- 1Marine Biology Station, National Institute of Biology, Piran, Slovenia
- 2Faculty of Maritime Studies and Transport, University of Ljubljana, Portoroz, Slovenia
A mid-term study (2003-2013) of polycyclic aromatic hydrocarbons in Gulf of Trieste sediments was conducted at seven sampling sites. The PAH spatial and temporal distribution and origin were determined. The sediments were sampled with a gravity core sampler. After extraction of PAH, their concentrations were determined by gas chromatography. The results of the study show PAH spatial and temporal variability. The highest mean concentration was measured in the marina of Portoroz (1168 ng/g dw), and the lowest at an offshore site (320 ng/g dw). The results show higher concentrations of PAH in coastal areas and a decreasing trend towards the central part of the Gulf of Trieste. The interannual differences in the concentrations of PAH showed a significant downward trend in the marina of Portorož, in the port of Koper and in the offshore site off Piran. No trend was observed at all other sites. Various evaluation indices showed that PAH are predominantly of pyrolytic origin, with a lower proportion of petrogenic origin, especially in the Bay of Koper. The ecological risk assessment found that concentrations of PAH are well below levels associated with adverse biological effects. According to the presented results, this area can be considered as only moderately contaminated with PAH. However, the applied measures to reduce pollution should be continued and intensified.
Introduction
Polycyclic aromatic hydrocarbons (PAH) are ubiquitous pollutants. They are considered priority pollutants and are listed in the Water Framework directive, Marine Strategy Framework Directive and the UNEP MEDPOL program because they are persistent and may have carcinogenic, mutagenic, and teratogenic effects. Their major environmental sources are oil seepage and spills, marine traffic, sewage, industrial wastewater and atmospheric deposition (GESAMP, 1993). They are hazardous environmental chemicals (GESAMP, 1993; IARC, 2010; Keith, 2014) and pose a threat to marine organisms and subsequently to human health.
PAH are hydrophobic compounds and have the ability to bind to suspended solids in seawater (Parinos and Gogou, 2016; Adhikari et al., 2019). The enriched suspended solids settle on bottom sediment, where PAH can accumulate to higher concentrations.
It is well known that PAH also accumulate in marine organisms (Bajt et al., 2019; Gan et al., 2021). They enter their bodies through various mechanisms such as adsorption, diffusion, ingestion, and inhalation (Kelly et al., 2007; Arias et al., 2016), resulting in elevated concentrations in their tissues. In this way, they pose a threat to the health of marine organisms (Mearns et al., 2017; Honda and Suzuki, 2020; Gan et al., 2021).
The composition of PAH usually reflects their sources. Various molecular indices have been used to determine the origin of PAH in the natural marine environment. For example, the ratio between low molecular weight (LMW) and high molecular weight (HMW) PAH (Sicre et al., 1987; Magi et al., 2002) or the ratio of some isomers, e.g., phenanthrene/anthracene, fluoranthene/pyrene, benzo[a]anthracene/chrysene (Sicre et al., 1987; Colombo et al., 1989; Budzinski et al., 1997; Magi et al., 2002). The isomeric composition of PAH, i.e., those with the same molecular weight but different structure, depends on their thermodynamic stability during low-temperature processes (e.g., formation of petroleum) or on the kinetics of their transformation during high-temperature processes (e.g., combustion of organic material). As a result, the LMW fraction predominates in the petrogenic PAH fraction, whereas the HMW PAH are mostly of pyrolytic origin. Identifying the distribution of these compounds in seawater, sediments and marine biota and their origin is important for assessing the extent of pollution. This could help decision makers in planning and applying pollution reduction measures.
In the last decade, some results on PAH pollution have been published in the literature for the northern Adriatic (Alebic Juretic, 2011; Marini and Frapiccini, 2013; Traven, 2013; Acquavita et al., 2014; Combi et al., 2020), but few for the Gulf of Trieste (Acquavita et al., 2014; Bajt, 2014).
Considering the above facts, the aim of our work was to evaluate the state of pollution with PAH, including their concentrations, spatial and temporal distribution and origin, in the study area. For this purpose, data on the content of these compounds in surface sediments from 2003-2013 were processed using different evaluation indices. The ecological risk was also assessed. The data obtained during this period allowed us to gain a better insight into the status of pollution in the study area, including temporal trends.
Experimental
Study Area
The study area is a part of the Gulf of Trieste in the northern Adriatic Sea (Figure 1). The coastal area of the Gulf of Trieste is highly urbanized and industrialized and characterized by a relatively high population density (> 250 inhabitants/km2). There are many sources of pollution in this study area (e.g. sewage treatment plants, local industry, maritime and road traffic, agriculture…). The maritime traffic to the three ports (Trieste, Koper, Monfalcone) is very intense (more than 15,000 ships per year), with over 40 million tons of oil and oil products among other cargo. Nautical tourism is also well developed (pleasure crafts).
The coastal area studied is also affected by intensive road traffic, especially in summer, associated with intensive tourism. This marine environment receives effluents from the wastewater treatment plants of the local cities and freshwater discharges from the rivers, which also bring runoff from the adjacent agricultural areas. Despite a decline in industrial activity in recent decades, some industrial wastewater is still discharged into the Gulf.The Gulf of Trieste is also generally characterized by important oceanographic conditions, such as a very complex circulation of water masses (Malačič and Petelin, 2009) and a strong stratification of seawater in summer, resulting in a limited exchange of water masses. The main water inflow into the gulf is at its lower part. During winter, a cyclonic turn is formed in the southern part, while in spring and summer a gyre is formed and coupled with an anticyclonic gyre near the eastern part of the gulf. Many local currents affecting water circulation are seasonal and wind-driven (Bogunovič and Malačič, 2008).The selected sampling sites are shown in Figure 1 and described in Table 1. They were distributed on two transects in the Bay of Koper and the Bay of Piran and offshore in the central part of the Gulf of Trieste, in order to obtain information on the influence of pollution from land-based sources.
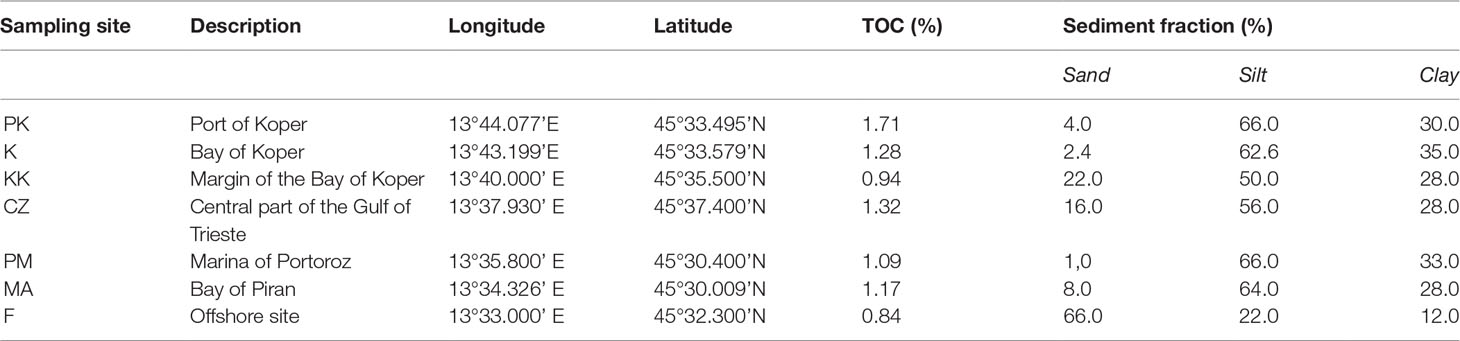
Table 1 Description of sampling sites; sediment fraction from Ogorelec et al. (1991), TOC from Bajt (2012).
Sampling, Extraction and Analyses
Sediments were collected by gravity corer in September each year from 2003 to 2013. The top 2 cm (approx. for recent ten years) of the sediment was frozen at -30°C in a glass jar. The water was removed by freeze-drying. The dried sediment sample was sieved through a 2-mm stainless steel sieve to remove small stones and shell particles. The use of the sediment fraction < 2 mm is in accordance with both the UNEP procedure and the proposed methodology developed under the Harmonia project (Berto et al., 2020), whose main objective was to harmonize monitoring methodologies in the Adriatic-Ionian subregion in accordance with the Marine Strategy Directive. About 10 g of the sieved sediment sample was used for further analysis. They were performed according to the procedure of UNEP/IOC/IAEA (1992). High purity solvents (Fluka) were used for the extraction of hydrocarbons. All other chemicals were of analytical grade, purchased from Merck. Sodium sulphate (Na2SO4), silicium dioxide (silica gel-SiO2), and aluminium oxide (alumina-Al2O3) were purified by Soxhlet extraction (methanol and hexane). Both oxides were activated at 200°C for 4 h and partially deactivated with MilliQ water (5 wt%). Cu (particle size <75 µm), which was used to remove sulphur, was activated with HCl (6 N).
Extraction of hydrocarbons from sediments was performed in a Soxhlet apparatus using hexane and dichloromethane (50:50) for 8 hours. After concentration of the solution on a rotary evaporator and under a stream of N2, the sulphur was removed with Cu powder. Purification and separation of hydrocarbons into aliphatic and aromatic fractions was performed by column chromatography (silica, alumina). The eluates obtained were concentrated and PAH concentrations determined with a HP 6890 gas chromatograph (GC). Separation was performed on a HP Ultra 2 column (25 m x 0.32 mm x 0.17 µm). The GC oven temperature program was as follows: initial column temperature 60°C, increased by 3°C min-1 to 290°C and hold at 290°C for 15 minutes. The initial temperature of the “on column” injector was 63°C, increased by 3°C min-1 to 293°C and held until the end. The detector temperature (flame ionization detector-FID) was 300°C. Helium was used as the carrier gas at a flow rate of 1 ml min-1.
PAH (16 EPA PAH + benzo[e]pyrene and perylene) were identified by comparing their retention times with those of an external PAH standard solution. Their concentrations were calculated based on their response factors.
Total organic carbon (TOC) was determined after acid digestion with HCl using a CHN -elemental analyzer (Vario Micro Cube, Elementar, Germany). Prior to analysis, the samples were homogenized in an agate mortar. Concentrations were calculated based on the initial sediment mass, and no corrections were made due to the carbonate removal process. Sulfonylamide was used as a standard.
Quality Control and Quality Assurance
Quality control procedures were used in the analyses. Concentrations of all target compounds in the blank samples were below the detection limit. Detection limits were determined by analyzing three replicates of a standard PAH solution at low PAH concentrations and considering the S/N ratio of 3. Two internal standards, hexamethylbenzene and cadalene (undeuterated), were added to each sediment sample to calculate recoveries and correct results. The recoveries were generally higher than 70% for hexamethylbenzene and from 85% up to 100% for cadalene. The precision of our work was evaluated using analyses of a sediment standard reference material IAEA-408, considering the 95% confidence interval (Table S1). The accuracy of the work was determined by analyzing three replicates of the standard reference material. The relative standard deviations for all target compounds were less than 15%.
Evaluation Indices
Different diagnostic ratios were applied for PAH origin determination:
the ratio between LMW PAH (2 and 3 aromatic rings) and HMW PAH (4, 5 and 6 aromatic rings); this ratio is higher than 1 for petrogenic origin and lower than 1 for pyrolytic origin (Gschwend and Hites, 1981)PAH isomer pairs; phenanthrene/anthracene (>15 for petrogenic origin and <10 for pyrolytic origin), fluoranthene/pyrene (<1 for petrogenic origin and >1 for pyrolytic origin), fluoranthene/fluoranthene+pyrene (<0.4 for petrogenic origin and >0.4 for pyrolytic origin) and benzo[a]anthracene/benzo[a]anthracene+chrysene (<0.2 for petrogenic origin and >0.35 for pyrolytic origin) (Gschwend and Hites, 1981; Yunker et al., 2002).
Results and Discussion
Distribution of PAH
Concentrations of PAH in sediments from the study area are shown in Table 2. The average total concentrations of PAH ranged from 320 ng g-1 dw to 1168 ng g-1 dw. The highest concentrations of PAH were observed at the PM station and the lowest at the offshore F station. In both bays, a gradual decrease in concentrations was observed toward the offshore areas. The slightly higher concentrations in the central part of the Gulf of Trieste (site CZ) are related to the city of Trieste and its port. The highest PAH concentrations at the PM site show the direct influence of boats in the marina. The higher concentrations are also due to very limited water circulation in the marina. Elevated concentrations in the port of Koper are directly related to maritime traffic and port activities. It should be mentioned that other factors could also contribute to the distribution of PAH. Perhaps the most important factor in the area studied is sediment grain size, as it increases toward the central part of the Gulf (Table 1). Indeed, TOC concentrations are higher at stations with smaller sediment grain size (Table 1), although this correlation is not statistically significant in our case. A similar correlation was found for total concentrations of PAH, with concentrations higher in sediment samples with a higher proportion of fine sediment, but also not statistically significant.
The total concentrations of PAH, obtained in this work, are generally comparable to the results of other studies from the Adriatic Sea from the last decade, although higher concentrations would be expected due to high pollution pressure and special oceanographic conditions (semi-enclosed area with limited exchange of water from the northern Adriatic). Marini and Frapiccini (2013) analyzed deep sediments at three sites in the northern Adriatic and determined quite low PAH concentrations of up to 108 ng/g. Much higher concentrations were detected in the Kvarner Bay in the northern Adriatic, 50 to 1150 ng/g (Traven, 2013). The detected PAH concentrations in the western Adriatic, in the Po Delta area, ranged from 193 to 533 ng/g (Combi et al., 2020). On the open sea at the SW Adriatic margin, concentrations were much lower, up to 74 ng/g (Combi et al., 2020). Similar concentrations were found in the open sea in the middle Adriatic, while concentrations in the Croatian coastal area were much higher, up to 1705 ng/g (Mandic et al., 2018). The highest concentrations were found in the Sibenik area, from 4629 to 18348 ng/g. Comparable concentrations were found in the western central Adriatic, in the Puglia region of Italy: 100-21500 ng/g (Mali et al., 2017). Sedimentary PAH concentrations from the Bay of Rijeka, an industrial region, exceeded 12000 ng/g (Alebic Juretic, 2011). Acquavita et al. (2014) reported concentrations up to 1056 ng/g in sediments from Marano and Grado lagoons in the northern Adriatic Sea. Even assuming higher enrichment in such lagoon systems, the concentrations were comparable to those from other coastal areas of the Adriatic Sea.
A classification according to PAH levels has been reported (Baumard et al., 1998a; Mostafa et al., 2009; Acquavita et al., 2014). The concentration range from 100 to 1000 ng/g was characteristic of moderately polluted areas. Almost all studied areas in our work fall within this range, with the exception of the Marina of Portoroz, a very limited area with higher pollution pressure. However, in recent years the situation in the marina has improved significantly.
As an EU member state, Slovenia had to incorporate the Water Framework Directive (WFD) and the Marine Strategy Framework directive (MSFD) into its legislation. Determining the quality of the marine environment is one of the main objectives of the MSFD. Descriptor 8 deals with marine pollution by various groups of pollutants. In addition to the concentrations of pollutants in seawater, sediments and organisms, the trends of these concentrations are also important. Member States are required to monitor the quality of their seas and, in the case of deterioration, to take appropriate measures to improve the status in accordance with criteria set by themselves. Member States should group themselves into sub-regions to facilitate harmonization of criteria for determining the quality of the marine environment. For the Adriatic-Ionian Sea sub region, the main recent attempt at harmonization was made in the Harmonia project (Berto et al., 2020). In addition to the proposals for the overall monitoring methodology, from sampling to analysis and reporting of results, the proposed ranges for quality control of the marine environment are also important. Comparison of our PAH concentrations shows that they are all much lower (one to two orders of magnitude) than the maximum concentrations listed under Harmonia. This confirms the assessment in the previous paragraph that the sediment in the study area is only moderately contaminated with PAH.
Origin of PAH
The distribution of PAH with different numbers of aromatic rings is shown in Figure 2. HMW-PAH, i.e., PAH with 4, 5, and 6 rings, are much more abundant compared to LMW-PAH (2 + 3 rings). PAH with 2 and 3 fused aromatic rings accounted for less than 20% at all sites, resulting in a LMW/HMW ratio below 1. This already indicates the predominant pyrolytic PAH origin in the studied area. PAH with 4 rings accounted for up to 40% of the total PAH concentrations. They can be either petrogenic or pyrolytic in origin. The results shown in Figure 3 indicate that they are predominantly pyrolytic in origin.
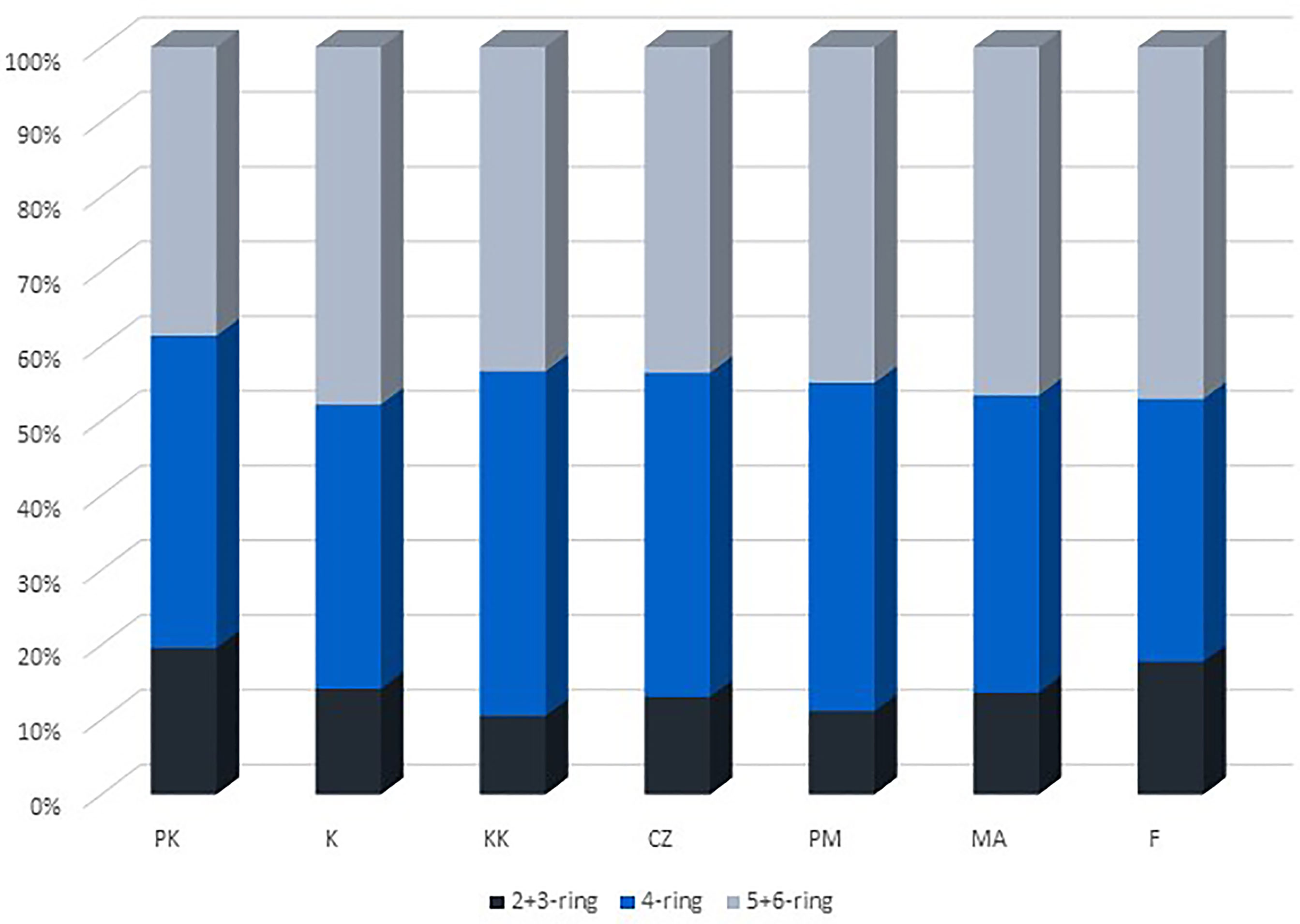
Figure 2 Distribution of PAHs with different number of aromatic rings in sediment samples (average values).
PAH isomer pairs with similar molecular weight but different structure and thermodynamic stability have also been widely used to identify potential PAH sources, e.g., phenanthrene/anthracene, fluoranthene/pyrene, benzo[a]anthracene/chrysene (Magi et al., 2002; Readman et al., 2002; Yunker et al., 2002; Zaghden et al., 2007). The cross-plot of the two evaluation indices used in this work is shown in Figure 3. Almost all sampling sites are grouped in one quadrant, showing the predominant pyrolytic origin of sedimentary PAH in the studied part of the Gulf of Trieste. Only at the sites PK and K a larger contribution of petrogenic origin was detected. This is likely related to the intense maritime traffic to and from the port of Koper, the effects of which reach the central part of the Koper Bay. The pronounced pyrolytic origin at site F, the site farthest from the coast, is rather the result of various processes affecting the transport of pollutants in the marine environment, in addition to the direct influence of maritime traffic. The dispersion of pollutants by seawater currents affects their distribution in the marine environment. Organic compounds can undergo various transformations (e.g., photochemical oxidation, microbial degradation, physical processes such as evaporation, dissolution, and sedimentation) during their transport (Oros and Ross, 2004; Haritash and Kaushik, 2009). Low molecular weight PAH are known to be more soluble and volatile and thus better substrates for microbial degradation (Mac Rae and Hall, 1998). In contrast, the high molecular weight compounds are more likely to be transported to the seafloor due to their resistance to degradation and increased particle sorption. The CZ site in the central part of the Gulf is also under the influence of the port and the city of Trieste with its coastal ironworks in close proximity to the port.
A somewhat more detailed insight into the sources of PAH was obtained by combining two other indices (Figure 4). Again, the main source is pyrolytic, but this varies little between stations. Thus, the main source at stations K, F, MA, and PK is the combustion of petroleum products, most likely fuels, while stations KK and CZ also show the influence of solid fuel combustion. The most probable influence is that of the city of Trieste with its large industrial area. At the station PK in the port, the petrogenic source is also significant, certainly indicating the influence of maritime traffic. For the PM station, a mixed origin can be assumed, but it is not very clear. The distribution and accumulation of PAH in the marina is certainly also influenced by the enclosure of the area itself, which leads to a higher accumulation of these substances, which in the long term may even somewhat mask the main source of PAH.
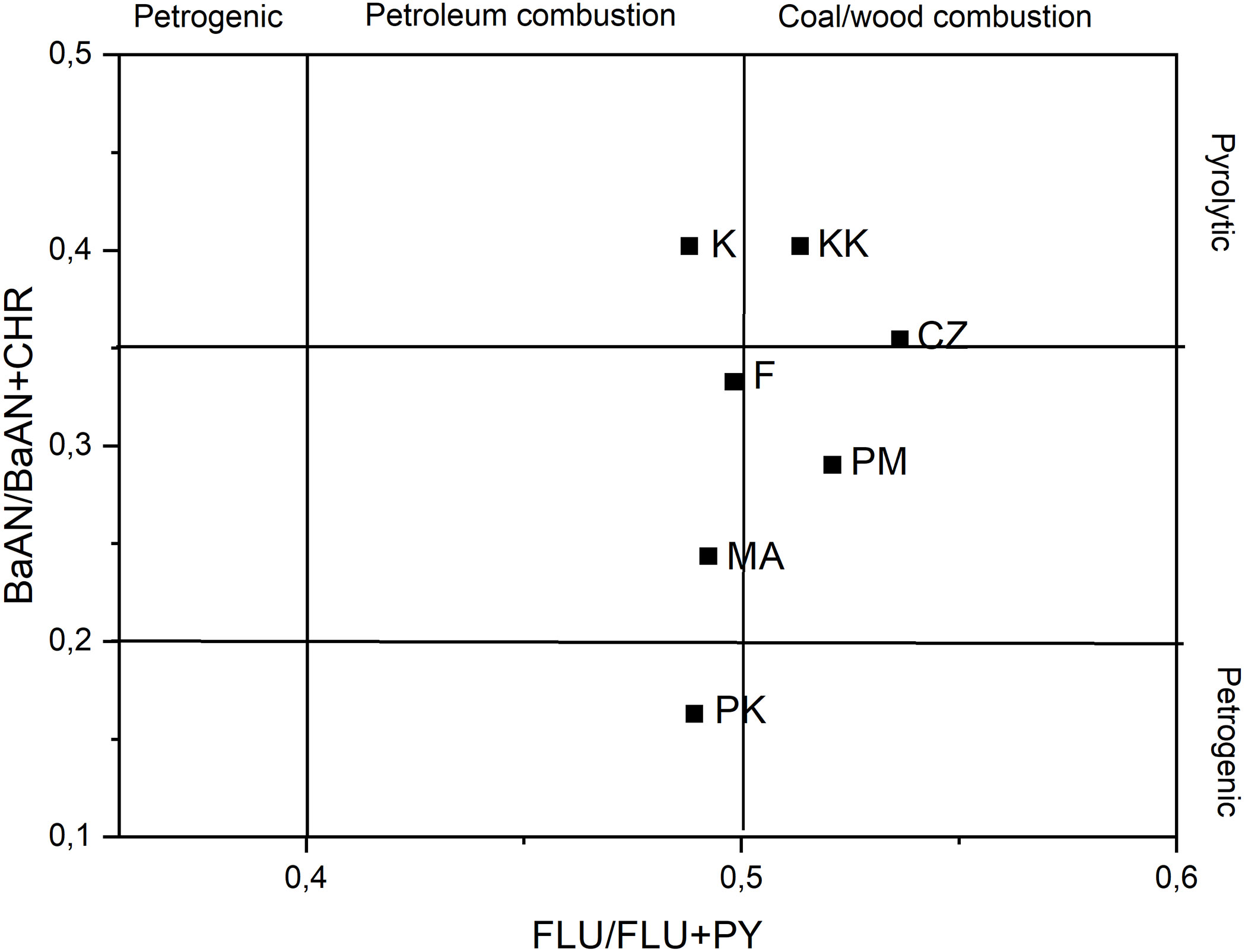
Figure 4 Cross plot of fluoranthene/fluoranthene+pyrene and benzo[a]anthracene/benzo[a]anthracene+chrysene (average values).
The predominant pyrolytic origin of PAH was also found in some other parts of the Adriatic. It seems that such a situation is more characteristic of coastal areas, especially in bays and lagoons near important cities. For example, PAH in the Bay of Rijeka were predominantly of pyrolytic origin with some petrogenic contribution (Alebic Juretic, 2011), a similar situation was found in the wider area of the Kvarner Bay (Traven, 2013), strongly pyrolytic origin PAH were found in the lagoons of Marano and Grado (Acquavita et al., 2014), in the Puglia region (Mali et al., 2017), and near Sibenik in Croatia (Mandic et al., 2018). The petrogenic origin seems to predominate in the open sea, as in the middle Adriatic transect (Mandic et al., 2018) and in the middle Adriatic (Mandic and Pavela Vrancic, 2017). This is consistent with the results presented in this paper for the Gulf of Trieste, as the entire Gulf can be considered coastal.
Perylene, a 5-ring PAH usually detected in coastal areas, can be of pyrolytic or natural origin (Readman et al., 2002). Combustion processes produce only small amounts of perylene. More important is its natural origin, as it is considered the major diagenetic PAH in sedimentary environments. It originates from terrestrial plant debris carried by rivers to the sea and undergoes diagenetic transformation in situ (Baumard et al., 1998b; Readman et al., 2002). The origin of perylene has been determined by the relative concentration of perylene to the sum of the 5-ring isomers. Values greater than 10% indicate its diagenetic origin (Readman et al., 2002; Chen et al., 2006). In our case, such proportion was observed at all sites examined. This is indicative of the predominant diagenetic origin of perylene. The Gulf of Trieste is namely under the strong influence of freshwater inputs from rivers. During the winter and spring periods, large amounts of freshwater are introduced into the Gulf, reflected in a decrease of salinity in the surface water of the Gulf of Trieste (Malačič et al., 2006). The highest perylene concentrations were found at the site PK, which is under the direct influence of the Rizana River, the outflow of which is located in the area of the Port of Koper. Higher perylene concentrations at the PM sampling site are due to the Dragonja River inflow. Perylene concentrations in both bays decreased toward the central part of the Gulf of Trieste, confirming its natural terrestrial origin.
Temporal Trends
Evaluation of temporal variations in pollutant distribution is very important in the implementation of various pollution reduction measures. Especially in coastal waters, temporal variations are more important due to the direct effects of various pollution sources, higher pollution levels, temporal differences in anthropogenic activities, more intense oceanographic phenomena, and so on. Even though this is important, such trends are not very often identified in scientific works because the results are needed for many years (Alebic Juretic, 2011; Traven, 2013). The results on PAH concentrations in sediments in this work for eleven individual years allowed us to assess at least medium-term trends. Most notably, year-to-year fluctuations in concentrations can be seen, which are more visible at sites with higher PAH concentrations. Only in three locations we could detect a significant decreasing trend of concentrations. These are the port in Koper, the marina in Portoroz and station F on the more open sea. At the station PK, concentrations decreased from about 900 ng/g to about 500 ng/g during the reporting period. At the PM station, the decrease was even more pronounced, from about 1800 ng/g to about 700 ng/g in recent years. A smaller absolute decrease was observed at station F, from about 400 ng/g to about 200 ng/g. Here the concentrations are the lowest among the studied areas. The determination of the Pearson correlation coefficient showed that the trend of concentrations at these three stations was even statistically significant (p < 0.05) (Figure S1).
It is difficult to give reliable reasons for such a decrease in PAH concentrations at these three stations. The port and marina have been using numerous activities and financial resources to reduce pollution for many years, which is reported in the annual sustainability reports of the Port of Koper and the annual reports of the Portoroz marina. This has certainly had a concrete effect on the reduction of PAH concentrations in sediments. However, the decreasing trend in the more open sea is probably due to all the efforts to reduce pollution in the coastal area, as well as actions in the maritime sector itself.
In processing these results, we have also seen that multi-year monitoring of pollution by specific substances gives us a more comprehensive view of the levels, distribution, and sources of PAH in a given area. Both concentrations and origin evaluation indices vary considerably from year to year depending on many parameters, the pollution itself, weather conditions and oceanographic conditions in a given year. Therefore, we can also get slightly different results about the distribution and sources of PAH in a single year (Bajt, 2014).
As written earlier, there is not much work describing the temporal trends of concentrations in sedimentary PAH. Only two recent works were found for the Adriatic Sea. Alebic Juretic (2011) found a decreasing trend from 1998 to 2006 in Rijeka Bay, while no trend was found in Kvarner Bay from 2007 to 2012 (Traven, 2013). Considering the Rijeka Bay as a part of the Kvarner Bay, we can see that the trends are more characteristic for areas close to important pollution sources (cities, ports etc.). Similarly, in our case in the Gulf of Trieste, some trends were observed mainly in the port of Koper and the marina of Portoroz, areas directly affected by important sources of pollution.
Ecological Risk Assessment
PAH are toxic compounds and can have adverse biological effects. Numerical sediment quality guidelines (SQGs) have been developed to assess the adverse biological effects of various contaminants. SQGs for PAH were derived from the results of numerous laboratory and field studies and various toxicity tests for various aquatic organisms (Long et al., 1995; MacDonald et al., 1996). The lower effect range (ERL) and the median effect range (ERM) were used to evaluate potential adverse toxicological effects (Long et al., 1995). These ranges are used to define contaminant concentration ranges that are rarely (<ERL), occasionally (≥ERL and <ERM) or frequently (≥ERM) associated with adverse biological effects.
In this study, the total concentrations of PAH and the individual concentrations of PAH were compared with the ERL and ERM values (Table 3). The results showed that the total concentrations of PAH for all years were well below the ERM and well below the ERL at all sites (77 results). Even the maximum value at the PM station was still two times below the ERL. At most sites, individual concentrations of PAH also did not exceed the ERL. Only the concentrations of acenaphthene and dibenzo[a,h]anthracene in five samples and benzo[g,h,i]perylene in one sample exceeded the ERL but were well below the ERM. Fluorene concentrations were most frequently above the ERL (22 of 77 samples), although the maximum value was still five times lower than the ERM. This means that the levels of PAH in the sediments of the studied area are below the ranges where adverse toxicological effects would be expected.
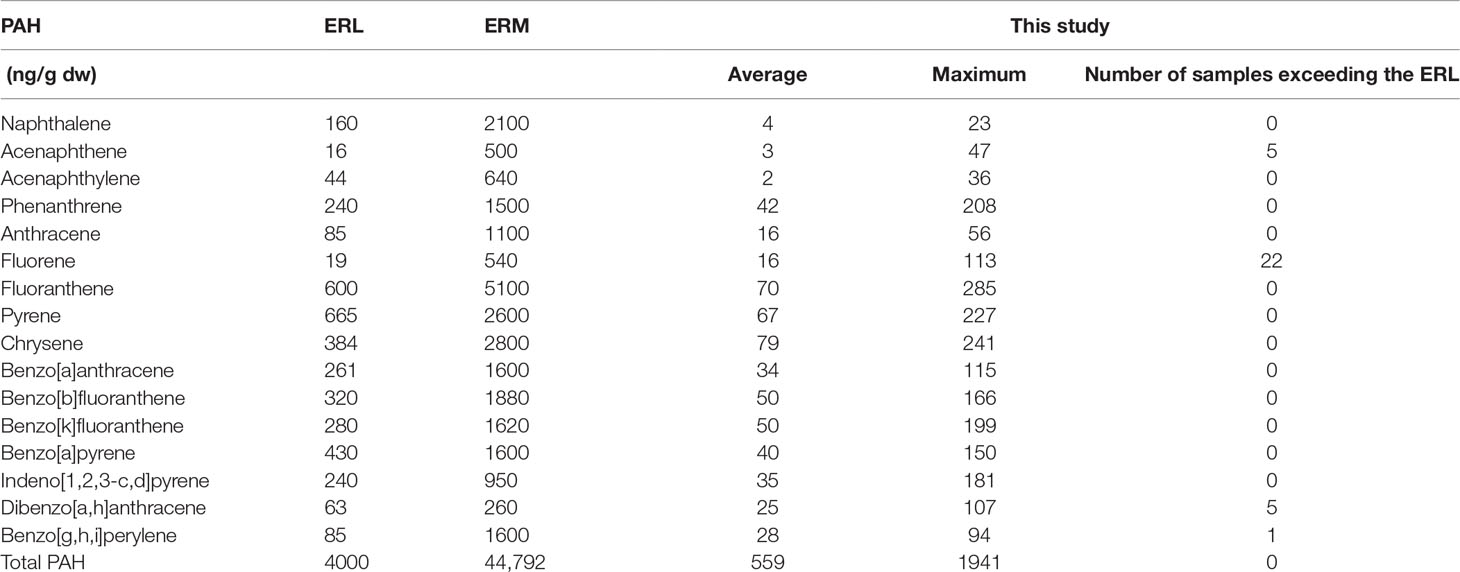
Table 3 Results of ecological risk assessment for sediment samples in the study area from 2003 to 2013.
Conclusions
In general, the studied area in the Gulf of Trieste can be considered as only moderately polluted by polycyclic aromatic hydrocarbons. The highest concentrations were detected near the pollution sources (port, marina). The concentrations then decreased towards the offhore areas.
The pyrolytic origin of PAH is the predominant one in the studied area, with a lower contribution of the petrogenic one, which is slightly more important in the port of Koper, most likely related to the intensive maritime traffic and port activities. Perylene is of diagenetic origin and is associated with terrestrial inputs (rivers).
The ecological risk assessment showed that the concentrations of PAH are far below the levels that could cause adverse biological effects.
Concentrations of PAH showed a decreasing trend in 11 years only at the stations in the port of Koper, the marina of Portoroz and the offshore area off Piran. No trend was observed at any other sites. These trends are most likely the result of better environmental management to reduce the impact of pollution from direct pollution sources. These activities should be continued and even intensified.
Data Availability Statement
The raw data supporting the conclusions of this article will be made available by the authors, without undue reservation.
Author Contributions
The author confirms being the sole contributor of this work and has approved it for publication.
Funding
This study was financially supported by the Slovenian Environmental Agency (National monitoring program) and Slovenian Research Agency (research program P1-0237 and project L7-1847).
Conflict of Interest
The author declares that the research was conducted in the absence of any commercial or financial relationships that could be construed as a potential conflict of interest.
Publisher’s Note
All claims expressed in this article are solely those of the authors and do not necessarily represent those of their affiliated organizations, or those of the publisher, the editors and the reviewers. Any product that may be evaluated in this article, or claim that may be made by its manufacturer, is not guaranteed or endorsed by the publisher.
Supplementary Material
The Supplementary Material for this article can be found online at: https://www.frontiersin.org/articles/10.3389/fmars.2022.946618/full#supplementary-material
References
Acquavita A., Falomo J., Predonzani S., Tamberlich F., Bettoso N., Mattassi G. (2014). The PAH Level, Distribution and Composition in Surface Sediments From a Mediterranean Lagoon: The Marano and Grado Lagoon (Northern Adriatic Sea, Italy). Mar. pollut. Bull. 81, 234–241. doi: 10.1016/j.marpolbul.2014.01.041
Adhikari P. L., Maiti K., Bam W. (2019). Fate of Particle-Bound Polycyclic Aromatic Hydrocarbons in the River-Influenced Continental Margin of the Northern Gulf of Mexico. Mar. pollut. Bull. 141, 350–362. doi: 10.1016/j.marpolbul.2019.02.046
Alebic Juretic A. (2011). Polycyclic Aromatic Hydrocarbons in Marine Sediments From the Rijeka Bay Area, Northern Adriatic, Croatia 1998–2006. Mar. pollut. Bull. 62, 863–869. doi: 10.1016/j.marpolbul.2011.01.035
Arias A. H., Souissi A., Roussin M., Ouddane B., Souissi S. (2016). Bioaccumulation of PAHs in Marine Zooplankton: An Experimental Study in the Copepod Pseudodiaptomus Marinus. Environ. Earth Sci. 75, 691. doi: 10.1007/s12665-016-5472-1
Bajt O. (2012). Aliphatic and Polycyclic Aromatic Hydrocarbons in Sediments of the Slovenian Coastal Area (Gulf of Trieste, Northern Adriatic). Environ. Monit. Assess. 184 (12), 7439–7452. doi: 10.1007/s10661-011-2511-y
Bajt O. (2014). Aliphatic and Polycyclic Aromatic Hydrocarbons in Sediments of the Gulf of Trieste (Northern Adriatic)-The Impact of Maritime Traffic. Bull. Environ. Contamination Toxicol. 93 (3), 299–305. doi: 10.1007/s00128-014-1321-7
Bajt O., Ramšak A., Milun V., Andral B., Romanelli G., Scarpato A., et al. (2019). Assessing Chemical Contamination in the Coastal Waters of the Adriatic Sea Using Active Mussel Biomonitoring With Mytilus Galloprovincialis. Mar. pollut. Bull. 141, 283–298. doi: 10.1016/j.marpolbul.2019.02.007
Baumard P., Budzinski H., Garrigues P. (1998a). Polycyclic Aromatic Hydrocarbons in Sediments and Mussels of the Western Mediterranean Sea. Environ. Toxicol. Chem. 17, 765–776. doi: 10.1002/etc.5620170501
Baumard P., Budzinski H., Mehin Q., Garrigues P., Burgeot T., Bellocq J. (1998b). Origin and Bioavailability of PAHs in the Mediterranean Sea From Mussel and Sediment Records. Estuar. Coast. Shelf Sci. 47, 77–90. doi: 10.1006/ecss.1998.0337
Berto D., Formalewicz M., Giorgi G., Manfra L., Lipizer M., Molina J. E., et al. (2020). HarmoNIA Methodological Proposals Part I: Harmonizing Monitoring of Marine Contaminants and Sharing Data Quality Control Procedures. 40 pp. doi: 10.6092/8c1f1204-b1f1-46d7-86e2-f173b3f84196
Bogunovič B., Malačič V. (2008). Circulation in the Gulf of Trieste: Measurements and Model Results. Nuovo cimento 31, 301–326. doi: 10.1393/ncc/i2008-10310-9
Budzinski H., Jones I., Bellocq J., Pierrad C., Garrigues P. (1997). Evaluation of Sediment Contamination by Polycyclic Aromatic Hydrocarbons in the Gironde Estuary. Mar. Chem. 58, 264–269. doi: 10.1016/S0304-4203(97)00028-5
Chen S. J., Luo X. J., Mai B. X., Sheng G. Y., Fu J. M., Zeng E. Y. (2006). Distribution and Mass Inventories of Polycyclic Aromatic Hydrocarbons and Organochlorine Pesticides in Sediments of the Pearl River Estuary and the Northern South China Sea. Environ. Sci. Technol. 40, 709–714. doi: 10.1021/es052060g
Colombo J. C., Pelletier E., Brochu C., Khalil M., Catoggio J. A. (1989). Determination of Hydrocarbon Sources Using N-Alkanes and Polycyclic Aromatic Distribution Indexes. Case Study: Rio De La Plata, Argentina. Environ. Sci. Technol. 23, 888–894. doi: 10.1021/es00065a019
Combi T., Pintado-Herrera M. G., Lara-Martín P. A., Lopes-Rocha M., Miserocchi S., Langone L., et al. (2020). Historical Sedimentary Deposition and Flux of PAHs, PCBs and DDTs in Sediment Cores From the Western Adriatic Sea. Chemosphere 241, 125029. doi: 10.1016/j.chemosphere.2019.125029
Gan N., Martin L., Xu W. (2021). Impact of Polycyclic Aromatic Hydrocarbon Accumulation on Oyster Health. Front. Physiol. 12, 734463. doi: 10.3389/fphys.2021.734463
GESAMP (1993). “Review of Potentially Harmful Substances: Oil and Other Hydrocarbons Including Used Lubricating Oils, Oil Spill Dispersants and Chemicals Used in Offshore Exploration and Exploitation. Impact of Oil and Related Chemicals and Wastes on the Marine Environment, XXII/4. Joint Group of Experts on the Scientific Aspects of Marine Pollution,” in Reports and Studies (Uk: IMO, London).
Gschwend P. M., Hites R. A. (1981). Fluxes of Polycyclic Aromatic Hydrocarbons to Marine and Lacustrine Sediments in the North-Eastern US. Geochimica. Cosmochimica. Acta 45, 2359–2367. doi: 10.1016/0016-7037(81)90089-2
Haritash A. K., Kaushik C. P. (2009). Biodegradation Aspects of Polycyclic Aromatic Hydrocarbons (PAHs): A Review. J. Hazardous Materials 169, 1–15. doi: 10.1016/j.jhazmat.2009.03.137
Honda M., Suzuki N. (2020). Toxicities of Polycyclic Aromatic Hydrocarbons for Aquatic Animals. Int. J. Environ. Res. Public Health 17 (4), 1363. doi: 10.3390/ijerph17041363
IARC (2010) International Agency for Research on Cancer. IARC Monographs on the Evaluation of Carcinogenic Risks to Humans. Volume 92: Some Non-Heterocyclic Polycyclic Aromatic Hydrocarbons and Some Related Exposures. Available at: http://monographs.iarc.fr/ENG/Monographs/vol92/mono92.pdf.
Keith L. H. (2014). The Source of U.S. Epa’s Sixteen PAH Priority Pollutants. Polycyclic Aromatic Compounds 35, 147–160. doi: 10.1080/10406638.2014.892886
Kelly B., Ikonomou M., Blair J. D., Morin A. E., Gobas F. (2007). Food Webspecific Biomagnifican of Persistent Organic Pollutants. Science 317, 236–239. doi: 10.1126/science.1138275
Long E. R., MacDonald D. D., Smith S. L., Calder F. D. (1995). Incidence of Adverse Biological Effects Within Ranges of Chemical Concentrations in Marine and Estuarine Sediments. Environ. Manage. 19, 81–97. doi: 10.1007/BF02472006
MacDonald D. D., Carr R. S., Calder F. D., Long E. R., Ingersoll C. G. (1996). Development and Evaluation of Sediment Quality Guidelines for Florida Coastal Waters. Ecotoxicology 5, 253–278. doi: 10.1007/BF00118995
Mac Rae J. D., Hall K. J. (1998). Biodegradation of Polycyclic Aromatic Hydrocarbons (PAH) in Marine Sediments After Denitrifying Conditions. Water Sci. Technol. 38, 177–185. doi: 10.2166/wst.1998.0463
Magi E., Bianco R., Ianni C., Di Carro M. (2002). Distribution of Polycyclic Aromatic Hydrocarbons in Sediment of the Adriatic Sea. Environ. pollut. 119, 91–98. doi: 10.1016/S0269-7491(01)00321-9
Malačič V., Celio M., Čermelj B., Bussani A., Comici C. (2006). Interannual Evolution of Seasonal Thermohaline Properties in the Gulf of Trieste (Northern Adriatic) 1991-2003. J. Geophys. Res. 111, 1–16. doi: 10.1029/2005JC003267
Malačič V., Petelin B. (2009). Climate Circulation in the Gulf of Trieste (Northern Adriatic). J. Geophys. Res. 114, 1–15. doi: 10.1029/2008JC004904
Mali M., Dell’Anna M. M., Mastrorilli P., Damiani L., Piccinni A. F. (2017). Assessment and Source Identification of Pollution Risk for Touristic Ports: Heavy Metals and Polycyclic Aromatic Hydrocarbons in Sediments of 4 Marinas of the Apulia Region (Italy). Mar. pollut. Bull. 114, 768–777. doi: 10.1016/j.marpolbul.2016.10.063
Mandic J., Pavela Vrancic M. (2017). Concentrations and Origin of Polycyclic Aromatic Hydrocarbons in Sediments of the Middle Adriatic Sea. Acta Adriatica 58 (1), 3–24. doi: 10.32582/aa.58.1.1
Mandic J., Tronczynski J., Kuspili G. (2018). Polycyclic Aromatic Hydrocarbons in Surface Sediments of the Mid-Adriatic and Along the Croatian Coast: Levels, Distributions and Sources. Environ. pollut. 242, 519–527. doi: 10.1016/j.envpol.2018.06.095
Marini M., Frapiccini E. (2013). Persistence of Polycyclic Aromatic Hydrocarbons in Sediments in the Deeper Area of the Northern Adriatic Sea (Mediterranean Sea). Chemosphere 90, 1839–1846. doi: 10.1016/j.chemosphere.2012.09.080
Mearns A. J., Reish D. J., Oshida P. S., Morrison A. M., Rempel-Hester M. A., Arthur C., et al. (2017). Effects of Pollution on Marine Organisms. Water Environ. Res. 89 (10), 1704–1798. doi: 10.2175/106143017X15023776270647
Mostafa A. R., Wade T. L., Sweet S. T., Al-Alimi A. K. A. (2009). Distribution and Characterization of Polycyclic Aromatic Hydrocarbons (PAHs) in Sediments of Hadhramout Coastal Area, Gulf of Aden, Yemen. J. Mar. Syst. 78, 1–8. doi: 10.1016/j.jmarsys.2009.02.002
Ogorelec B., Misic M., Faganeli J. (1991). Marine Geology of the Gulf of Trieste (Northern Adriatic): Sedimentological Aspects. Mar. Geol. 99, 79–92. doi: 10.1016/0025-3227(91)90084-H
Oros D. R., Ross J. R. M. (2004). Polycyclic Aromatic Hydrocarbons in San Francisco Estuary Sediments. Mar. Chem. 86, 169–184. doi: 10.1016/j.marchem.2004.01.004
Parinos C., Gogou A. (2016). Suspended Particle-Associated PAHs in the Open Eastern Mediterranean Sea: Occurrence, Sources and Processes Affecting Their Distribution Patterns. Mar. Chem. 180, 42–50. doi: 10.1016/j.marchem.2016.02.001
Readman J. W., Fillmann G., Tolosa I., Bartocci J., Villeneuve J. P., Catinni C., et al. (2002). Petroleum and PAH Contamination of the Black Sea. Mar. pollut. Bull. 44, 48–62. doi: 10.1016/S0025-326X(01)00189-8
Sicre M. A., Marty J. C., Saliot A., Aparicio X., Grimalt J., Albaiges J. (1987). Aliphatic and Aromatic Hydrocarbons in Different Size Aerosols Over the Mediterranean Sea: Occurrence and Origin. Atmosph. Environ. 21, 2247–2259. doi: 10.1016/0004-6981(87)90356-8
Traven L. (2013). Sources, Trends and Ecotoxicological Risks of PAH Pollution in Surface Sediments From the Northern Adriatic Sea (Croatia). Mar. pollut. Bull. 77, 445–450. doi: 10.1016/j.marpolbul.2013.08.043
UNEP/IOC/IAEA (1992). Determination of Petroleum Hydrocarbons in Sediments. Reference Methods for Marine Pollution Studies No. 20. IAEA-MEL, Monaco
Yunker M. B., Macdonald R. W., Vingarzan R., Mitchell R. H., Goyette D., Sylvestre S. (2002). PAHs in the Fraser River Basin: A Critical Appraisal of PAH Ratios as Indicators of PAH Source and Composition. Organic Geochem. 33 (4), 489–515. doi: 10.1016/S0146-6380(02)00002-5
Keywords: PAH, sediments, northern Adriatic, origin, distribution
Citation: Bajt O (2022) Sedimentary Record of Polycyclic Aromatic Hydrocarbons in the Gulf of Trieste (Northern Adriatic Sea) - Distribution, Origin and Temporal Trends. Front. Mar. Sci. 9:946618. doi: 10.3389/fmars.2022.946618
Received: 17 May 2022; Accepted: 23 June 2022;
Published: 22 July 2022.
Edited by:
Alessandra Roncarati, University of Camerino, ItalyReviewed by:
Bojan Hamer, Rudjer Boskovic Institute, CroatiaJean-Carlos Montero-Serrano, Université, du Québec à Rimouski, Canada
Alessandro Acquavita, Agenzia Regionale per la Protezione dell’Ambiente del Friuli Venezia Giulia (ARPA FVG), Italy
Copyright © 2022 Bajt. This is an open-access article distributed under the terms of the Creative Commons Attribution License (CC BY). The use, distribution or reproduction in other forums is permitted, provided the original author(s) and the copyright owner(s) are credited and that the original publication in this journal is cited, in accordance with accepted academic practice. No use, distribution or reproduction is permitted which does not comply with these terms.
*Correspondence: Oliver Bajt, oliver.bajt@nib.si