- 1Glasgow Polyomics, Wolfson Wohl Cancer Research Centre, College of Medical, Veterinary & Life Sciences, University of Glasgow, Glasgow, United Kingdom
- 2Sea Mammal Research Unit, School of Biology, University of St Andrews, St Andrews, United Kingdom
- 3Institute of Biodiversity, Animal Health & Comparative Medicine, Graham Kerr Building, College of Medical, Veterinary and Life Sciences, University of Glasgow, Glasgow, United Kingdom
True, phocid seals have the shortest known lactations relative to body mass, during which mass transfer of adipose stores from mother to offspring occurs at an unrivalled rate and extent. The mothers of most species of seal fast until weaning whilst their pups gorge on the most fat-rich milks known. This results in a dramatic reduction in maternal blubber mass while pups may triple their body weights before weaning. Mothers mobilise their blubber fat, transport it via blood to their mammary glands and into milk, whilst pups transfer fat in the opposite direction, from their intestines, via blood, to their blubber. Using proteomic analysis of mother and pup sera from Atlantic grey seals, we find that this mirror image flux of lipids between mothers and pups is reflected in an almost inverse relationship in the proteins in their blood specialised to transport fats, lipids, and fat-soluble vitamins. For instance, apolipoproteins ApoB-48/100, ApoA-II and ApoA-IV, which are structural components of the main lipid carrier complexes such as chylomicrons and HDL particles, occur at much higher levels in pups than mothers. Meanwhile, carriers of fat-soluble vitamins such as retinol- and vitamin D-binding proteins are lower in pups and gradually build towards weaning. In contrast, sex hormone-binding globulin occurs at remarkably high relative concentrations in pups. There are therefore dramatic differences between, and an unrealised complexity in, the balance of proteins involved in the rapid transfer of fats and other lipids from mother to pups in preparing their offspring for their post-weaning fasts on land and eventual survival at sea before they can feed again.
Introduction
True, phocid, seals engage in the most rapid transfer of adipose body mass from mother to offspring known among mammals, and have amongst the highest concentrations of fats in their milks (Berta et al., 2015; Crocker and Mcdonald, 2016). During their uniquely short lactation periods for their size, mother seals transfer sufficient nourishment to more than double their pups’ body masses (Berta et al., 2015; Crocker and Mcdonald, 2016), and this is achieved in most phocids while the mothers do not themselves feed (Schulz and Bowen, 2004; Rosen and Hindle, 2016). Mothers and pups have inverse fat mobilisation fluxes – mothers mobilise blubber fat into their blood, use it for fuel and also translocate it to milk - pups consume milk fats, digest, chemically process, and package them for release into blood, and thence for use as fuel or to build their blubber stores. At each stage the bulk of fat and other insoluble lipids are suspended in the aqueous phase by association with specific apolipoproteins and incorporated into large lipoprotein complexes. The proteins that do this in blood and milk are different, but their emulsification activities are similar. While the amount of lipids in transit to the mammary glands in a mother’s circulation may be similar in total to that in transit to blubber in their pups, the latter’s blood volumes are smaller, resulting in the need for pups to cope with higher fat concentrations in their sera. The fat and lipid transporter protein profiles may therefore be dramatically different between mothers and pups and change as lactation proceeds to its conclusion.
Blubber is a vascularized layer of subcutaneous adipose tissue found in all pinnipeds, sirenians and cetaceans to varying degrees according to differences in function. While it is the main lipid (and thus energy) store, the degree to which it is present can influence insulation, buoyancy, hydrodynamics and in some cases sound propagation [e.g. cetaceans; (Struntz et al., 2004)]. Most phocid species, with few exceptions (monk seals), live in temperate or polar seas, and many raise their pups exclusively on ice (e.g. Weddell, leopard, harp, hooded seals) (Schulz and Bowen, 2004). Mother seals must balance retention of sufficient blubber mass for survival during nursing and subsequent return to cold seas against providing their offspring with sufficient reserves to survive their own period of post-weaning fast before going to sea independently (Berta et al., 2015; Crocker and Mcdonald, 2016). At sea, seal pups have greater need for insulation and metabolic heating than adults because they have a higher surface area to volume ratio and consequent greater heat loss (Burns and Castellini, 1996; Noren et al., 2005). Moreover, since pups are not accompanied by their mothers they need to endure a period of time before they can hunt efficiently to replenish and enlarge their own blubber layers (Carter et al., 2017). Quite why phocids exhibit such short lactations remains in debate but is nevertheless compensated by the provision of such fat-rich milks. And the pups ingesting it must have sufficient means to process, package and distribute remarkably high levels of ingested fats through their blood to their own blubber.
Triglyceride fats ingested by pups will be emulsified by bile salts in their intestines, where they are hydrolysed to free fatty acids and monoacylglycerols (Sesorova et al., 2021). Along with cholesterol esters, these will be taken into enterocytes where monoacylglycerols will be synthesised back into triacylglycerols, loaded into lipid-rich particles that are rendered soluble by association with apolipoproteins, released into intestinal lacteals that feed into lymph entering the thoracic duct, and hence into the venous blood close to the heart (Sesorova et al., 2021). This route from the intestine to the blood avoids the portal vein and liver directly such that lipids can mostly bypass the active fat metabolism in the liver to reach adipocytes of the blubber. Smaller lipids, such as free fatty acids, may dissolve in the lipid cores of lipoprotein particles or be transported in proteins such as serum albumin that are not part of large lipoprotein complexes. Material in this route will also supply muscle, immune system, heart, and brain that need lipid for energy metabolism, cell growth, and development, and also provide developmentally important signalling lipids such as eicosanoids, lipidic hormones, and vitamins.
The Atlantic grey seal Halichoerus grypus is a typical capital-breeding phocid where mothers fast while rapidly provisioning offspring. In this species a pup may treble its initial body mass in eighteen days of nursing, during which a mother relies on stored reserves to maintain herself and her pup. Mothers consequently lose about 40% of their body mass comprising predominately lipid and protein stores (Pomeroy et al., 1999; Smout et al., 2020). We have examined the lipid transporter proteins engaged in the mass transfer of fats, those that carry small lipids such as fatty acids, and water-insoluble (“fat soluble”) vitamins and hormones in both mother and pup grey seals, from soon after birth until weaning. We found distinct differences in proportions of all these classes of transport proteins between mothers and pups, and there were dramatic changes in most of them from birth to weaning in pups. This highlights the dynamic complexity of the rapid provisioning by mothers and how their pups deal with an unrivalled flux of fats and developmentally important small lipids in a highly specialized lactation strategy.
Materials and methods
Animals and serum samples
Blood samples were collected from five free-ranging mother/pup pairs (see Supplementary A for details of animals sampled) on the Isle of May, Scotland (56.18°N 2.55°W), three of which were selected for serum proteomics. Four samples were collected from each mother and pup on days 2, 7, 13, and 17 or 18 or 19 days after birth – for simplicity, samples taken 17, 18, or 19 days are presented as being from day 18 throughout this report. In this species, mothers wean their pups between about 16 and 23 days after birth and return to sea, leaving their pups in the natal colony to continue development before they themselves go to sea independently after a further period of up to 40 days, during which time they do not feed (Bennett et al., 2010). All five pups had growth rates and weaned at masses within the normal range for grey seals in the British Isles (Pomeroy et al., 1996; Pomeroy et al., 1999). Their minimum durations of suckling ranged from 18-21 days. To avoid desertions by the mothers immediately after birth, the earliest samples were taken on day 2 post-partum. Mothers were tranquilised with an intramuscular mass-adjusted dose of Zoletil 100 (Virbac, Bury St Edmunds, Suffolk, UK), followed by intravenous oxytocin to stimulate milk let-down to provide milk samples as part of a larger study, blood was taken, and given a mass-adjusted intramuscular prophylactic dose of tetracycline after the day 7 and day 18 samples, as reported previously (Lowe et al., 2017; Watson et al., 2020; Watson et al., 2021; Mcgill et al., 2022). Blood was collected by venepuncture of either extradural vein or hind flipper plexus using 3.5in 16G spinal needle or 2in 19G Microlance needle (Becton Dickinson, UK) as appropriate into plain vacutainers and allowed to clot. Serum was separated by centrifugation within four hours of collection, frozen at -20°C and stored at that temperature or below and while sent to Glasgow for analysis. Samples from all five mother/pup pairs (pairs A to E) were collected, and three pairs (B, D and E) were selected for the proteomics. At each capture, mothers and pups were checked externally for signs of ill health or abnormal growth of pups which may have indicated underlying problems. Pups on breeding colonies risk being bitten or suffer injuries from neighbouring adults resulting in infections or trauma - no evidence of such was detected in our sample.
Tandem Mass Tag proteomics
This multiplex comparative proteomics technique provides relative abundances of detected proteins between six samples in one analysis run, and the technique used here is identical to that described in our previous paper (Mcgill et al., 2022). That paper used data acquired from the same three mother/pup pairs as here, having been selected from field observations indicating that birth had occurred within 36 hours after birth, and also that the final sample day occurred on the last day of lactation (pair E) or within three days of its termination (pairs B and D). Serum samples from a given mother that were taken on days 2, 7 and 18 after birth were analysed along with samples taken on the same days from her pup. This method thereby provides relative abundances (not absolute values) of serum proteins as they change in a mother and her pup within a single six-sample comparative multiplex run.
The overall protein concentrations in the sera of grey seal pups increases over time, most clearly due to immunoglobulin and other defence protein changes (Mcgill et al., 2022). We therefore chose to submit equal volumes of serum for TMT proteomics rather than equal amounts of protein in order to more accurately detect changes in individual proteins. Tryptic peptides were generated from each serum sample using the Filter-Assisted Samples Preparation (FASP) method (Wisniewski et al., 2009), omitting LysC enzyme digestion steps and using a 30kDa ultrafiltration spin column. Equal volumes of the resulting peptide samples were labelled with TMT 6-plex reagents according to the manufacturer’s instructions (Thermo Scientific). Each of the six samples derived from each mother/pup pair were labelled with a set of six isobaric TMT reagents. Samples were then combined in equal volumes to constitute three separate 6-plex sets, comprising sera from one mother and her pup. 6ug mixed, TMT-labelled peptides were dried and resolubilized in 20 µL 5% acetonitrile with 0.5% formic acid using the auto-sampler of a nanoflow uHPLC system (Thermo Scientific RSLCnano). Online detection of peptide ions was by electrospray ionisation (ESI) mass spectrometry MS/MS with an Orbitrap Elite MS (Thermo Scientific). Ionisation of LC eluent was performed by interfacing the LC coupling device to a NanoMate Triversa (Advion Bioscience) with an electrospray voltage of 1.7 kV. An injection volume of 5 µL of the reconstituted protein digest was desalted and concentrated for 12 min on trap column (0.3 × 5 mm) using a flow rate of 25 µl/min with 1% acetonitrile with 0.1% formic acid. Peptide separation was performed on a Pepmap C18 reversed phase column (50 cm × 75 µm, particle size 3 µm, pore size 100 Å, Thermo Scientific) using a solvent gradient at a fixed solvent flow rate of 0.3 μl/min for the analytical column. The solvent composition was A) 0.1% formic acid in water B) 0.08% formic acid in 80% acetonitrile 20% water. The solvent gradient was 4% B for 10 min, 4 to 60% for 170 min, 60 to 99% for 15 min, held at 99% for 5 min. A further 10 minutes at initial conditions for column re-equilibration were used before the next injection. The Orbitrap Elite was set to acquire a high-resolution precursor scan at 60 000 RP (over a mass range of m/z 380 – 1800) followed by CID fragmentation and detection of the top 3 precursor ions from the MS scan in the linear ion trap. The 3 precursor ions are also subjected to HCD in the HCD collision cell followed by detection in the Orbitrap. Singly charged ions were excluded from selection, while selected precursors were added to a dynamic exclusion list for 180 seconds.
MS/MS spectra were analysed for protein identification and quantification using Proteome Discoverer software 2.4 (Thermo Scientific). Protein identifications were assigned using the Mascot search engine (v2.6.2, Matrix Science) to interrogate protein sequences in the NCBIprot database using the taxonomy Caniformia and Phocidae datasets with a precursor mass tolerance of 10 ppm and fragment ion mass tolerance of 0.3 Da. Set modifications were addition of TMT 6-plex labels to lysines and N-termini, carbamidomethylation of cysteine (fixed) and methionine oxidation (variable), allowing for the possibility of iodination of tyrosines, deamination of asparagines and glutamines, and carbamidomethylation of peptide N-termini. Two missed cleavages for the trypsin digestion were permitted. Unique peptides were allowed for protein quantification in the consensus workflow, and normalization was designated for the total peptide amount with a scaling mode based on channels average per file. The quantification ratios were filtered based on fold change threshold equal to or greater than 1.5. The false discovery rate (FDR) was set at 5%.
Statistical analysis and graphing
Heatmaps with hierarchical clustering, variable importance projection (VIP) analysis, and Principal Component Analysis (PCA) were carried out using MetaboAnalyst 5.0 (https://www.metaboanalyst.ca/), for which the data were assembled for upload in MS Excel and then log transformed and normalised online with MetaboAnalyst 5.0. ANOVA with Tukey’s procedure and graphing were carried out using MetaboAnalyst and OriginPro 2021 software (OriginLab, Northampton, Massachusetts, USA), and both were used to create the figures and graphs. The Excel file in Supplementary B provides all the original data and statistical analysis from MetaboloAnalyst. OriginPro was used to create the statistical results tables for all of the graphs in the main text and are available in Supplementary A.
Results and discussion
Inverse abundances of lipid carrier proteins in the sera of mother and pups
The visual appearance of the serum of mother and pup grey seals taken from soon after birth to near weaning illustrates the dramatic differences in their fat concentrations and how they change with time (Figure 1). The earliest samples from pups are visibly less turbid than later (albeit also affected by recency of feeding), epitomised here by seal pup D (Figure 1). The differences in appearance likely reflect the concentrations of lipids, fats in particular, chemical modifications of the lipids in transit, or how they are packaged and emulsified for transport. An immediate question is how, if the quantities of fats in the bloodstream of pups are derived from fats transiting from blubber to mammary glands of the mothers, are maternal sera so much less turbid? This could be due at least partially to blood volume differences, but also how the lipids are transported in each. Triglyceride fats are particularly insoluble so are emulsified for transport in blood by inclusion in large, light-scattering, lipoprotein particles such as chylomicrons, LDL and HDL (Mehta and Shapiro, 2021), the structural components of which are the several apolipoproteins dealt with below. While we do not currently have direct measurements of fat content of mother and pup sera during lactation, and are aware of no published information on this for any phocid seal, the dramatic visual differences alone shown in Figure 1 prompt questions that can start to be answered by understanding the proteins involved in the mass distribution of fats in mothers and pups.
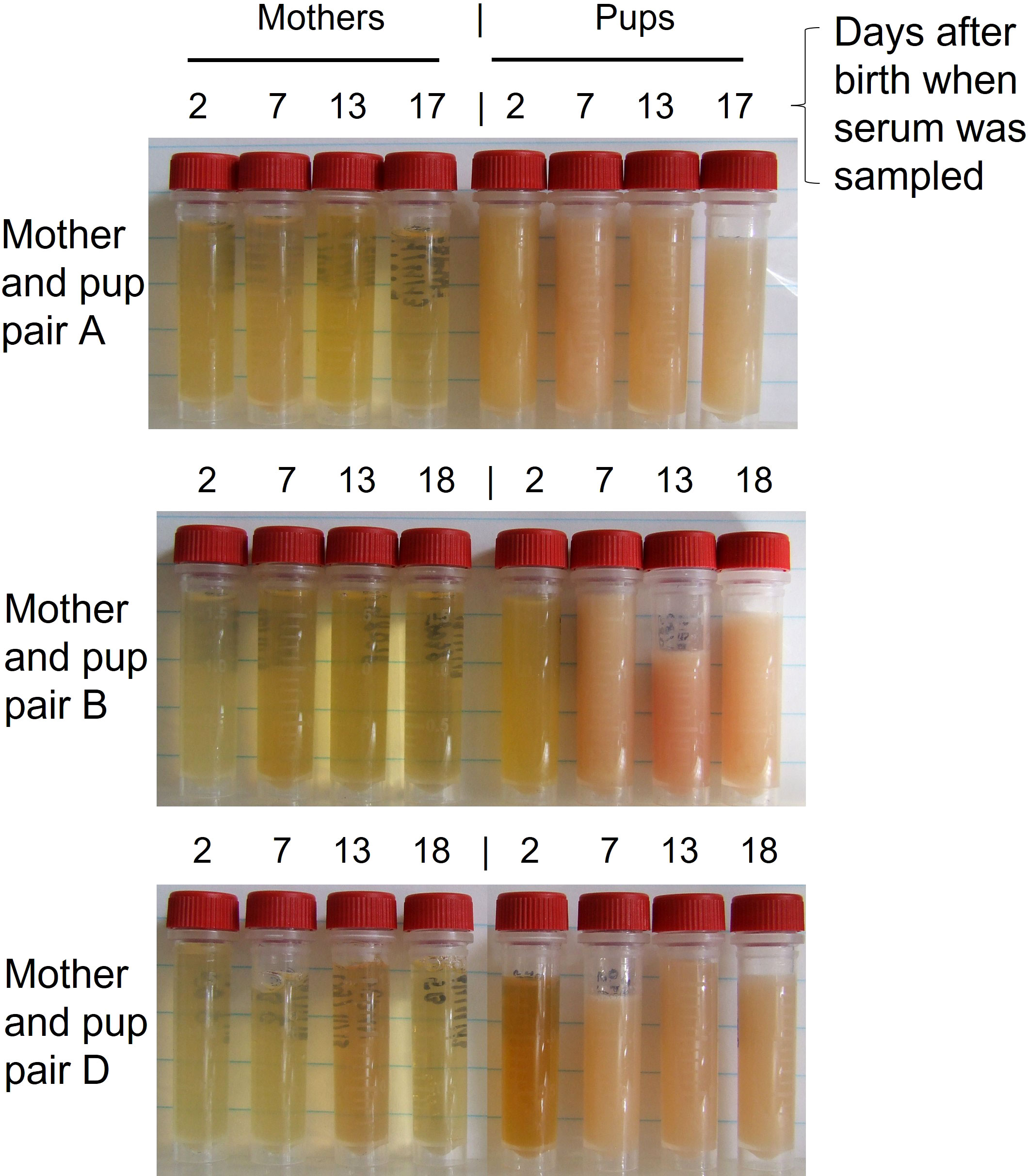
Figure 1 Appearance of serum samples of mother and pup Atlantic grey seals at the times indicated after birth. Pups’ sera showed initial and increasing turbidity with time (also likely affected by time since suckling), indicative of increasing content of fats and other lipids, whereas maternal sera show little change. The turbidity of sera will be affected by both the content of fats and other lipids and how they are emulsified by incorporation into lipoprotein particles. The samples had been stored frozen until thawed. Adapted from supplement to ref. (Watson et al., 2020).
We used tandem mass tag (TMT) proteomics to compare lipid transporter proteins in mother and pup sera. This technique yields the relative abundance of a given protein species, but not absolute concentrations, between a set of six samples analysed simultaneously. We collected samples from three mother and pup pairs of grey seals. Each individual was sampled on three occasions from shortly after birth to near weaning, and the three samples from each mother together with the three samples from her pup were examined in parallel, in the same analytical run. For this we chose a time shortly after lactation had begun (day 2), when main phase lactation would likely have established (day 7), and as weaning may be imminent (day 18), omitting day 13 as likely similar to day 7. Our analysis was confined to lactating mothers and how their protein levels compare with resting levels in non-breeding females, or during season changes, remains to be established. Our methods do, however, follow changes in mothers relative to their pups throughout lactation.
Principal component analysis (PCA) reveals a dramatic divergence between lipid carrier proteins in mother and pup sera at all times between birth and weaning (Figure 2A). This is further emphasised in a heatmap of relative abundances of individual protein types shown in Figure 2B, which also includes hierarchical clustering dendrograms that clearly separate mother and pup sera, in addition to demarcating the proteins into two groups. Some proteins are clearly at higher abundances in pups relative to their mothers, and others exhibit the opposite. Variable importance projection analysis (VIP) of the data identifies proteins that best segregate mother and pup sera - those with VIP scores greater than unity are potentially the best discriminators (Figure 2C). These comprise a mixture of proteins involved in bulk transport of lipids and fats (apolipoproteins) along with proteins that are not involved in such complexes but bind small insoluble lipids such as fatty acids, fat-soluble vitamins, and sex hormones. These proteins are considered as three main groups below and we examine how individual proteins within a group change with time.
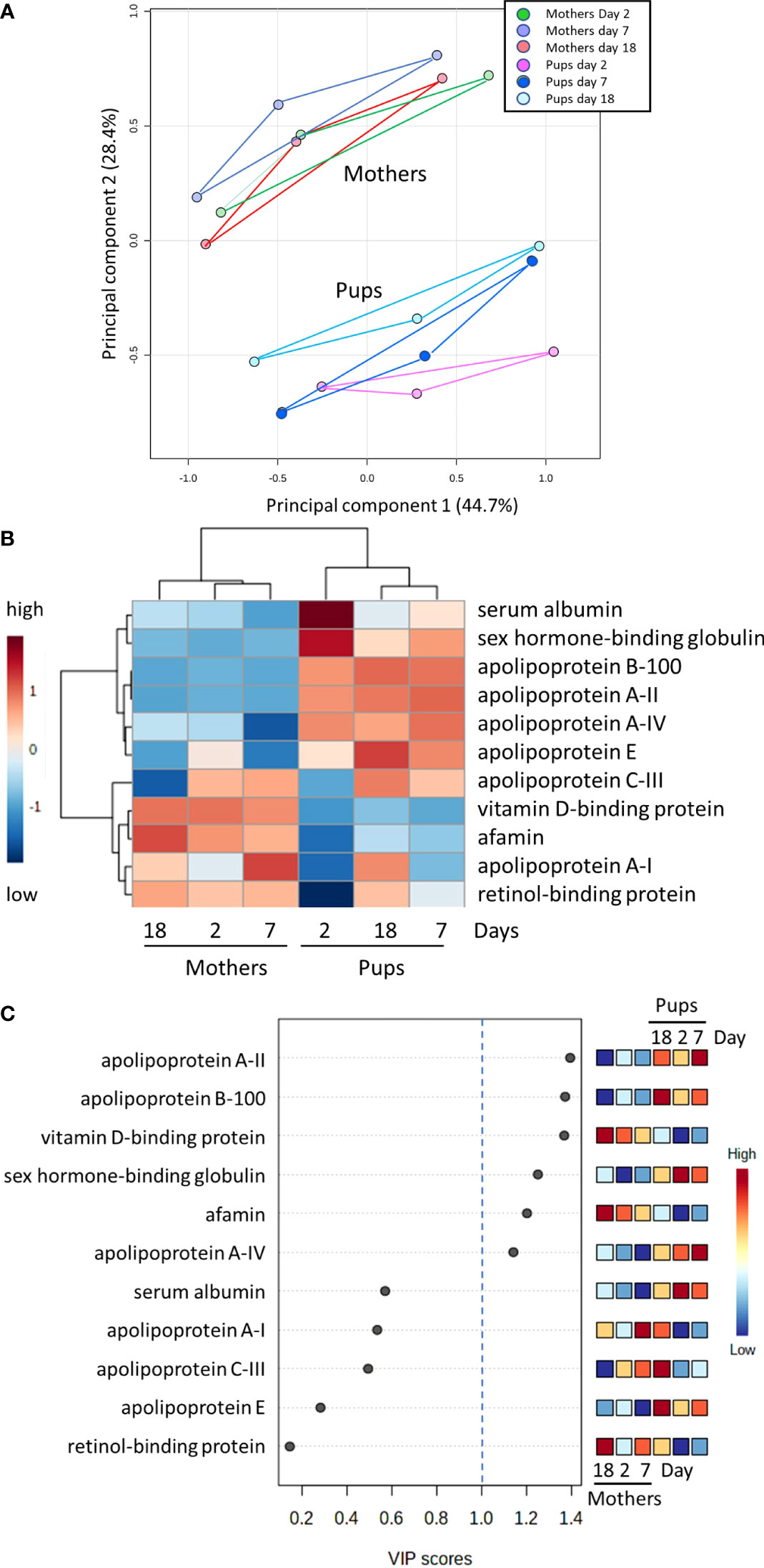
Figure 2 Contrasting profiles of serum lipid carrier proteins in mother and pup grey seals with time after birth. (A) Principal component analysls based on log transformed relative abundance data for the proteins listed in (B). Values were obtained from TMT proteomics from three mother and pup pairs (pairs B, D and E) see Supplementary B) sampled on days 2, 7, and 18 after birth. (B) Heatmap with hierarchical clustering of the lipid carrier proteins. The heatmap was created with group averages (three pups on the given day after birth, three mothers on the given day). Note how the cluster dendrogram above the heatmap differentiates mothers’ sera from pups on all days. The day order is as automatically determined in the analaysis in comparing the overall protein balance in the grouped samples. A similar heatmap from the same dataset but for individual mothers and pups is given in Supplementary Figure S1. (C) Variable importance projection analysis (VIP) of the data. Proteins that have scores greater than 1 (indicated by the dotted blue line) best segregate mother and pup sera.
Lipid particle apolipoproteins
The main lipid-carrier particles in blood are, in descending size order and increasing density, chylomicrons, very low density lipoproteins (VLDL), low density lipoproteins (LDL), and high density lipoproteins (HDL) (Wasan et al., 2008). These comprise lipid-rich cores containing triglycerides, phospholipids, non-polar lipids, and cholesterol derivatives, held together by peripheral, surface-embedded apolipoproteins that may differ in content and proportion between particle types (Mehta and Shapiro, 2021). The proteins serve as structural components of the particles and allow them to be soluble but also act as agents in the exchange of lipids between different lipoprotein particles, bind to receptors on cell surfaces, and activate or suppress enzymatic modifications of lipids (Wasan et al., 2008).
Chylomicrons have the highest proportion of triacylglycerol fats to protein, are mainly produced in the intestine and liver, and contain isoforms of ApoA, ApoB, ApoC, and ApoE (Wasan et al., 2008; Mehta and Shapiro, 2021). Isoforms ApoA-II, ApoA-IV, and ApoB-48/100 are of particular note, all of which occurred at higher levels in pups than mothers, with ApoE increasing with time (Figure 3).
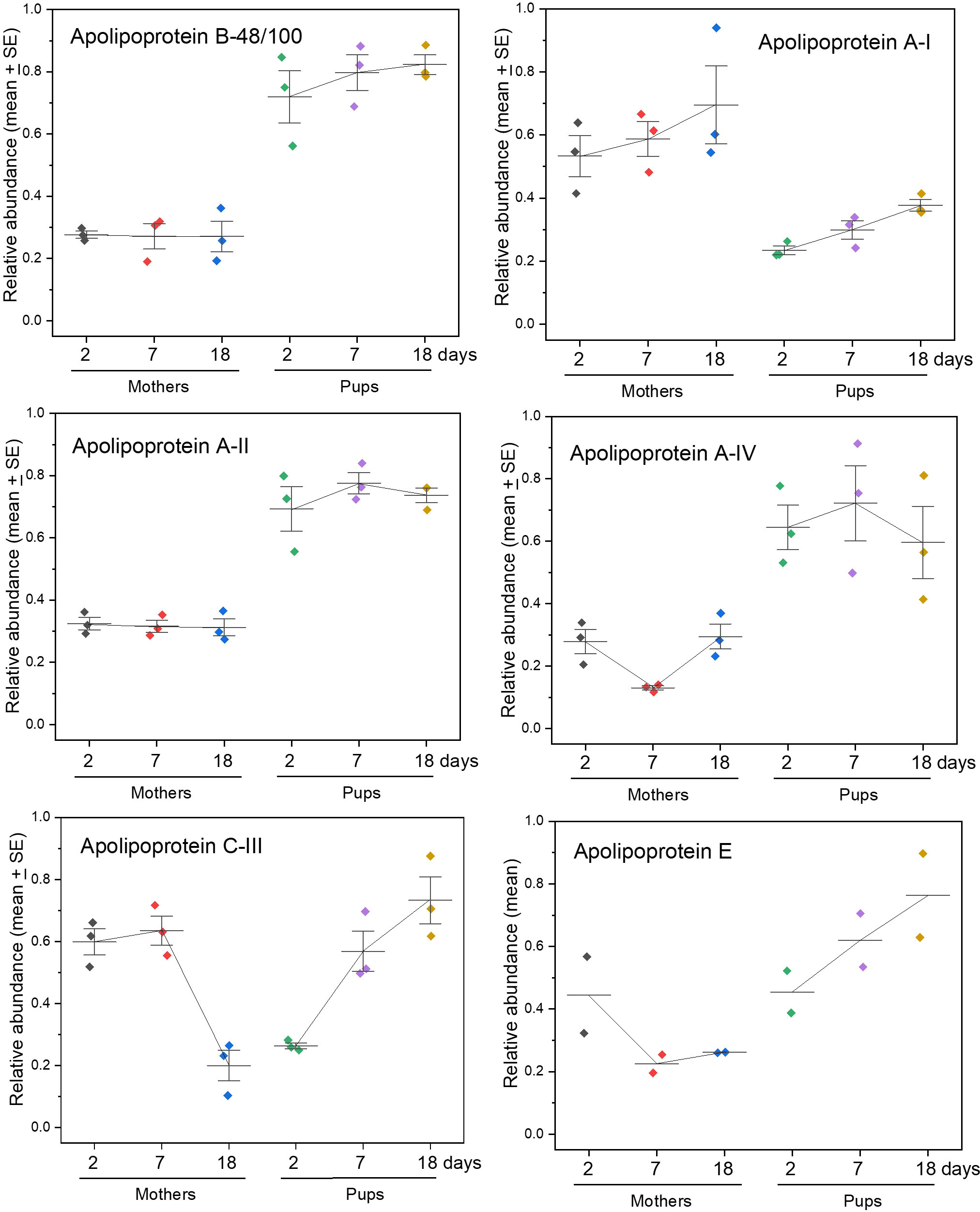
Figure 3 Changes in apolipoproteins that contribute to lipoprotein particles - chylomicrons (the largest lipoprotein particles), LDL, HDL, etc. ApoB-48 is the main component of chylomicrons synthesised in the intestine as an abbreviated form of ApoB-100 which is instead mainly produced in the liver - interrogation of genome databases with the TMT proteomics data amalgamates the two proteins. The data for ApoA-1 shown here were obtained using TMT proteomic searches that identified this protein from the Weddell seal genome, and data indicating a less distinct trend as derived from the harbour seal genome are given in Figure S2; the amino acid sequences of ApoA-1 are at least 97% identical or identical between these and grey seals. See Supplementary Files A, B for for the statistical analysis of differences between mothers and pups for each protein for each sampling day.
The largest protein component of chylomicrons produced in the intestine is ApoB-48, This is encoded in the ApoB-100 gene but its mRNA is truncated by RNA editing to yield a protein comprising the first 48% of ApoB-100’s amino acid sequence (Fisher and Mcleod, 2021; Mehta and Shapiro, 2021). Full length ApoB-100 is synthesised in the liver and is a structural component of VDL and LDL, but not HDL or chylomicrons (Wasan et al., 2008). Both isoforms will be found in circulation, but ApoB-48 will be specific to chylomicrons produced in the intestine (Wasan et al., 2008). A prediction would therefore be that pup sera will be enriched in ApoB-48 to carry lipids from their intestines, while mothers’ sera would be depleted in ApoB-48 because they are fasting. The genome information interrogated with our proteomics data will automatically list both ApoB-48 and ApoB-100 present as the latter. But, when we examined the peptides yielded by the proteomics that were above detection levels in pups and mothers, we found 14 peptides matching ApoB-100, 13 of which are in the ApoB-48 section, so only one from the remainder of ApoB-100 (Supplementary Table S1). This is consistent with pups making more B48 than B100 to cope with bulk absorption and distribution of fats and other lipids, and that mothers make little of either.
The size of chylomicrons in circulation increases in high fat-fed animals (Sesorova et al., 2020) (which may contribute to the turbidity of pup sera seen in Figure 1), so the ratio of ApoB-48 to ApoB-100 may change in pups as the levels of fat in milk change with time [the synthesis ratios of other apolipoproteins in intestine versus liver also change with feeding or withdrawal of fat (Wu and Windmueller, 1979; Windmueller and Wu, 1981)]. The very high levels of ApoB (as ApoB-48/100) in grey seal pups relative to their mothers is also seen in pups of southern elephant seals (Marquez et al., 2007; Tift et al., 2011).
ApoA-1 is present as a major component of HDL particles in circulation (Wasan et al., 2008; Mehta and Shapiro, 2021). It is also present in chylomicrons, but their ApoA-1 is rapidly transferred to HDL particles in the blood (Wasan et al., 2008). Our proteomics searches produced ambivalent trends in mothers and pups with time. In one there was little change in either (Supplementary Figure S2), and in the other the pups had low levels at first that increased with time but did not reach maternal levels by weaning (Figure 3). ApoA-I is a component of HDL, so is involved in transport of cholesterol and phospholipids from the body’s tissues to the liver from where they are redistributed to other tissues (presumably including the mammary gland) (Wasan et al., 2008; Mehta and Shapiro, 2021). It would therefore be understandable for ApoA-I to be higher in mothers than pups, as seen in Figure 3.
ApoA-II was consistently about twice as abundant in pups’ sera than in mothers’. ApoA-II is the second most abundant protein in HDL particles and absent in the others (Wasan et al., 2008). HDLs have the highest proportion of protein to lipid amongst the lipoprotein particles and function to carry lipids from cells and lipoproteins to other tissues (Wasan et al., 2008). HDLs are important in delivering cholesterol to steroidogenic tissues such as the gonads and adrenals (Kuhbandner et al., 2021). Given the contributions of both ApoA-I and ApoA-II to particles such as HDLs, the observed inverse relationship of these two lipoproteins in mother and pup sera is puzzling if true – i.e. that ApoA-II abundance was higher in pups than females, while ApoA-I was higher in females than pups. ApoA-II is absent from chylomicrons, and ApoA-1 is present in those particles only transiently. The answer may therefore lie in the protein composition of HDLs, subclasses of which may have both these proteins or only ApoA-I (Blanchard and Francis, 2021). Or that the two proteins have differing roles in other physiological processes such as metabolic regulation and inflammation, or that there is a different attrition rate of ApoA-I in the kidneys of mothers and pups (Francis, 2016; Blanchard and Francis, 2021), and their abundances thereby differ.
In humans, ApoA-IV, like ApoB-48, is confined to chylomicrons formed in the intestine and, like ApoB-48/100, is more abundant in pups than mothers throughout nursing (Figure 3) (Wu and Windmueller, 1979; Qu et al., 2019)). Synthesis and secretion of ApoA-IV on chylomicrons in the intestine increases dramatically upon ingestion of fat (Windmueller and Wu, 1981; Qu et al., 2019), which may already have begun in pups by the time our first samples were taken. Even though our grey seals mothers are not feeding, they have detectable amounts of ApoA-IV in their sera, which could be due to, for example, mobilising the lipid from their intestinal tissues to contribute to milk fat, or residual amounts still in circulation from before their fast. However, it is not yet known whether seals, like some other mammals (e.g. mice and rats), produce ApoA-IV in their livers (Wu and Windmueller, 1979; Qu et al., 2019).
ApoC-III occurs in the most triglyceride-rich lipoproteins, chylomicrons and VLDLs (Mehta and Shapiro, 2021). It inhibits hepatic lipase and lipoprotein lipase and may reduce hepatic uptake of triglyceride-rich particles such that their passage to pups’ blubber is unimpeded (Mendivil et al., 2010). Indeed, elevated levels of ApoC-III in humans associates with the development of hypertriglyceridemia, and in null variants there is a reduction in plasma triglyceride levels (Mehta and Shapiro, 2021). ApoC-III increases linearly with time in the pups, which may be consistent with increasing levels of fat in their mothers’ milk and triglycerides in circulation (Mehta and Shapiro, 2021). Mothers exhibited similar amounts of ApoC-III on days 2 and 7 after birth, but the levels then dropped dramatically (Figure 3). This may also signal a dramatic change in lipid management as weaning approaches and mothers may be reaching starvation.
We only detected ApoE in two out of three mother/pup pairs, but its abundance nevertheless appeared to increase steadily over lactation in pups, yet decreased in mothers (Figure 3). ApoE occurs in all types of lipoprotein particle (Wasan et al., 2008; Mehta and Shapiro, 2021; Van Valkenburgh et al., 2021) and is important in lipid distribution because it interacts with the LDL-receptor, which is central to cellular acquisition and processing of triglyceride-rich lipoproteins (Wasan et al., 2008; Mehta and Shapiro, 2021). It is also produced by astrocytes of the nervous system and functions as the main vehicle for cholesterol transport to neurons and occurs at higher concentrations in cerebrospinal fluid relative to plasma than do other apolipoproteins (Rhea and Banks, 2021; Van Valkenburgh et al., 2021). But of most direct pertinence to the rapid accumulation of blubber mass in seal pups is that ApoE is directly involved in adipogenesis (Chiba et al., 2003; Zhang et al., 2017), which may explain a match with its increasing levels in pup sera with time.
Our fasting mother grey seals show broadly similar results to those from fasting, moulting adult female elephant seals in which plasma Apo-CIII and ApoE decreased with time, and Apo-I increased, although we did not see a decrease in Apo-IV and an increase in Apo-AII (Khudyakov et al., 2022). While both species were fasting during the sampling periods, fasting during lactation (when milk production is important) and fasting during moulting are likely to involve different apolipoprotein dynamics.
Albumin and related proteins
Free fatty acids and small lipids such as fat-soluble vitamins that are not dissolved within the lipid-rich cores of lipoprotein complexes can be carried in a range of other plasma proteins. The principal carrier of small fatty acids in plasma is serum albumin, which is the most abundant protein in plasma, accounting for about 50% of protein by mass. It also binds and circulates a wide range of compounds such as hormones and bilirubin (Wilton, 1990; Peters and Peters, 1996) - the latter is a potentially toxic breakdown product of haem that occurs at elevated levels in the serum of pup and mother grey seals (Watson et al., 2020). Albumin levels appear to be steady in nursing mothers and their pups throughout lactation in southern elephant seals (Marquez et al., 2007). In grey seals, we find that albumin occurs at similar levels in mothers and pups except the earliest samples in pups (day 2 after birth), in which albumin was substantially higher (Figure 4). This may be residual of high levels of albumin required in uterine life, or reflects other roles for albumin such as regulating the osmotic activity of blood (partly balancing the low total protein levels evident in neonatal pups; (Marquez et al., 2007; Mcgill et al., 2022), or the need for albumin to carry a range of other compounds immediately postpartum.
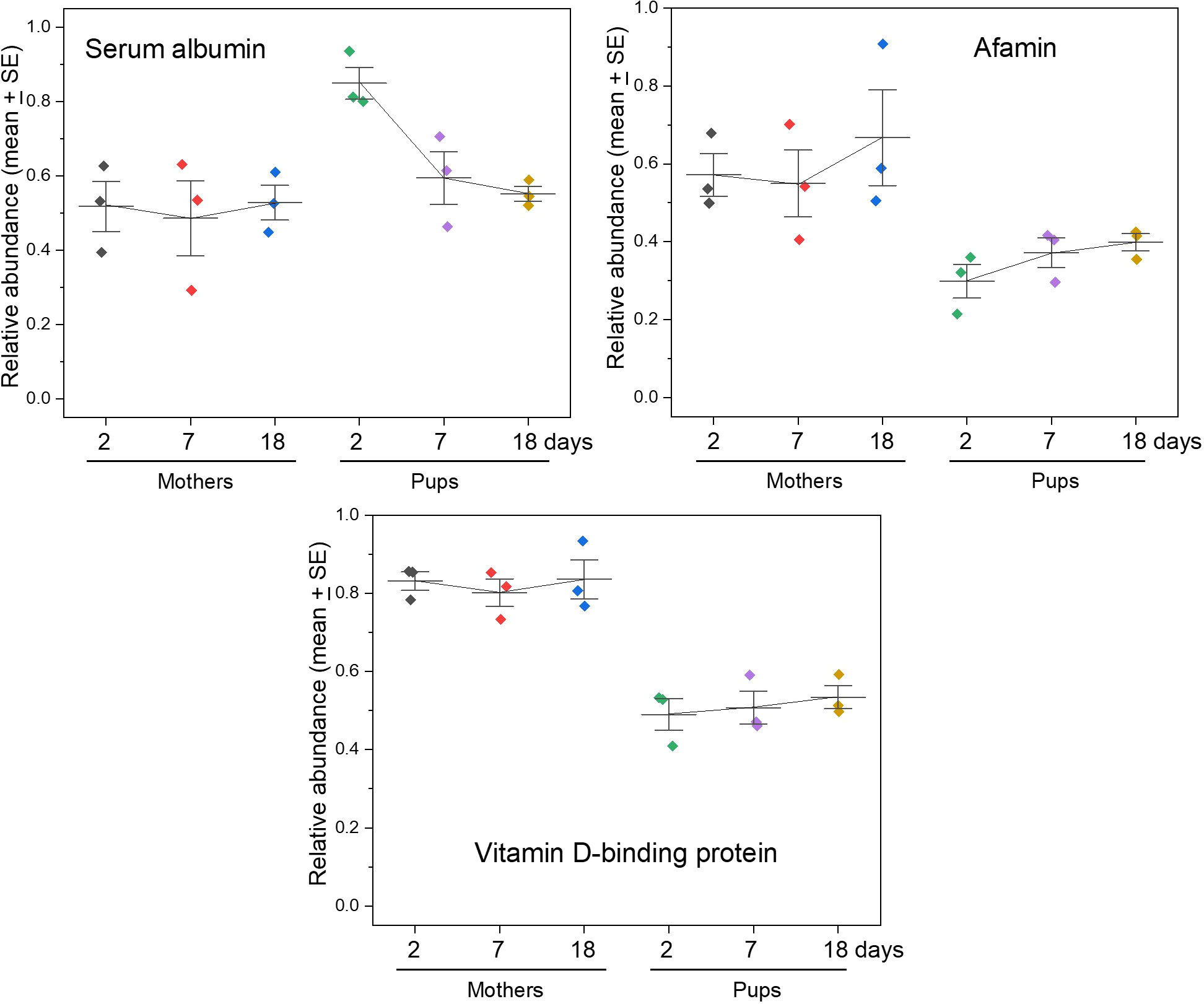
Figure 4 Albumin and related proteins. Serum albumin is the most abundant protein in the plasma of mammals and carries fatty acids, other lipids, and a range of other compounds and mineral ions. Afamin and vitamin D-binding protein are evolutionarily related to serum albumin, and each are known to bind lipids, though less is known about them, afamin in particular. Alpha foetoprotein is also in the albumin family, is considered to perform a similar role to albumin in the foetus, is usually found in the serum of mothers and offspring for a period after birth, but was not detected by our proteomic analysis in these grey seal sera.
Serum albumin is evolutionarily related to the proteins afamin, vitamin D-binding protein, and alpha-foetoprotein that transport small apolar compounds. Afamin is poorly understood but is considered to be a vitamin E carrier at least (Dieplinger and Dieplinger, 2015; Altamirano et al., 2018; Tramontana et al., 2018). Vitamin D-binding protein transports this fat-soluble vitamin which is best known for involvement in skeletal development but has a wide range of other activities including in the immune system (White and Cooke, 2000; Kew, 2019; Bouillon et al., 2020). Unlike albumin, however, these two proteins occurred at levels about half of maternal levels throughout the nursing period in grey seals (Figure 4). Alpha-foetoprotein is the most abundant plasma protein in the human foetus and is considered to be the functional equivalent of albumin in utero (Glowska-Ciemny et al., 2022). In some mammals it is thought to bind and sequester sex hormones so as to avoid aberrant development of gonadal tissue and brain (Bakker and Baum, 2008). In humans, alpha-foetoprotein decreases rapidly after birth in both neonates and mothers (Glowska-Ciemny et al., 2022). But, despite its presumed abundance in seal foetuses, we did not detect it in the serum of either pups or mothers.
Vitamin and hormone-binding proteins
Unexpectedly high relative levels of sex hormone binding globulin (SHBG) were found in pups on day 2 after birth, but SHBG fell to near maternal levels by weaning (Figure 5). Hormones such as testosterone and oestradiol bind to and circulate in both albumin and SHBG, with very little free in the aqueous phase (Simons et al., 2021). SHBG thereby sequesters these hormones and regulates their bioavailability that may otherwise have inappropriate effects in development of tissues including gonads and the brain (Simons et al., 2021). In contrast to pups, levels of SHBG in maternal serum were stable throughout the nursing period. Without reference samples from non-breeding adult females or males, however, it is not possible to determine the significance of its low abundance (relative to pups) and steady presence in lactating females during lactation.
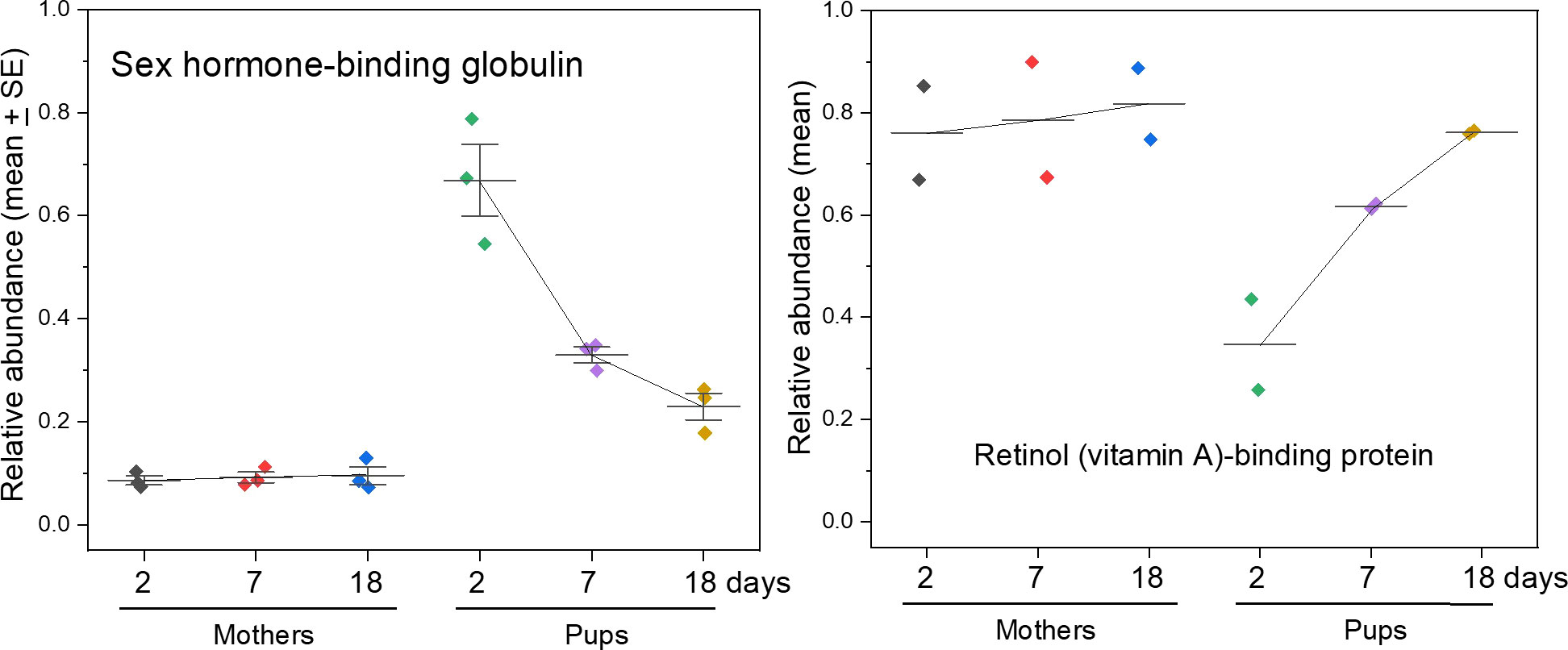
Figure 5 Vitamin and hormone-binding proteins. Sex hormone-binding globulin is present at substantially higher levels in pups than in mothers. Retinol (vitamin A)-binding protein (RBP4 isoform) carries retinol in plasma and is found non-covalently associated with transthyretin (which carries the thyroid hormone thyroxine), the resulting complex being large enough to prevent retinol binding protein passing through the kidney filter and excreted. See Supplementary Figure S2 for a graph of the relative concentrations of retinol-binding protein and transthyretin showing similar trends.
Retinol (vitamin A)-binding protein 4 (RBP4) levels were stable in mothers, whilst pups had low levels at first that eventually increased to maternal levels. Retinol has critical roles in physiology, development, and the immune system, in addition to providing the precursor for visual pigment, retinal (Steinhoff et al., 2022). It is also a precursor of a range of retinoic acid isoforms that are involved in development, cellular differentiation, and the immune system (Steinhoff et al., 2022). RBP4 is the main carrier of retinol in blood and is highly specific for its ligand (Steinhoff et al., 2022). Retinol is water-insoluble and highly susceptible to oxidation, so RBP4 is crucial to its safe distribution to cells and tissues requiring it (Steinhoff et al., 2022). RBP4 is a relatively small protein (~21,000 Da) that would pass through the kidney glomerular filter and be lost to urine were it not in complex with transthyretin, the thyroxine transporter, resulting in a 1:1 stoichiometric complex of about 80 kDa in mass that is above the kidney threshold (Cioffi et al., 2021). The gradual rise in RBP4 levels in pups was mirrored by that of transthyretin (Figure S3). This rise in RBP4 may accommodate or explain the increasing concentration of retinol in phocid seal milk as lactation proceeds (Simms and Ross, 2000; Debier et al., 2012), where it is then stored in blubber and liver (Mos and Ross, 2002), presumably to support developmental needs during both lactation and the post-weaning fast.
Intriguingly, RBP4 is now also recognised as an adipokine with several roles in metabolism including insulin regulation (Aigner and Datz, 2011; Nankam and Blueher, 2021; Flores-Cortez et al., 2022; Ji et al., 2022). It is secreted by the liver and adipocytes such that the increase in serum RBP4 in pups with time could be directly due to the increase in blubber adipocyte mass, and it may play other important roles in controlling the metabolism in pups as they accumulate extensive body stores in preparation for weaning.
Concluding remarks
Phocid seals exhibit a highly specialized lactation strategy in the quantity and rapidity with which they transfer lipid stores from mother to offspring. We asked in what ways the near inverse route of lipid flux (from blubber via blood to mammary glands in mothers/intestines via blood to blubber in pups) is reflected in the balance of lipid carrier proteins in their respective sera.
We found dramatic differences along with progressive changes in pups until weaning. Consistent with highly active absorption of fat from the intestines of pups, and its mass export from the intestines to the circulation of pups, the apolipoproteins that were persistently elevated in their sera were those associated with chylomicrons, and were at low levels in their fasting mothers. This prompts a question for future studies - how do the protein profiles in pups change when their fat-rich food supply abruptly terminates, and they enter and endure a post-weaning fast. Is there a full conversion to profiles similar to their fasting mothers as pups consume rather than build their blubber stores (Rosen and Hindle, 2016; Secor and Carey, 2016)?
The lipid carrier protein profiles we describe should be indicative of the state of the gut - highly active in the pups, quiescent in the mothers. Intestinal tissue mass in mothers may regress during lactation, and that may then apply also to pups during the post-weaning fast, perhaps alongside changes in their gut microbiomes. So, would the apolipoproteins they produce abruptly change upon weaning? For instance, do the intestine and chylomicron-specific ApoB-48 and ApoA-IV disappear from circulation in fasting pups, and the only form of ApoB-48/100 to persist is ApoB-100?
The increasing and continued presence of lipophilic pollutants such as polychlorinated biphenyls (PCBs) in polar mammals including seals is increasingly concerning (Debier et al., 2003a; Debier et al., 2003b; Wolkers et al., 2004; Mos et al., 2007; Vanden Berghe et al., 2010; Debier et al., 2012; Vanden Berghe et al., 2013). These toxins partition into adipocyte stores and are mobilised to lethal or hormone-disruptive effect under starvation conditions. Such pollutants that accumulate in blubber stores of mother seals may be mobilised into their blood, transferred via their fat-rich milk to pups, accumulate in their blubber, then released during post-weaning fast. The proteins considered here, the apolipoproteins and the lipidic particles they build, are likely the main conduits of the transfer of a potentially lethal legacy from milk to the blubber stores of seal pups.
While our focus here is on the lipid transporting activities of the apolipoproteins, they and their receptors on cells ranging from adipocytes to brain cells are involved in immune defence or as conduits for entry of viruses into cells (Clark et al., 2022). Isoforms of ApoE, for instance, are inhibitors of C1q, which is an early component of the classical pathway of complement, but not of the alternative or lectin pathways (Yin et al., 2019). C1q is activated by antigen:antibody complexes [though immunoglobulin levels are unusually low in seal pups (King et al., 1994; Mcgill et al., 2022)], or by C-reactive protein [associated with inflammation and also at low relative levels in grey seal pups (Mcgill et al., 2022)], to trigger cell lysis or opsonisation of pathogens, or initiate inflammatory reactions (Parham, 2021). It could therefore be that the elevated levels of ApoE in pups act to control inappropriate activation of C1q, which would align with our previous finding that complement and certain proteins of the innate immune system are of reduced capacity in grey seal pups (Mcgill et al., 2022). ApoA-1 is similarly involved in aspects of innate immunity, being involved in the control of inflammatory reactions (Yao et al., 2016); it binds bacterial lipopolysaccharide (Ma et al., 2004; Li et al., 2008), and, in humans, it is involved in the killing of trypanosomes (Harrington et al., 2009). In contrast to the activity of apolipoproteins in immune defence is the role of their cell surface receptors in infection - VLDL and ApoE, for instance, are known to facilitate cellular infections by alphaviruses (Clark et al., 2022).
The remarkable differences between the apolipoprotein profiles of mother and pup seals, and their time courses from birth to weaning, may not only be relevant to understanding the specialized lactation strategy of phocids, but could also have implications for the transfer of pollutants to pups, and their susceptibility to infections. Cessation of maternal feeding in advance of and during lactation is unusual amongst mammals, phocid seals, some cetaceans, and hibernating bears being notable exceptions (Crocker and Mcdonald, 2016). Although the brevity of lactations in phocids remains unique. It is tempting to consider that the changing pattern of lipid transporter protein profiles we found in true seals may also apply to these other groups of marine mammals, albeit with different time scales.
Data availability statement
The datasets presented in this study can be found in online repositories. The names of the repository/repositories and accession number(s) can be found in the article/Supplementary Material.
Ethics statement
Work involving animals in this study was licensed under the UK Home Office project 60/4009 or preceding versions and conformed to the UK Animals (Scientific Procedures) Act, 1986. Research was approved by the University of St Andrews Animal Welfare and Ethics Committee.
Author contributions
MK and PP conceived, designed and coordinated the study. PP organised collection of samples and was responsible for fieldwork. MK carried out the protein gel electrophoresis and processed samples for submission to proteomics. SM performed the TMT proteomics analysis and genome searches with advice from RJSB. MK and SM analysed the data. MK carried out statistical analysis and created the figures. MK wrote the paper with edits by PP. PP acquired funding and fieldwork permits through SMRU. All authors contributed amendments to drafts of the paper and approved the final version. All authors contributed to the article and approved the submitted version.
Funding
The work was funded from core support given to the Sea Mammal Research Unit, Scottish Oceans Institute, from the Natural Environmental Research Council (United Kingdom).
Acknowledgments
We are grateful to all involved in fieldwork, particularly Simon Moss. Scottish National Heritage granted permits for working on the Isle of May. Thanks also go to Monika Mihm, Neil Evans and David Watson for advice.
Conflict of interest
The authors declare that the research was conducted in the absence of any commercial or financial relationships that could be construed as a potential conflict of interest.
Publisher’s note
All claims expressed in this article are solely those of the authors and do not necessarily represent those of their affiliated organizations, or those of the publisher, the editors and the reviewers. Any product that may be evaluated in this article, or claim that may be made by its manufacturer, is not guaranteed or endorsed by the publisher.
Supplementary material
The Supplementary Material for this article can be found online at: https://www.frontiersin.org/articles/10.3389/fmars.2022.944214/full#supplementary-material
References
Aigner E., Datz C. (2011). Retinol-binding protein 4 as an adipokine. Adipokines. Boca Raton, FL, USA: CRC Press - Taylor and Francis. p. 110–118.
Altamirano A., Naschberger A., Fuemrohr B. G., Saldova R., Struwe W. B., Jennings P. M., et al. (2018). Expression, purification, and biochemical characterization of human afamin. J. Proteome Res. 17, 1269–1277. doi: 10.1021/acs.jproteome.7b00867
Bakker J., Baum M. J. (2008). Role for estradiol in female-typical brain and behavioral sexual differentiation. Front. Neuroendocrinol. 29, 1–16. doi: 10.1016/j.yfrne.2007.06.001
Bennett K. A., Mcconnell B. J., Moss S. E. W., Speakman J. R., Pomeroy P. P., Fedak M. A. (2010). Effects of age and body mass on development of diving capabilities of Gray seal pups: Costs and benefits of the postweaning fast. Physiol. Biochem. Zoology 83, 911–923. doi: 10.1086/656925
Berta A., Sumich J. L., Kovacs K. M. (2015). ““Reproductive structures, strategies, and patterns,”,” in Marine mammals - evolutionary biology, 3rd ed. Eds. Berta A., Sumich J. L., Kovacs K. M. (Amsterdam, Boston, New York: Academic Press), 465–532.
Blanchard V., Francis G. A. (2021). ““High-density lipoproteins: Metabolism and protective roles,”,” in Biochemistry of Lipids, Lipoproteins and Membranes. Eds. Ridgway N. D., Mcleod R. S. (Amsterdam, Netherlands: Elsevier), 487–514.
Bouillon R., Schuit F., Antonio L., Rastinejad F. (2020). Vitamin d binding protein: A historic overview. Front. Endocrinol. 10. doi: 10.3389/fendo.2019.00910
Burns J. M., Castellini M. A. (1996). Physiological and behavioral determinants of the aerobic dive limit in weddell seal (Leptonychotes weddellii) pups. J. Comp. Physiol. B-Biochemi. Syst. Environ. Physiol. 166, 473–483. doi: 10.1007/BF02338290
Carter M. I. D., Russell D. J. F., Embling C. B., Blight C. J., Thompson D., Hosegood P. J., et al. (2017). Intrinsic and extrinsic factors drive ontogeny of early-life at-sea behaviour in a marine top predator. Sci. Rep. 7.doi: 10.1038/s41598-017-15859-8
Chiba T., Nakazawa T., Yui K., Kaneko E., Shimokado K. (2003). VLDL induces adipocyte differentiation in ApoE-dependent manner. Arterioscler. Thromb. Vasc. Biol. 23, 1423–1429. doi: 10.1161/01.ATV.0000085040.58340.36
Cioffi C. L., Raja A., Muthuraman P., Jayaraman A., Jayakumar S., Varadi A., et al. (2021). Identification of transthyretin tetramer kinetic stabilizers that are capable of inhibiting the retinol-dependent retinol binding protein 4-transthyretin interaction: Potential novel therapeutics for macular degeneration, transthyretin amyloidosis, and their common age-related comorbidities. J. Medicinal Chem. 64, 9010–9041. doi: 10.1021/acs.jmedchem.1c00099
Clark L. E., Clark S. A., Lin C., Liu J., Coscia A., Nabel K. G., et al. (2022). VLDLR and ApoER2 are receptors for multiple alphaviruses. Nature 602, 479–480. doi: 10.1038/s41586-021-04326-0
Crocker D. E., Mcdonald B. I. (2016). ““Post-partum,”,” in Marine mammal physiology: Requisites for ocean living. Eds. Castellini M. A., Mellish J.-A.(Boca Raton, London, New York: CRC Press - Taylor & Francis), 219–241.
Debier C., Crocker D. E., Houser D. S., Vanden Berghe M., Fowler M., Mignolet E., et al. (2012). Differential changes of fat-soluble vitamins and pollutants during lactation in northern elephant seal mother-pup pairs. Comp. Biochem. Physiol. a-Mol. Integr. Physiol. 162, 323–330. doi: 10.1016/j.cbpa.2012.04.001
Debier C., Pomeroy P. P., Dupont C., Joiris C., Comblin V., Le Boulenge E., et al. (2003a). Dynamics of PCB transfer from mother to pup during lactation in UK grey seals halichoerus grypus: differences in PCB profile between compartments of transfer and changes during the lactation period. Mar. Ecol. Prog. Ser. 247, 249–256. doi: 10.3354/meps247249
Debier C., Pomeroy P. P., Dupont C., Joiris C., Comblin V., Le Boulenge E., et al. (2003b). Quantitative dynamics of PCB transfer from mother to pup during lactation in UK grey seals halichoerus grypus. Mar. Ecol. Prog. Ser. 247, 237–248. doi: 10.3354/meps247237
Dieplinger H., Dieplinger B. (2015). Afamin - a pleiotropic glycoprotein involved in various disease states. Clinica Chimica Acta 446, 105–110. doi: 10.1016/j.cca.2015.04.010
Fisher E. A., Mcleod R. S. (2021). ““Assembly and secretion of triacylglycerol-rich lipoproteins,”,” in Biochemistry of lipids, lipoproteins and membranes, 7th ed. Eds. Ridgway N. D., Mcleod R. S. (Amsterdam, The Netherlands:Elsevier), 515–546.
Flores-Cortez Y. A., Barragan-Bonilla M. I., Mendoza-Bello J. M., Gonzalez-Calixto C., Flores-Alfaro E., Espinoza-Rojo M. (2022). Interplay of retinol binding protein 4 with obesity and associated chronic alterations. Mol. Med. Rep. 26. doi: 10.3892/mmr.2022.12760
Francis G. A. (2016). ““High-density lipoproteins metabolism and protective roles against atherosclerosis,”,” in Biochemistry of lipids, lipoproteins and membranes, 6th ed. Eds. Ridgway N. D., Mcleod R. S. (Amsterdam: Elsevier B.V), 437–457. doi: 10.1016/C2013-0-18457-7
Glowska-Ciemny J., Pankiewicz J., Malewski Z., Von Kaisenberg C., Kocylowski R. (2022). Alpha-fetoprotein (AFP)-new aspects of a well-known marker in perinatology. Ginekol. Polska 93, 70–75. doi: 10.5603/GP.a2021.0226
Harrington J. M., Howell S., Hajduk S. L. (2009). Membrane permeabilization by trypanosome lytic factor, a cytolytic human high density lipoprotein. J. Biol. Chem. 284, 13505–13512. doi: 10.1074/jbc.M900151200
Ji Y., Song J., Su T., Gu X. (2022). Adipokine retinol binding protein 4 and cardiovascular diseases. Front. Physiol. 13. doi: 10.3389/fphys.2022.856298
Kew R. R. (2019). The vitamin d binding protein and inflammatory injury: A mediator or sentinel of tissue damage? Front. Endocrinol. 10. doi: 10.3389/fendo.2019.00470
Khudyakov J. I., Holser R. R., Vierra C. A., Ly S. T., Niel T. K., Hasan B. M., et al. (2022). Changes in apolipoprotein abundance dominate proteome responses to prolonged fasting in elephant seals. J. Exp. Biol. 225. doi: 10.1242/jeb.243572
King D. P., Lowe K. A., Hay A. W. M., Evans S. W. (1994). Identification, characterization, and measurement of immunoglobulin concentrations in grey (Haliocherus grypus) and common (Phoca vitulina) seals. Dev. Comp. Immunol. 18, 433–442. doi: 10.1016/0145-305X(94)90008-6
Kuhbandner K., Herz J., Pohlkamp T. (2021). ““Lipoprotein receptors,”,” in Biochemistry of lipids, lipoproteins and membranes, 7th ed. Eds. Ridgway N. D., Mcleod R. S. (Masterdam: Elsevier), 583–622. doi: 10.1016/C2020-0-00089-7
Li Y., Dong J.-B., Wu M.-P. (2008). Human ApoA-I overexpression diminishes LPS-induced systemic inflammation and multiple organ damage in mice. Eur. J. Pharmacol. 590, 417–422. doi: 10.1016/j.ejphar.2008.06.047
Lowe A. D., Bawazeer S., Watson D. G., Mcgill S., Burchmore R. J. S., Pomeroy P. P., et al. (2017). Rapid changes in Atlantic grey seal milk from birth to weaning – immune factors and indicators of metabolic strain. Sci. Rep. 7, 16093. doi: 10.1038/s41598-017-16187-7
Ma J., Liao X. L., Lou B., Wu M. P. (2004). Role of apolipoprotein a-1 in protecting against endotoxin toxicity. Acta Biochim. Et Biophys. Sin. 36, 419–424. doi: 10.1093/abbs/36.6.419
Marquez M. E. I., Carlini A. R., Baroni A. V., De Ferrer P., Slobodianik N. H. (2007). Shifts in specific serum protein concentrations: apolipoprotein-b and albumin in the southern elephant seal during the breeding and molting periods in Antarctica. Polar Biol. 30, 1253–1263. doi: 10.1007/s00300-007-0286-y
Mcgill S., Burchmore R. J. S., Pomeroy P. P., Kennedy M. W. (2022). Is a little enough? paucity of immune proteins in serum of precocial neonates of a marine carnivoran–the Atlantic grey seal. Front. Ecol. Evol. 9. doi: 10.3389/fevo.2021.802510
Mehta A., Shapiro M. D. (2021). Apolipoproteins in vascular biology and atherosclerotic disease. Nat. Rev. Cardiol. 19, 168–179. doi: 10.1038/s41569-021-00613-5
Mendivil C. O., Zheng C., Furtado J., Lel J., Sacks F. M. (2010). Metabolism of very-Low-Density lipoprotein and low-density lipoprotein containing apolipoprotein c-III and not other small apolipoproteins. Arterioscler. Thromb. Vasc. Biol. 30, 239–U210. doi: 10.1161/ATVBAHA.109.197830
Mos L., Ross P. S. (2002). Vitamin a physiology in the precocious harbour seal (Phoca vitulina): a tissue-based biomarker approach. Can. J. Zoology 80, 1511–1519. doi: 10.1139/z02-152
Mos L., Tabuchi M., Dangerfield N., Jeffries S. J., Koop B. F., Ross P. S. (2007). Contaminant-associated disruption of vitamin a and its receptor (retinoic acid receptor alpha) in free-ranging harbour seals (Phoca vitulina). Aquat. Toxicol. 81, 319–328. doi: 10.1016/j.aquatox.2006.12.017
Nankam P., Blueher M. (2021). Retinol-binding protein 4 in obesity and metabolic dysfunctions. Mol. Cell. Endocrinol. 531. doi: 10.1016/j.mce.2021.111312
Noren S. R., Iverson S. J., Boness D. J. (2005). Development of the blood and muscle oxygen stores in gray seals (Halichoerus grypus): Implications for juvenile diving capacity and the necessity of a terrestrial postweaning fast. Physiol. Biochem. Zoology 78, 482–490. doi: 10.1086/430228
Peters T. Jr. (1995). All about albumin: Biochemistry, genetics, and medical applications. Amsterdam, The Netherlands: Elsevier. doi: 10.1016/B978-0-12-552110-9.X5000-4.
Pomeroy P. P., Fedak M. A., Rothery P., Anderson S. (1999). Consequences of maternal size for reproductive expenditure and pupping success of grey seals at north Rona, Scotland. J. Anim. Ecol. 68, 235–253. doi: 10.1046/j.1365-2656.1999.00281.x
Pomeroy P. P., Green N., Hall A. J., Walton M., Jones K., Harwood J. (1996). Congener-specific exposure of grey seal (Halichoerus grypus) pups to chlorinated biphenyls during lactation. Can. J. Fish. Aquat. Sci. 53, 1526–1534. doi: 10.1139/f96-087
Qu J., Ko C.-W., Tso P., Bhargava A. (2019). Apolipoprotein a-IV: A multifunctional protein involved in protection against atherosclerosis and diabetes. Cells 8. doi: 10.3390/cells8040319
Rhea E. M., Banks W. A. (2021). Interactions of lipids, lipoproteins, and apolipoproteins with the blood-brain barrier. Pharm. Res. 38, 1469–1475. doi: 10.1007/s11095-021-03098-6
Rosen D., Hindle A. (2016). ““Fasting,”,” in Marine Mammal Physiology: Requisites for Ocean Living. Eds. Castellini M. A., Mellish J. A.,Boca Raton, FL, USA: CRC Press - Taylor and Francis 169–191.
Schulz T. M., Bowen W. D. (2004). Pinniped lactation strategies: Evaluation of data on maternal and offspring life history traits. Mar. Mammal Sci. 20, 86–114. doi: 10.1111/j.1748-7692.2004.tb01142.x
Secor S. M., Carey H. V. (2016). Integrative physiology of fasting. Compr. Physiol. 6, 773–825. doi: 10.1002/cphy.c150013
Sesorova I. S., Dimov I. D., Kashin A. D., Sesorov V. V., Karelina N. R., Zdorikova M. A., et al. (2021). Cellular and sub-cellular mechanisms of lipid transport from gut to lymph. Tissue Cell 72. doi: 10.1016/j.tice.2021.101529
Sesorova I. S., Karelina N. R., Kazakova T. E., Parashuraman S., Zdorikova M. A., Dimov I. D., et al. (2020). Structure of the enterocyte transcytosis compartments during lipid absorption. Histochem. Cell Biol. 153, 413–429. doi: 10.1007/s00418-020-01851-3
Simms W., Ross P. S. (2000). Developmental changes in circulatory vitamin a (retinol) and its transport proteins in free-ranging harbour seal (Phoca vitulina) pups. Can. J. Zoology 78, 1862–1868. doi: 10.1139/z00-129
Simons P. I. H. G., Valkenburg O., Stehouwer C. D. A., Brouwers M. C. G. J. (2021). Sex hormone-binding globulin: biomarker and hepatokine? Trends Endocrinol. Metab. 32, 544–553. doi: 10.1016/j.tem.2021.05.002
Smout S., King R., Pomeroy P. (2020). Environment-sensitive mass changes influence breeding frequency in a capital breeding marine top predator. J. Anim. Ecol. 89, 384–396. doi: 10.1111/1365-2656.13128
Steinhoff J. S., Lass A., Schupp M. (2022). Retinoid homeostasis and beyond: How retinol binding protein 4 contributes to health and disease. Nutrients 14. doi: 10.3390/nu14061236
Struntz D. J., Mclellan W. A., Dillaman R. M., Blum J. E., Kucklick J. R., Pabst D. A. (2004). Blubber development in bottlenose dolphins (Tursiops truncatus). J. Morphol. 259, 7–20. doi: 10.1002/jmor.10154
Tift M. S., Houser D. S., Crocker D. E. (2011). High-density lipoprotein remains elevated despite reductions in total cholesterol in fasting adult male elephant seals (Mirounga angustirostris). Comp. Biochem. Physiol. B-Biochem. Mol. Biol. 159, 214–219. doi: 10.1016/j.cbpb.2011.04.005
Tramontana A., Dieplinger B., Stangl G., Hafner E., Dieplinger H. (2018). First trimester serum afamin concentrations are associated with the development of pre-eclampsia and gestational diabetes mellitus in pregnant women. Clinica Chimica Acta 476, 160–166. doi: 10.1016/j.cca.2017.11.031
Vanden Berghe M., Mat A., Arriola A., Polain S., Stekke V., Thome J.-P., et al. (2010). Relationships between vitamin a and PCBs in grey seal mothers and pups during lactation. Environ. pollut. 158, 1570–1575. doi: 10.1016/j.envpol.2009.12.012
Vanden Berghe M., Weijs L., Habran S., Das K., Bugli C., Pillet S., et al. (2013). Effects of polychlorobiphenyls, polybromodiphenylethers, organochlorine pesticides and their metabolites on vitamin a status in lactating grey seals. Environ. Res. 120, 18–26. doi: 10.1016/j.envres.2012.09.004
Van Valkenburgh J., Meuret C., Martinez A. E., Kodancha V., Solomon V., Chen K., et al. (2021). Understanding the exchange of systemic HDL particles into the brain and vascular cells has diagnostic and therapeutic implications for neurodegenerative diseases. Front. Physiol. 12. doi: 10.3389/fphys.2021.700847
Wasan K. M., Brocks D. R., Lee S. D., Sachs-Barrable K., Thornton S. J. (2008). Impact of lipoproteins on the biological activity and disposition of hydrophobic drugs: implications for drug discovery. Nat. Rev. Drug Discovery 7, 84–99. doi: 10.1038/nrd2353
Watson D. G., Pomeroy P. P., Al-Tannak N. F., Kennedy M. W. (2020). Stockpiling by pups and self-sacrifice by their fasting mothers observed in birth to weaning serum metabolomes of Atlantic grey seals. Sci. Rep. 10, 7465–7465. doi: 10.1038/s41598-020-64488-1
Watson D. G., Pomeroy P. P., Kennedy M. W. (2021). Atlantic Grey seal milk shows continuous changes in key metabolites and indicators of metabolic transition in pups from birth to weaning. Front. Mar. Sci. 7. doi: 10.3389/fmars.2020.596904
White P., Cooke N. (2000). The multifunctional properties and characteristics of vitamin d-binding protein. Trends Endocrinol. Metab. 11, 320–327. doi: 10.1016/S1043-2760(00)00317-9
Wilton D. C. (1990). The fatty-acid analog 11-(dansylamino)undecanoic acid is a fluorescent-probe for the bilirubin-binding sites of albumin and not for the high-affinity fatty acid-binding sites. Biochem. J. 270, 163–166. doi: 10.1042/bj2700163
Windmueller H. G., Wu A. L. (1981). Biosynthesis of plasma apolipoproteins by rat small-intestine without dietary or biliary fat. J. Biol. Chem. 256, 3012–3016. doi: 10.1016/S0021-9258(19)69716-4
Wisniewski J. R., Zougman A., Nagaraj N., Mann M. (2009). Universal sample preparation method for proteome analysis. Nat. Methods 6, 359–U360. doi: 10.1038/nmeth.1322
Wolkers H., Lydersen C., Kovacs K. M. (2004). Accumulation and lactational transfer of PCBs and pesticides in harbor seals (Phoca vitulina) from Svalbard, Norway. Sci. Tot. Environ. 319, 137–146. doi: 10.1016/S0048-9697(03)00449-2
Wu A. L., Windmueller H. G. (1979). Relative contributions by liver and intestine to individual plasma apolipoproteins in the rat. J. Biol. Chem. 254, 7316–7322. doi: 10.1016/S0021-9258(18)50321-5
Yao X., Gordon E. M., Figueroa D. M., Barochia A. V., Levine S. J. (2016). Emerging roles of apolipoprotein e and apolipoprotein a-I in the pathogenesis and treatment of lung disease. Am. J. Respir. Cell Mol. Biol. 55, 159–169. doi: 10.1165/rcmb.2016-0060TR
Yin C., Ackermann S., Ma Z., Mohanta S. K., Zhang C., Li Y., et al. (2019). ApoE attenuates unresolvable inflammation by complex formation with activated C1q. Nat. Med. 25, 496–506. doi: 10.1038/s41591-018-0336-8
Keywords: Atlantic grey seals, Halichoerus grypus, apolipoproteins, hormone binding proteins, vitamin-binding proteins, blubber, lactation, serum
Citation: McGill S, Burchmore RJS, Pomeroy PP and Kennedy MW (2022) Mirror image serum lipid carrier protein profiles in pup and lactating mother Atlantic grey seals reflect contrasting resource mobilisation challenges. Front. Mar. Sci. 9:944214. doi: 10.3389/fmars.2022.944214
Received: 14 May 2022; Accepted: 22 August 2022;
Published: 16 September 2022.
Edited by:
Davina Derous, University of Aberdeen, United KingdomReviewed by:
Jane Khudyakov, The University of the Pacific, United StatesMiwa Suzuki, Nihon University, Japan
Copyright © 2022 McGill, Burchmore, Pomeroy and Kennedy. This is an open-access article distributed under the terms of the Creative Commons Attribution License (CC BY). The use, distribution or reproduction in other forums is permitted, provided the original author(s) and the copyright owner(s) are credited and that the original publication in this journal is cited, in accordance with accepted academic practice. No use, distribution or reproduction is permitted which does not comply with these terms.
*Correspondence: Patrick P. Pomeroy, cHA2QHN0LWFuZHJld3MuYWMudWs=; Malcolm W. Kennedy, bWFsY29sbS5rZW5uZWR5QGdsYXNnb3cuYWMudWs=