- 1Centre of Marine Sciences (CCMAR), CIMAR Associated Laboratory, University of Algarve, Campus de Gambelas, Faro, Portugal
- 2Phycology, Blue Biodiversity & Biotechnology RU, Laboratory of Plant Biotechnology, Ecology and Ecosystem Valorization – CNRST Labeled Research Unit n°10, Faculty of Sciences, University Chouaïb Doukkali, El Jadida, Morocco
Thermal tolerance is often interpreted as a species-wide thermal niche in the absence of studies focusing on the adaptive potential of populations to exhibit differential thermal tolerance. Thus, considering intraspecific thermal plasticity, local adaptation or both between populations along distributional gradients when interpreting and predicting species responses to warming is imperative. Removing the effect of environmental histories by raising kelp gametophyte generations in vitro under common garden conditions allows unbiased comparison between population-specific adaptive variation under different environmental conditions. Following this approach, this study aims to detect (potentially) adaptive differentiation in microscopic life-stages (gametophytes) between populations of a temperate forest forming kelp, Laminaria ochroleuca from locations with distinct thermal conditions. Gametophytes from four geographically distinct populations were subjected to different temperature treatments (17, marine heat waves of 23, 25 and 27°C) and gametophyte survival during thermal stress as well as reproductive success and photosynthetic responses during recovery were investigated. Intraspecific variation in resilience and reproductive output to thermal stress was found in L. ochroleuca; gametophytes from the most northern population (Brittany, France) were the most thermally sensitive, with mortality onset at 23°C, whereas mortality in the remaining populations was only apparent at 27°C. Gametophytes from northern Spain and Morocco exhibited very low reproductive success during recovery from 23 and 25°C. However, when recovering from the highest thermal treatment (27°C) the reproductive development and sporophyte output was higher than in the gametophytes from France and Italy (Mediterranean). The population-specific responses of gametophyte resilience and reproductive success to temperature stress suggest genetic differentiation in response to variation in local thermal regimes.
Introduction
Species with extensive geographical ranges are not homogeneous units, but rather a collection of conspecific populations inhabiting distinct environmental regimes (Linhart and Grant, 1996). Over evolutionary periods, populations may exhibit intraspecific variation driven by local thermal conditions (Valladares et al., 2014; King et al., 2018) resulting in locally adapted populations, with specific fitness advantages (e.g. thermal resilience, growth rate, etc.) that may surpass the species average response (Denny, 2017). Among the consequences of climate change, sudden and anomalous warm thermal events such as marine heat waves (MHWs) have drastically affected entire ecosystems (Garrabou et al., 2009; Wernberg et al., 2012; Duarte et al., 2020) particularly in warming hotspots, which may lead to population-specific responses. Nonetheless, most studies investigating responses of marine species to environmental stressors or models aiming to predict geographical distributions under future climate conditions, need to assume that tolerances are reflected solely on a species-level in the absence of localized adaptation studies, which can ultimately lead to the over- or under-estimated impacts of climate changing conditions on biodiversity and ecosystems (Bellard et al., 2012; Applebaum et al., 2014).
Kelp forests are predominantly composed of brown algae of the order Laminariales (Dayton, 1985), dominating rocky shores of the world’s temperate and cold-water marine ecosystems (Steneck et al., 2002; Smale et al., 2019). These forests provide a variety of ecosystem goods and services, either directly as a source of food or medicinal products, or indirectly as biogenic habitats for a variety of economically and ecologically important marine species (Steneck et al., 2002; Teagle et al., 2017). As the distribution and survival of habitat-forming algae is generally determined by species thermal limits and seawater temperatures (King et al., 2019), many kelp populations are severely threatened by global warming and large-scale declines in their abundance and range shifts have been reported worldwide (Wernberg, 2021; McPherson et al., 2021; Anderson et al., 2021). Habitat-forming algae such as kelps are good models to study intraspecific variability due to their high dependence on ocean temperatures, limited dispersal, strong spatial structuring (King et al., 2018; Miller et al., 2019) as well as relatively reduced connectivity which is facilitated by ocean currents and upwelling regions acting as thermal refugia for local genetic diversity (e.g. Coleman, 2013; Pereyra et al., 2013; Buonomo et al., 2016; Lourenço et al., 2016; Lourenço et al., 2017). Additionally, historical depth range shifts in Laminariales were found to preserve and isolate ancient gene pools during warming periods enabling long-term persistence and facilitating local adaption (Graham et al., 2007; Santelices, 2007; Assis et al., 2016).
Laminariales have a heteromorphic life cycle, alternating between a microscopic haploid gametophyte and a macroscopic diploid sporophyte life stage (Colin et al., 2003; Lane et al., 2006). Although microscopic gametophytes show higher thermal tolerance for growth and survival than the macroscopic sporophytes, a lower and narrower temperature window is required for successful reproduction between sexually mature gametophytes (tom Dieck, 1992; Bartsch et al., 2013; Martins et al., 2017). Therefore, temperature increases may delay reproductive development (Martins et al., 2020), with negative effects on kelp recruitment (Martins et al., 2017; Silva et al., 2022). Microscopic gametophytes may remain vegetative under unfavorable environmental conditions, acting as a “seed bank” (tom Dieck, 1993; Carney and Edwards, 2010; Martins et al., 2017; Silva et al., 2022) allowing the persistence of kelp populations following significant sporophyte mortality, e.g., due to extreme climatic events (Ladah and Zertuche-Gonzalez, 2007; Barradas et al., 2011).
The genus Laminaria presents one of the most diverse distribution ranges among kelp (Bolton, 2010; Rothman et al., 2017) which is assumed to have led to higher speciation rates compared to other genera and includes species that exhibit some of the highest temperature tolerances among kelp (tom Dieck, 1992). Laminaria ochroleuca, the golden kelp, is a warm temperate kelp species that can be found in the north-eastern Atlantic. Its distribution ranges from the southern UK to Morocco but also forms deeper populations in the Azores and Mediterranean Sea (Birkett et al., 1998; Assis et al., 2009; Flores-Moya, 2012; Ramos et al., 2016). Locally isolated biodiversity hotspots of L. ochroleuca were discovered at lower latitudes, exhibiting reduced connectivity as well as higher and unique genetic diversity (Assis et al., 2018). However, the level of population variability in thermal tolerance remains poorly understood for L. ochroleuca (but see Pereira et al., 2015). Thus, this species with a distributional range covering different thermal gradients provides an excellent model to investigate intraspecific adaptive variation. Investigating intraspecific adaptive variation to warming events is crucial in predicting the persistence of foundation species along their distributional ranges (Pearman et al., 2010) and therefore the fate of coastal communities and ecosystems. However, distinguishing genetic variation among populations from phenotypic short-term acclimation in species is difficult. By removing the influence of environmental history, common garden conditions allow us to investigate whether a generation of individuals from distinct populations raised in the same conditions exhibits different ecophysiological responses to environmental stress, and therefore the existence of adaptive differentiation rather than phenotypic plasticity.
This study aims to detect potential intraspecific adaptive differentiation to extreme thermal conditions in the habitat-forming kelp species L. ochroleuca. Using four populations spanning distinct thermal regimes and broadly representing the species distributional range in the NE Atlantic (NW France, NW Spain, Morocco) and Mediterranean (Italy), we compared the resilience and recovery capacity of gametophytes to simulated MHWs. Gametophyte survival during thermal stress as well as reproductive success and photosynthetic responses during recovery were investigated. The gametophytes were raised in vitro under common garden conditions from spores to remove the effect of environmental histories (although epigenetic inheritance cannot be discounted entirely, e.g., see Gauci et al., 2022), allowing comparison between population-specific adaptive variation under different environmental conditions. Inter-population variation in response to simulated MHW focused on detecting adaptive variation delivers fundamental insights into local marine coastal ecology and evolution, which can potentially be incorporated into models anticipating future vulnerability and geographic range shifts of this foundation species under climate change scenarios.
Materials and methods
Algal material
Mature specimens of Laminaria ochroleuca were collected in Roscoff, France (FR, November 2018), the Strait of Messina, Italy (IT, November 2019), Galicia, NW Spain (SP, May 2019) and El Jadida, Morocco (MO, August 2019) by SCUBA diving or snorkeling (see Table 1 for detailed sampling information, Figure 1). These four populations of L. ochroleuca originate from different latitudes and local habitat type (variable shallow coastal [FR, SP, MO] versus deeper stable [IT] habitat) with distinct local in situ temperatures (Table 1). The northernmost population (FR) is locally exposed to maximum sea surface temperature (SST) of 16.35°C while the southernmost (MO) is exposed to maximum SST of 23.19°C (Table 1, temperatures were obtained from Bio-Oracle (http://www.bio-oracle; Assis et al., 2017; supplementary material 1).
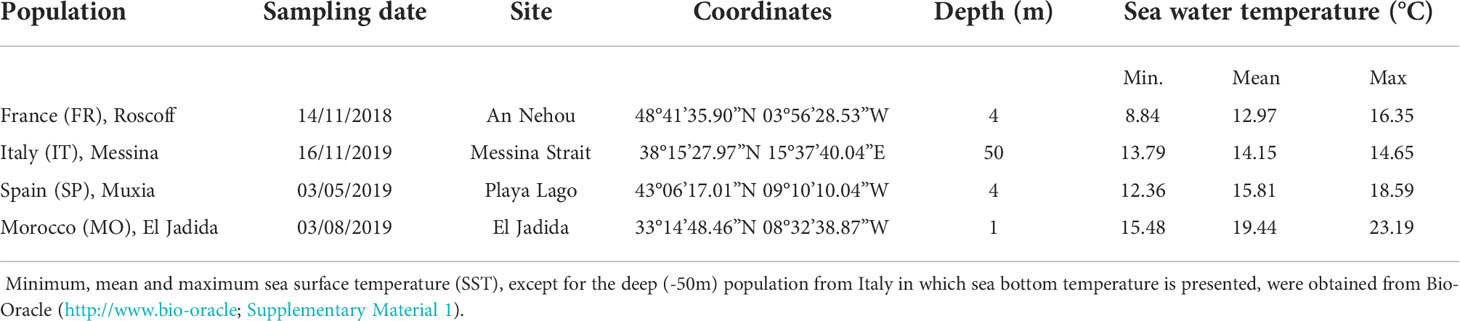
Table 1 Locations of Laminaria ochroleuca sampling sites (country, sampling date, geographical coordinates of each site, depth).
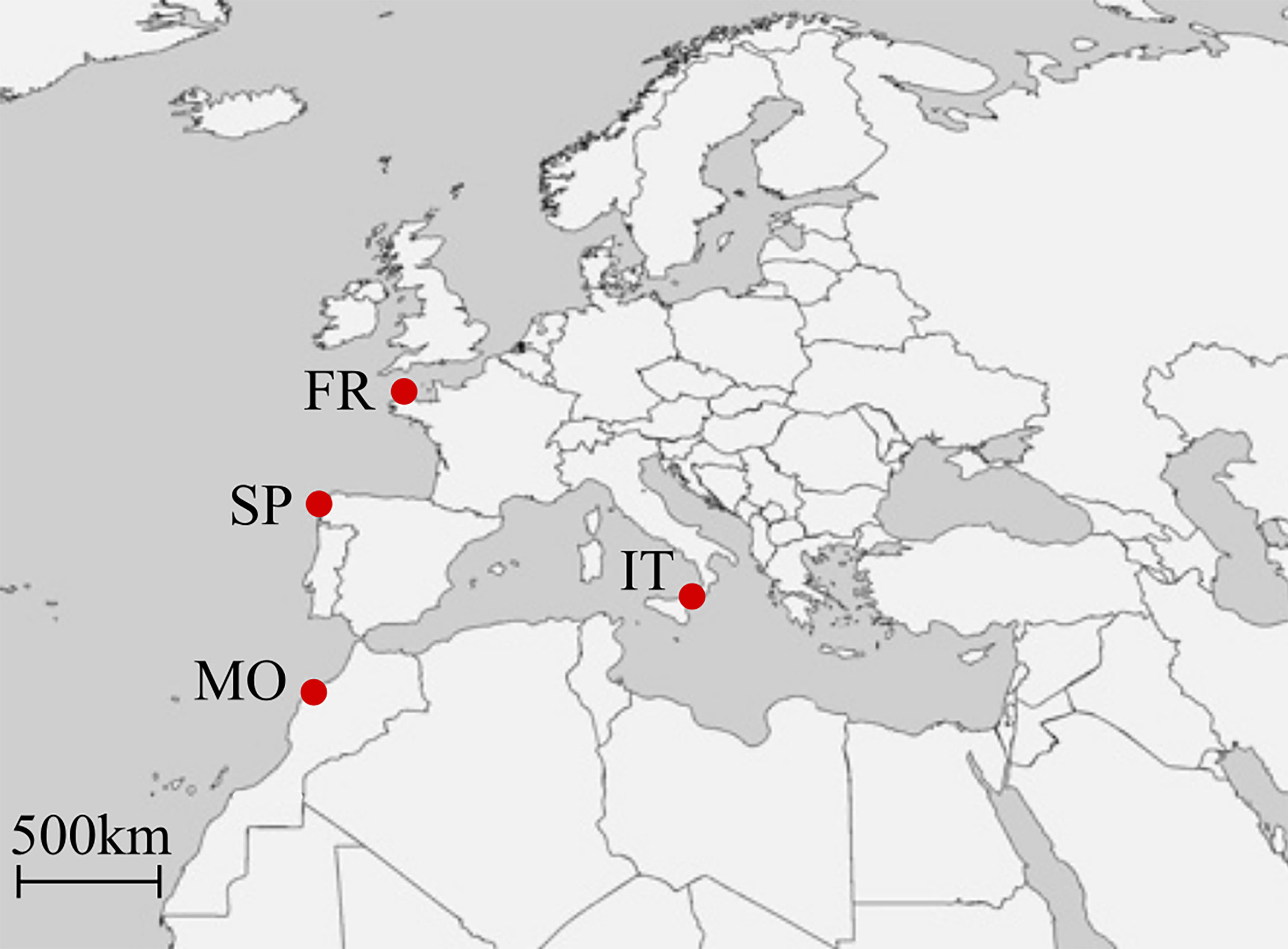
Figure 1 Sampling locations of Laminaria ochroleuca populations (red circles): France (FR), Italy (IT), Spain (SP) and Morocco (MO).
Two disks (2 cm Ø) were excised from reproductive tissue (sorus) of each mature adult sporophyte and cleaned with dry paper towel. The disks were placed into a Falcon tube with sterile seawater and two glass slides and left overnight in darkness to induce meiospore release. The seawater and the slides were then transferred to Petri dishes and left to germinate. The male and female gametophytes obtained from multiple L. ochroleuca sporophytes per population (IT: 6 individuals; FR, SP, MO: 5 individuals) were maintained together in a vegetative state in sterile half-strength Provasoli enriched seawater (PES; Provasoli, 1968, modifications: HEPES buffer instead of TRIS, double concentration of Na2-glycerophosphate). The cultures were maintained in climate-controlled chambers (Fitoclima, S600, Aralab, Lisboa, Portugal) at 13°C under 3-6 µmol photons m-2 s-1 of fluorescent red light to prevent gametogenesis under a 16:8 h light:dark photoperiod (controlled via Fitolog400R software). The culture medium was changed monthly, until the start of the experiment (ca. 1-2 y). Sterile artificial seawater (Tropic Marin Sea Salt, Dr. Biener, GmbH, Wartenberg, Germany) with 30-32 ppm salinity (hand refractometer ATAGO Co., Ltd) was used for culture maintenance and all experiments.
Experimental setup
The gametophyte cultures were acclimated for two days at 15°C before the start of the experiment for a gradual temperature increase. The same amount of gametophyte vegetative tissue derived from multiple L. ochroleuca individuals were combined per population, gently fragmented with a pestle and mortar, sieved (stainless stell sieve with 100 µm mesh), and diluted in sterile 10% PES to produce four stock solutions of gametophytes with lengths of ≤ 100 µm. The density of each stock solution was determined using an inverted microscope (100× magnification, Zeiss Axio Observer D1, Carl Zeiss MicroImaging GmbH, Göttingen, Germany) and the respective volume needed to obtain ~500-600 gametophytes cm-2 was added to glass beakers (5.5 cm diameter, 5.5 cm height) filled with 80 ml of 10% PES. Four replicate beakers were used per population and thermal treatment (4 populations × 4 temperatures × 4 replicates = 64 beakers in total). The gametophytes were allowed to settle and recover from the mechanical stress induced by fragmentation at 17°C under 3-6 µmol photons m-2 s-1 of red light for 5 days.
After this period, the gametophytes were transferred to each heat wave (HW) treatment (with a maximum temperature of 23°C, 25°C and 27°C) for 11 experimental days or left under control conditions at 17°C. To avoid gametophyte thermal shock and to mimic natural temperature changes the temperature was gradually increased in the HW treatments from 17°C to each maximum temperature at a warming rate of 2-3°C day-1. The maximum temperatures of 25°C and 27°C were kept for 5 days (following Hobday et al., 2016), while 23°C was kept over a period of 7 days. After this HW peak, the temperatures were gradually decreased back to 17°C again at a cooling rate of 2-3°C day-1, initiating a recovery phase for the subsequent 29 days (see Figure 2 for detailed experimental design). Experiments were conducted in temperature-controlled waterbaths (one waterbath per thermal treatment; Huber Variostat with Pilot ONE, Offenburg, Germany), provided with 20 μmol photons m-2 s-1 white LED light in a 16:8 h light:dark photoperiod. Culture medium (10% PES, 30-32 ppm) was 50% changed per beaker after 12 and 29 days. The irradiance of 20 μmol photons m-2 s-1 and the control temperature of 17°C chosen were reported as optimal conditions for L. ochroleuca gametogenesis (Izquierdo et al., 2002). Whereas the sub-lethal and lethal temperatures of 23°C, 25°C and 27°C for L. ochroleuca gametophytes (tom Dieck, 1992) were used to test for physiological and ontogenetic differences between populations.
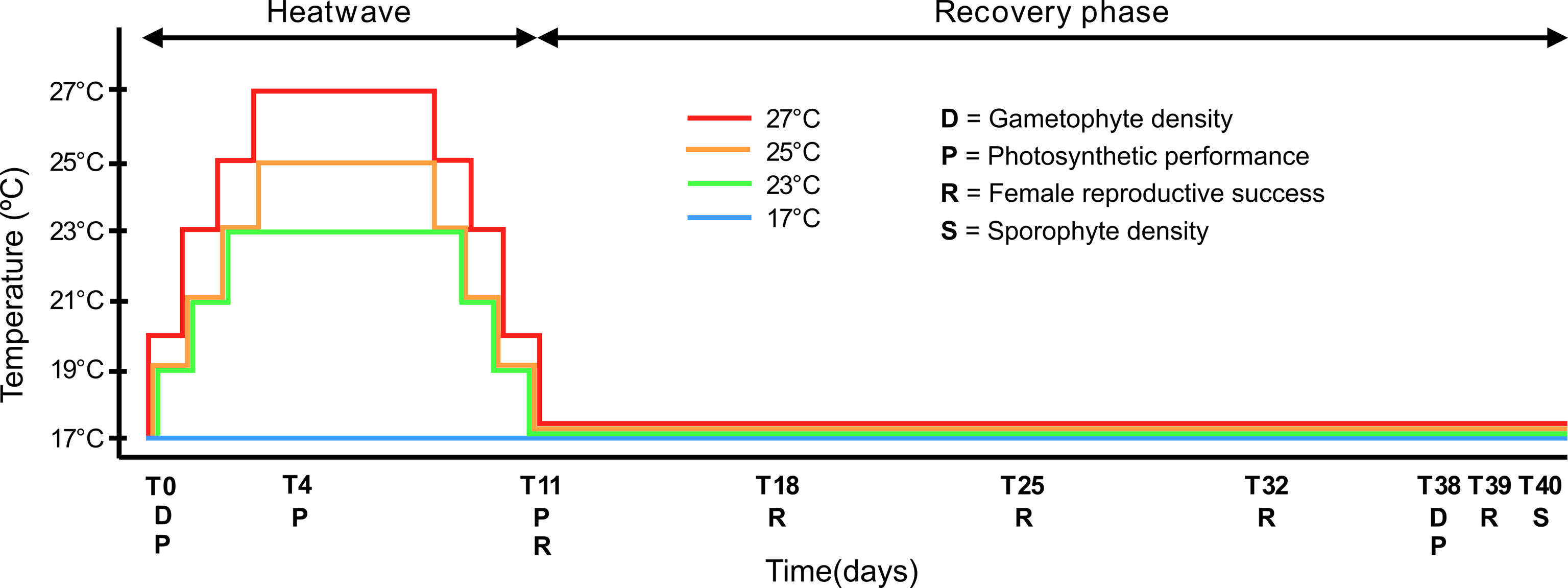
Figure 2 Design of the simulated MHW and recovery experiment. Laminaria ochroleuca gametophytes from four populations were exposed to different thermal treatments (17°C, 23°C, 25°C, 27°C) for 11 days and subsequently transferred to 17°C (recovery phase) for 29 days. Gametophyte density, photosynthetic performance, female reproductive success and sporophyte density were evaluated in different time periods.
Gametophyte density
To assess the survival capacity of gametophytes after simulated MHW, the density of combined male and female gametophytes (gametophytes cm-2) was determined at day 0 and 38 (11 days of heat treatment + 27 days of recovery). The number of gametophytes was quantified in a minimum of 70 fields of view per replicate using an inverted microscope (100× magnification).
Photosynthetic performance
To assess the physiological status of gametophytes during simulated MHW and subsequent recovery, maximum quantum yield of PSII (Fv/Fm) was estimated (AquaPen-P AP 110-P fluorometer; Photon Systems Instruments, Drásov, Czech Republic). Gametophytes were dark-acclimated for five minutes before the measurements. Measurements were taken throughout the course of the experiment (day 0, 4, 11 and 38) to allow continuous assessment of gametophyte performance.
Female reproductive success
During the recovery phase, gametogenesis development was quantified every seven days (day 11, 18, 25, 32 and 39) by assessing the relative occurrence of three ontogenetic stages of female gametophytes (vegetative state, egg released state and gametophytes with sporophytes attached). A minimum of 100 fields of view were examined per replicate using an inverted microscope (100× magnification). For each female gametophyte, the most advanced reproductive state was recorded. Female gametophytes were identified as being in the sporophyte stage as soon as the first cell division was visible in the zygote. The percentages of gametophytes with eggs and sporophytes were summed to obtain the proportion of reproductive female gametophytes.
Reproductive success was also evaluated as the absolute numbers of sexually-derived and putatively asexual sporophytes (i.e., possible partheno-sporophytes with irregular morphology sensu tom Dieck, 1992) per female gametophyte (sporophyte density) at the end of the recovery phase (day 40). Sporophytes with normal morphology (clear polar differentiation into the basal rhizoid and proximal elongated blade) and attached to the respective female oogonium are considered fertilized diploid sporophytes, whereas sporophytes with malformed shapes, normally missing rhizoids and unattached to the female gametophyte were considered to derive from parthenogenesis (tom Dieck, 1992). Most partheno-sporophytes in Laminaria are generally unable to complete development and thus represent unsuccessful recruits (tom Dieck, 1992). The absolute number of normal and parthenogenetic sporophytes was counted in a minimum of 100 fields of view (Zeiss Observer D1 inverted microscope; 100× magnification) per replicate. Final female gametophyte density was determined on day 39.
Statistics
Gametophyte density was analysed by 2-way ANOVA with population and temperature as fixed factors using SPSS (version 27.0, IBM Corp, 2017). The proportion of reproductive females and sporophyte density of each population were expressed relative to the respective controls at 17°C to account for significant variation between populations, allowing comparisons amongst them. Normality of the data (Shapiro-Wilk test) and homogeneity of variances (Levene’s test) were tested before the statistical analyses. Estimated marginal means pairwise comparisons (Bonferroni-corrected) were performed to determine differences between treatments when a significant interaction was found. Differences were considered significant at p < 0.05. Three-way repeated measures ANOVA was performed using SPSS to test for differences in the percentage of female reproductive gametophytes and Fv/Fm over time between populations and temperatures. Data were tested with the Mauchly test for sphericity and Greenhouse Geisser or Huynh-Feldt corrections were used if sphericity could not be assumed.
The sporophyte density data did not fulfil Levene’s assumption of homogeneity of variance even after transformation. Therefore, data were analysed with the PERMANOVA module within PRIMER 7 software (Anderson, 2001; McArdle and Anderson, 2001) under a two-factor design with population and temperature as fixed factors. PERMDISP tests were performed for homogeneity of multivariate dispersion (around the centroid), which was verified without variable transformation for grouped factors population and temperature (P (perm) = 0.063). Pair-wise t-test comparisons were performed to identify differences between treatments when significant interactions were found. Data analysis was performed with Euclidian distances and 9999 permutations.
Results
Gametophyte survival
The final gametophyte density differed significantly due to the interaction of population × temperature (Table 2, Figure 3; F = 14.539, p < 0.001). The most striking effect in all populations was the significant decline in gametophyte density at the highest temperature (27°C) compared to the control temperature. Gametophytes from the FR population showed the greatest thermal sensitivity, with density reduction apparent at all temperatures between 23°C and 27°C compared to controls at 17°C. In contrast, gametophyte densities of the other Atlantic populations from SP and MO were only affected following the highest MHW temperature (27°C). The Mediterranean population (IT) showed a slightly different trend of increasing density following MHW at intermediate temperatures (significantly so at 25°C), before declining at 27°C to a level not significantly different from the control, which also showed reduced density over time (Figure 3).

Table 2 Two-way ANOVA for the effects of population and temperature on the gametophyte density of Laminaria ochroleuca after 38 days.
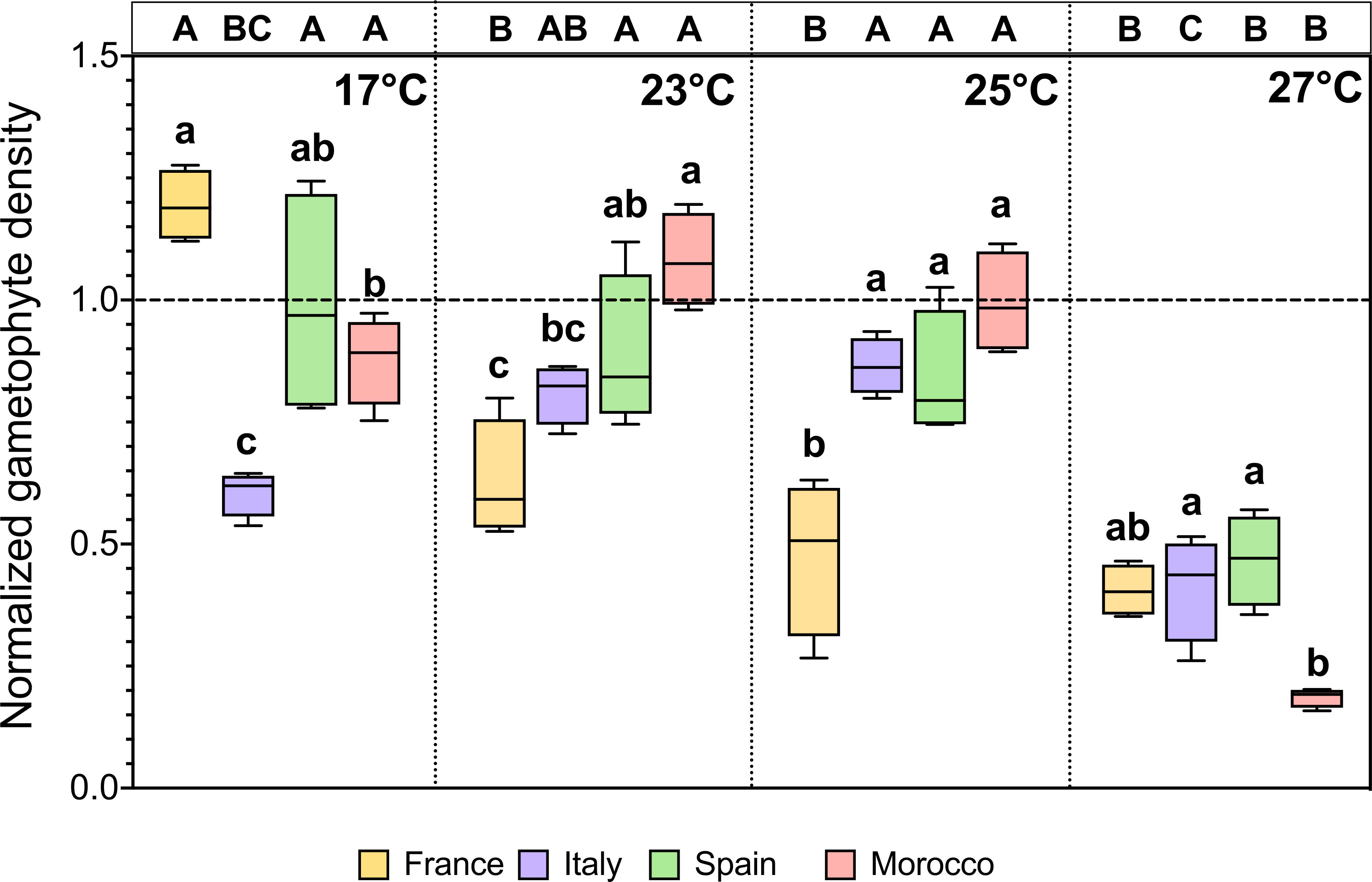
Figure 3 Gametophyte density from different populations (FR, IT, SP, MO) of Laminaria ochroleuca after recovery (day 38) at 17°C from different thermal treatments (17°C, 23°C, 25°C and 27°C). Note that density values were normalized to the respective initial value for each population (dotted line). Box plots with median, boxes for 25th and 75th percentiles and whiskers indicating min and max values (n = 4). For each temperature, different lowercase letters above boxplot bars indicate significant differences between populations. For each population, different uppercase letters indicate differences between temperatures (p < 0.05). See Table 2 for statistical analysis.
Photosynthetic efficiency during heat stress and recovery
The maximum quantum yield of photosystem II (Fv/Fm) showed significant time × population × temperature interactions (Table 3; F = 5.984, p < 0.001). Overall, during the 11 day simulated MHW Fv/Fm progressively decreased over time and with increasing MHW temperature (Figure 4), clearly indicating reduced maximum PSII efficiency resulting from photoinhibition and/or photodamage. At the end of recovery period (day 38), we observed some surprising effects with respect to the thermal history of the gametophytes: Fv/Fm in controls declined relative to initial values in all populations, suggesting the cumulative experimental irradiance (20 µmol m-2 s-1 PAR) induced progressive photoinhibition and/or photodamage, since temperature was a constant 17°C over this period (Figure 4A). Across all populations, Fv/Fm decreased (mean value of 0.298) in the gametophytes exposed to 17°C at the end of recovery compared to the initial 11 days (mean value of 0.561). Following intermediate MHW of 23 and 25°C, a trend for recovery of Fv/Fm after 38 d was observed, particularly in FR and IT populations, and less evident in MO (Figure 4B, C), while after the most severe MHW (27°C) all populations recovered to values not significantly different from initial Fv/Fm prior to the thermal stress (Figure 4D). Overall, although the mechanisms remain unclear, our results suggest that MHW of increasing intensity have positive effects on the resilience of PSII functioning at the population level, either through a positive interaction between irradiance and high temperature, selective effects of thermal stress favouring survival of the most resilient cells, or a shifting balance between mortality and production of new cells with increased resilience.
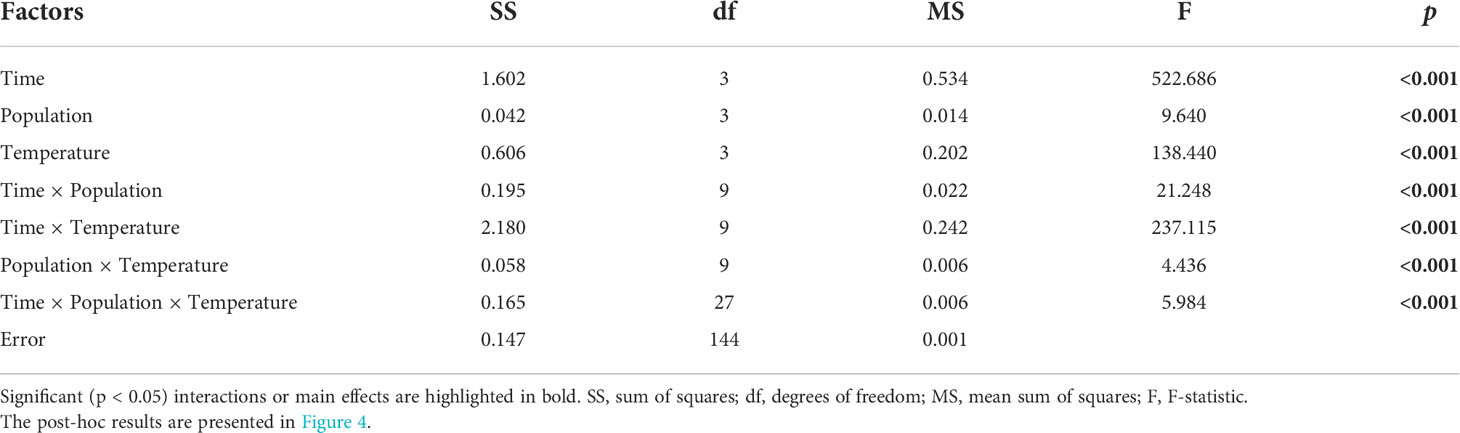
Table 3 Three-way repeated measures ANOVA for the effects of population, temperature, and time on the maximum quantum yield of photosystem II (Fv/Fm) of Laminaria ochroleuca gametophytes.
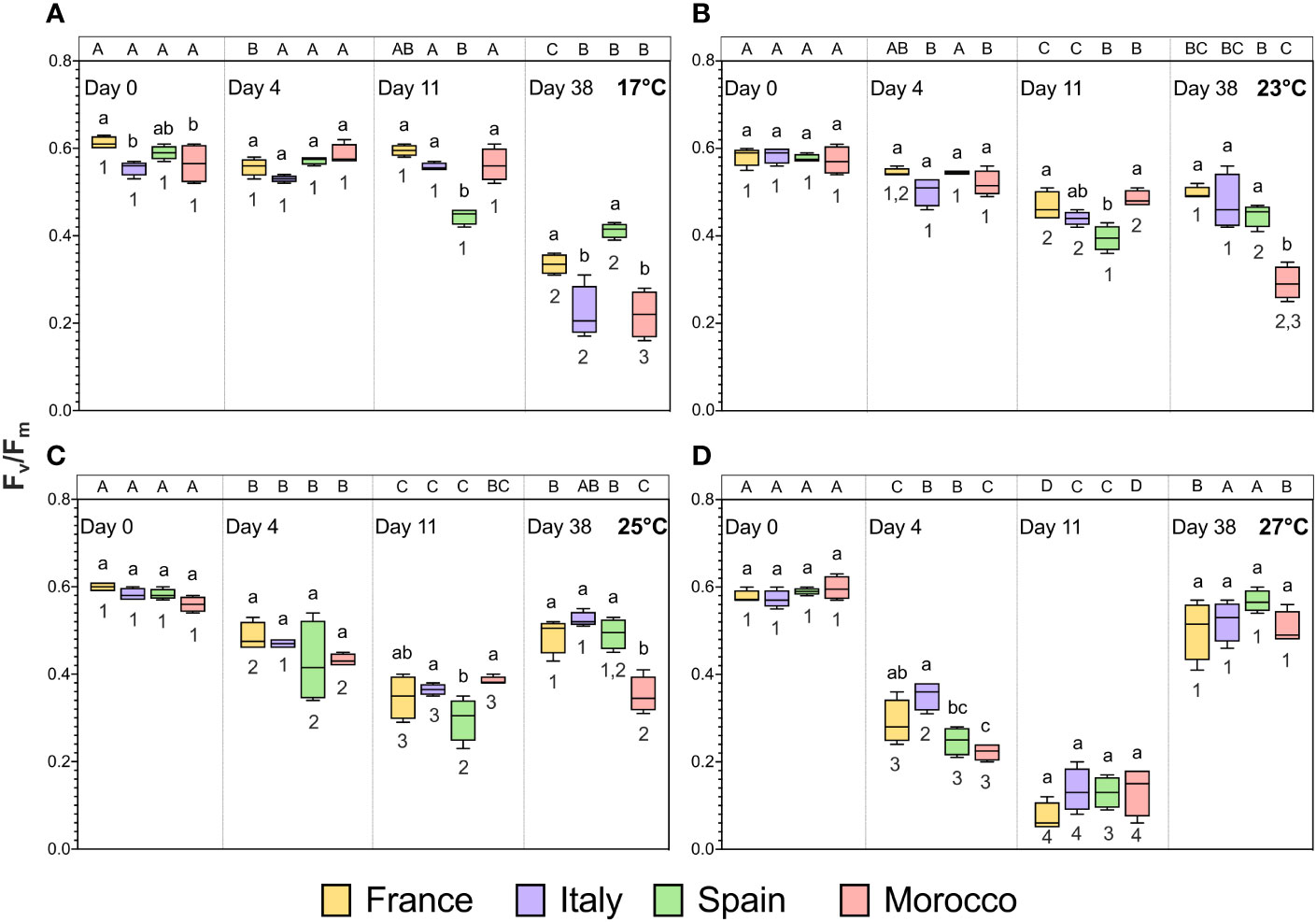
Figure 4 Maximum quantum yield of photosystem II (Fv/Fm) of Laminaria ochroleuca gametophytes from different populations (FR, IT, SP, MO) exposed to thermal treatments of 17°C (A), 23°C (B), 25°C (C) and 27°C (D) over time (heat wave: days 0, 4, 11 and recovery: day 38). Box plots with median, boxes for 25th and 75th percentiles and whiskers indicating min and max values (n = 4). For each temperature and time period, different lowercase letters above boxplot bars indicate significant differences between populations. For each population and temperature, different uppercase letters indicate differences between time periods. For each population and time period, different numbers indicate differences between temperatures (p < 0.05). See Table 3 for statistical analysis.
Gametophyte reproductive success during recovery from heat stress
The normalized female gametophyte reproductive success during the recovery phase showed significant time × population × temperature interactions (Table 4; F = 4.886, p < 0.001). On the first day of recovery (day 11), no reproduction was observed in the female gametophytes pre-exposed to all the HW treatments (23°C, 25°C and 27°C), indicating that the heat treatments delayed the onset of gametogenesis (Figure 5). From day 18 onwards, reproductive success significantly increased in the female gametophytes from FR and IT recovering from 23°C and 25°C, and from day 25 for those recovering from 27°C. In contrast, the SP (MHW of 23 and 25°C) and MO (MHW of 23°C) gametophytes remained mostly vegetative during the recovery phase (Figure 5A, B). In all four populations, the gametophytes pre-exposed to 27°C remained vegetative during the first two time periods (day 11 and 18), however the female reproduction significantly increased from day 25 onwards (Figure 5C). Notably, the SP and MO gametophytes pre-exposed to the MHW of 27°C exhibited the highest reproductive success from day 25 onwards (normalised reproduction ≥ 1), but with high variability compared to the other temperatures and populations and exceeded levels of reproductive success observed in their respective controls and in both FR and IT gametophyte populations (Figure 5C).
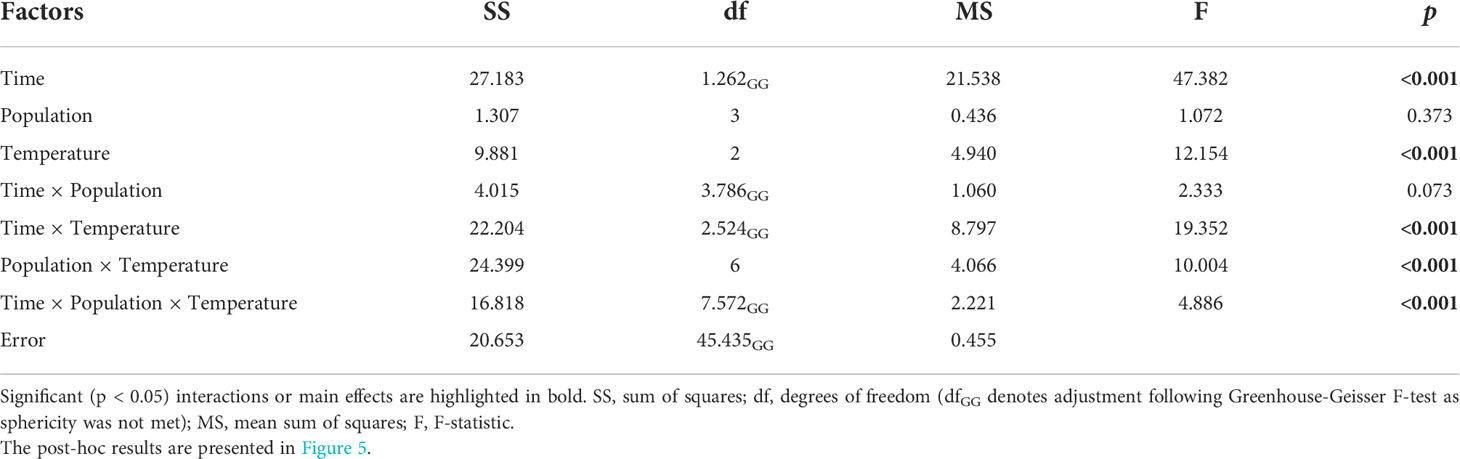
Table 4 Three-way repeated measures ANOVA for the effects of population, temperature, and time on the relative reproductive potential of female gametophytes of Laminaria ochroleuca.
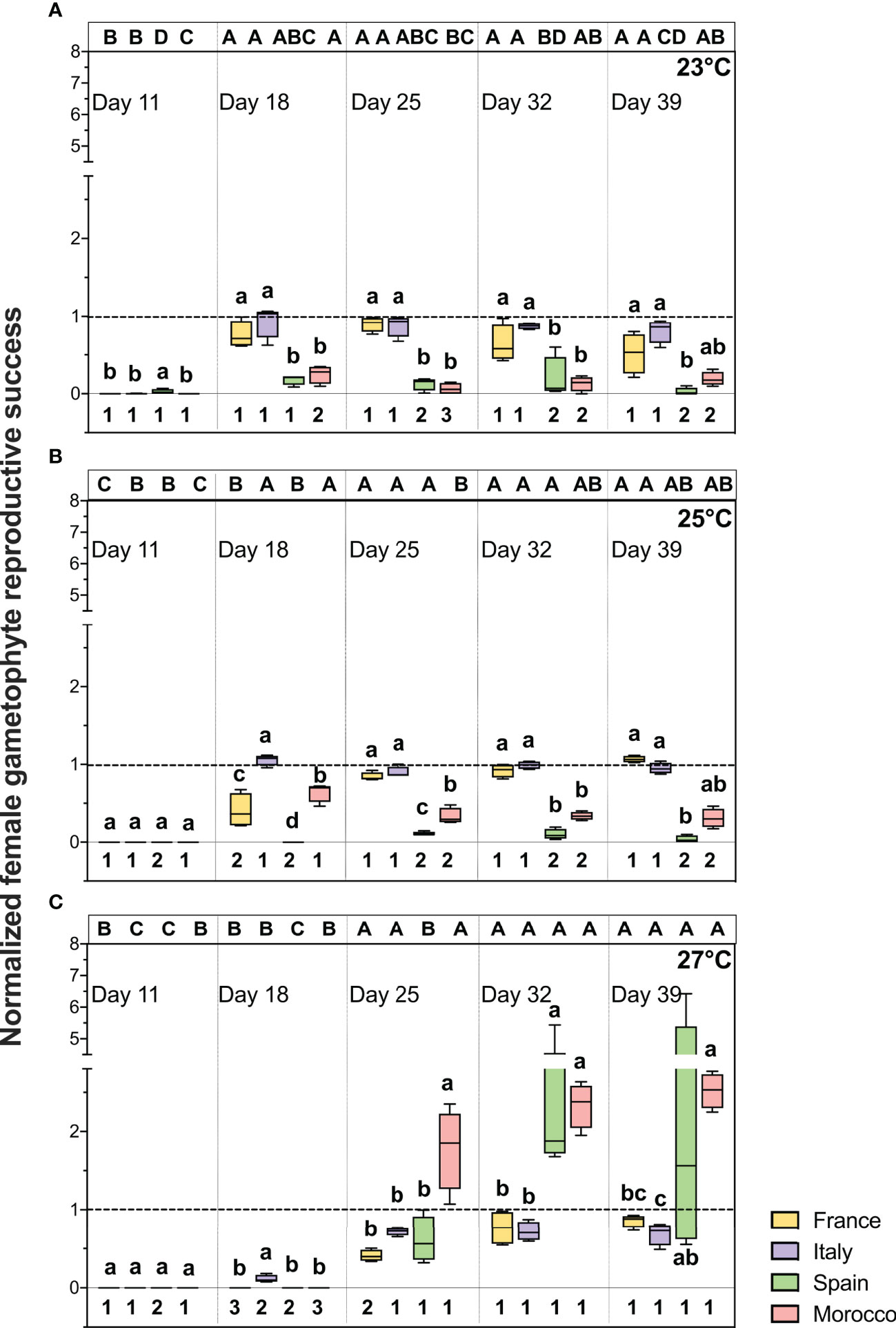
Figure 5 Female gametophyte reproductive success (proportion of female gametophytes with eggs and sporophytes) of different populations of Laminaria ochroleuca over recovery (day 39) at 17°C from MHW treatments of 23°C (A), 25°C (B) and 27°C (C). Note that the female reproductive values were normalized to the control treatment (17°C) value for each population (dotted line). Box plots with median, boxes for 25th and 75th percentiles and whiskers indicating min and max values (n = 4). For each temperature and time period, different lowercase letters above boxplot bars indicate significant differences between populations. For each population and temperature, different uppercase letters indicate differences between time periods. For each population and time period, different numbers indicate differences between temperatures (p < 0.05). See Table 4 for statistical analysis.
The normalized sporophyte density after 40 days showed significant population × temperature interactions (Table 5; pseudo-F = 2.2834; p = 0.011) and exhibits an overall similar pattern as the proportion of reproductive females (Figures 5, 6). Overall, extremely low normalised sporophyte densities (close to zero) were observed in the SP and MO gametophytes recovering from 23°C and 25°C compared to the FR and IT gametophytes (Figure 6), suggesting that intermediate heat stress negatively affects the subsequent reproductive success in these two populations. On the other hand, when pre-exposed to 27°C a high number of sporophytes developed (normalised density ≥ 1) in the gametophytes from the FR, SP and MO populations, indicating that a short pre-exposure to a critical high temperature enhanced subsequent sporophyte formation. In contrast to the Atlantic populations, sporophyte production in the Mediterranean IT population was temperature independent following simulated MHW exposure, and always lower than controls at 17°C. In the FR, SP and MO populations the gametophytes pre-exposed to 27°C had the highest sporophyte densities compared to 23°C.
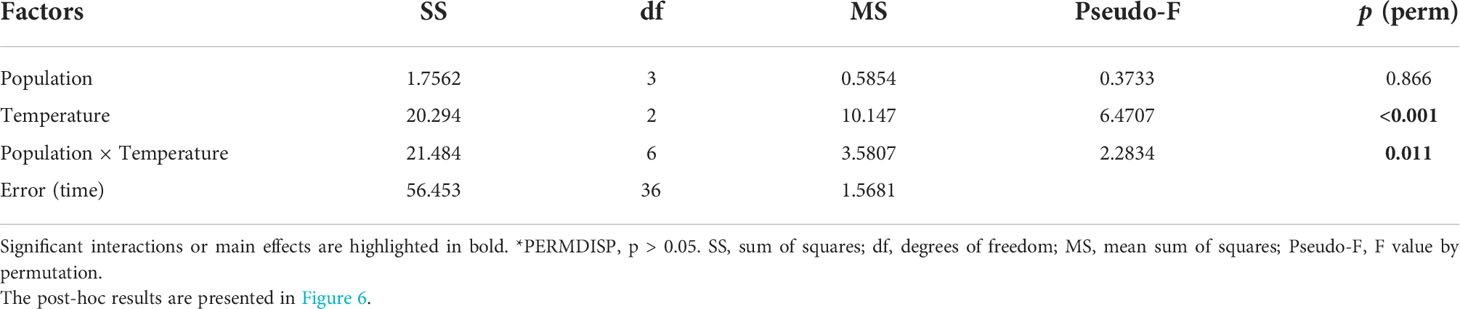
Table 5 PERMANOVA for the effects of population and temperature on the number of sporophytes per female gametophytes after 40 days in Laminaria ochroleuca.
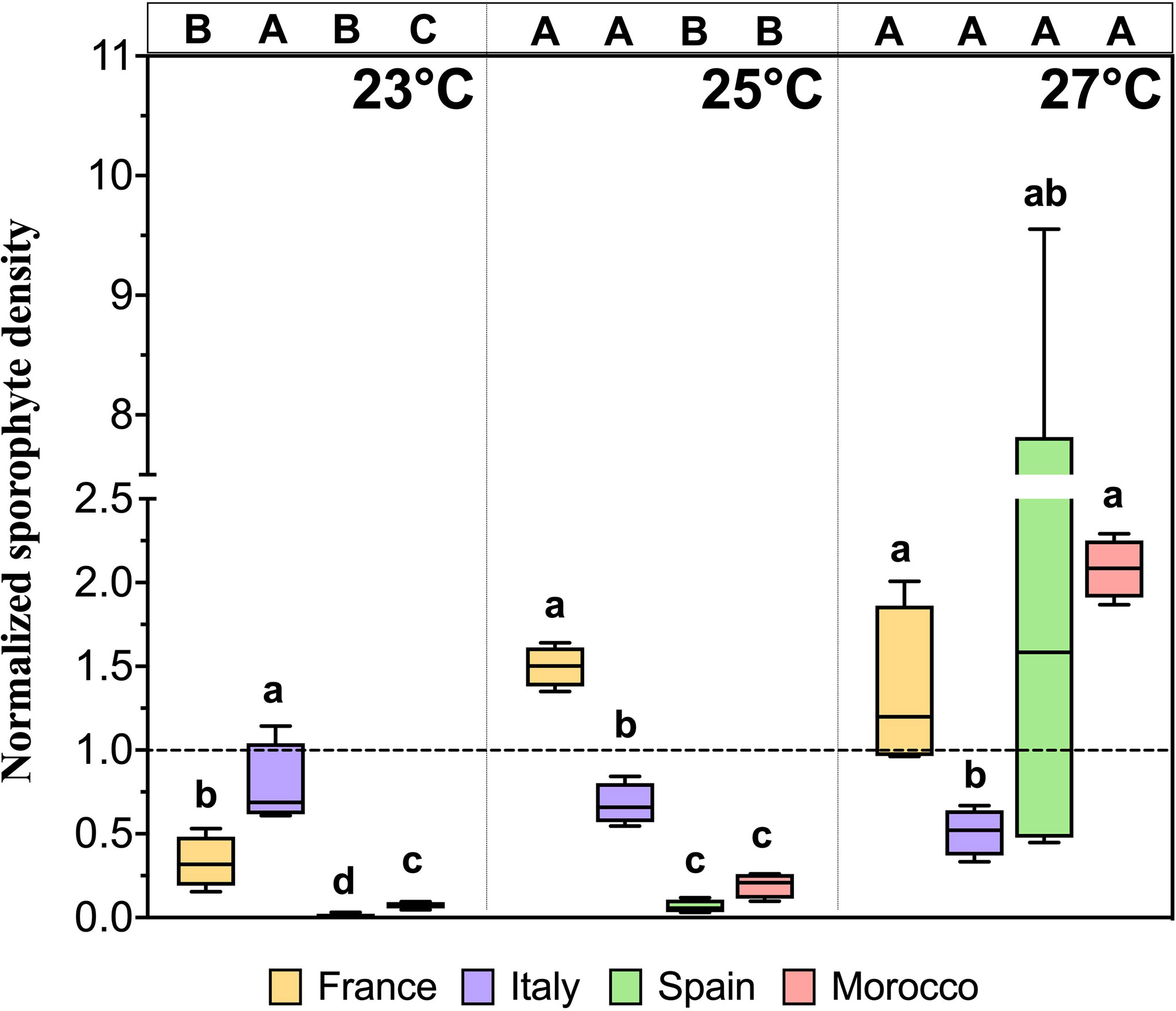
Figure 6 Absolute number of sporophytes per female gametophytes of different populations of Laminaria ochroleuca after recovery (day 40) from different MHW treatments. Note that the sporophyte density values were normalized to the mean control treatment value (17°C) for the respective population (dotted line). Box plots with median, boxes for 25th and 75th percentiles and whiskers indicating min and max values (n = 4). For each temperature, different lowercase letters above boxplot bars indicate significant differences between populations. For each population, different uppercase letters indicate differences between temperatures (p<0.05). See Table 5 for statistical analysis.
Interestingly, a considerable number of sporophytes with irregular morphology (i.e. likely partheno-sporophytes) developed after 40 days only in the FR gametophytes pre-exposed to 17°C (324 sporophytes cm-2), 23°C (1271 sporophytes cm-2), and 25°C (628 sporophytes cm-2) compared to sexually formed sporophytes (Supplementary Figure S2).
Discussion
This study revealed inter-population variability in the resilience, recovery, and subsequent reproductive success of microscopic gametophytes to thermal stress along the distribution range of L. ochroleuca, which potentially reflect evolutionary adaptations in response to local thermal regimes. The gametophytes from France (the most northern and cool-water population) were the most sensitive to thermal stress with mortality onset at 23°C compared to 27°C in the remaining populations. During recovery gametophytes from Spain and Morocco exhibited very low reproductive success after exposure to 23 and 25°C. However, when recovering from the highest thermal treatment (MHW of 27°C) the reproductive development and sporophyte output exceeded the levels obtained under control conditions (17°C). In contrast, the gametophytes from France and Italy (Mediterranean) pre-exposed to MHWs of 23°C, 25°C and 27°C were able to resume reproduction at levels similar to those of controls by the end of the recovery period. This highlights the need for fine scale regional data to accurately predict species response to future climate changes.
Gametophytes are the most thermally tolerant life cycle stage in kelps, withstanding higher temperatures than sporophytes (tom Dieck, 1992; tom Dieck, 1993; tom Dieck and Oliveira, 1993). The upper survival temperature of L. ochroleuca gametophytes was previously reported to be 25°C (tom Dieck, 1993). In our study, exposure to a simulated heat wave of 27°C negatively affected L. ochroleuca gametophyte density (more than 50% of mortality) in all four populations. However, the gametophytes from the most northern population, France, exhibited greater thermal sensitivity, with loss of gametophyte density (i.e., balance between growth and mortality) already apparent at 23°C. This intraspecific variation in gametophyte thermal tolerance suggests (potentially) adaptive divergence between populations of L. ochroleuca as all gametophytes were developed from meiospores under common garden conditions to remove the influence of previous thermal histories (although carry over effects cannot be discounted entirely, e.g., see Gauci et al., 2022). Inter-population variability (both adaptive and mal-adaptive) in the resistance to heat stress has been reported for other laminarian and fucoid macrophytes (Pearson et al., 2009; Saada et al., 2016; King et al., 2018). Locally adapted ecotypes were demonstrated for L. digitata, where trailing edge populations had a stronger heat shock response to thermal stress than range centre populations (King et al., 2019). In addition, gametophytes of L. digitata from the Arctic exhibited lower growth rates and reduced sporophyte recruitment after heat stress compared to Atlantic gametophytes (Martins et al., 2020). Evidence for adaptive variation was recently shown for the intertidal seaweed Hormosira banksii, as high temperatures (21°C) led to increased mortality in individuals from cooler locations, while individuals from warmer niches were relatively unaffected (Miller et al., 2019). Intraspecific variation in thermal tolerance was also found for Fucus vesiculosus, with southern individuals showing higher resilience to heat stress than northern ones (Saada et al., 2016).
It was recently shown that the northern populations (English Channel) of L. ochroleuca exhibit much lower genetic diversity and endemism compared to southern populations such as western Morocco (Assis et al., 2018). This skewed pattern of genetic diversity was found to be driven by past climate changes and oceanographic barriers (Lourenço et al., 2017; Assis et al., 2018). Genetic diversity can have substantial effects on the adaptive capacity of kelp populations to respond to environmental changes (Reed and Frankham, 2003; Coleman and Wernberg, 2020; Coleman and Wernberg, 2021; Vranken et al., 2021) as higher genetic diversity provides a greater range of functional responses (i.e., adaptive potential) and may increase the capacity to endure a stressor (Reusch et al., 2005; Wernberg et al., 2018). We may hypothesise that the lower resilience to heat stress of the gametophytes from the most northern population (France) might be associated with reduced genetic diversity. In the intertidal fucoid Fucus serratus, trailing edge populations showing reduced genetic diversity were also less resilient to desiccation and showed lower photosynthetic performance when exposed to heat shock compared to central populations (Pearson et al., 2009). In the seagrass Zostera marina, increased genetic diversity had a positive effect on shoot density after heat stress (Ehlers et al., 2008). Thus, the conservation of genetic diversity may be crucial for evolutionary adaptation of macrophytes to global climate change, but additional tests of this hypothesis are required.
Thermal stress causes photoinhibition in kelps (Bruhn and Gerard, 1996) and Fv/Fm is frequently used as an indicator of stress affecting photosystem II (PSII, Beer et al., 2014). The progressive decrease in Fv/Fm we observed in gametophytes with increasing temperature and exposure time during simulated MHWs in all populations of L. ochroleuca indicates dynamic downregulation (e.g., Pereira et al., 2015), or impaired function of PSII reaction centres in response to thermal stress (particularly at 27°C), potentially imposing individual fitness costs. Overall, gametophytes failed to fully recover Fv/Fm following simulated MHWs by the end of recovery period. Counterintuitively however, we observed an increasing trend for recovery of Fv/Fm with greater MHW temperature, notably including controls at 17°C, which were quite severely affected after 38 d. Indeed, full recovery to initial Fv/Fm values were only observed after moderate to severe MHW in gametophytes from Italy (25 and 27°C) and Spain (27°C).
Several factors may have contributed to these patterns. First, optimal thermal conditions for gametogenesis in the controls (tom Dieck, 1992; Izquierdo et al., 2002) and the earlier diversion of resources to reproduction may result in a trade-off between physiological performance and ontogeny, and/or competition for nutrients, space or light between gametophytes and microscopic sporophytes (Edwards and Connell, 2012). The inhibition of reproduction imposed by simulated MHWs may serve to delay this process, and partially explain higher Fv/Fm after the recovery period. In addition, the 27°C MHW reduced mean gametophyte density between > 50% (FR, SP, IT) to ca. 80% (MO), potentially alleviating sources of limitation. Furthermore, a positive interaction between irradiance and high temperature, selective effects of thermal stress favouring survival of the most resilient cells, or a shifting balance between mortality and production of new cells with increased resilience might also explain the positive effects of MHW of increasing intensity on the resilience of PSII functioning. Microscopic sporophytes are an actively growing early life stage, requiring nutrient levels that can exceed those of mature thalli (Thomas et al., 1985). Therefore, despite the medium being renewed twice through the experiment, the nutrient consumption by both life cycle stages (particularly by the actively growing sporophytes) might have created a nutrient deficit affecting physiological status. Negative effects of nutrient limitation on sporophyte photosynthesis have been described in several kelp species (Saccharina japonica: Gao et al., 2017; Liu et al., 2021; Macrocystis pyrifera: Fernández et al., 2020). Furthermore, potentially suboptimal or limiting irradiance for gametophytes developing beneath the growing sporophytes might have also contributed to the observed decrease in maximum photosynthetic efficiency of PSII.
The optimal reproductive development of L. ochroleuca gametophytes occurs between 11–18°C (tom Dieck, 1992; Izquierdo et al., 2002). However, studies investigating the influence of warming on the reproductive success of L. ochroleuca gametophytes are scarce (but see Pereira et al., 2011). In our study, simulated MHWs (23°C, 25°C and 27°C) prevented the onset of gametogenesis in all populations of L. ochroleuca, with gametophytes remaining in the vegetative state. Gametophytes can delay the formation of sexual cells and thus sporophyte production when exposed to unfavourable environmental conditions (tom Dieck, 1993; Carney and Edwards, 2010; Silva et al., 2022) such as extreme climatic events (Martins et al., 2020), but are able to resume their reproductive capacity as soon as conditions improve (Carney and Edwards, 2010; Martins et al., 2020). This may provide an advantage for populations living in habitats subjected to severe climatic events, which usually lead to drastic sporophyte mortality (Ladah et al., 1999; Barradas et al., 2011). However, the capacity of gametophytes to recover and reproduce after limiting conditions seems to be species-specific (Silva et al., 2022).
We found that the gametophyte potential to reproduce when recovering from heat stress varies among populations of L. ochroleuca. Overall, female gametophytes from Spain and Morocco showed very low ability to successfully reproduce and form sporophytes after exposure to 23°C and 25°C. In contrast, the gametophytes from France and Italy pre-exposed to 23°C, 25°C and 27°C were able to resume reproduction at levels similar to their respective controls by the end of the recovery period. Although, the gametophyte generation from each population were raised under common garden conditions from meiospores, intrinsic differences in reproductive investment after a heat stress were seen, which might suggest genetically based thermal responses (or strong carry-over effects) linked to their regional origin. Similarly, L. digitata gametophytes from the Atlantic produced a greater number of sporophytes during recovery from heat stress than those from the Arctic, suggesting differential thermal adaptation (Martins et al., 2020). Variations across populations in the reproductive response to temperature have previously been observed in the giant kelp (Macrocystis pyrifera) along its distributional range, indicating local adaption to thermal gradients (Hollarsmith et al., 2020).
Surprisingly, the gametophytes from Spain and Morocco exhibited the highest levels of reproductive success after the simulated MHW of 27°C, exceeding the reproductive levels obtained under control conditions (17°C). This extreme thermal treatment might have promoted the subsequent gametogenesis via an ultimate stress-induced survival mechanism, similar to heat-stimulated bolting and flowering observed in plants (Arabidopsis thaliana, Balasubramanian et al., 2006; Tonsor et al., 2008) and seagrasses (Blok et al., 2018; Ruiz et al., 2018; Marín-Guirao et al., 2019). Alternatively, thermal stress may have resulted in selective survival of extreme thermotolerant genotypes, a particularly interesting hypothesis for the core, high genetic diversity population from MO (Assis et al., 2018). The high variability in the MO and SP gametophyte reproductive success after the 27°C MHW compared to the other thermal treatments and populations might be a consequence of individual phenotypic plasticity. While it is tempting to search for population-specific reproductive responses that might be associated with regional thermal regimes, such conclusions are difficult to draw. For example, although reproductive success in the relatively cool and stenothermal IT population was poor after exposure to MHW > 23°C, the cool-water Atlantic population from FR was relatively successful after moderate to high MHW exposure. Similarly, gametophytes from the warmest thermal niches (SP and MO) successfully reproduced after a 27°C MHW, but were very unsuccessful at 23 and 25°C. Moreover, reproduction at 27°C may be a non-adaptive stress-induced response, since local seawater temperatures in Spain and Morocco do not naturally reach 27°C. There remains the potential for inbreeding depression to affect the thermal performance of the gametophytes that derived from a reduced number of fertile sporophytes (IT: 6 individuals; FR, SP and MO: 5 individuals). While negative effects on fecundity and reproduction due to inbreeding have been observed at least one other kelp (Macrocystis pyrifera; Raimondi et al., 2004), we are unaware of any analysis of inbreeding depression affecting temperature tolerance in L. ochroleuca. Similar thermal tolerance limits were detected in the kelp Alaria esculenta when using 4 donor sporophytes (Martins et al., 2022) or a wider gene pool (14 sporophytes; Fredersdorf et al., 2009), suggesting absence of inbreeding depression in this kelp species. Similarly, in the sea palm Postelsia palmaeformis there were low costs of self-fertilization in individuals size and reproduction (Barner et al., 2011).
Species of the order Laminariales can develop sporophytes via parthenogenesis from unfertilized eggs (tom Dieck, 1992; Oppliger et al., 2007; Martins et al., 2019). Most parthenogenetic sporophytes have an irregular morphology and show high mortality rates (Ar Gall et al., 1996), however in some species adult fertile partheno-sporophytes with normal morphology develop (Shan et al., 2013). In the most northern population of L. ochroleuca (France) that displays less genetic diversity, partheno-sporophytes sensu tom Dieck (1992) developed in controls at 17°C. Subsequent exposure to MHWs of 23°C and 25°C further increased the number of partheno-sporophytes. Species adaptation to marginal habitats has often been associated with parthenogenetic reproduction (Kawecki, 2008). It would be interesting to understand whether the geographical parthenogenesis found in L. ochroleuca (i.e., partheno-sporophytes developing near the northern distributional edge) plays an adaptive role in a marginal ecological niche, contributing to the geographic distribution of the species over evolutionary time. Similarly, a southern-limit population of L. digitata showed a high propensity for producing unreduced spores, a consequence of the life in a marginal habitat (Oppliger et al., 2014).
The in-vitro experimental setup using gametophytes raised under common garden conditions has shown to be efficient in revealing L. ochroleuca population differences in thermal traits while excluding the influence of environmental histories (but not potential transgenerational epigenetic effects). The study highlights the need for species distribution and climate niche models to incorporate intraspecific variation to generate more reliable predictions of species responses to future climates. Deciphering intraspecific variation may also provide valuable insight for future management of wild and farmed kelp populations, incorporating evolutionary potential for “climate-proofing” marine ecosystems.
Data availability statement
The raw data supporting the conclusions of this article will be made available by the authors, without undue reservation.
Author contributions
NM, GP, ES, BS, LB planned the main research question. F-ES, LB, GP and NM planned and designed the experiments. LB, NM, SK performed field sampling. F-ES conducted the experimental work and analysed the data, and LB the photosynthetic measurements. F-ES and NM wrote the manuscript. F-ES, LB, ES, GP and NM contributed to the data interpretation and discussion. All authors contributed to reviewing and revising the final manuscript and approved the submitted version.
Funding
This study received Portuguese national funds from FCT - Foundation for Science and Technology through SFRH/BPD/122567/2016 to NM (in transitional norm DL 57/2016/CP1361/CT0039), SFRH/BD/139189/2018 to LB, project UIDB/04326/2020, UIDP/04326/2020 and LA/P/0101/2020 and from the operational programmes CRESC Algarve 2020 and COMPETE 2020 through project EMBRC.PT ALG-01-0145-FEDER-022121. NM has received funding from H2020 (EU) through the Transnational Access program from ASSEMBLE PLUS (EC grant agreement No 730984) for the sampling in Messina, Italy. FCT Portugal – CNRST Morocco bilateral cooperation project ‘Variation of marine-forests traits in range-edge vs core NE Atlantic upwelling refugia in a context of climatic change’. Ref. 0504/19/CNR, 2019-2021. RESTORESEAS (Portuguese funds to EU-BiodivERsA BiodivRestore-253 - FCT DivRestore/0013/2020). Water JPI. MARAFRICA: AGA-KHAN/540316524/2019, and by a Pew Marine Fellowship to ES.
Acknowledgments
We would like to thank Francesco Smedile from the Istituto per le Risorse Biologiche e le Biotecnologie Marine (CNR - IRBIM) for the help provided during the field sampling in Messina. We would like to thank Myriam Valero for providing divers and laboratory space and resources during field sampling in Roscoff. We would like to thank João Neiva for providing assistance in the field sampling and laboratory processing in Morocco. We would like to thank both reviewers for the constructive comments and suggestions that greatly improved the quality of the manuscript.
Conflict of interest
The reviewer FA declared a past collaboration with one of the authors ES to the handling Editor.
The remaining authors declare that the research was conducted in the absence of any commercial or financial relationships that could be construed as a potential conflict of interest.
Publisher’s note
All claims expressed in this article are solely those of the authors and do not necessarily represent those of their affiliated organizations, or those of the publisher, the editors and the reviewers. Any product that may be evaluated in this article, or claim that may be made by its manufacturer, is not guaranteed or endorsed by the publisher.
Supplementary material
The Supplementary Material for this article can be found online at: https://www.frontiersin.org/articles/10.3389/fmars.2022.943511/full#supplementary-material
References
Anderson M. J. (2001). A new method for non-parametric multivariate analysis of variance. Austral. Ecol. 26, 32–46.
Anderson A. B., Assis J., Batista M. B., Serrão E. A., Guabiroba H. C., Delfino S. D. T., et al. (2021). Global warming assessment suggests the endemic Brazilian kelp beds to be an endangered ecosystem. Mar. Environ. Res. 168, 1–9. doi: 10.1016/j.marenvres.2021.105307
Applebaum S. L., Pan T. C. F., Hedgecock D., Manahan D. T. (2014). Separating the nature and nurture of the allocation of energy in response to global change. Integr. Comp. Biol. 54 (2), 284–295. doi: 10.1093/icb/icu062
Ar Gall E., Le Gall Y., Asensi A., Marie D., Kloareg B. (1996). Parthenogenesis and apospory in the laminariales: a flow cytometry analysis. Eur. J. Phycol. 31, 369–380. doi: 10.1080/09670269600651601
Assis J., Coelho N. C., Lamy T., Valero M., Alberto F., Serrão E.Á. (2016). Deep reefs are climatic refugia for genetic diversity of marine forests. J. Biogeogr. 43 (4), 833–844. doi: 10.1111/jbi.12677
Assis J., Serrão E.Á., Coelho N. C., Tempera F., Valero M., Alberto F. (2018). Past climate changes and strong oceanographic barriers structured low-latitude genetic relics for the golden kelp laminaria ochroleuca. J. Biogeogr. 45 (10), 2326–2336. doi: 10.1111/jbi.13425
Assis J., Tavares D., Tavares J., Cunha A., Alberto F., Serrão E. A. (2009). Findkelp, a GIS-based community participation project to assess Portuguese kelp. J. Coast. Res II (56), 1469–1473.
Assis J., Tyberghein L., Bosch S., Verbruggen H., Serrão E. A., De Clerck O. (2017). Bio-ORACLE v2.0: Extending marine data layers for bioclimatic modelling. Global Ecol. Biogeogr. 27 (3), 277–284. doi: 10.1111/geb.12693
Balasubramanian S., Sureshkumar S., Lempe J., Weigel D. (2006). Potent induction of arabidopsis thaliana flowering by elevated growth temperature. PloS Genet. 2 (7), 0980–0989. doi: 10.1371/journal.pgen.0020106
Barner A. K., Pfister C. A., Timothy Wootton J. (2011). The mixed mating system of the sea palm kelp postelsia palmaeformis: Few costs to selfing. Proc. R. Soc. B: Biol. Sci. 278 (1710), 1347–1355. doi: 10.1098/rspb.2010.1928
Barradas A., Alberto F., Engelen A. H., Serrão E. A. (2011). Fast sporophyte replacement after removal suggests banks of latent microscopic stages of Laminaria ochroleuca (Phaeophyceae) in tide pools in northern Portugal. Cahiers Biol Mar. 52, 435–439.
Bartsch I., Vogt J., Pehlke C., Hanelt D. (2013). Prevailing Sea surface temperatures inhibit summer reproduction of the kelp Laminaria digitata at helgoland (North Sea). J. Phycol. 49, 1061–1073. doi: 10.1111/jpy.12125
Beer S., Björk M., Beardall J. (2014). Photosynthesis in the marine environment. (Iowa: Wiley Blackwell).
Bellard C., Bertelsmeier C., Leadley P., Thuiller W., Courchamp F. (2012). Impacts of climate change on the future of biodiversity. Ecol. Lett. 15 (4), 365–377. doi: 10.1111/J.1461-0248.2011.01736.X
Birkett D. A., Maggs C. A., Dring M. J., Boaden P. J. S. (1998). Infralittoral reef biotipes with kelp species (volume VII). an overview of dynamic and sensivity characteristics for conservation management of marine SAC’s. Scottish Assoc. Mar. Sci. (UK Mar. SACs Project) VII (August), 1–174.
Blok S. E., Olesen B., Krause-Jensen D. (2018). Life history events of eelgrass zostera marina l. populations across gradients of latitude and temperature. Mar. Ecol. Prog. Ser. 590, 79–93. doi: 10.3354/MEPS12479
Bolton J. J. (2010). The biogeography of kelps (Laminariales, phaeophyceae): A global analysis with new insights from recent advances in molecular phylogenetics. Helgoland Mar. Res. 64 (4), 263–279. doi: 10.1007/s10152-010-0211-6
Bruhn J., Gerard V. A. (1996). Photoinhibition and recovery of the kelp laminaria saccharina at optimal and superoptimal temperatures. Mar. Biol. 125 (4), 639–648. doi: 10.1007/BF00349245
Buonomo R., Assis J., Fernandes F., Engelen A. H., Airoldi L., Serrão E. A. (2016). Habitat continuity and stepping-stone oceanographic distances explain population genetic connectivity of the brown alga cystoseira amentacea. Mol. Ecol. 26 (3), 766–780. doi: 10.1111/mec.13960
Carney L. T., Edwards M. S. (2010). Role of nutrients fluctuations and delayed development in gametophyte reproduction by Macrocystis pyrifera (Phaeophyceae) in southern California. J. Phycol. 46, 987–996. doi: 10.1111/j.1529-8817.2010.00882.x
Coleman M. A. (2013). Connectivity of the habitat-forming kelp, ecklonia radiata within and among estuaries and open coast. PloS One 8 (5), e64667. doi: 10.1371/JOURNAL.PONE.0064667
Coleman M. A., Wernberg T. (2020). The silver lining of extreme events. Trends Ecol. Evol. 35, 1065–1067. doi: 10.1016/j.tree.2020.08.013
Coleman M. A., Wernberg T. (2021). A glass half full: solutions-oriented management under climate change. Trends Ecol. Evol. 36, 385–386. doi: 10.1016/j.tree.2021.02.009
Colin C., Leblanc C., Wagner E., Delage L., Leize-Wagner E., Dorsselaer A., et al. (2003). The brown algal kelp laminaria digitata features distinct bromoperoxidase and iodoperoxidase activities *. J. Biol. Chem. 278 (26), 23545–23552. doi: 10.1074/JBC.M300247200
Dayton P. K. (1985). Ecology of kelp communities. Annu. Rev. Ecol. Syst 16 (1985), 215–245. doi: 10.1146/annurev.es.16.110185.001243
Denny M. (2017). The fallacy of the average: On the ubiquity, utility and continuing novelty of jensen’s inequality. J. Exp. Biol. 220 (2), 139–146. doi: 10.1242/jeb.140368
Duarte G. A. S., Villela H. D. M., Deocleciano M., Silva D., Barno A., Cardoso P. M., et al. (2020). Heat waves are a major threat to turbid coral reefs in Brazil. Front. Mar. Sci. 7, 179. doi: 10.3389/fmars.2020.00179
Edwards M. S., Connell S. D. (2012). “Competition, a major factor structuring seaweed communities,” in Seaweed biology. ecological studies, vol 219. Eds. Wiencke C. (Berlin, Heidelberg: Springer). doi: 10.1007/978-3-642-28451-9_7
Ehlers A., Worm B., Reusch T. B. H. (2008). Importance of genetic diversity in eelgrass zostera marina for its resilience to global warming. Mar. Ecol. Prog. Series. 355, 1–7. doi: 10.3354/meps07369
Fernández P. A., Gaitán-Espitia J. D., Leal P. P., Schmid M., Revill A. T., Hurd C. L. (2020). Nitrogen sufficiency enhances thermal tolerance in habitat-forming kelp: implications for acclimation under thermal stress. Sci. Rep. 10, 3186. doi: 10.1038/s41598-020-60104-4
Flores-Moya A. (2012). Warm temperate seaweed communities: A case study of deep water kelp forests from the alboran Sea (SW Mediterranean Sea) and the strait of Gibraltar (Berlin, Heidelberg: Springer), 315–327. doi: 10.1007/978-3-642-28451-9_15
Fredersdorf J., Müller R., Becker S., Wiencke C., Bischof K. (2009). Interactive effects of radiation, temperature and salinity on different life history stages of the Arctic kelp alaria esculenta (Phaeophyceae). Oecologia. 160 (3), 483–492. doi: 10.1007/s00442-009-1326-9
Gao X., Endo H., Nagaki M., Agatsuma Y. (2017). Interactive effects of nutrient availability and temperature on growth and survival of different size classes of saccharina japonica (Laminariales, phaeophyceae). Phycologia 56:3, 253–260. doi: 10.2216/16-91.1
Garrabou J., Coma R., Bensoussan N., Bally M., Chevaldonné P., Cigliano M., et al. (2009). Mass mortality in northwestern Mediterranean rocky benthic communities: Effects of the 2003 heat wave. Global Change Biol. 15 (5), 1090–1103. doi: 10.1111/j.1365-2486.2008.01823.x
Gauci C., Bartsch I., Martins N., Liesner D. (2022). Cold thermal priming of Laminaria digitata (Laminariales, phaeophyceae) gametophytes enhances gametogenesis and thermal performance of sporophytes. Front. Mar. Sci. 9. doi: 10.3389/fmars.2022.862923
Graham M. H., Vasquez J. A., Buschmann A. (2007). Oceanography and marine biology: An annual review. Oceanog. Mar. Biol: Annu. Rev. (Vol. 49 pp, 39–88). doi: 10.1016/0025-326x(94)90189-9
Hobday A. J., Alexander L. V., Perkins S. E., Smale D. A., Straub S. C., Oliver E. C. J., et al. (2016). A hierarchical approach to defining marine heatwaves. Prog. Oceanog. 141, 227–238. doi: 10.1016/j.pocean.2015.12.014
Hollarsmith J. A., Buschmann A. H., Camus C., Grosholz E. D. (2020). Varying reproductive success under ocean warming and acidification across giant kelp (Macrocystis pyrifera) populations. J. Exp. Mar. Biol. Ecol. 522, 151247. doi: 10.1016/j.jembe.2019.151247
Izquierdo J., Pérez-Ruzafa I. M., Gallardo T. (2002). Effect of temperature and photon fluence rate on gametophytes and young sporophytes of Laminaria ochroleuca pylaie. Helgol Mar. Res. 55, 285–292. doi: 10.1007/s10152-001-0087-6
Kawecki T. J. (2008). “Adaptation to marginal habitats,” in Annual review of ecology, evolution, and systematics, 39, 321–342. doi: 10.1146/annurev.ecolsys.38.091206.095622
King N. G., McKeown N. J., Smale D. A., Moore P. J. (2018). The importance of phenotypic plasticity and local adaptation in driving intraspecific variability in thermal niches of marine macrophytes. Ecography 41 (9), 1469–1484. doi: 10.1111/ecog.03186
King N. G., McKeown N. J., Smale D. A., Wilcockson D. C., Hoelters L., Groves E. A., et al. (2019). Evidence for different thermal ecotypes in range centre and trailing edge kelp populations. J. Exp. Mar. Biol. Ecol. 514–515, 10–17. doi: 10.1016/j.jembe.2019.03.004
Ladah L. B., Zertruche-Gonzalez J. A. (2007). Survival of microscopic stages of a perennial kelp (Macrocystis pyrifera) from the center and the southern extreme of its range in the northern hemisphere after exposure to simulated El niño stress. Mar. Biol. 152, 677–686. doi: 10.1007/s00227-007-0723-z
Ladah L. B., Zertuche-González J. A., Hernandez- Carmona G. (1999). Giant kelp (Macrocystis pyrifera, phaeophyceae) recruitment near its southern limit in Baja California after mass disappearance during ENSO 1997–1998. J. Phycol. 35, 1106–1112. doi: 10.1046/j.1529-8817.1999.3561106.x
Lane C. E., Mayes C., Druehl L. D., Saunders G. W. (2006). A multi-gene molecular investigation of the kelp (Laminariales, phaeophyceae) supports substantial taxonomic re-organization. J. Phycol. 42 (2), 493–512. doi: 10.1111/J.1529-8817.2006.00204.X
Linhart Y. B., Grant M. C. (1996). Evolutionary significance of local genetic differentiation in plants. Annu. Rev. Ecol. Syst. 27, 237–277. doi: 10.1146/annurev.ecolsys.27.1.237
Liu Y., Cao J., Chu Y., Liu Y., Wang Q., Gong Q., et al. (2021). The brown algae saccharina japonica and sargassum horneri exhibit species-specific responses to synergistic stress of ocean acidification and eutrophication. J. Ocean Univ. China. 20, 1253–1262. doi: 10.1007/s11802-021-4853-6
Lourenço C. R., Nicastro K. R., McQuaid C. D., Chefaoui R. M., Assis J., Taleb M. Z., et al. (2017). Evidence for rangewide panmixia despite multiple barriers to dispersal in a marine mussel. Sci. Rep. 7 (1), 1–16. doi: 10.1038/s41598-017-10753-9
Lourenço C. R., Zardi G. I., McQuaid C. D., Serrão E. A., Pearson G. A., Jacinto R., et al. (2016). Upwelling areas as climate change refugia for the distribution and genetic diversity of a marine macroalga. J. Biogeogr. 43 (8), 1595–1607. doi: 10.1111/jbi.12744
Marín-Guirao L., Entrambasaguas L., Ruiz J. M., Procaccini G. (2019). Heat-stress induced flowering can be a potential adaptive response to ocean warming for the iconic seagrass posidonia oceanica. Mol. Ecol. 28 (10), 2486–2501. doi: 10.1111/MEC.15089
Martins N., Barreto L., Bartsch I., Bernard J., Serrão E., Pearson G. (2022). Daylength influences reproductive success and sporophyte growth in the Arctic kelp species alaria esculenta. Mar. Ecol. Prog. Ser. 683, 37–52. doi: 10.3354/meps13950
Martins N., Pearson G. A., Bernard J., Serrão E. A., Bartsch I. (2020). Thermal traits for reproduction and recruitment differ between Arctic and Atlantic kelp Laminaria digitata. PLoS ONE. 16 (6), e0235388. doi: 10.1371/journal.pone.0235388
Martins N., Pearson G. A., Gouveia L., Tavares A. I., Ester A., Bartsch I. (2019). Hybrid vigour for thermal tolerance in hybrids between the allopatric kelps Laminaria digitata and L. pallida (Laminariales, phaeophyceae) with contrasting thermal affinities. Eur. J. Phycol. 54 (4), 1–14. doi: 10.1080/09670262.2019.1613571
Martins N., Tanttu H., Pearson G. A., Serrão E. A., Bartsch I. (2017). Interactions of daylength, temperature and nutrients affect thresholds for life stage transitions in the kelp Laminaria digitata (Phaeophyceae). Botanica Marina 60, 109–121. doi: 10.1515/bot-2016-0094
McArdle B. H., Anderson M. J. (2001). Fitting multivariate models to community data: a comment on distance-based redundancy analysis. Ecology 82, 290–297. doi: 10.1890/0012-9658(2001)082[0290:FMMTCD]2.0.CO;2
McPherson M. L., Finger D. J. I., Houskeeper H. F., Bell T. W., Carr M. H., Rogers-Bennett L., et al. (2021). Large-Scale shift in the structure of a kelp forest ecosystem co-occurs with an epizootic and marine heatwave. Commun. Biol. 4 (1), 1–9. doi: 10.1038/s42003-021-01827-6
Miller A. D., Coleman M. A., Clark J., Cook R., Naga Z., Doblin M. A., et al. (2019). Local thermal adaptation and limited gene flow constrain future climate responses of a marine ecosystem engineer. Evol Appl. 13 (5), 918–934. doi: 10.1111/EVA.12909
Oppliger L. V., Correa J. A., Peters A. F. (2007). Parthenogenesis in the brown alga Lessonia nigrescens (Laminariales, phaeophyceae) from central Chile. J. Phycol. 43, 1295–1301. doi: 10.1111/j.1529-8817.2007.00408.x
Oppliger L. V., von Dassow P., Bouchemousse S., Robuchon M., Valero M., Correa J. A., et al. (2014). Alteration of sexual reproduction and genetic diversity in the kelp species Laminaria digitata at the southern limit of its range. PloS One 9, e102518. doi: 10.1371/journal.pone.0102518
Pearman P. B., D’Amen M., Graham C. H., Thuiller W., Zimmermann N. E. (2010). Within-taxon niche structure: niche conservatism, divergence and predicted effects of climate change. Ecography. 33 (6), 990–1003. doi: 10.1111/J.1600-0587.2010.06443.X
earson G. A., Lago-Leston A., Mota C. (2009). Frayed at the edges: Selective pressure and adaptive response to abiotic stressors are mismatched in low diversity edge populations. J. Ecol. 97 (3), 450–462. doi: 10.1111/j.1365-2745.2009.01481.x
Pereira T. R., Engelen A. H., Pearson G. A., Serrão E. A., Destombe C., Valero M. (2011). Temperature effects on the microscopic haploid stage development of Laminaria ochroleuca and Sacchoriza polyschides, kelps with contrasting life histories. Cah. Biol. Mar. 52, 395–403.
Pereira T. R., Engelen A. H., Pearson G. A., Valero M., Serrão E. A. (2015). Response of kelps from different latitudes to consecutive heat shock. Journal of experimental marine biology and ecology. 463, 57–62. doi: 10.1016/j.jembe.2014.10.022
Pereyra R. T., Huenchuñir C., Johansson D., Forslund H., Kautsky L., Jonsson P. R., et al. (2013). Parallel speciation or long-distance dispersal? lessons from seaweeds (Fucus) in the Baltic Sea. J. Evol Biol. 26 (8), 1727–1737. doi: 10.1111/JEB.12170
Provasoli L.. (1968). “Media and prospects for the cultivation of marine algae. in Cultures and Collections of Algae,” in Proceedings of the US-Japan Conference, Hakone, September 1966, eds A. Watanabe, and A. Hattori (Tokyo: Japan Society of Plant Physiology) 63–75
Ramos M., Bertocci I., Tempera F., Calado G., Albuquerque M., Duarte P. (2016). Patterns in megabenthic assemblages on a seamount summit (Ormonde peak, gorringe bank, northeast Atlantic). Mar. Ecol. 37 (5), 1057–1072. doi: 10.1111/MAEC.12353
Raimondi P. T., Reed D. C., Gaylord B., Washburn L. (2004). Effects of self-fertilization in the giant kelp, macrocystis pyrifera. Ecology 85, 3267–3276. doi: 10.1890/03-0559
Reed D. H., Frankham R. (2003). Correlation between fitness and genetic diversity. Conserv. Biol. 17, 230–237. doi: 10.1046/j.1523-1739.2003.01236.x
Reusch T. B. H., Ehlers A., Hammerli A., Worm B. (2005). Ecosystem recovery after climatic extremes enhanced by genotypic diversity. Proc. Natl. Acad. Sci. 102, 2826–2831. doi: 10.1073/pnas.0500008102
Rothman M. D., Mattio L., Anderson R. J., Bolton J. J. (2017). A phylogeographic investigation of the kelp genus laminaria (Laminariales, phaeophyceae), with emphasis on the south Atlantic ocean. J. Phycol. 53 (4), 778–789. doi: 10.1111/jpy.12544
Ruiz J. M., Marín-Guirao L., García-Muñoz R., Ramos-Segura A., Bernardeau-Esteller J., Pérez M., et al. (2018). Experimental evidence of warming-induced flowering in the Mediterranean seagrass posidonia oceanica. Mar. pollut. Bull. 134, 49–54. doi: 10.1016/J.MARPOLBUL.2017.10.037
Saada G., Nicastro K. R., Jacinto R., McQuaid C. D., Serrao E. A., Pearson G. A., et al. (2016). Taking the heat: Distinct vulnerability to thermal stress of central and threatened peripheral lineages of a marine macroalga. Divers. Distrib. 22, 1060–1068. doi: 10.1111/ddi.12474
Santelices B. (2007). The discovery of kelp forests in deep-water habitats of tropical regions. Proc. Natl. Acad. Sci. 104 (49), 19163–19164. doi: 10.1073/PNAS.0708963104
Shan T. F., Pang S. J., Gao S. Q. (2013). Novel means for variety breeding and sporeling production in the brown seaweed Undaria pinnatifida (Phaeophyceae): crossing female gametophytes from parthenosporophytes with male gametophytes clones. Phycol Res. 61, 154–161. doi: 10.1111/pre.12014
Silva C. F., Pearson G. A., Serrão E. A., Bartsch I., Martins N. (2022). Microscopic life stages of Arctic kelp differ in their resilience and reproductive output in response to Arctic seasonality. Eur. J. Phycol. 00 (00), 1–15. doi: 10.1080/09670262.2021.2014983
Smale D. A., Wernberg T., Oliver E. C. J., Thomsen M., Harvey B. P., Straub S. C., et al. (2019). Marine heatwaves threaten global biodiversity and the provision of ecosystem services. Nat. Climate Change 2019 9:4 9 (4), 306–312. doi: 10.1038/s41558-019-0412-1
Steneck R. S., Graham M. H., Bourque B., Corbett D., Erlandson J., Estes J., Tegner M., et al. (2002). “Kelp forest ecosystems: Biodiversity, stability, resilience and future,” in Environ. Conserv., vol. 29 (4), 436–459. doi: 10.1017/S0376892902000322
Teagle H., Hawkins S. J., Moore P. J., Smale D. A. (2017). The role of kelp species as biogenic habitat formers in coastal marine ecosystems. J. Exp. Mar. Biol. Ecol. 492, 81–98. doi: 10.1016/j.jembe.2017.01.017
Thomas T. E., Harrison P. J., Taylor E. B. (1985). Nitrogen uptake and growth of the germlings and mature thalli of Fucus distichus. Mar. Biol. 84, 267–274. doi: 10.1007/BF00392496
tom Dieck I. (1992). North pacific and north Atlantic digitate laminaria species (Phaeophyta): hybridization experiments and temperature responses. Phycologia 31 (2), 147–163. doi: 10.2216/i0031-8884-31-2-147.1
tom Dieck I. (1993). Temperature tolerance and survival in darkness of kelp gametophytes (Laminariales, phaeophyta) - ecological and biogeographical implications. Mar. Ecol. Prog. Ser. 100 (3), 253–264. doi: 10.3354/meps100253
tom Dieck I., de Oliveira E. C. (1993). The section digitatae of the genus Laminaria (Phaeophyta) in the northern and southern Atlantic: crossing experiments and temperature responses. Mar. Biol. 115 (1), 151–160. doi: 10.1007/BF00349397
Tonsor S. J., Scott C., Boumaza I., Liss T. R., Brodsky J. L., Vierling E. (2008). Heat shock protein 101 effects in a. thaliana: Genetic variation, fitness and pleiotropy in controlled temperature conditions. Mol. Ecol. 17 (6), 1614–1626. doi: 10.1111/j.1365-294X.2008.03690.x
Valladares F., Matesanz S., Guilhaumon F., Araújo M. B., Balaguer L., Benito-Garzón M., et al. (2014). The effects of phenotypic plasticity and local adaptation on forecasts of species range shifts under climate change. Ecol. Lett. 17 (11), 1351–1364. doi: 10.1111/ele.12348
Vranken S., Wernberg T., Scheben A., Severn-Ellis A. A., Batley J., Bayer P. E., et al. (2021). Genotype–environment mismatch of kelp forests under climate change Molecular Ecology. 30(15), 3730–3746. doi: 10.1111/mec.15993
Wernberg T., Coleman M. A., Bennett S., Thomsen S., Tuya F., Kelaher P. (2018). Genetic diversity and kelp forest vulnerability to climatic stress. Sci. Rep. 8, 1851. doi: 10.1038/s41598-018-20009-9
Keywords: gametophyte, sporophyte, temperature, kelp, North Atlantic, adaptive potential
Citation: Strasser F-E, Barreto LM, Kaidi S, Sabour B, Serrão EA, Pearson GA and Martins N (2022) Population level variation in reproductive development and output in the golden kelp Laminaria ochroleuca under marine heat wave scenarios. Front. Mar. Sci. 9:943511. doi: 10.3389/fmars.2022.943511
Received: 13 May 2022; Accepted: 05 September 2022;
Published: 26 September 2022.
Edited by:
Anne Chenuil, Centre National de la Recherche Scientifique (CNRS), FranceReviewed by:
Diana Sofia Madeira, University of Aveiro, PortugalFilipe Alberto, University of Wisconsin–Milwaukee, United States
Copyright © 2022 Strasser, Barreto, Kaidi, Sabour, Serrão, Pearson and Martins. This is an open-access article distributed under the terms of the Creative Commons Attribution License (CC BY). The use, distribution or reproduction in other forums is permitted, provided the original author(s) and the copyright owner(s) are credited and that the original publication in this journal is cited, in accordance with accepted academic practice. No use, distribution or reproduction is permitted which does not comply with these terms.
*Correspondence: Luis Barreto, bGFiYXJyZXRvQHVhbGcucHQ=; Gareth A. Pearson, Z3BlYXJzb25AdWFsZy5wdA==
†These authors share first authorship