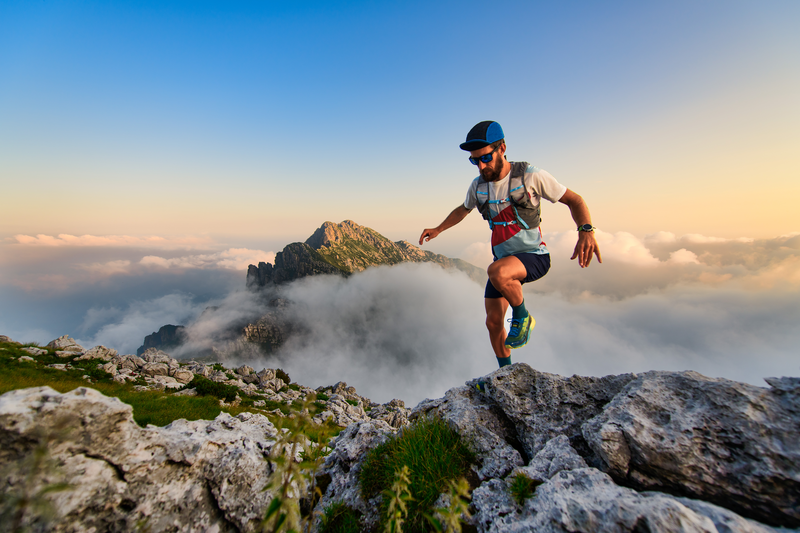
94% of researchers rate our articles as excellent or good
Learn more about the work of our research integrity team to safeguard the quality of each article we publish.
Find out more
ORIGINAL RESEARCH article
Front. Mar. Sci. , 18 November 2022
Sec. Marine Affairs and Policy
Volume 9 - 2022 | https://doi.org/10.3389/fmars.2022.943552
Gear entanglement and vessel collisions are the major known causes of injuries to the critically endangered North Atlantic right whale (Eubalaena glacialis) and other marine animals. Whale entanglements often occur in endlines (the fixed vertical lines between fishing traps and surface buoys) and removal of these lines in “on-demand” or “ropeless” fishing gear has been presented as a potential solution. These fishing systems use acoustic and timed releases to locate and retrieve a submerged trap, endline, and buoy (or lift bag or spool) to the surface. On-demand fishing gear commercially in use costs up to $8,000 for a deck unit and up to $4,000 per acoustic release. Developers expect on-demand fishing component costs to drop as production ramps up. The correlation between production cost or labor hours per unit and cumulative production have been described as ‘experience curves’ or ‘learning curves.’ The learning model is the most common approach to projecting production costs in electronics and other high-tech industries, with the log-linear model based on the Wright learning curve the most frequently used modelling approach. Wright’s equation is used to calculate on-demand fishing gear costs for northeastern U.S. commercial fishing vessels operating in federal lobster management areas. These vessels represent approximately 70% of entanglement risk, estimated by the National Marine Fisheries Service. The use of on-demand gear would therefore initially be most effective in reducing the risk of whale entanglement (and mortality) in commercial fishing areas in these waters. It is estimated that the cost of on-demand gear for the 1,494 vessels operating in federal waters would total $58.1 million, with an average of $38,899 per vessel if releases were used on all endlines. If vessels fishing 5 or more traps per trawl use a release on 1 endlines per trawl, the total cost is estimated at $38.2 million, with an average of $25,552 per vessel. The initial cost of on-demand fishing gear may be an obstacle to widespread adoption. Funding and loan programs are available and there is precedent for government and community assistance to workers in industries affected by resource management regulations.
The North Atlantic right whale has been listed as endangered under the Endangered Species Act since 1970 and designated as critically endangered by the International Union for Conservation of Nature in 2020 (Cooke, 2020). As of 2021, there were an estimated 336 right whales in existence, of which fewer than 100 were females of breeding age (Pettis et al., 2022). Entanglement in fishing gear and collisions with vessels are the major known causes of injuries to North Atlantic right whales (Moore et al., 2021). The static vertical lines used in fixed-gear fisheries, including trap/pot and gillnet gear, connect bottom gear to a surface buoy and can entangle the whales (as well as other large ocean animals), leading to injuries or death (Johnson et al., 2005). Between 1980 and 2017, evidence of fishing gear entanglement was documented for 86% of North Atlantic right whales, with entanglement deaths increasing in recent decades (Moore et al., 2021). NMFS has committed to implement measures designed to reduce entanglement risk to right whales in federal waters in four phases, with periodic evaluation of results (NOAA, 2021a). In this Conservation Plan, the risk of serious injury and mortality of right whales in federal fisheries is to be reduced to a level to ensure the likelihood of survival and recovery of the species by 2030 (NOAA, 2021a).
There have been increasing efforts over the past two decades to mitigate whale entanglement in lobster and crab trap/pot gear (NOAA, 2022a). Approaches including seasonal closures, weak links (in and between lines and buoys), sinking groundlines (lines between traps), and more traps per trawl may have helped - but have not solved - the problem of lethal entanglements (Pace et al., 2014; Baumgartner et al., 2019). Because of the increasing rate of serious entanglements (Knowlton et al., 2012; Henry et al., 2020; Knowlton et al., 2022) which often occur in endlines (Johnson et al., 2005; see entanglement reports at NOAA, 2022a), removal of these fixed lines by using on-demand fishing gear has been discussed as a potential solution (Baumgartner et al., 2019; Myers et al., 2019). The use of on-demand gear would provide an option to continue commercial lobster and crab fisheries while eliminating the threat of whale entanglement.
The 2021 amendment to regulations implementing the Atlantic Large Whale Take Reduction Plan (to reduce whale entanglement in northeast commercial lobster and crab trap/pot fisheries) allows for on-demand fishing under an Exempted Fishing Permit issued by the National Oceanic and Atmospheric Administration's (NOAA's) Greater Atlantic Regional Fisheries Office (Taking of Marine…2021). This permit authorizes a fishing vessel to conduct fishing activities that would otherwise be prohibited under regulation (NOAA, 2022b). The rule also modifies gear marking, gear configurations, and existing area closures, and adds new closed areas.
On-demand fishing gear relies on additional technology to detect the location of gear submerged on the sea floor and make it detectable by other vessels in addition to the owners. Also required are either a timed release or acoustic release for each trap (or trawl) and, for acoustic releases, a deck unit with an acoustic transducer on board the vessel. The transducer enables the fisher to trigger a release mechanism on the equipment located on the sea floor to signal the trap (or trawl) to the surface. There are 3 basic types of on-demand (or “ropeless”) fishing technologies in development: 1 with a bottom-stowed endline contained within a trap, 1 with an attached lift bag, and 1 with a buoyant spool (Figure 1). While these components are being refined specifically for on-demand fishing, underwater acoustic technology has previously been applied to other marine purposes (Hydro International, 2009; PACE, 2009; Myers et al., 2019; Ropeless Systems Inc., 2020).
Figure 1 Traditional and on-demand, or ropeless, fishing systems Source: NOAA, 2022c.
The bottom-stowed endline approach uses an endline and buoy stowed with the trap. When triggered by an acoustic signal, the line is released and brought to the water’s surface with a buoy or other flotation device. The lift bag approach has a compressed air canister linked to a flotation device (e.g., an inflatable bag) attached to the trap. The compressed air inflates the flotation device when it is remotely triggered with an acoustic mechanism. Once inflated, the trap (with air canister and device) floats to the surface. The spool technology is similar; stowed on the sea floor with the trap, it unwinds as it floats to the surface when signaled.
Using gear tracking applications (e.g., EdgeTech’s Trap Tracker), fishers can manually enter the location where they set their traps, then access this location at the desired retrieval time. Some systems can be set to appear at the surface on a specific date and time (e.g., Fiobuoy TD100 & TD200; Fiomarine Industries, 2022),
An on-demand system includes, at minimum, a deck unit with a transducer and at least 1 release per trap or trawl. Two on-demand fishing systems are currently used in commercial fisheries. One, from Desert Star Systems, LLC, has been used commercially since 2012. After testing in 2010, it was introduced in the New South Wales, Australia, rock lobster commercial fishery to eliminate the loss and theft of traps (Marino, 2011; Desert Star Systems, 2021). In South Africa, several octopus fishers have been using Desert Star’s on-demand gear since 2020 (FINSA, 2020); octopus fishing permit conditions were changed in 2019 to require acoustic or time-release buoys in response to whale entanglements in octopus fishing gear (Oirere, 2019). EdgeTech’s 5112 Ropeless Fishing System has been in commercial production since 2019. It is being used by crab fishers in Canada and being tested for on-demand fishing in both the United States and Canada (Rob Morris, emails, 2021).
Other on-demand fishing gear is being developed and tested by engineers and fishers (Baumgartner et al., 2019; Ropeless Consortium, 2020). NOAA’s Northeast Fisheries Science Center has partnered with gear engineers and manufacturers, fishers, researchers, and environmental organizations to fund the purchase and trial of on-demand fishing gear (NOAA, 2021b). As of February 2022, the “gear library” had 164 units from 6 developers available for testing both onshore and offshore (Gahm et al., 2022). Federal and private grant funding has contributed to the development of some systems. (e.g., NOAA’s Small Business Innovation Research Program and Bycatch Reduction Engineering Programs).
The use of acoustic on-demand fishing systems can provide operational advantages compared to traditional methods. Some (e.g., EdgeTech’s 5112 Ropeless Fishing System Trap Tracker App) collect real-time data from the traps, such as water temperature, and store user-provided trap count and catch quantities (Morris, 2020). This allows fishers to schedule trap retrieval when optimal, saving unnecessary trips and time at sea, and provides more reliable access to gear in high currents. If fishing with on-demand gear is allowed in restricted areas, fishers with these systems will be able to fish in these areas and generate income when otherwise they would not be allowed access.
Expanded use of on-demand fishing gear depends on the ability of fishers to identify gear deployed by others on the sea floor, as well as their own, to avoid setting traps in the same place and trawlers from dragging traps. Technology that shows the location of traps deployed using on-demand systems to lobster fishers using different systems, gillnet and other fishers, and regulators is being developed by EdgeTech, Ropeless Systems, SMELTS, and others (Morris, 2020; Riels, 2020; Vincent and Capotosto, 2020). Research comparing different methods is ongoing, with a goal of choosing a gear location method that meets the requirements of fishers and other stakeholders and developing manufacturing specifications (Baumgartner et al., 2021). NOAA Fisheries is testing the feasibility of the Allen Institute for Artificial Intelligence’s EarthRanger platform to solve the problem of geolocating underwater deployments of on-demand fishing gear (NOAA, 2022c).
An understanding of the potential cost of on-demand gear in the future, after production ramps up, is one important factor to assist fisheries managers, industry participants, and other stakeholders to make decisions about its use. This paper uses a learning model approach, which projects the correlation between production cost per unit and cumulative production, to estimate on-demand fishing gear costs for permitted vessels operating in federal lobster management areas in the northeastern U.S.
The on-demand fishing components commercially available cost up to $8,000 for the deck unit and up to $4,000 for one acoustic release (Rob Morris, email to author, July 9, 2021; Desert Star Systems, LLC, 2021). (Other gear not yet in commercial use may be more costly.) Developers expect on-demand fishing gear costs to drop as production increases (Ropeless Consortium, 2020). The economic concept of a reduction in production cost per unit with increased total output is referred to as “economies of scale.” Graphic representations of this correlation between production cost or labor hours per unit and cumulative production have been described as “experience curves,” “performance curves,” “improvement curves,” “learning curves,” and “progress functions” (Delionback, 1995; Brennan, 2020). Some authors distinguish between the terms (e.g., Dutton et al., 1984, in a review of 162 studies) while others do not. The Boston Consulting Group asserts that “learning curve” relates only to labor and production inputs and “experience curve” to manufacturing and distribution costs (Henderson, 1968). In recent literature, the terms appear to be used interchangeably, with “learning curve” used more frequently.
Wright (1936) has been credited with the concept of the learning curve (Anzanello and Fogliatto, 2011; Lolli et al., 2016; Glock et al., 20191). He observed that the direct labor required to produce an airframe (the body of an aircraft, without the engine) declined as the cumulative production increased; this reduction followed a constant rate as the number of assembled airframes doubled (Wright, 1936). His mathematical model became known as the “80% learning curve” and was widely applied in the aeronautical industry of the time (Anzanello and Fogliatto, 2011). According to that rule, cumulative assembly costs are reduced, on average, by 20% (from 100% to 80%) as the number of units produced is doubled (Anzanello and Fogliatto, 2011).2 He also developed curves for purchased materials, raw materials, and a composite curve for a complete airframe, and observed that labor-intensive operations had a more rapid performance improvement than machine-intensive operations (Wright, 1936).
The work of the Boston Consulting Group in the late 1960s further bolstered the general applicability of (what they term as) “the experience curve.” They observed a reduction of 20% to 30% in real costs with each doubling of cumulative production experience for a diverse range of industries and products (Henderson, 1968; Brennan, 2020). The improvement of worker performance and cost reductions has since been studied in many industries, such as electronics, automotive, construction, software, energy, and chemical production (Anzanello and Fogliatto, 2011; Grosse et al., 2015; Glock et al., 2019).
The log-linear model based on the Wright learning curve is the most frequently used modelling approach (Lolli et al., 2016; Glock et al., 2019) and can be applied to cost or time reductions:
where yx is the cumulative average cost to produce the xth unit, y1 is the cost to produce the first unit, and b is the learning exponent, which is computed as b = -log(LR)/log(2) with LR being the learning rate. For example, an 80% learning curve has a b = 0.3219 (Glock et al., 2019).
Other log-linear models adopted are those of Crawford3 and de Jong, as well as the Plateau, the Stanford-B, and the S curve model (Glock et al., 2019). Exponential and hyperbolic specifications have also been developed; Grosse et al. (2015) performed a meta-analysis of empirical learning curve data to identify characteristics important for selecting the appropriate learning model for an analysis.
Actual production cost (or time) data may be used to develop a learning curve, and the slope/learning rate can then be determined (see, for example, Adler, 1990). Lacking that, an estimate of the cost to produce the first unit and a projected learning rate can be used to approximate a learning curve. For guidance, the effect of different proportions of handwork versus machining on the learning curve slope was quantified by Delionback (1995); Table 1):
● 75% hand assembly/25% machining – 80% rate
● 50% hand assembly/50% machining – 85% rate
● 25% hand assembly/75% machining – 95% rate
For mature technologies (e.g., conventional gasoline engines), the learning rate is (close to) 100% as costs are no longer projected to fall as production increases.
The learning model is the most common approach to projecting production costs in electronics as well as computers, telecommunications, aerospace, and other high-tech industries ( Delionback, 1995; Anzanello and Fogliotto, 2011; Glock et al., 2019). In a systematic literature review of leading journals focusing on production and operations management over a 58-year period (1960 to 2018), Glock et al. (2019) identified 457 articles employing a learning approach, several applying it to electronics manufacturing (no studies of acoustic technology were among these works). The log-linear model based on the Wright learning curve was the most frequently used modelling approach (Glock et al., 2019). Similarly, results of a meta-analysis of empirical learning curve data in 44 studies4 confirmed prior findings that the Wright learning curve is able to approximate empirically observed learning (production cost or time) (Grosse et al., 2015). Wright’s equation is therefore used to estimate cumulative average on-demand fishing system costs at various levels of production. The costs of on-demand gear currently on the market are used in this analysis. Assuming a conservative 95% learning rate for electronics manufacturing (per Table 1), for example, the nth unit and cumulative average costs for a $4,000 deck unit is shown in Table 2 for production of 100 to 4,000 units.
To demonstrate the concept, on-demand fishing component costs per vessel are estimated using Wright’s model and shown in Table 3 (for deck units) and Table 4 (for acoustic releases). The modeled decline in deck unit costs (initial cost $4,000) for production of 1,000 to 25,000 is illustrated in Figure 2, showing the greatest cost decline (46%) at about 4,000 units. A 95% learning rate is assumed as the conservative end of the 90% to 95% range for electronics manufacturing5 (Table 2). This indicates that each doubling of production could result in a 5% reduction in average price per unit.
Table 3 Example of cumulative average cost for on-demand fishing deck units, per vessel, with a 95% learning rate.
Table 4 Example of cumulative average cost of acoustic releases (initial cost of $1,000) per vessel for 100 vessels, with a 95% learning rate.
Figure 2 Cumulative average cost of deck unit with an initial cost of $4,000, applying a 95% learning rate and Wright’s model.
The total per vessel cost for an on-demand fishing system can be calculated by adding the cost of the deck unit and acoustic releases. For example, if a deck unit with an initial cost of $4,000 is adopted by 100 vessels, the cumulative average cost of the deck unit drops to $2,845 each (using Wright’s model and a 95% learning rate6). If each vessel uses 20 releases on average, a total of 2,000 releases (100 x 20) would be needed. With an initial unit cost of $1,000 (95% learning rate), the cumulative average cost per release would be $570 each if 2,000 were produced, and the cost of 20 releases (for 1 vessel) would be $11,400 (20 x $570). The calculation for cost per vessel would then be $2,845 + $11,400, totaling $14,245 per vessel. This can be illustrated for a range of average acoustic releases per vessel in Figure 3.
Figure 3 Example of total cost per vessel for 100 vessels adopting an on-demand fishing system (initial costs: $4,000 per deck unit and $1,000 per release) using Wright’s model with a 95% learning rate.
There are an estimated 7,854 vessels in lobster and Jonah crab (Cancer borealis) trap/pot fisheries in the Northeast region (Industrial Economics, 2020, NOAA, 2020). In most areas of the northeastern United States permitted vessels are authorized to use up to 800 traps per vessel (NOAA, 2021c). The number of endlines per vessel depends on the number of traps fished per trawl, which varies with vessel size, distance from shore, bottom type, and other factors (McCarron and Tetreault, 2012). Considering the continued critical decline in North Atlantic right whale populations caused, in large part, by entanglement and importance of the lobster and other trap/pot fisheries to the region, the use of on-demand gear would be most effective in reducing the risk of right whale entanglement (and mortality) in commercial fishing areas in and around habitat used by whales. Comparing the relationship between trap/pot gear (measured by endlines) and risk in federal vs. state waters, the NMFS estimates 20% of fishing gear occurs in federal waters and these lines represent approximately 70% of entanglement risk (with line strength as the proxy for entanglement risk; Burton Shank, email to author, April 7, 2022). This suggests that on-demand gear adoption by the 1,494 vessels operating in federal waters would provide the greatest reduction in entanglement risk initially. (Some of these vessels may also operate in state waters.)
For maximum risk reduction, the cost of adopting on-demand fishing practices is therefore calculated based on the estimated 1,494 trap/pot fishing vessels operating in federal waters in the northeastern United States and the number of associated endlines. These estimates are from NMFS’ Vertical Line Model developed by Industrial Economics7 (2020). The model provides estimates of the number and distribution of vessels and endlines by month; vessel and endline data for the month of the year with the highest number were used in this analysis. Vessels were categorized as nearshore or offshore based on the location of fishing activity (in part from vessel tracking reports) (NOAA, 2020).
It is estimated there are 1,005 trap/pot fishing vessels operating in federally-managed waters between 3 and 12 nautical miles from the coast of Maine, New Hampshire, Massachusetts, and Rhode Island (Industrial Economics, 2020). Of these, according to the Vertical Line Model, 250 vessels are fishing 5 or fewer traps per trawl, and 755 vessels are fishing with more than 5 traps per trawl (Industrial Economics, 2020).
On-demand gear adoption costs are calculated for the 250 vessels fishing 5 or fewer traps per trawl assuming that acoustic releases would be used on all 25,127 endlines. For the 755 vessels fishing more than 5 traps per trawl, it is assumed that each trawl would employ 2 endlines. If acoustic releases are used on both endlines, the number of releases needed would be 48,514, the number of endlines associated with these 755 vessels.
The same procedure employed above is used to calculate potential adoption costs for the estimated 489 vessels fishing 12 or more nautical miles from the coast of the northeastern United States (Industrial Economics, 2020). According to the Vertical Line Model, these vessels are fishing more than 5 traps per trawl (Industrial Economics, 2020), and it is assumed that each trawl would employ 2 endlines (McCarron and Tetreault, 2012). There are an estimated 22,143 endlines associated with these 489 vessels (Industrial Economics, 2020).
The initial cost of on-demand fishing components is based on those commercially available from EdgeTech and Desert Star Systems, LLC, and in use by commercial fishers. It should be noted that because the costs of producing the first unit of gear components are not publicly available, the costs of purchasing the gear are used as proxies. This assumption will result in an overestimate of cumulative average cost if the market price is greater than the production cost of the first unit.
Components from both companies are in early production phase, with annual sales of 300 or fewer (Marco Flagg, email to author, March 2, 2021; Rob Morris, email to author, July 9, 2021). Orders of EdgeTech, available since 2019, have not reached a level to achieve large cost reductions (Rob Morris, email to author, July 9, 2021). The Desert Star CEO notes that the company, having a broad product line, is not dependent on any one product, has pricing flexibility, and experiments with pricing to see how it affects product demand (Marco Flagg, email to author, March 2, 2021).
Components of EdgeTech’s 5112 Ropeless Fishing System include the deck box with transducer, ranging in cost from $3,750 to $4,000, and acoustic releases at $4,000 each (Rob Morris, email to author, July 9, 2021). The system is designed to “easily withstand … shallow water and small boat operations” (EdgeTech, 2019). Acoustic releases for shallower, low- to medium-current waters nearshore/inshore are available from Desert Star Systems for $950 each, while those for deeper, high-current waters offshore are $1,995 each (with discounts available for the purchase of 10 or more releases) (Desert Star Systems, LLC, 2021). Desert Star deck units are $4,000 and $8,000 for nearshore and offshore use, respectively (Desert Star Systems, LLC, 2021).
Adoption costs are calculated separately for vessels 3 to 12 nautical miles from shore and for those 12 or more nautical miles from shore because, as noted above, the current cost of on-demand fishing gear designed for shallower, nearshore waters differ from those designed for offshore waters. For each distance category, costs are estimated assuming vessels use releases on all endlines.
An alternative, lower-cost estimate is calculated by assuming the vessels fishing more than 5 traps per trawl use acoustic releases on 1 of 2 endlines per trawl rather than both, based on results of a survey of lobster gear configurations in the Gulf of Maine (McCarron and Tetreault, 2012). They found that fishers generally use 1 buoy line (endline) when fishing trawls up to 5 traps and a second buoy line with more than 5 traps. Allowing deployment of a trawl of traps with only one fixed buoy line is an option to reducing fixed vertical lines suggested by NOAA Fisheries (NOAA, 2022c).
The cumulative average costs of on-demand gear components are estimated using Wright’s model (described above) with a 95% learning rate assuming all 1,494 vessels adopt the technology over a non-specified period of time. Calculations demonstrate substantial declines in average cost per unit with a ramp-up in production.
The cost of on-demand fishing gear is calculated for 1,005 vessels in federal waters 3 to 12 nautical miles from shore assuming initial costs of $4,000 for a deck box and $1,000 per acoustic release. The cumulative average cost for 1,005 deck boxes would be $2,398 each and for 73,641 releases $436 each, applying a 95% learning rate and Wright’s model.
The total on-demand gear adoption cost for 1,005 vessels is estimated at $34.5 million as follows:
● Deck boxes: 1,005 x $2,398 = $2.4 million
● Acoustic releases for 73,641 endlines = $32.1 million
● Total: $34.5 million
● Average per vessel: $34,346 (assuming endlines evenly distributed across vessels)
An alternative, lower-cost estimate can be calculated by assuming the 755 vessels fishing more than 5 traps per trawl use acoustic releases on 1 of 2 endlines per trawl rather than both. In this case, 24,258 releases would be needed (48,514 divided by 2) for those vessels The total number of acoustic releases needed nearshore would be 49,384 (24,258 + 25,127 for the 250 vessels fishing 5 or fewer traps/trawl, 1 endline/trawl). The cumulative average cost for 49,385 releases would be $449. The cumulative average cost for 1,005 deck boxes remains at $2,398.
The total on-demand gear adoption cost for 755 vessels fishing releases on 1 of 2 endlines is estimated at $12.7 million as follows:
● Deck boxes: 755 x $2,398 = $1.8 million
● Acoustic releases for 24,258 endlines = $10.9 million
● Total: $12.7 million
● Average per vessel: $16,824 (assuming endlines evenly distributed across vessels).
On-demand gear adoption cost for 250 vessels fishing releases on all endines is estimated at $11.9 million as follows:
● Deck boxes: 250 x $2,398 = $0.6 million
● Acoustic releases for 25,127 endlines = $11.3 million
● Total: $11.9 million
● Average per vessel: $47,526 (assuming endlines evenly distributed across vessels).
The total on-demand gear adoption cost for 1,005 vessels, with 755 vessels fishing with releases on 1 of 2 endlines per trawl rather than both, is estimated at $24.6 million.
Adoption costs are calculated for 489 vessels assuming initial costs of $8,000 for the deck box and $2,000 per acoustic release. The cumulative average cost for 489 deck boxes would be $5,059 each, and the cumulative average cost for 22,143 acoustic releases would be $954 each, applying a 95% learning rate and Wright’s model. Total adoption cost for 489 vessels is calculated at $23.6 million as follows:
● Deck boxes for 489 vessels: 489 x $5,059 = $2.5 million
● Acoustic releases for 22,143 endlines: 22,143 x $954 = $21.1 million
● Total: $2.5 million + $21.1 million = $23.6 million
● Average per vessel: $48,258
To calculate an alternative estimate, it is assumed releases are used on 1 of the 2 endlines per trawl. In this case, 11,072 acoustic releases would be needed (22,143 divided by 2), with a cumulative average cost of $1,004 each, (applying a 95% learning rate and Wright’s model). Total adoption cost for 489 vessels is calculated at $13.6 million as follows:
● Deck boxes: 489 x $5,059 = $2.5 million
● Acoustic releases for 489 vessels fishing more than 5 traps per trawl, release on 1 of 2 endlines: 11,072 x $1,004 = $11.1 million
● Total: $2.5 million + $11.1 million = $13.6 million
● Average per vessel: $27,792
The total cost of on-demand fishing technology adoption by the estimated 1,494 vessels operating in northeastern United States federal waters is the sum of the costs for the vessels fishing nearshore and offshore calculated above (Table 5). Assuming acoustic releases are used on all endlines, this is calculated as $34.5 million + $23.6 million, totaling $58.1 million. The average cost per vessel would be $38,899 (Figure 4).
Figure 4 Average cost of on-demand fishing gear per vessel for 1,494 commercial vessels in northeastern U.S. federally-managed waters.
If vessels fishing more than 5 traps per trawl use releases on 1 of 2 endlines per trawl, the total cost would be $38.2 million, $19.9 million (34%) less. The average cost per vessel would be $25,552 vessels (Table 6).
Table 6 Total on-demand fishing gear adoption costs for 1,494 vessels, releases on 1 of 2 endlines per trawl for vessels fishing more than 5 traps per trawl.
The cumulative average cost calculated using Wright’s learning model depends on the assumed initial cost, the number of units produced, and the learning rate. The on-demand gear adoption costs estimated above show the variability with changes in production and initial on-demand component costs at a 95% learning rate. To assess the sensitivity of the estimates to a more conservative learning rate, 97%, the adoption costs for 1,005 nearshore vessels and 489 offshore vessels in federally-managed waters is calculated, assuming acoustic releases are used on all endlines. This rate implies that a doubling in production could result in a 3% reduction in cumulative average price per unit.
Results show that the projected adoption costs increase substantially by varying the learning rate by only 2%. With a 97% learning rate, on-demand fishing adoption costs increase by $13.4 million to $48.0 million for 1,005 nearshore vessels, a 39% increase, assuming releases are used on all endlines. For 489 offshore vessels, adoption costs increase to $31.5 million, or 34%, with a 97% learning rate. For all 1,494 vessels, total adoption costs increase $21.4 million from $58.1 million to $79.5 million, or 37%.
Calculations assuming higher initial costs for on-demand fishing components and/or fewer vessels adopting the technology would result in higher cumulative average costs, and thus adoption costs, for both learning rates. It could be the case, for example, that on-demand gear may be used in restricted areas, which represent a subset of vessels operating in Federal waters. Conversely, lower initial costs for components and/or more vessels adopting the technology (e.g., some in state waters) would result in lower average cumulative costs.
The key limitations of using a learning model approach derive from the assumptions required for the calculations. In this analysis, both the initial costs of on-demand fishing systems at the production phase and the learning rate are estimates. The estimates of the initial costs are, however, guided by the cost of on-demand components commercially produced and in use.
It is likely that less expensive components and systems will eventually be produced (e.g., as a result of technology advances, increases in demand, and competitive pressures). For example, Desert Star Systems is developing other versions of their offshore acoustic release ARC-1 (now $1,950 each, or $1,550 each when purchased in quantities of 100 or more) that will be priced at around $500 each (Zimmer, 2019; Desert Star, 2021). Releases for EdgeTech’s Ropeless Fishing System currently sell for $4,000, and with sales of thousands of units, they expect at least a 1/3 cost reduction (Rob Morris, email to author, July 9, 2021); to approximately $2,670.
Fiomarine Industry is testing its current model, Fiobuoy AC100 (for shallower waters, deployable to 100m), which retails for $6,800 (Mike Shegog, email to author, April 6, 2021). They are designing a new model called the F-Series specifically for on-demand fishing (Mike Shegog, email to author, April 6, 2021). It is essentially the same design and technology as their other models but with alterations and additions for fishers: live tracking, automation, geo-fencing (restricting to seasons and zones), water temperature sensing, and other capabilities, if required. When fully implemented, the new system will add around a minute to deploy and to retrieve. Even with runs of around 3,000 units, the company expects prices would decline to around $2,000 for each Fiobouy unit (1 per trap or trawl) and decline further as time goes on. One deck unit with a transducer would be between $4,000 and $6,000 when fully optioned (Mike Shegog, email to author, April 7, 2021).
On-demand fishing gear is proving to be a viable option to traditional fishing methods and, used strategically, is a step towards the continued survival of whales and northeast commercial lobster and Jonah crab trap/pot fisheries. It could most effectively be initially employed to provide the greatest reduction in whale entanglement risk in federally-managed waters (greater than 3 nautical miles from shore). Use of the gear may be most critical for continued fishing in these waters given the gear modifications required by the 2021 amendment to the Atlantic Right Whale Take Reduction Plan, which include reductions in the number of vertical buoy lines, the establishment of weak rope requirements, and trawl length minima (Taking of Marine…2021). An analysis of these vertical line strength requirements in the lobster trap fishery in the Gulf of Maine found that weak ropes are not as feasible for use in the offshore lobster fishery, while inshore areas can be safely fished with vertical lines within the recommended breaking strength specification (Willse et al., 2022).
NOAA Fisheries has developed a “ropeless roadmap” describing a plan for integrating on-demand systems into U.S. commercial fisheries more broadly (NOAA, 2022c). The document provides details on how to address other challenges, such as gear geolocation, gear conflicts with mobile gear fisheries, enforcement, and current regulations. Successful implementation on the water will require continued guidance and support from developers, at least in the short-term. As on-demand gear is adopted, more fishers may find the technology would benefit them. In addition to preventing ocean animal entanglements, acoustic technology can result in less gear lost (e.g., from vandalism, vessel conflicts, bad weather, or sea conditions), less (if any) catch lost to poachers, and more efficient retrieval of lost gear (Desert Star Systems, 2021; EdgeTech, 2021).
This analysis considers only the potential market cost of on-demand gear; there are other costs that may become evident as the gear is more widely implemented. For example, the use of on-demand systems may require additional time on board compared to conventional traps if it takes longer for crew to retrieve each trap or trawl (Molyneaux, 2020; Simke, 2020). Some systems can be set to appear at the surface on a specific date and time (e.g., Fiobuoy TD100 & TD200; Fiomarine Industries, 2022), while other acoustic systems may take up to 10 minutes to surface after being signaled (Partan and Ball, 2016). Consequently, crew may be able to haul in fewer traps per day.
In addition, replacing release devices and re-packing traps with the line and buoy or liftbag (or reloading spools) will take crew time that would otherwise not be expended to redeploy conventional traps. These factors may be lessened or eliminated in the future with technology improvements. For example, prototype on-demand systems with the vertical line pre-spooled or pre-packed in traps ready for deployment as soon as a trap is hauled on board have been considered (Partan and Ball, 2016). While fewer lobsters caught per fishing trip may result in lower revenue per trip, it should be noted that there is evidence that reduced fishing effort (use of fewer traps) can increase landings and fishing profits (Myers and Moore, 2020).
The initial cost of on-demand fishing systems has often been given as an obstacle to adoption (Molyneaux, 2020; Ropeless Consortium, 2020). The costs above for the northeastern U.S. lobster and crab fishery provide estimates of the level of funding required should governments, non-governmental organizations, and/or other groups choose to implement programs to help fishers acquire gear. If on-demand gear is adopted more broadly throughout the United States and the rest of the world, costs would continue to decline.
Foundations, educational institutions, environmental organizations, fishing gear developers, and governments continue to provide funding to further the development and testing of on-demand fishing gear. Non-governmental organizations provide grants and other support for research and programs directed toward reducing risks to North Atlantic right whales, as well as other marine species harmed by vertical line entanglement. These include the National Fish and Wildlife Foundation, Island Foundation, and SeaWorld & Busch Gardens Conservation Fund. (Fishing Gear Location Marking Fund; SeaWorld Parks and Entertainment, 2019; Bogomolni and Baumgartner, 2020; National Fish and Wildlife Foundation, 2020). Most recently, NOAA has partnered with the non-profit AI2 to develop and test the geolocation tool and system with several on-demand gear developers (Gahm et al., 2022).
Beginning in FY 2020, additional federal funds have been provided for North Atlantic right whale-related research, development, and conservation in annual Department of Commerce Appropriations (Consolidated Appropriations Act, 2020). In addition to appropriations, two NOAA programs may provide financial assistance. Through the Fisheries Finance Program, NOAA has the authority to provide long-term fixed-rate loans to commercial fishers for a range of fishery-related activities (NOAA, 2021d). Eligible costs include fishing vessel reconstruction and purchase, and acquiring vessel equipment (NOAA n.d.). The Capital Construction Fund Program enables fishers to establish an account to use pre-tax dollars to construct, reconstruct, or (under some circumstances) acquire fishing vessels (NOAA, 2021e).
There is also precedent for federal and community aid to help fishers modify fishing gear to comply with regulations protecting other endangered marine species. Government-funded programs “buy out” fishing permits and/or gear to incentivize fishers to switch to gear that mitigates ocean mammal entanglements. Examples include the Maine Groundline Exchange Program (Schreiber, 2006; Lincoln County News, 2010; Ludwig, 2011) and the Shark and Swordfish Permit Transition Program (Commercial Fishing, 2017-2018; Bittenbender, 2020). There have been community funding initiatives for the purchase of gillnet “pingers” – acoustic alarms – required to reduce interactions between harbor porpoises and commercial gillnet gear in both New England and the Mid-Atlantic (Lessard, 2013; Talking Fish, 2013).
There may be other creative strategies to offset the acquisition cost of on-demand fishing gear. For example, fishers’ associations could obtain a supply of on-demand gear that could be rented out to fishers if an area is temporarily closed; governments and/or environmental organizations could assist in funding such a program (Terhune, 2018). And, associations within a state or management area could arrange a bulk purchase (or lease) of on-demand fishing systems for all fishers in the state or area to obtain lower costs per unit.
The adoption of on-demand fishing technology by lobster and other trap/pot fishers is one of many management strategies needed to allow recovery of the North Atlantic right whale. It would facilitate the survival of other marine species as well, and the continuation of profitable northeastern fisheries and related communities.
The raw data supporting the conclusions of this article will be made available by the author, without undue reservation.
The author confirms being the sole contributor of this work and has approved it for publication.
Prepared Under National Oceanic and Atmospheric Administration Contract #1305M418DNFFK0010, Task Order #1305M220FNFFM0140.
Many thanks to Chao Zou for providing the vessel and gear data required for estimates. Also, thanks to Eric Thunberg, Sean Hayes, Michael Asaro, and Kathryn Bisack for their assistance in framing this research and review of earlier versions of this document.
Author CA was employed by company Environmental Assessment Services, LLC.
All claims expressed in this article are solely those of the authors and do not necessarily represent those of their affiliated organizations, or those of the publisher, the editors and the reviewers. Any product that may be evaluated in this article, or claim that may be made by its manufacturer, is not guaranteed or endorsed by the publisher.
Anzanello M. J., Fogliatto F. S. (2011). Learning curve models and applications: Literature review and research directions. Int. J. Ind. Ergonomics. 41 (5), 573–583. doi: 10.1016/j.ergon.2011.05.001
Baumgartner M., Baumwell L., Brilliant S., Baker E. (2021). “Workshop on buoyless fishing gear location marking methods: Report on stakeholder engagement meetings,” in Report to the ropeless consortium. August 2021. https://ropeless.org/wp-content/uploads/sites/112/2021/08/GearLocationMarkingStakeholderReport_Aug2021.pdf?utm_source=North+Atlantic+Right+Whale+Consortium+List&utm_campaign=9b901d77f3-EMAIL_CAMPAIGN_2020_02_14_02_55_COPY_01&utm_medium=email&utm_term=0_4485741029-9b901d77f3-372853394.
Baumgartner M., Werner T., Moore M. (2019). Urgent need for ropeless fishing: Removing end lines to protect right whales. Sea Technology. 60 (3), 23–27. Available at: https://ropeless.org/wp-content/uploads/sites/112/2019/05/ST_Urgent-Need.pdf.
Bittenbender S. (2020). Oceana provides USD 1 million to California gillnet buyback program. Seafood Source. Available at: https://www.seafoodsource.com/news/environment-sustainability/oceana-provides-usd-1-million-to-californa-gillnet-buyback-program.
Bogomolni A., Baumgartner M. (2020). “The fishing gear location marking fund,” in Presented at ropeless consortium annual meeting. Virtual. 01.05. Available at: https://ropeless.org/2020-annual-meeting/2020-ropeless-meeting-agenda-and-presentations/
Brennan D. (2020). “Process technology evolution and adoption,” in Process industry economics, 2nd. Ed. Brennan D. (Amsterdam: Elsevier), 177–207.
Commercial Fishing (2017-2018). Drift gillnet shark and swordfish fishery: Permit transition program. California SB 1017.
Consolidated Appropriations Act of 2020. Available at: https://www.congress.gov/116/cprt/HPRT38678/CPRT-116HPRT38678.pdf. (2019).
Cooke J. G. The IUCN red list of threatened species 2020, Eubalaena glacialis (errata version published in 2020).
Delionback L. M. (1995). “Learning curves and progress functions,” in Cost estimator’s reference manual, 2nd. Eds. Steward R. D., Wyskida R. M., Johannes J. D. (New York: John Wiley & Sons, Inc), 169–192.
Desert Star Systems, LLC (2021). “Acoustic releases“. Available at: https://www.desertstar.com/page/arc-1xd, (Accessed June 22, 2021)
Dutton J. M., Thomas A., Butler J. E. (1984). The history of progress functions as a managerial technology. Business History Review. 58 (2), 204–233. doi: 10.2307/3115048
EdgeTech (2019). 5112 ropeless fishing system [PDF] (EdgeTech). Available at: https://www.edgetech.com/wp-content/uploads/2019/07/ETN-5112-RFS-9_17_19.pdf.
EdgeTech (2021) 5112 ropeless fishing system Available at: https://www.fishingindustrynewssa.com/2020/12/09/the-octopus-fishery-has-a-gripping-story-to-tell/ (Accessed June 22, 2021).
FINSA Fishing Industry News Southern Africa (2020) The Octopus Fishery Has a Gripping Story to Tell. FINSA.
Fiomarine Industries Fiobuoy models. Available at: https://fiomarine.com/fiobuoy-models/ (Accessed May 11, 2022).
Fishing Gear Location Marking Fund. Available at: https://www.gear-fund.org/ (Accessed June 22, 2021).
Gahm M., Long K., Good C., Matzen E., Milliken H., Hayes S. (2022). “U.S. efforts to develop ropeless fishing gear technology,” in International whaling commission annual scientific committee meeting. #SC/68D/HIM/07.
Glock C. H., Grosse E. H., Jaber M. Y., Smunt T. L. (2019). Applications of learning curves in production and operations management: A systematic literature review. Comput. Ind. Engineering. 131, 422–441. doi: 10.1016/j.cie.2018.10.030
Grosse E. H., Glock C. H., Müller S. (2015). Production economics and the learning curve: A meta-analysis. Int. J. Production Economics 170, 401–412. doi: 10.1016/j.ijpe.2015.06.021
Henderson B. (1968) The learning curve. Boston consulting group (BCG)Available at: https://www.bcg.com/publications/1968/business-unit-strategy-growth-experience-curve (Accessed June 22, 2021).
Henry A. G., Garron M., Morin D. M., Reid A., Ledwell W., Cole T. V. N. (2020). “Serious injury and mortality determinations for baleen whale stocks along the gulf of Mexico, UC T/O, and Atlantic Canadian provinces,” in Northeast Fisheries Science Center Reference Document 20-06. (Woods Hole, Massachusetts).
Hydro International (2009) Successful acoustic fiobuoy trials for AHS. Available at: https://www.hydro-international.com/content/news/successful-acoustic-fiobuoy-trials-for-ahs(Accessed June 22, 2021).
Johnson D. L. (1987). “The learning curve, which one to use,” in Defense systems management college newsletter (New York City (NY): Columbia University: Program Manager).
Johnson A., Salvador G., Kenney J., Robbins. J. Kraus S., Landry S., Clapham P. (2005). Fishing gear involved in entanglements of right and humpback whales. Mar. Mammal Sci. 21, 635–645. doi: 10.1111/j.1748-7692.2005.tb01256.x
Knowlton A. R., Clark J. S., Hamilton P. K., Kraus S. D., Pettis H. M., Rolland R. M., et al. (2022). Fishing gear entanglement threatens recovery of critically endangered north Atlantic right whale. Conserv. Sci. Pract. 4 (8), e12736. doi: 10.1111/csp2.12736
Knowlton A. R., Hamilton P. K., Marx M. K., Pettis H. M., Kraus S. D. (2012). Monitoring north Atlantic right whale Eubalaena glacialis entanglement rates: a 30 year retrospective. Mar. Ecology Prog. Ser. 466, 293–302. doi: 10.3354/meps09923
Lessard R. (2013). N.H. fishermen double down on new generation of pingers to save migratory porpoise, NHPR. Availabe at: https://www.nhpr.org/nh-news/2013-06-07/n-h-fishermen-double-down-on-new-generation-of-pingers-to-save-migratory-porpoise#stream/0
Liao S. S. (1988). The learning curve: Wright’s model vs. Crawford’s model. Issues Accounting Education. 3 (2), 302–215.
Lolli F., Messori M., Gamberini R., Rimini B., Balugani E. (2016). Modelling production cost with the effects of learning and forgetting. IFAC World Congress Proc. 49 (12), 503–508. doi: 10.1016/j.ifacol.2016.07.672
Marino M. (2011). Innovation ends spectre of ghost traps. Fish. 19 (4), 12–13. Available at: https://desertstarsystems.nyc3.digitaloceanspaces.com/ARC-Page/Marino,%20Melisa%20%20-%20Innovation%20Ends%20Spectre%20of%20Ghost%20Traps.pdf.
McCarron P., Tetreault H. (2012) Lobster pot gear configurations in the gulf of Maine. Available at: https://www.bycatch.org/sites/default/files/Lobster_Gear_Report.pdf.
Molyneaux P. Pop-up pots and the search for ‘whale-safe’ gear (National Fisherman). Available at: https://www.nationalfisherman.com/boats-gear/pop-up-pots-and-the-search-for-whale-safe-gear.
Moore M. J., Rowles T. K., Fauquier D. A., Baker J. D., Biedron I., Durban J. W., et al. (2021). Assessing north Atlantic right whale health: a review of threats, and development of tools critical to conservation of the species. Dis. Aquat. Organisms. 143, 205–226. doi: 10.3354/dao03578
Morris R. (2020). EdgeTech trap tracker application for ropeless fishing, Ropeless Consortium Annual Meeting: Virtual. Available at: https://ropeless.org/2020-annual-meeting/2020-ropeless-meeting-agenda-and-presentations/
Myers H. J., Moore M. J. (2020). Reducing effort in the U.S. American lobster ITAL T/O fishery to prevent north Atlantic right whale (Eubalaena glacialis) entanglements may support higher profits and long-term sustainability. Mar. Policy 118. doi: 10.1016/j.marpol.2020.104017
Myers H. J., Moore M. J., Baumgartner M. F., Brillant S. W., Katona S. K., Knowlton A. R., et al. (2019). Ropeless fishing to prevent large whale entanglements: Ropeless consortium report. Mar. Policy 107. doi: 10.1016/j.marpol.2019.103587
NFWF National Fish and Wildlife Foundation (2020). NFWF announces $500,000 in new support to help conserve north Atlantic right whales (NFWF).
NOAA National Oceanic and Atmospheric Administration (2020) Draft environmental impact statement: Atlantic Large whale take reduction plan risk reduction rule (NOAA Fisheries) Available at: https://www.fisheries.noaa.gov/resource/document/draft-environmental-impact-statement-atlantic-large-whale-take-reduction-plan (Accessed January 4, 2021).
NOAA (2021a) Endangered species act section 7 consultation biological opinion (National Marine Fisheries Service). Available at: https://www.greateratlantic.fisheries.noaa.gov/public/nema/PRD/Final%20Fisheries%20BiOp_05_28_21.pdf (Accessed August 1, 2022).
NOAA (2021b) Protected species gear research Available at: https://www.fisheries.noaa.gov/new-england-mid-atlantic/science-data/protected-species-gear-research (Accessed June 22, 2021).
NOAA (2021c). Lobster trap limit. greater Atlantic regional fisheries office (Data provided by Allison Murphy, Sustainable Fisheries Division).
NOAA (2021d) Fisheries finance program. Available at: https://www.fisheries.noaa.gov/national/funding-and-financial-services/fisheries-finance-program (Accessed May 6, 2022).
NOAA (2021e) Capital construction fund program (Capital Construction Fund Program | NOAA Fisheries) (Accessed June 22, 2021) Available at: https://www.fisheries.noaa.gov/national/funding-and-financial-services/capital-construction-fund-program.
NOAA (2022a) Atlantic Large Whale take reduction plan. Available at: https://www.fisheries.noaa.gov/new-england-mid-atlantic/marine-mammal-protection/atlantic-large-whale-take-reduction-plan (Accessed August 18, 2022).
NOAA (2022b). Scientific research and exempted fishing permits. (NOAA Fisheries). Available at https://www.fisheries.noaa.gov/new-england-mid-atlantic/sustainable-fisheries/scientific-research-and-exempted-fishing-permits (Accessed June 22, 2022).
NOAA (2022c). Draft ropeless roadmap: A strategy to develop on-demand fishing. Available at: https://media.fisheries.noaa.gov/2022-07/RopelessRoadmapDRAFT-NEFSC.pdf (Accessed August 1, 2022).
Oirere S. (2019). South Africa unveils octopus fishery rules to protect whales (Seafood Source News).
Pace III. R.M., Cole T. V., Henry A. G. Incremental fishing gear modifications fail to significantly reduce large whale serious injury rates. Endangered Species Res. 26, 115–126. doi: 10.3354/esr00635
Partan J., Ball K. (2016). Project 5 final report: Rope-less fishing technology development. Available at: https://www.bycatch.org/sites/default/files/Partan%26Ball_2016.pdf
Pettis H. M., Pace R. M. III, Hamilton P. K. (2022). North Atlantic right whale consortium 2021 annual report cardReport to the North Atlantic Right Whale Consortium.
Riels R. (2020). Fishing and testing SMELTS ropeless lift bag fishing gear in the gulf of Maine Presented at the 2020 Ropeless Consortium Annual Meeting. Virtual. Available at: https://ropeless.org/2020-annual-meeting/2020-ropeless-meeting-agenda-and-presentations/
Ropeless Consortium (2020). Session 1. marking systems Ropeless Consortium Annual Meeting: Virtual. Availabe at: https://ropeless.org/2020-annual-meeting/2020-ropeless-meeting-agenda-and-presentations/
Schreiber L. (2006). Groundline exchange scheduled for Maine, Fishermen’s Voice Monthly News. Availabe at: http://www.fishermensvoice.com/archives/0406groundlineexchange.html
SeaWorld Parks & Entertainment (2019). The SeaWorld & Busch gardens conservation fund commits $900,000 to protect critically endangered north Atlantic right whale (SeaWorld Parks & Entertainment).
Taking of Marine Mammals Incidental to Commercial Fishing Operations (2021). Atlantic Large Whale take reduction plan regulations; Atlantic coastal fisheries cooperative management act provisions (American Lobster Fishery). Federal register 86, 51970–52024.
Terhune J. (2018). An evaluation of at-sea field trials of a ropeless fishing method in LFA34 (Saint John New Brunswick, Canada: University of New Brunswick).
The Lincoln County News (2010). Lobster foundation announces final groundline exchanges for all fishermen, The Lincoln County News. Availabe at: https://lcnme.com/currentnews/lobster-foundation-announces-final-groundline-exchanges-for-all-fishermen/
Vincent H., Capotosto D. A. (2020). Ropeless RISER: A system for ropeless fishing Presented at the 2020 Ropeless Consortium Annual Meeting. Virtual. https://ropeless.org/2020-annual-meeting/2020-ropeless-meeting-agenda-and-presentations/
Willse N., Summers E., Chen Y. (2022). Vertical line requirements and the north Atlantic right whale entanglement risk reduction for the gulf of Maine American lobster fishery. Mar. Coast. Fisheries, 14(2), e10203. doi: 10.1002/mcf2.10203
Wright T. P. (1936). Factors affecting the cost of airplanes. J. Aeronautical Sci. 3 (4), 122–128. doi: 10.2514/8.155
Keywords: right whale, entanglement, bycatch, American lobster fishery, learning model, production, fishing gear, ropeless
Citation: Alkire C (2022) Decline in on-demand fishing gear costs with learning. Front. Mar. Sci. 9:943552. doi: 10.3389/fmars.2022.943552
Received: 13 May 2022; Accepted: 19 October 2022;
Published: 18 November 2022.
Edited by:
Kristy Wallmo, National Marine Fisheries Service, United StatesReviewed by:
Amy Richardson Knowlton, New England Aquarium, United StatesCopyright © 2022 Alkire. This is an open-access article distributed under the terms of the Creative Commons Attribution License (CC BY). The use, distribution or reproduction in other forums is permitted, provided the original author(s) and the copyright owner(s) are credited and that the original publication in this journal is cited, in accordance with accepted academic practice. No use, distribution or reproduction is permitted which does not comply with these terms.
*Correspondence: Carolyn Alkire, Y2Fyb2x5bi5hbGtpcmVAbm9hYS5nb3Y=
Disclaimer: All claims expressed in this article are solely those of the authors and do not necessarily represent those of their affiliated organizations, or those of the publisher, the editors and the reviewers. Any product that may be evaluated in this article or claim that may be made by its manufacturer is not guaranteed or endorsed by the publisher.
Research integrity at Frontiers
Learn more about the work of our research integrity team to safeguard the quality of each article we publish.