- 1College of Marine and Environmental Sciences, Tianjin University of Science and Technology, Tianjin, China
- 2Tianjin Key Laboratory of Marine Resources and Chemistry, Tianjin University of Science and Technology, Tianjin, China
- 3Department of Biology and Chemistry, City University of Hong Kong, Kowloon, Hong Kong SAR, China
Anthropogenic nutrient input to coastal waters is one of the most common disturbances within inshore marine benthic communities. Organic enrichment in sediments leads to the reduction or disappearance of sensitive organisms, and influences the quality and quantity of primary producers which serve as food sources for the benthic fauna. Such changes, in turn, affect the energy flow and food-web interactions in benthic communities. To examine how organic enrichment may alter marine benthic trophic relationship, a stable isotope (δ13C and δ15N) analysis of the potential food sources and a range of meiofauna and polychaetes from an organically polluted and a relatively unpolluted site was compared in subtropical waters of Hong Kong. Results indicated that some omnivorous infauna shifted from a mainly carnivorous diet at the unpolluted site to a largely herbivorous diet at the organically polluted site. This dietary shift is likely to be related to the oxygen stress, prey limitation and increased abundance and nutritional quality of primary producers in the eutrophic area, resulting in an increase in utilization of plant materials as the major food source. The present findings suggest that such changes in trophic position induced by organic enrichment can provide further insights into the structure and function of coastal benthic communities under pollution stress.
Introduction
Anthropogenic nutrient inputs to the coastal ecosystems particularly from discharges of untreated domestic sewage have led to widespread organic enrichment in inshore marine environments (Souza et al., 2013; Altieri and Diaz, 2019; Brauko et al., 2020). Such nutrient enrichment has impacted many biological communities, ranging from depletion of native populations, extinction of sensitive species to disappearance of diverse community structure (Nordström and Bonsdorff, 2017; Caswell et al., 2018; Culhane et al., 2019; Drylie et al., 2020). This becomes increasing important to understand how the species, community and ecosystem levels respond to such human-induced changes and whether ecosystem functions and services can be maintained (Riedel et al., 2014; Johansen et al., 2018; Guan et al., 2020).
The increase in nutrients can have both positive and negative effects on marine pelagic and benthic communities. Higher nutrient input can stimulate primary production in the water column, resulting in further deposition of organic matter onto the sediment that benefits both benthic grazers and detritus feeders (Aberson et al., 2016). However, increased sedimentation of organic matter can also lead to reduction or disappearance of some sensitive organisms through siltation, habitat modification and depletion of oxygen due to enhanced metabolic activities of microorganisms (Grall and Chauvaud, 2002; Dorgham, 2014; Hale et al., 2016). Changes in species composition and abundance associated with hypoxia caused by organic enrichment may in turn result in limitation of prey availability and alter consumers’ feeding habits (Fox et al., 2009; Zheng et al., 2020). Under low oxygen supply, there is also a change in predator-prey dynamics, in which the foraging ability of predators is reduced due to slower mobility (Riedel et al., 2014; Briggs et al., 2017). These changes in composition and feeding behaviour of consumers may alter trophic relationships in coastal areas subject to eutrophication.
Stable isotope analyses of carbon and nitrogen are commonly used to reveal trophic interactions of natural populations of consumers in the field (Lepoint et al., 2004; Braeckman et al., 2015; Du et al., 2020). δ13C is absorbed in the animal tissues with little or no change as compared to their food sources, so it can be used to determine the food sources; whereas δ15N is absorbed in the animals with constant trophic shift, so it can be used to determine the trophic level (Fry, 2006). Using stable isotope analysis, the diets of benthic consumers have been shown to follow the changes in primary producers under nutrient enrichment, resulting in different benthic pathways that may explain modifications in community diversity and food web structure (Armitage and Fourqurean, 2009; Mitwally and Fleeger, 2015).
Victoria Harbour in subtropical Hong Kong (Figure 1) is one of the busiest and heavily populated ports in the world. Historically, the harbour has received substantial loadings of pollutants from sewage discharge, leading to severe pollution especially within the harbour centre where water movement is restricted by embayments and vessel anchorage shelters (Nicholson et al., 2011). At present most sewage is treated and discharged via a submarine outfall west of the harbour and the general water quality in the harbour has been improved (Xu et al., 2011). However, the sediments there still contain high levels of organic matter in the central harbour area resulting in lower diversity and different benthic community structure compared with that outside the harbour (Liu et al., 2011; Xu et al., 2014).
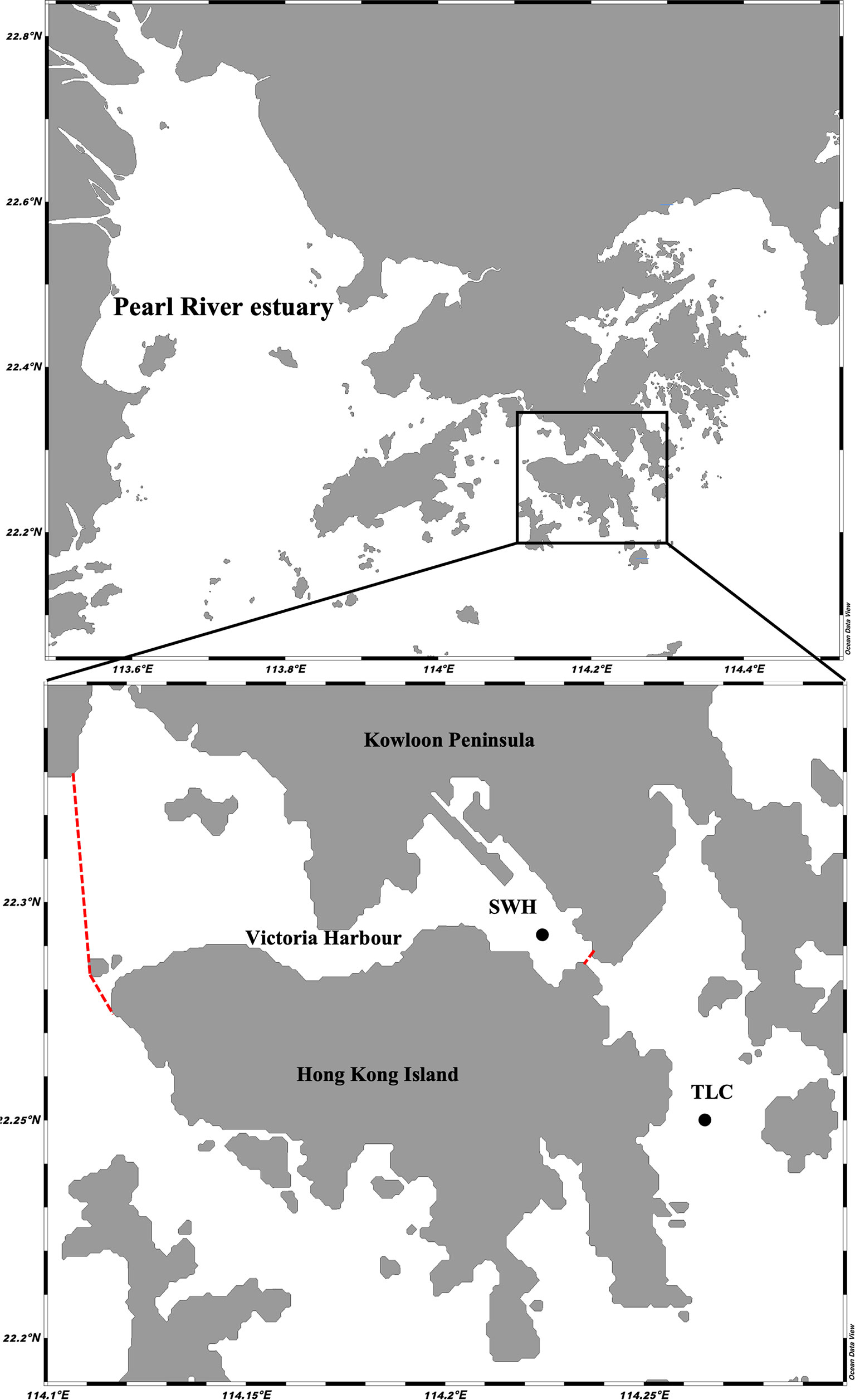
Figure 1 Map showing the relatively unpolluted site TLC and organically enriched site SWH in subtropical Hong Kong, the red dotted lines indicate the boundary of Victoria Harbour.
In the present study, we took advantages of coastal areas with different levels of pollution to examine whether increased organic enrichment can alter the benthic trophic relationship. To determine food web linkages, we measured stable isotopes (δ13C and δ15N) of the particulate organic matter (POM), sediment organic matter (SOM) and primary and secondary consumers at an organically enriched site within the harbour area and a relative unpolluted site outside the harbour. Identifying and understanding the shifts in food source availability and the feeding modes of the animals in inshore areas can help to assess how coastal ecosystems respond under a long history of organic enrichment, sedimentation and other anthropogenic disturbances.
Materials and Methods
Study Sites
The investigation and collection of samples for benthic infaunal community and stable isotope analysis were conducted in August 2010 at two sites around Victoria Harbour at a depth of 15-20 m (Figure 1). Tung Lung Chau (TLC) is located on the east outside the harbour, where anthropogenic activities are minimal and can be regarded as a relatively unpolluted site with low sediment nutrient levels. Sai Wan Ho (SWH) is located inside the harbour, where the sediment is organically enriched. A preliminary study on the taxonomic composition of macrofauna and nematode communities in relation to a number of sediment parameters were conducted at the same locations as in the present study (Xu et al., 2014). Data from the preliminary study showed that the sediment at TLC contained relative low nutrient levels (total organic carbon content: 0.37%, total phosphorus: 374.68 mg/kg, total Kjeldahl nitrogen: 632.97 mg/kg) and higher benthic faunal diversity (Shannon index H' = 2.74 for polychaetes and 3.61 for nematodes) as compared to that at SWH, with higher nutrient levels (total organic carbon content: 1.71%, total phosphorus: 807.07 mg/kg, total Kjeldahl nitrogen: 1926.72 mg/kg) and lower benthic faunal diversity (Shannon index H' = 2.35 for polychaetes and 2.59 for nematodes). The results also showed that the low diversity and different benthic community structure in the inner-harbour area were highly correlated with increased sediment nutrient levels.
Sampling
Triplicate water samples (~10 L per replicate) for particulate organic matter (POM) were collected at about 1 m above the sea bottom at each site using a water sampler. Sediment samples were collected with a 0.1 m2 van Veen grab. A total of twelve grab sediment samples were retrieved at each site. For the first three grab samples, the upper 8 cm of sediment was scraped and stored in plastic bags for subsequent collection of copepod and free-living nematode specimens. For the next three grab samples, sediment was sieved through a 0.5 mm screen and the residues retained were transferred into plastic bags for collection of polychaete specimens. For the following three grab samples, about 400 g surface sediment was scooped by a spatula for later sediment organic matter (SOM) analysis. Benthic microalgae (BMA) were sampled from the sediment in the last three grab samples using the method modified from Wainright et al. (2000) and Doi et al. (2005). In brief, surface sediment was scraped to a depth of 1 cm, and spread into a 1 cm thin layer on a 0.1 m2 plastic flat plate on board. For each plate sample, sediment was covered with a thin layer of silica sand (pre-combusted at 500°C for 2 h), a piece of 63 μm nylon screen, and another thin layer of silica sand. The plates were placed under sunlight for 2 h with continuous moisture from filtered seawater to allow vertical phototactic migration of microalgae onto the nylon screen. Then the screen was peeled off and stored in an ice box.
In the laboratory, meiofaunal nematodes and harpacticoid copepods were extracted from the sediment samples using the centrifugation technique with colloidal Ludox solution (Heip et al., 1985). Moens et al. (2002) showed that the isotopic values of meiofauna obtained under such handling procedures were not affected as compared with similar analysis of live specimens. Sorting of nematodes was restricted to species which were easily recognizable under a stereomicroscope and available in sufficient biomass. In order to obtain enough biomass, for the large nematodes Halichoanolaimus and Sphaerolaimus spp., at least 50 individuals were pooled. For the average-sized nematode Daptonema, Dorylaimopsis, Paradontophora and Terschellingia spp., at least 500 individuals were pooled. Harpacticoid copepods were pooled with at least 500 individuals, without identification to genus or species level. For macrofauna, only polychaetes were sorted and identified to species as far as practicable. The whole body of polychaetes was used for subsequent stable isotope analysis. At least five individuals per species were pooled to obtain enough biomass. All sorted faunal samples were then oven dried at 60°C and 1 mg of the homogenized samples was placed in pre-combusted aluminium cups and stored in screw-cap glass tubes for subsequent stable isotope analysis. Gut contents of all collected meio- and macrofaunal specimens were not separated from the tissue. Hence, the reported stable isotope ratios of these animals referred to the signatures of both assimilated and ingested matter.
In order to obtain enough BMA, the benthic microalgae and silica sand from the three screen replicates were rinsed by distilled water into one beaker, then swirled, and the suspended (non-sand) materials were filtered onto a pre-combusted Whatman GF/F glass fiber filter paper (0.7 μm porosity). For collection of POM, water samples obtained from each site were rinsed through a 63 μm screen to remove larger zooplankton before filtration on pre-combusted Whatman GF/F glass fiber filters (0.7 μm porosity). Sediment samples for SOM analysis were processed following the method of Riera et al. (1996). Each sediment sample was homogenized, freeze-dried and ground using a mortar and pestle. BMA, POM and SOM samples were acidified with 1.2 N HCl to remove inorganic carbon. To prevent any loss of dissolved organics, samples were not rinsed, but were dried overnight at 60°C under a fume extractor to evaporate the acid.
Stable Isotope Analysis
13C and 15N isotopic ratios of food source and consumer samples were determined using a PDZ Europa ANCA-GSL elemental analyzer interfaced to a PDZ Europa 20-20 isotope ratio mass spectrometer (Sercon Ltd., Cheshire, UK) in the Stable Isotope Facility, University of California, Davies, USA. Results of isotopic ratios were expressed in standard δ-unit notation, which is defined as follows:
where X is 13C or 15N, and R is either the 13C:12C ratio for carbon or the 15N:14N ratio for nitrogen. These values were reported relative to the Vienna-PeeDee Belemnite (V-PDB) standard for C and to air N2 for N.
Data Analysis
The use of different food sources by the meio- and macrofauna at each of the two sites was investigated by measurements of the isotopic niche widths. A small sample-size corrected standard ellipse area (SEAc) was calculated by the values of δ13C versus δ15N of all taxa at the two sites (Jackson et al., 2011; Jackson et al., 2012). This metric represents a measure of the total amount of niche occupied in the isotopic space and can be used to investigate the distinct food sources and their utilization (Layman et al., 2007; Layman et al., 2012). It also allows calculating the overlapping area of the standard ellipses between the two study sites, which can be used as a measure of diet similarity between the consumers. In addition, the Laymen’s metrics (Layman et al., 2007) can be estimated to describe these ellipses, as follows: (1) mean distance to centroid (CD), giving additional information about the isotopic niche amplitude and spacing between taxa; and (2) standard deviation of nearest neighbour distance (SDNND), a measure of the evenness of taxa displaying in a bi-plot space. All the metrics proposed by Layman et al. (2007), SEAc and overlapping of standard ellipses were calculated using the SIAR package (Jackson et al., 2011; Jackson et al., 2012) in R 3.10 software (R Development Core Team, 2014).
Results
Stable Isotope of Food Sources and Benthic Fauna
From the stable isotope food web bi-plot (Figure 2A), the δ13C and δ15N values of primary producers at the two sites were close to each other. At the relatively unpolluted TLC, the stable isotope ratios of the food sources ranged from -23.4‰ (POM) to -21.3‰ (SOM) and from 2.9‰ (POM) to 4.8‰ (SOM) for δ13C and δ15N, respectively. At the organically enriched SWH, the stable isotope ratios of the food sources ranged from -23.4‰ (POM) to -22.1‰ (BMA) and from 2.7‰ (POM) to 4.3‰ (SOM) for δ13C and δ15N, respectively. The stable isotope values of SOM at SWH were lighter than that at TLC (Table 1).
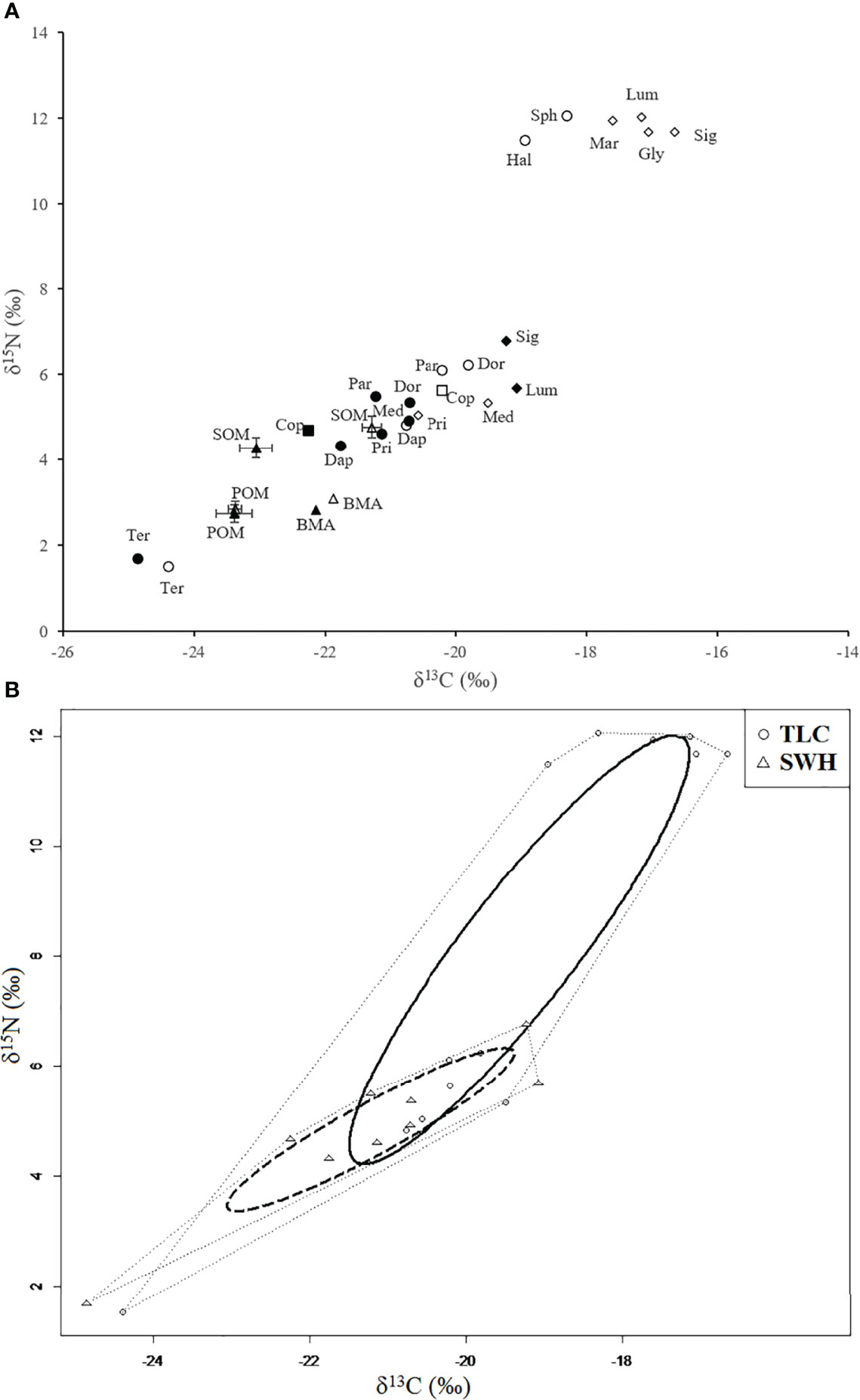
Figure 2 Stable isotope food web bi-plot for (A) all consumers and potential food sources at TLC (open symbols) and SWH (solid symbols). Each symbol represents the δ13C and δ15N values for nematodes (circles), copepods (squares), polychaetes (diamonds) and primary producers (triangles). Cop, Copepods; Dap, Daptonema sp.; Dor, Dorylaimopsis sp.; Gly, Glycera sp.; Hal, Halichoanolaimus sp.; Lum, Lumbrineris sp.; Mar, Marphysa stragulum; Med, Mediomastus sp.; Par, Paradontophora sp.; Pri, Prionospio sp.; Sig, Sigambra hanaokai; Sph, Sphaerolaimus sp.; Ter, Terschellingia sp.; (B) convex hull areas (thin dotted lines) and corrected standard ellipses areas (SEAc: bold lines) in the isotopic space for benthic communities sampled at the relatively unpolluted site TLC (circle) and organically enriched site SWH (triangle).
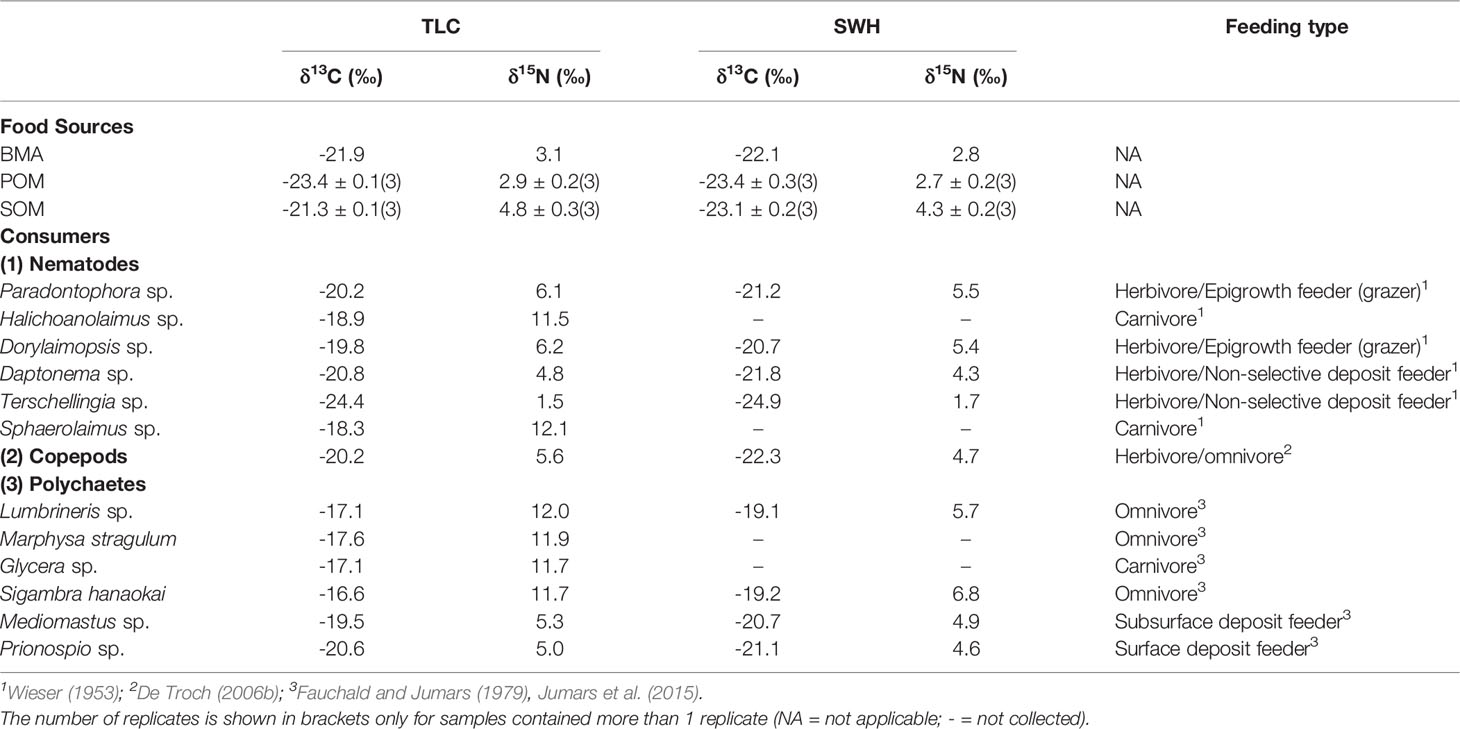
Table 1 Stable isotope composition of food sources and consumers in subtidal sediments at the relatively unpolluted site TLC and organically enriched site SWH in subtropical Hong Kong.
Table 1 also shows the δ13C and δ15N values of the consumers inhabiting in the sediments at TLC and SWH, together with their feeding types according to literature information. The number of species found at TLC was higher than that of species occurring at SWH. For nematodes, there were 6 abundant species (2 carnivores and 4 herbivores) included in the present analysis, with all the 6 species found at TLC and 4 at SWH (4 herbivores). For polychaetes, there were also 6 abundant species (1 carnivore, 3 omnivores and 2 herbivores) at the study sites, with all the 6 species found at TLC and 4 at SWH (2 omnivores and 2 herbivores). At TLC, δ13C ranged from -24.4‰ (nematode Terschellingia sp.) to -16.6‰ (polychaete Sigambra hanaokai) and δ15N ranged from 1.5‰ (nematode Terschellingia sp.) to 12.1‰ (nematode Sphaerolaimus sp.). At SWH, δ13C ranged from -24.9‰ (nematode Terschellingia sp.) to -19.1‰ (polychaete Lumbrineris sp.) and δ15N ranged from 1.7‰ (nematode Terschellingia sp.) to 6.8‰ (polychaete Sigambra hanaokai). The δ15N values of the consumers spanned 10.6‰ at TLC, while 5.1‰ at SWH. Most consumers in a given trophic level at the two sites had similar δ15N values. However, the δ15N values of omnivorous polychaetes Lumbrineris sp. and Sigambra hanaokai were about 5-7‰ lower at SWH compared to that at TLC. These two omnivorous polychaete species tended to become solely herbivorous at the organically enriched SWH, while they remained omnivorous with more prey in their diet and less primary producers at the relatively unpolluted TLC.
Community-Wide Dynamics of Benthic Communities
Layman’s metrics for benthic fauna sampled at TLC showed larger values than that at SWH (Table 2). The CD value at TLC (3.8‰) was more than two times larger than that at SWH (1.5‰). In contrast, SEAc measure was more than three times larger at TLC than that at SWH (SEAc = 11.0‰ and 3.1‰, respectively; Table 2). However, the ellipses overlapping area was lower (15.0%) for benthic fauna present at TLC as compared to that at SWH (53.2%) (Table 2). Comparing the relatively unpolluted TLC to the organically enriched SWH sites, there was a shift of the ellipse towards food sources with lower δ13C values and a shift towards low trophic levels at SWH (Figure 2B).
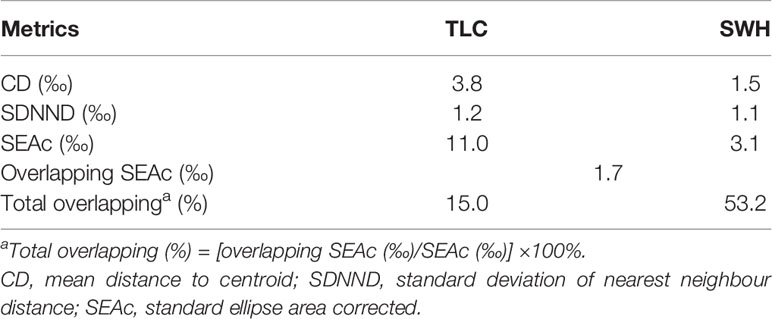
Table 2 Summary of niche community metrics of benthic communities sampled at the relatively unpolluted site TLC and organically enriched site SWH in subtidal sediments of subtropical Hong Kong.
Discussion
Food Sources for Benthic Consumers
Previous studies of benthic communities in subtidal sediment have emphasized the importance of the phytoplankton pathway as an energy source for the benthic food web (Thimdee et al., 2004; Jeffreys et al., 2013; Careddu et al., 2015). In the present study, POM was collected as an indication of phytoplankton content and detritus material. The composition of SOM is usually highly heterogeneous, which includes both in situ primary producers, i.e., microphytobenthos, microorganisms, sedimentation of phytoplankton, nutrients from human activities, and detritus from other origins. Increases in nutrient loading in coastal waters from anthropogenic activities can influence the quality and quantity of the primary producers (e.g. phytoplankton), and together with less prey availability due to hypoxic condition resulting from organic enrichment can further affect consumer population structure and diets (Tewfik et al., 2005; Fox et al., 2009; van der Lee et al., 2021).
Taking an average trophic level increase in δ15N of about 3‰ (Post, 2002), the within-site range of δ15N of consumers (7.3‰ at TLC and 2.5‰ at SWH, excluding the nematode Terschellingia sp.) spanned two trophic steps at the relatively unpolluted site TLC and one step at the organically enriched site SWH. Although feeding behaviour of species from the same trophic level may be different, most consumers in a given trophic level at these two sites had similar δ15N values.
In the present study, organisms that are regarded as herbivores from literature had relatively lower δ15N values than that of omnivores and carnivores. The nematode Daptonema sp. is a non-selective deposit feeder (Wieser, 1953), ingesting suitably-sized food particulates like microalgae (Moens et al., 2014; Xu et al., 2018). The stable isotope values of Daptonema sp. obtained from the present study also reflected BMA as its main diet. The nematode Dorylaimopsis and Paradontophora spp. are considered as epigrowth feeders or grazers based on their buccal morphology (Wieser, 1953). This was also confirmed from the isotopic values of these nematodes in this study, with BMA and other detritus in sediment as their major diet. In a microcosm experiment, Moens et al. (2014) found that epigrowth feeders can either ingest whole diatoms or use their teeth to puncture and suck out the cell contents. With similar isotope values, the harpacticoid copepods collected in the present study also mainly feed on BMA. Our results thus coincided with data from other studies that BMA was an important food source to many harpacticoid copepods (De Troch et al., 2006a; Cnudde et al., 2015) and epigrowth feeding nematodes (Moens and Vincx, 1997). The polychaete Prionospio sp. is considered a typical surface deposit feeder based on the study of its biology (Jumars et al., 2015), and its feeding mode was confirmed from the stable isotope values in the present study. The polychaete Mediomastus sp. is a small to medium-sized worm living in permanent sandy tubes in fine sand and mud, where it exploits subsurface diatoms and detritus as food sources (Hansen, 1993). Its stable isotope values obtained in the present study also revealed that BMA and other detritus in sediments are the major food sources.
The strongly depleted δ13C value of the nematode Terschellingia sp. suggested a different trophic pathway involving utilization of a carbon source that might not be included in the present study. Several chemoautotrophic processes could yield depleted δ13C values, such as sulphide-oxidizing bacteria, with δ13C values below -30‰ (Robinson and Cavanaugh, 1995). Therefore, our data could imply that Terschellingia sp. relies on such bacteria as a food source for its diets, as reported from a mangrove ecosystem in India (Bouillon et al., 2002), an estuarine intertidal flat in the Netherlands (Moens et al., 2011), and an estuarine Zostera noltii seagrass bed in Portugal (Vafeiadou et al., 2014).
Predators are usually more sensitive to stress due to increased metabolic demand, longer lifespan and late maturity (Mor et al., 2022). All the six carnivorous/omnivorous species (2 carnivorous nematodes, 1 carnivorous polychaete and 3 omnivorous polychaetes) involved in the present study were found at the relatively unpolluted site TLC, while only two omnivorous polychaetes were present at the organically enriched site SWH. At TLC, the δ15N values of omnivores overlapped with that of carnivores, whereas at SWH, the δ15N values of omnivores were considerably similar to that of herbivores. The nematodes Sphaerolaimus and Halichoanolaimus spp. were only found at TLC. Their higher δ15N values indicated a carnivorous role, which is in agreement with the trophic guild classification based on buccal morphology (Wieser, 1953; Moens and Vincx, 1997) and field observations (Chitwood and Timm, 1954). The nematode Sphaerolaimus sp. has also been reported having a predacious feeding mode from a stable isotope study from the Scheldt estuary, the Netherlands (Moens et al., 2005), a mudflat in Marennes-Oléron Bay, French Atlantic coast (Rzeznik-Orignac et al., 2008), and Zostera noltii seagrass beds and adjacent bare sediments in Mira estuary, Portugal (Vafeiadou et al., 2014). The polychaetes Marphysa stragulum, Sigambra hanaokai and Lumbrineris sp. are considered to be omnivores, whereas Glycera sp. is a carnivore (Fauchald and Jumars, 1979; Jumars et al., 2015). At TLC, the δ15N values of these omnivorous polychaetes were similar to those of carnivorous polychaete and nematodes, suggesting that they tend to rely on other animals as their main food source. In contrast, the polychaetes Sigambra hanaokai and Lumbrineris sp. at SWH had depleted δ15N values as compared to that collected at TLC, implying that they may assume mainly a herbivore role. Fox et al. (2009) observed organisms that have been reported to feed omnivorously shifted their diets from mainly a carnivorous diet in an oligotrophic estuary to mainly a herbivorous diet in an eutrophic estuary, where prey were limited and macroalgae were abundant. It thus suggested that under organically enriched condition in which the availability of prey is limited, some omnivores can alter their feeding mode to become herbivores by taking advantage of the increase in primary production so as to survive in such stressed environment (Zheng et al., 2020). A similar case was also noted from the present study.
Community-Wide Dynamics of Benthic Food Web
Larger CD and SDNND values were found in the benthic community sampled at the relatively unpolluted site TLC than those at the organically enriched site SWH, indicating a more complex functional community at TLC than that at SWH. This could be directly related to the presence of more trophic levels from herbivores to carnivores at TLC, as reflected in their wider isotopic niche. The largely narrower isotopic niche as revealed in the benthic community at SWH could be related to the high organic enrichment in the sediment, which is characterized by the presence of smaller organisms, usually deposit feeders (Mucha and Costa, 1999; Aberson et al., 2016). Larger SEAc measure was also found in the benthic community at TLC, further suggesting a more complex functional community there. Furthermore, the overlapping area of the ellipses of niche areas at TLC and SWH indicated the niches of benthic communities at the two sites can be different, owing to the different functional structures in their benthic community.
Apart from causing hypoxia with subsequent decrease in prey availability (Riedel et al., 2014) and predator foraging activity (Sagasti et al., 2001; Stover et al., 2013), nutrient enrichment can also lead to changes in the abundance and nutritional quality of primary producers, providing another explanation for the shifts in trophic position of some benthic omnivores (van der Lee et al., 2021). The evidence of a higher reliance on POM as the major food source at SWH can be indicated from difference in the position of the ellipse areas depicted from TLC and SWH (Figure 2B). Such change in food source reliance may also be reflected from the different δ13C values of SOM from the two sites, probably due to a higher contribution of phytoplankton-derived detritus to the overall resource pool at the organically enriched SWH. These dietary differences may be linked to the increase in phytoplankton production which is frequently occurred in eutrophic coastal waters, especially in harbour centre where water movement is restricted by embayments and breakwaters (Nixon and Buckley, 2002). It is noted that there has been an increase in algal biomass within Victoria Harbour in recent years, likely owing to the nutrients brought by the nearby Pearl River estuary from west of the harbour (Figure 1) and increased water transparency as a result of improvement in water quality (Xu et al., 2010). In contrast, chlorophyll a biomass is low in relatively open eastern waters at TLC which are dominated by nutrient-poor oceanic seawater off the coast (Ho et al., 2008). The bulk of the increased primary production may not be directly utilized as living phytoplankton, but may enter the benthic food web as an allochthonous subsidy of POM falling from the water column, and finally settle on the sediment and support the benthic community there (Bouillon et al., 2002; Tewfik et al., 2005). Moreover, the tidal-induced vertical mixing can breakdown the stratification in the water column and transport phytoplankton below the photic zone, as indicated by the fairly high chlorophyll a concentration at the bottom water of Victoria Harbour in summer (Yin and Harrison, 2007; Xu et al., 2010). Such process could increase the sinking rate of microalgae from the water column to the sediment. In addition, the dietary shift of benthic omnivores is likely to be related to the increased nutritional quality of primary producers in more eutrophic waters caused by urban expansion, which allows for an enhanced consumption of plant material (van der Lee et al., 2021). With the shift of the feeding preference of omnivores in organically enriched sediment, the energy flow through the trophic web thus changed by transferring energy from primary production directly to omnivores instead of the functional and trophic roles of herbivores under unpolluted conditions (Fox et al., 2009). Our present findings revealed that the ability of some benthic invertebrate omnivores to change their trophic position in response to organic enrichment, which results in the limited availability of prey and variation in the abundance and nutritional quality of primary producers, may have important implications for our understanding of changes to the structure and function of benthic communities under such pollution stress. Additionally, the traditional feeding type classification based on buccal morphology should be combined with empirical information such as stable isotope signatures in order to decipher the food web structure in areas suffering from prolonged organic enrichment.
Data Availability Statement
The original contributions presented in the study are included in the article/supplementary material. Further inquiries can be directed to the corresponding author.
Author Contributions
PS designed the experiment and revised the manuscript. WX conducted the experiment and wrote the manuscript. JS gave constructive comments and revised the manuscript. All the authors approved the final manuscript.
Funding
This work was supported by the Natural Science Foundation of China (No. 41706184), the Natural Science Foundation of Tianjin (18JCQNJC79000), and the General Research Fund (No. CityU 161009) from the Research Grants Council of the HKSAR Government.
Conflict of Interest
The authors declare that the research was conducted in the absence of any commercial or financial relationships that could be construed as a potential conflict of interest.
Publisher’s Note
All claims expressed in this article are solely those of the authors and do not necessarily represent those of their affiliated organizations, or those of the publisher, the editors and the reviewers. Any product that may be evaluated in this article, or claim that may be made by its manufacturer, is not guaranteed or endorsed by the publisher.
Acknowledgments
Thanks for Ankang Teng for his technical assistance in the field and laboratory.
References
Aberson M. J. R., Bolam S. G., Hughes R. G. (2016). The Effect of Sewage Pollution on the Feeding Behaviour and Diet of Hediste (Nereis Diversicolor (O.F. Müller 1776)) in Three Estuaries in South-East England, With Implications for Saltmarsh Erosion. Mar. Pollut. Bull. 105 (1), 150–160. doi: 10.1016/j.marpolbul.2016.02.033
Altieri A. H., Diaz R. J. (2019). “Chapter 24 Dead Zones: Oxygen Depletion in Coastal Ecosystems,” in World Seas: An Environmental Evaluation, 2nd ed. Ed. Sheppard C. (Massachusetts, MA: Academic Press), 453–473. doi: 10.1016/B978-0-12-805052-1.00021-8
Armitage A. R., Fourqurean J. W. (2009). Stable Isotopes Reveal Complex Changes in Trophic Relationships Following Nutrient Addition in a Coastal Marine Ecosystem. Estuar. Coast. 32 (6), 1152–1164. doi: 10.1007/s12237-009-9219-z
Bouillon S., Koedam N., Raman A., Dehairs F. (2002). Primary Producers Sustaining Macro-Invertebrate Communities in Intertidal Mangrove Forests. Oecologia 130 (3), 441–448. doi: 10.1007/s004420100814
Braeckman U., Provoost P., Sabbe K., Soetaert K., Middelburg J. J., Vincx M., et al. (2015). Temporal Dynamics in a Shallow Coastal Benthic Food Web: Insights From Fatty Acid Biomarkers and Their Stable Isotopes. Mar. Environ. Res. 108, 55–68. doi: 10.1016/j.marenvres.2015.04.010
Brauko K. M., Cerveira A. F., Freitas R. F., Hadlich H. L., Beras T., Fonseca A. L., et al. (2020). Comparing Structural and Functional Approaches to Distinguish Benthic Communities From Nutrient and non-Eutrophic Estuaries. Mar. Pollut. Bull. 157, 111290. doi: 10.1016/j.marpolbul.2020.111290
Briggs K. B., Craig J. K., Shivarudrappa S., Richards T. M. (2017). Macrobenthos and Megabenthos Responses to Long-Term, Large-Scale Hypoxia on the Louisiana Continental Shelf. Mar. Environ. Res. 123, 38–52. doi: 10.1016/j.marenvres.2016.11.008
Careddu G., Costantini M. L., Calizza E., Carlino P., Bentivoglio F., Orlandi L., et al. (2015). Effects of Terrestrial Input on Macrobenthic Food Webs of Coastal Sea are Detected by Stable Isotope Analysis in Gaeta Gulf. Estuar. Coast. Shelf. Sci. 154, 158–168. doi: 10.1016/j.ecss.2015.01.013
Caswell B. A., Paine M., Frid C. L. J. (2018). Seafloor Ecological Functioning Over Two Decades of Organic Enrichment. Mar. Pollut. Bull. 136, 212–229. doi: 10.1016/j.marpolbul.2018.08.041
Chitwood B., Timm R. (1954). “Free-Living Nematodes of the Gulf of Mexico,” in Gulf of Mexico Its Origins, Waters and Marine Life. Fishery Bulletin of the Fish and Wildlife Service, vol. 55 . Ed. Galtsoff P. S. (USA: United States Government Printing Office), 313–323.
Cnudde C., Moens T., Werbrouck E., Lepoint G., Van Gansbeke D., De Troch M. (2015). Trophodynamics of Estuarine Intertidal Harpacticoid Copepods Based on Stable Isotope Composition and Fatty Acid Profiles. Mar. Ecol. Prog. Ser. 524, 225–239. doi: 10.3354/meps11161
Culhane F. E., Briers R. A., Tett P., Fernandes T. F. (2019). Response of a Marine Benthic Invertebrate Community and Biotic Indices to Organic Enrichment From Sewage Disposal. J. Mar. Biol. Assoc. U. K. 99, 1721–1734. doi: 10.1017/S0025315419000857
De Troch M., Chepurnov V., Gheerardyn H., Vanreusel A., Ólafsson E. (2006a). Is Diatom Size Selection by Harpacticoid Copepods Related to Grazer Body Size? J. Exp. Mar. Biol. Ecol. 332 (1), 1–11. doi: 10.1016/j.jembe.2005.10.017
De Troch M., Houthoofd L., Chepurnov V., Vanreusel A. (2006b). Does Sediment Grain Size Affect Diatom Grazing by Harpacticoid Copepods? Mar. Environ. Res. 61, 265–277. doi: 10.1016/j.marenvres.2005.10.004
Doi H., Matsumasa M., Toya T., Satoh N., Mizota C., Maki Y., et al. (2005). Spatial Shifts in Food Sources for Macrozoobenthos in an Estuarine Ecosystem: Carbon and Nitrogen Stable Isotope Analyses. Estuar. Coast. Shelf. Sci. 64 (2), 316–322. doi: 10.1016/j.ecss.2005.02.028
Dorgham M. M. (2014). “Effects of Eutrophication,” in Eutrophication: Causes, Consequences and Control, vol. Vol. 2 . Eds. Ansari A. A., Gill S. S. (Dordrecht: Springer), 29–44. doi: 10.1007/978-94-007-7814-6_3
Drylie T. P., Lohrer A. M., Needham H. R., Pilditch C. A. (2020). Taxonomic and Functional Response of Estuarine Benthic Communities to Experimental Organic Enrichment: Consequences for Ecosystem Function. J. Exp. Mar. Biol. Ecol. 532, 151455. doi: 10.1016/jembe.2020.151455
Du J. G., Makatipu P. C., Tao L. S. R., Pauly D., Cheung W. W. L., Peristiwady T., et al. (2020). Comparing Trophic Levels Estimated From a Tropical Marine Food Web Using an Ecosystem Model and Stable Isotopes. Estuar. Coast. Shelf. Sci. 233, 106518. doi: 10.1016/j.ecss.2019.106518
Fauchald K., Jumars P. A. (1979). The Diet of Worms: A Study of Polychaete Feeding Guilds. Oceanogr. Mar. Biol. Annu. Rev. 17, 193–284.
Fox S. E., Teichberg M., Olsen Y. S., Heffner L., Valiela I. (2009). Restructuring of Benthic Communities in Eutrophic Estuaries: Lower Abundance of Prey Leads to Trophic Shifts From Omnivory to Grazing. Mar. Ecol. Prog. Ser. 380, 43–57. doi: 10.3354/meps07917
Grall J., Chauvaud L. (2002). Marine Eutrophication and Benthos: The Need for New Approaches and Concepts. Glob. Change Biol. 8 (9), 813–830. doi: 10.1046/j.1365-2486.2002.00519.x
Guan Y., Hohn S., Wild C., Merico A. (2020). Vulnerability of Global Coral Reef Habitat Suitability to Ocean Warming, Acidification and Eutrophication. Glob. Change Biol. 26 (10), 5646–5660. doi: 10.1111/gcb.15293
Hale S. S., Cicchetti G., Deacutis C. F. (2016). Eutrophication and Hypoxia Diminish Ecosystem Functions of Benthic Communities in a New England Estuary. Front. Mar. Sci. 3. doi: 10.3389/fmars.2016.00249
Hansen B. (1993). Aspects of Feeding, Growth and Stage Development by Trochophora Larvae of the Boreal Polychaete Mediomastus Fragile (Rasmussen) (Capitellidae). J. Exp. Mar. Biol. Ecol. 166 (2), 273–288. doi: 10.1016/0022-0981(93)90224-C
Heip C., Vincx M., Vranken G. (1985). The Ecology of Marine Nematodes. Oceanogr. Mar. Biol. Annu. Rev. 23, 313–328.
Ho A. Y. T., Xu J., Yin K., Yuan X. C., He L., Jiang Y. L., et al. (2008). Seasonal and Spatial Dynamics of Nutrients and Phytoplankton Biomass in Victoria Harbour and its Vicinity Before and After Sewage Abatement. Mar. Pollut. Bull. 57 (6), 313–324. doi: 10.1016/j.marpolbul.2008.04.035
Jackson M. C., Donohue I., Jackson A. L., Britton J. R., Harper D. M., Grey J. (2012). Population-Level Metrics of Trophic Structure Based on Stable Isotopes and Their Application to Invasion Ecology. PLoS One 7 (2), e31757. doi: 10.1371/journal.pone.0031757
Jackson A. L., Inger R., Parnell A. C., Bearhop S. (2011). Comparing Isotopic Niche Widths Among and Within Communities: SIBER – Stable Isotope Bayesian Ellipses in R. J. Anim. Ecol. 80 (3), 595–602. doi: 10.1111/j.1365-2656.2011.01806.x
Jeffreys R. M., Burke C., Jamieson A. J., Narayanaswamy B. E., Ruhl H. A., Smith K. L. Jr., et al. (2013). Feeding Preferences of Abyssal Macrofauna Inferred From in Situ Pulse Chase Experiments. PLoS One 8 (11), e80510. doi: 10.1371/journal.pone.0080510
Johansen P.-O., Isaksen T. E., Bye-Ingebrigtsen E., Haave M., Dahlgren T. G., Kvalø S. E., et al. (2018). Temporal Changes in Benthic Macrofauna on the West Coast of Norway Resulting From Human Activities. Mar. Pollut. Bull. 128, 483–495. doi: 10.1016/j.marpolbul.2018.01.063
Jumars P. A., Dorgan K. M., Lindsay S. M. (2015). Diet of Worms Emended: An Update of Polychaete Feeding Guilds. Annu. Rev. Mar. Sci. 7 (1), 497–520. doi: 10.1146/annurev-marine-010814-020007
Layman C. A., Araujo M. S., Boucek R., Hammerschlag-Peyer C. M., Harrison E., Jud Z. R., et al. (2012). Applying Stable Isotopes to Examine Food-Web Structure: An Overview of Analytical Tools. Biol. Rev. 87, 545–562. doi: 10.1111/j.1469-185X.2011.00208.x
Layman C. A., Arrington D. A., Montaña C. G., Post D. M. (2007). Can Stable Isotope Ratios Provide for Community-Wide Measures of Trophic Structure? Ecology 88 (1), 42–48. doi: 10.1890/0012-9658(2007)88[42:csirpf]2.0.co;2
Lepoint G., Dauby P., Gobert S. (2004). Applications of C and N Stable Isotopes to Ecological and Environmental Studies in Seagrass Ecosystems. Mar. Pollut. Bull. 49 (11), 887–891. doi: 10.1016/j.marpolbul.2004.07.005
Liu X. S., Xu W. Z., Cheung S. G., Shin P. K. (2011). Marine Meiobenthic and Nematode Community Structure in Victoria Harbour, Hong Kong Upon Recovery From Sewage Pollution. Mar. Pollut. Bull. 63 (5-12), 318–325. doi: 10.1016/j.marpolbul.2011.03.027
Mitwally H. M., Fleeger J. W. (2015). Long-Term Nutrient Enrichment Alters Nematode Trophic Structure and Body Size in a Spartina Alterniflora Salt Marsh. Mar. Ecol. 36 (4), 910–925. doi: 10.1111/maec.12181
Moens T., Bouillon S., Gallucci F. (2005). Dual Stable Isotope Abundances Unravel Trophic Position of Estuarine Nematodes. J. Mar. Biol. Assoc. U. K. 85 (6), 1401–1407. doi: 10.1017/S0025315405012580
Moens T., Luyten C., Middelburg J. J., Herman P. M. J., Vincx M. (2002). Tracing Organic Matter Sources of Estuarine Tidal Flat Nematodes With Stable Carbon Isotopes. Mar. Ecol. Prog. Ser. 234, 127–137. doi: 10.3354/meps234127
Moens T., Moodley L., Steyaert M., Van Colen C., Van Oevelen D., Boschker H. T., et al. (2011). “The Structure and Functional Roles of Tidal Flat Meiobenthos,” in Aspects of Coastal Research in Contribution to LOICZ in the Netherlands and Flanders, (2002–2010). LOICZ Reports and Studies, vol. 38 . Eds. Heip C., Laane R. (Helmholtz-Zentrum Geesthacht, Germany: LOICZ International Project Office), 171–184.
Moens T., Vafeiadou A.-M., De Geyter E., Vanormelingen P., Sabbe K., De Troch M. (2014). Diatom Feeding Across Trophic Guilds in Tidal Flat Nematodes, and the Importance of Diatom Cell Size. J. Sea. Res. 92, 125–133. doi: 10.1016/j.seares.2013.08.007
Moens T., Vincx M. (1997). Observations on the Feeding Ecology of Estuarine Nematodes. J. Mar. Biol. Assoc. U. K. 77 (1), 211–227. doi: 10.1017/S0025315400033889
Mor J. R., Muñoz I., Sabater S., Zamora L., Ruhi A. (2022). Energy Limitation or Sensitive Predators? Trophic and non-Trophic Impacts of Wastewater Pollution on Stream Food Webs. Ecology 103 (2), e03587. doi: 10.1002/ecy.3587
Mucha A. P., Costa M. H. (1999). Macrobenthic Community Structure in Two Portuguese Estuaries: Relationship With Organic Enrichment and Nutrient Gradients. Acta Oecol. 20 (4), 363–376. doi: 10.1016/S1146-609X(99)00130-7
Nicholson S., Hui Y. H., Lam P. K. S. (2011). Pollution in the Coastal Waters of Hong Kong: Case Studies of the Urban Victoria and Tolo Harbours. Water Environ. J. 25 (3), 387–399. doi: 10.1111/j.1747-6593.2010.00234.x
Nixon S. W., Buckley B. A. (2002). “A Strikingly Rich Zone” - Nutrient Enrichment and Secondary Production in Coastal Marine Ecosystems. Estuaries 25 (4), 782–796. doi: 10.1007/BF02804905
Nordström M. C., Bonsdorff E. (2017). Organic Enrichment Simplies Marine Benthic Food Web. Limnol. Oceanogr. 62 (5), 2179–2188. doi: 10.1002/lno.10558
Post D. M. (2002). Using Stable Isotopes to Estimate Trophic Position: Models, Methods, and Assumptions. Ecology 83, 703–718. doi: 10.1890/0012-9658(2002)083[0703:usitet]2.0.co;2
R Development Core Team (2014). R: A Language and Environment for Statistical Computing (Vienna, Austria: R Foundation for Statistical Computing). Available at: http://www.R-project.org/.
Riedel B., Pados T., Pretterebner K., Schiemer L., Steckbauer A., Haselmair A., et al. (2014). Effect of Hypoxia and Anoxia on Invertebrate Behaviour: Ecological Perspectives From Species to Community Level. Biogeosciences 11 (6), 1491–1518. doi: 10.5194/bg-11-1491-2014
Riera P., Richard P., Grémare A., Blanchard G. (1996). Food Source of Intertidal Nematodes in the Bay of Marennes-Oleron (France), as Determined by Dual Stable Isotope Analysis. Mar. Ecol. Prog. Ser. 142, 303–309. doi: 10.3354/meps142303
Robinson J. J., Cavanaugh C. M. (1995). Expression of Form I and Form II Rubisco in Chemoautotrophic Symbioses: Implications for the Interpretation of Stable Carbon Isotope Values. Limnol. Oceanogr. 40 (8), 1496–1502. doi: 10.4319/lo.1995.40.8.1496
Rzeznik Orignac J., Boucher G., Fichet D., Richard P. (2008). Stable Isotope Analysis Position of Intertidal of Food Source and Trophic Nematodes and Copepods. Mar. Ecol. Prog. Ser. 359, 145–150. doi: 10.3354/meps07328
Sagasti A., Schaffner L. C., Duffy J. E. (2001). Effects of Periodic Hypoxia on Mortality, Feeding and Predation in an Estuarine Epifaunal Community. J. Exp. Mar. Biol. Ecol. 258 (2), 257–283. doi: 10.1016/S0022-0981(01)00220-9
Souza F. M., Brauko K. M., Lana P. C., Muniz P., Camargo M. G. (2013). The Effect of Urban Sewage on Benthic Macrofauna: A Multiple Spatial Scale Approach. Mar. Pollut. Bull. 67 (1-2), 234–240. doi: 10.1016/j.marpolbul.2012.10.021
Stover K. K., Burnett K. G., McElroy E. J., Burnett L. E. (2013). Locomotory Fatigue During Moderate and Severe Hypoxia and Hypercapnia in the Atlantic Blue Crab, Callinectes Sapidus. Biol. Bull. 224 (2), 68–78. doi: 10.1086/BBLv224n2p68
Tewfik A., Rasmussen J., McCann K. S. (2005). Anthropogenic Enrichment Alters a Marine Benthic Food Web. Ecology 86 (10), 2726–2736. doi: 10.1890/04-1537
Thimdee W., Deein G., Sangrungruang C., Matsunaga K. (2004). Analysis of Primary Food Sources and Trophic Relationships of Aquatic Animals in a Mangrove-Fringed Estuary, Khung Krabaen Bay (Thailand) Using Dual Stable Isotope Techniques. Wetl. Ecol. Manage. 12 (2), 135–144. doi: 10.1023/b:wetl.0000021674.76171.69
Vafeiadou A. M., Materatski P., Adão H., De Troch M., Moens T. (2014). Resource Utilization and Trophic Position of Nematodes and Harpacticoid Copepods in and Adjacent to Zostera Noltii Beds. Biogeosciences 11 (14), 4001–4014. doi: 10.5194/bg-11-4001-2014
van der Lee G. H., Vonk J. A., Verdonschot R. C., Kraak M. H., Verdonschot P. F., Huisman J. (2021). Eutrophication Induces Shifts in the Trophic Position of Invertebrates in Aquatic Food Webs. Ecology 102 (3), e03275. doi: 10.1002/ecy.3275
Wainright S. C., Weinstein M. P., Able K. W., Currin C. A. (2000). Relative Importance of Benthic Microalgae, Phytoplankton and the Detritus of Smooth Cordgrass Spartina Alterniflora and the Common Reed Phragmites Australis to Brackish-Marsh Food Webs. Mar. Ecol. Prog. Ser. 200, 77–91. doi: 10.3354/meps200077
Wieser W. (1953). Die Beziehungen Zwischen Mundhohlengestalt, Ernahrungsweise Und Vorkommen Beifreilebenden Marinen Nematoden. Ark. Zool. 4, 439–484.
Xu W. Z., Cheung S. G., Shin P. K. S. (2014). Structure and Taxonomic Composition of Free-Living Nematode and Macrofaunal Assemblages in a Eutrophic Subtropical Harbour, Hong Kong. Mar. pollut. Bull. 85 (2), 764–773. doi: 10.1016/j.marpolbul.2014.01.023
Xu W. Z., Cheung S. G., Zhang Z. N., Shin P. K. (2018). Dual Isotope Assessment of Trophic Dynamics of an Intertidal Infaunal Community With Seasonal Shifts in Food Sources. Mar. Biol. 165 (1), 1–13. doi: 10.1007/s00227-017-3278-7
Xu J., Lee J. H., Yin K., Liu H., Harrison P. J. (2011). Environmental Response to Sewage Treatment Strategies: Hong Kong’s Experience in Long Term Water Quality Monitoring. Mar. Pollut. Bull. 62 (11), 2275–2287. doi: 10.1016/j.marpolbul.2011.07.020
Xu J., Yin K., Liu H., Lee J. H. W., Anderson D. M., Ho A. Y. T., et al. (2010). A Comparison of Eutrophication Impacts in Two Harbours in Hong Kong With Different Hydrodynamics. J. Mar. Syst. 83 (3), 276–286. doi: 10.1016/j.jmarsys.2010.04.002
Yin K., Harrison P. J. (2007). Influence of the Pearl River Estuary and Vertical Mixing in Victoria Harbor on Water Quality in Relation to Eutrophication Impacts in Hong Kong Waters. Mar. Pollut. Bull. 54 (6), 646–656. doi: 10.1016/j.marpolbul.2007.03.001
Keywords: organic enrichment, benthic community, nematodes, polychaetes, food web, trophic structure
Citation: Xu W, Shin PKS and Sun J (2022) Organic Enrichment Induces Shifts in the Trophic Position of Infauna in a Subtropical Benthic Food Web, Hong Kong. Front. Mar. Sci. 9:937477. doi: 10.3389/fmars.2022.937477
Received: 06 May 2022; Accepted: 23 May 2022;
Published: 16 June 2022.
Edited by:
Meilin Wu, South China Sea Institute of Oceanology (CAS), ChinaReviewed by:
Yongfen Du, Nanjing University, ChinaHaichao Zhou, Shenzhen University, China
Er Hua, Ocean University of China, China
Copyright © 2022 Xu, Shin and Sun. This is an open-access article distributed under the terms of the Creative Commons Attribution License (CC BY). The use, distribution or reproduction in other forums is permitted, provided the original author(s) and the copyright owner(s) are credited and that the original publication in this journal is cited, in accordance with accepted academic practice. No use, distribution or reproduction is permitted which does not comply with these terms.
*Correspondence: Wenzhe Xu, xuwenzhe@tust.edu.cn