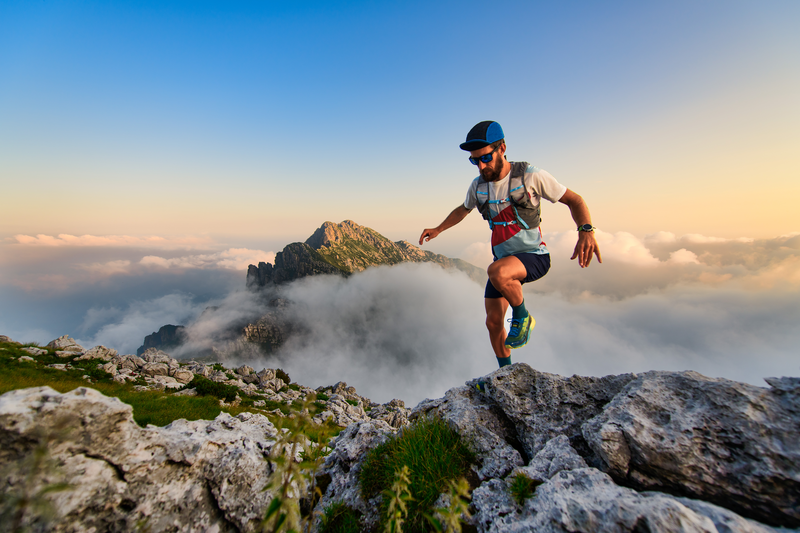
94% of researchers rate our articles as excellent or good
Learn more about the work of our research integrity team to safeguard the quality of each article we publish.
Find out more
ORIGINAL RESEARCH article
Front. Mar. Sci. , 12 August 2022
Sec. Aquatic Physiology
Volume 9 - 2022 | https://doi.org/10.3389/fmars.2022.945417
This article is part of the Research Topic Nutritional Physiology of Aquacultured Species View all 12 articles
A correction has been applied to this article in:
Corrigendum: Effect of dietary soybean meal on growth performance, apparent digestibility, intestinal digestive enzyme activity and muscle growth-related gene expression of Litopenaeus vannamei
Soybean meal is one of the major components of aquatic animal diets, whereas little information is available about the evaluation of soybean meal growth suppression mode of action. A 42-day feeding trial was performed to assess the effects of dietary soybean meal on growth performance, apparent digestibility, intestinal digestive enzyme activity, and muscle growth–related gene expression of Litopenaeus vannamei. A total of 600 shrimp were randomly distributed into 20 tanks with 30 shrimp per tank and four tanks per group. The soybean meal was added to the diets at the rate of 20% (T20), 28% (T28), 35% (T35), 42% (T42), and 50% (T50), respectively. Shrimp were fed with apparent satiation three times daily. Results indicated that the final body weight, weight gain rate, specific growth rate, feed intake, intestinesomatic index, dressed weight percentage, and the apparent digestibility coefficients of dry matter, crude protein, crude lipid, and ash were linearly decreased (p < 0.05), but feed coefficient was linearly increased (p < 0.05) as dietary soybean meal increased from 20% to 50%. The intestinal trypsin and amylase activities were decreased (p < 0.05) as dietary soybean meal increased from 20% to 50%, and reached significance at the level of 35%, 42%, and 50%. Shrimp fed with T20 had higher (p < 0.05) intestinal lipase activity than those fed with other diets. The mRNA relative expression of growth hormone, myogenic regulatory factor 5, and target of rapamycin was downregulated (p < 0.05) as dietary soybean meal increased from 20% to 50%. To conclude, dietary soybean meal exceeded 28% significantly inhibited growth performance of L. vannamei, mainly due to the negative impact of soybean meal on digestion and feed utilization and also the inhibition on the muscle growth and related gene expressions.
Fishmeal is one of the most important feed protein sources in aquatic feed. It has been reported that more than two-thirds of fishmeal is used as aquatic feed in the world and about 60% in China (Mai et al., 2021). As the global population increase, the demand of people for aquatic products consumption is unabated. However, the production of fishmeal is maintained at around 5 million tons in recent years, which could no longer continue to increase in the further. Combined with the increasing need of fishmeal in the development of aquaculture, professionals have spared no effort to find alternatives to fishmeal and solutions to improve the utilization of non-fishmeal protein. Over the last decade, the plant protein such as soybean meal has been widely used to replace fishmeal in aquafeed and exhibits as a promising alternative in various species (Jiang et al., 2015a; Chakraborty et al., 2019; Shukla et al., 2019; Bae et al., 2020; Bruce et al., 2022; Wu et al., 2021; Zhao et al., 2021; Ding et al., 2022). Even so, soybean meal is considered to have deficiencies including the presence of anti-nutritional factors (e.g., soybean agglutinin, glycinin, and β-conglycinin), imbalance of essential amino acids, and absence of certain fishmeal components (e.g., taurine, hydroxyproline, and vitamin D3) (Mai et al., 2021), which may result in poor palatability and digestibility of diets and lead to the reduced growth performance of animals.
Litopenaeus vannamei belongs to crustacean species that is being widely cultured and welcomed in China. In 2020, China contributed 1.19 million tons of the farmed L. vannamei, which account for 70% of shrimp production (Fishery Administration of Ministry of Agriculture and Rural Affairs, 2021). Although replacing fishmeal with soybean meal in L. vannamei diet has been well reported, the suitable substitution ratio is inconsistent among studies. For comprehensive assessment from growth performance and nutrient utilization of L. vannamei, Amaya et al. (2007) indicated that fishmeal could be totally replaced by soybean meal (dietary concentration at 39.5%); Lim and Dominy (1990) suggested that the suitable substitution ratio of fishmeal (dietary concentration at 11.3%) by soybean meal is 60%; and the suitable substitution ratio were proposed to be 28% (dietary fishmeal at 38.2%) (Xu et al., 2021) and 20% (dietary fishmeal at 30.0%) (Yang et al., 2015). On the other hand, high dose of dietary soybean meal caused inhibition of growth and digestion of L. vannamei, whereas the reason and related mechanism are still not fully understood. Previous studies documented that the inhibition effect of dietary soybean meal on growth of aquatic animals is mainly attributed to endogenous anti-nutritional factors (Elumalai et al., 2019; Zhao, 2021; Zhou, 2021). A recent study screened the growth and development related functional genes (e.g., myogenic regulatory factors Myf5 and MyoG) in the muscle of L. vannamei by transcriptome sequencing, but the information regarding the effects of dietary soybean meal on the muscle growth–related gene expressions is rare. Therefore, this study is conducted to evaluate the effects of dietary soybean meal on growth performance, apparent digestibility, intestinal digestive enzyme activity, and muscle growth–related gene expression of L. vannamei.
The ingredients and proximate compositions of experimental diets were shown in Table 1. The soybean meal (crude protein 46%) was added to the diets at the rate of 20% (T20), 28% (T28), 35% (T35), 42% (T42), and 50% (T50), to replace fishmeal (crude protein 66%) at the rate of 0%, 20%, 40%, 60%, and 80%, respectively. All ingredients were ground (AHZC1265 Hammer Mill, Buhler Machinery Co., Ltd., Guanghzou, China) to pass through a 320-μm sieve, mixed (AHML2000 Mixer, Buhler Machinery Co., Ltd., Changzhou, China) thoroughly and then extruded (SLX-80 Twin-screw Extruder, South China University of Technology Machinery Factory, Guangzhou, China) into 2-mm pellets, dried (HMO-205 Oven Dryer, Haiming Electronic Technology Co., Ltd., Dongguan, China) at 55°C for 12 h, and stored at −20°C until use.
The protocol (No. GAAS20210501) and all procedures performed in this study were approved by the Institutional Animal Care and Use Committee of Institute of Animal Science, Guangdong Academy of Agricultural Sciences (Guangdong, China). A 42-day feeding trial was conducted by randomly distributing 600 L. vannamei (initial body weight, 5.8 ± 0.15 g) into 20 tanks (30 shrimp per tank) with four tanks being assigned to each diet. Shrimp were hand-fed with apparent satiation (approximately 4% of body weight per day) three times daily (08:00, 14:00, and 20:00). Uneaten feed was collected in 1 h after each meal, analyzed for dry matter, and subtracted from feed offered (dry matter basis) to calculate feed intake (FI) as described by Wang et al. (2019). During the feeding trial, water was continuously aerated and filtered through sand filter system at a rate of 1.6 L/min. Water temperature ranged from 25°C to 27°C, dissolved oxygen was above 5.0 mg/L, pH ranged from 7.6 to 8.0, salinity was 5‰–6‰, ammonia nitrogen and nitrite were below 0.01 mg/L, and the photoperiod regime was 12-h light and 12-h dark.
Feces were continuously collected from each tank daily at the last 14 days of the feeding trial according to the siphon method described by Gumus (2011). The intact feces were picked out with a tweezers and stored at −20°C. Fecal samples collected from each tank were pooled and dried at 105°C to a constant weight to determine the apparent digestibility coefficients (ADCs) of dry matter, crude protein, crude lipid, and ash. Indigestible acid insoluble ash (AIA) in diet ingested and in feces samples was used as marker to estimate nutrient digestibility according to Peng et al. (2021).
At the end of the feeding trial, all shrimp were fasted and anesthetized with MS-222 (40 mg/L; Sigma, USA) prior to sampling. Shrimp per tank were counted and weighted to analyze for survival rate (SR), final body weight (FBW), weight gain rate (WGR), specific growth rate (SGR), and feed coefficient (FC). Six shrimp per tank were randomly selected, determined individual body and muscle weight, and slaughtered to analyze the hepatosomatic index (HSI), intestinesomatic index (ISI), and dressed weight percentage (DWP).
Intestines of three shrimp in each tank were sampled to determine trypsin (Ultraviolet colorimetry), lipase (colorimetry), and amylase (colorimetry) activities using commercial kits (Nanjing Jiancheng Bioengineering Institute, Nanjing, China) following the corresponding instructions of the manufacturer.
The dorsal muscle of three shrimp in each tank was collected and immediately stored at −80°C for subsequent RNA extraction and real-time qPCR analysis of muscle growth–related gene expression.
The nutrient compositions of experimental diets including moisture, crude protein, crude lipid, and ash were measured following the AOAC methods (AOAC, 1999). The contents of lysine and methionine were analyzed by chromatography using an automated amino acid analyzer (L8900, Hitachi, Japan) with a lithium high-performance column.
Total RNA of muscle samples was isolated using the RNAiso Plus kit (TaKaRa Biotechnology Co., Ltd.). The real-time qPCR analysis method was the same as described by Xin (2016). The primer sequences of the growth hormone (GH), myogenic regulatory factors (Myf5 and MyoG), and target of rapamycin (TOR) were listed in Table 2. The 2−ΔΔCT method was used to analyze the gene expression levels (Livak and Schmittgen, 2001).
Data were summarized and averaged for each tank. Growth performance parameters were calculated as follows:
SR (%) = 100 × (final number of shrimp/initial number of shrimp)
WGR (%) = 100 × [final body weight (g) − initial body weight (g)]/initial body weight (g)
SGR (%/d) = 100 × [ln final body weight (g) − ln initial body weight (g)]/days
FI (g/shrimp) = feed intake (g)/[(final number of shrimp + initial number of shrimp)/2]
FC = total feed intake (g)/[final body weight (g) − initial body weight (g)]
HSI (%) = 100 × hepatopancreas weight (g)/body weight (g)
ISI (%) = 100 × intestinal weight (g)/body weight (g)
DWP (%) = 100 × muscle weight (g)/body weight (g)
ADCs (%) = 100 × [1 − (a × b)/(c × d)]
where a and c are the AIA concentration in diet ingested and feces, respectively; b and d are the nutrient concentration in feces and diets ingested, respectively. a and d were calculated as difference between the diet offered and orts.
All data were subjected to normality test and homogeneity of variance by using Shapiro–Wilk and Levene’s test for equal variance, respectively. If the data conform to the normal distribution, one-way ANOVA by using SPSS 17.0 analysis software (SPSS(Statistical Packages for Social Sciences, 2007) for Windows followed by the Tukey test with tank as statistical unit and treatment as fixed effect. Polynomial contrasts were used to determine linear and/or quadratic responses to the dietary soybean meal levels. Differences were regarded as significance when p < 0.05.
All shrimp had similar (p > 0.05) SR and HSI regardless of the treatments (Table 3). The FBW, WGR, SGR, FI, ISI, and DWP were linearly decreased (p < 0.05), but FC was linearly increased (p < 0.05) as dietary soybean meal increased from 20% to 50%.
The ADC of dry matter, crude protein, crude lipid, and ash was linearly decreased (p < 0.001) as dietary soybean meal increased from 20% to 50% (Table 4).
Table 4 Apparent digestibility coefficients (ADCs) of nutrients by L. vannamei fed with experimental diets.
The intestinal trypsin and amylase activities were decreased (p < 0.05) as dietary soybean meal increased from 20% to 50% and reached significance at the level of 35%, 42%, and 50% (Figure 1). Shrimp fed with T20 had higher (p < 0.05) intestinal lipase activity than those fed with other diets.
Figure 1 Intestinal digestive enzyme activities of L. vannamei fed with experimental diets. Different letters above the bars denote significant differences among diets (p < 0.05). T20–T50, diets containing 20%–50% of soybean meal..
All shrimp has similar (p > 0.05) mRNA relative expression of MyoG among diets (Figure 2). The mRNA relative expression of GH, Myf5, and TOR was downregulated (p < 0.05) as dietary soybean meal increased from 20% to 50%. Compared with T20, T28, T35, T42, and T50 had lower (p < 0.05) mRNA relative expression of GH and TOR, and T35, T42, and T50 had lower (p < 0.05) mRNA relative expression of Myf5.
Figure 2 Muscle growth–related gene expression of L. vannamei fed with experimental diets. GH, growth hormone; Myf5 and MyoG, myogenic regulatory factors; TOR, target of rapamycin. Different letters above the bars denote significant differences among diets (p < 0.05). T20–T50, diets containing 20%–50% of soybean meal.
The similar SR among diets in this study indicated that dietary soybean meal at the rate of 20% to 50% did not affect survival of L. vannamei. However, the decreased SGR and FI suggested that the increased dietary soybean meal depressed feed palatability and growth performance of shrimp. This is consistent with the report by Yun et al. (2017) that the replacement ratio of dietary fishmeal with soybean meal at 33% reduced growth of L. vannamei. Xu et al. (2021) also reported that dietary fishmeal replaced by soybean meal at 56% reduced FI of L. vannamei. Similar results were also observed in various species, such as European catfish (Silurus glanis) (Kumar et al., 2017), Tiger puffer (Takifugu rubripes) (Lim et al., 2011), sharpsnout seabream (Diplodus puntazzo) (Hernandez et al., 2007), red snapper (Lutjanus campechanus) (Davis et al., 2005), Pseudobagrus ussuriensis (Wang et al., 2016), stellate sturgeon (Acipenser stellatus) (Emdadi et al., 2013), Jian carp (Cyprinus carpio var. Jian) (Jiang et al., 2015b), rainbow trout (Oncorhynchus mykiss) (Harlioglu, 2011), Channa argus (Zhang et al., 2020), Epinephelus Fuscoguttatus (Liu et al., 2018), bullfrog (Rana catesbeiana) (Ding et al., 2019; Wang et al., 2020), and Chinese mitten carb (Eriocheir sinensis) (Liu et al., 2021). In this study, the increased FC with increasing dietary soybean meal indicated that supplementation of soybean meal decreased feed utilization; this may account for the depressed growth performance of shrimp. It has been reported that FI is generally regulated by both digestibility and palatability of diet (Peng et al., 2016). The decreased FI as dietary soybean meal increased may partly attribute to decreased apparent digestibility of nutrients as observed in this study. In addition, soybean meal contains several anti-nutritional factors, e.g., soybean agglutinin, glycinin, and β-conglycinin, which have been well reported to reduce the palatability of diet and thereby decrease FI and growth performance of aquatic animals (Feng, 2006; Elumalai et al., 2019; Duan, 2019; Zhao, 2021; Zhou, 2021). Moreover, the decreased ISI and DWP as dietary soybean meal increased suggested that the inclusion of soybean meal in diet inhibited growth and development of the intestine and muscle of L. vannamei. This inhibition effect on the intestine may be due to the injury directly caused by soybean meal or indirectly induced by anti-nutritional factors. Zhang et al. (2020) documented that replacing 30% to 60% fishmeal with soybean meal induced intestinal injury of Channa argus. Ding et al. (2019) indicated that replacing 50% to 100% fishmeal with soybean meal damaged intestinal villus structure and caused enteritis of bullfrog (Rana catesbeiana). Zhou (2021) reported that dietary glycinin and β-conglycinin induced intestinal damage and inflammation and thus inhibited growth and development of intestine in Songpu mirror carp by upregulating mRNA levels of apoptosis-related genes. Similar result was also reported by Duan (2019), in which the inclusion of β-conglycinin in grass carp diet caused enterocytes apoptosis and significantly reduced the ISI of fish. Similarly, Lu et al. (2018) also indicated that totally replacement of fishmeal by soybean meal in crayfish (Cherax quadricarinatus) diet significantly decreased crude protein content and depressed muscle growth. Xu et al. (2021) reported that replacing 28% to 70% fishmeal with soybean meal reduced the muscle nutrient compositions of L. vannamei.
Digestibility of nutrients as reflected by the ADC values of crude protein, crude lipid, and ash usually indicates digestion and utilization of diets. These are closely related to the corresponding digestive enzyme activities in the intestine, because intestinal digestive enzymes, e.g., trypsin, lipase, and amylase, are commonly used as critical indicators to evaluate the changes in diets (Santigosa et al., 2008). In this study, the decreased ADCs of crude protein, crude lipid, and ash as dietary soybean meal increased suggest that the supplementation of soybean meal in shrimp diets decreased the digestion and utilization of diets. This is most likely due to the decreased intestinal digestive enzyme activities of trypsin, lipase, and amylase as observed in this study. The inhibition effect of intestinal digestive enzyme activities by replacing fishmeal with soybean meal in aquatic feed is a common phenomenon that has been well reported in previous studies. For instance, replacing 57% and 100% of fishmeal by soybean meal in Chinese mitten crab (Eriocheir sinensis) diets significantly reduced the intestinal digestive enzyme activities of trypsin, lipase, and amylase (Liu et al., 2021). Similar result was also reported by Zhang et al. (2015) that replacing 12% to 48% fishmeal with soybean meal significantly decreased the intestinal lipase and amylase activities of rice filed eel (Monopterus albus). Furthermore, Hernandez et al. (2007) documented that the inclusion of 60% soybean meal (approximately replacing 68% fishmeal) in sharpsnout seabream (Diplodus puntazzo) diet significantly reduced the ADCs of dry matter and crude protein. Yang et al. (2015) indicated that replacing 40% and 60% of fishmeal by soybean meal significantly decreased ADCs of dry matter and crude protein of L. vannamei. Tibaldi et al. (2006) also documented that substitution of 50% fishmeal by soybean meal significantly decreased ADCs of dry matter, crude protein, crude lipid, and ash. Although the reason why supplementation of soybean meal inhibited the intestinal digestive enzyme activities is not fully understood, this may be attributed to the increasing levels of the anti-nutritional factors (such as soybean agglutinin, glycinin, and β-conglycinin) with the increase of soybean meal in diets (Ding et al., 2019; Liu et al., 2021). Yue et al. (2012) also reported that anti-nutritional factors in soybean meal reduced the digestive enzyme activities in the gut of L. vannamei.
The growth and development of muscle that involves hyperplasia and hypertrophy is generally controlled by diverse genetic factors, such as growth hormone (GH), myogenic regulatory factors (Myf5 and MyoG), and target of rapamycin (TOR) (Xin, 2016; Asaduzzaman et al., 2017). Therefore, the effects of formulated diets on muscle growth of aquatic animals were mainly investigated from the aspects of these muscle growth–related genes (Alami-Durante et al., 2018; Wei et al., 2020a; Wei et al., 2020b; Yang et al., 2021). GH stimulates the growth of muscle through inducing proliferation of myogenic cells and muscle hyperplasia and hypertrophy (Asaduzzaman et al., 2017). Myogenic regulatory factors, e.g., Myf5 and MyoG, are the critical muscle development– and growth–related transcription factors (Funkenstein et al., 2007). TOR, an important regulatory factor in cellular central control system, plays a key role in cell growth and proliferation (Liang et al., 2020). Therefore, all of these genes act as a positive regulator of muscle growth that promote hyperplastic and hypertrophic muscular growth. In this study, the downregulated mRNA levels of GH, Myf5, and TOR as dietary soybean meal increased suggested that supplementation of soybean meal in shrimp diets decreased the growth and development of muscle. This may also account for the concomitantly decreased DWP as observed in this study. To our best knowledge, this study is the first to assess the effects of dietary soybean meal on these muscle-related gene expressions of L. vannamei. This is similar with the observation by Hu et al. (2018) that replacing 40% and 50% of fishmeal by fermented soybean meal in Nibea albiflora diets significantly decreased mRNA levels of muscle growth–related gene (insulin-like growth factor І). Ulloa et al. (2013) also reported that the dietary inclusion of plant proteins reduced the muscle growth–related gene expressions in the male zebrafish (Danio rerio). Despite the reason why the dietary inclusion of soybean meal downregulated the gene expression of muscle growth–related genes is not clear, this is most likely ascribed to the adverse effects of anti-nutritional factors exist in soybean meal on the proliferation of intestinal epithelial cells and thereby decreased digestion and absorption of nutrients in shrimp diet to provide necessary energy serving as basis contents for muscle growth. Numbers of studies documented that anti-nutritional factors extracted from soybean meal injured intestinal epithelial cells of animals (Feng, 2006; Guo, 2006; Xu, 2009; Duan, 2019; Peng, 2020; Zhou, 2021). These studies combined with the observation that supplementation of soybean meal inhibited nutrient digestibility of shrimp in this study may be the potential mechanisms to support this hypothesis. Further study is still needed to confirm this.
Dietary soybean meal that exceeded 28% significantly inhibited growth performance of L. vannamei. This growth inhibition effect may partly attribute to the negative impact of anti-nutritional factors that exist in soybean meal on the digestion and utilization of nutrients in shrimp diets and also the inhibition on the muscle growth and development as reflected by the decreased DWP and muscle growth–related gene expressions. This study provides a caution for the application of soybean meal in aquaculture, and further study is needed to clarify mechanisms regarding the inhibition effect of soybean meal on muscle growth of shrimp.
The original contributions presented in the study are included in the article/supplementary material. Further inquiries can be directed to the corresponding author.
The animal study was reviewed and approved by the Animal Care and Use Committee of Guangdong Academy of Agricultural Sciences, Guangzhou, China.
KP and WH conceived and designed the experiments. KP, XC, HJL, JZ, YC, CL, and HL performed the experiments. KP analyzed the data and wrote the paper. All authors contributed to the article and approved the submitted version.
This study was funded by Innovation Fund project of Guangdong Academy of Agricultural Sciences (202137 and 202138), Special Fund for Scientific Innovation Strategy-Construction of High-Level Academy of Agriculture Science (R2021PY-QY001), and Independent Research and Development Projects of Maoming Laboratory (2021TDQD004 and 2021ZZ007).
We acknowledge those who donated the experimental animals and those who helped support this study at the Institute of Animal Science, Guangdong Academy of Agricultural Sciences.
Author HL is employed by Guangdong Jinyang Biotechnology Co. Ltd.
The remaining authors declare that the research was conducted in the absence of any commercial or financial relationships that could be construed as a potential conflict of interest.
The reviewer (HZ) declared a shared affiliation with the author (CL) to the handling editor at the time of review.
All claims expressed in this article are solely those of the authors and do not necessarily represent those of their affiliated organizations, or those of the publisher, the editors and the reviewers. Any product that may be evaluated in this article, or claim that may be made by its manufacturer, is not guaranteed or endorsed by the publisher.
Alami-Durante H., Bazin D., Cluzeaud M., Fontagne-Dicharry S., Kaushik S., Geurden I. (2018). Effect of dietary methionine level on muscle growth mechanisms in juvenile rainbow trout (Oncorhynchus mykiss). Aquaculture 483, 273–285. doi: 10.1016/j.aquaculture.2017.10.030
Amaya E., Davis D. A., Rouse D. B. (2007). Replacement of fish meal in practical diets for the pacific white shrimp (Litopenaeus vannamei) reared under pond conditions. Aquaculture 262, 393–401. doi: 10.1016/j.aquaculture.2006.11.015
AOAC (1999). Official methods of analysis of the association of official agricultural chemists, 16th ed (Gaithersburg: AOAC International).
Asaduzzaman M., Ikeda D., Abol-Munafi A. B., Bulbul M., Ali M. E., Kinoshita S., et al. (2017). Dietary supplementation of inosine monophosphate promotes cellular growth of muscle and upregulates growth-related gene expression in Nile tilapia oreochromis niloticus. Aquaculture 468, 297–306. doi: 10.1016/j.aquaculture.2016.10.033
Bae J., Hamidoghli A., Djaballah M. S., Maamri S., Hamdi A., Souffi I., et al. (2020). Effects of three different dietary plant protein sources as fishmeal replacers in juvenile whiteleg shrimp, litopenaeus vannamei. Fish. Aquat. Sci. 23 (1), 2. doi: 10.1186/s41240-020-0148-x
Bruce T. J., Oliver L. P., Ma J., Small B. C., Hardy R. W., Brown M. L., et al. (2021). An initial evaluation of fishmeal replacement with soy protein sources on growth and immune responses of burbot (Lota lota maculosa). Aquaculture 545, 737157. doi: 10.1016/j.aquaculture.2021.737157
Chakraborty P., Mallik A., Sarang N., Lingam S. S. (2019). A review on alternative plant protein sources available for future sustainable aqua feed production. Int. J. Chem. Stud. 7 (3), 1399–1404.
Davis D. A., Miller C. L., Phelps R. P. (2005). Replacement of fish meal with soybean meal in the production diets of juvenile red snapper, lutjanus campechanus. J. Word. Aquacult. Soc 36 (1), 114–119. doi: 10.1111/j.1749-7345.2005.tb00137.x
Ding L., Wang L., Lu K., Song K., Zhang C. (2019). Effects of replacement of fish meal with soybean meal on growth performance, digestive enzyme activity and intestinal health of rana catesbeiana. Fresh. Fish. 49 (2), 69–75. doi: 10.13721/j.cnki.dsyy.2019.04.011
Ding Z., Zhou D., Zheng J., Chen X., Kong Y., Qi C., et al. (2022). Replacing fishmeal with soybean meal affects survival, antioxidant capacity, intestinal microbiota, and mRNA expression of TOR and S6K1 in macrobrachium rosenbergii. Chin. J. Oceanol. Limn. 40, 805–817. doi: 10.1007/s00343-021-0494-2
Duan X. (2019). Effects of β-conglycinin on apoptosis in grass carp enterocytes and the involved mechanism (Chengdu, China: Sichuan Agricultural University).
Elumalai P., Rubeena A. S., Arockiaraj J., Wongpanya R., Cammarata M., Ringo E., et al. (2019). The role of lectins in finfish: a review. Rev. Fish. Sci. Aquacult. 27 (2), 152–169. doi: 10.1080/23308249.2018.1520191
Emdadi B., Sajadi M. M., Yazdani M. A., Shakoorian M. (2013). Influence of replacing fish meal with soybean meal on growth rate, feed conversion ratio and chemical composition of carcass, fillet and liver in juvenile stellate sturgeon (Acipenser stellatus). Iran. Sci. Fish. J. 22 (2), 23–34. doi: 10.22092/ISFJ.2017.110116
Feng L. (2006). Effects of soybean agglutinin on the proliferation, differentiation and functions of carp intestinal epithelial cells in primary culture (Chengdu, China: Sichuan Agricultural University).
Fishery Administration of Ministry of Agriculture and Rural Affairs (2021). China Fishery statistical yearbook (Beijing, China: China Agriculture Press).
Funkenstein B., Skopal T., Rapoport B., Rebhan Y., Du S. J., Radaelli G. (2007). Characterization and functional analysis of the 5′-flanking region of myosin light chain-2 gene expressed in fast skeletal muscle of the gilthead sea bream (Sparus aurata). Comp. Biochem. Phys. D. 2 (3), 187–199. doi: 10.1016/j.cbd.2007.04.001
Gumus E. (2011). Effect of replacement of fishmeal with sand smelt (Atherina boyeri) meal on growth, feed utilization and body composition of mirror carp fry (Cyprinus carpio). Kafkas. Univ. Vet. Fak. Derg. 17 (3), 363–369. doi: 10.1038/laban0111-9a
Guo L. (2006). Effects of soybean β-conglycinin extract on the proliferation and function of carp intestinal-epithelial cells in primary culture (Chengdu, China: Sichuan Agricultural University).
Harlioglu A. G. (2011). The influence of replacing fish meal partially in diet with soybean meal and full-fat soya on growth and body composition of rainbow trout (Oncorhynchus mykiss). Pakistan J. Zool. 43 (1), 175–182. doi: 10.1515/MAMM.2010.076
Hernandez M. D., Martinez F. J., Jover M., Garcia B. G. (2007). Effects of partial replacement of fish meal by soybean meal in sharpsnout seabream (Diplodus puntazzo) diet. Aquaculture 263, 159–167. doi: 10.1016/j.aquaculture.2006.07.040
Hu S., Wang L., Lou B., Zeng W., Tan P., Zhan W., et al. (2018). Effects of replacing fish meal with fermented soybean meal on amino acids in muscle and expression of IGF-I in liver and muscle of juvenile nibea albiflora. J. Zhejiang. Ocean. Univ. 37 (3), 196–202.
Jiang S., Yang Q., Huang J., Lin H., Li X., Jiang S. (2015a). Impacts of diets with different protein levels on the growth and mRNA expression of growth-related genes in penaeus monodon families. Mar. Fish. 37 (3), 244–252. doi: 10.7668/hbnxb.2015.S1.020
Jiang J., Zhou X., Yin L., Feng L., Jiang W., Liu Y., et al. (2015b). The influence of replacing fish meal with soybean meal on growth, body composition and digestive ability of jian carp in practical extruded diet. Acta Agric. Bor. Sin. 30, 107–111. doi: 10.13233/j.cnki.mar.fish.2015.03.006
Kumar S., Sandor Zs J., Nagy Z., Fazekas G., Havasi M., Sinha A. K., et al. (2017). Potential of processed animal protein versus soybean meal to replace fish meal in practical diets for European catfish (Silurus glanis): growth response and liver gene expression. Aquacult. Nutr. 23, 1179–1189. doi: 10.1111/anu.12487
Liang Q., Ou M., Li Z., Ren Y., Wei W., Qiao X., et al. (2020). Functional analysis target of rapamycin (TOR) on the penaeus vannamei in response to acute low temperature stress. Fish. Shell. Immunol. 96, 53–61. doi: 10.1016/j.fsi.2019.11.070
Lim C., Dominy W. (1990). Evaluation of soybean meal as a replacement for marine animal protein in diets for shrimp (Penaeus vannamei). Aquaculture 87, 53–63. doi: 10.1016/0044-8486(90)90210-E
Lim S., Kim S., Ko G., Song J., Oh D., Kim J., et al. (2011). Fish meal replacement by soybean meal in diets for tiger puffer, takifugu rubripes. Aquaculture 313, 165–170. doi: 10.1016/j.aquaculture.2011.01.007
Liu Y., Meng L., Zhang J. (2018). Effect of partial fish meal replacement by soybean meal on the growth performance, nutritional composition and blood biochemical indices of juvenile epinephelus fuscoguttatus. China Feed. 4, 60–64. doi: 10.15906/j.cnki.cn11-2975/s.20180412
Liu Q., Wen B., Man D., Jiang Y., Li X., Zuo R. (2021). Substitution of fish meal with soybean meal for juvenile Chinese mitten crab (Eriocheir sinensis) reared at three salinities: Effects on survival, growth, antioxidant capacity and body composition. Aquacult. Res. 52, 3723–3735. doi: 10.1111/are.15217
Livak K. J., Schmittgen T. D. (2001). Analysis of relative gene expression data using real-time quantitative PCR and the 2-ΔΔCT method. Method. 25, 402–408. doi: 10.1006/meth.2001.1262
Lu Y., Li J., Zhang X., Wang A., Xian J., Wang D. (2018). Effects of dietary replacement of fish meal protein by soybean meal protein on growth, muscle composition and enzyme activities of juvenile crayfish cherax quadricarinatus. Hebei. Fish. 4, 1–18. doi: 10.3969/j.issn.1004-6755.2018.04.001
Mai K. S., Lv M. D., He G. (2021). The issue of protein sources of aquafeed-a new approach to improve utilization efficiency of feed protein. Feed. Ind. 42 (1), 2–6. doi: 10.13302/j.cnki.fi.2021.01.001
Peng C. (2020). The mechanism of β-conglycinin and glycinin induced IPEC-J2 cell damage (Hefei, China: Anhui Agricultural University).
Peng K., Huang W., Zhao H., Sun Y., Chen B. (2021). Dietary condensed tannins improved growth performance and antioxidant function but impaired intestinal morphology of litopenaeus vannamei. Aquacult. Rep. 21, 100853. doi: 10.1016/j.aqrep.2021.100853
Peng K., Shirley D. C., Xu Z., Huang Q., McAllister T. A., Chaves A. V., et al. (2016). Effect of purple prairie clover (Dalea purpurea vent.) hay and its condensed tannins on growth performance, wool growth, nutrient digestibility, blood metabolites and ruminal fermentation in lambs fed total mixed rations. Anim. Feed. Sci. Technol. 222, 100–110. doi: 10.1016/j.anifeedsci.2016.10.012
Santigosa E., Sanchez J., Medale F., Kaushik S., Perez-Sanchez J., Gallardo M. A. (2008). Modifications of digestive enzymes in trout (Oncorhynchus mykiss) and sea bream (Sparus aurata) in response to dietary fish meal replacement by plant protein sources. Aquaculture 282, 68–74. doi: 10.1016/j.aquaculture.2008.06.007
Shukla A., Kumar M., Gupta G., Pathak N., Mishra V. (2019). A review on replacing fish meal in aqua feeds using plant and animal protein sources. Int. J. Chem. Stud. 7 (3), 4732–4739. doi: 10.13140/RG.2.2.13085.13285
SPSS (Statistical Packages for Social Sciences) (2007). Statistical packages for social sciences (version 17.0) (Chicago, IL, USA: SPSS Inc.).
Tibaldi E., Hakim Y., Uni Z., Tulli F., Francesco M., Luzzana U., et al. (2006). Effects of the partial substitution of dietary fish meal by differently processed soybean meals on growth performance, nutrient digestibility and activity of intestinal brush border enzymes in the European sea bass (Dicentrarchus labrax). Aquaculture 261, 182–193. doi: 10.1016/j.aquaculture.2006.06.026
Ulloa P. E., Pena A. A., Lizama C. D., Araneda C., Iturra P., Neira R., et al. (2013). Growth response and expression of muscle growth-related candidate genes in adult zebrafish fed plant and fishmeal protein-based diets. Zebrafish 10 (1), 99–109. doi: 10.1089/zeb.2012.0823
Wang G., Peng K., Hu J., Yi C., Chen X., Wu H., et al. (2019). Evaluation of defatted black soldier fly (Hermetia illucens l.) larvae meal as an alternative protein ingredient for juvenile Japanese seabass (Lateolabrax japonicus) diets. Aquaculture 507, 144–154. doi: 10.1016/j.aquaculture.2019.04.023
Wang L., Wang J., Lu K., Song K., Mai K., Zhang C., et al. (2020). Total replacement of fish meal with soybean meal in diets for bullfrog (Lithobates catesbeianus): Effects on growth performance and gut microbial composition. Aquaculture 524, 735236. doi: 10.1016/j.aquaculture.2020.735236
Wang Y., Yu S., Wang Y., Che J., Zhao L., Bu X., et al. (2016). Effect of replacing fish meal with soybean meal on growth, feed utilization and nitrogen and phosphorus excretion of juvenile pseudobagrus ussuriensis. Aquacult. Res. 47, 3145–3155. doi: 10.1111/are.12765
Wei Y., Li B., Xu H., Liang M. (2020a). Fish protein hydrolysate in diets of turbot affects muscle fibre morphometry, and the expression of muscle growth-related genes. Aquacult. Nutr. 26, 1780–1791. doi: 10.1111/anu.13129
Wei Y., Xu H., Liang M. (2020b). Amino acid absorption and protein synthesis responses of turbot scophthalmus maximus to lysine and leucine in free, dipeptide and tripeptide forms. Aquacult. Nutr. 26, 358–367. doi: 10.1111/anu.12998
Wu Y., Wang Y., Ren X., Huang D., Si G., Chen J. (2021). Replacement of fish meal with gamma-ray irradiated soybean meal in the diets of largemouth bass micropterus salmoides. Aquacult. Nutr. 27, 977–985. doi: 10.1111/anu.13239
Xin F. (2016). Gene cloning and functional study on the important genes of mTOR signalingin litopenaeus vannamei (Beijing, China: University of Chinese Academy of Sciences).
Xu J. (2009). The influence of the extracted soybean glycinin and β-conglycinin on the function and molecular mechanism of mice intestinal-epithelial cells in primary cultivation (Chengdu, China: Sichuan Agricultural University).
Xu T., Xu D., Liu X., Zhao W. (2021). Effect of dehulled soybean meal instead of fish meal on growth and body composition of litopenaeus vannamei. Feed. Res. 13, 72–76. doi: 10.13557/j.cnki.issn1002-2813.2021.13.018
Yang C., Chen H., Peng M., Li Q., Zeng D., Liu Q., et al. (2021). Transcriptome sequencing and screening of genes related to muscle growth and development in litopenaeus vannamei. J. Sou. Agric. 52 (9), 2319–2328. doi: 10.3969/j.issn.2095-1191.2021.09.001
Yang Q., Yan B., Dong X., Chi S., Liu H. (2015). Effect of replacing fish meal with extruded soybean meal on growth, feed utilization and apparent nutrient digestibility of juvenile white shrimp (Litopenaeus vannamei). J. Ocean. Univ. China 14 (5), 865–872. doi: 10.1007/s11802-015-2572-6
Yue Y. R., Liu Y. J., Tian L. X., Gan L., Yang H. J., Liang G. Y. (2012). Effects of replacing fishmeal with soybean meal and peanut meal on growth, feed utilization and haemolymph indexes for juvenile white shrimp litopenaeus vannamei. Boone. Aquacult. Res. 43, 1687–1696. doi: 10.1111/j.1365-2109.2011.02976.x
Yun H., Shahkar E., Hamidoghli A., Lee S., Won S., Bai S. C. (2017). Evaluation of dietary soybean meal as fish meal replacer for juvenile whiteleg shrimp, litopenaeus vannamei reared in biofloc system. Int. Aquat. Res. 9, 11–24. doi: 10.1007/s40071-017-0152-7
Zhang X., Han P., Hu J., Liu L., Chen Y., Xu W., et al. (2020). Effects of replacement of fish meal by soybean meal on growth performance, protein utilization and intestinal tissue morphology of channa argus. Chin. J. Anim. Nutr. 32 (6), 2799–2807. doi: 10.3969/j.issn.1006-267x.2020.06.040
Zhang J., Lv F., Huang Z., Liu Z., Hu Y., Wang P., et al. (2015). Effects of fish meal replacement by different proportions of extruded soybean meal on growth performance, body composition, intestinal digestive enzyme activities and serum biochemical indices of rice filed eel (Monopterus albus). Chin. J. Anim. Nutr. 27 (11), 3567–3576. doi: 10.3969/j.issn.1006-267x.2015.11.030
Zhao X. (2021). Effects of dietary glycinin or β-conglycinin addition on the growth performance and intestinal health of grouper (Epinephelus coioides) (Xiamen, China: Jimei University).
Zhao X., Wang Y., Wang X., Ye J. (2021). Growth performance, plasma components, and intestinal barrier in grouper (Epinephelus coioides) are altered by dietary fish meal replacement with extruded soybean meal. Aquacult. Rep. 21, 100863. doi: 10.1016/j.aqrep.2021.100863
Keywords: fishmeal, soybean meal, growth, digestion, muscle growth, Litopenaeus vannamei
Citation: Peng K, Chen X, Lu H, Zhao J, Chen Y, Li C, Li H and Huang W (2022) Effect of dietary soybean meal on growth performance, apparent digestibility, intestinal digestive enzyme activity and muscle growth-related gene expression of Litopenaeus vannamei. Front. Mar. Sci. 9:945417. doi: 10.3389/fmars.2022.945417
Received: 16 May 2022; Accepted: 21 July 2022;
Published: 12 August 2022.
Edited by:
Helena Peres, University of Porto, PortugalReviewed by:
Hongliang Zuo, Sun Yat-sen University, ChinaCopyright © 2022 Peng, Chen, Lu, Zhao, Chen, Li, Li and Huang. This is an open-access article distributed under the terms of the Creative Commons Attribution License (CC BY). The use, distribution or reproduction in other forums is permitted, provided the original author(s) and the copyright owner(s) are credited and that the original publication in this journal is cited, in accordance with accepted academic practice. No use, distribution or reproduction is permitted which does not comply with these terms.
*Correspondence: Wen Huang, aHVhbmd3ZW41NDlAMTI2LmNvbQ==
Disclaimer: All claims expressed in this article are solely those of the authors and do not necessarily represent those of their affiliated organizations, or those of the publisher, the editors and the reviewers. Any product that may be evaluated in this article or claim that may be made by its manufacturer is not guaranteed or endorsed by the publisher.
Research integrity at Frontiers
Learn more about the work of our research integrity team to safeguard the quality of each article we publish.