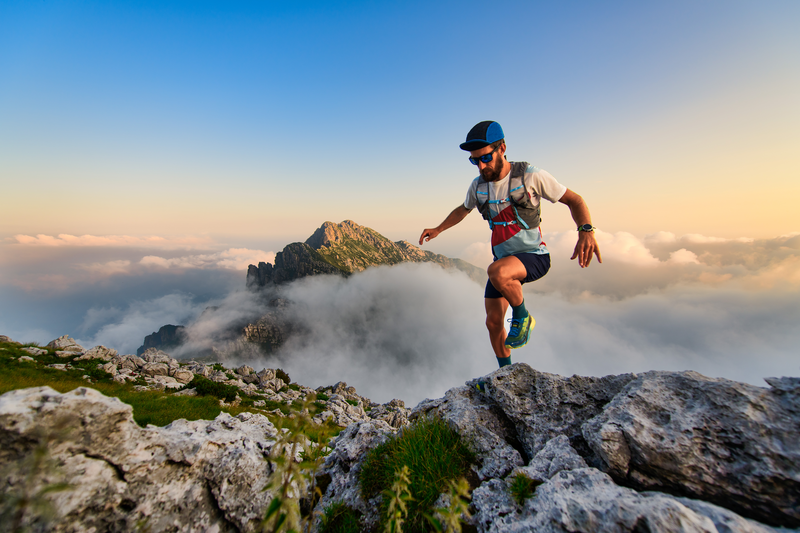
94% of researchers rate our articles as excellent or good
Learn more about the work of our research integrity team to safeguard the quality of each article we publish.
Find out more
ORIGINAL RESEARCH article
Front. Mar. Sci. , 11 November 2022
Sec. Marine Conservation and Sustainability
Volume 9 - 2022 | https://doi.org/10.3389/fmars.2022.947124
This article is part of the Research Topic Aquatic One Health—The Intersection of Marine Wildlife Health, Public Health, and Our Oceans View all 10 articles
The oceans contain trillions of plastic particles, mostly microplastics (i.e., particles < 5 mm diameter; 92.4% of plastic particles), which have been detected in organisms at all levels of the marine food web. The ubiquity of marine plastic debris has created a monumental environmental pollution problem with extensive public health consequences, as more than 40% of the world’s population lives near the coast and shares coastal resources. For decades, common bottlenose dolphins (Tursiops truncatus) have been used as sentinels of marine pollution risks for coastal communities that rely on seafood. Recently, prevalent phthalate exposure was documented in bottlenose dolphins residing in Sarasota Bay, FL, at concentrations exceeding those of human reference populations. While the source of their exposure is uncertain, the types of compounds detected suggest a plastic origin. The objective of this study was to screen for plastic ingestion among free-ranging dolphins in Sarasota Bay using gastric samples collected during catch-and-release health assessments. Gastric samples were collected from seven live bottlenose dolphins in 2019, and suspected microplastic particles were detected in all samples. The number of particles per sample ranged from <10 to >100, and the most common types were transparent films and white foams. Similar to other marine mammal studies, fibers were also present. Given that dolphins are likely exposed to microplastics via contaminated prey, findings from this and additional studies will help to evaluate the potential of contaminated seafood as an additional source of microplastic exposure for humans, as well as help to inform intervention and risk communication needs regarding seafood safety.
Plastics are of increasing concern to environmental health because of their ubiquitous presence in the global oceans (Eriksen et al., 2014), combined with the fact that plastic waste is slow to degrade and therefore persists in the environment (Andrady, 2011). Geyer et al. (2017) estimated a global plastic production of 380 million tons annually, of which approximately 60% ends up in landfills or the natural environment. Marine plastic debris is now recognized as a pollutant of international concern due to impacts on wildlife and seafood safety, stimulating the initiation of a formal plan at the 2015 G7 Summit targeting improvements in the management and reduction of marine litter G-7 Leaders' Declaration (2015).
Factors impacting the degree of marine microplastic pollution include land use (i.e., commercial, industrial, transport), proximity to urban centers, current and water flow, polymer composition, particle characteristics (e.g., density, size; Su et al., 2020), and wastewater treatment practices (Freeman et al., 2020). Coastal microplastic ‘hotspots’ are suspected to occur in urbanized areas near industrial and commercial activities, especially those located downstream of large rivers and waterways (Su et al., 2020). Marine plastic debris is categorized by size (Andrady, 2011). Macro- and mesoplastics (≥ 5mm diameter) often enter the marine environment directly as waste, while marine microplastics (<5mm diameter) may come from wastewater treatment facilities or fragmentation of larger plastic items (Cole et al., 2011; Geyer et al., 2017). Eriksen et al. (2014) estimated that the oceans contain more than 5.25 trillion plastic particles, of which 92.4% are microplastics.
Previous studies have demonstrated microplastic ingestion by filter-feeding and lower-trophic level organisms (Autian, 1973; Farrell and Nelson, 2013; Fossi et al., 2014) as well as translocation of microspheres (1 to 5 µm) into muscle tissue (Zeytin et al., 2020), suggesting the potential for trophic transfer to apex predators. In fact, microplastics have been detected in the stomachs, intestines, and feces of many marine mammal species (Zantis et al., 2021; Table 1), but the health consequences of microplastic exposure are still uncertain. In vitro and in vivo laboratory studies have linked microplastic exposure with gastrointestinal inflammation, altered gut microbiota, metabolic changes, antibiotic resistance, and oxidative stress, among other impacts from particles ranging from nano to 250 µm (Lu et al., 2018; Jin et al., 2019; González-Acedo et al., 2021). Recent evidence from Leslie et al. (2022) suggest that many organ systems can be exposed as plastic polymers can enter and be transported by the bloodstream. The toxic threat of microplastics may be two-fold due to both the plastic particle itself and endocrine disrupting chemical additives (e.g., phthalate acid esters, “phthalates”; Rochman et al., 2019). In fact, plasticizers compose up to 70% of some plastics, and it is anticipated that the demand and market for these additives will continue to grow (Benjamin et al., 2017).
Sarasota Bay, located on the central west coast of Florida, is a semi-closed estuarine system with minimal tidal exchange (SBEP, 2014). As an urban watershed, this region houses multiple residential, industrial, and commercial centers (USF, 2020). Bottlenose dolphins in this area have been the focus of numerous studies since 1970, including recent investigations of phthalate exposure (Hart et al., 2018; Hart et al., 2020; Dziobak et al., 2021). These studies found prevalent exposure among live dolphins sampled during 2010-2019 (75%; n=51; Hart et al., 2020; Dziobak et al., 2021) and heightened exposure to a metabolite that suggests a plastic origin (mono-(2-ethylhexyl) phthalate, MEHP; Hart et al., 2020). We suspect incidental ingestion of microplastics could be a source of phthalate exposure for bottlenose dolphins, evidenced by studies of phthalate exposure in laboratory mice that were fed plastic particles laced with chemical additives (Deng et al., 2020). Additionally, previous studies have demonstrated that plastic particles in general are highly resistant to digestive juices (Stock et al., 2020), suggesting the potential for chronic exposure.
Prior studies investigating microplastic exposure and ingestion among cetaceans (i.e., dolphins, porpoises, whales) have relied primarily upon investigations of gastrointestinal tracts from dead stranded, or bycaught animals (Lusher et al., 2015; Lusher et al., 2018; Nelms et al., 2019; Zantis et al., 2021; Table 1), as well as computer simulation models that predicted geospatial overlap of microplastic contaminated waters and large-whale foraging areas (Fossi et al., 2017). Additionally, most of the studies on marine mammal exposure to microplastics have focused on pinniped species and regions in Europe (Hudak and Sette, 2019; Zantis et al., 2021). To our knowledge, this the first study to screen for ingested microplastics in free-ranging bottlenose dolphins, and one of the few studies characterizing marine mammal microplastic exposure in the southeastern United States. Using gastric samples collected from long-term resident bottlenose dolphins inhabiting an urban estuary (i.e., Sarasota Bay, Florida), the objective of this descriptive study was to screen for evidence of microplastic ingestion and evaluate the characteristics of ingested microplastics (e.g., shape, color, quantity, size). Findings from this study will provide a foundation for a future systematic assessment of ingested microplastics in Sarasota Bay dolphins and contribute to a larger effort to understand potential sources of phthalate exposure among Sarasota Bay dolphins.
Bottlenose dolphins have been the focus of extensive study in Sarasota Bay, FL since 1970 to understand the behavior, movement patterns, and health of the approximately 170 individuals that inhabit the region year-round, over decades and across generations. Using well-established catch-and-release techniques developed and refined over more than 50 years, dolphins were encircled by a net and temporarily restrained to collect biological samples indicative of an individual’s health (Wells et al., 2004). Gastric samples were collected by passing a small tube (9-15mm diameter) through the esophagus into the stomach (Twiner et al., 2011), and gastric fluid was stored in amber glass jars and frozen at -20°C until analysis. Approximately 20mL of gastric fluid was collected from each dolphin. Environmental blanks were collected by passing deionized water through the stomach tubes prior to the collection of dolphin samples. One environmental blank was collected per sampled dolphin to control for potential field contamination. All samples for this study came from bottlenose dolphin health assessments that were conducted during June 3 -June 14, 2019. Samples for this study were collected under Scientific Research Permit No. 20455 from the National Oceanic and Atmospheric Administration’s (NOAA) National Marine Fisheries Service (NMFS). All catch-and-release and sampling methodologies for the health assessments were reviewed and approved by Mote Marine Laboratory’s Institutional Animal Care and Use Committee (IACUC).
For the processing of microplastics, bottlenose dolphin gastric samples were emptied into a 250 mL glass beaker. Organic (non-plastic) material in the samples was digested by adding 150 mL of KOH (10% solution) and incubated at 60°C for 24 hours (Valton et al., 2014). Following digestion, samples were vacuum filtered onto GF/A 1.6 µm glass fiber filters in a fume hood. Samples were left to dry in covered glass petri dishes. Particles of at least 35 µm in size were characterized visually using a dissection microscope (Leica EZ4, magnification 8-35x) according to physical attributes including shape (e.g., fiber, film, fragment, foam), surface texture, and color (e.g., transparent, blue, black; Shim et al., 2017). Various parameters were examined to indicate potential plastic material. Suspected plastic fibers were indicated by a smooth, uniform surface with a length that exceeded the width (Lusher et al., 2020). Suspected plastic fragments were indicated by smooth or angular edges that appeared to be broken from a larger piece of debris (Lusher et al., 2020). Fragments were further categorized as films if they were flexible and able to be folded, or foams if they were distorted when physically handled, but eventually returned to their original shape (Lusher et al., 2020). Particles (at least 100 µm in size) were tested with a hot needle (250°C) and suspected to be of plastic origin if the needle melted or left a mark on the particle surface (De Witte et al., 2014; Devriese et al., 2015). A subsample of suspected plastic particles ranging between 500 µm and 5mm in diameter was further examined by Fourier Transform Infrared (FTIR) spectroscopy to determine composition. Particles were analyzed using a Nicolet iS20 FTIR (Thermo Scientific) with diamond attenuated total reflectance (ATR). Recorded spectra were processed and matched to a reference spectral library using Open Specy Software (Cowger et al., 2021). Polymers were noted if the spectral match was at least 50%.
Rigorous precautions were taken while handling and processing samples. A 100% cotton lab coat and nitrile gloves were worn during laboratory analyses. All tools and glassware were carefully rinsed with distilled water that was filtered through 90 mm GF/A 1.6 µm glass fiber filters. For QA/QC purposes, one lab/procedural blank extraction without tissue was performed simultaneously with each set of sample digestions to correct for potential procedural contamination, and three positive controls with commercially purchased polyethylene, polystyrene, and polyester microplastic particles were used to determine recovery efficiency. Mean recovery percentages were 90% for film, 87% for foam, and 90% for fibers. During the dolphin health assessments, environmental blanks were collected concurrently with gastric fluid, using the same sampling methods and equipment. Also, the sample collection and processing teams wore green cotton shirts, and the caps for the glass sampling tubes were only removed once the gastric sample was collected from the dolphin.
Methods to characterize microplastic exposure in bottlenose dolphins followed the methods of Nelms et al. (2019) and best practice recommendations by Zantis et al. (2021). Briefly, we estimated the number of suspected microplastic particles per gastric sample, calculated the proportion of samples containing at least one suspected plastic particle, and identified key physical characteristics (e.g., shape, color). On a random subset of suspected plastic particle types (e.g., films, foams, fibers), we assessed polymer composition via FTIR and measured particle dimensions. As this study was exploratory and sampling volumes were not standardized, systematic exposure assessments were not performed for each individual. Further, given the small sample size, statistical comparisons between age groups or sexes were not performed.
Gastric samples were collected from seven free-ranging, long-term resident bottlenose dolphins during health assessments in June 2019. Four of the dolphins were females, and ages ranged from 4 to 44 years (Table 2). Dolphins were sampled from four locations throughout Sarasota Bay: 1) one dolphin in Palma Sola Bay; 2) one dolphin near Tidy Island; 3) three dolphins near the southern end of Longboat Key; 4) two dolphins near the northern end of Siesta Key (Figure 1; Table 2).
Table 2 Characteristics of bottlenose dolphins sampled in Sarasota Bay, Florida during health assessments (2019).
Figure 1 Locations of sampling sites for Bottlenose Dolphins Sampled during 2019 Catch-and-Release Health Assessments in Sarasota Bay, Florida.
Of the total 92 particle types remaining in samples after digestion and filtration, 62% (n=57) were of suspected plastic origin, based on the hot needle test. All suspected plastic particles examined were less than 5mm in diameter, suggesting microplastic ingestion in bottlenose dolphins. At least one suspected microplastic particle was observed in gastric samples of every dolphin (Table 3). Suspected microplastic quantity was variable across dolphin samples (less than 10 to greater than 100), and particle types included several colors of films, fibers, and foams (Figures 2–4; Table 3). Transparent films and white foams were the most commonly observed particle types in bottlenose dolphin gastric samples (Table 3). FTIR was not conducted on fibers due to their small widths, and many of the white foams were too small to yield conclusive results. However, polymers (e.g., PVC zinc, polyethylene, polyamide) were detected in transparent films, providing evidence of microplastic ingestion in five of the seven dolphins (Table 4).
Table 3 Microplastic characteristics in environmental/laboratory blanks and gastric samples collected during catch and release bottlenose dolphin health assessments conducted in Sarasota Bay, FL, USA (June 2019).
Figure 2 Example of White Foam from a Gastric Sample of a Bottlenose Dolphin Sampled in Sarasota Bay, Florida (2019).
Figure 3 Example of Transparent Film from a Gastric Sample of a Bottlenose Dolphin Sampled in Sarasota Bay, Florida (2019).
Figure 4 Example of Blue Fiber from a Gastric Sample of a Bottlenose Dolphin Sampled in Sarasota Bay, Florida (2019).
Suspected plastic particles were also present in all environmental and laboratory/procedural blanks, ranging from one to 12 particles per blank (Tables 3, 4). The majority of suspected plastic particles in the environmental and laboratory blanks were fibers; transparent films and foams were not observed in any of the blanks (Tables 3, 4).
Overall, our findings are consistent with previous studies. In a review of 30 publications reporting results of microplastics screening of gastrointestinal tracts (primarily from dead stranded or bycaught cetaceans) and scat (primarily from live pinnipeds), microplastics were highly prevalent (Zantis et al., 2021). In our study, all dolphin samples contained at least one suspected plastic particle, and most contained more than 50 particles. Particle types observed in Sarasota Bay dolphin samples were similar to those reported in previous studies, including fibers, foams, and films. However, previous studies reported a predominance of fibers (Zantis et al., 2021; Table 1), while foams and films dominated the particle types ingested by Sarasota Bay dolphins (Table 3). Our findings agree with previous cetacean studies in which blue and black fibers were commonly observed (Zantis et al., 2021), but the most abundant particle colors in Sarasota Bay dolphin samples were transparent and white.
Given widespread reports of microplastic ingestion among many stranded cetaceans of a variety of species (Table 1), it is not entirely surprising to detect evidence of microplastic ingestion in Sarasota Bay dolphins. Although there are no published microplastic surveys for Sarasota Bay, microplastic contamination along the coastal regions of the Gulf of Mexico is among the highest found worldwide, with reported concentrations ranging from 60-1,940 items/kg in sediment, 12-381 particles/L in water, and 1.3-4.7 particles per fish (reviewed by Shruti et al., 2021). Microplastic concentrations in sediments, which serve as a sink for these particles, were strongly influenced by degree of urbanization (Shruti et al., 2021). Further, a study conducted in Tampa Bay, which is directly north of Sarasota Bay, estimated approximately 4 billion particles in the bay (McEachern et al., 2019). As a result, it is reasonable to expect similar levels of contamination in Sarasota Bay.
The differences in particle characteristics observed between Sarasota Bay dolphins and previous cetacean studies could possibly be explained by geography, sample type (gastric fluid vs. intestine), or retention/passage time. For example, Giani et al. (2019) found geographic differences in the composition of microplastic types among fish inhabiting distinct regions of the Mediterranean Sea. Also, given the likelihood for trophic exposure (Nelms et al., 2018), the types of particles observed in Sarasota Bay dolphins could also be attributed to geographic differences in prey distribution. Alternatively, Nelms et al. (2019) note that microplastic distribution is inconsistent across the gastrointestinal tract, resulting in differences that may be dependent on the anatomical location of sampling (e.g., stomach vs. intestine). It also seems plausible that differences in suspected microplastic characteristics observed in this study could be attributed to differences in retention time for different types of microplastics. For example, in a study comparing ingested microplastic characteristics between grey seals (Halichoerus grypus) and their prey fish, Nelms et al. (2018) found that microplastic fragments were most common in seal scat samples, while fish gastrointestinal tracts were dominated by fibers. Perhaps foams and fragments are egested quickly, whereas fibers may accumulate in the intestinal tract.
One of the limitations in microplastic research is the recurring issue of sample contamination by ambient microplastics during field collection or sample processing (Foekema et al., 2013). Despite our QA/QC efforts, suspected plastic particles (primarily fibers) were observed in nearly all laboratory and environmental blanks, suggesting the possibility of dolphin sample contamination (Tables 3, 4). To help control for contamination levels in the field, environmental blanks were collected and processed in a manner identical to that of the gastric samples. Furthermore, while we were not able to control for synthetic clothing during field collection, the individuals involved in the sample collection and sample processing wore bright green shirts, and green fibers were not prevalent in gastric samples or associated environmental blanks. While overlap in fiber color between the gastric samples and both sets of blanks could provide evidence of sample contamination, the rate of contamination in these samples was less than 10% of the total number of suspected microplastics in the gastric samples (Table 3). Additionally, clear films and white foams were not observed in any blank, suggesting true ingestion of these particles by Sarasota bottlenose dolphins.
Although suspected microplastics were observed in every sample collected from Sarasota Bay dolphins, plastic ingestion could only be confirmed in five dolphins due to size limitations of FTIR. Additionally, given that blue and black fibers were observed in gastric and blank samples, laboratory or environmental contamination cannot be ruled out, particularly for dolphin “F266” (Table 3). It is difficult to contextualize microplastic exposure in Sarasota Bay bottlenose dolphins due to differences in sample type and collection methods across research studies, as well as limitations within our own study. For example, considering that our analyses focused only on particles contained in gastric fluid, results presented herein are an underestimate of trophic consumption since particles may adhere to, or be trapped in, tissue lining the gastrointestinal tract. Secondly, understanding individual dosage is complicated by the fact that repeated sampling of individuals is not allowable by permit or feasible since gastric samples were taken opportunistically as other health-related samples and measurements were being obtained. As this is the first study to collect gastric samples from live free-ranging bottlenose dolphins for microplastic screening, future efforts will be made to facilitate microplastic exposure assessments. For example, standardized volumes will be collected to calculate and compare microplastic load between individuals and demographic cohorts (Zantis et al., 2021). Additionally, particle sizes will be systematically measured, and suspected plastic particles identified by the hot needle test will be confirmed using FTIR or Raman spectroscopy, which will enable the determination of polymer composition for suspected particles of a broader size range.
Our recent studies detected prevalent phthalate exposure among free-ranging bottlenose dolphins in Sarasota Bay, FL (~75%; n=51; 2010-2019; Hart et al., 2020; Dziobak et al., 2021), which is located in the tenth fastest-growing metropolitan region in the United States (U.S. Census Bureau, 2017). The most commonly detected metabolites were monoethyl phthalate (MEP), and mono-(2-ethylhexyl) phthalate (MEHP; Dziobak et al., 2021), which are metabolites of parent compounds commonly added to personal care products and plastic. In fact, Sarasota Bay bottlenose dolphins had significantly higher concentrations of MEHP, the metabolite of di-(2-ethylhexyl) phthalate (DEHP), than human reference populations (Hart et al., 2020). This finding is significant as DEHP is most commonly used in polyvinyl chloride products, food packaging, wire covering, toys, and medical tubing (ATSDR, 2002). While the source of this phthalate exposure is uncertain for bottlenose dolphins, our findings suggest a plastic origin. The link between MEHP detection and DEHP contamination is not clear, but previous studies demonstrated that MEHP concentrations measured in blubber and muscle samples from fin whales and basking sharks were higher in individuals sampled in regions with significantly higher water and plankton microplastic concentrations (Fossi et al., 2012; Fossi et al., 2014).
The ubiquity of plastic has created a monumental environmental pollution problem. The public health consequences of this extensive pollution are significant, as an estimated 41% of the world’s population lives within 100 km of the coast (Martínez et al., 2007). As apex predators with a long lifespan (>60 years; Wells, 2014), year-round resident estuarine bottlenose dolphins can serve as gauges to detect disturbances in their local environment (Wells et al., 2004). This has been demonstrated by epidemiologic studies of exposure to chemical contaminants (e.g., polychlorinated biphenyls, “PCBs”; Wells et al., 2005; Schwacke et al., 2012), toxins produced during harmful algal blooms (Twiner et al., 2011), and oil-associated compounds (Schwacke et al., 2014; Smith et al., 2017), making them excellent sentinels of environmental contaminant exposure for human communities, particularly coastal communities that rely on seafood as a primary source of food or economic export (Backer et al., 2019). In fact, Backer et al. (2019) suggest that because of trophic concurrence, dolphins can warn of local environmental pollution risks for coastal human populations. Findings from this research may assist with efforts to monitor seafood contamination and help to inform intervention and risk communication needs regarding seafood safety.
The datasets presented in this study can be found in online repositories. The names of the repository/repositories and accession number(s) can be found below: The datasets generated for this study can be found in the 4TU.Research Data international data repository for science, engineering, and design. https://doi.org/10.4121/19768477.v1.
The animal study was reviewed and approved by Mote Marine Laboratory’s Institutional Animal Care and Use Committee (IACUC). Samples for this study were collected under Scientific Research Permit No. 20455 from the National Oceanic and Atmospheric Administration’s (NOAA) National Marine Fisheries Service (NMFS).
Conceptualizaton: LH. Data Curation: MD, BE, RW. Formal Analysis: MD, BE, LH. Funding Acquisition: LH, RW. Investigation: MD, RW, LH. Methodology: MD, BE, LH, JW. Project Administration: LH, RW. Resources: BE, JW, RW. Supervision: JW, BE, RW, LH. Validation: MD, BE. Visualization: MD, LH. Writing Original Draft: LH, MD. Writing Review and Editing: RW, BE, JW. All authors contributed to the article and approved the submitted version.
Dolphin samples were obtained through health assessments supported primarily by Dolphin Quest, Inc., and microplastic analysis was supported by research and development funding from the Department of Health and Human Performance at the College of Charleston. This study received funding from Dolphin Quest, Inc. The funder was not involved in the study design, collection, analysis, interpretation of data, the writing of this article or the decision to submit it for publication.
We are grateful to the staff, collaborators, and volunteers of the Sarasota Dolphin Research Program for ensuring the safe capture, sampling, and release of the dolphins. Additionally, we would like to thank Christina Toms and Amanda Moors for assistance with the collection of gastric samples and environmental blanks for this study.
The authors declare that the research was conducted in the absence of any commercial or financial relationships that could be construed as a potential conflict of interest.
All claims expressed in this article are solely those of the authors and do not necessarily represent those of their affiliated organizations, or those of the publisher, the editors and the reviewers. Any product that may be evaluated in this article, or claim that may be made by its manufacturer, is not guaranteed or endorsed by the publisher.
Andrady A. L. (2011). Microplastics in the marine environment. Mar. Pollut. Bull. 62 (8), 1596–1605. doi: 10.1016/j.marpolbul.2011.05.030
ATSDR (2002). Toxicological profile for di(2-ethylhexyl)phthalate (Atlanta, Ga: U.S. Dept. of Health and Human Services, Public Health Service, Agency for Toxic Substances and Disease Registry).
Autian J. (1973). Toxicity and health threats of phthalate esters: review of the literature. Environ. Health Perspect. 4, 3–26. doi: 10.1289/ehp.73043
Backer L. C., Bolton B., Litz J. A., Trevillian J., Kieszak S., Kucklick J. (2019). Environmental contaminants in coastal populations: Comparisons with the national health and nutrition examination survey (NHANES) and resident dolphins. Sci. Total Environ. 696, 134041. doi: 10.1016/j.scitotenv.2019.134041
Battaglia F. M., Beckingham B. A., McFee W. E. (2020). First report from north America of microplastics in the gastrointestinal tract of stranded bottlenose dolphins (Tursiops truncatus). Mar. Pollut. Bull. 160, 111677. doi: 10.1016/j.marpolbul.2020.111677
Benjamin S., Masai E., Kamimura N., Takahashi K., Anderson R. C., Faisal P. A. (2017). Phthalates impact human health: Epidemiological evidences and plausible mechanism of action. J. Hazardous Materials 340, 360–383. doi: 10.1016/j.jhazmat.2017.06.036
Besseling E., Foekema E. M., Van Franeker J. A., Leopold M. F., Kühn S., Rebolledo E. B., et al. (2015). Microplastic in a macro filter feeder: Humpback whale megaptera novaeangliae. Mar. Pollut. Bull. 95 (1), 248–252. doi: 10.1016/j.marpolbul.2015.04.007
Cole M., Lindeque P., Halsband C., Galloway T. S. (2011). Microplastics as contaminants in the marine environment: A review. Mar. Pollut. Bull. 62 (12), 2588–2597. doi: 10.1016/j.marpolbul.2011.09.025
Cowger W., Steinmetz Z., Gray A., Munno K., Lynch J., Hapich H., et al. (2021). Microplastic spectral classification needs an open source community: Open specy to the rescue! Analytical Chem. 93 (21), 7543–7548. doi: 10.1021/acs.analchem.1c00123
Deng Y., Yan Z., Shen R., Wang M., Huang Y., Ren H., et al. (2020). Microplastics release phthalate esters and cause aggravated adverse effects in the mouse gut. Environ. Int. 143, 105916. doi: 10.1016/j.envint.2020.105916
Devriese L. I., van der Meulen M. D., Maes T., Bekaert K., Paul-Pont I., Frère L., et al. (2015). Microplastic contamination in brown shrimp (Crangon crangon, Linnaeus 1758) from coastal waters of the southern north Sea and channel area. Mar. Pollut. Bull. 98 (1-2), 179–187. doi: 10.1016/j.marpolbul.2015.06.051
De Witte B., Devriese L., Bekaert K., Hoffman S., Vandermeersch G., Cooreman K., et al. (2014). Quality assessment of the blue mussel (Mytilus edulis): Comparison between commercial and wild types. Mar. Pollut. Bull. 85 (1), 146–155. doi: 10.1016/j.marpolbul.2014.06.006
Dziobak M. K., Wells R. S., Pisarski E. C., Wirth E. F., Hart L. B. (2021). Demographic assessment of mono (2-ethylhexyl) phthalate (MEHP) and monoethyl phthalate (MEP) concentrations in common bottlenose dolphins (Tursiops truncatus) from Sarasota bay, FL, USA. GeoHealth 5, e2020GH000348. doi: 10.1029/2020GH000348
Eriksen M., Lebreton L. C., Carson H. S., Thiel M., Moore C. J., Borerro J. C., et al. (2014). Plastic pollution in the world's oceans: More than 5 trillion plastic pieces weighing over 250,000 tons afloat at sea. PLoS One 9 (12), e111913. doi: 10.1371/journal.pone.0111913
Farrell P., Nelson K. (2013). Trophic level transfer of microplastic: Mytilus edulis (L.) to carcinus maenas (L.). Environ. pollut. 177, 1–3. doi: 10.1016/j.envpol.2013.01.046
Foekema E. M., De Gruijter C., Mergia M. T., van Franeker J. A., Murk A. J., Koelmans A. A. (2013). Plastic in north sea fish. Environ. Sci. Technol. 47 (15), 8818–8824. doi: 10.1021/es400931b
Fossi M. C., Coppola D., Baini M., Giannetti M., Guerranti C., Marsili L., et al. (2014). Large Filter feeding marine organisms as indicators of microplastic in the pelagic environment: The case studies of the Mediterranean basking shark (Cetorhinus maximus) and fin whale (Balaenoptera physalus). Mar. Environ. Res. 100, 17–24. doi: 10.1016/j.marenvres.2014.02.002
Fossi M. C., Panti C., Guerranti C., Coppola D., Giannetti M., Marsili L., et al. (2012). Are baleen whales exposed to the threat of microplastics? a case study of the Mediterranean fin whale (Balaenoptera physalus). Mar. Pollut. Bull. 64 (11), 2374–2379. doi: 10.1016/j.marpolbul.2012.08.013
Fossi M. C., Romeo T., Baini M., Panti C., Marsili L., Campani T., et al. (2017). Plastic debris occurrence, convergence areas and fin whales feeding ground in the Mediterranean marine protected area pelagos sanctuary: A modeling approach. Front. Mar. Sci. 4, 167. doi: 10.3389/fmars.2017.00167
Freeman S., Booth A. M., Sabbah I., Tiller R., Dierking J., Klun K., et al. (2020). Between source and sea: The role of wastewater treatment in reducing marine microplastics. J. Environ. Manage. 266, 110642. doi: 10.1016/j.jenvman.2020.110642
G-7 Leaders' Declaration (2015). Washington: Federal information & news dispatch, LLC. retrieved from research library prep. Available at: https://search.proquest.com/docview/1690245200.
Geyer R., Jambeck J. R., Law K. L. (2017). Production, use, and fate of all plastics ever made. Sci. Adv. 3 (7), e1700782. doi: 10.1126/sciadv.1700782
Giani D., Baini M., Galli M., Casini S., Fossi M. C. (2019). Microplastics occurrence in edible fish species (Mullus barbatus and merluccius merluccius) collected in three different geographical sub-areas of the Mediterranean Sea. Mar. Pollut. Bull. 140, 129–137. doi: 10.1016/j.marpolbul.2019.01.005
González-Acedo A., García-Recio E., Illescas-Montes R., Ramos-Torrecillas J., Melguizo-Rodríguez L., Costela-Ruiz V. J. (2021). Evidence from in vitro and in vivo studies on the potential health repercussions of micro-and nanoplastics. Chemosphere 280, 130826. doi: 10.1016/j.chemosphere.2021.130826
Hart L. B., Beckingham B., Wells R. S., Alten Flagg M., Wischusen K., Moors A., et al. (2018). Urinary phthalate metabolites in common bottlenose dolphins (Tursiops truncatus) from Sarasota bay, FL, USA. GeoHealth 2 (10), 313–326. doi: 10.1029/2018GH000146
Hart L. B., Dziobak M. K., Pisarski E. C., Wirth E. F., Wells R. S. (2020). Sentinels of synthetics–a comparison of phthalate exposure between common bottlenose dolphins (Tursiops truncatus) and human reference populations. PLoS One 15 (10), e0240506. doi: 10.1371/journal.pone.0240506
Hernandez-Gonzalez A., Saavedra C., Gago J., Covelo P., Santos M. B., Pierce G. J. (2018). Microplastics in the stomach contents of common dolphin (Delphinus delphis) stranded on the Galician coasts (NW Spain 2005–2010). Mar. Pollut. Bull. 137, 526–532. doi: 10.1016/j.marpolbul.2018.10.026
Hudak C. A., Sette L. (2019). Opportunistic detection of anthropogenic micro debris in harbor seal (Phoca vitulina vitulina) and gray seal (Halichoerus grypus atlantica) fecal samples from haul-outs in southeastern Massachusetts, USA. Mar. Pollut. Bull. 145, 390–395. doi: 10.1016/j.marpolbul.2019.06.020
Jin Y., Lu L., Tu W., Luo T., Fu Z. (2019). Impacts of polystyrene microplastic on the gut barrier, microbiota and metabolism of mice. Sci. Total Environ. 649, 308–317. doi: 10.1016/j.scitotenv.2018.08.353
Leslie H. A., Van Velzen M. J., Brandsma S. H., Vethaak A. D., Garcia-Vallejo J. J., Lamoree M. H. (2022). Discovery and quantification of plastic particle pollution in human blood. Environ. Int. 163, 107199. doi: 10.1016/j.envint.2022.107199
Lusher A. L., Bråte I. L. N., Munno K., Hurley R. R., Welden N. A. (2020). Is it or isn’t it: The importance of visual classification in microplastic characterization. Appl. Spectrosc. 74 (9), 1139–1153. doi: 10.1177/0003702820930733
Lusher A. L., Hernandez-Milian G., Berrow S., Rogan E., O'Connor I. (2018). Incidence of marine debris in cetaceans stranded and bycaught in Ireland: Recent findings and a review of historical knowledge. Environ. pollut. 232, 467–476. doi: 10.1016/j.envpol.2017.09.070
Lusher A. L., Hernandez-Milian G., O'Brien J., Berrow S., O'Connor I., Officer R. (2015). Microplastic and macroplastic ingestion by a deep diving, oceanic cetacean: The true's beaked whale mesoplodon mirus. Environ. pollut. 199, 185–191. doi: 10.1016/j.envpol.2015.01.023
Lu L., Wan Z., Luo T., Fu Z., Jin Y. (2018). Polystyrene microplastics induce gut microbiota dysbiosis and hepatic lipid metabolism disorder in mice. Sci. Total Environ. 631, 449–458. doi: 10.1016/j.scitotenv.2018.03.051
Martínez M. L., Intralawan A., Vázquez G., Pérez-Maqueo O., Sutton P., Landgrave R. (2007). The coasts of our world: Ecological, economic and social importance. Ecol. Economics 63 (2-3), 254–272. doi: 10.1016/j.ecolecon.2006.10.022
McEachern K., Alegria H., Kalagher A. L., Hansen C., Morrison S., Hastings D. (2019). Microplastics in Tampa bay, Florida: Abundance and variability in estuarine waters and sediments. Mar. Pollut. Bull. 148, 97–106. doi: 10.1016/j.marpolbul.2019.07.068
Moore R. C., Loseto L., Noel M., Etemadifar A., Brewster J. D., MacPhee S., et al. (2020). Microplastics in beluga whales (Delphinapterus leucas) from the Eastern Beaufort Sea. Mar. Pollut. Bull. 150, 110723. doi: 10.1016/j.marpolbul.2019.110723
Nelms S. E., Barnett J., Brownlow A., Davison N. J., Deaville R., Galloway T. S., et al. (2019). Microplastics in marine mammals stranded around the British coast: ubiquitous but transitory? Sci. Rep. 9 (1), 1–8. doi: 10.1038/s41598-018-37428-3
Nelms S. E., Galloway T. S., Godley B. J., Jarvis D. S., Lindeque P. K. (2018). Investigating microplastic trophic transfer in marine top predators. Environ. pollut. 238, 999–1007. doi: 10.1016/j.envpol.2018.02.016
Philipp C., Unger B., Ehlers S. M., Koop J. H., Siebert U. (2021). First evidence of retrospective findings of microplastics in harbour porpoises (Phocoena phocoena) from German waters. Front. Mar. Sci. 8, 508. doi: 10.3389/fmars.2021.682532
Rochman C. M., Brookson C., Bikker J., Djuric N., Earn A., Bucci K., et al. (2019). Rethinking microplastics as a diverse contaminant suite. Environ. Toxicol. Chem. 38 (4), 703–711. doi: 10.1002/etc.4371
SBEP (2014) Sarasota Bay comprehensive conservation and management plan update and state of the bay report. Available at: https://www.epa.gov/sites/production/files/2015-09/documents/ccmp.stateofthebay-for-website-august2014.pdf.
Schwacke L. H., Smith C. R., Townsend F. I., Wells R. S., Hart L. B., Balmer B. C., et al. (2014). Health of common bottlenose dolphins (Tursiops truncatus) in barataria bay, Louisiana, following the deepwater horizon oil spill. Environ. Sci. Technol. 48 (1), 93–103. doi: 10.1021/es403610f
Schwacke L. H., Zolman E. S., Balmer B. C., De Guise S., George R. C., Hoguet J., et al. (2012). Anaemia, hypothyroidism and immune suppression associated with polychlorinated biphenyl exposure in bottlenose dolphins (Tursiops truncatus). Proc. R. Soc. B: Biol. Sci. 279 (1726), 48–57. doi: 10.1098/rspb.2011.0665
Shim W. J., Hong S. H., Eo S. E. (2017). Identification methods in microplastic analysis: A review. Analytical Methods 9 (9), 1384–1391. doi: 10.1039/C6AY02558G
Shruti V. C., Pérez-Guevara F., Kutralam-Muniasamy G. (2021). The current state of microplastic pollution in the world's largest gulf and its future directions. Environ. pollut. 291, 118142. doi: 10.1016/j.envpol.2021.118142
Smith C. R., Rowles T. K., Hart L. B., Townsend F. I., Wells R. S., Zolman E. S., et al. (2017). Slow recovery of Barataria Bay dolphin health following the Deepwater Horizon oil spill, (2013-2014), with evidence of persistent lung disease and impaired stress response. Endangered Species Res. 33, 127–142. doi: 10.3354/esr00778
Stock V., Fahrenson C., Thuenemann A., Dönmez M. H., Voss L., Böhmert L., et al. (2020). Impact of artificial digestion on the sizes and shapes of microplastic particles. Food Chem. Toxicol. 135, 111010. doi: 10.1016/j.fct.2019.111010
Su L., Sharp S. M., Pettigrove V. J., Craig N. J., Nan B., Du F., et al. (2020). Superimposed microplastic pollution in a coastal metropolis. Water Res. 168, 115140. doi: 10.1016/j.watres.2019.115140
Twiner M. J., Fire S., Schwacke L., Davidson L., Wang Z., Morton S., et al. (2011). Concurrent exposure of bottlenose dolphins (Tursiops truncatus) to multiple algal toxins in Sarasota bay, Florida, USA. PLoS One 6 (3), e17394. doi: 10.1371/journal.pone.0017394
U.S. Census Bureau (2017). Press kit: County and metro area population estimates. table 4: Top 25 fastest-growing metro areas (percent change): July 1, 2015 to july 1, 2016. Available at: https://www.census.gov/content/dam/Census/newsroom/press-kits/2017/Top%2025%20Fastest%20Metros.pdf.
USF (2020). Sarasota Bay watershed. Available at: https://www.sarasota.wateratlas.usf.edu/watershed/geography.asp?wshedid=5&wbodyatlas=watershed (Accessed 2.14.21).
Valton A. S., Serre-Dargnat C., Blanchard M., Alliot F., Chevreuil M., Teil M. J. (2014). Determination of phthalates and their by-products in tissues of roach (Rutilus rutilus) from the orge river (France). Environ. Sci. pollut. Res. 21 (22), 12723–12730. doi: 10.1007/s11356-014-3213-0
Van Franeker J. A., Bravo Rebolledo E. L., Hesse E., IJsseldijk L. L., Kühn S., Leopold M., et al. (2018). Plastic ingestion by harbour porpoises Phocoena phocoena in the Netherlands: Establishing a standardised method. Ambio 47 (4), 387–397. doi: 10.1007/s13280-017-1002-y
Wells R. S. (2014). “Social structure and life history of bottlenose dolphins near Sarasota bay, Florida: insights from four decades and five generations,” in Primates and cetaceans (Tokyo: Springer), 149–172.
Wells R. S., Rhinehart H. L., Hansen L. J., Sweeney J. C., Townsend F. I., Stone R., et al. (2004). Bottlenose dolphins as marine ecosystem sentinels: developing a health monitoring system. EcoHealth 1 (3), 246–254. doi: 10.1007/s10393-004-0094-6
Wells R. S., Tornero V., Borrell A., Aguilar A., Rowles T. K., Rhinehart H. L., et al. (2005). Integrating life-history and reproductive success data to examine potential relationships with organochlorine compounds for bottlenose dolphins (Tursiops truncatus) in Sarasota bay, Florida. Sci. Total Environ. 349 (1-3), 106–119. doi: 10.1016/j.scitotenv.2005.01.010
Xiong X., Chen X., Zhang K., Mei Z., Hao Y., Zheng J., et al. (2018). Microplastics in the intestinal tracts of East Asian finless porpoises (Neophocaena asiaeorientalis sunameri) from yellow Sea and bohai Sea of China. Mar. Pollut. Bull. 136, 55–60. doi: 10.1016/j.marpolbul.2018.09.006
Zantis L. J., Carroll E. L., Nelms S. E., Bosker T. (2021). Marine mammals and microplastics: A systematic review and call for standardisation. Environ. pollut. 269, 116142. doi: 10.1016/j.envpol.2020.116142
Zeytin S., Wagner G., Mackay-Roberts N., Gerdts G., Schuirmann E., Klockmann S., et al. (2020). Quantifying microplastic translocation from feed to the fillet in European sea bass dicentrarchus labrax. Mar. Pollut. Bull. 156, 111210. doi: 10.1016/j.marpolbul.2020.111210
Keywords: plastic, contaminant, pollution, cetacean, marine mammal
Citation: Hart LB, Dziobak M, Wells RS, Ertel B and Weinstein J (2022) Microplastics in gastric samples from common bottlenose dolphins (Tursiops truncatus) residing in Sarasota Bay FL (USA). Front. Mar. Sci. 9:947124. doi: 10.3389/fmars.2022.947124
Received: 18 May 2022; Accepted: 24 October 2022;
Published: 11 November 2022.
Edited by:
Stephanie Plön, Stellenbosch University, South AfricaReviewed by:
Olga Pantos, Institute of Environmental Science and Research (ESR), New ZealandCopyright © 2022 Hart, Dziobak, Wells, Ertel and Weinstein. This is an open-access article distributed under the terms of the Creative Commons Attribution License (CC BY). The use, distribution or reproduction in other forums is permitted, provided the original author(s) and the copyright owner(s) are credited and that the original publication in this journal is cited, in accordance with accepted academic practice. No use, distribution or reproduction is permitted which does not comply with these terms.
*Correspondence: Leslie B. Hart, aGFydGxiQGNvZmMuZWR1; Miranda Dziobak, bWR6aW9iYWtAZW1haWwuc2MuZWR1
Disclaimer: All claims expressed in this article are solely those of the authors and do not necessarily represent those of their affiliated organizations, or those of the publisher, the editors and the reviewers. Any product that may be evaluated in this article or claim that may be made by its manufacturer is not guaranteed or endorsed by the publisher.
Research integrity at Frontiers
Learn more about the work of our research integrity team to safeguard the quality of each article we publish.