- 1Faculty/Graduate School of Fisheries Sciences, Hokkaido University, Hakodate, Japan
- 2Biology Department, Woods Hole Oceanographic Institution, Woods Hole, MA, United States
- 3Graduate School of Oceanography, University of Rhode Island, Narragansett, RI, United States
- 4Arctic Research Center, Hokkaido University, Sapporo, Japan
Aetideidae is a calanoid copepod family dominant in the mesopelagic layer of the Arctic Ocean for which little ecological information is available because species identification, especially of early copepodite stages, is difficult. In this study, we developed a species identification flow for the whole copepodite stages of five sympatric aetideid copepods (Chiridius obtusifrons, Gaetanus tenuispinus, G. brevispinus, Aetideopsis minor, and A. rostrata). Vertical distributions and seasonal population structures of these species were evaluated using a year-round sample time-series collected at the drifting ice station (SHEBA) in the western Arctic Ocean. Combinations of morphological characteristics (prosome length, cephalosome, and prosome widths) were used to identify the early copepodite stages to species. Aetideopsis rostrata was distributed in deep waters (1,032–1,065 m) throughout the year. The other species all were found at 600–700 m during the midnight sun. However, during the polar night, the vertical distributions of each species were distinct, resulting from ascent, descent, or depth maintenance, indicating seasonal vertical migration which may function to reduce inter-specific competition during the polar night when food resources are scarce. Reproduction timing varied among four aetideid copepods: C.obtusifrons and G. tenuispinus showed polar night ascent and reproduction at the end of the polar night, whereas G. brevispinus and A. minor showed descent or depth maintenance during the polar night and reproduction at the beginning of the polar night. There was not sufficient data to examine reproduction timing of A. rostrata. Common for all aetideid species, δ15N values of the adult females indicate more carnivorous feeding modes during the polar night than those in the midnight sun. Such vertical distribution and timing of reproduction variation among these five aetideid copepods may function to reduce species competition in the mesopelagic layer of the Arctic Ocean.
1 Introduction
In the Arctic Ocean marine ecosystem, the zooplankton community is an important secondary producer and plays a role in energy transfer to higher trophic level organisms as well as mediating vertical material transportation (Lowry et al., 2004; Wassmann et al., 2006). Copepods are the dominant taxon of the zooplankton community in the Arctic Ocean (Thibault et al., 1999; Ashjian et al., 2003). Because of seasonal ice coverage, most information about Arctic Ocean copepod ecology has been obtained during the summer (under midnight sun conditions) (cf. Laakmann et al., 2009a), and few studies have been conducted to investigate copepod ecology during the winter (under polar night conditions) (Zhang et al., 2010). Consequently, ecological and standing stock information about the Arctic copepods during the winter and their seasonal changes is scarce. To fill this knowledge gap, we considered that zooplankton sampling conducted from the drifting ice station in the Arctic could facilitate the year-round observation of standing stocks, population structure, and vertical distribution of Arctic copepods (Ashjian et al., 2003).
Most of the existing ecological information on Arctic planktonic copepods is available for surface-dwelling, numerically dominant copepod species (e.g., Pseudocalanus spp.) and interzonal, particle-feeding copepods that have a diapause phase at deeper depths (e.g., Calanus spp.). However, the species diversity of copepods is known to be high in the deep sea, especially in the mesopelagic zone (Lee, 2000; Norris, 2000; Goetze, 2003). The Aetideidae is a family of copepods that dominates the copepod community in the mesopelagic zone, and is characterized by a high species diversity (Richter, 1995; Markhaseva, 1996; Auel, 1999). Members of the Aetideidae are known to be omnivores or detritivores and are described as opportunistic feeders (Richter, 1995; Auel, 1999; Sano et al., 2013; Sano et al., 2015). The occurrence of species-specific food preferences (Laakmann et al., 2009a; Laakmann et al., 2009b; Sano et al., 2013; Sano et al., 2015) and species-specific vertical segregation within the family (Kosobokova and Hirche, 2000; Auel and Hagen, 2002; Laakmann et al., 2009a; Smoot and Hopcroft, 2017) is well documented. Although the hydrographic condition of the deep Arctic Ocean is homogenous (cold and fresh), coexistence of Aetideidae species is thought to occur by vertical and food segregation (Laakmann et al., 2009a; Laakmann et al., 2009b; Laakmann and Auel, 2010). Stable isotopes especially δ15N are a useful guide for food preference (Laakmann et al., 2009a; Laakmann et al., 2009b; Laakmann and Auel, 2010). Mesopelagic copepods are reported to have a substantial effect on vertical material flux to the deep sea by feeding on sinking particles and excretion in the form of faecal pellets (Dilling et al., 1998; Wilson et al., 2010), and aetideid copepods in the Greenland Sea are reported to consume 40% of the particulate organic carbon flux that reaches at their habitat depth (Auel, 1999). These facts suggest that Aetideidae is an important copepod taxon in the Arctic Ocean, both from species diversity and vertical material flux perspectives.
A key challenge to conducting an ecological study of Aetideidae is the difficulty of species identification, especially for the early copepodite stages (C1–C3). In part, this is because morphological identification keys for each sympatric species have not yet been created, and the early copepodite stages of the Aetideidae have been treated as aetideid copepods C1–C3 in previous studies conducted in the Arctic Ocean (e.g., Kosobokova and Hirche, 2000; Auel and Hagen, 2002). Consequently, little is known about the life history, seasonal population structure, and timing of reproduction of the aetideid copepods in the Arctic Ocean. To improve our knowledge of the ecology of aetideid copepods, it is necessary to create species identification criteria based on morphological characteristics of all the copepodite stages.
In this study, we examined a year-round time series of zooplankton samples collected from the drifting Surface Heat Budget of the Arctic Ocean (SHEBA) ice station in the western Arctic Ocean. We conducted morphological identification of all the copepodite stages of five dominant sympatric aetideid copepods. We also determined the seasonal changes in the vertical distribution and population structure of these five species. We measured δ15N of adult females of all aetideid copepods with midnight sun and polar night for evaluation of their food preference. We compared the seasonal dynamics of the five sympatric aetideid copepods and considered the mechanism of coexistence of these copepod species in the mesopelagic layer of the Arctic Ocean.
2 Materials and methods
2.1. Field sampling
Zooplankton sampling was conducted from the drifting SHEBA ice station from 27 October 1997 to 29 September 1998. Vertical stratification samples were collected from 2–7 depth layers over a depth range of 0–3,500 m depth using a closing net with a 1 m2 mouth area and 150 µm or 53 µm mesh. The small mesh (53 µm) was used for the shallow samples (<200 m) of the restricted period (February-August 1998). Samples were collected at 10–14 day intervals (Figure 1). The SHEBA ice station drifted from the Canadian Basin to the Mendeleyev Basin during the one-year sampling period (Ashjian et al., 2003). Because of this drift, the water depth varied from 352 m to 3,800 m during the sampling period, consequently, the zooplankton collection depth and the number of layers sampled ranged from 0–100 m to 0–3,500 m and from 2 to 7 layers, respectively. The details of the sampling layer at each sampling date are available in Ashjian and Campbell (2007). The zooplankton samples were preserved using 4% buffered formaldehyde seawater. On each sampling day, water temperature, salinity, and chlorophyll a (Chl. a) fluorescence values were measured for the upper water column (maximum depth: 300 or 700 m) with a SeaBird SB11 Seacat CTD (SeaBird Scientific, Bellevue, WA, USA), equipped with a calibrated fluorometer. Daylight hours for each sampling day were calculated based on the latitude of the sampling site (cf. Brock, 1981).
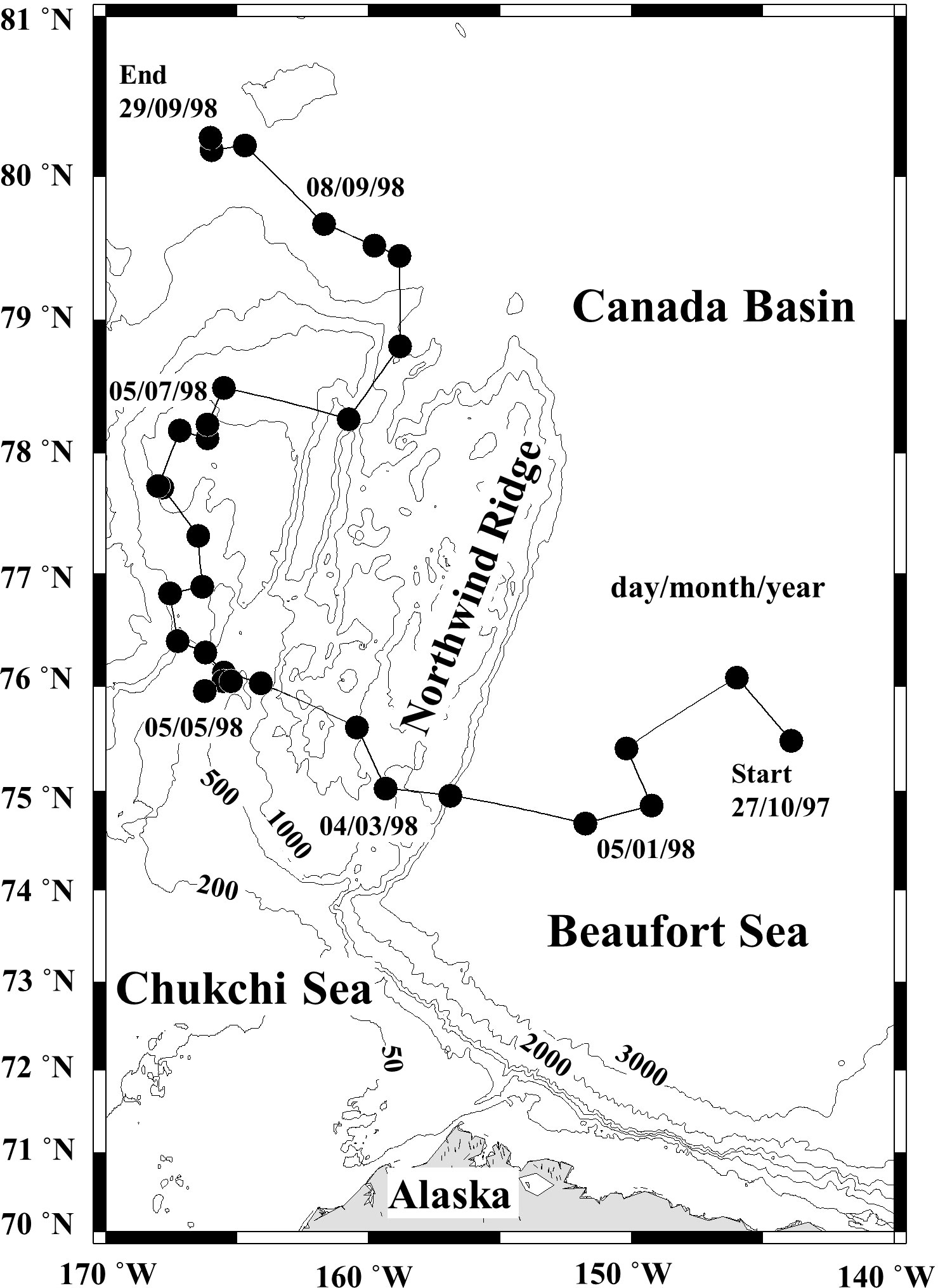
Figure 1 Location of the zooplankton sampling sites (black circles) along the drift track of the Surface Heat Budget of the Arctic Ocean (SHEBA) ice station through the Canada Basin to the Mendeleev Plain from 27 October 1997 to 29 September 1998 (cf. Ashjian et al., 2003).
2.2. Microscope observations
Using a stereomicroscope, each copepodite stage of the five dominant aetideid copepods (Chiridius obtusifrons, Gaetanus tenuispinus, G. brevispinus, Aetideopsis minor, and A. rostrata) was identified and enumerated. Based on the presence (male) or absence (female) of a fifth swimming leg, males and females were distinguishable for specimens of the C4–C6 stages (hereafter referred to as C4F–C6F and C4M–C6M). We provide a flow diagram showing species identification of the whole copepodite stages of the aetideid copepods in Table 1.
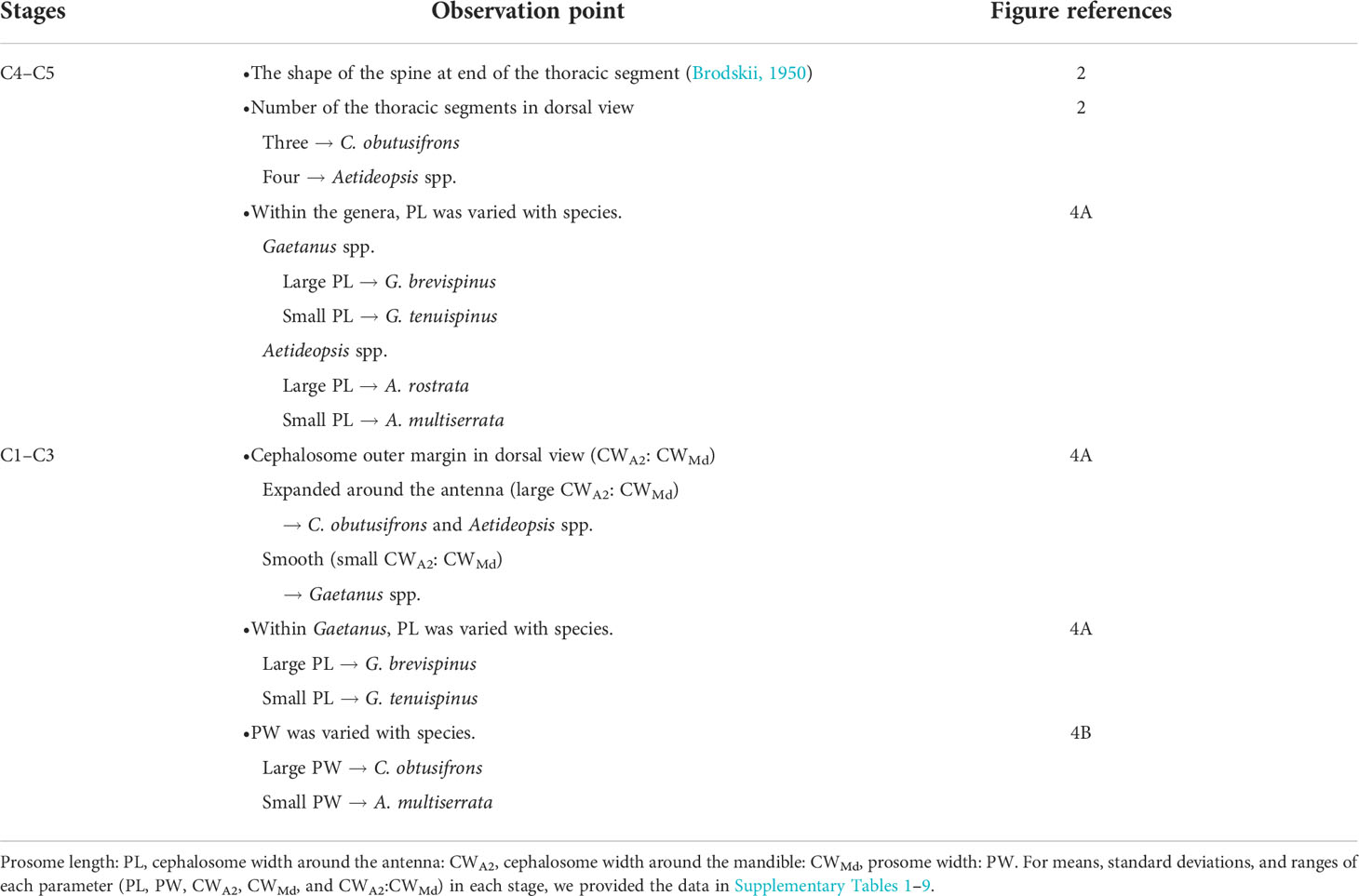
Table 1 Flow diagram for species identification on juvenile copepodite stages of five sympatric Aetideidae copepods (Chiridius obtusifrons, Gaetanus tenuispinus, Gaetanus brevispinus, Aetideopsis minor, and Aetoideopsis rostrata) in the western Arctic Ocean.
For species identification of stages C4–C6, we used the shape of the spine at the end of the thoracic segment (Brodskii, 1950). Since the shape of the spine was similar for C. obtusifrons and Aetideopsis spp., we used the number of thoracic segments (three for C. obtusifrons and four for Aetideopsis) which was identifiable from the dorsal view as an identification guide (Figure 2). For the congener species, body sizes determined as prosome length (PL) varied: for Gaetanus spp., G. brevispinus was larger than G. tenuispinus, and for Aetideopsis spp., A. rostrata was larger than A. minor. Because the spine at the end of the thoracic segment was not developed in stages C1–C3, we used the cephalosome width around the antenna (CWA2) and cephalosome width around the mandible (CWMd) (Figure 2) as alternative species-identification characteristics for C1–C3. The cephalosome outer margin of Gaetanus was smooth, whereas the outer margins of Chiridius and Aetideopsis were expanded around the antenna. Based on these morphological features, the C1–C3 copepodite stages were identified to the genus level based on the CWA2: CWMd ratio, thus, a specimen with a large CWA2: CWMd ratio was identified as Chiridius and Aetideopsis, and specimens with a small CWA2: CWMd ratio as Gaetanus. Identification of species within a genus was based on PL. Because the C1–C3 stages of C. obtusifrons and A. minor were similar in PL and morphology of the outer cephalosome margin, we observed the prosome width at the middle of the prosome (PW) and identified large PW specimens as C. obtusifrons and small PW specimens as A. minor. To define the size ranges used to discriminate species (Figure 4), the PL, CWA2, and CWMd were measured for 5–10 individuals of each copepodite stage of each species sorted from the most abundant samples under a stereomicroscope using an eyepiece micrometre with a precision of 10 µm. For the C1–C3 stages of C. obtusifrons and A. minor, PW was also measured. For means, standard deviations, and ranges of each parameter (PL, PW, CWA2, CWMd, and CWA2: CWMd) at each stage, we provide the data in Supplementary Tables 1–9.
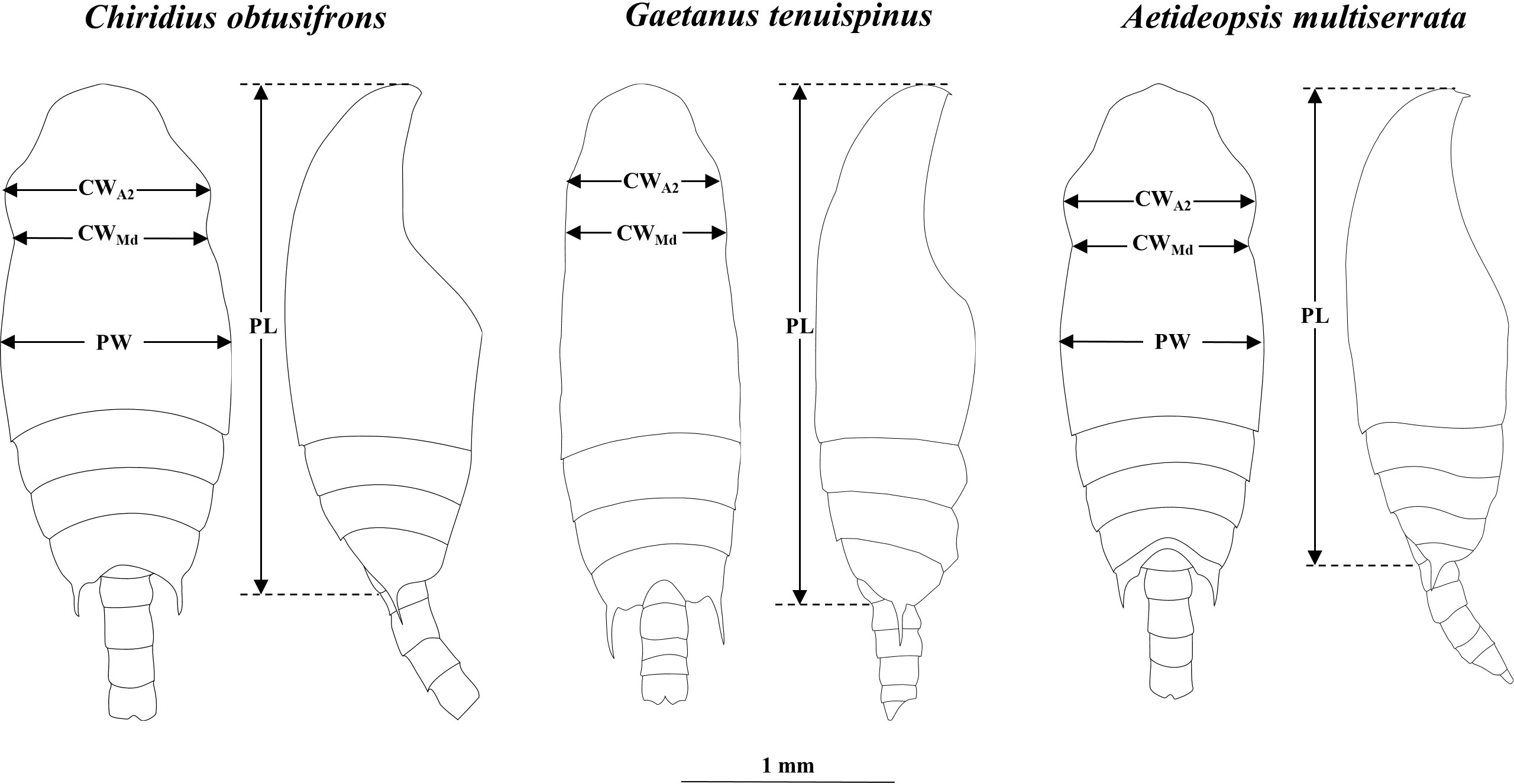
Figure 2 Diagrams showing body size measurements: cephalosome width at antenna (CWA2), cephalosome width at mandible (CWMd), prosome width at middle of the prosome (PW), and prosome length (PL) for Chiridius obtusifrons (left), Gaetanus tenuispinus (centre) and Aetideopsis minor (right). Scale bar represents 1 mm.
It should be noted that the identification for the juvenile copepodite stages of this study is not confirmed by molecular identification. Thus, the identification should be termed the open nomenclature term incerta (Horton et al., 2021) to indicate uncertain identification (e.g. C. obtusifrons sp. inc., G. tenuispinus sp. inc., G. brevispinus sp. inc., A. minor sp. inc., and A. rostrata sp. inc.).
2.3. Population structure
The depth topography below the ice station varied temporally as a consequence of drift, and this determined the maximum sampling depth on any one sampling day. Because Aetideidae species are distributed in the mesopelagic layer (Kosobokova and Hopcroft, 2010; Kosobokova et al., 2011), shallower sampling depths on some sampling days may have resulted in failure to collect representative samples of the Aetideidae populations at every sampling site. The likelihood of this is evidenced by the low abundance of Aetideidae species at the shallower sampling depths (see Supplementary Tables 10–13). Therefore, we used population structure data of the four species: C. obtusifrons, G. tenuispinus, G. brevispinus, and A. minor only from sampling sites where the maximum sampling depths were greater than 500 m and omitted the data for the shallower sampling depths (<500 m maximum sampling depth) (Supplementary Tables 10–13). This resulted in a reduction in the number of sampling days from 30 to 19; however, the reduced data set comprised approximately one-month intervals, except for July 1998. The impact of maximum sampling depth was most severe for the deepest dwelling species, A. rostrata, and population structure data collected at depths shallower than 900 m were omitted for this species (Supplementary Table 14). As a result, time-series data for A. rostrata were not available from April to July 1998. To reduce the abrupt changes in the population structure data related to hydrographic and geographical changes, we standardized the abundance data for each copepodite stage by calculating the moving average of three consecutive sampling dates. For each sampling date, the mean copepodite stage (MCS) was calculated using the following formula (Marin, 1987):
where i is the number of copepodite stages (1 for C1, 6 for C6) and Ai is the number of individuals at copepodite stage i (ind. m−2).
2.4. Vertical distribution
The vertical distribution core (D50%) where 50% of the population was distributed, was calculated based on abundances (ind. m−2) in each sampling layer (Pennak, 1943):
where d1 is the depth (m) of the upper depth of the 50% individual occurrence layer, d2 is the maximum depth (m) of the 50% individual occurrence layer, p1 is the cumulative individual percentage (%) that occurred at depths shallower than the 50% individual occurrence layer, and p2 is the individual percentage (%) at the 50% individual occurrence layer. The difference in D50% between the polar night and midnight sun for all copepodite stages of each species was tested using the Mann-Whitney U-test. Differences in D50% among copepodite stages within a species during the same period (i.e., during the polar night and during the midnight sun) were tested by one-way ANOVA. In addition, differences in D50% among species during the same period were tested by one-way ANOVA and a post hoc test (Tukey-Kramer test). For the evaluation of D50%, we excluded the sampling dates when the maximum depth of collection was shallower than 900 m for all species because Aetideidae species are distributed in the mesopelagic layer. Excluding data based on this criterion, we analysed six sampling dates for the polar night and three sampling dates for the midnight sun for comparison of the vertical distribution of Aetideidae (Supplementary Tables 10–14).
2.5. δ15N value
For evaluation of food preference, δ15N value was measured for C6F of each species with polar night and midnight sun. From the most abundant samples at each period, the batches of C6F specimens (since 5 mg DW was required for each measurement, 9–18 individuals which varied with species were set for each batch) were sorted, rinsed with distilled water then dried in a 60°C oven for five hours. Dried samples were ground into a fine powder with a ceramic mortar and pestle and used for measurement of 15N and 14N by using an elemental analyzer/isotope-ratio mass spectrometer (Flash EA1112-Delta V Plus He-Flow System, Thermo Fisher Scientific, Germany). The measurements were made in triplicate (three batches). δ15N was calculated from the following equation:
where 15Nsample and 14Nsample are the values of the samples, and 15Nstandard and 14Nstandard are the values of the standard. Species differences in δ15N values were tested by one-way ANOVA and Tukey-Kramer test. Temporal changes (polar night vs midnight sun) within the species were tested by Mann-Whitney U-test.
3 Results
3.1 Hydrography
Seasonal variations in the daily daylight length, water temperature, salinity, and Chl a fluorescence determined at the SHEBA ice station are shown in Figure 3. Based on the daylight length at the SHEBA ice station, the polar night was demarcated to have occurred from early November 1997 to early February 1998, and the midnight sun from late April to late August 1998. The remaining periods were defined as transition periods. The water temperatures ranged between −1.76 and 0.91°C, and were lower in the shallower water and higher at depth. The salinity range was 26.60−34.91, being lower near the surface, and the halocline was observed at a depth range of approximately 100–200 m throughout the year. The Chl. a fluorescence ranged from 0.002 to 4.259 and was high above a depth of 30 m from June to August. During the course of the drifting ice station, three water masses were present for the shallower depths (< 100 m), but the remaining deep waters contained Arctic Intermediate Water and Polar Intermediate Water throughout the year (Ashjian et al., 2003).
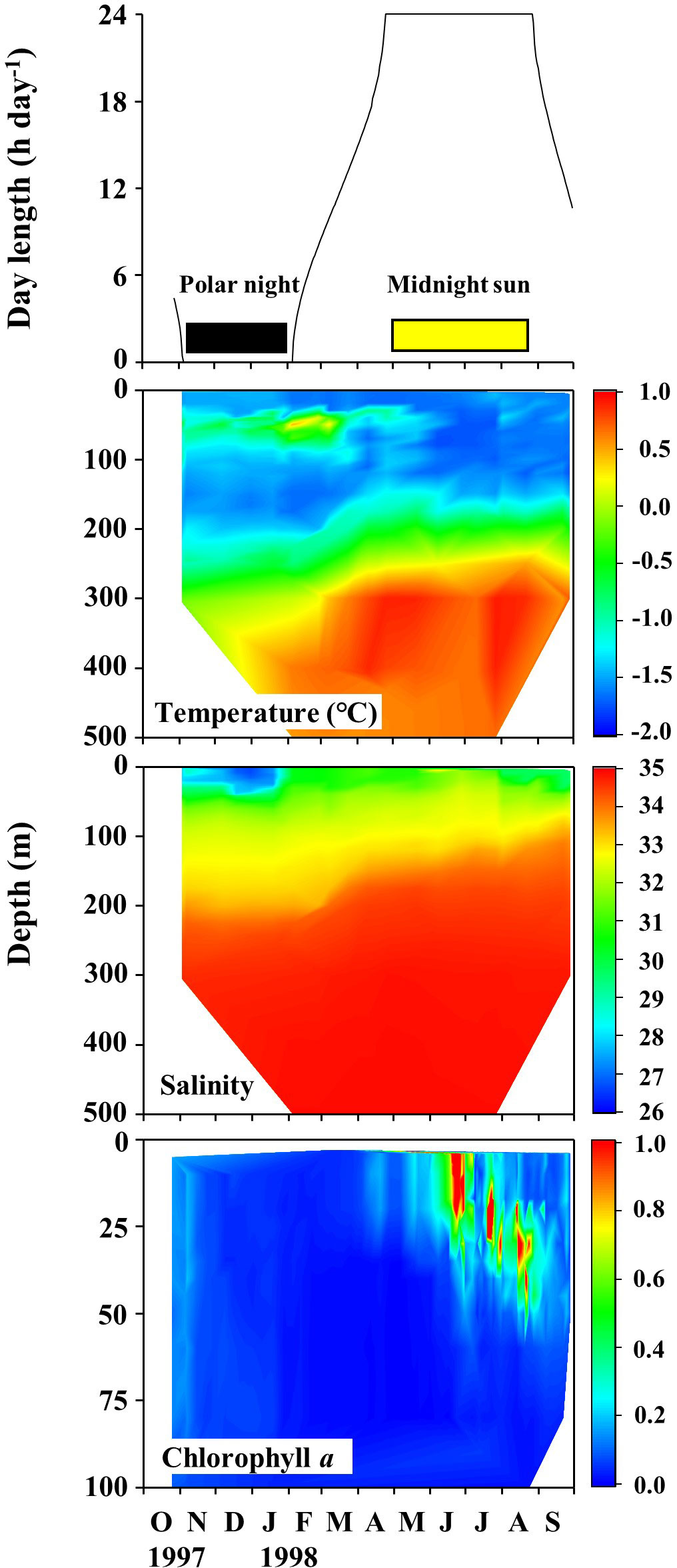
Figure 3 Seasonal changes in daylength, temperature, salinity, and chlorophyll a fluorescence recorded at the Surface Heat Budget of the Arctic Ocean (SHEBA) ice station as it drifted through the Canada Basin to the Mendeleev Plain from 27 October 1997 to 29 September 1998.
3.2. Morphological characteristics
Scatter plots showing the relationship between the CWA2: CWMd ratio and PL for each copepodite stage of the five aetideid copepods are shown in Figure 4A. For all copepodite stages except C6M, CWA2: CWMd was low (approximately 0.9–1) for Gaetanus spp., whereas this ratio was high (approximately 1–1.1) for Chiridius and Aetideopsis species. This finding reflected the morphological difference of the cephalosome outer margin which was fused around the antenna in Chiridius and Aetideopsis species, but smooth in Gaetanus spp. Note that some variability was present for CWA2: CWMd ratio, especially for early copepodite stages (C1 and C3). Within the congener species, PL varied with species, where, for Gaetanus spp., the PL of G. brevispinus was larger than that of G. tenuispinus, and for the Aetideopsis spp. the PL of A. rostrata was larger than that of A. minor. It should be noted that CWA2: CWMd was similar for C. obtusifrons and A. minor, while some overlap was present, the PL of C. obtusifrons was larger than that of A. minor. For identification of C. obtusifrons and A. minor, the number of thoracic segments was used for stages older than C4. For identification of earlier stages (C1–C3) of these species, PW was used, where a large PW distinguished C. obtusifrons, and a small PW distinguished A. minor (Figure 4B). For means, standard deviations, and ranges of each parameter (PL, PW, CWA2, CWMd, and CWA2: CWMd) in each stage, we provide the data in Supplementary Tables 1–9.
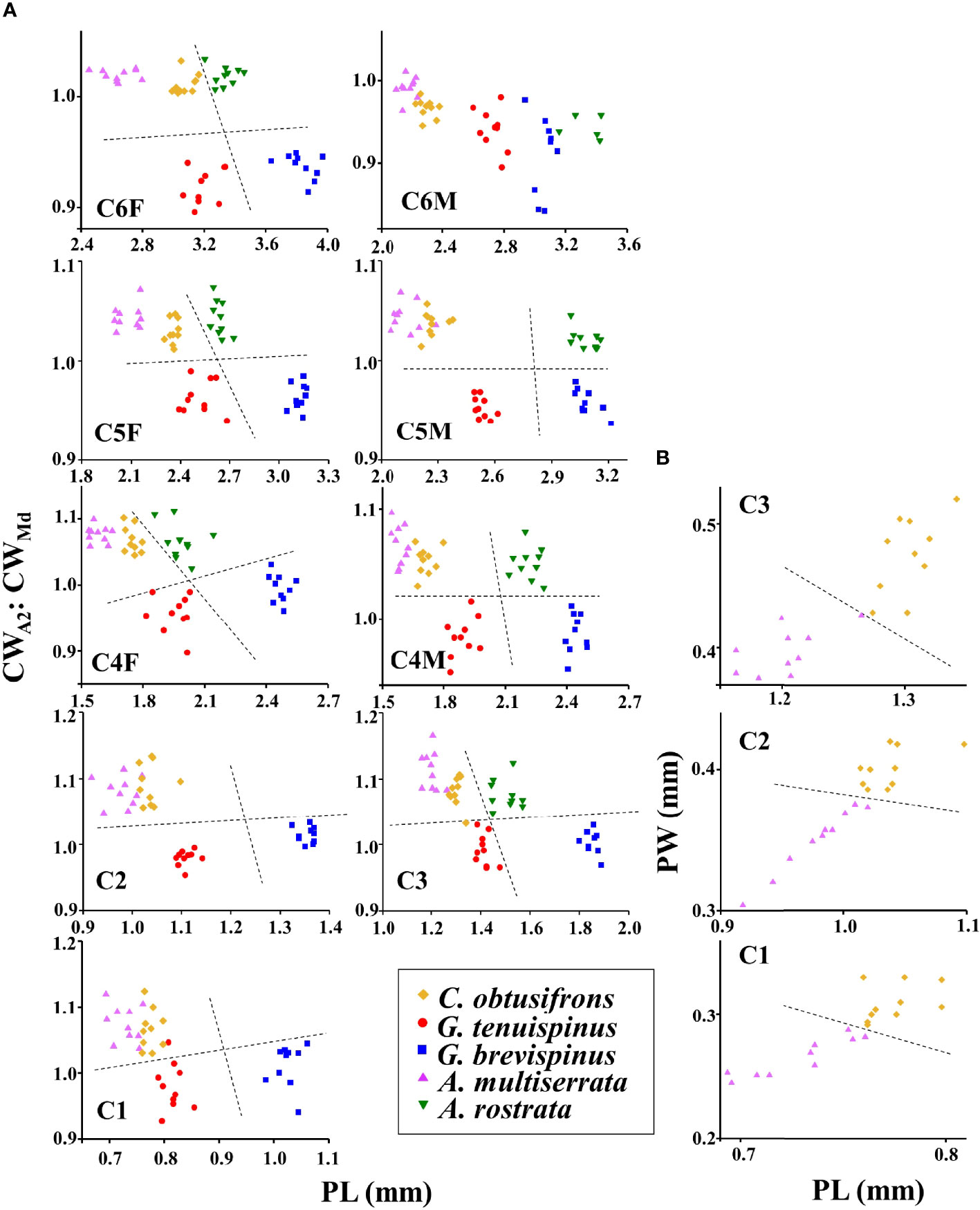
Figure 4 (A) Scatter plots of the ratio of the cephalosome width at the antenna (CWA2): cephalosome width at the mandible (CWMd) and the prosome length (PL) for each copepodite stage of the five Aetideidae copepods dominant in the western Arctic Ocean. The species are: Chiridius obtusifrons, Gaetanus tenuispinus, G. brevispinus, Aetideopsis minor, and A. rostrata. (B) Scatter plots of prosome width (PW) and prosome length (PL) for the early copepodite stages of C. obtusifrons and A. minor. F, female; M, male. Dashed lines represent approximate separation for each species at each copepodite stage. Because the scatter plots for C. obtusifrons and A. minor in (A) were similar, additional plots were created for these two species in (B).
3.3. Vertical distribution
The vertical distribution core (D50%) of each copepodite stage of the five studied Aetideidae species during the polar night and the midnight sun is shown in Figure 5. Within the same stages, significant differences in vertical distribution between the polar night and midnight sun were observed only for C. obtusifrons C6F (p<0.05, Mann-Whitney U-test). Within the same season, significant differences in vertical distribution between the copepodite stages of the same species were observed only for A. rostrata during the polar night (p<0.05, one-way ANOVA). Comparisons between species and temporal changes within species based on the mean D50% of all the copepodite stages during the polar night and midnight sun are shown in Table 2. During the polar night, C. obtusifrons and G. tenuispinus distributed at the shallowest depths (298–381 m), whereas G. brevispinus and A. minor occurred 300–400 m deeper (628–791 m), and A. rostrata showed the deepest distribution (1,065 m). In contrast, during the midnight sun, four of the species: C. obtusifrons, the two Gaetanus spp., and A. minor distributed at similar depths (within a 100 m range), and only A. rostrata showed a significantly different distribution (p<0.01), at a depth approximately 300 m deeper than that of the other species. C. obtusifrons and G. tenuispinus showed shallower distributions during the polar night than during the midnight sun (p<0.001, Mann-Whitney U-test), whereas G. brevispinus showed a deeper distribution during the polar night compared to that during the midnight sun (p<0.01). The deepest dwelling species, the two Aetideopsis spp., showed no differences in their D50% between the polar night and the midnight sun (p>0.05).
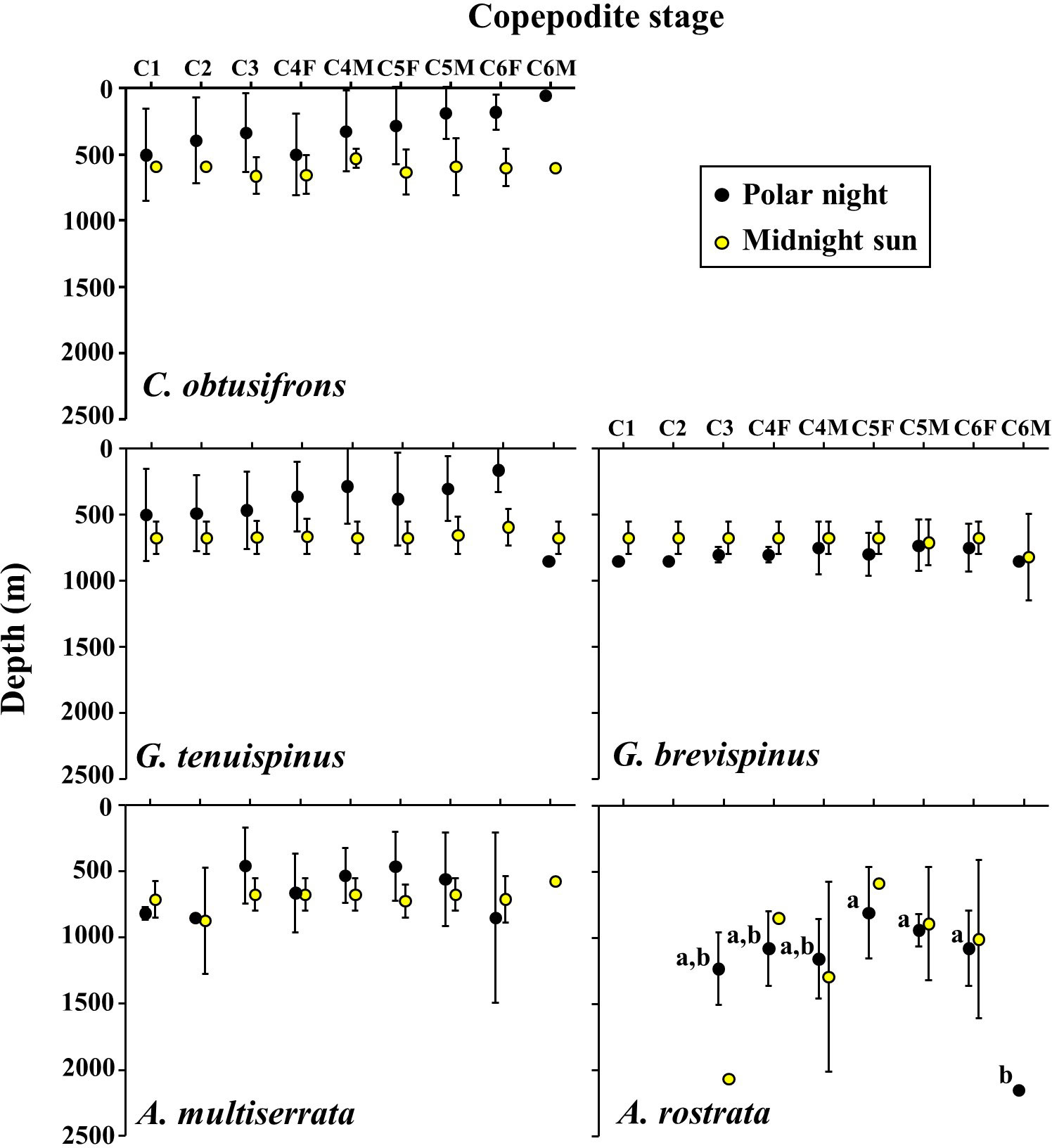
Figure 5 Ontogenetic changes in vertical distribution of the copepodite stages of the five Aetideidae copepods dominant in the western Arctic Ocean during the polar night and midnight sun. Symbols and bars represent means of the distribution centres (D50%) and their standard deviations, respectively. F, female; M, male. The species are: Chiridius obtusifrons, Gaetanus tenuispinus, G. brevispinus, Aetideopsis minor, and A. rostrata. Alphabet letters for A. rostrata during the polar night indicate significant differences between stages.
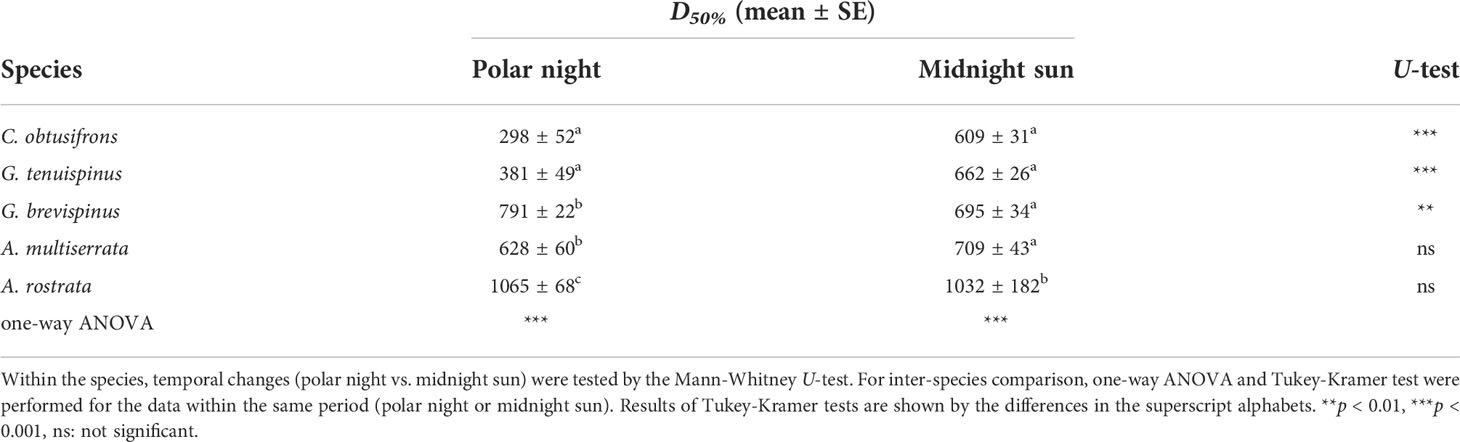
Table 2 Summary of vertical distribution (vertical distribution core: D50%, mean ± SE) of five Aetideidae copepods in the western Arctic Ocean during the polar night and midnight sun.
3.4. Population structure
Seasonal changes in abundance, copepodite stage composition, MCS, and the proportion of C4–C6 in the female and male populations of the five aetideid copepods studied are shown in Figure 6. The season of peak abundance varied with species. The abundance of C. obtusifrons and G. tenuispinus was highest in May, whereas the abundance of G. brevispinus and A. minor peaked in March. In all species, the MCS values were lowest when the recruitment of the early copepodite stages occurred. The lowest MCS value was observed in April–May for C. obtusifrons and G. tenuispinus, whereas it was recorded for G. brevispinus from January to March, and from February to March for A. minor. The seasonal timing of early stage copepodite recruitment corresponded with the peak abundance seasons of each species. A common feature of all five species was a much lower proportion of C6 individuals in the male population compared to that in the female population. The proportion of females (%) in each copepodite stage (C4–C6) is shown in Table 3. For all species, females were dominant (56.6%–66.7%) in the C4–C6 populations, whereas males dominated the C4 and C5 populations. In all species, the C6 population was predominantly female (76.3%–97.3%), which may account for the higher overall proportion of females in the C4–C6 populations.
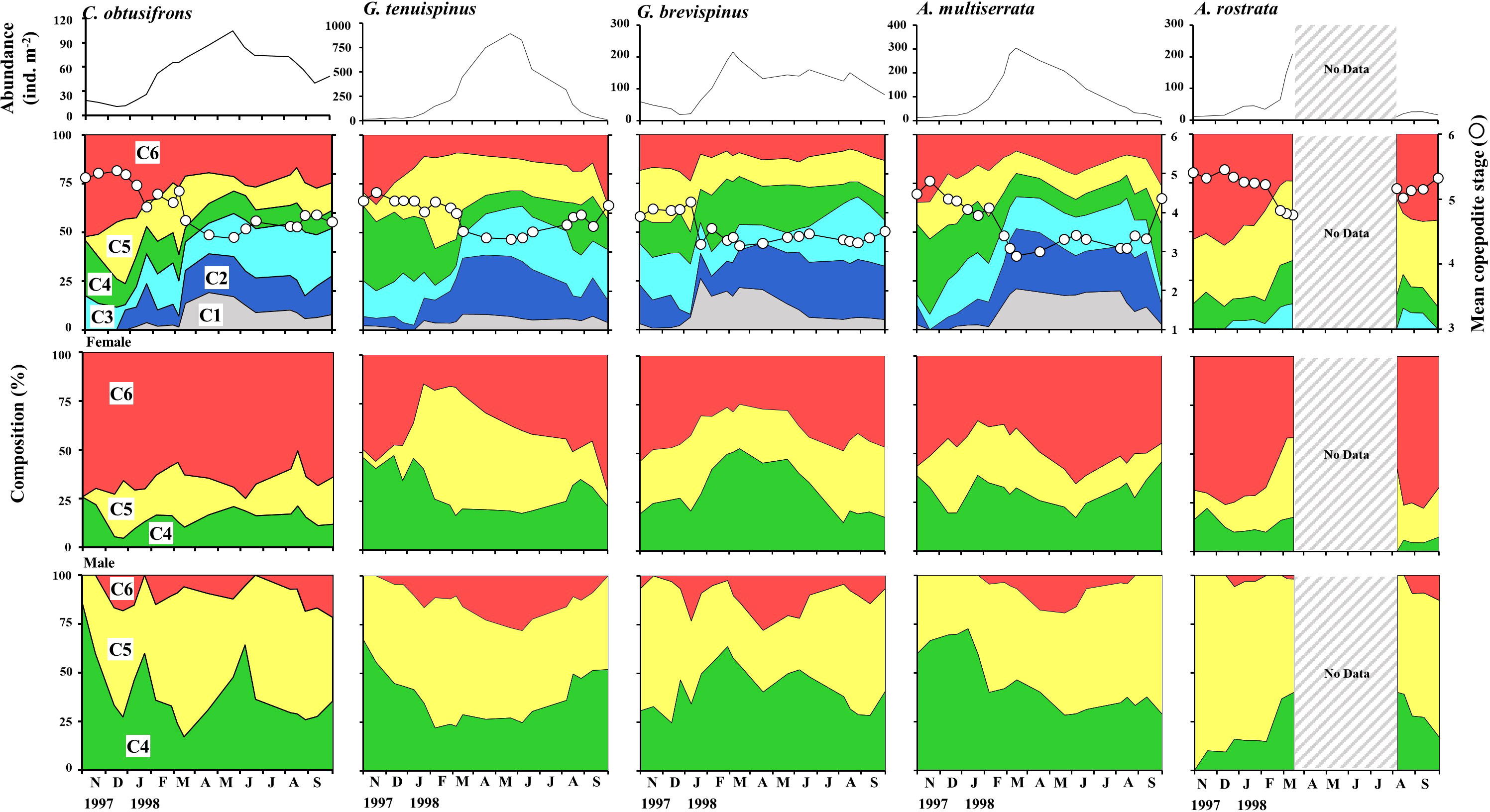
Figure 6 Seasonal changes in total abundance, stage composition, and population structure (for C4–C6) of females and males of the five dominant Aetideidae copepods in the western Arctic Ocean from 27 October 1997 to 29 September 1998. Mean copepodite stage (white circles) was calculated for each species for each sampling date. Populations of Aetideopsis rostrata, the deepest dwelling species, were not quantified between March and August 1998 because zooplankton collection was restricted to the shallower depths related to drifting of the ice station. The shallower dwelling species are Chiridius obtusifrons, Gaetanus tenuispinus, G. brevispinus, and Aetideopsis minor.
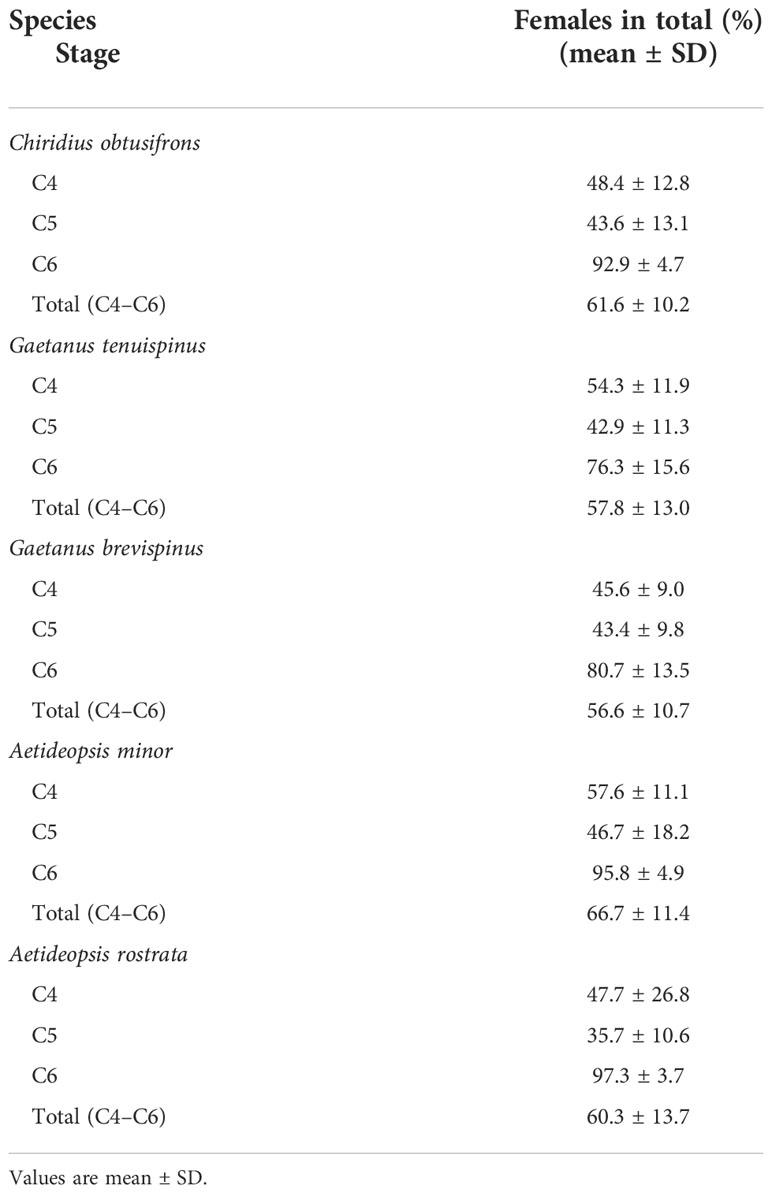
Table 3 Sex ratio (% of females in total population) of the five Aetideidae copepods abundant in the western Arctic Ocean during October 1996 to September 1997.
3.5. δ15N value
δ15N values of adult females of the aetideid copepods during the polar night and midnight sun are summarized in Table 4. During the polar night, δ15N values were 14.1–16.4, with the lower values for Gaetanus spp. and higher values for C. obtusifrons and Aetideopsis spp. During the midnight sun, δ15N values were 13.4–15.4 somewhat lower than the polar night values within the species, although there was no significant difference between the polar night and the midnight sun. The species order in δ15N values of the aetideid copepods was the same for the polar night and midnight sun.
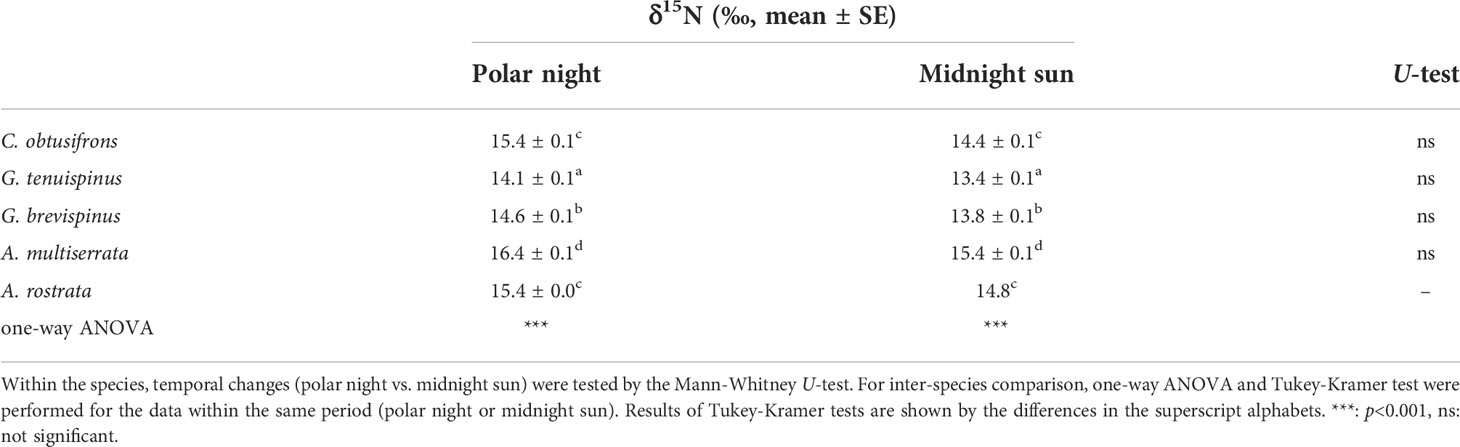
Table 4 Summary of δ15N value (‰, mean ± SE) of five Aetideidae copepods in the western Arctic Ocean during the polar night and midnight sun.
4 Discussion
4.1. Vertical distribution
A schematic diagram of the seasonal vertical distributions and reproductive periods of the five aetideid copepods in the western Arctic Ocean is shown in Figure 7. During the midnight sun, the four species with the shallower distributions: C. obtusifrons, G. tenuispinus, G. brevispinus, and A. minor were distributed at similar depths. However, during the polar night, C. obtusifrons and the two Gaetanus species changed their habitat depths (C. obtusifrons and G. tenuispinus ascended, whereas G. brevispinus descended), and their habitat depths were clearly separated during this period (Table 2). In contrast, the A. rostrata populations were distributed in deep waters throughout the year. Most of our knowledge about the vertical distribution of the aetideid copepods in the Arctic Ocean is derived from the summer season, particularly August–September; the end of the midnight sun. At this time, C. obtusifrons was reported to be distributed mainly at depths of 0–2,500 m, G. tenuispinus at 0–1,000 m, G. brevispinus at 100–2,000 m, and A. rostrata at 200–2,000 m (Kosobokova and Hirche, 2000; Laakmann et al., 2009a; Smoot and Hopcroft, 2017). Although little information is available for the vertical distribution of A. minor, this species is treated as a meso- to bathypelagic species and was reported to distribute at depths of approximately 500–1,500 m (Richter, 1994; Richter, 1995). Thus, the vertical distribution ranges of the five aetideid copepods recorded in this study correspond with the reported vertical distribution depths in previous Arctic Ocean studies (Figure 5).
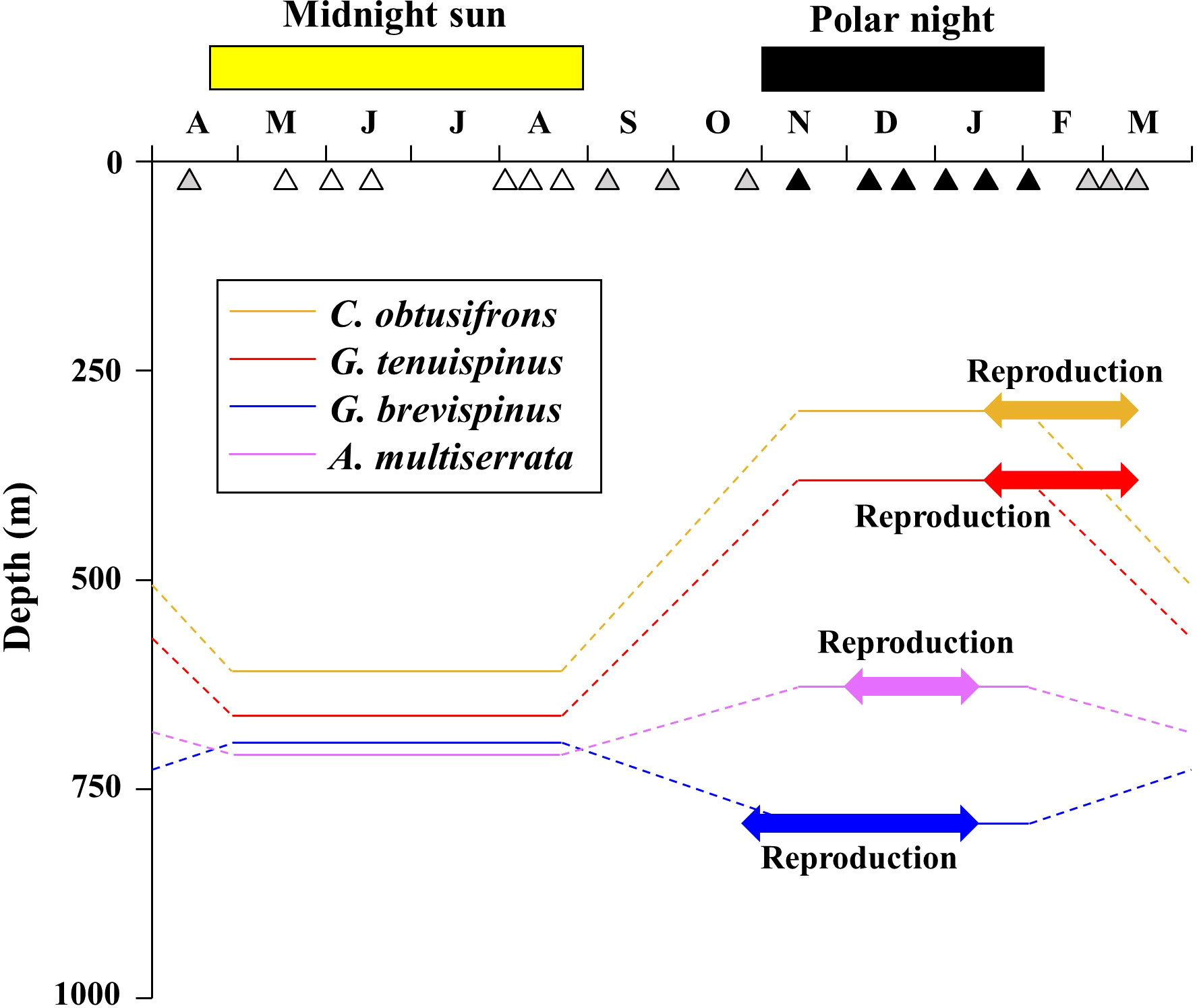
Figure 7 Schematic diagram showing seasonal changes in vertical distribution and timing of reproduction of four of the dominant Aetideidae copepods in the western Arctic Ocean. The open, solid, and grey triangles indicate the sampling dates during the midnight sun, polar night, and their transition periods, respectively. The shallower-dwelling species are Chiridius obtusifrons, Gaetanus tenuispinus, G. brevispinus, and Aetideopsis minor.
Information about the vertical distribution of aetideid copepods during the polar night is scarce. Moreover, limited information is available about the seasonal dynamics of their vertical distribution. As a notable exception, Richter (1994); Richter (1995) reported that there are no seasonal changes in the vertical distribution of Aetideidae in the Greenland Sea. In contrast to this Greenland Sea finding, results of the present study indicate that the four shallower-dwelling aedtideid copepods (C. obtusifrons, G. tenuispinus, G. brevispinus, and A. minor) showed clear vertical depth separation during the polar night and exhibited seasonal vertical migration (Table 2). The coexistence mechanisms of the sympatric sibling copepods in the deep-sea of the Arctic Ocean are reported to include separation in vertical distribution and different food preferences (Laakmann et al., 2009a; Laakmann et al., 2009b; Laakmann and Auel, 2010). In terms of their feeding modes, the aetideid copepods are considered to be omnivores or detritivores and are reported to perform opportunistic feeding, utilising passive, sinking, particulate organic matter as their primary food sources (Richter, 1995; Auel, 1999; Sano et al., 2013; Sano et al., 2015). In the western Arctic Ocean, the amount of passive, sinking particle organic carbon flux is reported to be high for the midnight sun during spring to autumn, and low for the polar night during winter (O’Brien et al., 2006; Honjo et al., 2010). These seasonal changes in the amount of sinking particle flux may explain the seasonal vertical distribution of the sympatric aetideid copepods. It may be that during the midnight sun, a sufficiently high availability of sinking particles may provide sufficient food resources to support all four of the aetideid copepods, thus enabling their distribution and coexistence at similar depths. However, during the polar night, because of the low amount of sinking particles available across the depth range, the four copepod species may separate their habitat depths to reduce competition for food resources, a dynamic that may function as niche separation. Vertical separation in habitat depths and preference for carnivory are reported for the aetideid copepods (Laakmann et al., 2009a; Laakmann et al., 2009b; Laakmann and Auel, 2010).
4.2. Population structure
In this study, seasonal changes in population structure were observed for four of the aetideid copepods, except A. rostrata. The low MCS values indicated that population recruitment occurred for each species, and, common to all species, the seasonal timing of the low MCS values corresponded closely with the seasonal timing of the abundance peak of each species (Figure 6). By combining the timing of recruitment of early copepodite stages and the time taken for development of the eggs and nauplii, the seasonal reproduction period of each species can be estimated (Yamaguchi et al., 2020). Regarding aetideid copepod reproduction, egg diameters have been reported as 315 ± 10 μm (mean ± 1sd) for G. tenuispinus, 440 ± 66 μm for G. brevispinus, 290 ± 12 µm for C. obtusifrons, and 270–300 µm (range) for A. rostrata (Kosobokova et al., 2007), and it has been observed that the development time of early copepod stages is affected by temperature, food availability, and body size (egg diameter) (Mauchline, 1998). Because the egg diameters of the deep-sea aetideid copepods are much larger compared to those of the surface-dwelling copepods, their naupliar stages are suggested to be able to develop without feeding (Kosobokova et al., 2007). These facts indicate that the development times of eggs and nauplii could be estimated by two factors: habitat temperature and body size (egg diameter).
The relationships between temperature and the development time of early mesopelagic copepod stages with non-feeding naupliar stages have been reported for Gaetanus variabilis (= Gaidius variabilis) and Paraeuchaeta elongata, which have egg diameters of 325 ± 11 μm (G. variabilis) and 460 ± 30 μm (P. elongata) (Ozaki and Ikeda, 1997; Yamaguchi and Ikeda, 2000). These egg sizes are comparable to those of the aetideid copepods researched in this study (270–440 µm). Applying published Bělehrádek equations for G. variabilis and P. elongata (Ozaki and Ikeda, 1997; Yamaguchi and Ikeda, 2000) and habitat temperature of the aetideid copepods in this study: 0.57°C (mean temperature below 300 m; range: −0.23 to 1.07°C, Figure 3), the development times for eggs and nauplii to reach C1 are estimated to be 67.6–83.1 days. From field data, the recruitment of early copepodite stages was observed to be April–May for C. obtusifrons and G. tenuispinus, January–March for G. brevispinus, and February–March for A. minor (Figure 6). Considering the estimated development time of eggs and nauplii (67.6–83.1 days), the reproductive period of each species was back-calculated as: February–March for C. obtusifrons and G. tenuispinus, November–January for G. brevispinus, and December–January for A. minor (Figure 7). These reproductive periods corresponded with the timing of the polar night or occurred during the sunlight recovery season in the field.
Of the factors that determine the timing of copepod reproduction, food availability is known to be the most important (cf. Mauchline, 1998). At the SHEBA ice station, increases in phytoplankton and microplankton were reported to occur from late May to June (Sherr and Sherr, 2003; Sherr et al., 2003). This period corresponds with the growth season of the early copepodite stages of the aetideid species observed in this study (Figure 6). Thus, as an energy source for the initiation of the reproduction of the aetideid copepods, other food resources may be important. Although Aetideidae species are known to be omnivorous, the importance of carnivorous feeding has been reported, especially for the adult stages, and their food is reported to comprise the energy-rich eggs and nauplii of the large-sized Calanus copepods, and adults of small-sized copepods such as Microcalanus and Pseudocalanus species (Richter, 1995; Auel, 1999; Sano et al., 2013; Sano et al., 2015). These carnivorous feeding modes of the aetideid copepods have been confirmed by fatty acid analysis (Laakmann et al., 2009a; Laakmann et al., 2009b; Laakmann and Auel, 2010). These facts suggest that the link between food resource availability and the initiation of reproduction of four of the aetideid copepods investigated in this study may be related to carnivorous feeding on small-sized copepods. Since δ15N values of adult females were higher for C. obtusifrons and Aetideopsis spp. than those of Gaetanus spp., the carnivorous feeding modes would be more pronounced for the former species. And common for all aetideid copepods, carnivorous feeding modes were more common during the polar night than those in the midnight sun (Table 4).
In the present study, the reproduction of the four shallower-dwelling aetideid copepods could be classified into two types based on vertical distribution and seasonality (Figure 7). In the first type of reproduction, exhibited by C. obtusifrons and G. tenuispinus, both species ascended to shallower depths during the polar night, and their reproductive period occurred from the end of the polar night into the sunlight recovery period (Figure 7). The timing of reproduction of these species corresponded with the seasonal population increase of the small-sized and abundant copepod: Microcalanus pygmaeus (Ashjian et al., 2003). Since M. pygmaeus is distributed mainly in the upper mesopelagic layer (Auel and Hagen, 2002; Ashjian et al., 2003), the upward migration of C. obtusifrons and G. tenuispinus during the polar night may be an adaptive strategy for prey capture at shallower depths, which may provide an important energy source to support their reproduction. The other type of reproduction was exhibited by G. brevispinus and A. minor. Both these species remained in deeper waters, even during the polar night, and began reproducing at the beginning of the polar night (Figure 7), which was comparatively earlier than for the two shallower-dwelling species. The timing of reproduction of these two deeper-dwelling species corresponded with the timing in reproduction of the large-sized copepod Calanus hyperboreus, which dominates the zooplankton biomass in part of the Arctic Ocean (Conover and Huntley, 1991; Hirche and Niehoff, 1996; Ashjian et al., 2003). During the winter to spring period, C. hyperboreus descends to the mesopelagic layer and sheds floating eggs (Conover and Huntley, 1991; Hirche and Niehoff, 1996; Ashjian et al., 2003). To perform raptorial feeding on the eggs and nauplii of C. hyperboreus, the two deeper-dwelling aetideid copepods, G. brevispinus and A. minor, may remain at the deeper depths during the polar night in order to use the abundance of C. hyperboreus eggs and nauplii as their main energy source for reproduction. These seasonal trends in feeding modes were evidenced by the δ15N values of the aetideid adult specimens (Table 4). The differences in vertical distribution and timing of reproduction among the five sympatric aetideid copepods observed in this study may function to reduce interspecific competition within the resource-limited mesopelagic layers and could be a key mechanism maintaining high biodiversity at these depths in the Arctic Ocean.
5 Conclusions
In this study, we developed a set of metrics to aid species identification of early copepodite stages of five sympatric aetideid copepods (Chiridius obtusifrons, Gaetanus tenuispinus, G. brevispinus, Aetideopsis minor, and A. rostrata), and evaluated vertical distribution and seasonal population structure of these species using a year-round sample time-series collected at the drifting ice station (SHEBA) in the western Arctic Ocean. Combinations of morphological characteristics (prosome length, cephalosome, and prosome widths) were used to identify the early copepodite stages to species. Aetideopsis rostrata was distributed in deep waters throughout the year. The other species distributed at similar depths during the midnight sun, whereas their vertical distribution varied with species during the polar night. Reproduction timing also varied among these four aetideid copepods and occurred at the start or at the end of the polar night, possibly related to food availability. Seasonal distribution dynamics and different reproductive periods may reduce inter-specific competition among aetideid copepods in the Arctic Ocean.
Data availability statement
The original contributions presented in the study are included in the article/Supplementary Material. Further inquiries can be directed to the corresponding author.
Author contributions
YK, KT, CA, RC, and AY designed the study; CA and RC collected the samples; YK and KT analysed the samples; YK and AY analysed the data; YK and AY wrote the paper with contributions from all authors. All authors read and approved the final manuscript.
Funding
Collection of the samples was supported in part by grants #OCE9707184 to CA and #OCE9707182 to RC from the US National Science Foundation. This work was partially supported by the Arctic Challenge for Sustainability II (ArCS II), Program Grant Number JPMXD1420318865. This research was also supported by the Environment Research and Technology Development Fund (JPMEERF20214002) of the Environmental Restoration and Conservation Agency of Japan. In addition, this work was partly supported by a Grant-in-Aid for Challenging Research (Pioneering) JP20K20573, and Scientific Research JP20H03054 (B), JP19H03037 (B), JP21H02263 (B), and JP17H01483 (A) from the Japanese Society for the Promotion of Science (JSPS).
Conflict of interest
The authors declare that the research was conducted in the absence of any commercial or financial relationships that could be construed as a potential conflict of interest.
Publisher’s note
All claims expressed in this article are solely those of the authors and do not necessarily represent those of their affiliated organizations, or those of the publisher, the editors and the reviewers. Any product that may be evaluated in this article, or claim that may be made by its manufacturer, is not guaranteed or endorsed by the publisher.
Acknowledgments
We thank the crew members of the CCGS Des Groseilliers and the SHEBA project members, most especially Harold “Buster” Welch, for assistance in collecting the samples and CTD data.
Supplementary material
The Supplementary Material for this article can be found online at: https://www.frontiersin.org/articles/10.3389/fmars.2022.943100/full#supplementary-material
References
Ashjian C., Campbell R. (2007) Mesozooplankton abundance and biomass. version 1.0 (UCAR/NCAR - Earth Observing Laboratory) (Accessed 27 Jun 2022).
Ashjian C. J., Campbell R. G., Welch H. E., Butler M., VanKeuren D. (2003). Annual cycle in abundance, distribution, and size in relation to hydrography of important copepod species in the western Arctic ocean. Deep-Sea. Res. Part I.: Oceanogr. Res. Pap. 50 (10–11), 1235–1261. doi: 10.1016/S0967-0637(03)00129-8
Auel H. (1999). The ecology of Arctic deep-sea copepods (Euchaetidae and aetideidae). aspects of their distribution, trophodynamics and effect on the carbon flux. Berichte. Zur. Polarforschung. (Report. Pol. Res.) 319, 1–97. doi: 10.2312/BzP_0319_1999
Auel H., Hagen W. (2002). Mesozooplankton community structure, abundance and biomass in the central Arctic ocean. Mar. Biol. 140 (5), 1013–1021. doi: 10.1007/s00227-001-0775-4
Brock T. D. (1981). Calculating solar radiation for ecological studies. Ecol. Model. 14 (1–2), 1–19. doi: 10.1016/0304-3800(81)90011-1
Brodskii K. A. (1950). Calanoida of the far Eastern seas and polar basin of the USSR. Key. To. Fauna. USSR. 35, 1–440.
Conover R. J., Huntley M. (1991). Copepods in ice-covered seas–distribution, adaptations to seasonally limited food, metabolism, growth patterns and life cycle strategies in polar seas. J. Mar. Syst. 2 (1–2), 1–41. doi: 10.1016/0924-7963(91)90011-l
Dilling L., Wilson J., Steinberg D., Alldredge A. (1998). Feeding by the euphausiid Euphausia pacifica and the copepod Calanus pacificus on marine snow. Mar. Ecol. Prog. Ser. 170, 189–201. doi: 10.3354/meps170189
Goetze E. (2003). Cryptic speciation on the high seas; global phylogenetics of the copepod family eucalanidae. Proc. R. Soc. Lond. Ser. B.: Biol. Sci. 270 (1531), 2321–2331. doi: 10.1098/repb.2003.2505
Hirche H. J., Niehoff B. (1996). Reproduction of the Arctic copepod Calanus hyperboreus in the Greenland Sea-field and laboratory observations. Pol. Biol. 16 (3), 209–219. doi: 10.1007/BF02329209
Honjo S., Krishfield R. A., Eglinton T. I., Manganini S. J., Kemp J. N., Doherty K., et al. (2010). Biological pump processes in the cryopelagic and hemipelagic Arctic ocean: Canada basin and chukchi rise. Prog. Oceanogr. 85 (3–4), 137–170. doi: 10.1016/j.pocean.2010.02.009
Horton T., Marsh L., Bett B. J., Gates A. R., Jones D. O. B., Benoist N. M. A., et al. (2021). Recommendations for the standardisation of open taxonomic nomenclature for image-based identifications. Front. Mar. Sci. 8. doi: 10.3389/fmars.2021.620702
Kosobokova K., Hirche H. J. (2000). Zooplankton distribution across the lomonosov ridge, Arctic ocean: species inventory, biomass and vertical structure. Deep-Sea. Res. Part Ⅰ: Oceanogr. Res. Pap. 47 (11), 2029–2060. doi: 10.1016/S0967-0637(00)00015-7
Kosobokova K. N., Hirche H. J., Hopcroft R. R. (2007). Reproductive biology of deep-water calanoid copepods from the Arctic ocean. Mar. Biol. 151 (3), 919–934. doi: 10.1007/s00227-006-0528-5
Kosobokova K. N., Hopcroft R. R. (2010). Diversity and vertical distribution of mesozooplankton in the arctic’s Canada basin. Deep-Sea Res Part. II: Trop. Stud. Oceanogr. 57 (1–2), 96–110. doi: 10.1016/j.dsr2.2009.08.009
Kosobokova K. N., Hopcroft R. R., Hirche H. J. (2011). Patterns of zooplankton diversity through the depths of the arctic’s central basins. Mar. Biodivers. 41 (1), 29–50. doi: 10.1007/s12526-010-0057-9
Laakmann S., Auel H. (2010). Longitudinal and vertical trends in stable isotope signatures (δ13C and δ15N) of omnivorous and carnivorous copepods across the south Atlantic ocean. Mar. Biol. 157, 463–471. doi: 10.1007/s00227-009-1332-9
Laakmann S., Kochzius M., Auel H. (2009a). Ecological niches of Arctic deep-sea copepods: Vertical partitioning, dietary preferences and different trophic levels minimize inter-specific competition. Deep-Sea. Res. Part Ⅰ: Oceanogr. Res. Pap. 56 (5), 741–756. doi: 10.1016/j.dsr.2008.12.017
Laakmann S., Stumpp M., Auel M. (2009b). Vertical distribution and dietary preferences of deep-sea copepods (Euchaetidae and aetideidae; calanoida) in the vicinity of the Antarctic polar front. Pol. Biol. 32 (5), 679–689. doi: 10.1007/s00300-008-0573-2
Lee C. E. (2000). Global phylogeography of a cryptic copepod species complex and reproductive isolation between genetically proximate “populations”. Evolution 54 (6), 2014–2027. doi: 10.1111/j.0014-3820.2000.tb01245.x
Lowry L. F., Sheffield G., George C. (2004). Bowhead whale feeding in the alaskan Beaufort Sea, based on stomach contents analyses. J. Cetacean. Res. Manage. 6 (3), 215–223.
Marin V. (1987). The oceanographic structure of the eastern Scotia–IV. distribution of copepod species in relation to hydrography in 1981. Deep-Sea. Res. Part A. Oceanogr. Res. Pap. 34 (1), 105–121. doi: 10.1016/0198-0149(87)90125-7
Markhaseva E. L. (1996). Calanoid copepods of the family aetideidae of the world ocean. Proc. Zool. Inst. Russian Acad. Sci. St. Petersburg. 268, 1–331.
Norris R. D. (2000). Pelagic species diversity, biogeography, and evolution. Paleobiology 26 (S4), 236–258. doi: 10.1017/S0094837300026956
O’Brien M. C., Macdonald R. W., Melling H., Iseki K. (2006). Particle fluxes and geochemistry on the Canadian Beaufort shelf: Implications for sediment transport and deposition. Continent. Shelf. Res. 26 (1), 41–81. doi: 10.1016/j.csr.2005.09.007
Ozaki K., Ikeda T. (1997). The effect of temperature on the development of eggs and nauplii of the mesopelagic copepod Paraeuchaeta elongata. Plankt. Biol. Ecol. 44 (1/2), 91–95.
Pennak R. W. (1943). An effective method of diagramming diurnal movements of zooplankton organisms. Ecology 24 (3), 405–407. doi: 10.2307/1930542
Richter C. (1994). Regional and seasonal variability in the vertical distribution of mesozooplankton in the Greenland Sea. Berichte. Zur. Polarforschung. (Report. Pol. Res.) 154, 1–87. doi: 10.2312/BzP_0154_1994
Richter C. (1995). Seasonal changes in the vertical distribution of mesozooplankton in the Greenland Sea gyre (75°N): distribution strategies of calanoid copepods. ICES J. Mar. Sci. 52 (3–4), 533–539. doi: 10.1016/1054-3139(95)80067-0
Sano M., Maki K., Nishibe Y., Nagata T., Nishida S. (2013). Feeding habits of mesopelagic copepods in sagami bay: Insights from integrative analysis. Prog. Oceanogr. 110, 11–26. doi: 10.1016/j.pocean.2013.01.004
Sano M., Nishibe Y., Tanaka Y., Nishida S. (2015). Temporally sustained dietary niche partitioning in two mesopelagic copepod species and their mouthpart morphology. Mar. Ecol. Prog. Ser. 518, 51–67. doi: 10.3354/meps11045
Sherr B. F., Sherr E. B. (2003). Community respiration/production and bacterial activity in the upper water column of the central Arctic ocean. Deep-Sea. Res. Part Ⅰ: Oceanogr. Res. Pap. 50 (4), 529–542. doi: 10.1016/S0967-0637(03)00030-X
Sherr E. B., Sherr B. F., Wheeler P. A., Thompson K. (2003). Temporal and spatial variation in stocks of autotrophic and heterotrophic microbes in the upper water column of the central Arctic ocean. Deep-Sea. Res. Part Ⅰ: Oceanogr. Res. Pap. 50 (5), 557–571. doi: 10.1016/S0967-0637(03)00031-1
Smoot C. A., Hopcroft R. R. (2017). Depth-stratified community structure of Beaufort Sea slope zooplankton and its relations to water masses. J. Plankt. Res. 39 (1), 79–91. doi: 10.1093/plankt/fbw087
Thibault D., Head E. J. H., Wheeler P. A. (1999). Mesozooplankton in the Arctic ocean in summer. Deep-Sea. Res. Part Ⅰ: Oceanogr. Res. Pap. 46 (8), 1391–1415. doi: 10.1016/S0967-0637(99)00009-6
Wassmann P., Reigstad M., Haug T., Rudels B., Carroll M. L., Hop H., et al. (2006). Food webs and carbon flux in the barents Sea. Prog. Oceanogr. 71 (2–4), 232–287. doi: 10.1016/j.pocean.2006.10.003
Wilson S. E., Steinberg D. K., Chu F. L. E., Bishop J. K. B. (2010). Feeding ecology of mesopelagic zooplankton of the subtropical and subarctic north pacific ocean determined with fatty acid biomarkers. Deep-Sea. Res. Part Ⅰ: Oceanogr. Res. Pap. 57 (10), 1278–1294. doi: 10.1016/j.dsr.2010.07.005
Yamaguchi A., Ashjian C. J., Campbell R. G., Abe Y. (2020). Vertical distribution, population structure and developmental characteristics of the less studied but globally distributed mesopelagic copepod Scaphocalanus magnus in the western Arctic ocean. J. Plankt. Res. 42 (3), 368–377. doi: 10.1093/plankt/fbaa021
Yamaguchi A., Ikeda T. (2000). Vertical distribution, life cycle, and developmental characteristics of the mesopelagic calanoid copepod Gaidius variabilis (Aetideidae) in the oyashio region, western north pacific ocean. Mar. Biol. 137 (1), 99–109. doi: 10.1007/s002270000316
Keywords: Aetideidae, sympatric mesopelagic copepods, vertical distribution, population structure, reproduction, the Arctic Ocean
Citation: Koguchi Y, Tokuhiro K, Ashjian CJ, Campbell RG and Yamaguchi A (2022) Inter-species comparison of the copepodite stage morphology, vertical distribution, and seasonal population structure of five sympatric mesopelagic aetideid copepods in the western Arctic Ocean. Front. Mar. Sci. 9:943100. doi: 10.3389/fmars.2022.943100
Received: 13 May 2022; Accepted: 28 July 2022;
Published: 19 August 2022.
Edited by:
Daniel J. Mayor, National Oceanography Centre, United KingdomReviewed by:
Kathryn Barbara Cook, University of Southampton, United KingdomMarianne Wootton, Marine Biological Association, United Kingdom
Copyright © 2022 Koguchi, Tokuhiro, Ashjian, Campbell and Yamaguchi. This is an open-access article distributed under the terms of the Creative Commons Attribution License (CC BY). The use, distribution or reproduction in other forums is permitted, provided the original author(s) and the copyright owner(s) are credited and that the original publication in this journal is cited, in accordance with accepted academic practice. No use, distribution or reproduction is permitted which does not comply with these terms.
*Correspondence: Yunosuke Koguchi, bWFrYXJvbmkxMDA5QGVpcy5ob2t1ZGFpLmFjLmpw