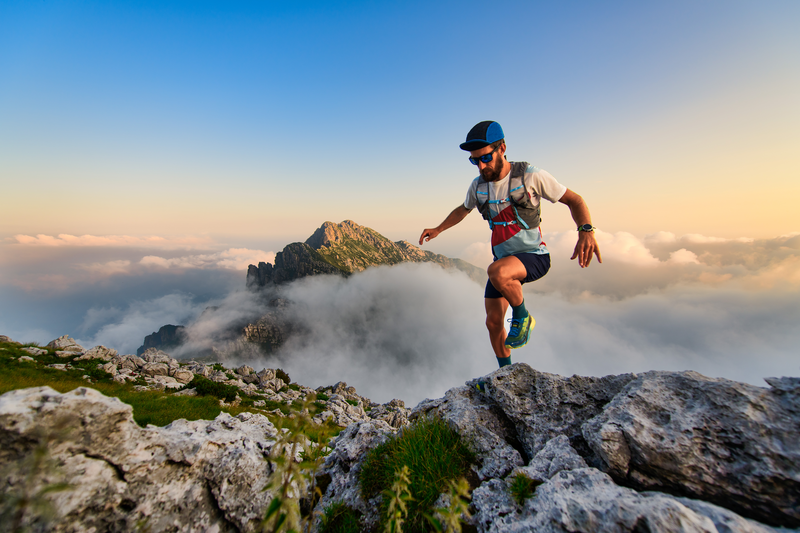
95% of researchers rate our articles as excellent or good
Learn more about the work of our research integrity team to safeguard the quality of each article we publish.
Find out more
ORIGINAL RESEARCH article
Front. Mar. Sci. , 02 August 2022
Sec. Marine Conservation and Sustainability
Volume 9 - 2022 | https://doi.org/10.3389/fmars.2022.946114
Marine fishes are sensitive to the environment during their early life stages. This study adopts a habitat suitability index (HSI) model to evaluate the environmental suitability for early stages of anchovy (Engraulis japonicus) in Laizhou Bay. Instead of calculating the suitability of spawning grounds and nursery grounds independently or the simple average of the two, an individual-based model is used to simulate the drifting trajectories of anchovy eggs to establish the link between a spawning ground and its corresponding nursery ground. The HSI of early life habitat is determined by averaging the HSI value of the paired two grounds. The model results suggest that a small patch at eastern Laizhou Bay (near 120.1 E°, 37.6 N°) is the most critical and suitable area for anchovy in early life stages. It can provide an appropriate habitat for anchovy eggs and allow the eggs to migrate to the inside of the bay with plenty of plankton, which would benefit the newly hatching larvae. The results indicate the significant impact of hydrodynamic transport on fishery recruitment process, which should not be neglected in habitat quality evaluation. The model adopted in this study is applied to anchovy as a case study, but it is also exportable to other species of commercial interest.
In the ocean, the early life stages of fishes are the most vulnerable and sensitive stages in their life where small-scale environmental changes could dramatically impact the recruitment process (Houde and Hoyt, 1987). As early as 1914, Johan Hjort of Norway proposed the pioneering “critical period hypothesis”, which holds the belief that the amount of population recruitment is mainly controlled by the prey abundance of the environment when fish larvae first transitioned to exogenous feeding (Hjort, 1914). In 1988, known as the “growth mortality” hypothesis, Anderson and Gerbing (1988) proposed that fast-growing individuals have higher chances of surviving than slow-growing ones. After that, the “match–mismatch hypothesis” assumed that the time span of temporal overlap between juvenile fish and plankton is a key factor influencing population growth in the later period (Cushing, 1990), stressing the importance of prey availability in early life stages. Despite different views on the mechanism of interactions between fishery species and the environment among researchers, the significant impact of the environment on fishes in early life stages has become a consensus. An assessment on the quality of early life habitats can help us in environment management and obtaining sustainable fishery productions.
The habitat suitability index (HSI) model, first used to evaluate the suitability of terrestrial habitats (U.S.Fish and WildLife Services, 1981), has now been widely applied in marine fishery research and management. From climate-scale impacts on habitat variation (Yu and Chen, 2021) to offshore scale habitat assessment (Theuerkauf and Lipcius, 2016), the HSI model assists us in quantitatively characterizing the environmental constraints to a non-dimensional value ranging from 0 to 1, providing concise and readable assessments on habitats.
HSI can be used to evaluate the quality of early life habitats, which include spawning grounds and nursery grounds. However, the environmental preferences of fishes at different life stages may vary (Rijnsdorp et al., 2009)—for example, anchovy tends to live at depths of 0 to 100 m (Ohshimo and Hamatsu, 1996), and the optimal temperature for anchovy is about 21°C (Oozeki et al., 2007), whereas anchovy eggs are generally in the upper 10–30 m of the water column (Coombs et al., 2004), and the preferred temperature is about 16.5°C (Lluch-Belda et al., 1991). It is better to independently evaluate the suitability of spawning grounds and nursery grounds and integrate the suitability assessment results using appropriate methods.
For most marine fish species, their eggs would be drifted by wave and tidal current for long distances away from the spawning grounds (Van der Molen et al., 2007). The hydrodynamic process enables eggs to migrate to new nursery grounds, significantly influencing potential population recruitment (Hjort, 1926). A suitable early life habitat should not only meet the requirements for egg incubation but also ensure that the eggs can be drifted to an adequate location for larvae to grow (Ottersen et al., 2014). Most of the larvae are not produced locally but drift from other areas. The connectivity between spawning grounds and nursery grounds of massive eggs cannot be detected by field observation, while the coupled bio-physical individual-based model (IBM) is a reliable tool to probe the hydrodynamic effects on marine organisms in the larval stage, especially in estimating connectivity within stocks (Patti et al., 2018; Falcini et al., 2020) and the responding of recruitment to environment variation (Patti et al., 2020; Russo et al., 2022). A bio-physical method has been applied in the Mediterranean and clarified the link between ocean dynamics and the fate of small pelagic fish larvae, including anchovy, which is also proven to be effective (Cuttitta et al., 2018; Torri et al., 2018).
In Laizhou Bay, anchovy has suffered from severe overfishing since the 1990s, which caused the population of anchovy to decrease significantly over the last three decades (Li et al., 2015). This study aims to evaluate the early life habitat quality of anchovy in Laizhou Bay in order to evaluate the effectiveness of the HSI model on the environmental suitability for the survival of larvae and eggs of fish species. In this sense, a coupled bio-physical IBM is constructed to simulate the spatial connectivity of anchovy eggs and larvae, and based on eggs–larvae connectivity, the suitability of early life habitats for anchovy is evaluated using the HSI method. Both laboratory-based and field-based observation indicate that the survival probability of anchovy larvae is significantly affected by the growth rate in early life stage (Takahashi and Watanabe, 2004), while the growth rate is greatly influenced by adequate ambient temperature (Takasuka and Aoki, 2006). Anchovies tend to feed and live in shallow ashore areas because of higher food availability in the coastal sea (Yu et al., 2020), and river-diluted freshwater strongly affects the concentration of dissolved nutrients for potential prey plankton (Pinckney et al., 2001). Therefore, depth, SST, and SSS are used to construct the HSI model. Since anchovy spawns in coastal shelf seas (Oozeki et al., 2007) and anchovy eggs are positively buoyant (Coombs et al., 2004), the surface current field from the hydrodynamic model is used to drive the eggs’ movement in the IBM.
The Bohai Sea Fishery Resources Oceanographic Survey, which was carried out by the Yellow Sea Fisheries Research Institute in May 2015, provided the spatial distribution data of anchovy eggs and larvae used in this paper. Macroplankton net was used for horizontal trawling, each trawling lasted for 10 minutes, the inlet diameter of the net was 80 cm, and the size of the net mesh was 0.5 mm. The specimens were fixed with 5% seawater formaldehyde solution and were manually counted in morphological identification under a stereomicroscope. Water depth was extracted from high-resolution sea chart. SST and SSS came from observation during the survey.
The abundance of eggs and larvae is reflected by the normalized relative abundance index (RAI):
where i is the index of one of the monitoring stations, niis the number of eggs or larvae counted at that monitoring station, and max(ni) is the maximum number of ni. The logarithmic normalization of RAI is employed as the indicator of habitat quality:
where RAIiis the relative abundance index of station indexed by i, and RAImax and RAIminare the maximum and minimum values of RAI among all stations, respectively.
As is seen in Figure 1, the Laizhou Bay is shallow, with few areas deeper than 20 m. The SST in June decreases from 20°C ashore to about 12°C at the mouth. Due to the influence of freshwater from the Yellow River, the salinity close to the southern part of the estuary is low at 29, while that outside the bay can reach 32.
Figure 1 Spatial distribution of some environmental factors. (A) Water depth in Laizhou Bay. The length of the colored lines above the contour map represents the relative abundance index of the larvae and eggs at the observation stations. Horizontal lines stand for eggs, and vertical lines stand for larvae. (B) Sea surface temperature. (C) Sea surface salinity.
The suitability index (SI) describes species’ preference for the environment, so it is also called the preference curve or suitability curve (Gillenwater et al., 2006; Yi et al., 2017). Some researchers in previous studies used “experts’ experience” to set the preference curve, reflecting practitioners’ comprehension of the interaction between the environment and species (Barnes et al., 2007; Kim et al., 2015). More recent studies adopted the regression method (Gómez et al., 2007; Cho et al., 2012; Lee et al., 2019) because it can objectively depict the relationship between species and the environment. In this article, regression based on normal distribution is used to get the SI. Organisms have the highest preference when some environmental factors reach the optimal value, and in extreme environments, organisms cannot grow well. This dome shape of the SI curve can be well described with a normal distribution curve:
where SI is the suitability index, E is the measured value of environmental factors, and i represents SST, SSS, or DEP. A, b, and c are the fitting parameters that control the height, the extent, and the peak location of the preference curve, respectively.
Larvae prefer to live in shallow waters, and there are almost no larvae existing in waters deeper than 20 m (Figure 2). Eggs are widely dispersed in waters between 15 and 20 m in depth. The larvae are mainly in areas with SST of 15–20°C, while the eggs prefer areas with lower SST, i.e., 10–18°C. The majority of the larvae are found in an area with a salinity of 28 to 32, and the eggs are found in salty waters (salinity >30) more often, which only appear outside the bay.
Figure 2 Preference curves for DEP, SST, and SSS. The first row represents larvae, and the second row represents anchovy eggs. The three columns are the suitability indexes for DEP, SST, and SSS. The bar shows the relative abundance index (RAI) data, grouped by the value of environmental factors. The average RAI is taken as representative in the group. The orange curve is the regression result.
The HSI for anchovy eggs is considered as the arithmetic mean of the SI values of eggs for SST, SSS, and DEP, which is presented as follows:
Similarly, HSIlarvae is calculated using:
Early life habitat consists of the spawning ground for anchovy eggs and the nursery ground. Eggs (green ones in area i in Figure 3) drift from area i to area k—area i is their spawning ground, while area k is the nursery ground where larvae live and grow. The larvae in area i (orange ones) came from somewhere else and have no biological relationship with the eggs in area i (green ones). It does not make sense to pair the HSI of the green eggs and orange larvae together. In a lagrangian view, instead of observing in one fixed spot, we track one batch of eggs in moving and evaluate their response to the environment. Therefore, the HSI for early life stages of area i is defined as:
To determine the connectivity between the spawning ground and the nursery ground, a biophysical model is used to simulate the anchovy behaviors in the hatching process. A three-dimensional ocean circulation model FVCOM (an unstructured grid, finite-volume coastal ocean model) is introduced to generate the hourly current field. Briefly, FVCOM is an ocean circulation model using the finite volume numerical scheme, which works on triangular unstructured grids to fit complex coastlines (Chen et al., 2003). The hydrodynamic model used here has already been validated and published (Lou et al., 2022), and it demonstrated excellent consistency between simulation results and observational data.
The domain of the model is 117.57 to 124.00°E and 37.08 to 40.94° N. The maximum grid size is 5,000 m at open sea, and the minimum grid is 200 m nearshore. The model runs on five vertical sigma layers and is driven by the tide-induced water level change at the open boundary across Bohai Strait. The tidal elevation is predicted by harmonic constants extracted from TPXO7 (Egbert and Erofeeva, 2002), where four major tidal components, including M2, S2, K1, and O1, are considered. The wind is taken into account in the model, and it is derived from the ERA5 dataset (Hersbach et al., 2020).
We use virtual lagrangian particles to explore the trajectories of eggs in Laizhou Bay. Fish eggs have very small volume and mass; therefore, tidal current governs their movement, which means that they are passively advected by the flow field. The trajectories are obtained by solving this differential equation through the fourth-order Runge–Kutta method:
where it is the time, and is the location of the particle. Because the anchovy eggs in Laizhou Bay are mostly in the upper layer of the sea (Coombs et al., 2004; Bian et al., 2022), is the flow field of the surface sigma layer in the model described in Section 2.3.1.
The accumulated temperature (Taccu) of the eggs, which is the integral of ambient temperature over time, largely determines the anchovy’s incubation state. Anchovy eggs start growing only when the temperature is above 10.26°C (Wan, 2008), which is known as the “biological zero”. Anchovy eggs develop into larvae when Taccu exceeds 423°C* hours (Wan, 2008).
The temperature:
Taccu is calculated by:
where T is the ambient temperature of the particle at location and time t Taccu(t) is the accumulated temperature of anchovy eggs at time t, and Tbiozero is the minimum temperature at which anchovy eggs can still grow (10.26°C for anchovy eggs).
Since the model intends to explore the spatial connectivity between spawning ground and nursery ground instead of the number of successful incubation cases, all particles are set immortal, and they are homogeneously distributed in the study domain. In total, 10,000 particles are seeded in the model to represent the anchovy eggs, and the releasing time is 1 o’clock, May 15, during the main spawning seasons of anchovy (Bian et al., 2022). For each timestep, the temperature and salinity around the particle are recorded to calculate the Taccu(t). Taccu(t) is set to zero at the initial location.
Figure 4 shows the spatial distribution of the HSI of larvae and eggs. We can observe that anchovy eggs (Figure 4A) prefer deep waters that are distant from the coastline. The HSI of eggs in Laizhou Bay decreases from outside to inside the bay and reaches a very low value at the bottom of the bay in shallow coastal waters. In addition, longer-term data also shows that anchovy eggs prefer high salinity and high transparency in the northeastern part of Laizhou Bay (Bian et al., 2022), which supports the validity of our HSI model. On the contrary, anchovy larvae (Figure 4B) prefer shallow waters near the shore, where the SST is higher and the SSS is lower than those in deeper areas. It provides the larvae with much more plankton to feed on, which could reduce mortality and increase population recruitment.
Figure 4 Habitat suitability of eggs and larvae. (A) Habitat suitability index (HSI) of eggs. (B) HSI of larvae.
The current in Laizhou Bay is tide-dominated and influenced by the wind. At low water (Figure 5A) or high water (Figure 5C) phase, the current speed is less than 0.3 m·s·s-¹ at most areas of the domain. At the flood (Figure 5B) or ebb (Figure 5D) phase, the current direction is southwest or northeast, and the current speed is about 0.4 m·s·s-¹ at most areas and can reach 0.7 m·s·s-¹ near the headlands.
Figure 5 Current field in Laizhou Bay in May 11 at times (A) 11:00, (B) 15:00, (C) 18:00, and (D) 22:00. The illustration on the lower right corner of each figure is the water elevation of the red dot in the center of Laizhou Bay.
According to the results of the IBM, eggs from the west side of Laizhou Bay are significantly transported to the northwest during incubation (Figure 6). The drifting distance is mostly above 20 km, while eggs from the east side of Laizhou Bay generally move to the southwest, and the drifting distances are about 10–20 km. Near 119.5° E and 37.4° N, the residual current is weak, and the eggs and their descendant larvae are almost at the same location, with a drifting distance of less than 5 km. Near the islands on the northeast corner of the domain, the trajectories are more erratic due to the effect of island bypass currents. Eggs travel along complicated trajectories between the islands and the coastline, with a considerable drifting distance larger than 25 km.
Figure 6 Drifting trajectories of eggs. The black dots are the release locations of anchovy eggs, and the colored lines are their drifting trajectories.
Some intriguing spatial patterns are marked in red in Figure 7A. Longer transport distances are more likely to appear close to headlands or long, projecting artificial constructions, e.g., the wharves, which could induce intense vortices around them (Black et al., 2005) and cause a stronger material transport than areas at the center of Laizhou Bay. The average drifting distance during incubation is 17.8 km. The 25, 50, and 75% percentiles are 11.9, 19.2, and 23.4 km, respectively.
The time duration increases from inside to outside the bay because of the inverse relationship between incubation time duration and ambient temperature. At the bottom of the bay, the time duration is about 60 h, and it exceeds 2 h in the deep waters in the outer Bohai Sea. The average time for egg incubation is 73.3 h, where the 25, 50, and 75% percentiles are 48.2, 59.0, and 87.0 h, respectively.
Hydrodynamic process significantly influenced the distribution pattern of the HSI inside Laizhou Bay. HSIELS (Figure 8A) and HSI′ELS (Figure 8B) have similar patterns, which appear to have a high value in the center of the bay and a smaller value on the other two sides. Nevertheless, comparing the distribution of HSI and HSI′ in Laizhou Bay, it is notable in Figure 8 that HSIELS reaches its highest value (HSIELS > 0.67) in the eastern Laizhou Bay (near 120.1 E° and 37.6 N°). Eggs laid in this favorable environment have a large chance to survive (HSIegg > 0.8), and when this batch of eggs turns into larvae, they will be transported to shallower and warmer waters where they would feed on a lot of plankton and grow well (HSIlarvae > 0.7). Overall, this area can be thought of as the most suitable and critical habitat for anchovy in Laizhou Bay.
Figure 8 Habitat suitability index (HSI) for early life stages. (A) The spatial distribution of HSIELS. (B) In contrast, the HSI′ELS without drifting process.
Contrary to the HSIELS discussed above, HSI′ELS (drifting excluded) has different spatial characteristics. HSI′ELS presents a striped region of the highest values (HSI′ELS > 0.67), spanning from the western to the eastern side of the bay. We could be misled into thinking that this whole area in the striped shape is suitable for larval anchovy. However, the eggs released from the west part of the stripe are more likely to be transported outwards and die in cold and barren seas (HSIlarvae < 0.4). It is hardly a good habitat for anchovy.
The differences between HSIELS and HSI′ELS are plotted in Figure 9. The positive value (marked in yellow) means that the drifting process increases the suitability of this habitat—the current brings the eggs to a better habitat, while the negative value (marked in green) means that the drifting process reduces the HSI of the area. It can be seen that almost the whole western Laizhou Bay is negative, while some small areas in the northeast part of the domain are positive. In Section 3.3, we discussed the outward transportation of the eggs in western Laizhou Bay, making the eggs in this area hatch under unsuitable nursery grounds, which results in the large negative areas. Meanwhile, the eggs in the northeastern Laizhou Bay are transported inshore to a shallow, warm, and low-salinity area, which is highly suitable for larvae as presented in positive areas in Figure 9.
Figure 9 Spatial distribution of HSIELS minus HSI′ELS. Yellow indicates a positive value, and green indicates a negative value.
In this study, we simulated the drifting trajectories and spatial connectivity of anchovy eggs over their whole incubation period in Laizhou Bay with an IBM. Based on that, we evaluated the habitat suitability index for anchovy in their early life stages with/without the drifting process (HSIELS and HSI′ELS, respectively). The model indicates that a small patch at the east side of the bay mouth (near 120.1 E° and 37.6 N°) has the highest score, where the environment is suitable for eggs to survive, and the final incubation location of those eggs is in warm and shallow offshore areas, which is also highly suitable for larvae to grow. The environment quality in this particular area has a significant impact on the prospective population recruitment. It is an important location for the cultivation, artificial egg release, and other fishery management activities.
Compared with HSIELS, HSI′ELS showed an unrealistic high-value striped area in the bay mouth. The difference is controlled by the current field in Laizhou Bay, where the residual currents in the west mostly direct outwards, and in the east, they mostly direct inwards. As a result, the eggs released in the west are transported to areas where larvae cannot survive, while the eggs in the east are transported to suitable offshore areas. Currents can significantly affect the recruitment process, especially when they transport biological particles to an entirely different environment. The method used in this study can also be used for other species’ habitat assessment.
The raw data supporting the conclusions of this article will be made available by the corresponding author, without undue reservation, to any qualified researcher upon request.
XZ proposed the idea of using IBM to explore the connectivity between spawning ground and nursery ground and designed the outline of this article. XX wrote the paper and the model codes, including HSI and particle tracking module. XB offered the fishery data including anchovy eggs and larvae from in situ observation. QL helped to build the hydrodynamic model and performed data analysis and model verification. YX reviewed the manuscript and helped to rewrite the introduction part. All authors contributed to the article and approved the submitted version.
This work is funded by the Special Financial Fund of Spawning Ground Survey in the Bohai Sea and the Yellow Sea of the Ministry of Agriculture and Rural Affairs (125C0505) and the National Natural Science Foundation of China (grant no. 31802297).
The authors would like to express their gratitude to the editors and reviewers for their constructive suggestions and comments that greatly improved this article. We appreciate EditSpring (https://www.editsprings.cn) for the expert linguistic services provided.
The authors declare that the research was conducted in the absence of any commercial or financial relationships that could be construed as a potential conflict of interest.
All claims expressed in this article are solely those of the authors and do not necessarily represent those of their affiliated organizations, or those of the publisher, the editors and the reviewers. Any product that may be evaluated in this article, or claim that may be made by its manufacturer, is not guaranteed or endorsed by the publisher.
Anderson J. C., Gerbing D. W. (1988). Structural equation modeling in practice: A review and recommended two-step approach. Psychol. Bull. 103, 411–423. doi: 10.1037/0033-2909.103.3.411
Barnes T. K., Volety A. K., Chartier K., Mazzotti F. J., Pearlstine L. (2007). A habitat suitability index model for the Eastern oyster (Crassostrea virginica), a tool for restoration of the caloosahatchee estuary, Florida. J. Shellfish. Res. 26, 949–960. doi: 10.2983/0730-8000(2007)26[949:AHSIMF]2.0.CO;2
Bian X., Wan R., Shan X., Jin X. (2022). Preliminary analysis on recruitment variation and the exogenous driving factors to early life stages of small pelagic fishes in the laizhou bay. J. Fish. Sci. China 29, 446–468.
Black K., Oldman J., Hume T. (2005). Dynamics of a 3-dimensional, baroclinic, headland eddy. N. Z. J. Mar. Freshw. Res. 39, 91–120. doi: 10.1080/00288330.2005.9517294
Chen C., Liu H., Beardsley R. C. (2003). An unstructured grid, finite-volume, three-dimensional, primitive equations ocean model: Application to coastal ocean and estuaries. J. Atmosph. Ocean. Technol. 20, 159–186. doi: 10.1175/1520-0426(2003)020<0159:AUGFVT>2.0.CO;2
Cho Y., Lee W.-C., Hong S., Kim H.-C., Kim J. B. (2012). GIS-based suitable site selection using habitat suitability index for oyster farms in geoje-hansan bay, Korea. Ocean. Coast. Manage. 56, 10–16. doi: 10.1016/j.ocecoaman.2011.10.009
Coombs S. H., Boyra G., Rueda L. D., Uriarte A., Santos M., Conway D. V. P., et al. (2004). Buoyancy measurements and vertical distribution of eggs of sardine (Sardina pilchardus) and anchovy (Engraulis encrasicolus). Mar. Biol. 145, 959–970. doi: 10.1007/s00227-004-1389-4
Cushing D. H. (1990). ““Plankton production and year-class strength in fish populations: An update of the Match/Mismatch hypothesis,”,” in Advances in Marine Biology. Eds. Blaxter J. H. S., Southward A. J. (Academic Press), 249–293. doi: 10.1016/S0065-2881(08)60202-3
Cuttitta A., Torri M., Zarrad R., Zgozi S., Jarboui O., Quinci E. M., et al. (2018). Linking surface hydrodynamics to planktonic ecosystem: The case study of the ichthyoplanktonic assemblages in the central Mediterranean Sea. Hydrobiologia 821, 191–214. doi: 10.1007/s10750-017-3483-x
Egbert G. D., Erofeeva S. Y. (2002). Efficient inverse modeling of barotropic ocean tides. J. Atmosph. Ocean. Technol. 19, 183–204. doi: 10.1175/1520-0426(2002)019<0183:EIMOBO>2.0.CO;2
Falcini F., Corrado R., Torri M., Mangano M. C., Zarrad R., Di Cintio A., et al. (2020). Seascape connectivity of European anchovy in the central Mediterranean Sea revealed by weighted Lagrangian backtracking and bio-energetic modelling. Sci. Rep. 10, 18630. doi: 10.1038/s41598-020-75680-8
Gillenwater D., Granata T., Zika U. (2006). GIS-based modeling of spawning habitat suitability for walleye in the Sandusky river, Ohio, and implications for dam removal and river restoration. Ecol. Eng. 28, 311–323. doi: 10.1016/j.ecoleng.2006.08.003
Gómez S. E., Menni R. C., Naya J. G., Ramirez L. (2007). The physical–chemical habitat of the Buenos Aires pejerrey, odontesthes bonariensis (Teleostei, atherinopsidae), with a proposal of a water quality index. Environ. Biol. Fish. 78, 161–171. doi: 10.1007/s10641-006-9086-4
Hersbach H., Bell B., Berrisford P., Hirahara S., Horányi A., Muñoz-Sabater J., et al. (2020). The ERA5 global reanalysis. Q. J. R. Meteorol. Soc 146, 1999–2049. doi: 10.1002/qj.3803
Hjort J. (1914). Fluctuations in the great fisheries of northern Europe viewed in the light of biological research. Rapp. Proces-Verbaux. Reun. Cons. Int. Pour. L’Explorat. Mer. 20, 1–228.
Hjort J. (1926). Fluctuations in the year classes of important food fishes. ICES. J. Mar. Sci. 1, 5–38. doi: 10.1093/icesjms/1.1.5
Houde E. D., Hoyt R. (1987). Fish early life dynamics and recruitment variability. Am. Fish. Soc Symp. 2, 17–29.
Kim S., Kim D., Cho K.-H. (2015). Evaluation of habitat improvement using two-dimensional fish habitat modeling after the connectivity restoration in an isolated former channel. Ecol. Resilient. Infrastruct. 2, 137–146. doi: 10.17820/eri.2015.2.2.137
Lee D., Son S. H., Lee C.-I., Kang C.-K., Lee S. H. (2019). Spatio-temporal variability of the habitat suitability index for the todarodes pacificus (Japanese common squid) around south Korea. Remote Sens. 11 2720–2734. doi: 10.3390/rs11232720
Li Q., Zou Y., Zhang S., Wang Y., Zhao D., Song A. (2015). Changes in main fish stocks in bohai Sea around Shandong province. Fish. Sci. 34, 647–651. doi: 10.16378/j.cnki.1003-1111.2015.10.009
Lluch-Belda D., Lluch-Cota D. B., Hernandez-Vazquez S. H., Salinas-Zavala C. A., Schwartzlose R. A. (1991). Sardine and anchovy spawning as related to temperature and upwelling in the California current system. Calif. Coop. Ocean. Fish. Investig. Rep. U. S. 32, 105–111
Lou Q., Li Z., Zhang Y., Feng Y., Zhang X. (2022). Impact of typhoon lekima, (2019) on material transport in laizhou bay using Lagrangian coherent structures. J. Oceanol. Limnol. (40), 922–933. doi: 10.1007/s00343-021-0384-7
Ohshimo S., Hamatsu T. (1996). Vertical distribution and acoustic estimation of biomass of walleye Pollock theragra chalcogramma, and anchovy engraulis japonicus in the pacific coast of Eastern Hokkaido [Japan]. Bull. Hokkaido. Natl. Fish. Res. Inst. Jpn. 60, 225–237.
Oozeki Y., Takasuka A., Kubota H., Barange M. (2007). Characterizing spawning habitats of Japanese sardine (Sardinops melanostictus) Japanese anchovy (Engraulis japonicus) and pacific round herring (Etrumeus teres) in the northwestern pacific. Calif. Coop. Fish. Investig. CalCOFI. Rep. 48, 191–203.
Ottersen G., Bogstad B., Yaragina N. A., Stige L. C., Vikebø F. B., Dalpadado P. (2014). A review of early life history dynamics of barents Sea cod (Gadus morhua). ICES. J. Mar. Sci. 71, 2064–2087. doi: 10.1093/icesjms/fsu037
Patti B., Torri M., Cuttitta A. (2020). General surface circulation controls the interannual fluctuations of anchovy stock biomass in the central Mediterranean Sea. Sci. Rep. 10, 1554. doi: 10.1038/s41598-020-58028-0
Patti B., Zarrad R., Jarboui O., Cuttitta A., Basilone G., Aronica S., et al. (2018). Anchovy (Engraulis encrasicolus) early life stages in the central Mediterranean Sea: Connectivity issues emerging among adjacent Sub-areas across the strait of Sicily. Hydrobiologia 821, 25–40. doi: 10.1007/s10750-017-3253-9
Pinckney J. L., Paerl H. W., Tester P., Richardson T. L. (2001). The role of nutrient loading and eutrophication in estuarine ecology. Environ. Health Perspect. 109, 699–706. doi: 10.1289/ehp.01109s5699
Rijnsdorp A. D., Peck M. A., Engelhard G. H., Möllmann C., Pinnegar J. K. (2009). Resolving the effect of climate change on fish populations. ICES. J. Mar. Sci. 66, 1570–1583. doi: 10.1093/icesjms/fsp056
Russo S., Torri M., Patti B., Musco M., Masullo T., Di Natale M. V., et al. (2022). Environmental conditions along tuna larval dispersion: Insights on the spawning habitat and impact on their development stages. Water 14, 1568. doi: 10.3390/w14101568
Takahashi M., Watanabe Y. (2004). Growth rate-dependent recruitment of Japanese anchovy engraulis japonicus in the kuroshio-oyashio transitional waters. Mar. Ecol. Prog. Ser. 266, 227–238. doi: 10.3354/meps266227
Takasuka A., Aoki I. (2006). Environmental determinants of growth rates for larval Japanese anchovy engraulis japonicus in different waters. Fish. Oceanogr. 15, 139–149. doi: 10.1111/j.1365-2419.2005.00385.x
Theuerkauf S. J., Lipcius R. N. (2016). Quantitative validation of a habitat suitability index for oyster restoration. Front. Mar. Sci. 3. doi: 10.3389/fmars.2016.00064
Torri M., Corrado R., Falcini F., Cuttitta A., Palatella L., Lacorata G., et al. (2018). Planktonic stages of small pelagic fishes (Sardinella aurita and engraulis encrasicolus) in the central Mediterranean Sea: The key role of physical forcings and implications for fisheries management. Prog. Oceanogr. 162, 25–39. doi: 10.1016/j.pocean.2018.02.009
U.S.Fish and WildLife Services (1981). Standards for the development of habitat suitability index models Technical Report 103 ESM, USDI Fish and Wildlife Service, Division of Ecological Services, Washington, DC.
Van der Molen J., Rogers S. I., Ellis J. R., Fox C. J., McCloghrie P. (2007). Dispersal patterns of the eggs and larvae of spring-spawning fish in the Irish Sea, UK. J. Sea. Res. 58, 313–330. doi: 10.1016/j.seares.2007.07.003
Wan R. (2008). Spawning ecology of the anchovy engraulis japonicus in the spawning ground of the southern Shandong Peninsula II. spawning habits and embryonic developmental characteristics of the anchovy. Acta Zool. Sin., (54), 988–997.
Yi Y., Cheng X., Yang Z., Wieprecht S., Zhang S., Wu Y. (2017). Evaluating the ecological influence of hydraulic projects: A review of aquatic habitat suitability models. Renew. Sustain. Energy Rev. 68, 748–762. doi: 10.1016/j.rser.2016.09.138
Yu W., Chen X. (2021). Habitat suitability response to Sea-level height changes: Implications for ommastrephid squid conservation and management. Aquac. Fish. 6, 309–320. doi: 10.1016/j.aaf.2020.06.001
Keywords: habitat suitability index, fish early life stages, hydrodynamics, individual-based model, anchovy, coastal habitats
Citation: Xiang X, Zhang X, Bian X, Lou Q and Xiong Y (2022) HSI model for early life stages of anchovy considering transport processes in Laizhou Bay. Front. Mar. Sci. 9:946114. doi: 10.3389/fmars.2022.946114
Received: 17 May 2022; Accepted: 06 July 2022;
Published: 02 August 2022.
Edited by:
Bernardo Patti, Institute for the Study of Anthropogenic Impacts and Sustainability in Marine Environment, National Research Council (CNR), ItalyReviewed by:
Barbara Zorica, Institute of Oceanography and Fisheries (IZOR), CroatiaCopyright © 2022 Xiang, Zhang, Bian, Lou and Xiong. This is an open-access article distributed under the terms of the Creative Commons Attribution License (CC BY). The use, distribution or reproduction in other forums is permitted, provided the original author(s) and the copyright owner(s) are credited and that the original publication in this journal is cited, in accordance with accepted academic practice. No use, distribution or reproduction is permitted which does not comply with these terms.
*Correspondence: Xueqing Zhang, enhxQG91Yy5lZHUuY24=
Disclaimer: All claims expressed in this article are solely those of the authors and do not necessarily represent those of their affiliated organizations, or those of the publisher, the editors and the reviewers. Any product that may be evaluated in this article or claim that may be made by its manufacturer is not guaranteed or endorsed by the publisher.
Research integrity at Frontiers
Learn more about the work of our research integrity team to safeguard the quality of each article we publish.