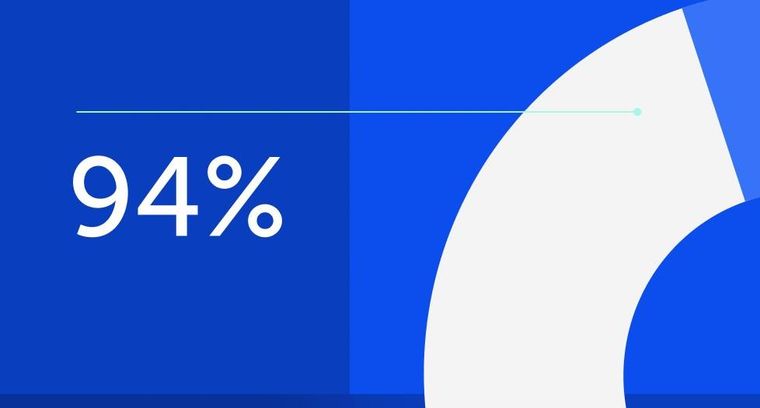
94% of researchers rate our articles as excellent or good
Learn more about the work of our research integrity team to safeguard the quality of each article we publish.
Find out more
REVIEW article
Front. Mar. Sci., 13 September 2022
Sec. Marine Biotechnology and Bioproducts
Volume 9 - 2022 | https://doi.org/10.3389/fmars.2022.946220
This article is part of the Research TopicBioremediation for a Greener Planet: Removal of Excess Nutrients, Heavy Metals, Hydrocarbons, and Other Pollutants by Aquatic MicroorganismsView all 4 articles
The application of manure and sewage sludge on farmland is one key element in building up soil organic matter and is contributing to soil carbon sequestration. These organic fertilizers are often contaminated with anthropogenic pollutants that can cause serious harm to ecosystems and persist over a long period. Bioremediation approaches have gained attention as clean-up treatment in recent decades due to their cost-effectiveness and environmental friendliness. Besides phytoremediation with plants and bacterial bioremediation, fungi have also been successfully applied for pollution control. While land-based species, especially white-rot fungi, are extensively studied for mycoremediation, fungi from marine environments are underrepresented but show adaptive traits (e.g., halophilic, anaerobic) that are beneficial under certain conditions and may improve the degradation portfolio. This review highlights application examples of marine-derived fungi for organopollutant removal and evaluates the overall future potential of this underexplored group, which might become an interesting extension for remediation of manure and sewage sludges with high osmotic pressure.
Soil is the largest terrestrial reservoir of organic carbon, holding more carbon (C) than the atmosphere and vegetation combined. Therefore, slight changes in soil carbon concentration can significantly affect the atmosphere: a loss in soil organic carbon implies a release of CO2 to the atmosphere, while an accumulation indicates the sequestration of carbon in soil (negative emission) (Flessa et al., 2018). The soil organic carbon content for a specific site is affected by local conditions (climate, water saturation, etc.) and the type of land use. Since the Neolithic Revolution and the advent of agriculture 12,000 years ago, land cover change from native vegetation to agricultural land has resulted in the estimated loss of 133 gigatons (Gt) of carbon (488 Gt CO2) from soil (Sanderman et al., 2017). Cropland soils are especially depleted in organic carbon because plant biomass is mostly removed during harvest, reducing organic matter input into soil. Depleted soils offer opportunities for soil carbon sequestration, and hence, cropping regions and degraded grazing lands should be targeted for soil organic carbon restoration efforts (Sanderman et al., 2017). Most studies estimate the global biophysical potential for soil carbon sequestration to be 4-5 Gt CO2 per year, equaling 1.09-1.36 Gt C per year if techniques that aim to increase soil carbon, or “best management practices,” are completely adopted (Paustian et al., 2019). However, social and economic constraints are likely to reduce this number (Sanderman et al., 2017).
Considering the current global greenhouse gas emissions of almost 60 Gt of CO2 equivalents per year (United Nations Environment Programme, 2021), it becomes clear that soil carbon sequestration can compensate for a small part. With these high emissions, every effort to reduce greenhouse gases is required, and soil carbon sequestration should be pursued as part of a suite of climate change mitigation tools and actions embedded in the systemic transformation of society.
In order to restore soil organic carbon on croplands, the organic matter content in soil has to be increased, which ultimately leads to more humus. Several regenerative practices support humus growth, such as the cultivation of cover crops, leaving crop residues on the field, crop rotation, tillage changes, and applying organic fertilizers. Some of these measures add organic matter to the soil and also reduce soil carbon losses. Building up humus is beneficial for soil health, soil fertility, and yield stability (Flessa et al., 2018). An important aspect here is that these measures must be continued permanently, even after reaching a new soil carbon equilibrium. The whole process could be reversed when humus is eroded, and soil carbon diminishes (Flessa et al., 2018).
Organic fertilizers are the central tool in plant nutrient recycling. They also return organic carbon to the crop field from where it was removed during harvest. Farm manure, compost, biogas digestate, and sewage sludge are common organic fertilizers. One issue with organic fertilizers is the contamination with anthropogenic pollutants. Sewage sludges often contain heavy metals, pharmaceuticals and other organic pollutants, and micro- and nanoscale contaminants (Roskosch & Heidecke, 2018). As for manure, veterinary drug residues are a frequent problem (Vidaurre et al., 2016; Adler et al., 2018). This presents the dilemma of applying manure and sewage sludge as organic fertilizers. Since they are generated anyway in livestock farming and wastewater treatment, they should be preferred over mineral fertilizers that rely on finite resources and involve high energy consumption and environmental damages during production. In addition, organic fertilizers contribute to carbon sequestration in soil. On the other hand, farm manure and sewage sludge could pose an environmental risk due to contamination. Therefore, polluted sewage sludge and farm manure should be treated before their use as fertilizer.
In recent decades, bioremediation approaches have gained attention as clean-up treatment due to their cost-effectiveness and environmental friendliness. Biological remediation – as a natural process – is highly applicable for treatments, and plants and bacteria have been mainly used for this purpose. Mycoremediation offers an interesting combination: Many fungi are able to break down organic pollutants. This power results from their ecological role: fungi are saprotrophs and play a key part as decomposers of complex biological polymers, e.g., cellulose and lignin. They release enzymes and have metabolic pathways to degrade these polymers into smaller molecules. Industrial chemicals such as polychlorinated biphenyls (PCBs) and pharmaceuticals such as diclofenac are examples of environmentally relevant organic pollutants that fungi can biodegrade (Esterhuizen-Londt et al., 2017; Sadañoski et al., 2019; Kaewlaoyoong et al., 2020).
In the following review, research works in the field of mycoremediation of organic compounds will be examined with a closer look at studies involving marine-derived fungi.
As the name implies, persistent organic pollutants (POPs) are stable organic compounds that are difficult to break down and accumulate in living plants and animals along the food chain (bioaccumulation). Their often xenohormonal (hormone-like) to carcinogenic effects bear a risk for many organisms. POPs are typically biocides, industrial chemicals, and by-products of combustion processes. The following are some POPs under regulation in the European Union and relevant for the analysis of sewage sludge in Germany (Roskosch & Heidecke, 2018; European Commission, 2019):
- Adsorbable organic halides (AOX)
- Benzo[a]pyrene (B[a]P)
- Polychlorinated dibenzodioxins (PCDDs, “dioxins”) and dibenzofurans (PCDFs), including dioxin-like PCBs (dl-PCBs)
- Polychlorinated biphenyls (PCBs)
- Perfluorooctanoic acid (PFOA) and perfluorooctanesulfonic acid (PFOS)
B[a]P, representative of the polycyclic aromatic hydrocarbons (PAHs), PCDDs, and PCDFs (PCDD/Fs), are formed during the incomplete combustion of organic substances. B[a]P mainly originates from wood and coal heating in private homes, diesel engines, forest fires, and industrial emissions. Illegal incineration in private households is the most significant source of PCDDs and PCDFs. B[a]P, PCDDs, and PCDFs are carcinogenic substances (U.S. EPA, 2006; International Agency for Research on Cancer, 2012).
PCBs are industrial chemicals formerly used, e.g., in coolants, capacitors, transformers, hydraulic fluids, and plasticizers, to increase chemical stability. They are carcinogenic, and their production and use have been banned worldwide since 2001 (Stockholm Convention on Persistent Organic Pollutants (POPs), 2001; International Agency for Research on Cancer, 2016).
PFOA and PFOS are technical chemicals used to manufacture water-repellent textiles such as Gore-Tex® or coatings such as Teflon® or ski wax. These per- and polyfluoroalkyl substances (PFAS) are associated with elevated cholesterol levels, thyroid disease, liver damage, infertility, inflammatory bowel disease, various cancers, and developmental disorders in unborn children. There is a growing concern in science and politics about associated health and environmental risks accompanying the increased use of these compounds (International Agency for Research on Cancer, 2017; Roskosch & Heidecke, 2018).
POPs can be detected everywhere in the environment due to their persistence, mobility, and long-standing use in the past. Even substances such as PCBs, which have not been produced or used for a long time, are ubiquitously found (International Agency for Research on Cancer, 1997; International Agency for Research on Cancer, 2012; International Agency for Research on Cancer, 2016; International Agency for Research on Cancer, 2017). Such accumulation can be observed in sewage and manure.
Drug residues in the environment come from both human and veterinary medicine. Around 2,300 active ingredients are currently approved for human medicinal products in Germany, of which around 1,200 substances, i.e., around half, are considered environmentally relevant. 8,120 t of these environmentally relevant substances were administered in 2012, an increase of more than 20% since 2002 (6,200 t) (Ebert et al., 2014). Due to demographic change, a further increase in pharmaceutical consumption by humans by up to 68.5% is expected for the coming decades in Germany and worldwide (civity Management Consultants, 2017).
Pharmaceuticals for human use enter the environment via wastewater. The entry route of veterinary medicinal products is mainly via animal excretions, applied on agricultural land as manure. More than 150 active substances have already been found in various environmental media. Finding more compounds is limited by the respective analytical capabilities and legal regulations. Pharmaceutical residues can be detected almost everywhere in German surface waters (Ebert et al., 2014).
In this context, medicinal products for human use, e.g., X-ray contrast agents, diclofenac (painkiller), and metformin (antidiabetic), are regularly found in high concentrations. Diclofenac, sold under the brand Voltaren®, is a very common analgesic and infamous for its devastating effects on ecosystems, most notably as the cause of the vulture population collapse on the Indian subcontinent (Green et al., 2004; Oaks et al., 2004). For the European flora and fauna, it has been reported that diclofenac damages the internal organs of certain fish species (Triebskorn et al., 2007). The impact on ecosystems is further exacerbated by its widespread use in human medicine and the fact that diclofenac is poorly removed in wastewater treatment processes (Luo et al., 2014). Moreover, diclofenac is obtainable over the counter in most countries, thus, leading to a high input load into the environment. The antidiabetic drug metformin is one of the most commonly found pharmaceuticals in aquatic environments, occurring in different environmental matrices, such as sewage, sludges and effluents from wastewater treatment plants, surface waters, drinking water, and sediments. Metformin is bioactive in many organisms changing the expression of different genes, including some responsible for endocrine pathways (Ambrosio-Albuquerque et al., 2021). Metformin is quite persistent and its degradation leads to another persistent compound, guanylurea, a “dead-end” compound in waste water treatment (Tassoulas et al., 2021). Synthetic hormones used for medication can have devastating effects on ecosystems even in the slightest concentration. The best example is ethinylestradiol, used in human birth control pills. It caused drastic changes in the reproductive system of a fish species and led to population collapse in field experiments (Kidd et al., 2007). An antiparasitic drug used in livestock farming has reportedly reduced dung-insect populations (Liebig et al., 2010).
Important pharmaceuticals in veterinary medicine are antibiotics, antiparasitics, hormones, and analgesics. Antibiotics have come into public focus in recent years, primarily due to antibiotic residues and multiresistant germs found in animal products such as poultry meat and the directly associated risks to human health. Antibiotics are also problematic in an environmental context as many have persistent properties (Ebert et al., 2014). Concerning the environmental impact, the sorption of the compounds does not make a difference: All degrees of expression are disadvantageous for the environment. A strong sorption tendency, e.g., with tetracycline, implies an accumulation of the substance in the soil. A weak sorption tendency, e.g., with sulfamethazine, indicates the substance is leached and dispersed in the environment (Vidaurre et al., 2016). The only sensible options for the environment are the prevention or targeted degradation of the substances.
There has been a positive development in Germany regarding the quantity of antibiotics that veterinarians administer. The delivery volumes have fallen from 1,706 t to 670 t (2011 to 2019), corresponding to a decrease of 60.7%. Since 2015, however, the decline has been less steep, suggesting that current supply levels have gradually approached a new equilibrium. Despite the reduced use of antibiotics, its environmental impact is still pressing. Due to rare data sets, the extent to which manure and agricultural soils are contaminated with antibiotics is difficult to state. As a result of antibiotic use in human and veterinary medicine, increasing resistance can be observed in bacteria in the environment. Compared to soils from 1940, a more than 15-fold increase has been reported for individual antibiotic resistance genes (Knapp et al., 2010). In addition, antibiotics inhibit the growth of plants and algae (Ebert et al., 2014) and have sub-lethal biological activities (Andersson & Hughes, 2014).
In summary, drug residues affect the quality of soil, surface water, and groundwater, have a toxic effect on organisms, and promote the development and spread of antibiotic resistance (Vidaurre et al., 2016). Despite these serious effects, there are no thresholds for drug residues in Germany, neither for manure or biogas digestates nor for sewage sludges (Roskosch & Heidecke, 2018). On the one hand, this is because the analytical proof is quite complex. On the other hand, there are no agreements on specific thresholds at the political level (Roskosch & Heidecke, 2018). Environmental quality standards are neither available on the European nor the national level, but analytical campaigns are performed and comprised in the EU watch list to propose predicted no-effect concentrations (PNEC).
For the mycoremediation of organic pollutants, both fruiting body-forming fungi and purely filamentous fungi (molds) can be used. In both cases, the filamentous state (stage of mycelium growth) is of interest for the degradation of organic pollutants. White-rot fungi are particularly interesting among fruitbody-forming fungi because they are specialists in lignin decomposition. White-rot fungi frequently discussed for mycoremediation include Phanerochaete chrysosporium, Pleurotus ostreatus (oyster mushroom), and Trametes versicolor (turkey tail). Among the purely filamentous fungi, species of the genera Aspergillus, Trichoderma, Penicillium, and Fusarium are often studied for mycoremediation (Bosco & Mollea, 2019). Many Aspergillus and Penicillium species are culturally and economically essential microorganisms. On the one hand, they cause diseases and spoil foods. On the other hand, they are the source of antibiotics and important substances like citric acid and are used for food fermentation (e.g., soy sauce). Using these filamentous fungi on an industrial scale is well-established and state-of-the-art (Troiano et al., 2020). Table 1 and Table 2 provide an overview of selected fungi and their removal rates of organic contaminants.
Most fungi feed by osmotrophy. This form of nutrition involves the release of exoenzymes into the environment to break down complex biological polymers, followed by the uptake of simplified monomers into the cell. The fungal cell structure and anatomy have adapted to this life of constant osmotic pressure generated during osmotrophic feeding. This partly explains why they thrive in nutritionally rich substrates such as plant and animal biomasses, soils, sediments, and detritus (Richards et al., 2012).
White-rot fungi are known for the ability to decompose lignin, the highly recalcitrant “wood-polymer” that provides rigidity to trees and other woody plants. The primary fungal enzymes associated with lignin modification are laccases (class: phenol oxidases), lignin peroxidase, manganese peroxidase, and versatile peroxidase (the latter three classified as heme-containing peroxidases) (Janusz et al., 2017). These extracellular enzymes are nonspecific and attack a broad array of organic compounds, including contaminants of anthropogenic origin. The second mechanism that has been described in organopollutant degradation by fungi is the intracellular cytochrome P450 monooxygenase pathway (Batista-García et al., 2017; Stella et al., 2017; Kaewlaoyoong et al., 2020).
Among white-rot fungi, P. chrysosporium is the best-studied for remediation applications and is regarded as a model organism in mycoremediation (Bosco & Mollea, 2019). In recent years, the edible white-rot fungus P. ostreatus has also gained recognition as an effective species for mycoremediation and has been applied to remove PCBs, PAHs, and drugs among others.
Oxytetracycline is a widespread antibiotic in livestock farming and aquaculture and is discharged in large amounts into the environment globally. To address this issue, a research group has tested the degradation potential of P. ostreatus for oxytetracycline in a liquid medium with two different concentrations (50 and 100 µg mL-1), demonstrating that P. ostreatus can almost completely degrade oxytetracycline in 14 days, leaving less than 3% of the initial concentration in both media (Migliore et al., 2012). Furthermore, laccase activity from P. ostreatus can be used to degrade a number of pharmaceutical compounds. Therefore, lignocellulosic substrate colonized by the oyster mushroom can be directly used for such approaches (Hultberg et al., 2020).
In another research, PCBs were removed by at least 91% in two different liquid media by white-rot fungus Pleurotus sajor-caju. The observed increase in hyphal diameter in the presence of PCBs is believed to be a strengthening of the cell wall in response to environmental stress. Furthermore, the cost-effectiveness of this mycoremediation technique was evaluated. At the end of a 35-day period, the cost of bioremediation in the complex medium was $3.12 g-1 d-1, and in the N-limited medium $0.69 g-1 d-1 (Sadañoski et al., 2019).
In an applied study, soil from a long-closed pentachlorophenol plant heavily contaminated with PCDD/Fs was treated with the white-rot fungus Pleurotus pulmonarius. After 72 days, the overall decontamination rate of 17 investigated PCDD/F compounds was 96%. Interestingly, the contaminated soil was not sterilized for this study, leaving the indigenous microbiome alive. However, PCDD/Fs levels in the non-inoculated control soils did not change, thus making the addition of P. pulmonarius the actual driver for the removal. The study found a correlation (r = 0.88) between PCDD/F degradation and manganese peroxidase production by the fungus during the first 35 days. Furthermore, there was a sharp reduction in PCDD/F concentrations of 55% during the first 7 days. It was suggested that this rapid drop at the beginning was powered by metabolites produced by the fungus while growing on the pre-inoculum substrate prior to the degradation experiment. These metabolites could have served as both mediators and biosurfactants – the former promoting the oxidative reaction of enzymes and the latter improving the bioavailability of PCDD/Fs, altogether enhancing the biodegradation capability (Kaewlaoyoong et al., 2020).
PFAS are highly stable chemical compounds because of the strong carbon-fluorine bond and are difficult to break down. This is probably one reason why there is only a little data on the bioremediation of PFAS. Two studies justify at least cautious optimism: Tseng (2012) showed that P. chrysosporium degrades 50-70% of certain fluorotelomer alcohols (precursor compounds that are converted to PFAS in the environment). Furthermore, in the experiment by Luo et al. (2015), perfluorooctanoic acid was decomposed by approximately 50% upon addition of the enzyme laccase and 1-hydroxybenzotriazole (as laccase mediator) after 157 days. This experiment was done with the laccase obtained from P. ostreatus; no living fungus was involved in the actual degradation experiment.
There is a substantial osmotic pressure coming from the high salinity in marine environments. Nevertheless, even here, fungi are ubiquitous, and they play a significant part in keeping the ecosystem intact. These marine fungi do not form a separate taxonomic clade but are phenotypically determined by their environment. Many live as symbionts in and on other marine organisms, such as sponges, tunicates, corals, and algae. They are also found in coastal habitats, the water column, and the seabed (Gladfelter et al., 2019). Like on land, marine fungi take on the ecological role of decomposers, mineralizing organic matter and driving nutrient cycles (Ariole & Ofuase, 2016; Amend et al., 2019). Some studies revealed a potential for biotechnological application, including biopulping, biobleaching, and dye bleaching. Marine fungi were also described as a valuable source for new natural products. The use of these organisms in a biorefinery approach, including a remediation step for “zero-waste” approaches, was proposed (Silber et al., 2016).
Since marine fungi are a relatively new discovery compared to their relatives on land and hence are still generally underrepresented in research, there are not as many studies regarding mycoremediation with marine-derived fungi. Nevertheless, promising findings have been made (Table 2). Passarini et al. (2011) demonstrated that Aspergillus sclerotiorum and Mucor racemosus, both isolated from cnidarians, are capable of metabolizing the polycyclic aromatic hydrocarbons pyrene and benzo[a]pyrene in liquid media. A. sclerotiorum degraded pyrene by 99.7% after 8 days and benzo[a]pyrene by 76.6% after 16 days, while degradation rates for M. racemosus were 33.8% in 8 days and 51.7% in 16 days for pyrene and benzo[a]pyrene, respectively. The authors suggest that metabolization occurred in the cytochrome P450 pathway.
A research group investigated the diclofenac biodegradation potential of the aquatic fungus Mucor hiemalis. In liquid media of different diclofenac concentrations (10, 25, and 50 µg/L), the removal rate was between 91.4% and 95% after 24 h. As diclofenac concentrations inside the mycelium were very low across all sampling times, bioaccumulation and intracellular degradation were dismissed as the main cause for this rapid decline. Bioadsorption on the mycelium surface was not analyzed and could theoretically be accounted for the diclofenac removal, although the authors consider it more likely to be due to extracellular enzymes secreted by the fungus (Esterhuizen-Londt et al., 2017).
Oxytetracycline is also widely used in intensive aquaculture, posing an environmental risk. In a study devoted to the mycoremediation of oxytetracycline, fungal strains were isolated from sediments collected under salmon cages. In the laboratory, these fungi were exposed to the antibiotic in a liquid medium (250 mg/L oxytetracycline) and achieved degradation between 68% and 78% after 15 days. Here, too, enzymatic metabolization was suggested to be the reason for the removal (Ahumada-Rudolph et al., 2021).
The potential of marine fungi for the degradation of many other compounds of interest and the urgent need for removal strategies have not been studied so far. A good example is the compound metformin. The compound and its degradation product guanylurea (both are endocrine disrupting compounds) have become a problem in many water bodies. Reduction by naturally occurring microbial communities and phytoremediation was shown to be effective in laboratories, but the antimicrobial activity may inhibit degradation (Balakrishnan et al., 2022). Still, due to their highly oxidizing capabilities, fungi might have a potential for the degradation of guanidines, especially in marine soil, as a final sink in the oceans.
Some aspects are worth taking a closer look at: firstly, most research work presented in this review (Table 1, Table 2) has investigated the removal of organic pollutants by fungi in typical laboratory culture media. Only a few used natural matrices. Kaewlaoyoong et al. (2020) have tested the mycoremediation potential in a soil matrix with encouraging results. Batista-García et al. (2017) and González-Abradelo et al. (2019) have examined the fungal remediation ability in polluted wastewaters, likewise with good results. Considering the ongoing severe environmental pollution, the ultimate goal of any research in bioremediation is real-life application. In the future, more applied experiments will be helpful to push the frontier of mycoremediation further towards large-scale applications. A large-scale application does not necessarily ask for industrial infrastructure. Introducing allochthonous fungi to contaminated sites (bioaugmentation) or stimulating indigenous fungi with nutrients (biostimulation) could be ways of treating large amounts of contamination without industry-like efforts in terms of money and energy (Bosco & Mollea, 2019). However, if contaminated substrates are already present in an industrial context (e.g., wastewater treatment plant; agriculture in industrialized countries), retrofitting the facilities in order to enable mycoremediation is conceivable.
The second aspect worth noting is that in the majority of reviewed experiments (Tables 1, 2), alternative carbon sources were present in the culture media, which is a strong indication that organic pollutants were cometabolized by the fungi in question. However, some studies explored the potential of fungi to use organic contaminants as sole carbon source (Wu et al., 2009; Pezzella et al., 2017; González-Abradelo et al., 2019). Among these studies, the minimum removal observed was with a marine-derived Aspergillus species removing 60% of initial benzo[a]pyrene concentration in a mineral salt medium (containing trace elements and some other nutrients except carbon) after 12 days (Wu et al., 2009). The best result was achieved with Trametes versicolor removing 100% of a mixture of endocrine disrupting chemicals in deionized water during each cycle (1 day) in a semi-batch bioreactor. This exceeded the preceding test, where T. versicolor needed 4 days to remove the same chemicals in a potato dextrose yeast extract broth (Pezzella et al., 2017). The redundancy of nutrient addition, especially carbon sources, at least in these examples, is an essential finding in terms of cost-effectiveness and simplicity of mycoremediation technologies. However, Pezzella et al. (2017) raised the question of whether the organic contaminants are actually being used as carbon sources or if these compounds are rather cometabolized at the expense of fungal storage energy.
Another aspect to consider is that it is necessary to distinguish between the removal and degradation of a contaminant (Table 1, Table 2). Fungi could bioaccumulate a contaminant inside the cells or adsorb contaminants on the cell surface, thereby clearing the medium or substrate. While this mode of action is intended for heavy metal remediation, it does not exploit the full potential when it comes to organic pollutants where a degradation to non-toxic compounds or, if possible, to CO2 is desired. Studying the effective degradation will be helpful in defining the remediation potentials of a fungus.
The last aspect to highlight is that some studies used non-sterile substrates (soil or wastewater; Table 1, Table 2) (Batista-García et al., 2017; González-Abradelo et al., 2019; Kaewlaoyoong et al., 2020). Kaewlaoyoong et al. (2020) emphasized that indigenous microbiota could not remove the contaminants in untreated controls, suggesting that the addition of the fungus is responsible for the pollutant removal. Although the number of studies involving non-sterilized substrates is still sparse, these results are further strong arguments regarding the cost-effectiveness and viability of mycoremediation solutions because sterilization is often not an option.
The described findings demonstrate the powerful capability of marine fungi to degrade organic pollutants. The growth behavior and filamentous morphology are advantageous to pervade complex matrices. By virtue of their natural habitat, marine fungi also bring a high resistance to osmotic pressure, which is a good premise for their growth in sewage sludge and manure (Gladfelter et al., 2019).
Many studies comprise marine species and genera also present in terrestrial habitats. Considering the ecological role as saprotrophs, it is likely that degrading activities are also found in true marine taxa, enabling remediation in challenging, high osmotic matrices. Moreover, the marine environment, too, is affected by pollution with POPs, pharmaceuticals, and other anthropogenic pollutants (Strehse et al., 2022). A deeper understanding of mycoremediation with marine fungi can also be helpful for the application in the natural environment. In future research, the stability of the fungal degradation activity in microbial (remediation) communities has to be proven in scaled approaches and real-life conditions.
Fungi from all habitats play a role in mineralization processes and possess metabolic pathways to use complex and even toxic organic compounds as carbon sources. All these characteristics contribute to the distinguished potential of fungi for bioremediation, especially the use of marine strains. To harness this potential, in and ex situ studies are urgently needed to establish mycoremediation processes for contaminated sewage sludges and manure.
Mycoremediation is not the single solution to reduce organic contaminations in sludges and manure. Measures that prevent or reduce pollution in the first place must be ramped up and consistently implemented. All applicable environmentally friendly remediation techniques should be put into practice in existing or planned wastewater treatment plants, e.g., using other organisms (plants, bacteria, archaea). Approaching the issue of pollution with a set of different solutions is necessary for generating contamination-free sewage sludges and manure suitable for responsible soil fertilization.
DAS and AL drafted the manuscript, DAS did the in-depth literature research and wrote the major part of the review, DAS and AL reviewed and edited the manuscript. All authors contributed to the article and approved the submitted version.
DAS receives a doctoral scholarship (No. 14/12-26) from the Gesellschaft für Energie und Klimaschutz Schleswig-Holstein GmbH (EKSH), Germany. The ZAiT is part of the project Grenzland INNOVATIV Schleswig-Holstein [innovative border region Schleswig-Holstein] funded by the German Federal Ministry of Education and Research (BMBF) in the context of “Innovative Hochschule” [innovative university]. We also acknowledge financial support by the state of Schleswig-Holstein within the funding program Open Access-Publikationsfonds.
The authors declare that the research was conducted in the absence of any commercial or financial relationships that could be construed as a potential conflict of interest.
All claims expressed in this article are solely those of the authors and do not necessarily represent those of their affiliated organizations, or those of the publisher, the editors and the reviewers. Any product that may be evaluated in this article, or claim that may be made by its manufacturer, is not guaranteed or endorsed by the publisher.
Adler N., Balzer F., Blondzik K., Brauer F., Chorus I., Ebert I., et al. (2018). Antibiotika und antibiotikaresistenzen in der umwelt: Hintergrund, herausforderungen und handlungsoptionen (Dessau-Roßlau, Germany: German Environment Agency) 2363–829X.
Ahumada-Rudolph R., Novoa V., Becerra J., Cespedes C., Cabrera-Pardo J. R. (2021). Mycoremediation of oxytetracycline by marine fungi mycelium isolated from salmon farming areas in the south of Chile. Food Chem. Toxicol. 152, 112198. doi: 10.1016/j.fct.2021.112198
Ahumada-Rudolph R., Novoa V., Sáez K., Martínez M., Rudolph A., Torres-Diaz C., et al. (2016). Marine fungi isolated from Chilean fjord sediments can degrade oxytetracycline. Environ. Monit. Assess. 188 (8), 468. doi: 10.1007/s10661-016-5475-0
Ambrosio-Albuquerque E. P., Cusioli L. F., Bergamasco R., Sinópolis Gigliolli A. A., Lupepsa L., Paupitz B. R., et al. (2021). Metformin environmental exposure: A systematic review. Environ. Toxicol. Pharmacol. 83, 103588. doi: 10.1016/j.etap.2021.103588
Amend A., Burgaud G., Cunliffe M., Edgcomb V. P., Ettinger C. L., Gutiérrez M. H., et al. (2019). Fungi in the marine environment: Open questions and unsolved problems. MBio 10 (2), e01189-18. doi: 10.1128/mBio.01189-18
Andersson D. I., Hughes D. (2014). Microbiological effects of sublethal levels of antibiotics. Nat. Rev. Microbiol. 12 (7), 465–478. doi: 10.1038/nrmicro3270
Ariole C. N., Ofuase N. (2016). Anaerobic denitrification and biotechnological potentials of filamentous fungi isolated from coastal marine sediment. Int. J. Microbiol. Mycol 4 (3), 8–15.
Balakrishnan A., Sillanpää M., Jacob M. M., Vo D.-V. N. (2022). Metformin as an emerging concern in wastewater: Occurrence, analysis and treatment methods. Environ. Res. 213, 113613. doi: 10.1016/j.envres.2022.113613
Batista-García R. A., Kumar V. V., Ariste A., Tovar-Herrera O. E., Savary O., Peidro-Guzmán H., et al. (2017). Simple screening protocol for identification of potential mycoremediation tools for the elimination of polycyclic aromatic hydrocarbons and phenols from hyperalkalophile industrial effluents. J. Environ. Manage. 198 (Pt 2), 1–11. doi: 10.1016/j.jenvman.2017.05.010
Birolli W. G., Santos A., de D., Alvarenga N., Garcia A. C. F. S., Romão L. P. C., et al. (2018). Biodegradation of anthracene and several PAHs by the marine-derived fungus. Cladosporium sp. CBMAI 1237. Mar. pollut. Bull. 129 (2), 525–533. doi: 10.1016/j.marpolbul.2017.10.023
Bosco F., Mollea C. (2019). “Mycoremediation in soil,” in Environmental chemistry and recent pollution control approaches. Eds. Saldarriaga-Noreña H., Alfonso Murillo-Tovar M., Farooq R., Dongre R., Riaz S. (London, United Kingdom: IntechOpen).
Chatterjee A., Abraham J. (2019). Mycoremediation of 17 β-estradiol using Trichoderma citrinoviride strain AJAC3 along with enzyme studies. Environ. Prog. Sustain. Energy 38 (4), 13142. doi: 10.1002/ep.13142
civity Management Consultants (2017). Arzneimittelverbrauch im Spannungsfeld des demografischen Wandels (Berlin, Germany: civity Management Consultants GmbH & Co. KG).
Cvančarová M., Křesinová Z., Filipová A., Covino S., Cajthaml T. (2012). Biodegradation of PCBs by ligninolytic fungi and characterization of the degradation products. Chemosphere 88 (11), 1317–1323. doi: 10.1016/j.chemosphere.2012.03.107
Ebert I., Amato R., Hein A., Konradi S. (2014). Arzneimittel in der Umwelt - vermeiden, reduzieren, überwachen (Dessau-Roßlau, Germany: German Environment Agency).
EPA U. S. (2006). An inventory of sources and environmental releases of dioxin-like compounds in the united states for the years 1987, 1995, and 2000. united states environmental protection agency (Washington, DC, USA: National Center for Environmental Assessment).
Esterhuizen M., Behnam Sani S., Wang L., Kim Y. J., Pflugmacher S. (2021). Mycoremediation of acetaminophen: Culture parameter optimization to improve efficacy. Chemosphere 263, 128117. doi: 10.1016/j.chemosphere.2020.128117
Esterhuizen-Londt M., Hendel A.-L., Pflugmacher S. (2017). Mycoremediation of diclofenac using Mucor hiemalis. Toxicol. Environ. Chem. 99 (5-6), 795–808. doi: 10.1080/02772248.2017.1296444
European Commission (2019). Report from the commission to the European parliament, the council, the European economic and social committee and the committee of regions: On the review and update of the second European community implementation plan in accordance with article 8(4) of regulation no 850/2004 on persistent organic pollutants (Brussels, Belgium: European Commission).
Flessa H., Don A., Jacobs A., Dechow R., Tiemeyer B., Poeplau C. (2018). Humus in landwirtschaftlich genutzten Böden Deutschlands: Ausgewählte Ergebnisse der Bodenzustandserhebung. Thünen-Inst Clim -Smart Agric. [Bonn, Germany: Bundesministerium für Ernährung und Landwirtschaft (Federal Ministry of Food and Agriculture)].
Gladfelter A. S., James T. Y., Amend A. S. (2019). Marine fungi. Curr. Biol. 29 (6), R191–R195. doi: 10.1016/j.cub.2019.02.009
González-Abradelo D., Pérez-Llano Y., Peidro-Guzmán H., Del Sánchez-Carbente M. R., Folch-Mallol J. L., Aranda E., et al. (2019). First demonstration that ascomycetous halophilic fungi (Aspergillus sydowii and Aspergillus destruens) are useful in xenobiotic mycoremediation under high salinity conditions. Bioresour. Technol. 279, 287–296. doi: 10.1016/j.biortech.2019.02.002
Green R. E., Newton I., Shultz S., Cunningham A. A., Gilbert M., Pain D. J., et al. (2004). Diclofenac poisoning as a cause of vulture population declines across the Indian subcontinent. J. Appl. Ecol. 41 (5), 793–800. doi: 10.1111/j.0021-8901.2004.00954.x
Hultberg M., Ahrens L., Golovko O. (2020). Use of lignocellulosic substrate colonized by oyster mushroom (Pleurotus ostreatus) for removal of organic micropollutants from water. J. Environ. Manage. 272, 111087. doi: 10.1016/j.jenvman.2020.111087
International Agency for Research on Cancer (1997). Polychlorinated dibenzo-para-Dioxins and polychlorinated dibenzofurans. IARC monographs on the evaluation of carcinogenic risks to humans (Lyon, France: International Agency for Research on Cancer).
International Agency for Research on Cancer (2012). Chemical agents and related occupations. IARC monographs on the evaluation of carcinogenic risks to humans (Lyon, France: International Agency for Research on Cancer).
International Agency for Research on Cancer (2016). Polychlorinated biphenyls and polybrominated biphenyls. IARC monographs on the evaluation of carcinogenic risks to humans (Lyon, France: International Agency for Research on Cancer).
International Agency for Research on Cancer (2017). “Some chemicals used as solvents and in polymer manufacture,” in IARC monographs on the evaluation of carcinogenic risks to humans (Lyon, France: International Agency for Research on Cancer).
Janusz G., Pawlik A., Sulej J., Swiderska-Burek U., Jarosz-Wilkolazka A., Paszczynski A. (2017). Lignin degradation: microorganisms, enzymes involved, genomes analysis and evolution. FEMS Microbiol. Rev. 41 (6), 941–962. doi: 10.1093/femsre/fux049
Kaewlaoyoong A., Cheng C.-Y., Lin C., Chen J.-R., Huang W.-Y., Sriprom P. (2020). White rot fungus Pleurotus pulmonarius enhanced bioremediation of highly PCDD/F-contaminated field soil via solid state fermentation. Sci. Total Environ. 738, 139670. doi: 10.1016/j.scitotenv.2020.139670
Kidd K. A., Blanchfield P. J., Mills K. H., Palace V. P., Evans R. E., Lazorchak J. M., et al. (2007). Collapse of a fish population after exposure to a synthetic estrogen. Proc. Natl. Acad. Sci. United States America 104 (21), 8897–8901. doi: 10.1073/pnas.0609568104
Knapp C. W., Dolfing J., Ehlert P. A. I., Graham D. W. (2010). Evidence of increasing antibiotic resistance gene abundances in archived soils since 1940. Environ. Sci. Technol. 44 (2), 580–587. doi: 10.1021/es901221x
Liebig M., Fernandez A. A., Blübaum-Gronau E., Boxall A., Brinke M., Carbonell G., et al. (2010). Environmental risk assessment of ivermectin: A case study. Integr. Environ. Assess. Manage. 6 Suppl, 567–587. doi: 10.1002/ieam.96
Luo Y., Guo W., Ngo H. H., Nghiem L. D., Hai F. I., Zhang J., et al. (2014). A review on the occurrence of micropollutants in the aquatic environment and their fate and removal during wastewater treatment. Sci. Total Environ. 473–474, 619–641. doi: 10.1016/j.scitotenv.2013.12.065
Luo Q., Lu J., Zhang H., Wang Z., Feng M., Chiang S.-Y. D., et al. (2015). Laccase-catalyzed degradation of perfluorooctanoic acid. Environ. Sci. Technol. Lett. 2 (7), 198–203. doi: 10.1021/acs.estlett.5b00119
Migliore L., Fiori M., Spadoni A., Galli E. (2012). Biodegradation of oxytetracycline by Pleurotus ostreatus mycelium: A mycoremediation technique. J. Hazard. Mater. 215–216, 227–232. doi: 10.1016/j.jhazmat.2012.02.056
Oaks J. L., Gilbert M., Virani M. Z., Watson R. T., Meteyer C. U., Rideout B. A., et al. (2004). Diclofenac residues as the cause of vulture population decline in Pakistan. Nature 427 (6975), 630–633. doi: 10.1038/nature02317
Passarini M. R. Z., Rodrigues M. V. N., Da Silva M., Sette L. D. (2011). Marine-derived filamentous fungi and their potential application for polycyclic aromatic hydrocarbon bioremediation. Mar. pollut. Bull. 62 (2), 364–370. doi: 10.1016/j.marpolbul.2010.10.003
Paustian K., Larson E., Kent J., Marx E., Swan A. (2019). Soil c sequestration as a biological negative emission strategy. Front. Clim. 1, 8. doi: 10.3389/fclim.2019.00008
Pezzella C., Macellaro G., Sannia G., Raganati F., Olivieri G., Marzocchella A., et al. (2017). Exploitation of Trametes versicolor for bioremediation of endocrine disrupting chemicals in bioreactors. PloS One 12 (6), e0178758. doi: 10.1371/journal.pone.0178758
Richards T. A., Jones M. D. M., Leonard G., Bass D. (2012). Marine fungi: Their ecology and molecular diversity. Annu. Rev. Mar. Sci. 4, 495–522. doi: 10.1146/annurev-marine-120710-100802
Roskosch A., Heidecke P. (2018). Klärschlammentsorgung in der Bundesrepublik Deutschland (Dessau-Roßlau, Germany: German Environment Agency).
Sadañoski M. A., Benítez S. F., Fonseca M. I., Velázquez J. E., Zapata P. D., Levin L. N., et al. (2019). Mycoremediation of high concentrations of polychlorinated biphenyls withPleurotus sajor-caju LBM 105 as an effective and cheap treatment. J. Environ. Chem. Eng. 7 (6), 103453. doi: 10.1016/j.jece.2019.103453
Sanderman J., Hengl T., Fiske G. J. (2017). Soil carbon debt of 12,000 years of human land use. Proc. Natl. Acad. Sci. United States America 114 (36), 9575–9580. doi: 10.1073/pnas.1706103114
Shang Z., Salim A. A., Khalil Z., Bernhardt P. V., Capon R. J. (2016). Fungal biotransformation of tetracycline antibiotics. J. Org. Chem. 81 (15), 6186–6194. doi: 10.1021/acs.joc.6b01272
Silber J., Kramer A., Labes A., Tasdemir D. (2016). From discovery to production: Biotechnology of marine fungi for the production of new antibiotics. Mar. Drugs 14 (7), 137. doi: 10.3390/md14070137
Stella T., Covino S., Čvančarová M., Filipová A., Petruccioli M., D’Annibale A., et al. (2017). Bioremediation of long-term PCB-contaminated soil by white-rot fungi. J. Hazard. Mater. 324 (Pt B), 701–710. doi: 10.1016/j.jhazmat.2016.11.044
Strehse J. S., Bünning T. H., Maser E. (2022). “Ocean pollution–a selection of anthropogenic implications,” in Transitioning to sustainable life below water, vol. pp . Eds. Ekau W., Hornidge A.-K. (Basel, Switzerland: MDPI) 14, 11–58.
Tassoulas L. J., Robinson A., Martinez-Vaz B., Aukema K. G., Wackett L. P. (2021). Filling in the gaps in metformin biodegradation: A new enzyme and a metabolic pathway for guanylurea. Appl. Environ. Microbiol. 87 (11), e03003-20. doi: 10.1128/AEM.03003-20
Triebskorn R., Casper H., Scheil V., Schwaiger J. (2007). Ultrastructural effects of pharmaceuticals (carbamazepine, clofibric acid, metoprolol, diclofenac) in rainbow trout (Oncorhynchus mykiss) and common carp (Cyprinus carpio). Anal. Bioanal. Chem. 387 (4), 1405–1416. doi: 10.1007/s00216-006-1033-x
Troiano D., Orsat V., Dumont M. J. (2020). Status of filamentous fungi in integrated biorefineries. Renewable Sustain. Energy Rev. 117, 109472. doi: 10.1016/j.rser.2019.109472
Tseng N. S. (2012). Feasibility of biodegradation of polyfluoroalkyl and perfluoroalkyl substances (Los Angeles, CA: University of California, Los Angeles). ProQuest ID: Tseng_ucla_0031N_10983. Merritt ID: ark:/13030/m5vq36g8. Available at: https://escholarship.org/uc/item/2x47296b.
United Nations Environment Programme (2021). “Emissions gap report 2021: The heat is on - a world of climate promises not yet delivered,” in UNEP emissions gap reports: 12 (Nairobi, Kenya: United Nations Environment Programme).
Vidaurre R., Lukat E., Steinhoff-Wagner J., Ilg Y., Petersen B., Hannappel S., et al. (2016). Konzepte zur Minderung von Arzneimitteleinträgen aus der landwirtschaftlichen Tierhaltung in die Umwelt (Dessau-Roßlau, Germany: German Environment Agency).
Keywords: soil carbon sequestration, bioremediation, persistent organic pollutants, pharmaceutical residues, contaminants of emerging concern, drug removal, negative emissions technology, agriculture
Citation: Stiebeling DA and Labes A (2022) Mycoremediation of sewage sludge and manure with marine fungi for the removal of organic pollutants. Front. Mar. Sci. 9:946220. doi: 10.3389/fmars.2022.946220
Received: 17 May 2022; Accepted: 24 August 2022;
Published: 13 September 2022.
Edited by:
Sergio Balzano, Stazione Zoologica Anton Dohrn Napoli, ItalyReviewed by:
Prem Chandra, BBA University, IndiaCopyright © 2022 Stiebeling and Labes. This is an open-access article distributed under the terms of the Creative Commons Attribution License (CC BY). The use, distribution or reproduction in other forums is permitted, provided the original author(s) and the copyright owner(s) are credited and that the original publication in this journal is cited, in accordance with accepted academic practice. No use, distribution or reproduction is permitted which does not comply with these terms.
*Correspondence: Antje Labes, YW50amUubGFiZXNAaHMtZmxlbnNidXJnLmRl
Disclaimer: All claims expressed in this article are solely those of the authors and do not necessarily represent those of their affiliated organizations, or those of the publisher, the editors and the reviewers. Any product that may be evaluated in this article or claim that may be made by its manufacturer is not guaranteed or endorsed by the publisher.
Research integrity at Frontiers
Learn more about the work of our research integrity team to safeguard the quality of each article we publish.