- 1Chinese Academy of Sciences (CAS) and Shandong Province Key Laboratory of Experimental Marine Biology, Center for Ocean Mega-Science, Institute of Oceanology, Chinese Academy of Sciences, Qingdao, China
- 2Laboratory for Marine Biology and Biotechnology, Qingdao National Laboratory for Marine Science and Technology, Qingdao, China
- 3University of Chinese Academy of Sciences, Beijing, China
- 4Southern Marine Science and Engineering Guangdong Laboratory (Guangzhou), Guangzhou, China
- 5School of Life Science and Engineering, Qingdao Agricultural University, Qingdao, China
Sebastes schlegelii is a commercially important fishery resource that is also a representative viviparous fish species in the Northeast Asian Sea. The mode of nutritional supply of embryos fertilized and developed in vivo is not clear. To screen biomarkers for nutrient transfer between mother and embryo, we studied the composition of and changes in maternal plasma at different developmental stages. The results showed that the plasma of S. schlegelii was rich in nutrients, including 16 hydrolysed amino acids, 20 fatty acids, glucose and fructose. The contents of amino acids in the plasma reached 3.44-4.58 g/100 g, which is approximately 3-5 times that of fatty acids and 44-155 times that of sugar. Thirteen amino acids, 15 fatty acids and glucose showed an increasing trend in the early stage of embryonic development and a decreasing trend in the later stage. The contents of leucine, as an essential amino acid in early development, changed significantly in maternal blood and was therefore selected as a biomarker. The results of isotope labelling showed that 15N leucine was not detected in the eggs, gastrula embryos or organogenesis embryos, but a high abundance of 14N leucine was detected in the eggs and embryos of the control and experimental groups, indicating that leucine, an essential amino acid for aquatic animals, plays an important role in gamete and embryo development. An abundance in 15N-labelled leucine was detected in the embryonic blood and ovarian villi but not in the embryos, which indicates that the ample free naturally abundant leucine in maternal blood during the middle and late developmental stages of the eggs and embryos was not absorbed or utilized. These results showed that eggs and embryos had certain autonomy and selectivity when absorbing nutrients and gave priority to the active transport of certain complex macromolecular nutrients that could meet their needs for a variety of nutrients at the same time.
Introduction
Sebastes schlegelii is a cold-temperate species belonging to the family Sebastidae and the genus Sebastes (Matsubara, 1943; Cheng and Zheng, 1987), its common name called “black rockfish”. It is widely distributed along the coast of northern China, the Korean Peninsula, Japan and south of Okhotsk (Yamada et al., 2007; Nakabo, 2010). As a benthic reef loving fish, S. schlegelii prefers to live in an environment with abundant gravel, rock reef and algae. This fish is an important part of the nearshore ecosystem (Chen, 1991). To protect the ecosystem, black rockfish have become an essential value-added release subject, and the release of offspring with different specifications are carried out every year (Liu et al., 2009; Lv, 2013). The value of S. schlegelii is reflected in both ecology and the economy. S. schlegelii produces a large number of eggs and provides rich nutrition and delicious meat. It is deeply loved by people and is an important breeding subject along the northern coast (Feng and Zhang, 1995; Li et al., 2017).
Sugar, fat and protein are the three most important nutrients of life, and they are also necessary nutritional elements for embryonic growth and development. Most marine bony fish are oviparous and undergo in vitro fertilization and in vitro development. Thus, maternal nutrition supply is not involved in the embryonic development stage. After the hatched larvae open their mouths, the mode of nutrition changes from endogenous to exogenous, and the absorption of nutrients is mainly completed through digestive organs (Xinwei et al., 2020). As a typical viviparous fish, the embryonic development of S. schlegelii is completed in the mother’s body (Yamada and Kusakari, 1991; Lei et al., 2005). It was found that fertilized embryos of S. schlegelii can complete their early development and enter the stage of delivery only after 1-1.5 months in females (Chen et al., 1994; Mori et al., 2003; Koya, 2008). The way in which typical nutrients are obtained, absorbed and utilized by these embryos in different developmental stages remain unclear. This leads to a lack in the suitability of nutrition enhancement during the later stage of reproduction regulation and before delivery, resulting in uneven quality of the offspring after delivery, affecting the stable supply of high-quality offspring and restricting the sustainable development of the breeding industry (Liu et al., 2009; Kai and Soes, 2009).
Previous studies have found that, compared with the ovarian structure of female parent oviparous fish, the ovaries of S. schlegelii are rich in ovarian fluid before fertilization and throughout the process of embryo development and have developed villous connective tissue (Zhao, 2017; Yang, 2018; Du et al., 2021). As a large number of eggs are conceived by S. schlegelii, the number of offspring reaches 314000/female fish (Zhu and Sui, 1999). Accordingly, there is a demand for high-energy nutrition during long-term in vivo development, as the nutrition from the endogenous yolk sac is not sufficient to support the completion of embryo development. Thus, does the mother use special ovarian connective tissue to provide through the blood nutrients for embryo development? Therefore, in view of the unique and developed connective tissue in the ovaries of S. schlegelii, we first selected the typical development stages (prefertilization, gastrula stage, somite stage and organ formation stage) to clarify the changes in the nutrient composition and contents in the blood and ovarian connective tissue of the female parent S. schlegelii at different development times. Second, by using amino acid stable isotope tracing technology, we tracked and confirmed whether the typical amino acids in the blood of S. schlegelii can enter the ovarian connective tissue or the embryo to provide direct research evidence for whether the mother can provide the embryo with nutrients.
Materials and methods
Experimental materials
The S. schlegelii used in this experiment were collected from the fixed-point breeding cage of S. schlegelii in Yantai, Shandong Province. According to the water environment and the ovarian situation, samples were taken irregularly. Twelve female fish were selected, including 3 female fish before fertilization (the fourth stage ovary, which has basically completed the accumulation of yolk material), 3 female fish in the gastrula stage, 3 female fish in the somite stage and 3 female fish in the organogenesis stage, from March to May. In the process of sampling, MS-222 at a concentration of 0.05% was used to anaesthetize the fish, and ovarian development was then determined by anatomical and stereoscopic observation. Finally, the samples were fixed according to the experimental requirements. The blood samples used in this experiment were processed as follows. First, blood was collected under the spine with a 10 ml disposable sterile syringe and placed in an anticoagulant tube. The samples were then centrifuged in a frozen centrifuge at 5000 rpm and for 15 min. Serum was placed in a frozen tube and stored in a freezer at -80°C for later analysis.
Four S. schlegelii in the same cage at the same stage of development were selected. One was treated to observe the development of the ovaries, and the remaining three were labelled with isotopes. Finally, three periods were selected to complete the isotope labelling experiment, including prefertilization, gastrulation and organogenesis. 15N-labelled stable isotope leucine powder (Shanghai Research Institute of Chemical Industry) was diluted with 0.9% sodium chloride to a final concentration of 0.02 g/ml. Leucine injections was injected in three times with 3 ml of solution injected each time (Li et al., 2003; Tian et al., 2011; Hou et al., 2016). The second injection was conducted 12 hours after the first injection, and the third injection was conducted 12 hours after the second injection. Three injections within 24 hours. After the last injection, samples were taken 36-48 h according to the state of the fish (Li et al., 2003; Tian et al., 2011; Hou et al., 2016). Oocyte and embryo sample processing was then carried out as follows. The ovarian wall was cut open, the oocytes and embryos were quickly rinsed with DEPC(diethy pyrocarbonate)treated water, placed in cryopreserved tubes, quick-frozen in liquid nitrogen, and stored in a -80°C refrigerator for later component analysis. Stable isotope samples were taken from the maternal blood, periovarian tissue and oocytes or embryos at the three developmental stages.
Experimental methods
Amino acid determination
The determination of amino acids was carried out in accordance with method GB5009.124-2016. Pretreatment method: In this experiment, a 0.5 g sample was put into a hydrolysis tube, 20 ml of HCl (8.3 mol/L) was added, and hydrolysis was carried out in an electric blast drying oven at 110°C. After 22-24 h, the solution was removed for cooling and transferred to a 25 ml volumetric tube and diluted to volume. One millilitre of supernatant was separated and dried in a water bath (85°C), and 1 ml of water was added before drying again. Then, 10 ml of 0.02 mol/l HCl was added, and the solution was mixed well. This study used 500 μL of mixed liquid with 250 μL of phenyl isothiocyanate in acetonitrile (0.1 mol/L) and 250 μL of triethylamine in acetonitrile (1 mol/L) for derivatization. After 1 hour, 2 ml of n-hexane was added followed by oscillation and static delamination. The lower layer was taken through a 0.45 μm organic film and tested on the instrument.
Instrument method: The selected chromatographic column was a C18 SHISEIDO (4.6 mm × 250 mm × 5 μm), the injection volume was 10 μL, the column temperature was 40°C, and the detection wavelength was 254 nm. The mobile phase consisted of solvents A and B, where solvent A was 0.1 mol/L anhydrous sodium acetate + acetonitrile (volume ratio of 97:3). After mixing, the pH was adjusted to 6.5 (31.815 g of sodium acetate +3880 mL water +120 mL of acetonitrile). Solvent B was acetonitrile + water (volume ratio of 80:20). The elution gradient is shown in Table 1.
Formula to calculate the amino acid content: where X is the amino acid content; C is the sample concentration in mg/L; C0 is the normal concentration in mg/L; V is the constant volume in mL; N is the dilution ratio; and M is the sample mass in g.
Fatty acid determination
The determination of fatty acids was carried out in accordance with method GB5009.168-2016. Sample hydrolysis: We weighed 0.3 g of sample into a beaker, and added 100 mg of pyrogallic acid, several grains of zeolite and 2 ml of 95% ethanol. After mixing well 10 ml of hydrochloric acid solution was added. In this study, after fully mixing, the sample was hydrolysed in a water bath at 70-80°C with oscillation every 10 min for a total hydrolysis time of 40-50 min.
Fat extraction: First, 10 ml of ethanol (95%) was added to the hydrolysed sample, which was mixed well and transferred to a separatory funnel. The flask was rinsed and stoppered after the addition of 50 ml of a mixture of ether and petroleum ether followed by shaking for 5 minutes and then standing. After 10 min, the ether layer was collected into a flask. After repeating the above procedure 3 times, the contents of the separatory funnel were washed with an ether–petroleum ether mixture and collected into a flask. The solvent was evaporated in a water bath and the residue was dried in an oven at 100°C ± 5°C for 2 h.
Fat saponification and fatty acid methyl esterification: First, 2 ml of a 2% sodium hydroxide methanol solution was added to the fat extract, and this mixture was heated in an 85°C water bath for 30 min. After adding 3 ml of a 4% boron trifluoride methanol solution, and heating continued for 30 min. After heating, the temperature was reduced to room temperature, 1 ml of n-hexane was added to the centrifuge tube followed by shaking, extracting for 2 min, and centrifuging again. After 1 hour, 100 μL of the supernatant was diluted to 1 ml with n-hexane. A sample filtered through a 0.45 μM filter membrane was analysed on the instrument.
Instrument method: The selected chromatographic column was a cd-2560 (100 m) × 0.25 mm × 0.02 μm). The heating procedure was as follows: holding at 130°C for 5 min, increasing the heating rate at 4°C/min, and holding at 240°C for 30 min. The temperature of the injection port was 250°C; the flow rate of the carrier gas was 0.5 ml/min; a 10:1 split injection was performed; the detector was an FID; and the detector temperature was 250°C.
Calculation formula: The fatty acid content was calculated according to the formula where W is the fatty acid content in mg/kg; C is the fatty acid methyl ester concentration in mg/L; V is the constant volume in mL; k is the fatty acid methyl ester to fatty acid conversion coefficient as shown in Table 2; N is the dilution multiplier; and m is the sample weight in g.
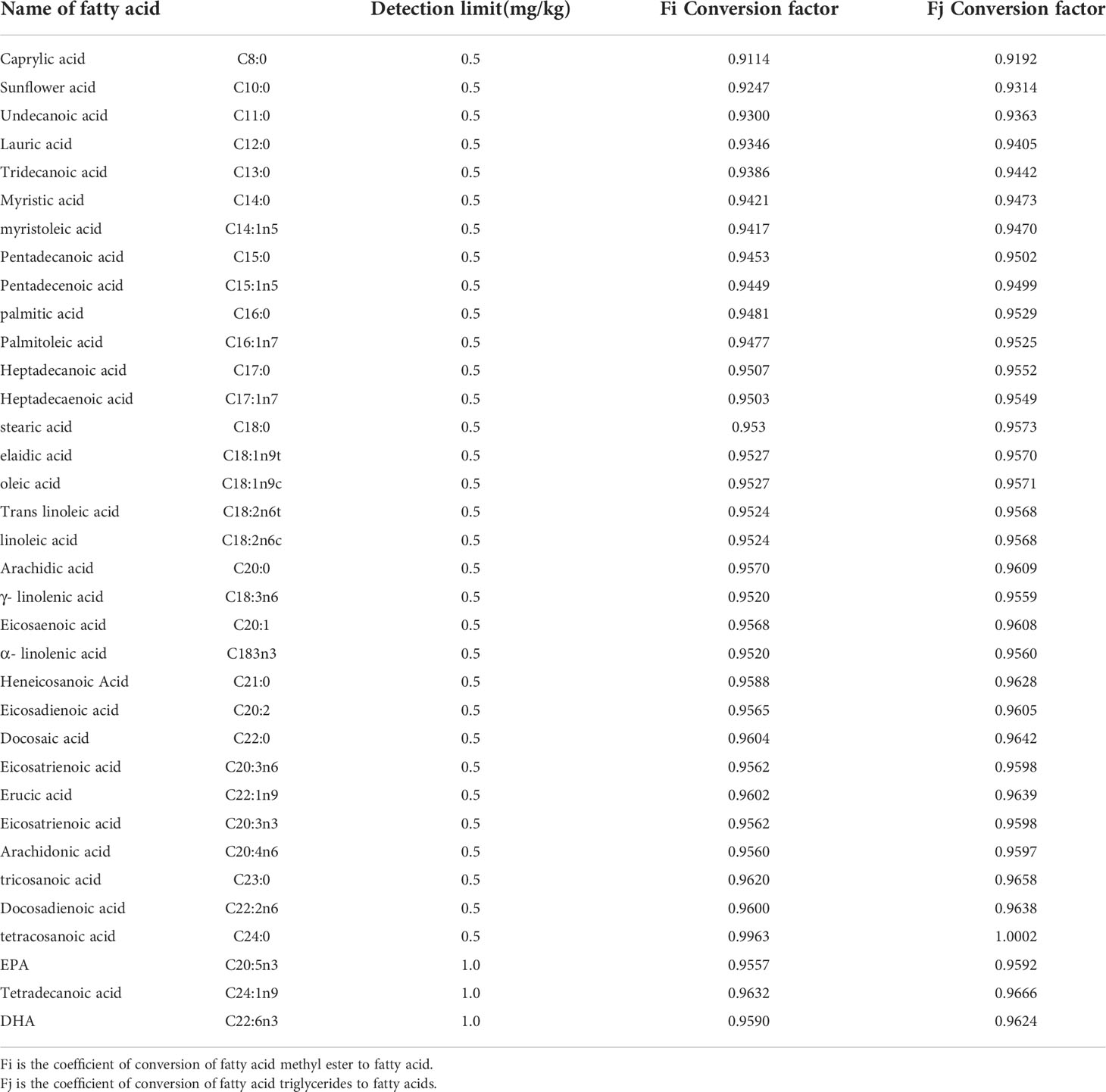
Table 2 Fatty acid types and detection limits and conversion coefficients of fatty acid methyl esters or fatty acid triglycerides to fatty acids.
Glucose and fructose determination
The determination of glucose and fructose were carried out in accordance with method “GB5009.8-2016”. We weighed 0.5 g of sample, added 2 ml of acetonitrile, and centrifuged the solution at 6000 r/min for 5 min. The supernatant was passed through a 0.45 μM filter membrane for analysis. The chromatographic column used was an Agilent amino column (250 × 4.6 mm × 5 µm). The detector was a differential detector. The column temperature was 35°C. The injection volume was 10 μL. The mobile phase was acetonitrile: water = 70:30 (volume ratio). The flow rate was 1.0 ml/min.
Formula to calculate the sugar content: where X is the glucose content; C is the sample concentration in mg/L; C0 is the normal concentration in mg/L; V is the constant volume in mL; N is the dilution ratio; and M is the sample weight in g.
Stable isotope tracer experiment
First, 0.5 g of sample was frozen and crushed with liquid nitrogen. Second, 3-5 ml of ultrapure water was added, the solution was mixed and shaken for 30 min and then centrifuged, and the supernatant was collected. Leucine was extracted by shaking on a shaking table for 1 hour. Third, each sample was centrifuged at 10000 r/min for 20 min, and the supernatant was collected for analysis.
The supernatant was placed in a vacuum freeze dryer, and the excess water was removed under vacuum at -60°C by freeze-drying. A total of 80 μL of pyridine and 80 μL of N-Methyl-N-tert-butyldimethylsilyl trifluoroacetamide (MTBSTFA) were added to the dry sample and incubated at 70°C for 4 hours. After cooling to room temperature, the derivatized samples were briefly centrifuged, and the transparent liquid was transferred to a gas chromatographic vial for gas chromatography/mass spectrometry analysis. An Agilent 7890b gas chromatography system and an HP-5MS capillary column (30 m, 0.25 mmi. D, 0.25 µm) were connected to an Agilent 7000c mass spectrometer and operated under 70 eV of electron impact ionization (EI). The obtained target isotope data were corrected according to the natural isotope abundance.
At the injector, 1 µL of sample was injected and helium was used as the carrier gas at a flow rate of and 1 ml/min. A glass wool super inert liner was used, and the injection temperature was 285°C. The injection needle was washed with methanol 4 times before and after each injection. Temperature gradient for GC-MS analysis of the derivatized samples: The starting temperature was 80°C, increased to 150°C at a rate of 8°C/min, further increased at a rate of 10°C/min to 280°C, which was maintained for 20 minutes.
KingDraw was used to generate the chemical structure diagram, Origin was used to construct the total ion flow diagram (TIC), Excel was used to make the isotope EI mass spectrum diagram, and PPT and Photoshop were used to process the pictures. The significance of the experimental data was analysed by the Tukey method in SPSS.
Results
The results showed that there were significant differences in the contents of amino acids, fatty acids and sugars in the plasma of female fish in the prefertilization stage, gastrula stage, somite stage and organogenesis stage. Additionally, the contents of amino acids were the highest, fatty acids were the second highest, and glucose was the lowest during the different developmental stages. There were also significant differences in the composition and contents of the same nutrient elements; notably, there were differences in the contents of the three major nutrient elements, and second, significant differences in the same nutrient element at different developmental stages (Figure 1). In the three samples measured at each developmental stage, the concentration range of all amino acids was 0.00-1.38 g/100g; The concentration range of fatty acids was 0.00-0.45 g/100g; The total concentration range of glucose and fructose was 0.00-0.15 g/100g; The content of total amino acids in maternal plasma before fertilization, early embryonic development, middle embryonic development and late embryonic development was 4.58 g/100g, 5.33 g/100g, 4.31 g/100g and 3.44 g/100g respectively. The content of total fatty acids in maternal plasma in the four development stages was 1.06 g/100g, 1.26 g/100g, 1.48 g/100g and 0.65 g/100g respectively. The content of total glucose and fructose in maternal plasma was 0.04 g/100g, 0.08 g/100g, 0.10 g/100g and 0.08 g/100g respectively. In the early stage of fertilization, the average amino acid content in the plasma of the female fish was 4.33 times that of the contents of fatty acids and 114.92 times that of sugar. During gastrulation, the average amino acid contents in the plasma of the female fish was 4.24 times that of the contents of fatty acids and 63.93 times that of sugar. At the somite stage, the average amino acid contents in the plasma of the female fish was 2.92 times that of the contents of fatty acids and 43.64 times that of sugar. During organogenesis, the average amino acid contents in the plasma of the female fish was 5.26 times that of the contents of fatty acids and 43.62 times that of sugar.
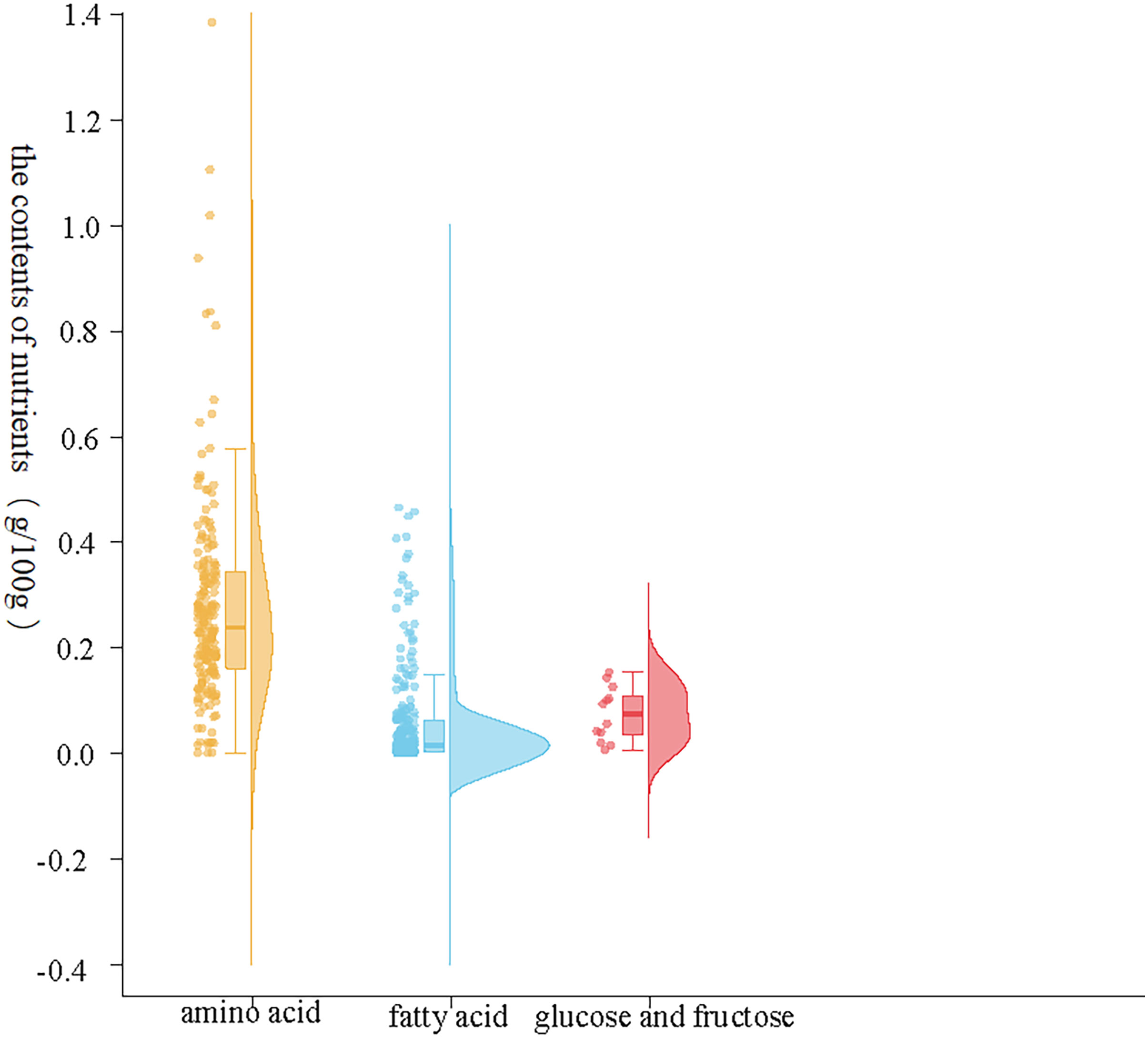
Figure 1 Contents of the three major nutrients in maternal plasma. The abscissa represents nutrient elements, in which orange represents the change of amino acid content, blue represents the change of fatty acid content, red represents the change of glucose and fructose content, and the ordinate represents the content composition value.
The abscissa represents nutrient elements, in which orange represents the change of amino acid content, blue represents the change of fatty acid content, red represents the change of glucose and fructose content, and the ordinate represents the content composition value.
Changes in amino acid contents in the plasma of female S. schlegelii
The determination results from 17 hydrolysed amino acids in maternal plasma before fertilization, early embryonic development (gastrula stage), middle stage (somite stage) and late stage (organogenesis stage) showed that there were 16 hydrolysed amino acids in S. schlegelii maternal plasma. There were 9 essential amino acids (histidine, arginine, threonine, valine, isoleucine, methionine, phenylalanine, leucine and lysine) and 7 nonessential amino acids (proline, glutamate, alanine, tyrosine, serine, aspartic acid and glycine) that could be synthesized by the organisms. The composition and contents of amino acids in the four developmental stages are shown in Table 3. Among the five amino acids with the highest contents (proline, leucine, glutamate, valine and alanine), there were two essential amino acids, leucine and valine. The proline content was the highest among all amino acids. The proline content in 100 g of plasma reached 0.8 g. The proline content in the plasma of S. schlegelii was particularly high, and the histidine content was particularly low, at 0.04 g/100 g. In addition, the contents of the other 14 amino acids were between 0.14-0.42 g/100 g, and their average value was 0.26 g/100 g. In general, the nutritional composition was relatively balanced.
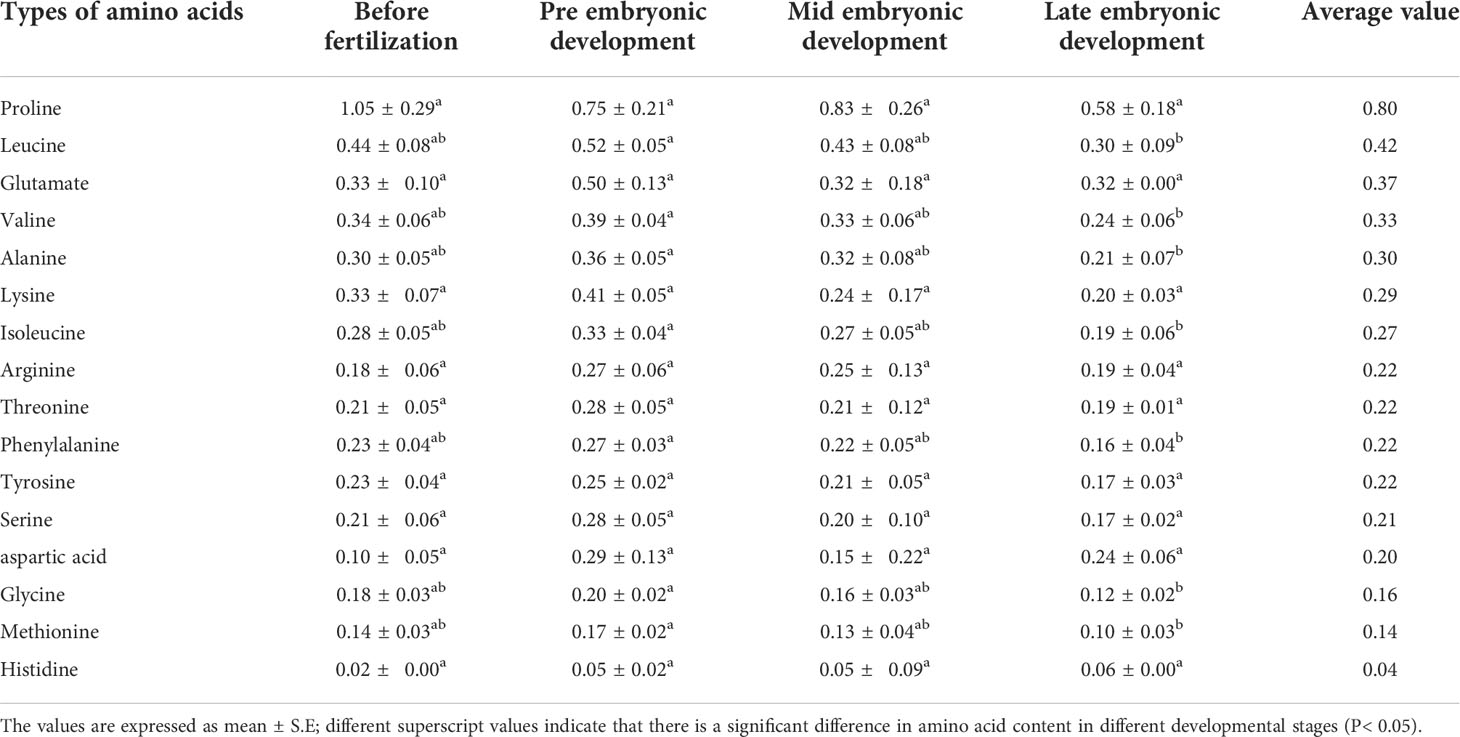
Table 3 Plasma amino acids content of three samples of S. schlegelii in different developmental stages (g/100 g).
The Tukey method in SPSS was used to analyse the significance of the experimental data. The results showed that there were 7 kinds of amino acids with significant differences. According to the contents in plasma from high to low, the amino acids with significant differences were leucine, valine, alanine, isoleucine, phenylalanine, glycine and methionine. Except for alanine and glycine, these amino acids are all essential. Moreover, these seven amino acids showed the same change trend, was as they were present in significantly lower quantities during the late stage of embryonic development than in the early stage of embryonic development.
In the different developmental stages, the species composition of amino acids was the same, but the contents were different. The proline content increased in the later stage of the embryo, but decreased in the early stage (Figure 2). The difference between proline and the other amino acids was that the content of proline decreased in the early stage of embryonic development. The contents of aspartic acid and histidine in the later stage of embryonic development did not decrease significantly and were higher than those before fertilization, and the contents throughout the embryonic development stage were higher than those before fertilization. The change trends of the other four amino acids with high contents in plasma were as follows. The contents of leucine, valine and alanine in the early stage of embryonic development were the highest, which were higher than those before fertilization, and the contents declined throughout embryonic body development. The content of glutamate was the highest in the early stage of embryonic development, and the content then decreased followed by an increase during embryonic development.
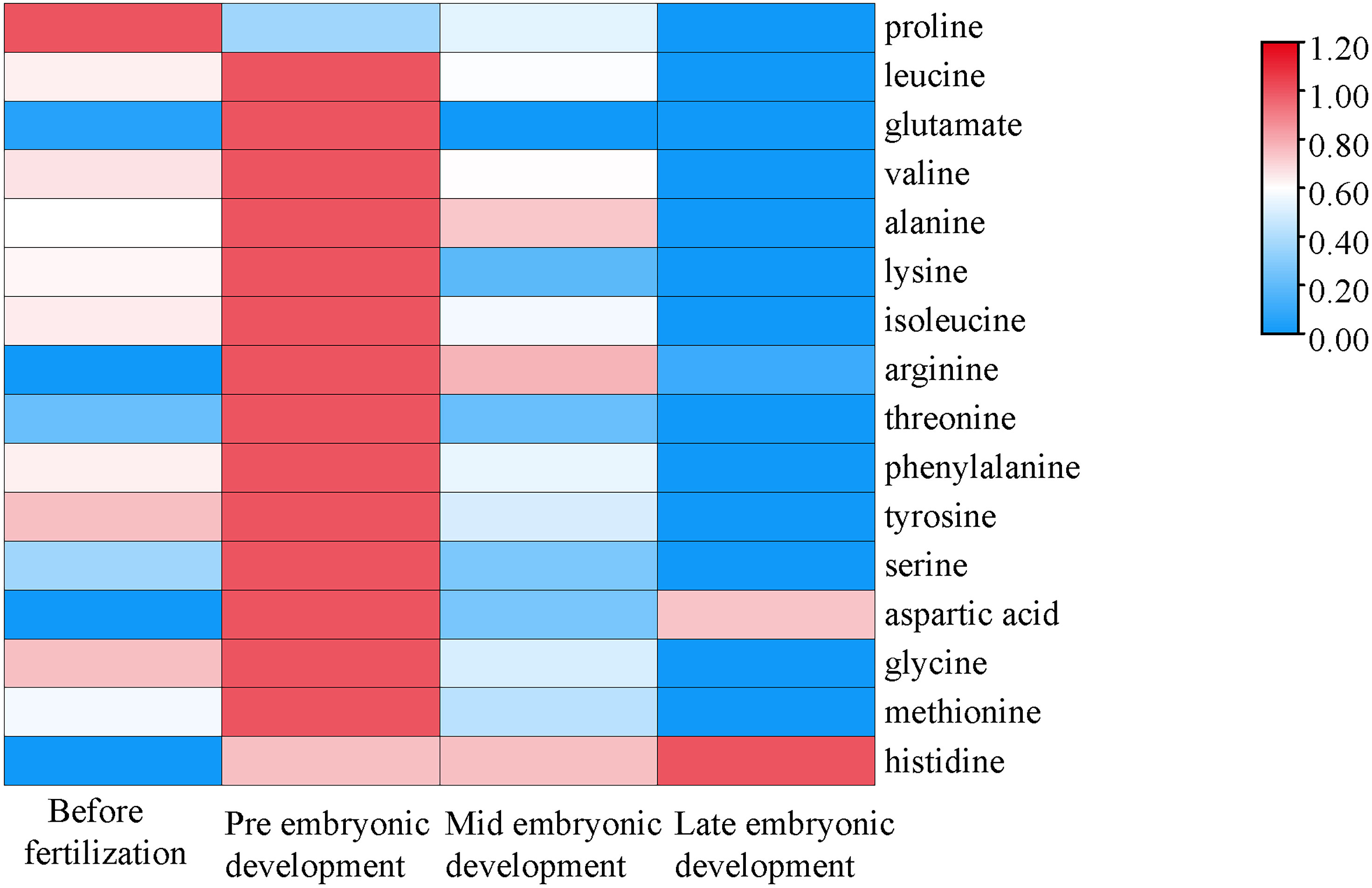
Figure 2 Changes in amino acid contents during different developmental stages. The abscissa represents the time sequence of development, respectively before fertilization, pre embryonic development, mid embryonic development and late embryonic development and the ordinate represents the changes in plasma composition of amino acids at different development stages.
The abscissa represents the time sequence of development, respectively before fertilization, pre embryonic development, mid embryonic development and late embryonic development and the ordinate represents the changes in plasma composition of amino acids at different development stages.
Changes in fatty acid content in the plasma of female S. schlegelii
The fatty acid determination results from the four developmental periods showed that there were 20 kinds of fatty acids in maternal plasma, including the essential fatty acids linoleic acid, α-linolenic acid, arachidonic acid, EPA and DHA. The composition and contents of fatty acids during the four developmental periods are shown in Table 4. There were five kinds of fatty acids with contents greater than 0.05 g/100 g, including DHA (0.2922 g/100 g), palmitic acid (0.2899 g/100 g), EPA (0.1557 g/100 g), oleic acid (0.1071 g/100 g) and erucic acid (0.0711 g/100 g). Generally, the fatty acid contents were not as high as those of the amino acids and were also very uneven. There were 8 kinds of fatty acids with contents lower than 0.005 g for every 100 g of plasma, and the fatty acid with the highest content was 365 times greater than that with the lowest content.
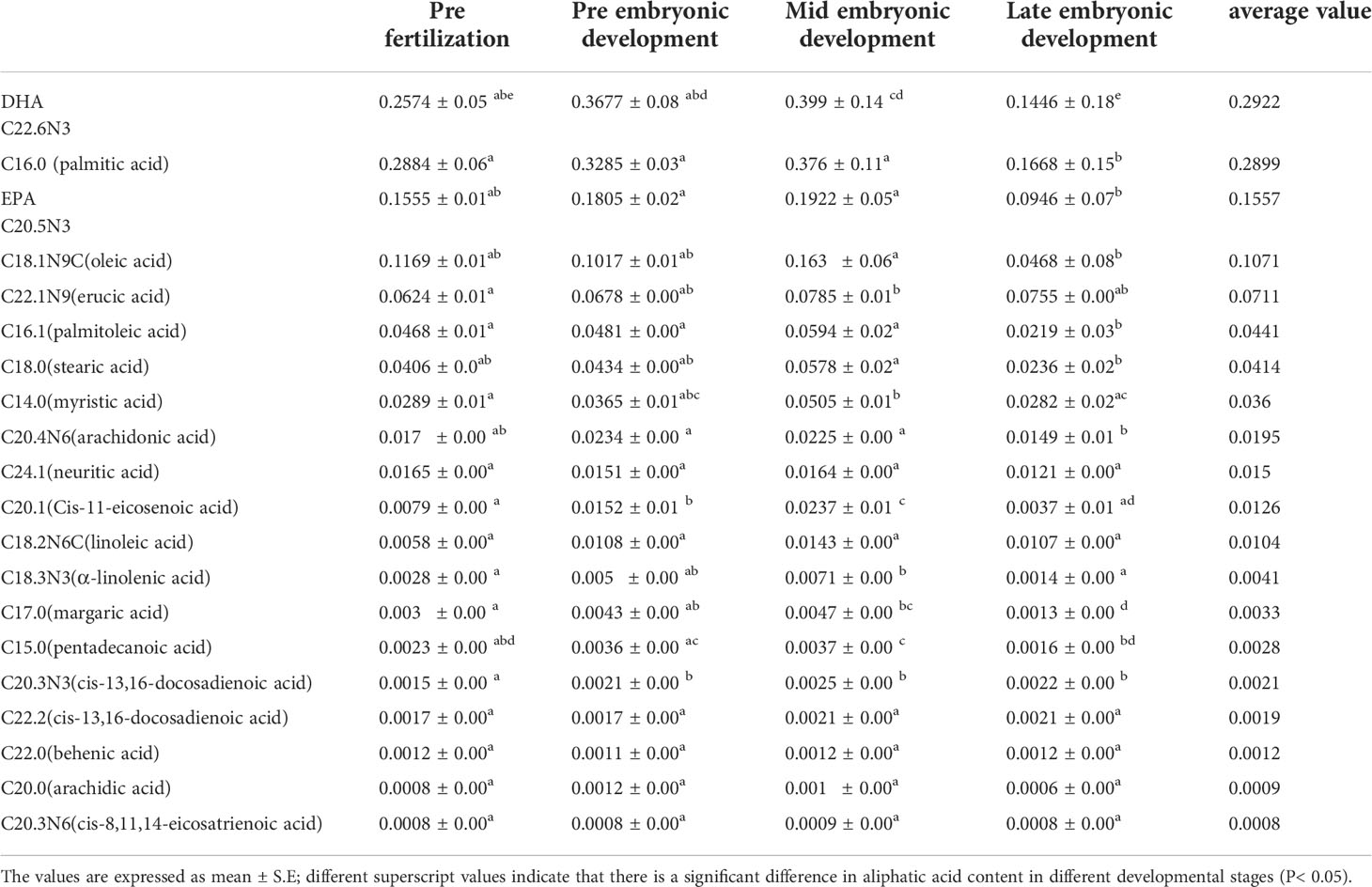
Table 4 Plasma fatty acids content of three samples of S. schlegelii in different developmental stages (g/100 g).
Significance analysis of the experimental data showed that there were more fluctuations in plasma fatty acids during the different periods, and there were no significant differences in only 6 out of the 22 fatty acids. The significance analysis results of the five fatty acids with the highest contents (DHA, palmitic acid, EPA, oleic acid and erucic acid) showed that the content of DHA first increased and then decreased during the different developmental stages of S. schlegelii (Table 4). Its content in the middle of embryonic development was the highest, reaching 0.399 g/100 g of plasma, which was significantly higher than that in the early stage of fertilization. After the middle stage, the content declined, and was significantly lower in the late stage of embryonic development than in the early and middle stages. The content of palmitic acid in the plasma also increased first and then decreased, with the highest content in the middle stage (0.376 g/100 g), and the content during the later stage of embryonic development was significantly lower than that in the first three stages. The overall change trend in EPA content was similar to that of the above two fatty acids, as it was significantly lower in the later stage than in the early and middle stages of embryonic development. Oleic acid decreased slightly during embryonic development, from 0.1169 g/100 g before fertilization to 0.1017 g/100 g in the early stage of embryonic development. The content then increased in the middle stage and significantly decreased in the later stage compared with the middle stage of embryonic development. The content of erucic acid in plasma first increased and then decreased, reached the highest level in the middle stage, which was significantly higher than that before fertilization, but its content in the plasma showed little change throughout embryonic development (0.0678 g/100 g in the early stage, 0.0785 g/100 g in the middle stage and 0.0755 g/100 g in the later stage).
In the different developmental stages, the types and composition of fatty acids were the same whereas their contents were different. In general, the fatty acid contents in the plasma were high in the middle of embryonic development and low in the later stage (Figure 3). The fatty acids with high contents were C22.6N3 (DHA) >C16.0 (palmitic acid) > C20.5N3 (EPA) >C18.1N9C (oleic acid) > C22.1N9 (erucic acid). There were 15 kinds of fatty acids that all showed similar patterns; the exceptions were oleic acid, C20.3N6, neuritic acid, behenic acid and arachidonic acid. Although their content ranges were different, they all showed a trend of first increasing and then decreasing with continued embryonic development. C18.1N9C (oleic acid), C20.3N6 (cis-8,11,14-eicosatrienoic acid), C24.1 (neuritic acid) and C22.0 (behenic acid) decreased first, then increased and then decreased again; the content of C20.0 (arachidonic acid) increased first and decreased from the early stage of embryonic development.
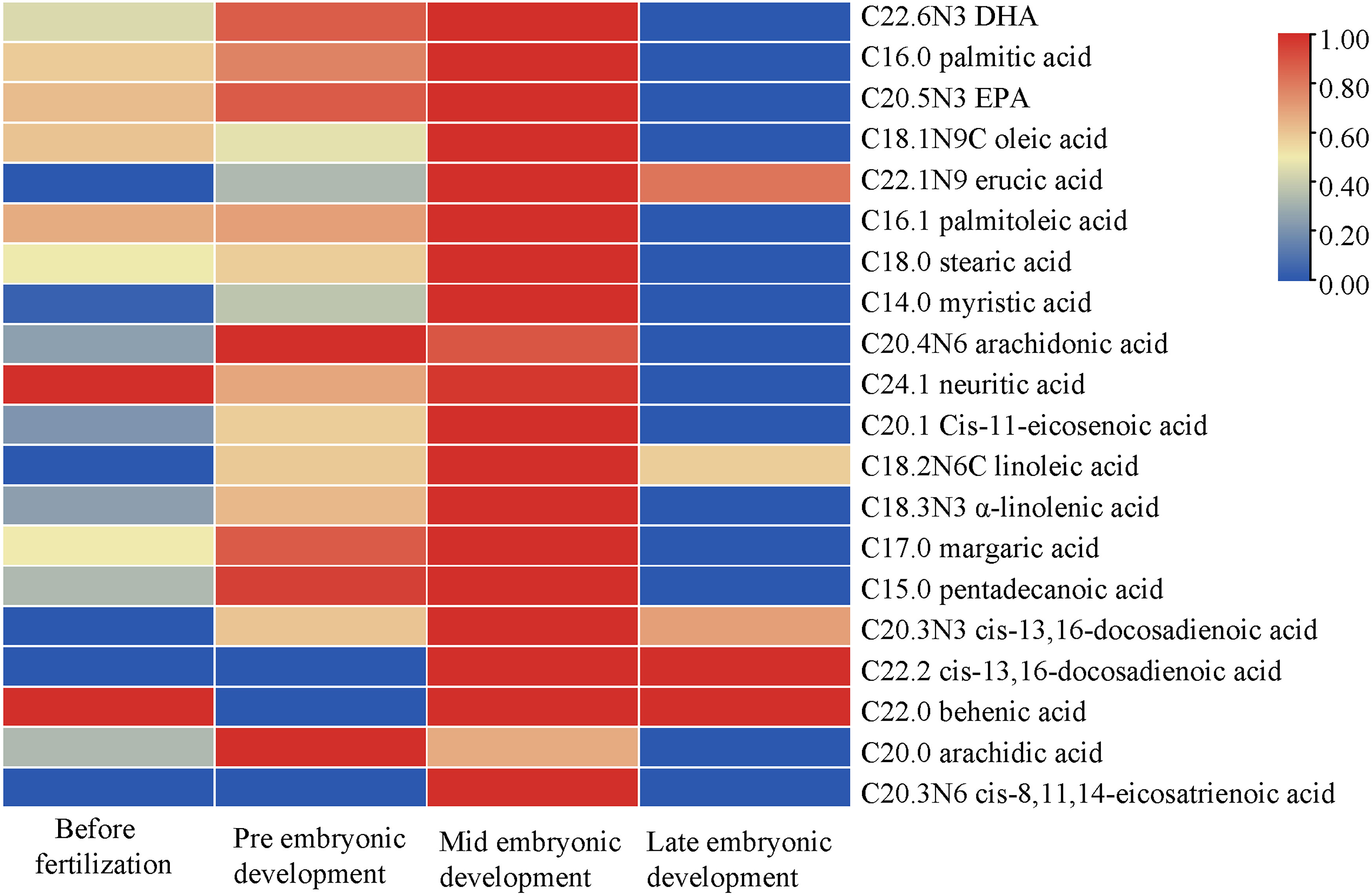
Figure 3 Changes in fatty acid contents during different developmental stages. The abscissa represents the time sequence of development, respectively before fertilization, pre embryonic development, mid embryonic development and late embryonic development and the ordinate represents the changes in plasma composition of fatty acids at different development stages.
The abscissa represents the time sequence of development, respectively before fertilization, pre embryonic development, mid embryonic development and late embryonic development and the ordinate represents the changes in plasma composition of fatty acids at different development stages
Changes in the glucose and fructose contents in the plasma of S. schlegelii females
During embryonic development, the content of glucose in the mother’s plasma was different but this difference was not significant. Overall, the glucose content first increased and then decreased. The content before fertilization was 0.04 g/100 g, the content during early embryonic development was 0.08 g/100 g, the content during middle embryonic development was 0.10 g/100 g, and the content during late embryonic development was 0.07 g/100 g. Fructose was not detected in the plasma before fertilization or in the early and middle stages of embryonic development, and only a small amount (0.004992 g/100 g) was present in the plasma at the later stage of embryonic development (Table 5).

Table 5 Plasma fructose and glucose content of three samples of S. schlegelii in different developmental stages (g/100 g).
Leucine stable isotope tracer
For this experiment, the gas chromatography method was optimized. Under the optimized chromatographic conditions, the peak from naturally abundant leucine was separated from the baseline and had a good shape. The total ion flow diagram (TIC) is shown in Figure 4. The TIC was further analyzed to obtain the EI mass spectrum of leucine. The EI mass spectrum of the leucine natural abundance standard is shown in Figure 4. The structures and molecular weights of the amino acids changed after derivatization with MTBSTFA. The hydrogen atoms at the amino and carboxy termini reacted with the silylation reagent, and the relative molecular weight changed from 131 to 359 (as shown in Figure 5).
In the EI spectrum of the above derivatives, the molecular ion peak is almost completely broken into fragment ions. To calculate 15N isotope abundance, the fragment ions with N atoms need to be selected, and to reduce the error, the fragment ions should preferably be the base peak or have higher peak intensities. The molecular ion peak of the leucine derivative is completely broken into fragment ions, and the structure of the fragment at m/z 200 is shown in the box in Figure 6, in which the N atom is the labelling site (represented as * in the figure), so this fragment ion can be used for the isotope abundance calculation.
The characteristic ion peak cluster from EI mass spectrometry was analysed for different tissues during the different development periods (as shown in Figure 7), and the isotopic abundance values were calculated. The results showed that 15N-labelled leucine was detected in the serum and peripheral tissues of oocytes in the early stage of fertilization, and the average isotopic abundances were 86.90% and 4.73%, respectively, but 15N-labelled leucine was not detected in oocytes (Table 6). Additionally, the nutrients in the blood of the female fish could be transported to the ovaries and the peripheral tissues of the egg cell through all connective tissue, such as blood vessels, to reserve and provide energy materials for the development of the ovaries, egg cell and embryo in real time.
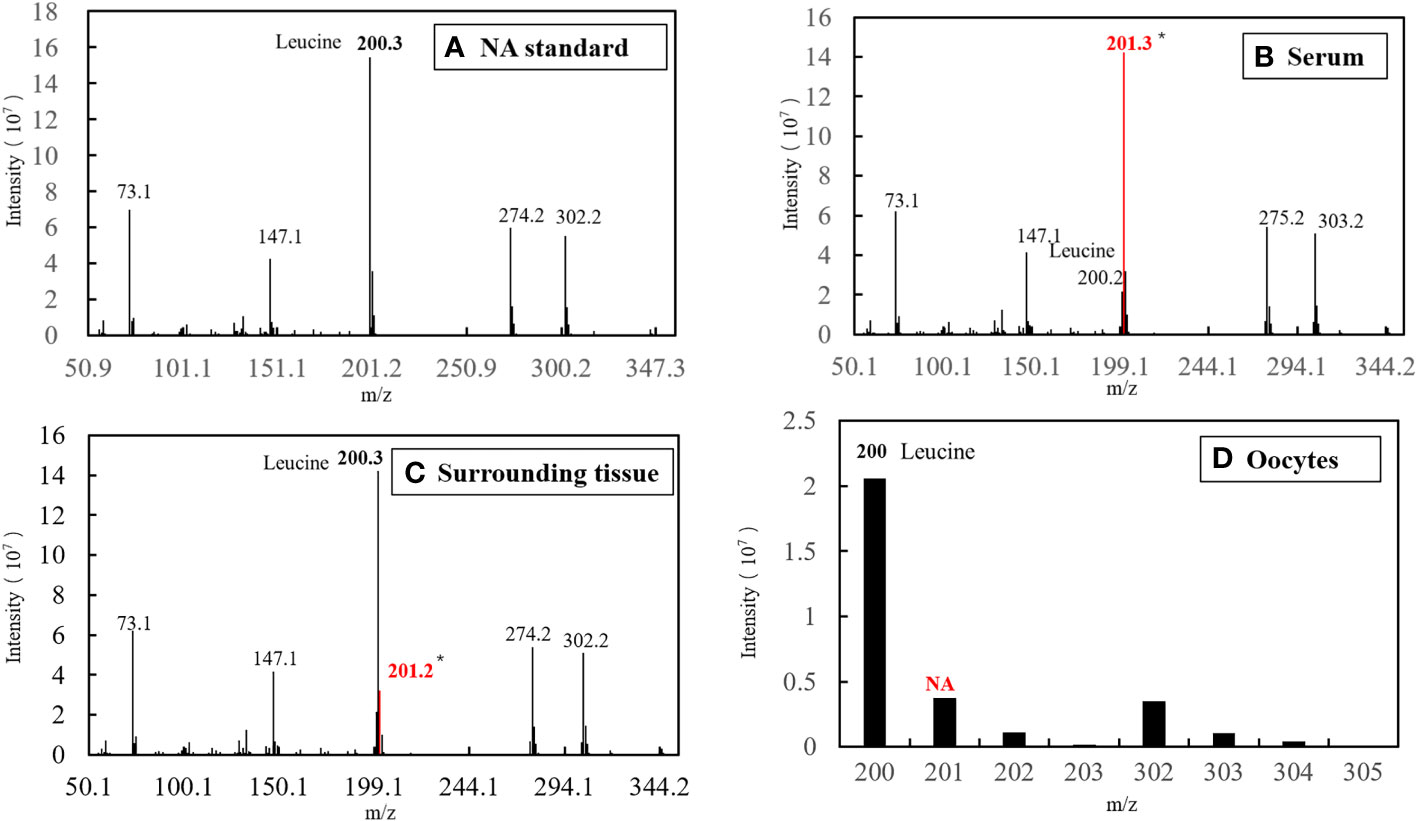
Figure 7 EI diagram of different samples before fertilization. (A) Standard of Natural Abundance (NA). (B) Sample of serum. (C) Sample of surrounding tissue. (D) Sample of oocytes.
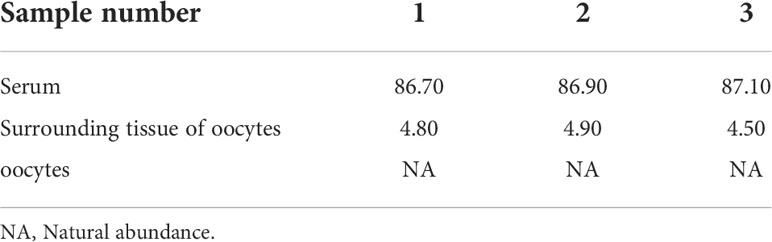
Table 6 Isotopic abundance in the different tissues of S. schlegelii before fertilization (unit: 15N atom%).
The serum samples from S. schlegelii in the gastrula stage showed that the average isotopic abundance of 15N-labelled leucine was 70.60% whereas its abundance in the peripheral tissues of the embryos was 3.43%. 15N-labelled leucine was not detected in embryos (Table 7 and Figure 8). 15N-labelled leucine was also not detected in the embryos of S. schlegelii during organogenesis (Figure 9). Although 15N leucine was not detected in 15N-labelled eggs, gastrula embryos or embryos during organogenesis, a high abundance of 14N leucine (conventional 200/200.1/200.3) was detected in the eggs and embryos of the control group and experimental group, indicating that leucine, as an essential amino acid of aquatic animals, plays an important role in gamete and embryo development.
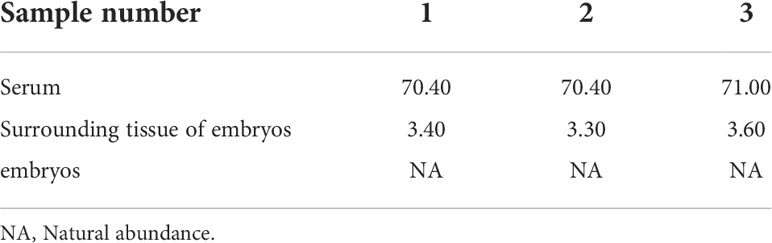
Table 7 Isotopic abundance in the different tissues of S. schlegelii at the gastrulation stage (unit: 15N atom%).
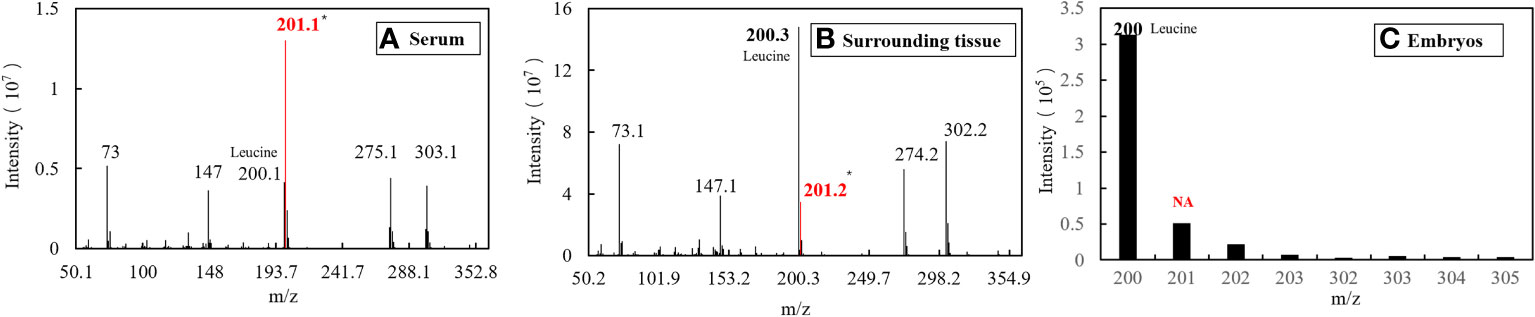
Figure 8 EI diagram of different samples in the gastrula phase. (A) Sample of serum. (B) Sample of surrounding tissue. (C) Sample of embryos.
The results of the comprehensive isotopic labelling study (Table 8) showed that the mother could transport the small molecule nutrient leucine to the ovaries through the blood before and after embryonic development, but it is not clear whether the embryo uses the nutrients transported by the mother and in what way. No labelled isotopes were detected in somite embryos.
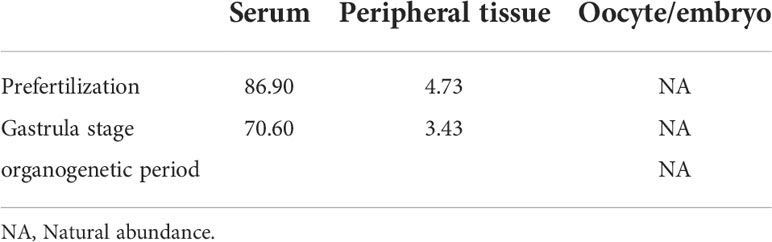
Table 8 Isotopic abundance in the different tissues of S. schlegelii at different development stages (unit: 15N atom%).
Discussion
In this study, analysis of the plasma components of S. schlegelii showed that the contents of amino acids in maternal plasma reached 3.44-4.58 g/100 g, which was approximately 3-5 times the contents of fatty acids and 44-155 times that of sugar during the different developmental stages. Amino acids are an important energy source during embryonic development. These results are consistent with the research results of eggs and early embryos of other bony fish; that is, the demand for amino acids in the early development is higher than that for fat and sugar (Rønnestad and Fyhn, 1993; Rønnestad et al., 1999; Rønnestad et al., 2003). Amino acids are small molecules that make up proteins. The synthesis of proteins can promote the rapid development of embryos. Other studies have shown that offspring nutrition mainly depends on the nutrition of the parents (Araújo et al., 2012; Callan et al., 2012; Sahar et al., 2017). Based on the above two factors, we can try to strengthen the nutrition of the mother to improve the contents of nutrients in the blood to provide sufficient amounts of these compounds needed for the embryonic development of black rockfish. Although we have not seen any research reports on black rockfish, from the overall perspective of aquaculture, optimizing the feed diet via supplementing protein as the main component has never been interrupted (Debnath and Saikia, 2021). For example, in Channa striata (Mohanty and Samantaray, 1996), Ctenopharyngodon idella (Dabrowski, 1977), Tifapia nilotica (Santiago et al., 1982) and Dicentrarchus labrax (Péres et al., 1996), fish meal and hydrolysed casein were used as the main nutrients to increase the protein content in the larvae feed to improve their specific growth rate and protein deposition rate.
If we want to improve nutrient utilization, choosing which period to strengthen the nutrient contents is another issue that needs to be considered. The amounts and types of nutrients used during different stages of development are different. Analysis of the transcriptome data from the beginning of hatching to the fourth day of development determined that the first day involved the main life activities of protein synthesis and protein and fat digestion and absorption. On the second day, after the yolk sac was absorbed, fat began to be stored for energy. On the fourth day, protein synthesis was important (Danitzia et al., 2021). There were 38 kinds of small molecules examined in this study. Except for fructose, which was only found in the later stage of embryonic development and had a very low content, 75.68% of the small molecules showed a trend of increasing contents in maternal plasma during the early stage of embryonic development and then decreasing during the later stage. However, whether the demand for nutrients in the process of embryonic development changes, resulting in the contents of nutrients in the blood first increasing and then decreasing, still needs to be further explored. Docosahexaenoic acid (DHA) and eicosapentaenoic acid (EPA) were the first and third most abundant fatty acids during the embryonic development of S. schlegelii, respectively. These two unsaturated fatty acids are also essential fatty acids for fish egg and embryo development (Sargent et al., 1999). In factory culture, adding DHA to the feed improved the survival rate of the larvae and accelerated the growth of yellowtail flounder (Limanda ferruginea) (Copeman et al., 2002). The high contents of DHA and EPA in black rockfish also explains why they are favoured as a cultured fish by people. The most well-known important role of DHA is to promote brain development (Lauritzen et al., 2001; Uavy et al., 2005). EPA is a blood vessel scavenger that can promote the health of the circulatory system and also promote development (Qian et al., 2020).
This study determined the composition and contents of the three major nutrients in the blood and also found the trends mentioned above, which are necessary basic materials for further research. In addition, we also conducted stable isotope tracing, hoping to find direct evidence that the mother provides nutrition to the embryo. We selected leucine, which showed a change trend that was consistent those of 13 amino acids, as it had a high content and is an essential amino acid for aquatic animals (Ye et al., 2003). The isotope labelling results showed that 15N-labelled leucine was detected only in blood and embryonic ovarian villi but not in embryos. Through this study, we found that there was a high abundance of leucine in oocytes and embryos, but 15N-labelled leucine was not detected. The results of this study also showed that the free naturally abundant leucine in the maternal blood during the early developmental stage of eggs and embryos (organ formation stage) was not absorbed and utilized by the eggs and embryos. This result showed that early in the development process, the absorption of nutrients by eggs and embryos had a certain degree of autonomy and selectivity. Priority was given to active transportation of certain functional macromolecular nutrients, that is, complex macromolecules, which could meet the needs of the eggs and embryos for a variety of nutrients at the same time. In a study on S. flavidus embryo nutrition (Macfarlane and Bowers, 1995), it was found that the embryo could absorb the macromolecular nutrient phosphatidylcholine but not the small molecule ruthenium red. Reports indicated that the nutrient uptake by embryos is mainly completed in the female’s maternal ovary before delivery, and mainly depend on the yolk but not the maternal supply (Mourente and Vazquez, 1996; Feng, 2015). Maternal nutrition for oocytes and embryos can be a continuous supply. It may also be a phased supply, and will not continue to supply once the material reserve is completed. These results suggested that the uptake of the leucine needed by eggs and embryos might be accomplished before fertilization. Because all the samples used in our experiment have completed yolk accumulation. Therefore, there is no material supply model for the complete development time sequence of S. schlegelii. In addition, the nutrients in maternal blood could be transported to the ovary through all levels of blood vessels. Leucine might be metabolized in the tissues surrounding the embryo to provide energy for development in the form of another substance; as such, 15N-labelled leucine was not detected. To follow up, we should conduct a broader spectrum study in combination with metabonomics. This research is an exploratory work. In the later research, we will take more intensive samples, whether before fertilization or during embryonic development, to make the results more convincing. On the other hand, in addition to essential amino acids, we will also discuss the supply of other nutrients.
Conclusion
We studied the composition and changes of maternal plasma at different developmental stages. The results showed that the plasma of S. schlegelii contained rich nutrients, including 16 hydrolyzed amino acids, 20 fatty acids, glucose and fructose. The content of amino acids in the plasma of the mother was the highest, followed by fatty acids and sugar. The contents of the three nutrients changed in different development stages, showing an upward trend in early embryonic development and a downward trend in late embryonic development. Studies that selected leucine, an essential amino acid rich in maternal blood, as a biomarker showed that amino acids rich in blood and ovarian villi did not enter oocytes and embryos after the fourth stage ovary. Therefore, we propose the hypothesis that the embryo’s absorption of leucine is selective, and will complete the reserve with the accumulation of yolk in the oocyte, and will no longer absorb it after the reserve is completed. And we speculate that oocytes and embryos have certain autonomy and selectivity in absorbing nutrients, and will preferentially transport some complex macromolecular nutrients, while meeting their needs for various nutrients. In the next step of research, we will take more samples of development stages, including the early, middle and late stages of yolk accumulation in oocytes, to make the research results more adequate. In addition, we will select other essential amino acids and nutrients as markers to study, in order to find out the law of nutrient absorption of S. schlegelii, and contribute to improving the survival rate in the process of breeding.
Data availability statement
The original contributions presented in the study are included in the article/Supplementary Material. Further inquiries can be directed to the corresponding authors.
Ethics statement
The animal study was reviewed and approved by the Institutional Animal Care and Use Committee, Institute of Oceanology, Chinese Academy of Sciences. Written informed consent was obtained from the owners for the participation of their animals in this study.
Author contributions
TD was responsible for the experimental design,implementation and writing of the whole paper. YS was responsible for the construction of the whole paper framework and the revision of the paper. QL provided opinions on the implementation of the paper. JL was responsible for the overall planning of the whole paper. TG and GG participated in the collection of samples. All authors contributed to the article and approved the submitted version.
Funding
Supported by a grant from the National Key Research and Development Program (2018YFD0901204), Key Deployment Projects of Center for Ocean Mega-Science, Chinese Academy of Sciences (Frontier Cross category, COMS2020Q05), Key Special Project for Introduced Talents Team of Southern Marine Science and Engineering Guangdong Laboratory (Guangzhou) (GML2019ZD0402), China Agriculture Research System (CARS-47), Major Agricultural Application Technology Innovation Project of Shandong Province (SD2019YY011), STS project (KFZD-SW-106, ZSSD-019, 2017T3017, KFJ-STS-QYZX-020), Qingdao National Laboratory for Marine Science and Technology (2018SDKJ0502-2, 2015ASKJ02).
Acknowledgments
We thank Qingdao Kechuang Quality Testing Co., Ltd. for blood component detection and Shanghai Chemical Research Institute Co., Ltd. for the detection of stable isotope abundance.
Conflict of interest
The authors declare that the research was conducted in the absence of any commercial or financial relationships that could be construed as a potential conflict of interest.
Publisher’s note
All claims expressed in this article are solely those of the authors and do not necessarily represent those of their affiliated organizations, or those of the publisher, the editors and the reviewers. Any product that may be evaluated in this article, or claim that may be made by its manufacturer, is not guaranteed or endorsed by the publisher.
References
Araújo B., Honji R., De Mello P., Moreira R. (2012). The influence of captive breeding on the fatty acid profiles of Salminus hilarii (Characiformes: Characidae) eggs and larvae. Aquaculture 20 (6), 1161–1181. doi: 10.1007/s10499-011-9472-6
Callan C. K., Laidley C. W., Forster I. P., Liu K. M., Kling L. J., Place A. R. (2012). Examination of broodstock diet effects on egg production and egg quality in flame angelfish (Centropyge loriculus). Aquaculture 43(5), 696–705. doi: 10.1111/j.1365-2109.2011.02877.x
Chen D. G., Ye Z. J., Duan Y., Feng D. Y. (1994). Preliminary study on the biology of breeding population and seedling cultivation of sebastes schlegelii. Acta Oceanologica Sin. 16, 94–101.
Copeman L. A., Parrish C. C., Brown J. A., Harel M. (2002). Effects of docosahexaenoic, eicosapentaenoic, and arachidonic acids on the early growth, survival, lipid composition and pigmentation of yellowtail flounder (Limanda ferruginea): a live food enrichment experiment. Aquaculture 210 (1), 285–304. doi: 10.1016/S0044-8486(01)00849-3
Dabrowski K. (1977). Protein requirements of grass carp fry (Ctenopharyngodon idella Val). Aquaculture 12 (1), 63–73. doi: 10.1016/0044-8486(77)90047-3
Danitzia G. T., Grecia V. I., Rafael C. R. (2021). A transcriptome insight during early fish larval development followed by starvation in Seriola rivoliana. Mar. Biotechnol. 23, 749–765. doi: 10.1007/s10126-021-10061-4
Debnath S., Saikia S. K. (2021). Absorption of protein in teleosts: a review. Fish Physiol. Biochem. 47, 313–326. doi: 10.1007/s10695-020-00913-6
Du T. F., Xiao Y. S., Zhao H. X., Zhou L., Liu Q. H., Wang X. Y., et al. (2021). Multiple fetal nutritional patterns before parturition in viviparous fish Sebastes schlegelii (Hilgendorf, 1880). Front. Mar. Sci. 7, 571946. doi: 10.3389/fmars.2020.571946
Feng Q. C. (2015). Embryos culture in vitro and larvae studies on postembryonal development of sebastes schlegelii hilgendorf (Ocean University of China, Qingdao).
Feng D. Y., Zhang M. Z. (1995). Experimental study on artificial seedling raising of black rockfish. China: Trans. Oceanol. Limnol. 4, 47–52.
Hou J., Lei W., Du X. N. (2016). Isotope abundance characterization of 15N labeled amino acids in animal plasma by liquid chromatography-mass spectrometry. Chem. World 57 (08), 475–480.
Kai Y., Soes D. M. (2009). A record of sebastes schlegelii hilgendorf, 1880 from Dutch coastal waters. Aquat. Invasions 4, 417–419. doi: 10.3391/ai.2009.4.2.23
Koya Y. (2008). “Reproductive physiology in viviparous teleost,” in Fish reproduction (Florida: The Chemical Rubber Company Press), 245–275.
Lauritzen L., Hansen H. S., Jorgensen M. H., Michaelsen K. F. (2001). The essentiality of long chain n-3 fatty acids in relation to development and function of the brain and retina. Prog. Lipid Res. 40 (1-2), 1–94. doi: 10.1016/S0163-7827(00)00017-5
Lei J. L., Lu J. W., Xu D. S., et al. (2005). Theory and technology of marine fish culture. 711 (Beijing: China Agricultural Press).
Li T. T., Chen L. M., Liu F. (2017). Indoor industrialized seedling raising technology of Sebastes schlegelii. Aquaculture 38 (11), 38–39.
Liu Q., Zhang X. M., Zhang P. D., Nwafili S. A. (2009). The use of alizarin red s and alizarin complexone for immersion marking Japanese flounder Paralichthys olivaceus (T.). Fish. Res. 98 (1), 67–74. doi: 10.1016/j.fishres.2009.03.014
Li G. S., Zhou L. H., Liang X. F. (2003). Study on the absorption and accumulation of two kinds of amino acids in juvenile mud carp. Reservoir Fish. 6, 60–62.
Lv H. J. (2013). Marker technology and marker release tracking evaluation of sebastes schlegelii and paralichthys olivaceus (Ocean University of China).
Macfarlane R. B., Bowers M. J. (1995). Matrotrophic viviparity in the yellowtail rockfish sebastes flavidus. J. Exp. Biol. 198, 1197–1206. doi: 10.1242/jeb.198.5.1197
Matsubara K. (1943). Studies on the scorpaenoid fishes of japan. anatomy, phylogeny and taxonomy, I, II (Tokyo: Trans Sigenkagaku Kenkyusyo), 486.
Mohanty S. S., Samantaray K. (1996). Effect of varying levels of dietary protein on the growth performance and feed conversion efficiency of snakehead channa striata fry. Aquac. Nutr. 2 (2), 89–94. doi: 10.1111/j.1365-2095.1996.tb00013.x
Mori H., Nakagawa M., Soyano K., Koya Y. (2003). Annual reproductive cycle of black rockfish Sebastes schlegelii in captivity. Fish. Sci. 69 (5), 910–923. doi: 10.1046/j.1444-2906.2003.00707.x
Mourente G., Vazquez R. (1996). Changes in the content of total lipid, lipid classes and their fatty acids of developing eggs and unfed larvae of the Senegal sole, solea senegalensis kaup. Fish Physiol. Biochem. 15 (3), 221–235. doi: 10.1007/BF01875573
Nakabo T. (2010). Fishes of Japan with pictorial keys to the species (second edition) (Kanagawaken, Japan: Tokai University Press).
Péres A., Cahu C. L., Infante J. L. Z., Le Gall M. M., Quazuguel P. (1996). Amylase and trypsin responses to intake of dietary carbohydrate and protein depend on the developmenta stage in sea bass (Dicentrarchus labrax) larvae. Fish Physiol. Biochem. 15 (3), 237–242. doi: 10.1007/BF01875574
Qian C., Hart B., Colombo S. M. (2020). Re-evaluating the dietary requirement of EPA and DHA for Atlantic salmon in freshwater. Aquaculture 518, 734870. doi: 10.1016/j.aquaculture.2019.734870
Rønnestad I., Fyhn H. J. (1993). Metabolic aspects of free amino acids in developing marine fish eggs and larvae. Rev. Fish. Sci. 1 (3), 239–259. doi: 10.1080/10641269309388544
Rønnestad I., Thorsen A., Finn R. N. (1999). Fish larval nutrition: a review of recent advances in the roles of amino acids. Aquaculture 177 (1), 201–216. doi: 10.1016/S0044-8486(99)00082-4
Rønnestad I., Tonheim S. K., Fyhn H. J., Rojas-García C. R., Kamisaka Y., Koven W., et al. (2003). The supply of amino acids during early feeding stages of marine fish larvae: a review of recent findings. Aquaculture 227, 147–164. doi: 10.1016/S0044-8486(03)00500-3
Sahar M., Tremblay R., Grant V., Mari M., Ines Ben K., Céline A. (2017). Differences in nutrient content of eggs and larvae as indicators for improvement of broodstock nutrition in walleye (Sander vitreus) production. Can. J. Zool. 95 (5), 299–310. doi: 10.1139/cjz-2016-0176
Santiago C. B., Banes-Aldaba M., Laron M. A. (1982). Dietary crude protein requirement of tilapia nilotica fry. Kalikasan: J. Philippine Biol. 11 (2-3), 255–265.
Sargent J., McEvoy L., Estevez A., Bell G., Bell M., Henderson J., et al. (1999). Lipid nutrition of marine fish during early development: current status and future directions. Aquaculture 179, 217–229. doi: 10.1016/S0044-8486(99)00191-X
Tian Y., Piao G. H., Zhang Y. H., Liu J. M., Tian Y., Yang X. G. (2011). Comparison of the study about human protein requirement through two stable isotope labelled amino acids. Acta Nutrimenta Sin. 33 (1), 5–9.
Uavy R., Rojas C., Llanos A., Mena P.. (2005). Dietary essential fatty acids in early postnatal life: long-term outcomes. Nestle Nutr. Workshop Ser. Pediatr. Program 55, 101–133. doi: 10.1159/000082597
Xinwei P., Chao F., Zengqiang Y. (2020). Analysis of stomach contents of sebastes schlegelii in tangshan marine ranch. Hebei Fish. 3, 8–12+49.
Yamada J., Kusakari M. (1991). Staging and the time course of embryonic development in kurosoi, sebastes schlegeli. Environ. Biol. Fishes. 30, 103–110. doi: 10.1007/BF02296881
Yamada U., Tokimura M., Horikawa H., Nakabo T. (2007). Fishes and fisheries of the East China and yellow seas (Kanagawa: Tokai University Press. Tokyo Japan).
Yang Y. (2018). Study on the molecular mechanism and pattern of germ cell proliferation and differentiation in Japanese flounder (Paralichthys olivaceus) (Chinese Academy of Sciences: University of Chinese Academy of Sciences (Institute of Oceanography).
Ye Y. T., Wang Y. H., Lin S. H., Luo L. (2003). Transmural fluxes of ten essential amino acids in intestinal gut of Ctenopharyngodon idellus in vitro. Chin. Fish. Sci. 4, 311–317.
Zhao C. Y. (2017). Study on differentiation of gonad and the characterization of mtnrs, kisslkissr and GnRH during breading season of turbot (Scophthalmus maximus) (Chinese Academy of Sciences: University of Chinese Academy of Sciences (Institute of Oceanography).
Keywords: Sebastes schlegelii, maternal nutrition, nutrients, stable isotope, plasma
Citation: Du T, Xiao Y, Liu Q, Guo T, Gao G and Li J (2022) Maternal nutrients and 15N Leucine tracing in Sebastes schlegelii. Front. Mar. Sci. 9:937805. doi: 10.3389/fmars.2022.937805
Received: 13 May 2022; Accepted: 03 October 2022;
Published: 18 October 2022.
Edited by:
Stephen J. Newman, Department of Primary Industries and Regional Development of Western Australia (DPIRD), AustraliaReviewed by:
Morteza Yousefi, Peoples’ Friendship University of Russia, RussiaYongkang Gai, Huazhong University of Science and Technology, China
Copyright © 2022 Du, Xiao, Liu, Guo, Gao and Li. This is an open-access article distributed under the terms of the Creative Commons Attribution License (CC BY). The use, distribution or reproduction in other forums is permitted, provided the original author(s) and the copyright owner(s) are credited and that the original publication in this journal is cited, in accordance with accepted academic practice. No use, distribution or reproduction is permitted which does not comply with these terms.
*Correspondence: Jun Li, junli@qdio.ac.cn; Yongshuang Xiao, dahaishuang1982@163.com
†These authors share first authorship