by physical and edaphic conditions (substrate type and morphology, predominant currents, etc.) (Ingrosso et al., 2018).
In the Mediterranean Sea, the most common bioconstruction is represented by the coralligenous assemblage, a carbonate structure widespread under moderate light conditions, whose distribution along the Italian coast was recently revised (Ingrosso et al., 2018). According to the literature, calcareous algae are the basic builders of coralligenous outcrops (Laborel, 1961), with the variable contributions of sessile invertebrate calcium carbonate depositors (Ballesteros, 2006; UNEP-MAP-RAC/SPA, 2019). However, with the progressive decrease in light intensity, the input of calcareous algae becomes increasingly negligible, while the contribution of various species of invertebrates increases. Some of these species, due to their capability to produce carbonatic structures, take on the role of main reef builders (Corriero et al., 2019; Cardone et al., 2020), giving rise to specific mesophotic bioconstructions.
Overall, similar to coralligenous formations, mesophotic bioconstructions provide essential ecosystem services, such as support for high habitat complexity and local biodiversity and are a source of recruits for deep but also shallow-water species. Moreover, as the mesophotic zone is more stable than shallow-water environments, with respect to climate change, this zone plays a key role as a refuge for shallow-water species, with relevant conservation implications (Eyal and Pinheiro, 2020). In contrast, mesophotic bioconstructions suffer various anthropogenic threats (for example the presence of anchoring tools and lost fishing gear, accumulation of various types of waste and invasion by alien species), which require a thorough assessment in order to mitigate the present degradation and prevent the future one (Cerrano et al., 2019).
Despite this scenario, most of the current knowledge concerns tropical areas, and very little information is available on the Mediterranean mesophotic zone (Eyal and Pinheiro, 2020; Lesser et al., 2019). Recently, Cerrano et al. (2019) reviewed the scientific literature and proposed the best possible definition of the “temperate mesophotic ecosystem”. In the Mediterranean, this definition encompasses different geomorphological features (Gori et al., 2017), resulting in different local benthic assemblages. They are dominated by various ecosystem engineers (sensu Jones et al., 199411) or habitat-forming species (Bertness and Callaway, 1994; Etnoyer and Morgan, 2005; Cerrano et al., 2006), which may form distinctive “animal forests” (Enrichetti et al., 2018: Chimienti et al., 2020; Enrichetti et al., 2020; Mastrototaro et al., 2020) and true reefs, such as those built by the nonsymbiotic scleractinians Phyllangia americana mouchezii and Polycyathus muellerae (Corriero et al., 2019) and by the deep-sea oyster Neopycnodonte cochlear (Angeletti and Taviani, 2020; Cardone et al., 2020), described along the southeastern Italian coast. These carbonate bioconstructions differ from coralligenous structures in their pattern of structuring species, leading to different morphologies (e.g., general shape, thickness, and cavitation) (Corriero et al., 2019; Cardone et al., 2020) and host a rich and highly diversified zoobenthic fauna, with many species not recorded in the coralligenous biocenoses (Corriero et al., 2019; Giampaoletti et al., 2020). To date, the available data on both the structuring and associated species of these bioconstructions are still fragmented and refer to a few scattered sites, thus preventing the framing of the aforementioned biogenic communities (Corriero et al., 2019; Cardone et al., 2020) within the mesophotic ecosystem.
In the present study, additional mesophotic bioconstructions are described and these, together with those already recorded (Corriero et al., 2019; Angeletti and Taviani, 2020; Cardone et al., 2020), contribute to the definition of a true mesophotic reef system along the southeastern Italian coast. To achieve this general objective, a multiscale approach that combines marine biology and geology methods was employed, with the final aim of conducting the following:
i. developing a definition of the geographical distribution and extension of the mesophotic bioconstructions, upgrading the map of the Apulian coast;
ii. investigating seabed morphology and bathymetry to describe the geomorphological features of the substrates where the bioconstructions occur and to infer possible causes of local geographical differences;
iii. iii.characterizing the benthic fauna to identify the colonization patterns of the different bioconstructions, both in terms of primary bioconstructors and associated megabenthos.
Material and methods
The research was carried out in six areas along the Apulian coast, where preliminary surveys (Tremiti Islands – TRM; Capitolo – CAP and San Foca – SFC) and published author’s data (Monopoli – MON; Otranto - OTR and Santa Maria di Leuca – SML; (Corriero et al., 2019; Cardone et al., 2020) indicated the occurrence of mesophotic bioconstructions (Figure 1A). In all the areas, the following investigations were carried out: i) in situ estimations of bioconstruction thickness; ii) collection of bioconstruction samples to describe the basal substrate (where possible) and the pattern of primary builder species, and iii) evaluation of the substrate covering values of the main benthic taxa by analyzing underwater photographic and video images.
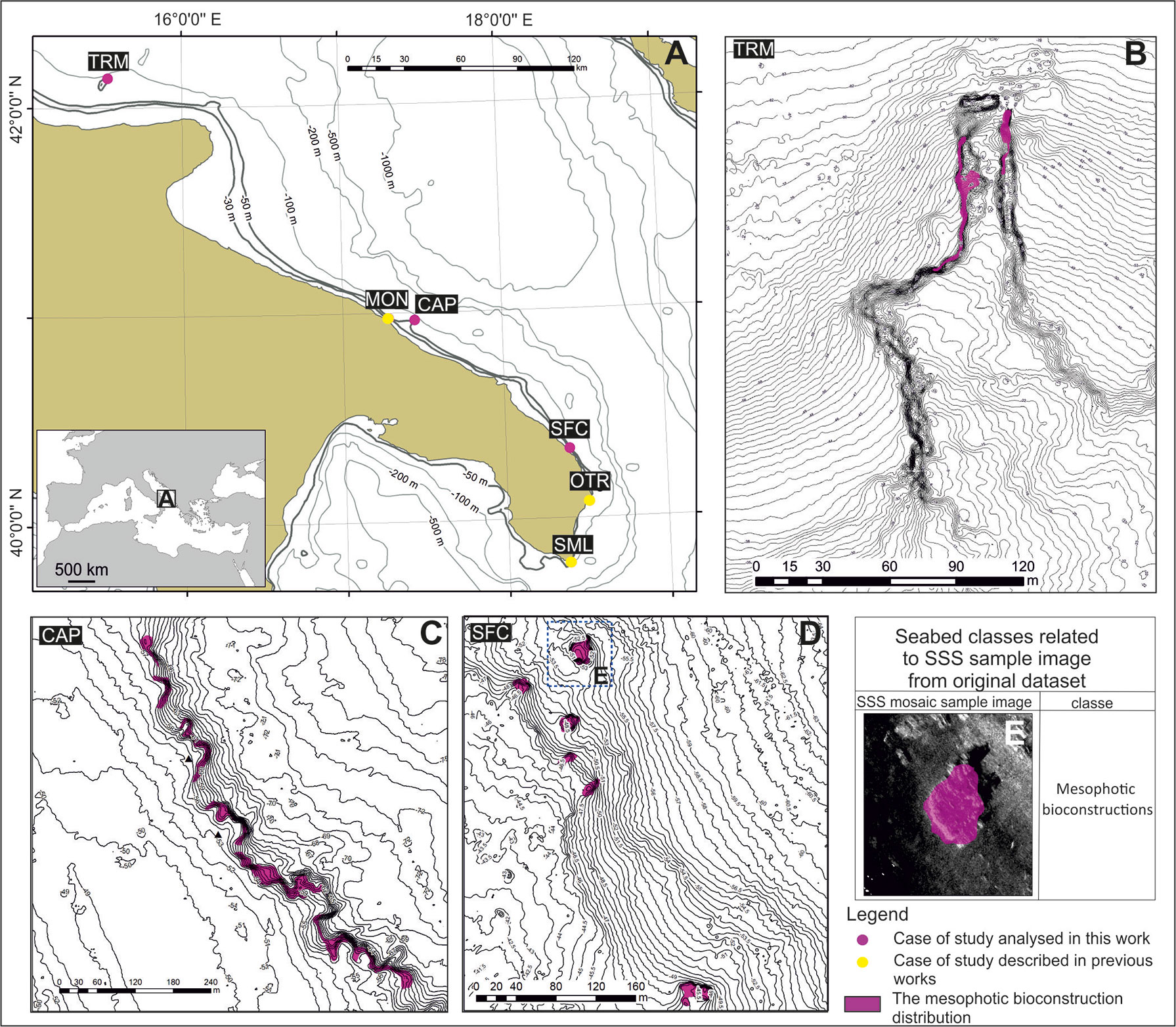
Figure 1 (A) Distribution of the mesophotic reefs along the Apulian coast. Yellow and purple circles show the already described reefs (MON – Corriero et al., 2019; OTR and SML – Cardone et al., 2020) and the mesophotic reefs described in the present paper (TRM, CAP, and SFC), respectively; (B-D) seafloor mapping (TRM, CAP and SFC shelf areas); (E) detail of the map in (D): example of seabed classes recognized on SSS images.
In addition, TRM, CAP and SFC were investigated using geophysical techniques to characterize the morphobathymetric features of the seafloor.
Seafloor mapping
Navigation was conducted with DGPS (Differential Global Positioning System) TRIMBLE SPS551 by means of the navigation software RESON PDS2000. Morphobathymetric maps were developed by using a CHIRP pulse side-scan sonar (SSS, BENTHOS SIS1500, 190-210 kHz frequency) and by processing data with CARIS SIPS. The results were inserted into a Geographic Information System (GIS ESRI ArcView 10.2; projection UTM33N-WGS84). Different bathymetric datasets from past surveys carried out by other geophysical teams were reviewed and merged into a final unique bathymetric map in a GIS environment. A multibeam survey (MBES) was carried out to obtain a high-resolution digital elevation model (DEM) of the seafloor that was used to identify the main morphological features associated with the bioconstructions. High-resolution SSS grayscale images (0.2 m pixel - raw dataset and processed mosaicked image) were used for the identification of the largest bioconstructions. In the GIS environment, detailed mapping based on the geophysical features of the bioconstructions was executed on georeferenced images; geophysical-based polygons were used to drive preliminary ROV analysis and subsequent video transect and sampling procedures. To validate the interpretation of the mosaic sonograms and preliminarily describe the “architecture” of the reef, ROV (Mariscope FO III) surveys were performed. ROV profiles were chosen according to the bionomic map, corresponding to passages from one facies to another or to locations where the signal returned from geophysics was not sufficient to exactly define the type of biological association present. The ROV was equipped with an underwater acoustic tracking position system (Applied Acoustic Smart Track) that provided records of its track along the seabed. The navigation software RESON PDS2000 continuously recorded the position of both the ROV and ship to georeference the images of the seabed.
Structure of the bioconstructions
With the aim of analyzing the general architecture of the bioconstructions, two samples of substrate (approximately 2-3 L) were collected by scuba divers from each study area. The original orientation of the samples was maintained by tagging in situ the main reference points (top, bottom, right and left sides and attachment point to the bioconstruction). Each sample, after being dried in the laboratory oven for 48 h at a temperature of 80°C, was impregnated with an epoxy resin to keep the original structure unaltered. The resin-embedded samples were cut into transverse slices by a stone saw. The surfaces of the slides were polished, measured with a high-resolution scanner and analyzed with image analysis procedures using ImageJ software. This procedure allowed us to evaluate the porosity of the bioconstruction and identify the builder taxa, leading to the production of 2D detailed maps of its inner structure.
Analysis of the images
Based on the geophysical surveys and preliminary ROV outputs, three vertical video transects were performed from the top to the bottom of the bioconstruction in each study area to describe the pattern of the megabenthic assemblage. Additional scuba dives were performed to collect small biological samples to improve taxonomic resolution. All the dives were conducted by technically trained divers equipped with high-definition video cameras (Sony PMW-EX1 and Sony Alpha 7III), high-performance LED strobe illuminators (EasyDive, 13,000 lumens) and 3 laser beams providing a 10-cm scale for measuring sampling areas on the substrate and obtaining quantitative data of the community. Video images were then evaluated using VLC Media Player Free software. The megabenthic organisms were recorded within 50-cm visual fields and then identified by taxonomists to the lowest possible taxonomic level. The percent contribution of each taxon was evaluated by analyzing 20 video frames obtained from each video transect for a total of 360 frames. The frames were extracted using freely available DVDVideoSoft Free Studio software, and image analysis was performed using ImageJ software. The covering values were calculated by superimposing a grid of 9 subsquares onto each image and counting the number of subsquares within which each taxon was found.
The data were used to create a matrix of occurrence and abundance data for subsequent statistical analyses. Prior to the analysis, the covering data of the detected species were square-root transformed, and a triangular similarity matrix was obtained by applying the Bray–Curtis index (Bray and Curtis, 1957). Nonmetric multidimensional scaling (nMDS) via Bray–Curtis distances on square-root transformed data, combined with clustering analysis, was used to visualize changes in the composition of the benthic assemblages (nMDS plot). Species that contributed most to the similarities among the groups of samples were identified using similarity percentage analysis (SIMPER). The cutoff criterion for the identification of species covering was 90%. Mean values of species richness and megabenthic percent covering were also calculated and graphed over time. PERMANOVA, nMDS, and SIMPER analyses were run using PRIMER v6 + PERMANOVA (Anderson et al., 2008).
Results
Distribution, maps and main morphological features
The geographic distribution and detailed locations of the studied mesophotic bioconstructions are shown in Figure 1. The main measures recorded are reported in Table 1. The bioconstructions developed on a vertical cliff or a subhorizontal soft bottom in the bathymetric range from 35 to 70 m along the southern Adriatic (TRM, MON, CAP, SFC, and OTR) and Ionian (SML) Italian coasts. Their extension (length of the major axis) ranged from a minimum of 30 m (SFC) to a maximum of 460 m (SML) (Table 1). Overall, the bioconstructions showed different orientations with respect to the coastline, and different structural and biological traits were recorded among the study sites (Figure 1; Table 1).
TRM
The bioconstruction developed in the approximate bathymetric range 40/55 m, on a steep (~ 90°) rocky cliff north-south oriented, uniformly occupying its northwestern and western sides and only partially its northeastern portion (Figure 1B). The biogenic substrate was organized in globose structures up to 0.5 m in diameter, superimposed and side by side, which gave the bioconstruction high structural complexity (Figure 2A). The maximum thickness detected in situ did not exceed 0.5 m.
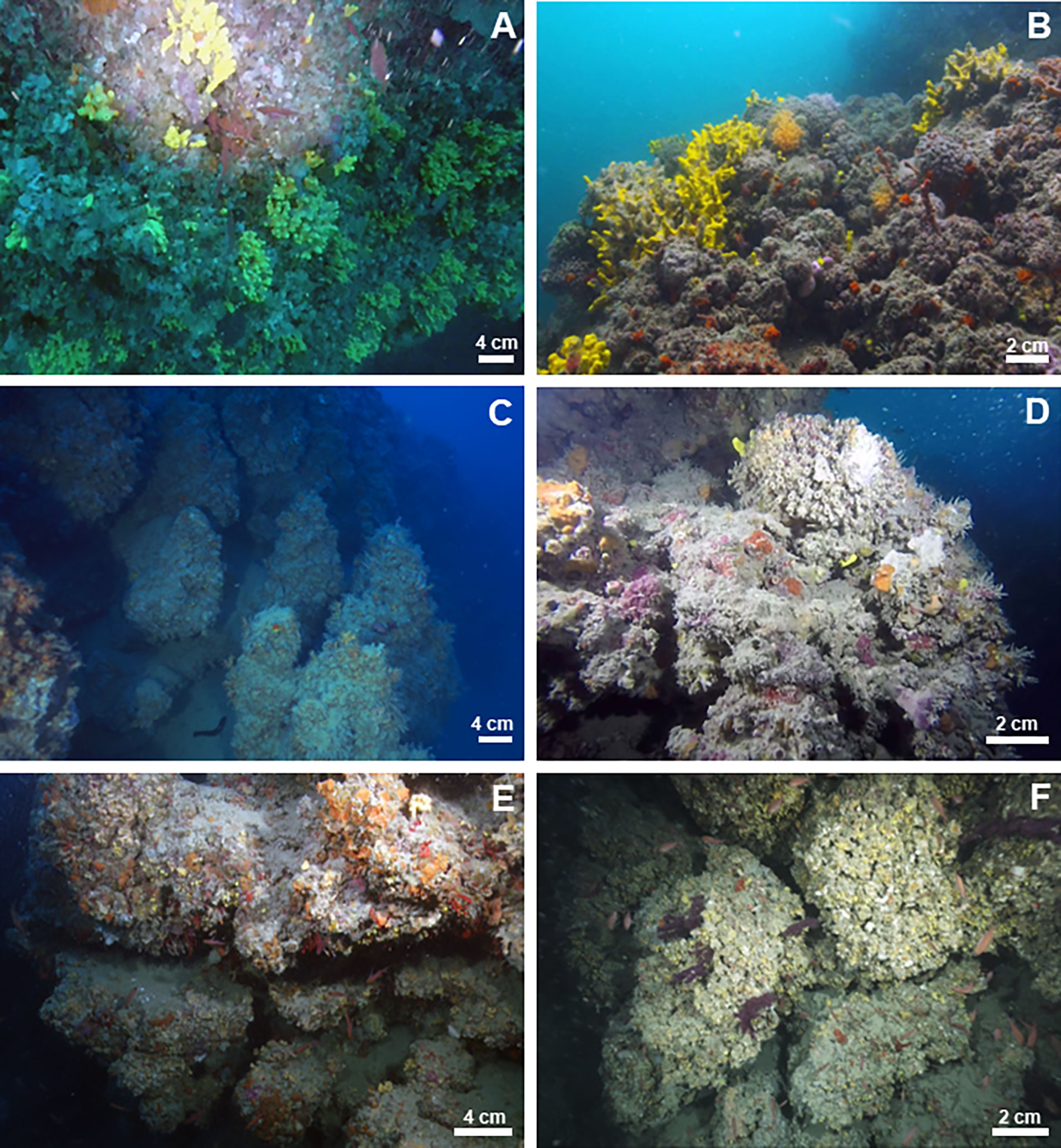
Figure 2 Underwater images of the morphological features of studied mesophotic bioconstructions: (A) TRM, (B) MON, (C) CAP, (D) SFC, (E) OTR, and (F) SML.
MON
The bioconstruction developed within a bathymetric range of 35-47 m on a subvertical cliff. It appeared as a thick (up to 1.5 m) and continuous framework of coral blocks organized into an interlocking meshwork that provided reef stiffness (Figure 2B). More details are contained in Corriero et al. (2019).
CAP
The bioconstruction, consisting of a series of promontories, developed along a moderately steep slope running in the north–south direction, parallel to the coast. The biogenic structure was grafted to the substrate in a bathymetric range between 53 and 64 m (Figure 1C). It was organized in globose formations from 0.5 to 1.5 m in diameter that protruded perpendicularly from the cliffs, were superimposed and occurred side by side (Figure 2C). In addition, on subhorizontal substrates, the bioconstruction developed as coniform pinnacles up to 1 m high.
SFC
A mosaic of scleractinian buildups, rising to a height of 0.5 to 4 m from a muddy bottom, was distributed between 45 and 53 m in depth on a moderately steep slope (Figure 1D). The single scleractinian colonies were globular in shape and did not exceed 25 cm in diameter. They grew embedded with each other and formed a framework of high structural complexity with a maximum thickness of 0.4 m (Figure 2D).
OTR
The bioconstruction developed on steep vertical cliffs rising from a detritic bottom at approximately 65 m in depth to a height of approximately 20 m. It consisted of globose formations, approximately 0.5 m in diameter, protruding perpendicularly with respect to the cliff and interconnected with one another (Figure 2E). The maximum thickness was 0.8 m. More details are reported in Cardone et al. (2020).
SML
The bioconstruction almost uniformly covered the northern and eastern sides of the cliff within the bathymetric range of 45–70 m, for a total length of approximately 460 m. It consisted of thick pinnacles 1.5 m high, perpendicular to the cliff and interconnected with one another, forming a framework of high structural complexity (Figure 2F). More details are supplied in Cardone et al. (2020).
Framework of the bioconstructions
The bioconstructions were mainly built by the nonsymbiotic scleractinians Phyllangia americana mouchezii (Lacaze-Duthiers, 1897) and Polycyathus muellerae (Abel, 1959) at MON and SFC and the Gryphaeidae bivalve Neopycnodonte cochlear (Poli, 1795) at TRM, CAP, OTR and SML (Table 1).
TRM
The bioconstruction architecture was mainly supported by the bivalve N. cochlear (Figures 3A, B). The valves were only partially articulated, and the disarticulated valves were also covered by other mollusks. The contacts between the valves were very localized or involved small surfaces of contiguous valves (Figure 3A). Bryozoans, polychaete tubes and single corals represented accessory elements of the general structure. Boring traces along the framework of the carbonate structure and sponge perforations on the valves of N. cochlear were also common (Figure 3B). Overall, the structure showed marked porosity, and there was no sedimentary infill in the large voids.
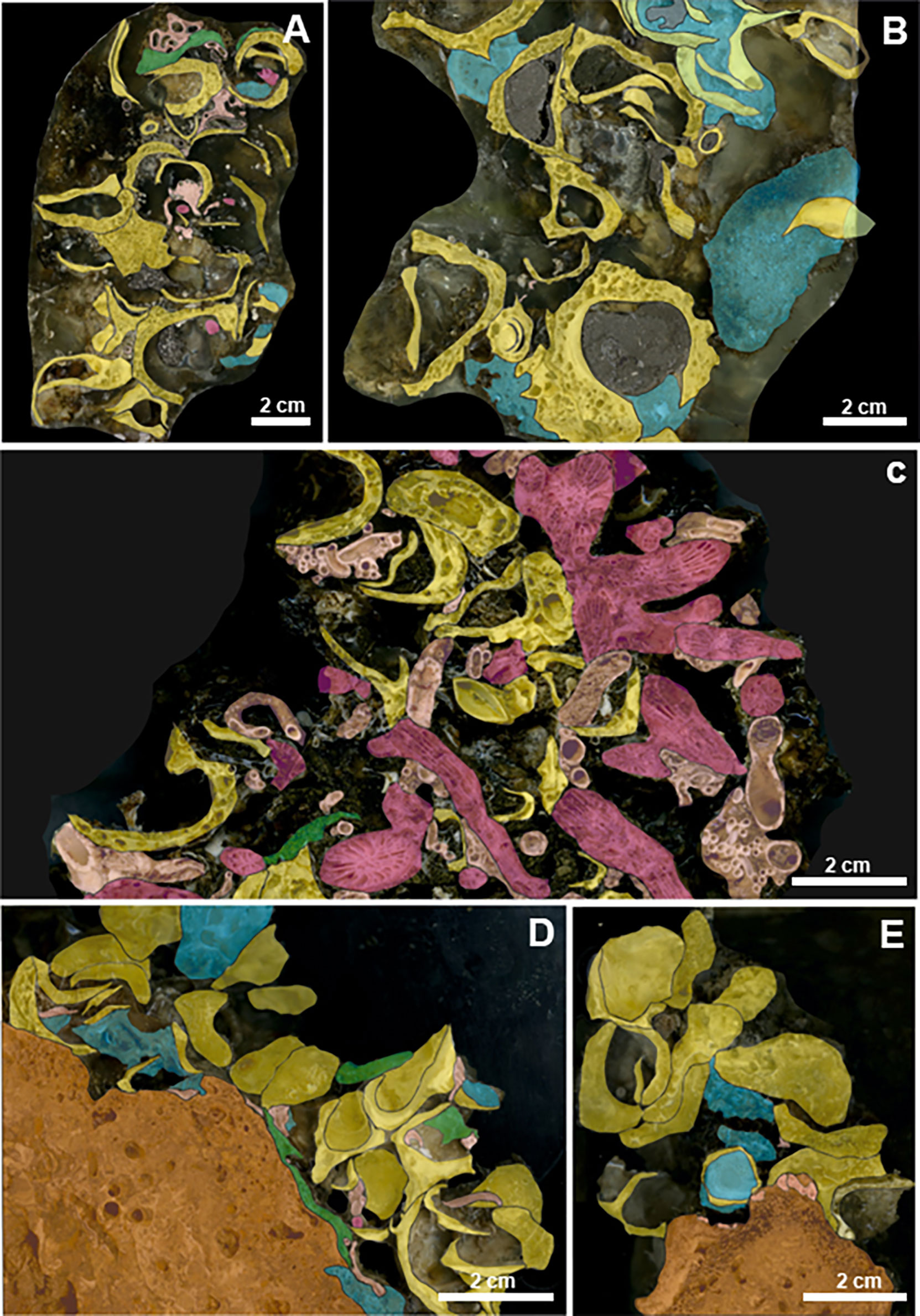
Figure 3 HD digitized images of impregnated sample slices of the bioconstructions. Scale bar = 2 cm. Legend: yellow = bivalves; violet = scleractinians; blue = sponges; green = bryozoans; pink = serpulids; and orange = biogenic substrate. (A) TRM. The bioconstruction is made up of valves of N. cochlear. (B) The biogenic framework at TRM is deeply bioeroded by sponges. (C) MON. Scleractinians and mollusks within the framework of the bioconstruction. (D) CAP. N. cochlear bioconstruction develops on an older biogenic substrate. (E) CAP. Details of the framework.
MON
Analysis of recent samples confirmed that the reef was mainly constituted by the skeletal remains of the scleractinians P. a. mouchezii and P. muellerae (see more details in Corriero et al., 2019). Some scattered disarticulated valves of the bivalve N. cochlear also contributed to the edification of the carbonate structure. The serpulid and vermetid calcareous tubes played an important role in increasing the architectural stability by cementing the boundary surfaces among adjacent corallites (Figure 3C).
CAP
The bivalve N. cochlear was the primary builder and formed a complex biogenic structure (Figure 2C). The spatial architecture of the bioconstruction mainly resulted from articulated valves with multiple points of contact with one another (Figures 3D, E), which fostered structural stability. In contrast, the marked bioerosion processes of sponges occurred on the valves and produced a high degree of porosity in the structure, even though porosity was lower than that recorded at other sites (see TRM). No sedimentary filling was recorded in the structural holes and crevices. Other recognizable taxa were bryozoans and serpulids (Figures 3D, E), contributing to the stability of the structure.
SFC
The bioconstruction was mainly built by the scleractinian P. muellerae and further by P. a. mouchezii, both directly anchored to a compact biogenic substrate (Figure 4A). The architecture was very similar to that found at MON, even if the framework of the bioconstruction was less compact and stable. Indeed, unlike at MON, at SFC, the spaces between adjacent corallites were only occasionally occupied by epibionts such as serpulids and sponges.
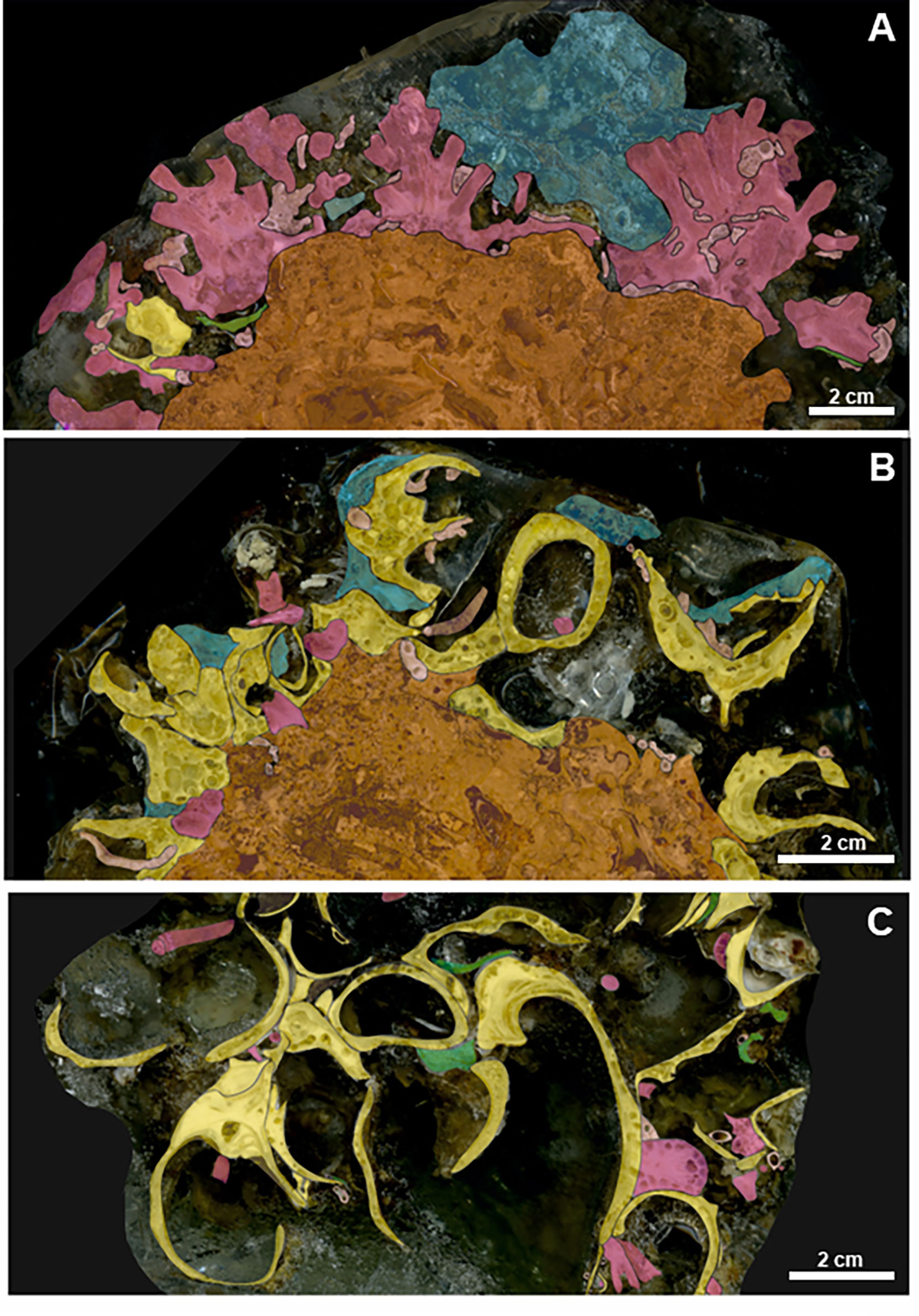
Figure 4 HD digitized images of impregnated sample slices of the bioconstructions. Scale bar = 2 cm. Legend: yellow = bivalves; violet = scleractinians; blue = sponges; green = bryozoans; pink = serpulids; and orange = biogenic substrate. (A) SFC bioconstruction shows a compact, moderately porous framework. It is made up of scleractinians growing on a pre-existing biogenic substrate. (B) At OTR, the bioconstruction is made up of closed shells of N. cochlear laying on a previous biogenic structure. (C) SML. The bioconstruction frequently shows disarticulated mollusk valves that are deeply bored by sponges.
OTR and SML
At OTR, occasional colonies of bryozoans and serpulid tubes contributed to the bioconstruction, while scleractinians were more common at SML. Moreover, the two bioconstructions differed in porosity, which was more marked at SML than at OTR. At both sites, the valves of the oysters were affected by the intense bioerosion activity of the sponges (Figures 4B, C).
Megabenthic assemblages
A total of 54 megabenthic taxa of invertebrates belonging to 6 phyla (Porifera, Cnidaria, Annelida, Mollusca, Bryozoa and Chordata) were recognized from the video analysis of the bioconstructions. Not all the taxonomic identifications were conducted down to a species level in relation to the video recognition technique. Eighteen of the taxa recorded appeared exclusively on the Neopycnodonte cochlear bioconstructions (oyster bioconstructions, OBs), 6 were exclusive of the scleractinian bioconstructions (SBs) and 28 were shared between them (Supplementary Table S1). Overall, most of the taxa detected were in the phylum Porifera with 33 taxa, 27 of which were recorded in OBs and 22 in SBs. Nine taxa were Cnidaria (9 in OBs and 6 in SBs), 5 were Bryozoa (5 in OBs and 4 in SBs), 3 were Chordata (3 in OBs and 2 in SBs), and finally, 1 taxon was in Annelida and 1 in Mollusca, both recorded in OBs only.
In general, Porifera and Cnidaria were dominant (about 65%) in the epibenthic fauna of the SBs, while Porifera, Cnidaria and Mollusca accounted for about 80% of the epibenthic covering of the OBs (Figure 5).
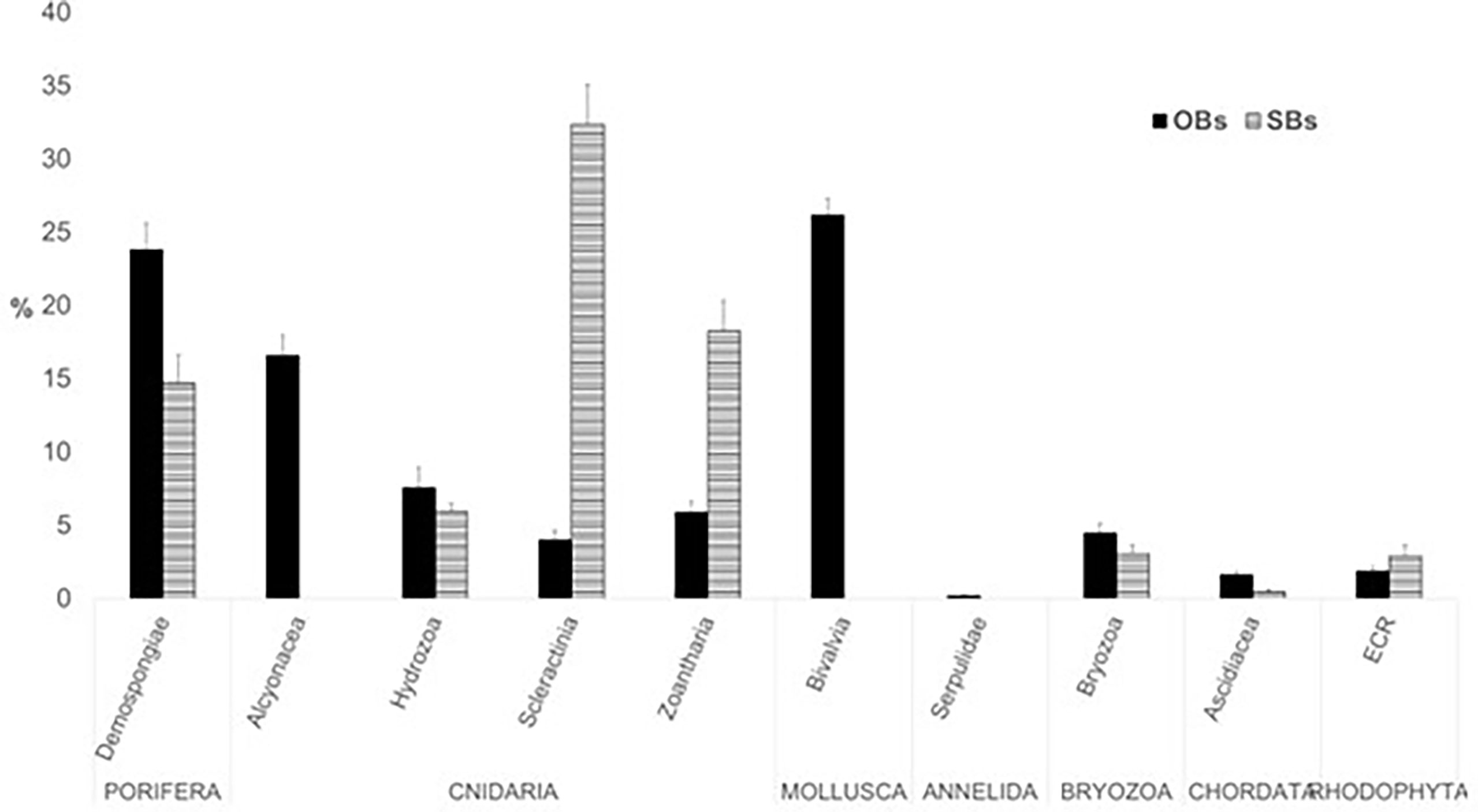
Figure 5 Percent covering (mean ± St. Dev. of covering values) of the main epibenthic taxa in the studied mesophotic bioconstructions (OBs, oyster bioconstructions; SBs, scleractinian bioconstructions, ECR, encrusting coralline Rhodophyta).
Even though the species distribution patterns varied among the sampling areas, they showed some recurrent features. The SIMPER analysis, based on a 90% cutoff of structural biodiversity (Table 2), showed the taxa that contributed most to the characterization of the mesophotic assemblages. The assemblage with the highest number of prominent taxa (7) was recorded at SML, where in addition to N. cochlear, the scleractinian Leptopsammia/Cladopsammia complex, demosponges and alcyonaceans such as Corallium rubrum and Paramuricea clavata mainly accounted for the covering pattern. On the contrary, the benthic assemblage from MON showed the lowest number of taxa contributing to the total covering pattern, with the remarkable presence (more than 37%) of the zoantharian Parazoanthus axinellae. Finally, OTR was characterized by the presence of C. rubrum, while at TRM, the bioconstruction was dominated by P. clavata.
The nMDS analysis highlighted differences among the sites, with the first two axes representing 65% of the total variance, and the third one representing 16%. The nMDS plot (Figure 6) showed a first separation between TRM and all the other sites, with the other sites sharing 20% of the taxa covering pattern recorded. Within this last cluster, the scleractinian bioconstructions from MON and SFC were further isolated from all the oyster bioconstructions, which were subdivided into two sets (CAP vs OTR and SML), each characterized by a 40% similarity value. Finally, each of the bioconstructions from CAP and OTR showed the highest values of similarity (60%) with respect to all the other study areas (Figure 6).
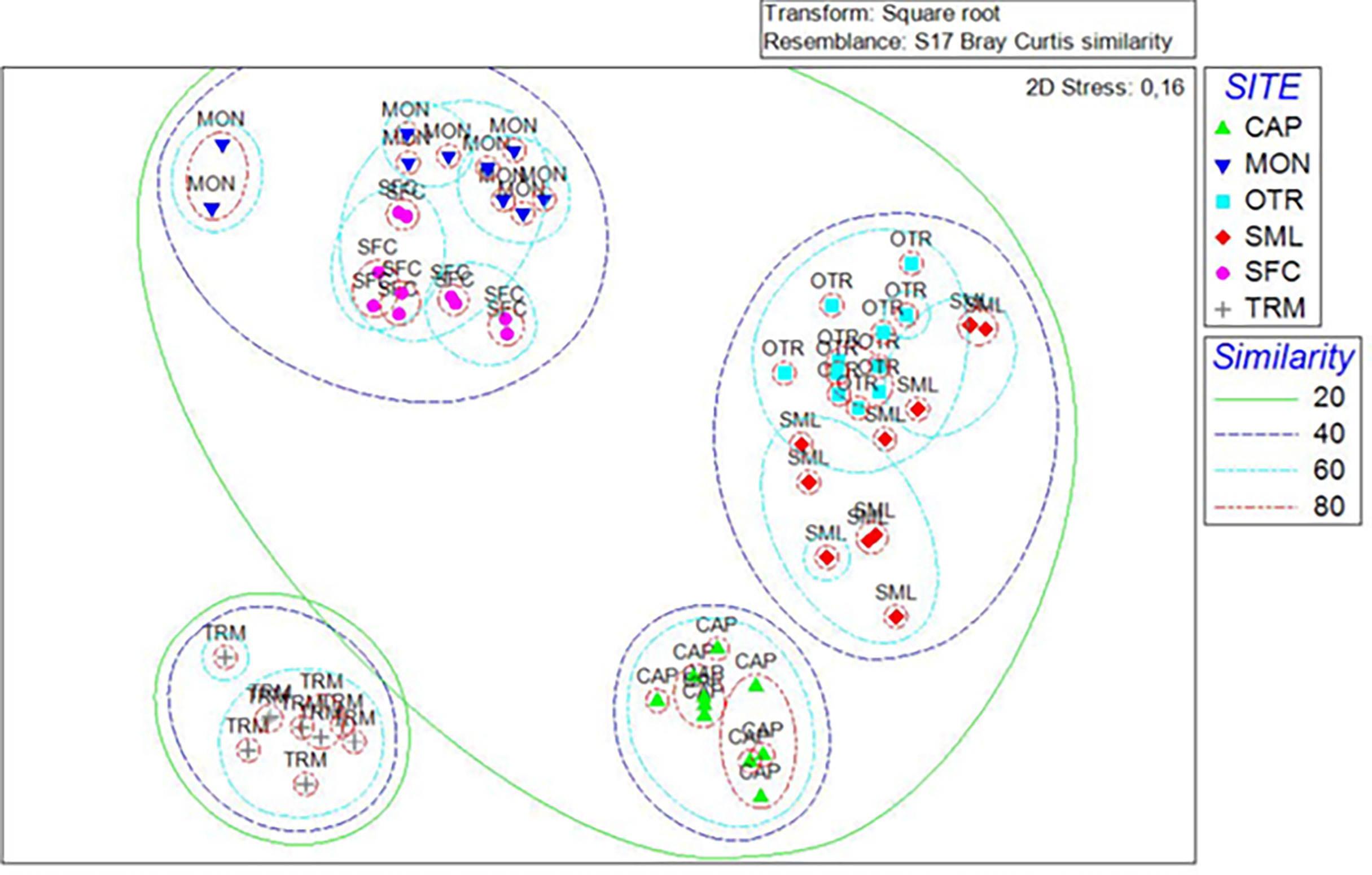
Figure 6 nMDS plot showing the differences between mesophotic bioconstructions based on the covering values of the associated megabenthic taxa. Sets of different colors include samples with different similarity levels (shown in the legend). Stress = 0.16.
The detailed distribution of megabenthic taxa determined from the analysis of the ROV videos of the bioconstructions is reported in Figure 7
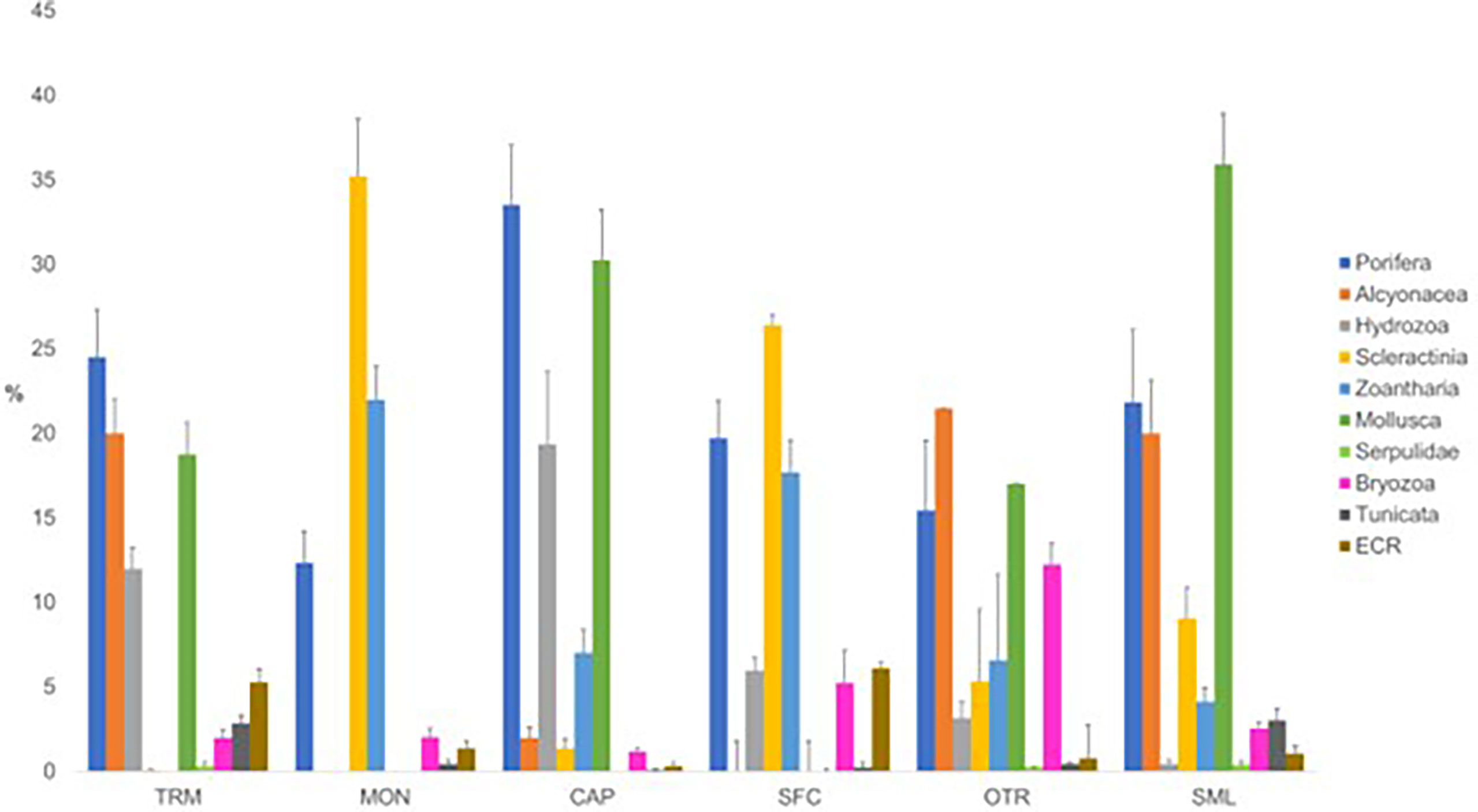
Figure 7 Percentage distribution (mean ± St. Dev. of covering values) of the main megabenthic taxa in each investigated area. TRM, Tremiti; MON, Monopoli; CAP, Capitolo; SFC, San Foca; OTR, Otranto; and SML, Santa Maria di Leuca.
TRM
Overall, 81% of the oyster bioconstruction was covered by epibionts. Poriferans were dominant within the associated community, with average covering values of approximately 25%. This taxon was mainly represented by large specimens of Aplysina cavernicola, which were detected in 100% of the video frames. Other common sponge species were Dysidea cf. incrustans, Topsentia pachastrelloides and Oscarella lobularis. Together with A. cavernicola, the gorgonian Paramuricea clavata (20% mean covering values) formed an additional 3D epibenthic substrate, adding a distinctive characteristic to the seascape. Hydroids were frequent (82%), although with moderate covering values (12%), and they included unidentified erect species colonizing the valves of Neopycnodonte and forming a mat that became locally dominant. Other represented taxa, although to a lesser extent, were the encrusting bryozoans Schizomavella spp. and colonial tunicates (Clavelina cf. dellavallei). Encrusting coralline rhodophyte (ECR) species were detected in 87% of the frames analyzed, although they covered limited portions of substrate (less than 6%) (Figure 8A).
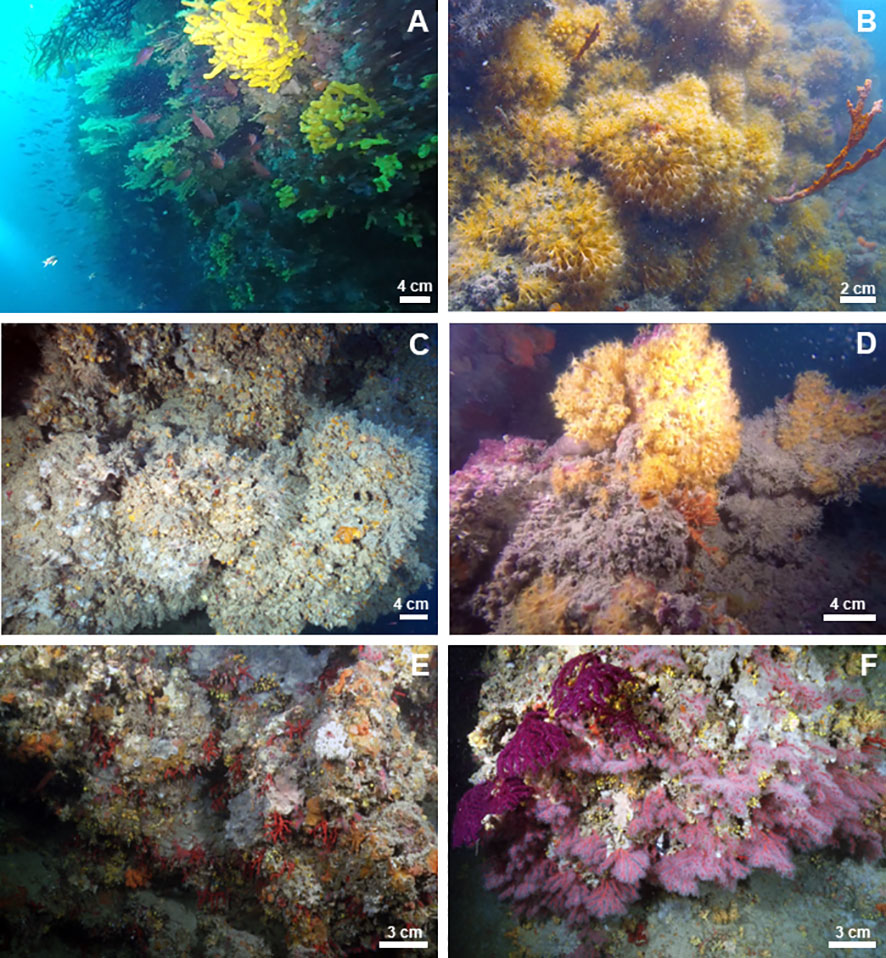
Figure 8 Underwater images of the megabenthic assemblages associated with the studied mesophotic bioconstructions: (A) TRM, (B) MON, (C) CAP, (D) SFC, (E) OTR, and (F) SML.
MON
On the scleractinian bioconstruction, 66% of the substrate was covered by epibionts. In addition to the main bioconstructors, other contributors were represented by the Leptopsammia/Cladopsammia complex, at low covering values (frequency: 95%; covering value: 1%). The zoantharian P. axinellae was the most represented of the epibenthic community, identified in 91% of the video frames analyzed, with covering values of approximately 22%. Porifera (12%) were mainly represented by A. cavernicola, Sarcotragus spinosulus and Petrosia ficiformis. Among the bryozoans, the encrusting Schizomavella spp. were frequent (50%), with average covering values of 2%. Algae, represented by ECR, were detected in 28% of the video frames analyzed, with a low covering value (1.3%) (Figure 8B).
CAP
In general, 70% of the oyster bioconstruction was covered by epibionts. The associated epibenthic community was characterized by a few dominant taxa. Porifera accounted for 34% of the benthic assemblage, almost exclusively represented by the encrusting sponge Dendroxea lenis, which covered wide portions of the substrate. The bioconstruction was also plentifully colonized by the erect hydroid Synthecium evansi, which showed a patchy distribution forming true forests on some portions of the substrate, while on others, it was almost completely absent. A similar pattern was also observed for the alcyonacean P. clavata, occurring only in some areas of the bioconstruction and in particular on the upper portion of the walls. Other recurrent taxa, although with low covering values, were Parazoanthus axinellae (frequency: 92%; covering value: 5.9%) and the bryozoans Schizomavella spp. (frequency: 65%; covering value: 1.2%), and they were mainly detected on the exposed portion of the substrate. The contribution of ECR was minimal. They were recorded with a frequency of 13% and covering values less than 1% (Figure 8C).
SFC
Seventy-five percent of the scleractinian bioconstruction was covered by epibionts. Porifera, mainly represented by the demosponge A. cavernicola, and P. axinellae were the main epibenthic components, recurring in 95% and 60% of the video frames, respectively, and covering 38% of the substrate. Other sponge taxa frequently occurring in the video frames were Sarcotragus foetidus, Spongia lamella and A. cannabina. Together with A. cavernicola, they conferred a marked 3D aspect to the habitat. Among taxa with carbonate structure, the encrusting bryozoans Schizomavella spp. and the Leptopsammia/Cladopsammia complex were present, with the latter covering large portions of substrate under overhangs and crevices. Algae, mainly represented by ECR, were limited to the subhorizontal upper portion of the bioconstruction, while they were significantly reduced on the vertical substrate (frequency: 60%; covering value: 6%). (Figure 8D)
OTR
In general, 87% of the bioconstruction was covered by epibionts. The encrusting sponge D. lenis frequently occurred in the study area, with average covering values of 15%. The bryozoans Schizomavella spp. were recorded in 100% of the video frames analyzed, at a 12% covering value. They formed thin crusts on the surface, increasing the compactness of the structure. The zoantharian P. axinellae was detected in 78% of the video frames, with approximately 7% covering. The gorgonian P. clavata characterized the seascape of this habitat, representing the main 3D habitat builder; however, this species was limited to a few areas of the bioconstruction. ECR were frequent (65% of the analyzed frames) but occurred at very low covering values (approximately 1%) (Figure 8E).
SML
Sixty-four percent of the bioconstruction was covered by epibenthic organisms. Sponges, mainly represented by the encrusting D. lenis, were the most frequent taxon in this area, with an average covering value of 22%. The alcyonacean C. rubrum (58% frequency and 10% covering value) and the gorgonian P. clavata (58% frequency and 2.5% covering value) were the most important contributors in terms of 3D habitat builders. They both showed a patchy distribution, and the former was concentrated below the pinnacles of the structure with aggregates of several specimens. The bryozoans Schizomavella spp. were recorded in 92% of the video frames analyzed, with a low covering value (3%). The zoantharian P. axinellae was detected in 75% of the video frames, with covering values of approximately 4%. ECR were frequently recorded (65%), at a 1% average covering value (Figure 8F).
Discussion
In the present paper, we provide new data on the distribution of mesophotic bioconstructions, their structure and associated megabenthic assemblages along the Southern Adriatic and Ionian Italian coasts. The study suggests the occurrence of a widespread and diversified mesophotic system of carbonate bioconstructions mainly built by scleractinians and oysters. Our investigation, based on a multidisciplinary, geological and biological approach, adds new elements to what is already known about the topographic and biological characteristics of the area, significantly increasing the knowledge on the distribution, diversity and complexity of bioconstructions from the Mediterranean mesophotic zone.
The bioconstructions were found along the Apulian coast between approximately 35 and 70 m in depth, associated with areas where the seascape was highly heterogeneous. ROV exploration coupled with geophysical surveys revealed marked morphological escarpments, most of them with N- and E-dipping slopes, characterized by a basal substrate consisting of meso-cenozoic carbonate rocks that are sometimes superimposed by ancient bioconstructions. Here, two biogenic structure types were observed, one mainly built by the nonsymbiotic scleractinians Phyllangia americana mouchezii and Polycyathus muellerae and the other by the deep oyster Neopycnodonte cochlear. At the study sites, the bioconstructors were found thriving on both rocky and biogenic substrates and were able to form generally continuous structures, depending on the characteristic geomorphological features of the area.
Massive bioconstructions built by suspension feeders invertebrates have never been recognized in the Western Mediterranean Basin, where the mesophotic zone has been widely explored in order to map coral banks (Bo et al., 2009; 2019). The occurrence of such biogenic structures along the eastern Italian coast could be linked to the general water movement of the Adriatic Sea, which exhibits a cyclonic circulation resulting in a prevailing current flowing southwards along the Italian coast (Orlic et al., 1992). This latter carries along Apulian coasts a large amount of nutrients that are released into the Northern Adriatic basin by the Po River (Boldrin et al., 2002; Trincardi et al., 2007), allowing the development of rich and diversified communities of suspensivorous invertebrates, such as nonsymbiotic scleractinians and bivalves. Indeed, the thickest bioconstructions were recorded on steeper cliffs showing a northward orientation, such as those present at TRM, MON and SML, while at CAP, SFC and OTR thinner formations occurred.
According to our observations, scleractinian bioconstructions occurred at depths between approximately 35 and 55 m, while oyster bioconstructions were found from approximately 40 to 70 m. Moreover, Angeletti and Taviani (2020) reported the occurrence of N. cochlear bioconstructions up to -125 m off the coast of SML. These results agree with previous data on the bathymetric distribution of these species, with P. a. mouchezii and P. muellerae usually recorded on shallower bottoms in comparison to N. cochlear (Corriero et al., 2019; Cardone et al., 2020). The bathymetric layer between approximately -40 and -55 m seems to represent an area where the two types of bioconstructions overlap and where scleractinians and bivalves can alternate or even co-exist. At these depths, N. cochlear is represented by clusters of few individuals, although as the depth increases, it tends to become dominant.
Nonsymbiotic scleractinians and deep oysters share the same sospensivorous habitus, since they are polytrophic taxa, both able to feed on several categories of plankton, on particulate and even dissolved organic matter (Johnson et al., 2013; Conlan et al., 2017; Conlan et al., 2018). However, according to the literature (Johnson et al., 2013), the greater filtering capacity of the bivalve may cope better with inconstant food supply, whereas nonsymbiotic scleractinians could benefit from a more regular supply of food particles. Therefore, we hypothesize that hydrodynamic features at different depths among the investigated areas may determine differences in the availability of food and therefore in the prevalence of suspension feeders bioconstructor species among Apulian mesophotic bioconstructions.
Overall, the megabenthic assemblages associated with the bioconstructions under study showed a rather homogeneous taxa richness, with the only exception of CAP, where a lower value was recorded. As regards taxonomic composition, a scarce overlap was registered, with a few taxa present in at least 5 over 6 study areas. A marked differentiation of the assemblages is highlighted by the nMDS analysis performed on taxa covering values, which shows a remarkable separation of the megabenthic assemblages associated with the bioconstructions built by scleractinians (MON and SFC) from those associated with the deep oyster. On one hand, this distributional pattern could be due to the same hydrological factors affecting the distribution of primary bioconstructors; on the other hand, it should be considered that different benthic invertebrates such as scleractinians and bivalves are likely to display a different chemical arsenal directed towards space competitors and predators, this latter contributing to shape the associated community in a different way.
However, some differences in covering values of the megabenthic taxa were pointed out by the results of the SIMPER analysis, which revealed an overall increase in the number of taxa most contributing to substrate covering, following a north-south latitudinal gradient. In particular, as regards scleractinian bioconstructions, at MON only the species Parazoanthus axinellae exhibited a remarkable contribution to the assemblage; contrarily, at the southern site of SFC, four taxa were responsible for more than 90% of substrate coverage. Similarly, on bioconstructions dominated by the oyster, the number of prominent taxa increased from CAP to SML, with the exception of TRM which showed intermediate values notwithstanding its northernmost location.
The relevant role of hydrodynamic factors in driving the colonization pattern of mesophotic benthic fauna off the coast of Apulia had already been suggested for bryozoan and polychaete assemblages (Giampaoletti et al., 2020; Gravina et al., 2021). However, the heterogeneity of the megabenthic assemblages here studied was partly congruent with the local current circulation pattern of the area. In fact, the clear distinction of the assemblage of TRM was supported by the distinctive location of the archipelago, which is part of a unique and very complex geomorphological area on the border between the Middle and Southern Adriatic Sea (Miccadei et al., 2011). According to the literature, geographical distinctions hinder connectivity among benthic populations, while hydrological dynamics may promote population dynamics (Boero et al., 2016). Furthermore, the spatial heterogeneity between the megabenthic assemblages could be influenced by biological species-specific processes closely related to the reproductive traits of the species, e.g., gamete release, larval pelagic duration, and propagule supply, which support the self-recruitment of the populations rather than the dispersal and connectivity between the populations (Cowen and Sponaugle, 2009; Giangrande et al., 2017). In this view, the occurrence of P. axinellae at all the sites is congruent with its widespread geographic distribution related to the low genetic differentiation among populations (Villamor et al., 2020).
Based on their structural aspects, distribution and faunal traits, the bioconstructions off the Apulian coast may be included as a peculiar, coherent system into the Mediterranean Temperate Mesophotic Ecosystem (sensu Cerrano et al., 2019). On the one hand, the characteristic hydrological regime of the area promoted connectivity between the assemblages. On the other hand, the megabenthic assemblages partially differentiated themselves at a local scale, depending on both the wide morphological heterogeneity of the seabed and the probable historical factors that, together with the life traits of the species, have driven the bioconstruction colonization patterns.
Finally, the present research highlighted the role of the investigated mesophotic bioconstructions as biodiversity hotspots, which enhanced the species richness and diversity of the associated benthic assemblages. Although during our study we recorded a moderate-to-low impact due to lost fishing gears, these habitats are subjected to numerous other threats due to anthropic activities (Ponti et al., 2021), thus deserving the adoption of valid conservation measures, taking into account the holistic ecosystem approach for the management of coastal marine areas.
Data availability statement
The original contributions presented in the study are included in the article/Supplementary Material. Further inquiries can be directed to the corresponding author.
Author contributions
The present work has required a multidisciplinary approach, thus involving the collaboration of a large number of experts. All authors contributed extensively to the research, interpreted and discussed the results and approved the final manuscript. In detail, FC, MMe, CL, and CP coordinated field activities. FC, MG, AG, CN, and GG elaborated and interpreted the data. MMo, SL, and FG designed and carried out geophysical surveys, prepared the maps and elaborated and interpreted the data. Taxonomists, FC and CL of poriferans, FC and GG general megabenthic taxa. CP and GG performed the comparative analysis between the communities of the study areas and edited the analysis. GG, FC, SL, and FG prepared figures and tables. GC, FC, MG, MMo, and AG wrote the original draft and review. All authors contributed to the article and approved the submitted version.
Funding
This work was partially funded by CoNISMa as part of Marine Strategy Framework Directive.
Acknowledgments
The authors wish to thank Alex Gubello, Gianluca Cassano, Stefano Levanto, Riccardo Cingillo, Massimiliano Piccolo, Michele Tancredi, Graziano Servello, Francesco Della Folgore, Eddy Cannoletta for their logistical help in performing underwater sampling. A special thanks to the Marine Protected Area “Tremiti Islands” for the support in the research activities. The authors wish also to thank Jacopo Giampaoletti from CNR-IAS, for the identification of bryozoans.
Conflict of interest
The author FG is employed by Environmental Surveys S.r.l. (ENSU), Via de Gasperi - 74123, Taranto, Italy.
The remaining authors declare that the research was conducted in the absence of any commercial or financial relationships that could be construed as a potential conflict of interest.
Publisher’s note
All claims expressed in this article are solely those of the authors and do not necessarily represent those of their affiliated organizations, or those of the publisher, the editors and the reviewers. Any product that may be evaluated in this article, or claim that may be made by its manufacturer, is not guaranteed or endorsed by the publisher.
Supplementary material
The Supplementary Material for this article can be found online at: https://www.frontiersin.org/articles/10.3389/fmars.2022.948836/full#supplementary-material
References
Abel E. F. (1959). Zur Kenntnis der marinen Hohlenfauna unter besonderer Beriicksichtigung der Anthozoen. Pubblicazioni della Stazione Zoologica di Napoli 30 (1), 1–94.
Anderson M., Gorley R., Clarke K. P. (2008). For PRIMER: guide to software and statistical methods Vol. 32 (Plymouth, UK: Primer-e).
Angeletti L., Taviani M. (2020). Offshore Neopycnodonte oyster reefs in the Mediterranean Sea. Diversity 12 (3), 92. doi: 10.3390/d12030092
Ballesteros E. (2006). Mediterranean Coralligenous assemblages: a synthesis of present knowledge. Oceanogr. Mar. Biol. Ann. Rev. 44, 123–195. doi: 10.1201/9781420006391.ch4
Bertness M. D., Callaway R. (1994). Positive interactions in communities. Trends Ecol. Evol. 9 (5), 191–193. doi: 10.1016/0169-5347(94)90088-4
Bo M., Montgomery A. D., Opresko D. M., Wagner D., and Bavestrello G. (2019). Characteristics of a black coral meadow in the twilight zone of the central Mediterranean Sea. Mar. Ecol. Prog. Ser. 397, 53–61. doi: 10.3354/meps08185
Bo M., Montgomery A. D., Opresko D. M., Wagner D., Bavestrello G.. (2019). “Antipatharians of the Mesophotic Zone: Four Case Studies,” in Mesophotic Coral Ecosystems Coral Reefs of the World Eds. Loya Y., Puglise K. A., Bridge T. C.L. (Cham: Springer International Publishing), 603–708. doi: 10.1007/978-3-319-92735-0_37
Boero F., Foglini F., Fraschetti S., Goriup P., Macpherson E., Planes S., et al. (2016). CoCoNet: towards coast-to-coast networks of marine protected areas (from the shore to the high and deep sea), coupled with sea-based wind energy potential. SCIRES-IT 6, 1–95. doi: 10.2423/i22394303v6Sp1
Boldrin A., Miserocchi S., Rabitti S., Turchetto M. M., Balboni V., Socal G. (2002). Particulate matter in the southern Adriatic and Ionian Sea: characterisation and downward fluxes. J. Mar. Syst. 33, 389–410. doi: 10.1016/S0924-7963(02)00068-4
Bray J. R., Curtis J. T. (1957). An ordination of the upland forest communities of southern Wisconsin. Ecol. Monogr. 27 (4), 326–349. doi: 10.2307/1942268
Cardone F., Corriero G., Longo C., Mercurio M., Onen Tarantini S., Gravina M. F., et al. (2020). Massive bioconstructions built by Neopycnodonte cochlear (Mollusca, bivalvia) in a mesophotic environment in the central Mediterranean Sea. Sci. Rep. 10 (1), 1–16. doi: 10.1038/s41598-020-63241-y
Cerrano C., Bastari A., Calcinai B., Di Camillo C., Pica D., Puce S., et al. (2019). Temperate mesophotic ecosystems: gaps and perspectives of an emerging conservation challenge for the Mediterranean Sea. Eur. Zool. J. 86 (1), 370–388. doi: 10.1080/24750263.2019.1677790
Cerrano C., Danovaro R., Gambi C., Pusceddu A., Riva A., Schiaparelli S. (2010). Gold coral (Savalia savaglia) and gorgonian forests enhance benthic biodiversity and ecosystem functioning in the mesophotic zone. Biodivers. Conserv. 19 (1), 153–167. doi: 10.1007/s10531-009-9712-5
Chimienti G., De Padova D., Mossa M., Mastrototaro F. (2020). A mesophotic black coral forest in the Adriatic Sea. Sci. Rep. 10 (1), 1–15. doi: 10.1038/s41598-020-65266-9
Conlan J. A., Bay L. K., Severati A., Humphrey C., Francis D. S. (2018). Comparing the capacity of five different dietary treatments to optimise growth and nutritional composition in two scleractinian corals. PloS One 13 (11), e0207956. doi: 10.1371/journal.pone.0207956
Conlan J. A., Rocker M. M., Francis D. S. (2017). A comparison of two common sample preparation techniques for lipid and fatty acid analysis in three different coral morphotypes reveals quantitative and qualitative differences. PeerJ 5, e3645. doi: 10.7717/peerj.3645
Corriero G., Pierri C., Mercurio M., Nonnis Marzano C., Onen Tarantini S., Gravina M. F., et al. (2019). A Mediterranean mesophotic coral reef built by non-symbiotic scleractinians. Sci. Rep. 9 (1), 1–17. doi: 10.1038/s41598-019-40284-4
Cowen R. K., Sponaugle S. (2009). Larval dispersal and marine population connectivity. Annu. Rev. Mar. Sci. 1, 443–466. doi: 10.1146/annurev.marine.010908.163757
Enrichetti F., Bavestrello G., Betti F., Coppari M., Toma M., Pronzato R., et al. (2020). Keratose-dominated sponge grounds from temperate mesophotic ecosystems (NW Mediterranean Sea). Mar. Ecol. 41 (6), e12620. doi: 10.1111/maec.12620
Enrichetti F., Bavestrello G., Coppari M., Betti F., Bo M. (2018). Placogorgia coronata first documented record in Italian waters: Use of trawl bycatch to unveil vulnerable deep-sea ecosystems. Aquat. Conserv.: Mar. Freshw. Ecosyst. 28 (5), 1123–1138. doi: 10.1002/aqc.2930
Etnoyer P., Morgan L. E. (2005). “Habitat-forming deep-sea corals in the northeast pacific ocean,” in Cold-water corals and ecosystems (Berlin, Heidelberg: Springer), 331–343. doi: 10.1007/3-540-27673-4_16
Eyal G., Pinheiro H. T. (2020). Mesophotic ecosystems: the link between shallow and deep-sea habitats. Diversity 12 (11), 411. doi: 10.3390/d12110411
Giampaoletti J., Cardone F., Corriero G., Gravina M. F., Nicoletti L. (2020). Sharing and distinction in biodiversity and ecological role of bryozoans in Mediterranean mesophotic bioconstructions. Front. Mar. Sci. 1040. doi: 10.3389/fmars.2020.581292
Giangrande A., Gambi M. C., Gravina M. F. (2017). Paradigm shifts in community ecology: Open versus closed units, challenges and limits of connectivity studies. Mar. Ecol. 38 (6). doi: 10.1111/maec.12480
Gori A., Bavestrello G., Grinyó J., Dominguez-Carrió C., Ambroso S., Bo M. (2017). Animal forests in deep coastal bottoms and continental shelf of the Mediterranean Sea. Mar. Anim. Forests.: Ecol. Benthic Biodivers. hotspots. (Cham: Springer International Publishing), 1–27. doi: 10.1007/978-3-319-17001-5_5-1
Gravina M. F., Pierri C., Mercurio M., Nonnis Marzano C., Giangrande A. (2021). Polychaete diversity related to different mesophotic bioconstructions along the southeastern Italian coast. Diversity 13 (6), 239. doi: 10.3390/d13060239
Ingrosso G., Abbiati M., Badalamenti F., Bavestrello G., Belmonte G., Cannas R., et al. (2018). Mediterranean Bioconstructions along the Italian coast. Adv. Mar. Biol. 79, 61–136. doi: 10.1016/bs.amb.2018.05.001
Johnson M. P., White M., Wilson A., Würzberg L., Schwabe E., Folch H., et al. (2013). A vertical wall dominated by Acesta excavata and Neopycnodonte zibrowii, part of an undersampled group of deep-sea habitats. PloS One 8 (11), e79917. doi: 10.1371/journal.pone.0079917
Jones C. G., Lawton J. H., Shachak M. (1994). Organisms as ecosystem engineers in ecosystem management (New York: Springer), 130–147.
Laborel J. (1961). Le concrétionnement algal “coralligène” et son importance géomorphologique en méditerranée. Rec. Trav. Stat. Mar. Endoume. 23 (37), 37–60.
Lacaze-Duthiers H. (1897). Faune du golfe du Lion: coralliaires, zoanthaires, sclérodermés (deuxième mémoire). Archives de Zoologie expérimentale et générale, Sér. 3 (5), 1–249 pls. 1-12.
Lesser M. P., Slattery M., Laverick J. H., Macartney K. J., Bridge T. C. (2019). Global community breaks at 60 m on mesophotic coral reefs. Global Ecol. Biogeogr. 28 (10), 1403–1416. doi: 10.1111/geb.12940
Mastrototaro F., Aguilar R., Alvarez H., Blanco J., García S., Montesanto F., et al. (2020). Mesophotic rocks dominated by Diazona violacea: a Mediterranean codified habitat. Eur. Zool. J. 87 (1), 688–695. doi: 10.1080/24750263.2020.1837972
Miccadei E., Mascioli F., Piacentini T. (2011). Quaternary geomorphological evolution of the tremiti islands (Puglia, Italy). Quaternary. Int. 233 (1), 3–15. doi: 10.1016/j.quaint.2010.04.028
Orlic M., Gacic M., Laviolette P. E. (1992). The currents and circulation of the Adriatic Sea. Oceanol. Acta 15 (2), 109–124.
Poli J. X. (1795). Testacea utriusque siciliae eorumque historia et anatome tabulis aeneis illustrata. Parma, Regio Typographeio. 2, i-xlix, 1-2 (75-264), pls 19–39.
Ponti M., Linares C., Cerrano C., Rodolfo Metalpa R., Hoeksema B. W. (2021). Biogenic reefs at risk: facing globally widespread local threats and their interaction with climate change. Front. Mar. Sci. 1688. doi: 10.3389/fmars.2021.793038
Trincardi F., Foglini F., Verdicchio G., Asioli A., Correggiari A., Minisini D., et al. (2007). The impact of cascading currents on the bari canyon system, SW-Adriatic margin (Central Mediterranean). Mar. Geol. 246 (2-4), 208–230. doi: 10.1016/j.margeo.2007.01.013
UNEP-MAP-RAC/SPA (2019). Monitoring protocols of the ecosystem approach common indicators 1 and 2 related to marine benthic habitats in meeting of the ecosystem approach of correspondence group on monitoring (CORMON). Biodivers. Fish. (Rome, Italy). 1–171.
Keywords: marine bioconstructions, mesophotic zone, macrobenthic taxa, central Mediterranean Sea, Ostreida, Scleractinia
Citation: Cardone F, Corriero G, Longo C, Pierri C, Gimenez G, Gravina MF, Giangrande A, Lisco S, Moretti M, De Giosa F, Mercurio M and Marzano CN (2022) A system of marine animal bioconstructions in the mesophotic zone along the Southeastern Italian coast. Front. Mar. Sci. 9:948836. doi: 10.3389/fmars.2022.948836
Received: 20 May 2022; Accepted: 25 July 2022;
Published: 17 August 2022.
Edited by:
Massimo Ponti, University of Bologna, ItalyReviewed by:
Paula Mariela González, University of Buenos Aires, ArgentinaPetar Kružić, University of Zagreb, Croatia
Daniele Grech, International Marine Centre Foundation, Italy
Copyright © 2022 Cardone, Corriero, Longo, Pierri, Gimenez, Gravina, Giangrande, Lisco, Moretti, De Giosa, Mercurio and Marzano. This is an open-access article distributed under the terms of the Creative Commons Attribution License (CC BY). The use, distribution or reproduction in other forums is permitted, provided the original author(s) and the copyright owner(s) are credited and that the original publication in this journal is cited, in accordance with accepted academic practice. No use, distribution or reproduction is permitted which does not comply with these terms.
*Correspondence: Maria Mercurio, maria.mercurio@uniba.it