- 1State Key Laboratory of Marine Resource Utilization in South China Sea, Hainan University, Haikou, China
- 2Key Laboratory of Tropical Hydrobiology and Biotechnology of Hainan Province, College of Marine Sciences, Hainan University, Haikou, China
Some symbiotic probiotics have antioxidant activities and could improve the antioxidant capacity of the host. There is still no report on the screening of host-derived antioxidant probiotics for grouper farming. In this study, 369 out of 583 isolates were screened from the gut of hybrid grouper (Epinephelus fuscoguttatus ♀ × Epinephelus lanceolatus ♂) based on their non-hemolytic characteristics. Subsequent preliminary screening with 2,2-Diphenyl-1-Picrylhydrazyl (DPPH) scavenging rate assay resulted in 36 potential antioxidant isolates. After comprehensive evaluation with nine different antioxidant assays (DPPH scavenging rate, 2,2’-azino-bis (3-ethylbezothiazoline)-6-sulfonic acid radical scavenging rate, iron ion reducing ability, reducing activity, O2-· scavenging rate,·OH scavenging rate, ferrous ion chelating rate, hydrogen peroxide tolerance, oxygen-free radical scavenging ability, et al.), 10 isolates with strong antioxidant abilities were screened from 36 potential antioxidant isolates. Then some other probiotic properties, such as simulated gastrointestinal fluid tolerance, adhesion, digestive enzyme activity, and antibacterial activity of the 10 selected isolates were evaluated. All 10 isolates were also identified using the molecular method. Finally, Vibrio rhodolitus GO 91 and Shewanella corallii GO 310, as representatives of the two genera resulting from the identification of the 10 isolates, and with the best overall probiotic properties, were selected from the 10 isolates. Isolates GO 91 and GO 310 were further tested for their safety performances. Antibiotic sensitivity tests showed that GO91 and GO310 were sensitive to many commonly used aquaculture antibiotics. The in vivo challenge test of GO 91 and GO 310 didn’t cause any disease symptoms or death in hybrid grouper. Therefore, isolates GO 91 and GO 310 showed great potential to be used as probiotics in hybrid grouper farming.
Introduction
Oxidative stress is caused by the excessive increase in the production or inactivation of reactive oxygen species (ROS) in the cell (Ashoori and Saedisomeolia, 2014; Yang et al., 2020). Reactive oxygen species is a type of highly active oxygen free radicals which includes superoxide anion radicals, hydroxyl radicals, hydrogen peroxide, nitric oxide, et al. (Raza et al., 2017). Though a small amount of ROS is required for many different cellular activities, when in excess, ROS damage cellular membrane components, induce oxidative stress, and finally results in multiple deleterious events, such as functional loss, apoptosis, or necrosis of cells (Schieber and Chandel, 2014; Feng and Wang, 2020). Many aquaculture conditions could induce oxidative stress in farmed grouper fish: unfavorable water environmental conditions such as temperature, pH, DO, or ammonia nitrogen, for example. Aquaculture operations (catch, transportation, disinfection) also induce oxidative stress in fish, leading to histopathological changes in muscle/liver/gill/intestine, resulting in host-microflora imbalance, and even immune system impairment (Malek et al., 2004; Rueda-Jasso et al., 2004; Bojarski et al., 2020; Ciji and Akhtar, 2021; Limbu et al., 2021).
Natural antioxidant systems in fish body were involved in detoxification of ROS. Within these systems, antioxidants composed of certain enzymes (e.g., superoxide dismutase, Catalase, Thioredoxin reductase, glutathione peroxidase, glutathione S transferase, and glutathione reductase) and non-enzymatic molecules (e.g., vitamins A, C, and E, flavonoids, and glutathione) interact with free radicals produced in cells and stop the chain reaction before the free radicals cause damage to vital molecules (Ashoori and Saedisomeolia, 2014; Mishra et al., 2015). But these natural antioxidant systems are usually not sufficient to protect organisms from oxidative damage (Wang et al., 2017a). Recent studies have shown that some probiotics have significant antioxidant capacity both in vivo and in vitro (Capcarova et al., 2011; Shen et al., 2011; Ejtahed et al., 2012; Persichetti et al., 2014; Wang et al., 2017b). Probiotics such as lactic acid bacteria show a large number of antioxidant activities in the host intestinal tract, promote the production of antioxidant enzymes, and help the host intestinal tract to remove reactive oxygen species, so as to reduce oxidative damage (Feng and Wang, 2020). A number of other probiotics also exhibited significant antioxidant properties by enhancing fish’s tolerance to oxidant stress (Giri et al., 2012; Yan et al., 2016; Gobi et al., 2018; Dawood et al., 2019). In addition to antioxidant activities, probiotics also bring other benefits to the host: promoting the bacterial disease resistance of fish by producing inhibitory compounds, competing for nutrients and chemicals, competing for adhesion sites, inhibiting virulence gene expression or interfering with quorum sensing, providing micronutrients, and helping the digestion of fish intestines (Vine et al., 2006; Pandiyan et al., 2013; Sopková et al., 2017). Therefore, it may be a high-quality substance to alleviate oxidative stress in the fish body.
An ideal probiotic should be able to colonize, establish, and multiply in the host (Lazado et al., 2015). Until now, a large portion of commercial probiotics currently used in aquaculture practices derived from terrestrial animals. They are commonly reported to be ineffective or with minimal effects in aquaculture (Giri et al., 2013; Beck et al., 2015; Doan et al., 2016; Tovar-Ramirez et al., 2010; Daruosh et al., 2018). The reason may be the unfavorable growth conditions of fish or the resistance of fish to foreign probiotics obstructed the successful colonization and multiplication of these terrestrial probiotics. After all, the physiological activities of microorganisms are optimum when in their natural habitat (Kuebutornye et al., 2019). For this reason, seeking probiotics from the host species itself presents a natural advantage over commercial probiotics.
Reports on screening of antioxidant probiotics from fish are rare. In vitro screening of probiotics was economical and practical. The methods for determining the antioxidant activity of probiotics in vitro include 2,2-Diphenyl-1-Picrylhydrazyl (DPPH) assay, 2,2’-azino-bis (3-ethylbezothiazoline)-6-sulfonic acid (ABTS+) assay, ferric ion reducing antioxidant power (FRAP) assay, hydroxyl radical scavenging activity assay, reducing activity assay, chelating ability on ferrous ions assay, hydrogen peroxide resistance assay, and oxygen-radical absorbance capacity (ORAC) assay (Liu et al., 2011; Gao et al., 2013; Li et al., 2013; Mu et al., 2018; Son et al., 2018; Ramalho et al., 2019; Sui et al., 2020). Each determination method has its own advantages and disadvantages, and each has a specific target in the well-defined matrix, therefore comprehensive evaluation by using a variety of methods is more convincing (Mishra et al., 2015).
Hybrid grouper (E. fuscoguttatus♀ × E. lanceolatus♂) is a high-priced mariculture fish species widely reared in China (Song et al., 2018; Wei et al., 2020). To our best knowledge, no research concerning screening antioxidant probiotics from grouper fish has been reported so far. To help alleviate oxidative stress and improve the health status of cultivated hybrid grouper, host-gut derived antioxidant probiotics should be explored. In this study, isolates with strong in vitro antioxidant activity were screened from the gut of hybrid grouper based on comprehensive evaluation of a variety of antioxidant activity indexes. Then these isolates were further screened based on their tolerance to gastrointestinal fluid, adhesion, digestive enzyme activities, and antibacterial activities. Finally, the obtained potential probiotics were identified molecularly and their safety was evaluated through antibiotic susceptibility test and in vivo challenge test.
Methods
Sample Collection and Gut Bacteria Isolation
Ten healthy hybrid grouper (E. fuscoguttatus ♀ × E. lanceolatus ♂) (average weight 650 ± 45 g) individuals were purchased from the local market of Haikou, Hainan, China.All marketable-sized (about 500-800g) hybrid groupers in Hainan market came from Hainan local hybrid grouper farms and farmed hybrid groupers were the aim host of our potential probiotics. Therefore, we used hybrid groupers directly bought from the local market as a source of potential probiotics. Fish were acclimated to laboratory conditions for a week before they were anaesthetized with tricaine methanesulfonate (Sigma, St. Louis, MO, USA) and dissected. For fish acclimation and in vivo challenge test, the fish rearing conditions were maintained as: water temperature 27.0-31.0°C, salinity 30%-32%, pH 7.9 - 8.3, dissolved oxygen 5.5 - 6.0 mg L−1, total ammonia< 0.2 mg L−1.
The fish intestines were taken and grinded with saline solution (0.85% NaCl). The continuously diluted grinding solution was plated on Luria-Bertani (LB) agar at 30°C overnight and plated on De Man, Rogosa and Shape (MRS) (Huankai Microbial, Guangzhou, China) agar containing 1% CaCO3 (MRS-CaCO3) at 37°C for 48 h.
Hemolysis Assay
The selected colonies were plated on Blood Agar Plate (Huankai Microbial, Guangzhou, China) and incubated at 30°C overnight. Non-hemolytic isolates were continuously purified to ensure that these isolates were stable single strains. The obtained isolates were then stored in 50% glycerol at − 80°C for further analysis.
Preliminary Screening of Potential Antioxidant Isolates
In order to quickly screen isolates with antioxidant activity from non-hemolytic isolates, assay of 2,2-Diphenyl-1-Picrylhydrazyl (DPPH) free radical scavenging rate was conducted for preliminary screening, according to Sui et al. (2020) and slightly modified. Briefly, the isolates were resuspended with saline (0.85%), the bacteria solution and DPPH methanol solution (0.2mM) were mixed at 1: 1. The mixture was shaken well and incubated at 30°C for 30 min without light. The supernatant solution was harvested by centrifugation (4000 rpm, 10 min). The absorbance of supernatant solution was measured at 517 nm. The DPPH radical scavenging ability was defined as:
DPPH radical scavenging ability % = [A0− (Ax− A1)]/A0× 100
Ax: The absorbance of sample and DPPH solution
A1: The absorbance of sample and methanol
A0: The absorbance of saline solution and DPPH solution
Further Evaluation of Antioxidant Properties Using Different Assays
Preparation of Isolate Samples
Isolates selected from preliminary screening were cultured overnight at 30°C. Then the isolates were washed with saline solution (0.85%) twice and resuspended in saline solution to a concentration of 2 × 108 CFU/mL (OD600 = 0.4).
Assay of Scavenging Activity Against Superoxide Anion Radical
The assay of scavenging activity against superoxide anion radical was carried out according to Gao et al. (2013) and slightly modified. Briefly, 200 µL of sample was added to 50 µL of Tris-HCl buffer (0.1 M, pH 8.0), mixed evenly, and the absorbance was determined at 320 nm. Then 25 μL pyrogallol solution (3 mM) was added, mixed evenly, and the absorbance was measured again under 320 nm. The scavenging activity against superoxide anion radical is defined as:
Superoxide radical scavenging ability % = [A0− (Ax− A1)]/A0× 100
Ax: The absorbance of sample after reacting with pyrogallol solution
A1: The absorbance of sample without pyrogallol solution
A0: The absorbance of the blank control which reacts with saline solution instead of the sample
Assay of Hydrogen Peroxide Resistance
The assay of resistance to hydrogen peroxide was carried out according to Mu et al. (2018) and slightly modified. Briefly, the overnight cultures of the selected isolates were inoculated into LB Broth containing 0.5 mM hydrogen peroxide solution (30 wt.%) at a concentration of 2% (v/v) and incubated at 30°C for 8 h. The absorbance of bacterial solution was measured at 600 nm. The ability of resistance to hydrogen peroxide is defined as:
Resistance to hydrogen peroxide ability % = (Ax− A0)/(A1− A0)×100
Ax: The absorbance of sample after reacting with 0.5 mM hydrogen peroxide solution
A1: The absorbance of sample without 0.5 mM hydrogen peroxide solution
A0: The absorbance of 0.5 mM hydrogen peroxide solution
Assay of Scavenging Activity Against Hydroxyl Radical
The assay of scavenging activity against superoxide hydroxyl radical was carried out according to Li et al. (2013) and slightly modified. Briefly, the reaction mixture containing 300 μL of FeSO4 solution (2.25 mM), 300 μL of salicylic acid methanol solution (9 mM), 300μL of the isolate sample, and 300μL of H2O2 methanol solution (8.8 mM) was incubated at 37°C for 10 min. The supernatant solution was harvested by centrifugation (4000 rpm, 10 min). The absorbance of supernatant solution was measured at 510 nm. The scavenging activity against superoxide hydroxyl radical is defined as:
Hydroxyl radical scavenging ability % = [A0– (Ax– A1)]/A0× 100
Ax: The absorbance of isolate sample after reacting with solution
A1: The absorbance of methanol added to the isolate sample
A0: The absorbance of the blank control which reacts with saline solution instead of the isolate sample
Assay of Chelating Ability on Ferrous Ions
The assay of chelating ability on ferrous ions was carried out according to Liu et al. (2011) and slightly modified. Briefly, 800 µL of isolate sample was added to 100 µL of FeCl2 solution (0.1 M, pH 8.0), mixed well, and incubated at 37°C for 10 min. Then 25 μL ferrozine solution (5 mM) was added to the mixture and centrifuged at 4000 rpm for 10 min. The absorbance of supernatant solution was measured at 562 nm. The chelating ability on ferrous ions is defined as:
Chelating ability on ferrous ions ability % = (A0– Ax)/A0× 100
Ax: The absorbance of solate sample after reacting with solution
A0: The absorbance of blank control which reacts with saline instead of the solate sample
Assay of Ferric Ion Reducing Antioxidant Power
The assay of FRAP was carried out according to Ramalho et al. (2019) and slightly modified. Briefly, sodium acetate buffer (pH 3.6), FeCl3 solution (20 mM), and 2, 4, 6-Tris (2-pyridyl)-s-triazine hydrochloric acid solution (10 mM) were mixed evenly at the ratio of 10: 1: 1 (V/V/V) to form the FRAP working solution. Then, 40 µL of isolate sample was mixed with 1200 µL of FRAP working solution and incubated in the dark at room temperature for 10 min. The supernatant solution was harvested by centrifugation (4000 rpm, 10 min). The absorbance of supernatant solution was measured at 595 nm. The standard curve was prepared with 50, 100, 200, 400, 800, and 1600 μM FeSO4 solution. The FRAP power of samples were calculated according to the standard curve, and the results were expressed as the equivalent amount of FeSO4 solution (μM). The standard curve was: y = 0.0004x + 0.4322, r2 = 0.996 (x: The concentration of FeSO4 solution; y: The absorbance at 595 nm).
Assay of Reducing Activity
The assay of reducing activity was carried out according to Son et al. (2018) and slightly modified. Briefly, 100 µL isolate sample was mixed with 300 μL sodium phosphate buffer (0.2 mM, pH 6.6) and 300 μL potassium ferricyanide solution (1%, M/V). After incubating at 50°C for 20 min, the mixture was quickly cooled in ice water until it reached room temperature. The reaction was stopped by adding 300 μL of trichloroacetic acid solution (10%, w/v). The supernatant solution was harvested by centrifugation (4000 rpm, 10 min). Then 400 µL supernatant solution was mixed with 400 μL saline solution (0.85%) and 200 μL FeCl3 solution (0.1%, M/V). The absorbance of the mixture was measured at 700 nm. The standard curve was prepared with 0, 100, 200, 300, and 400 μM L-cysteine solution. The reducing activity of samples were calculated according to the standard curve, and the results were expressed as the equivalent amount of L-cysteine solution (μM). The standard curve was: y = 0.0003x + 0.1988, r2 = 0.999 (x: The concentration of L-cysteine solution; y: The absorbance at 700 nm).
Assay of Oxygen-Radical Absorbance Capacity
The assay of resistance to hydrogen peroxide was carried out according to Mu et al. (2018) and slightly modified. Briefly, 25 μL of isolate sample and 150 μL of fluorescein (FL) solution (adjusted to 86.1 nM using 75mM phosphate buffer) were added to 96-well black microplate assay plates and incubated at 37°C for 10 min, then 25 μL of AAPH solution (adjusted to 153 nM using 75 mM phosphate buffer) was quickly added to start the reaction. The fluorescence values of 2 h were measured at 37°C, excitation wavelength of 485nm, emission wavelength of 538 nm, and interval of 2 min. The relative fluorescence intensity is recorded as fn, and the area under the curve (AUG) of each isolate sample is defined as:
The Net AUG values were obtained by subtracting the AUG value of the blank from that of the sample. The standard curve was prepared with Net AUG of 12.5, 25, 50, and 100 μM Trolox solution. The ORAC of samples were calculated according to the standard curve, and the results were expressed as the equivalent amount of Trolox solution (μM). The standard curve is: y=0.3805x-2.5934, r2 = 0.998 (x: The concentration of Trolox solution; y: The values of Net AUG).
Assay of Scavenging of DPPH Free Radical
Same as preliminary screening (DPPH).
Assay of Scavenging of 2,2’-Azino-bis(3-Ethylbezothiazoline)-6-Sulfonic Acid (ABTS+) Free Radical
The assay of scavenging ABTS+ free radical was carried out according to Son et al. (2018) and slightly modified. Briefly, the ABTS+ preparation solution (7 mM) and the K2S2O8 preparation solution (2.45 mM) were mixed at 1: 1 and placed in the dark for 12 h. The mixture was diluted 10-20 times with ethanol until its absorbance was reduced to 0.7 ± 0.02 at 734 nm. This would be the ABTS+ working solution. Then 100 µL of isolate samples were mixed with 1000 µL of ABTS+ working solution and incubated in the dark at room temperature for 6 min. The supernatant solution was harvested by centrifugation (4000 rpm, 10 min). The absorbance of supernatant solution was measured at 734 nm. The ABTS+ free radical scavenging ability was defined as:
ABTS+ radical scavenging ability % = [A0− (Ax− A1)]/A0× 100
Ax: The absorbance of isolate sample with ABTS+ working solution added
A1: The absorbance of isolate sample with methanol added
A0: The absorbance of the blank control which reacts with saline instead of isolate sample
Comprehensive Evaluation of Antioxidant Properties
The comprehensive evaluation of the screened potential probiotics was carried out according to (Mishra et al., 2015; Schaich et al., 2015). The weighting of each antioxidant index is shown in Table 1. Scavenging hydroxyl radical and ORAC accounted for 20% respectively, DPPH free radical and hydrogen peroxide resistance scavenging are 5% respectively, and the other antioxidant indexes accounted for 10% respectively. In order to screen the strains with a strong comprehensive ability of antioxidation, the score of each index was calculated according to the formula:
i: Antioxidant index i
k: Isolate k
N: The total number of isolates, N = 36
Ai: The weighting of antioxidant index i, Ai = 5%, 10%, or 20%
nki: The ranking of isolate k in N at antioxidant index i, the ranking range is 1-36.
Rki: Score of antioxidant index i of isolate k
Tolerance to Simulated Gastrointestinal Juice
The assay of survival in simulated gastrointestinal tract was carried out according to (Mortezaei et al., 2020; Meidong et al., 2021) and slightly modified. Briefly, the culture was carried out in LB broth and incubated overnight at 30°C, followed by centrifugation at 4000rpm for 10 min. The obtained pellet was washed twice with saline solution (0.85%), then resuspended in saline solution (0.85%). The survival of the isolates was evaluated by survival in simulated gastric juice (3mg/mL pepsin adjusted to pH 2.5 and pH 4.0) and simulated intestinal juice (1mg/mL trypsin, 3mg/mL bile salt and adjusted to pH 6.8 and pH 8.0). The surviving isolates were enumerated by dilution plate count on LB agar after exposure to SGJ for 3 h and SIJ for 6 h. The ability of percentage survival is defined as:
Survival % = (log10Ax/log10A0) × 100
Ax: The number of isolates cultured in simulated gastric juice and intestinal juice
A0: The number of isolates cultured in saline solution (0.85%)
Adhesion Ability
Preparation of Isolate Samples
Isolates selected from further evaluation were cultured overnight at 30°C. Then the isolates were washed with saline solution (0.85%) twice and resuspended in saline solution to a concentration of 2 × 108 CFU/mL (OD600 = 0.4).
Bacterial Auto-Aggregation
The assay of bacterial auto-aggregation test was carried out according to Meidong et al. (2021) and slightly modified. Briefly, the isolate samples were placed at room temperature for 5 h, and the absorbance of upper suspension at 600 nm was measured. The auto-aggregation percentage is defined as:
Agg% =[(A0− Ax)/A0]× 100
Ax: The absorbance of sample after 5 h
A0: The absorbance of sample
Bacterial Cell Surface Hydrophobicity
The assay of bacterial cell surface hydrophobicity test was carried out according to (Kavitha et al., 2018; Govindaraj et al., 2021) and slightly modified. Briefly, three organic solvents (xylene, chloroform, and ethyl acetate) were used to detect the surface hydrophobicity of selected isolates. Then 1 mL of isolate samples was evenly mixed with 200 µL solvent and kept at room temperature for 30 min, and the absorbance of upper suspension was measured at 600 nm. The bacterial cell surface hydrophobicity is defined as:
Cell surface hydrophobicity % = [(A0− Ax)/A0]× 100
Ax: The absorbance of sample reacted with solvent after 30min
A0: The absorbance of sample
Digestive Enzyme Activities
The assay of digestive enzyme activities was carried out according to Kavitha et al. (2018) and slightly modified. Briefly, the 10 isolates were washed with saline solution (0.85%) twice and resuspended in saline solution to a concentration of 3 × 108 CFU/mL (OD600 = 0.6). The extracellular protease and amylase of the selected isolates were quantitatively determined by agar diffusion method in skimmed milk agar and starch agar. Lugo’s iodine solution was added to the soluble starch agar to detect amylase activity.
Antibacterial Activity
The assay of antibacterial activity was carried out according to Kavitha et al. (2018) and slightly modified. Vibrio harveyi KR003733.1 (Xu et al., 2017), Vibrio anguillarum (ATCC43309), Photobacterium damselae (ATCC33536), and Edwardsiella tarda PBMM02142 (Sun et al., 2019) were used as the indicator fish pathogens. Briefly, the overnight grown cells of indicator pathogens were collected by centrifugation at 4000 rpm, washed twice, and resuspended in 0.85% saline solution and adjusted to concentration of 2 × 108 CFU/mL (OD600 = 0.4). The 10 isolates were washed with saline solution (0.85%) twice and resuspended in saline solution to a concentration of 3 × 108 CFU/mL (OD600 = 0.6). The antibacterial activity was detected by agar well diffusion assay and the antibacterial activity was evaluated by measuring the diameter of the inhibition zone (mm) around the well.
Identification of Selected Isolates
Bacterial DNA was extracted using the MiniBEST Bacteria Genomic DNA Extraction Kit (TaKaRa, Dalian, China) following the manufacturer’s protocol. The 16S ribosomal RNA gene was amplified using universal primer of Beijing Genomics Institute (BGI) (Beijing, China). The sequences of the primer were: 27F (5´- AGAGTTTGATCCTGGCTCAG -3´), and 1942R (5´- GGTTACCTTGTTACGACTT -3´). The PCR products were sequenced at the Beijing Genomics Institute (BGI) (Beijing, China). The obtained sequences were blasted against GenBank sequences. Multiple sequence alignments were obtained using software package MEGA-X. Phylogenetic tree was constructed using neighbor-joining (NJ) method. The robustness of each topology was checked by 1000 bootstrap replications.
Safety Tests
Antibiotic Susceptibility Test
The susceptibility of selected isolates to 20 antibiotics was determined by the disc diffusion method (Kavitha et al., 2018). The susceptibility was judged according to the instructions given by the antibiotic paper disk manufacturer (HANGWEI™, Hangzhou Microbial Reagent Co., LTD. Zhejiang, China). That instruction was in accordance with the Clinical and Laboratory Standards Institute (CLSI) Guidelines (CLSI M100, Ed28th. 2018). The antibiotics used in the susceptibility test were Cefoperazone, Cephalosporin card, Cefradine, Guacillin, Alozlocillin, Compound sulfamethoxazole, Ofloxacin, Ciprofloxacin, Enoxacin, Levofloxacin, Madimycin, Chloramphenicol, Neomycin, Clindamycin, Minocycline, Spectinomycin, Doxycycline, Tobramycin, Nitrofurantoin, and Polymyxin B. The two isolates were washed with saline solution (0.85%) twice and resuspended in saline solution to a concentration of 3 × 108 CFU/mL (OD600 = 0.6). The antibiotic discs were placed on LB agar plates and incubated at 30°C for 24 h. The inhibition zone (mm) was measured and the antibiotic sensitivity was recorded according to its activity.
In vivo Challenge Test
The in vivo challenge test was carried out according to Gutiérrez Falcón et al. (2021) and slightly modified. Selected isolates were cultivated overnight in LB broth, harvested by centrifugation (4000 rpm, 10 min), washed, and re-suspended in PBS to obtain a cell concentration of 1 × 108 CFU/mL. A total of 90 healthy juvenile hybrid groupers (average weight: 82 ± 4 g) were used for in vivo challenge test. The fish were randomly divided into nine tanks with 10 fish per tank. Each challenge group (two experimental groups and one control group) contained three tanks (three parallels). For experimental groups, one of two selected bacterial suspension was injected intraperitoneally into hybrid grouper at a dose of 100 µL; while for the control group, fish was injected with the same volume of PBS. The numbers of dead fish of all groups were recorded daily for 2 weeks.
An overview of the applied methods is illustrated in the diagram of the applied methodology (Figure 1).
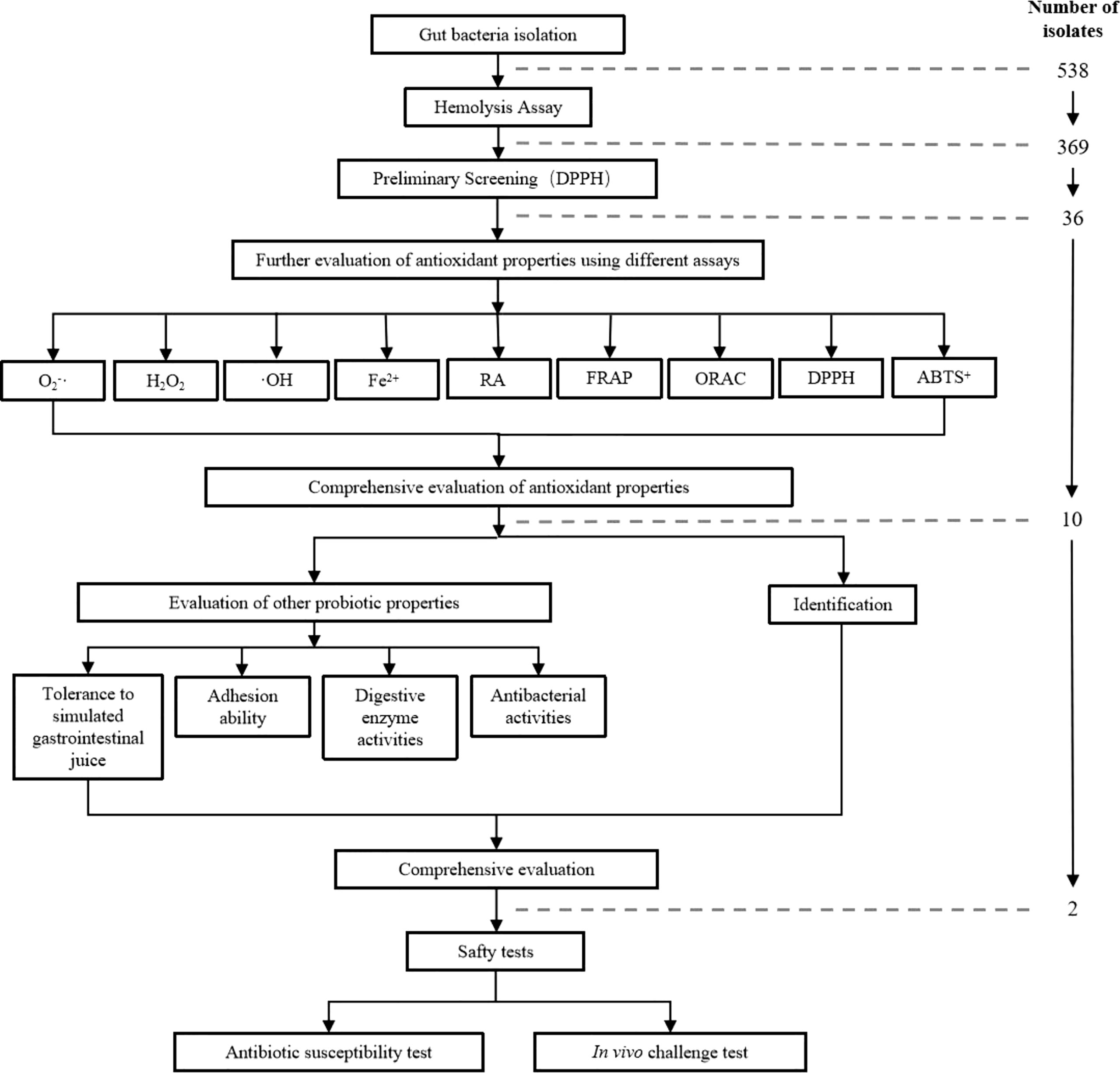
Figure 1 Diagram of the applied methodology. O2-·, Assay of scavenging activity against superoxide anion radicals; H2O2, Assay of hydrogen peroxide resistance; ·OH, Assay of scavenging activity against hydroxyl radical; Fe2+, Assay of chelating ability on ferrous ions; RA, Assay of reducing activity; FRAP, Assay of ferric ion reducing antioxidant power; ORAC, assay of oxygen-radical absorbance capacity; DPPH, Assay of scavenging of DPPH free radical; ABTS+, Assay of scavenging of ABTS+ free radical.
Statistical Analysis
All experiments were performed in triplicate (n=3) and data are presented as mean ± SD. Statistical significance was determined by one-way ANOVA using SPSS 26.0. Significant differences between means were examined using the Duncan multiple range test. P-values of< 0.05 were considered significant.
Result
Hemolytic Activity
A total of 583 colonies were isolated from the intestinal gut of hybrid grouper, and only 369 isolates showed non-hemolytic activity. The 369 non-hemolytic isolates were chosen for further experiments.
Preliminary Screening of Potential Antioxidant Isolates
The results of DPPH free radical scavenging tests are shown in Table S1. Three hundred sixty-nine isolates of potential probiotics have different degrees of DPPH radical scavenging ability, ranging from 17.65% to 67.06%. The highest DPPH radical scavenging rates were observed in GO 295, GO 303, GO 292, GO 26, and GO 231, which were 67.06%, 62.75%, 62.22%, 61.58%, and 61.44%, respectively. The DPPH radical scavenging rate distribution of the 369 isolates are shown in Figure 2.
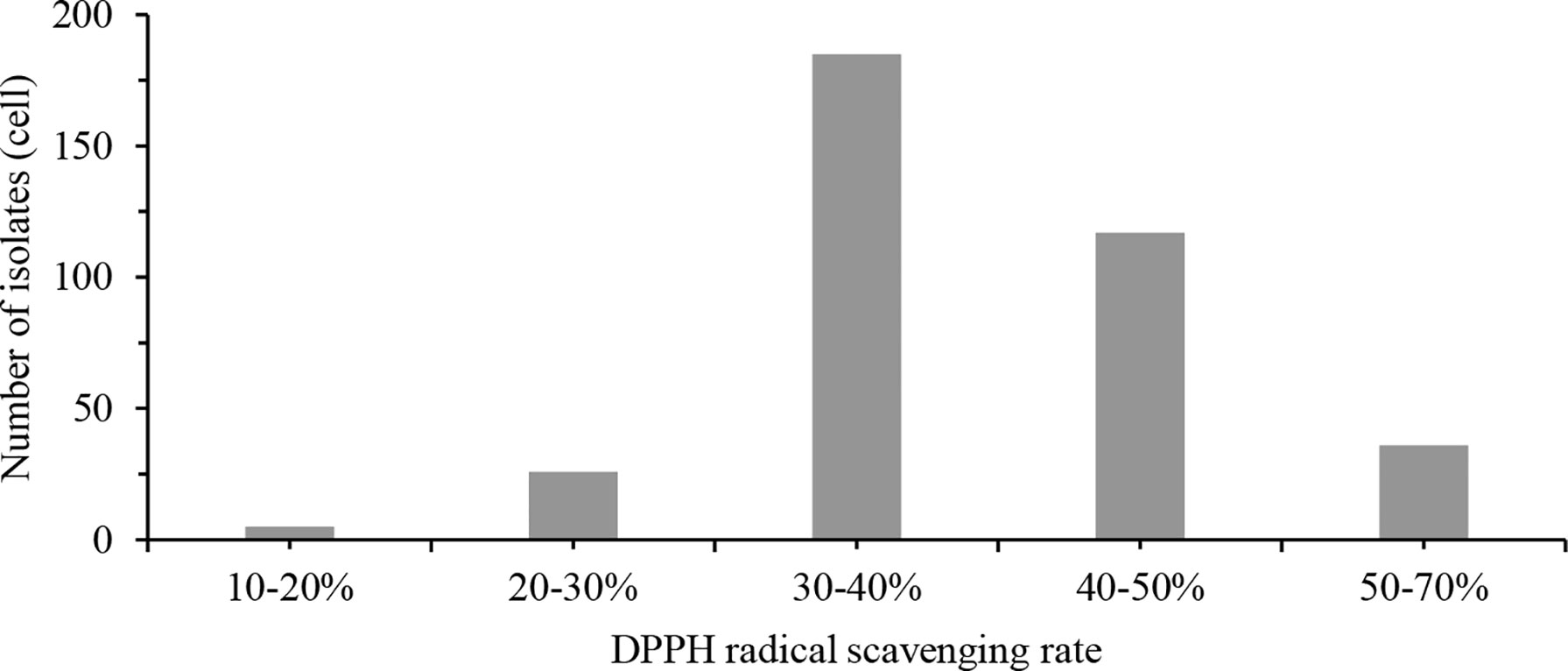
Figure 2 DPPH radical scavenging rate distribution of 369 isolates. DPPH, 2,2-Diphenyl-1-Picrylhydrazyl.
Further Evaluation of Antioxidant Properties Using Different Assays
The results of nine different antioxidant index assays are shown in Figure 3, Table S2. The same isolate showed varying degrees of antioxidant activity in different antioxidant indexes, and the activity of different isolates also varied in the same antioxidant index.
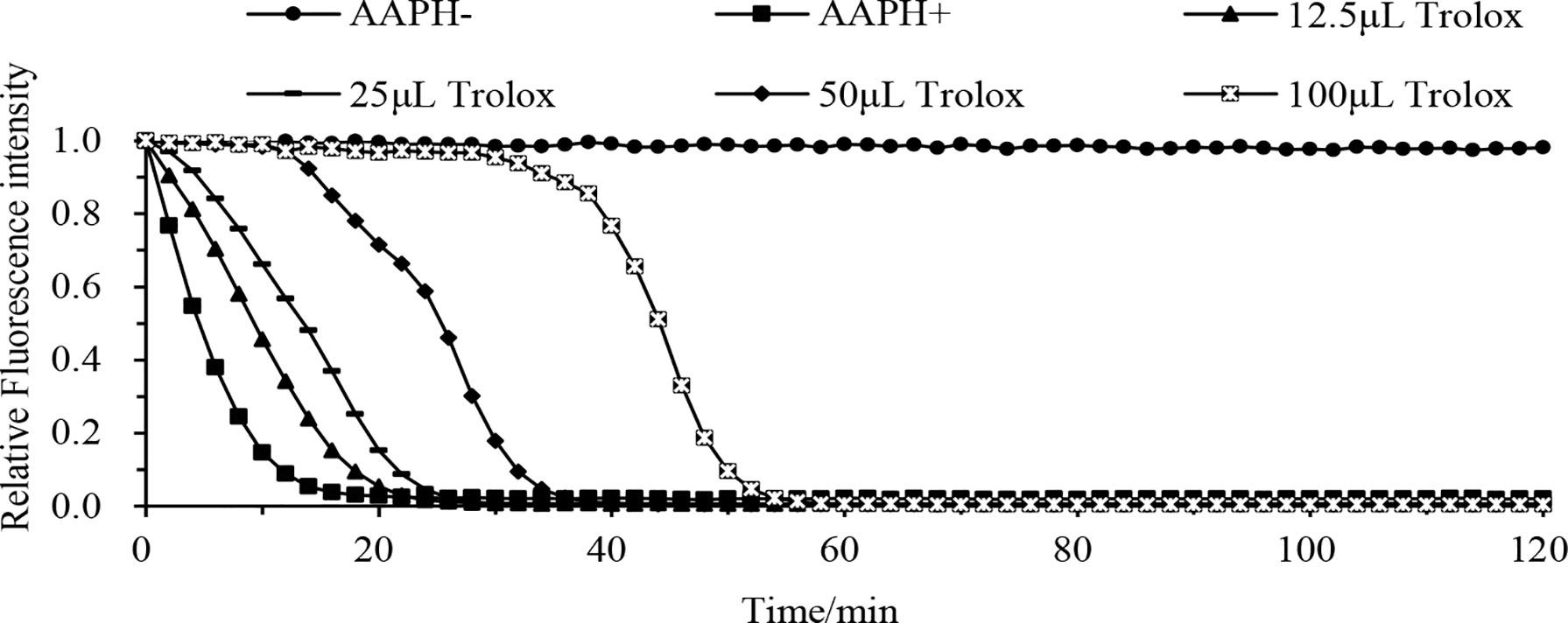
Figure 3 Fluorescence decay curve of different concentrations of Trolox with time. AAPH- is the fluorescence decay curve without adding AAPH solution and Trolox solution, AAPH+ is the fluorescence decay curve with the addition of AAPH solution without Trolox solution, Trolox is the fluorescence decay curve with the addition of AAPH solution and different concentrations of Trolox solution (12.5 µL, 25 µL, 50 µL, or 100 µL). Abbreviation: AAPH, 2,2’-azobis (2-methyl-propanimidamide) dihydrochloride.
Comprehensive Evaluation of Antioxidant Properties
The comprehensive antioxidant scores of the 36 experimental isolates are shown in Table 2. The antioxidant score value varied from 12.14 (GO 380) to 85.43 (GO 261). The 10 isolates with the highest scores (GO 261, GO 318, GO 91, GO 90, GO310) were selected for follow-up experiments.
Tolerance to Simulated Gastrointestinal Juice
The tolerance to simulated gastrointestinal juice assay results are shown in Table 3. All 10 selected isolates could survive at pH 2.5, pH 4, pH 6.8, and pH 8.0. For simulated stomach juice, at pH 2.5, the maximum survival rate was 49.80% in GO 91 followed by 42.84% in GO 90 and 35.71% in GO 295; At pH 4, the maximum survival rate was 90.81% in GO 261 followed by 89.64% in GO 264 and 85.31% in GO 90. For simulated intestinal juice, at pH 6.8, the maximum survival rate was GO 76.83% in GO 261, followed by 71.63% in GO 264 and 65.93% in GO 91; at pH=8, all 10 strains were higher than 60%.
Adhesion Ability
Auto-aggregation assay indicated that the auto-aggregation ability of the 10 selected isolates ranged from 18.70% to 84.81% (Figure 4). The highest auto-aggregation values (>75%) were observed in GO 284, GO 294, GO 299, GO 310, and GO 318. Cell surface hydrophobicity assay showed that all 10 selected isolates displayed relatively strong affinity with xylene, chloroform, and ethyl acetate (Figure 5).
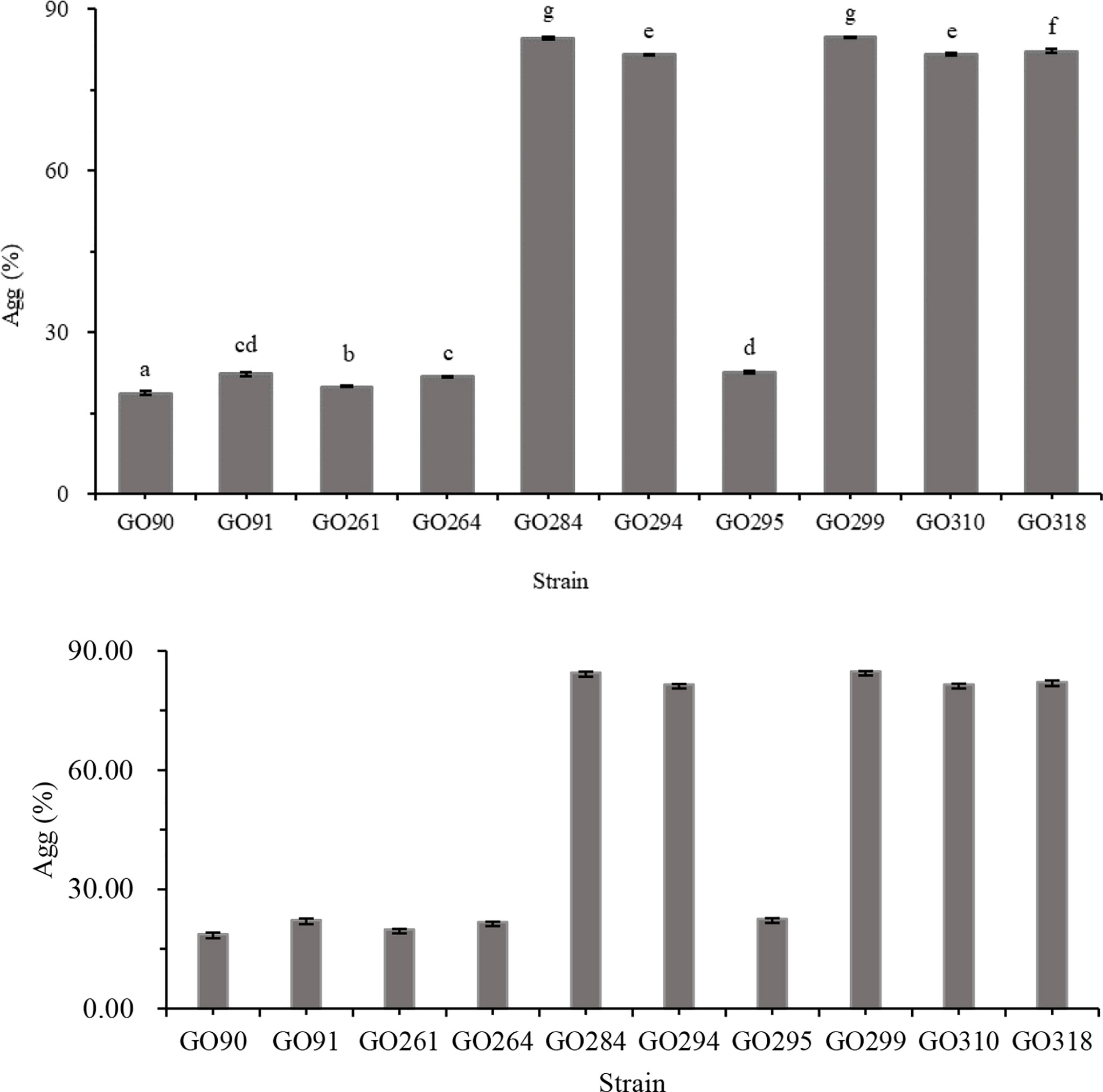
Figure 4 Auto-aggregation of 10 isolates (GO 90, GO91, GO261, GO264, GO284, GO294, GO295, GO299, GO310, GO318). Data are expressed as the mean ± SD (n = 3). Values not sharing a common superscript are significantly different (P< 0.05).
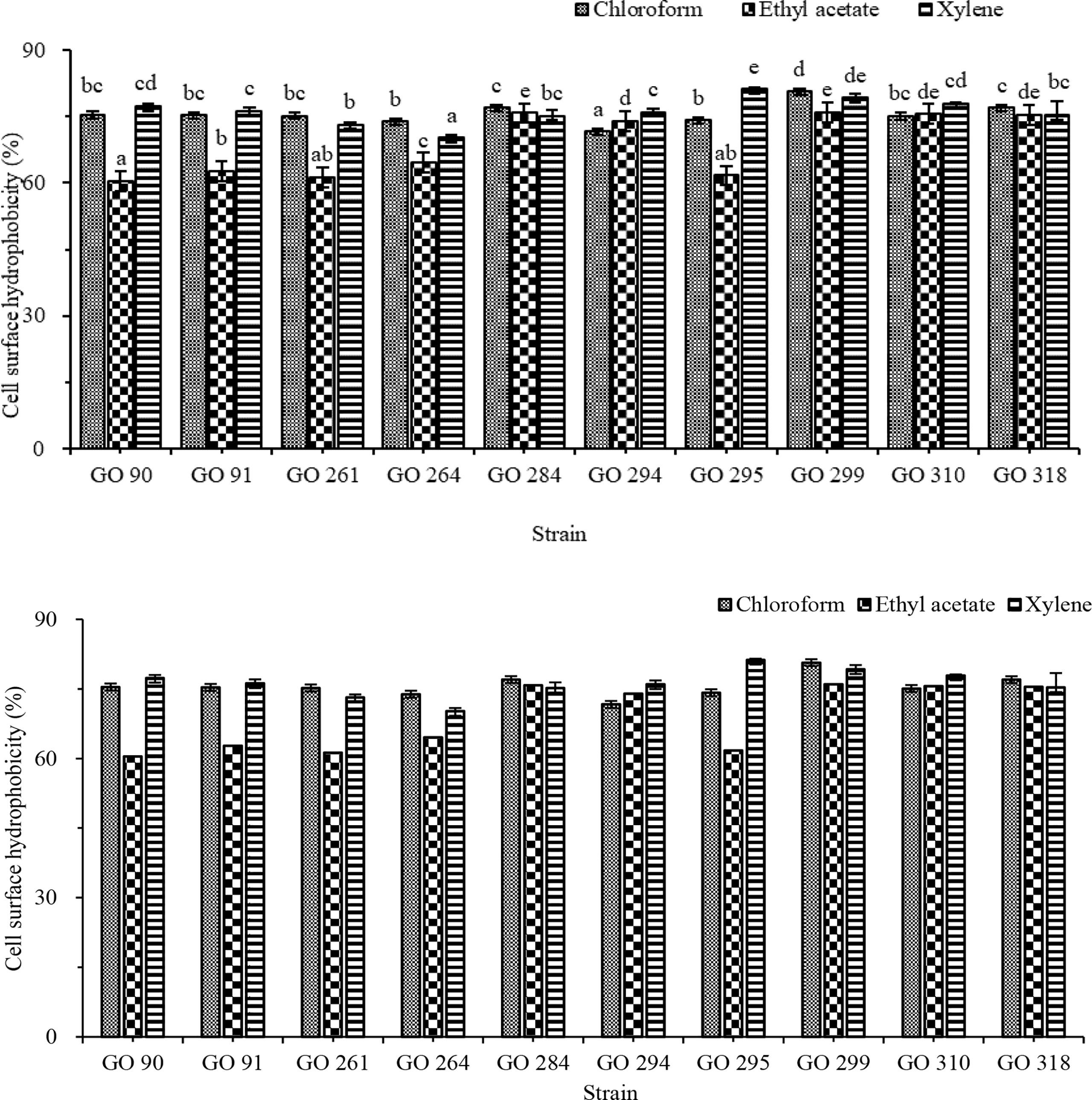
Figure 5 Surface hydrophobic activity of isolates. Data are expressed as the mean ± SD (n = 3). Values not sharing a common superscript are significantly different (P< 0.05).
Digestive Enzyme Activities and Antibacterial Activities
Digestive enzyme production assay results of the 10 selected isolates are shown in Table 4. Isolate GO 91 displayed the strongest protease activity; while isolate GO 261 displayed the strongest amylase activity.
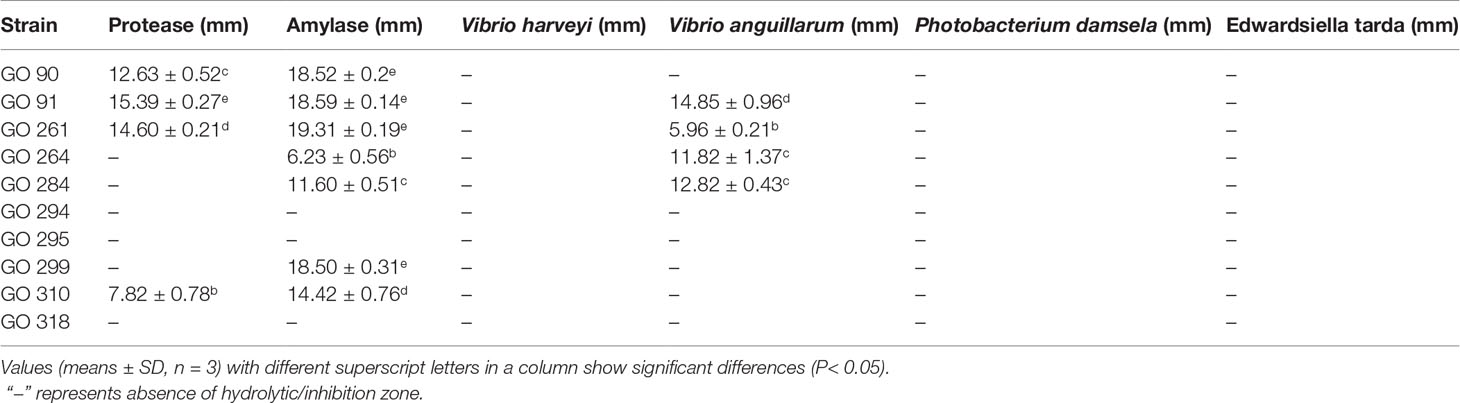
Table 4 Digestive enzyme activities (illustrated by hydrolytic zone diameter and antibacterial activities (illustrated by inhibition zone diameter) of 10 selected isolates.
Antibacterial activity tests showed that three isolates (GO 91, GO 264, and GO 284) exhibited marked antagonistic activity against Vibrio anguillarum. No isolate displayed antagonistic activity against Vibrio harveyi, Photobacterium damsela, or Edwardsiella tarda (Table 4).
Identification of Selected Isolates
The 16s rRNA sequence analysis revealed that the 10 selected isolates belong to two bacterial genera: Vibrio rhodolitus and Shewanella corallii. Isolate GO 90 (GenBank Accession No. ON415288), GO 91 (GenBank Accession No. ON386274), GO 261 (GenBank Accession No. ON415290), GO 264 (GenBank Accession No. ON415287), and GO 295 (GenBank Accession No. ON415291) all had high sequence homology (≥99.80%) with Vibrio rhodolitus (GenBank Accession No. NZQLYZ01000042.1), while GO 284 (GenBank Accession No.ON415320), GO 294 (GenBank Accession No.ON415292), GO 299 (GenBank Accession No. ON415311), GO 310 (GenBank Accession No. ON386273), and GO 318 (GenBank Accession No. ON415289) all had high sequence homology (≥99.22%) with Shewanella corallii (GenBank Accession No. NR116537.1). The constructed phylogenetic tree is shown in Figure 6.
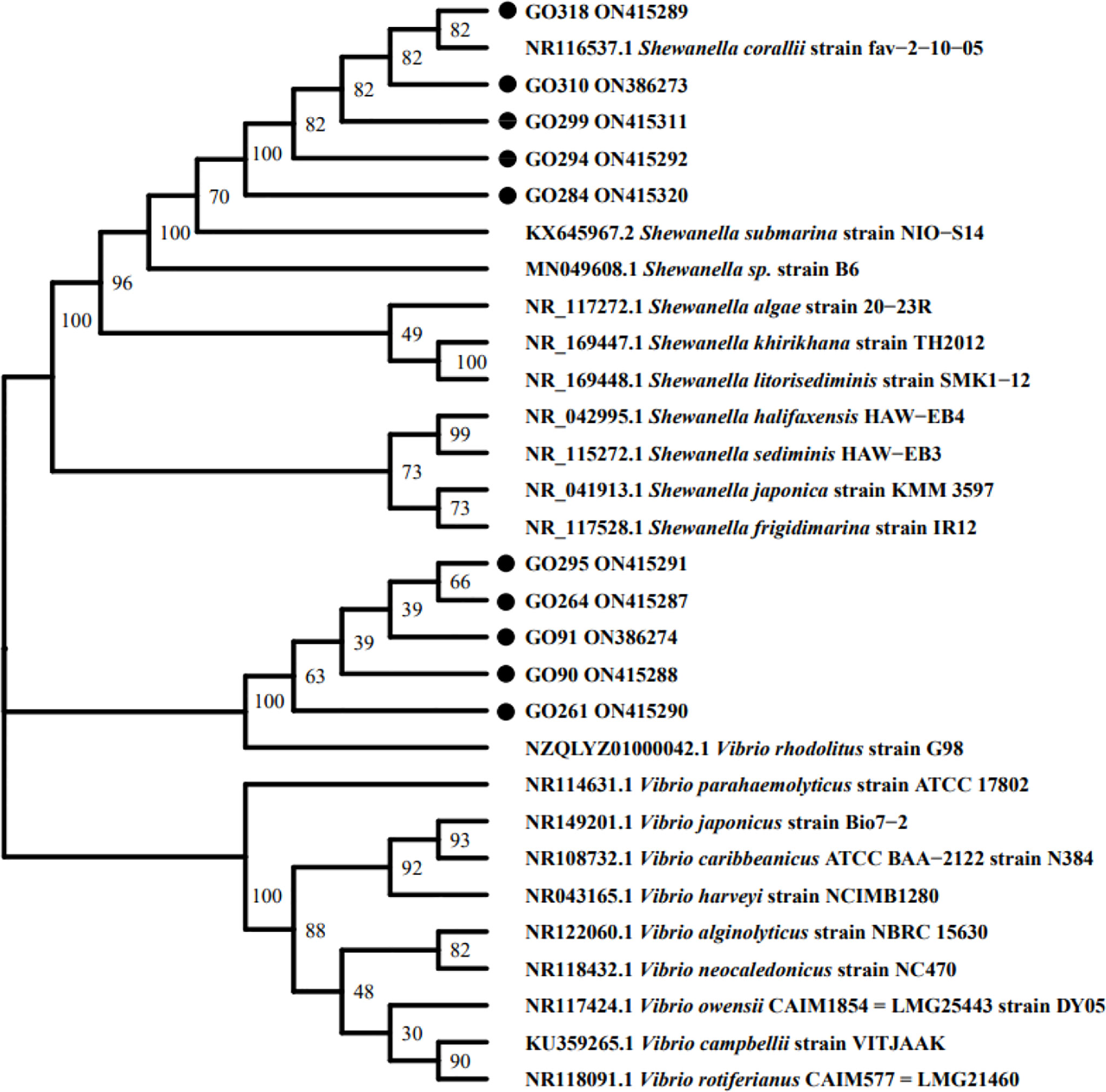
Figure 6 Phylogenetic tree of 10 selected isolates based on 16S rDNA sequence. The tree was constructed with MEGA-X software package using the Neighbor-Joining method.
Based on the results of other probiotic properties tests and identification results, Vibrio rhodolitus GO 91 and Shewanella corallii GO 310 were chosen for the follow-up safety tests.
Safety Tests
Antibiotics sensitivity test results of isolate GO 91 and GO 310 are shown in Table 5. Results indicated that among the 20 tested antibiotics, GO 91 is sensitive to six antibiotics, resistant to nine antibiotics, and intermediate to five antibiotics, while GO 310 is sensitive to 12 antibiotics, resistant to five antibiotics, and intermediate to three antibiotics. Strains were sensitive to many commonly used aquacultural antibiotics approved by the Chinese government (Lulijwa et al., 2020)
The in vivo challenge test of GO 91 or GO 310 didn’t cause any disease symptoms or death in hybrid grouper in 14 days.
Discussion
Previous antioxidant aquacultural probiotics studies mainly investigated the in vivo antioxidant effects of the potential isolates in aquacultural species, which inevitably involve feeding trials spanning 8 weeks or more (Giri et al., 2012; Yan et al., 2016; Gobi et al., 2018; Dawood et al., 2019). What’s more, though a large number of probiotics feeding experiments have shown that probiotics can improve the antioxidant indexes in the fish serum (such as SOD, CAT, or MDA), the action mechanism of these antioxidant probiotics (whether the bacteria have antioxidant activity themselves or somehow indirectly improve the antioxidant activity of the host) were essentially unclear. In this study, in vitro screening antioxidant methods, which are both time-saving and specific-antioxidant targeted, were employed to pre-screen probiotics with antioxidant properties for potential grouper farming application. Each of these in vitro methods targeted at one or one type of specific antioxidants. For example, DPPH assay determines the ability of antioxidants that could scavenge DPPH free radicals; ABTS assay estimates the ability of antioxidants that could quench free radical molecules ABTS•+ (Mishra et al., 2015); FRAP method measures the ability of antioxidants to reduce [Fe3+-(Tptz)2]3+ to [Fe2+-(Tptz)2]2+ (Mishra et al., 2015); The reducing assay evaluates the ability of antioxidants to directly reduce Fe3+ (CN-)6 to Fe2+ (CN-)6 (Gülçin, 2009); The ORAC method measures the ability of antioxidants to compete with fluorescein to react with peroxyl radicals (Schaich et al., 2015). A combination of these methods has been adopted for screening antioxidant probiotics in research areas such as food and beverage and waste water treatment. Das and Goyal (2015) showed that hydroxyl radical scavenging rate, superoxide anion radical scavenging rate, DPPH radical scavenging rate, and reducing activity of Lactobacillus plantarum isolated from Sikkim fermented beverage Marcha were 49%, 48%, 55%, and 149 mM (cysteine equivalent), respectively. Gaisawat et al. (2019) showed that using FRAP, DPPH assay, and iron and copper chelation to detect the redox state of normal fecal water and Clostridium difficile-infected fecal water in simulated upper digestive tract digestion caused by single-strain and multiple probiotic supplements. It was found that normal fecal water containing probiotic supplement (e.g., Lactobacillus rhamnosus R0011) showed significant iron chelation (p<0.05). In fecal water infected with C. difficile, all probiotic supplements showed a significant increase in FRAP and copper chelation (p<0.05). The scavenging rate of DPPH free radical, however, had no effect on the treatment of these two kinds of fecal water. Jang et al. (2018) showed that Lactobacillus plantarum LN1 was isolated from kimchi. With Lactobacillus plantarum KCTC3108 as a commercial probiotic as control, the antioxidant activity of live and heat-killed L. plantarum LN1 was evaluated by means of DPPH radical scavenging, ABTS+ radical scavenging, β-carotene and linoleic acid inhibition, and reducing activity. The results showed that the antioxidant activity of live L. plantarum LN1 was higher than that of the control strain. In L. plantarum LN1 heat inactivated cells, the bleaching inhibitory activity and reducing power of b-carotene were higher than those of DPPH and ABTS free radical scavenging activity. To our best knowledge, up to date only one aquacultural probiotic study adopted in vitro antioxidant methods (Giri et al., 2019). In that research, two in vitro antioxidant indexes were measured along with Pb-binding activities and other probiotic characteristics to screen Pb-resistant lactic acid bacteria. The present study, comprehensively evaluating nine antioxidant indexes in vitro, was the first aquaculture probiotic screening study focused on antioxidant abilities of the potential probiotics. in vitro In addition, considering that physiological activities of microorganisms are optimum when in their natural habitat (Liao et al., 2021), antioxidant isolates from the gut of grouper fish were screened in the present study to obtain local and host-derived probiotics for local aquaculture usage.
In the present study, a total of nine antioxidant assays (assay of scavenging activity against superoxide anion radicals, assay of hydrogen peroxide resistance, assay of scavenging activity against hydroxyl radical, assay of chelating ability on ferrous ions, assay of reducing activity, assay of ferric ion reducing antioxidant power, assay of oxygen-radical absorbance capacity, assay of scavenging of DPPH free radical, assay of scavenging of ABTS+ free radical) were used to comprehensively evaluate the antioxidant activity of the isolates. Each determination method of antioxidant activity has its advantages and disadvantages, and each determination of antioxidant activity has a specific target in a clear matrix, so it is best to use a variety of methods to determine it (Mishra et al., 2015). Compared with other methods, DPPH assay is a widely used method to evaluate antioxidant activity in a relatively short time (Elmastas et al., 2006). Therefore, DPPH assay was used to preliminary screen 36 isolates (DPPH scavenging rate > 50%) from 369 non-hemolytic isolates. Compared with ABTS•+ and DPPH assay, ORAC assay uses peroxyl radicals which are better models of antioxidant reaction with oxidizing lipids and reactive oxygen species in food and in vivo, and the continuous production of free radicals in time is more like the actual reaction (Schaich et al., 2015). Hydroxyl radicals are the main reactive oxygen species that cause lipid peroxidation and other large-scale biological damage to cells (Das and Goyal, 2015). Superoxide is a relatively weak oxidant, although its response is limited, but it will turn into more dangerous substances (e.g., singlet oxygen, hydroxyl radicals) which lead to lipid peroxidation (Gülçin, 2009). Hydrogen peroxide itself is not very active, but it produces hydroxyl radicals in the cells, which are toxic to the cells (Gülçin, 2009). Compared with HO•, O2-•, or active lipid oxygen free radicals in vivo, ABTS+• and DPPH assay use spatially blocked stable free radicals (Schaich et al., 2015). Iron exists in nature as ferrous (Fe2+) ion and iron ion (Fe3+). It will trigger the Fenton reaction in the body, and the reduced metal may form highly active hydroxyl radicals, resulting in oxidative stress. Fe2+ is the most effective oxidant in the food system, and good chelation will be beneficial. Chelating ferrous ions may be a promising method to prevent diseases caused by oxidative stress (Gülçin, 2009). The ability of scavenging 1-diphenyl-2-picrylhydrazine free radical, oxygen free radical absorption, anti-H2O2 and hydroxyl radical scavenging ability of lactic acid bacteria were determined by Mu et al. (2018). When screening lactic acid bacteria with strong antioxidant activity, it was found that the DPPH radical scavenging activity of lactic acid bacteria was not significantly correlated with the activity of other antioxidants (p > 0.05). There was a significant correlation among the antioxidant activity, 0.5mM H2O2 resistance, and hydroxyl radical scavenging ability of the strain in ORAC test (p < 0.05), and there was a significant correlation among the results of hydroxyl radical scavenging test, HT-29 cell protection against 0.5mM H2O2 damage, and cellular antioxidation test (p < 0.05). Based on previous antioxidant activity research using a variety of chemical methods and cell models, different weightings were assigned to each antioxidant index and an overall antioxidant score was calculated for each isolate, so that the antioxidant abilities of isolates could be comprehensively evaluated. The top 10 strains with the strongest antioxidant activities were screened for follow-up experiments.
The viability of probiotics under gastrointestinal conditions is also the key to the selection of probiotic strains (Meidong et al., 2021). Mortezaei et al. (2020) investigated the tolerance of 10 isolates at different pH (pH = 2.5, 3,3.5,4). The results showed that only five isolates could tolerate pH2.5 for 1.5 h. Meidong et al. (2021) used simulated gastric juice (SGJ, 3 mg/mL pepsin regulated to pH 2.5) and simulated intestinal juice (SIJ, 1 mg/mL trypsin, 3 mg/mL bile salt and adjusted to pH 8.0) to determine the survival rate of screening probiotics. The number of viable bacterial cells was counted by MRS agar dilution plate method after SGJ and SIJ were treated for 0.5, 1, 2, 3 h and 1, 2, 3, 6 h, respectively, and the potential probiotics with tolerance more than 50% were screened. In the present research, the survival rates of 10 isolates in pH = 2.5 and pH = 4.0 simulated gastric juice (3 mg/mL pepsin) for 3 h and in pH = 6.8 and pH=8.0 simulated intestinal juice (1 mg/mL trypsin, 3 mg/mL bile salt) for 6 h were determined. The results showed that only GO 90 and GO 91 could tolerate low pH 2.5. However, all isolates showed relatively high survival rates (>50%) at pH 4.0, 6.8, and 8.0. Literature (Murashita et al., 2021) and a preliminary experiment in our lab both showed that the pH of the stomach and intestine contents in carnivorous fish (tuna fish and hybrid grouper) ranged between 4.0 to 7.5. Therefore all 10 isolates tested could well tolerate the gut environment of hybrid grouper.
The auto-aggregation and hydrophobicity of probiotics cells are the indicators of bacterial cells colonizing and adhering to the surface of host intestinal mucosa (Meidong et al., 2021). Kavitha et al. (2018) used the Congo red method to determine the formation of biofilm and used toluene, chloroform, and ethyl acetate to determine the surface hydrophobicity of the isolates. Meidong et al. (2021) determined the auto-agglutination of the strain and used bacterial cells attached to hydrocarbons (xylene) to detect the hydrophobic activity of the strain. In this research, the auto-agglutination of the strain was determined, and the hydrophobic activity of the strain was determined by using toluene, chloroform, and ethyl acetate. Probiotics can also stimulate the host appetite, feed palatability, and host growth by secreting digestive enzymes, thus helping decompose indigestible ingredients in feed (Dawood et al., 2019). Therefore, amylase and protease activities of 10 isolates were determined in this study. The results showed that GO 90, GO 91, GO 261, and GO 310 produced protease, while GO 90, GO 91, GO 261, GO 264, GO 284, GO 299, and GO 310 produced amylase. In addition, probiotics regulate the growth and selectivity of intestinal microflora by inhibiting harmful bacteria and enhancing the natural defense mechanism of the host by producing inhibitors (Dawood et al., 2019). In this research, the antagonism of 10 isolates against Vibrio harveyi, V. anguillarum, Photobacterium damselae, and Edwardsiella tarda were detected. The results showed that GO 91, GO 261, GO 264, and GO2 84 could antagonize V. anguillarum. The 16s rRNA sequence analysis of 10 isolates showed that GO 90, GO 91, GO 261, GO 264, and GO 295 had high sequence homology with V. rosenbergii, while GO 284, GO 294, GO 299, GO 310, and GO 318 had high sequence homology with S. corallii. Combining antioxidant activity, gastrointestinal tolerance, adhesion, amylase, and protease in V. rosenbergii, the antagonistic selection of GO91 against pathogens was carried out in the follow-up experiment. Similarly, in S. corallii, GO310 was selected to carry on the follow-up experiment. The safety of strains is one of the evaluation criteria of probiotics. Intraperitoneal injection of isolate GO91 and GO 310 (100 µL, 1 × 108 CFU/mL) didn’t cause any disease symptoms or death to the tested hybrid grouper during the 2 weeks challenge period, indicating that the two strains were relatively safe if used to culture hybrid grouper.
Nowadays, more and more probiotic species have been found to be suitable for aquaculture. Probiotics used in aquaculture include: lactic acid bacteria, Bacillus, Aeromonas, Alteromonas, Arthrobacter, Bifidobacterium, Clostridium, Paenibacillus, Phaeobacter, Pseudoalteromonas, Pseudomonas, Rhodosporidium, Roseobacter, Streptomyces, Vibrio, Tetraselmis, Debaryomyces, Phaffia, and Saccharomyces (Gatesoupe, 1999; Merrifield et al., 2010; Yücel and Balci, 2010; Hai, 2015; Hoseinifar et al., 2018; Ringø et al., 2020). Among them, the main species of animal probiotics are lactic acid bacteria and Bacillus subtilis. Strains belonging to Vibrio are generally considered to be pathogens of aquaculture animals, but some Vibrio strains can be used as probiotics to play a probiotic role in the host. Ninawe and Selvin (2009) found that the non-pathogenic strain V. alginolyticus could significantly improve the survival rate of juvenile fish. Mujeeb Rahiman et al. (2010) researched the effects of Vibrio NE17 isolated from Macrobrachium rosenbergii larvae and egg samples on M. rosenbergii larvae (mixing, water bath, mixing and water bath). The results showed that SGR and weight gain were significantly improved in the experimental group (P< 0.001). The survival rate of experimental groups was improved comparing to that of the control group, and the water quality indexes such as nitrate concentration and ammonia nitrogen concentration of each experimental group were also significantly improved (P< 0.05). The immune indexes such as blood cell count, phenoloxidase activity, and respiratory burst were also significantly improved (P< 0.001). The strains (GO 91 and GO 310) showing the best overall antioxidant capacities in this experiment belong to two new probiotic species, V. rhodolitus and S. corallii. No available literature has reported their potential probiotic characteristics so far. Safety experiments in the present study showed that these two strains have no pathogenic effects on hybrid grouper. Their beneficial effects (antioxidant activities and other probiotic characteristics), however, need to be further verified through in vivo feeding experiments in future research.
Data Availability Statement
The datasets presented in this study can be found in online repositories. The names of the repository/repositories and accession number(s) can be found in the article/Supplementary Material.
Ethics Statement
This experiment was reviewed and approved by the Hainan University Institutional Animal Use and Care Committee, China (approval number: HNUAUCC-2020-00011). The animal experiments strictly abide by the principles of experimental animal welfare ethics, and accept the supervision and inspection of the Hainan University Institutional Animal Use and Care Committee.
Author Contributions
QZ and YC designed and carried out majority of the experiments and drafted the article. ZC participated in aquaculture experiment, sample collecting. HS participated in aquaculture experiment and sample collecting. YZ helped with antioxidant assays. YY helped with data collecting, RT participated in data analyzing. GC helped with sequencing. SW provided funding and was involved in writing-review and editing. All authors read and approved the final manuscript.
Funding
This study was funded by the National Natural Science Foundation of China (No. 31860739, 32173009).
Conflict of Interest
The authors declare that the research was conducted in the absence of any commercial or financial relationships that could be construed as a potential conflict of interest.
Publisher’s Note:
All claims expressed in this article are solely those of the authors and do not necessarily represent those of their affiliated organizations, or those of the publisher, the editors and the reviewers. Any product that may be evaluated in this article, or claim that may be made by its manufacturer, is not guaranteed or endorsed by the publisher.
Supplementary Material
The Supplementary Material for this article can be found online at: https://www.frontiersin.org/articles/10.3389/fmars.2022.933304/full#supplementary-material
References
Ashoori M., Saedisomeolia A. (2014). Riboflavin (Vitamin B2) and Oxidative Stress: A Review. Br. J. Nutr. 111, 1985–1991. doi: 10.1017/S0007114514000178.
Bojarski B., Kot B., Witeska M. (2020). Antibacterials in Aquatic Environment and Their Toxicity to Fish. Pharmaceuticals 13 (8), 189. doi: 10.3390/ph13080189
Beck B.R., Kim D., Jeon J., Lee S.M., Kim H.K., Kim O.J., et al. (2015). The Effects of Combined Dietary Probiotics Lactococcus Lactis BFE920 and Lactobacillus Plantarum FGL0001 on Innate Immunity and Disease Resistance in Olive Flounder (Paralichthys Olivaceus). Fish Shellfish Immunol. 42, 177–183. doi: 10.1016/j.fsi.2014.10.035
Capcarova M., Hascik P., Kolesarova A., Kacaniova M., Mihok M., Pal G. (2011). The Effect of Selected Microbial Strains on Internal Milieu of Broiler Chickens After Peroral Administration. Res. Veterinary Sci. 91, 132–137. doi: 10.1016/j.rvsc.2010.07.022
Ciji A., Akhtar M. S. (2021). Stress Management in Aquaculture: A Review of Dietary Interventions. Rev. Aquaculture 13, 2190–2247. doi: 10.1111/raq.12565
Clinical and Laboratory Standards Institute (2018). “Development of In Vitro Susceptibility Testing Criteria and Quality Control Parameters: Approved Guideline,” in CLSI Document M100, 28th ed (Wayne, PA: CLSI).
Das D., Goyal A. (2015). Antioxidant Activity and γ-Aminobutyric Acid (GABA) Producing Ability of Probiotic Lactobacillus Plantarum DM5 Isolated From Marcha of Sikkim. LWT-food Sci. Technol. 61 (1), 263–268. doi: 10.1016/j.lwt.2014.11.013
Daruosh A.A., Elahe S., Hojatollah J., Mehdi S., Mahsa N.S., Angel I.C.C.(2018). Efficacy of Two Commercial and Indigenous Probiotics, Bacillus Subtilis and Bacillus Licheniformis on Growth Performance, Immuno-Physiology and Resistance Response of Juvenile White Shrimp (Litopenaeus Vannamei). Aquaculture496, 43–49. doi: 10.1016/j.aquaculture.2018.06.082
Dawood M. A., Koshio S., Abdel-Daim M. M., Van Doan H. (2019). Probiotic Application for Sustainable Aquaculture. Rev. Aquaculture 11, 907–924. doi: 10.1111/raq.12272
Doan H., Doolgindachbaporn S., Suksri A. (2016). Effect of Lactobacillus Plantarum and Jerusalem Artichoke (Helianthus Tuberosus) on Growth Performance, Immunity and Disease Resistance of Pangasius Catfish (Pangasius Bocourti, Sauvage 1880). Aquac. Nutr. 22, 444–456. doi: 10.1111/anu.12263
Ejtahed H. S., Mohtadi-Nia J., Homayouni-Rad A., Niafar M., Asghari-Jafarabadi M., Mofid V. (2012). Probiotic Yogurt Improves Antioxidant Status in Type 2 Diabetic Patients. Nutrition 28 (5), 539–543. doi: 10.1016/j.nut.2011.08.013
Elmastas M., Turkekul I., Ozturk L., Gulcin I., Isildak O., Aboul-Enein H. Y. (2006). Antioxidant Activity of Two Wild Edible Mushrooms (Morchella Vulgaris and Morchella Esculanta) From North Turkey. Combinatorial Chem. High Throughput Screening 9, 443–448. doi: 10.2174/138620706777698544
Feng T., Wang J. (2020). Oxidative Stress Tolerance and Antioxidant Capacity of Lactic Acid Bacteria as Probiotic: A Systematic Review. Gut Microbes 12, 1801944. doi: 10.1080/19490976.2020.1801944
Gaisawat M. B., Iskandar M. M., MacPherson C. W., Tompkins T. A., Kubow S. (2019). Probiotic Supplementation is Associated With Increased Antioxidant Capacity and Copper Chelation in C. Difficile-Infected Fecal Water. Nutrients 11 (9), 2007. doi: 10.3390/nu11092007
Gao D., Gao Z., Zhu G. (2013). Antioxidant Effects of Lactobacillus Plantarum via Activation of Transcription Factor Nrf2. Food Funct. 4, 982–989. doi: 10.1016/j.carbpol.2013.07.027
Gatesoupe F. J. (1999). The Use of Probiotics in Aquaculture. Aquaculture 180, 147–165. doi: 10.1016/S0044-8486(99)00187-8
Giri S. S., Jun J. W., Yun S., Kim H. J., Kim S. G., Kang J. W., et al. (2019). Characterisation of Lactic Acid Bacteria Isolated From the Gut of Cyprinus Carpio That may be Effective Against Lead Toxicity. Probiotics antimicrobial Proteins 11, 65–73. doi: 10.1007/s12602-017-9367-6
Giri S. S., Sen S. S., Sukumaran V. (2012). Effects of Dietary Supplementation of Potential Probiotic Pseudomonas Aeruginosa VSG-2 on the Innate Immunity and Disease Resistance of Tropical Freshwater Fish, Labeo Rohita. Fish shellfish Immunol. 32, 1135–1140. doi: 10.1016/j.fsi.2012.03.019
Giri S.S, Sukumaran V, Oviya M (2013). Potential probiotic Lactobacillus plantarum VSG3 improves the growth, immunity, and disease resistance of Tropical Freshwater Fish, Labeo Rohita. Fish Shellfish Immunol. 34,660–666. doi: 10.1016/j.fsi.2012.12.008
Gobi N., Vaseeharan B., Chen J. C., Rekha R., Vijayakumar S., Anjugam M., et al. (2018). Dietary Supplementation of Probiotic Bacillus Licheniformis Dahb1 Improves Growth Performance, Mucus and Serum Immune Parameters, Antioxidant Enzyme Activity as Well as Resistance Against Aeromonas Hydrophila in Tilapia Oreochromis Mossambicus. Fish shellfish Immunol. 74, 501–508. doi: 10.1016/j.fsi.2017.12.066
Govindaraj K., Samayanpaulraj V., Narayanadoss V., Uthandakalaipandian R. (2021). Isolation of Lactic Acid Bacteria From Intestine of Freshwater Fishes and Elucidation of Probiotic Potential for Aquaculture Application. Probiotics Antimicrobial Proteins 13, 1598–1610. doi: 10.1007/s12602-021-09811-6
Gülçin İ.(2009). Antioxidant Activity of L-Adrenaline: A Structure–Activity Insight. Chemico-biological Interact. 179, 71–80. doi: 10.1016/j.cbi.2008.09
Gutiérrez Falcón A., Padilla D., Real F., Ramos Sosa M. J., Acosta-Hernández B., Sánchez Henao A., et al. (2021). Screening of New Potential Probiotics Strains Against Photobacterium Damselae Subsp. Piscicida for Marine Aquaculture. Animals 11, 2029. doi: 10.3390/ani11072029
Hai N. V. (2015). The Use of Probiotics in Aquaculture. J. Appl. Microbiol. 119, 917–935. doi: 10.1111/jam.12886
Hoseinifar S. H., Sun Y. Z., Wang A., Zhou Z. (2018). Probiotics as Means of Diseases Control in Aquaculture, a Review of Current Knowledge and Future Perspectives. Front. Microbiol., 9, 2429. doi: 10.3389/fmicb.2018.02429
Jang H. J., Song M. W., Lee N. K., Paik H. D. (2018). Antioxidant Effects of Live and Heat-Killed Probiotic Lactobacillus Plantarum Ln1 Isolated From Kimchi. J. Food Sci. Technol. 55 (8), 3174–3180. doi: 10.1007/s13197-018-3245-4
Kavitha M., Raja M., Perumal P. (2018). Evaluation of Probiotic Potential of Bacillus Spp. Isolated From the Digestive Tract of Freshwater Fish Labeo Calbasu (Hamilton 1822). Aquaculture Rep. 11, 59–69. doi: 10.1016/j.aqrep.2018.07.001
Kuebutornye F. K. A., Abarike E. D., Lu Y. (2019). A Review on the Application of Bacillus as Probiotics in Aquaculture. Fish Shellfish Immunol. 87, 820–828. doi: 10.1016/j.fsi.2019.02.010
Lazado C.C., Caipang C.M.A., Estante E.G.(2015). Prospects of Host-Associated Microrganisms in Fish and Penaeids as Probiotics with Immunomodulatory Functions. Fish Shellfish Immunol. 45, 2–12. doi: 10.1016/j.fsi.2015.02.023
Liao J., Cai Y., Wang X., Shang C., Zhang Q., Shi H., et al. (2021). Effects of a Potential Host Gut-Derived Probiotic, Bacillus Subtilis 6-3-1, on the Growth, Non-Specific Immune Response and Disease Resistance of Hybrid Grouper (Epinephelus Fuscoguttatus ♂ × Epinephelus Lanceolatus ♂). Probiotics Antimicrobial Proteins 13, 1119–1137. doi: 10.1007/s12602-021-09768-6
Li J. Y., Jin M. M., Meng J., Gao S. M., Lu R. R. (2013). Exopolysaccharide From Lactobacillus Planterum LP6: Antioxidation and the Effect on Oxidative Stress. Carbohydr. polymers 98, 1147–1152. doi: 10.1016/j.carbpol.2013.07.027
Limbu S. M., Chen L. Q., Zhang M. L., Du Z. Y. (2021). A Global Analysis on the Systemic Effects of Antibiotics in Cultured Fish and Their Potential Human Health Risk: A Review. Rev. Aquaculture 13, 1015–1059. doi: 10.1111/raq.12511
Liu C. F., Tseng K. C., Chiang S. S., Lee B. H., Hsu W. H., Pan T. M. (2011). Immunomodulatory and Antioxidant Potential of Lactobacillus Exopolysaccharides. J. Sci. Food Agric. 91, 2284–2291. doi: 10.1002/jsfa.4456
Lulijwa R., Rupia E. J., Alfaro A. C. (2020). Antibiotic Use in Aquaculture, Policies and Regulation, Health and Environmental Risks: A Review of the Top 15 Major Producers. Rev. Aquaculture 12, 640–663. doi: 10.1111/raq.12344
Malek R. L., Sajadi H., Abraham J., Grundy M. A., Gerhard G. S. (2004). The Effects of Temperature Reduction on Gene Expression and Oxidative Stress in Skeletal Muscle From Adult Zebrafish. Comp. Biochem. Physiol. Part C: Toxicol. Pharmacol. 138 (3), 363–373. doi: 10.1016/j.cca.2004.08.014
Meidong R., Nakao M., Sakai K., Tongpim S. (2021). Lactobacillus Paraplantarum L34b-2 Derived From Fermented Food Improves the Growth, Disease Resistance and Innate Immunity in Pangasius Bocourti. Aquaculture 531, 735878. doi: 10.1016/j.aquaculture.2020.735878
Merrifield D. L., Dimitroglou A., Foey A., Davies S. J., Baker R. T., Bøgwald J., et al. (2010). The Current Status and Future Focus of Probiotic and Prebiotic Applications for Salmonids. Aquaculture 302, 1–18. doi: 10.1016/j.aquaculture.2010.02.007
Mishra V., Shah C., Mokashe N., Chavan R., Yadav H., Prajapati J. (2015). Probiotics as Potential Antioxidants: A Systematic Review. J. Agric. Food Chem. 63, 3615–3626. doi: 10.1021/jf506326t
Mortezaei F., Royan M., Allaf Noveirian H., Babakhani A., Alaie Kordghashlaghi H., Balcázar J. L. (2020). In Vitro Assessment of Potential Probiotic Characteristics of Indigenous Lactococcus Lactis and Weissella Oryzae Isolates From Rainbow Trout (Oncorhynchus Mykiss Walbaum). J. Appl. Microbiol. 129, 1004–1019. doi: 10.1111/jam.14652
Mu G., Gao Y., Tuo Y., Li H., Zhang Y., Qian F., et al. (2018). Assessing and Comparing Antioxidant Activities of Lactobacilli Strains by Using Different Chemical and Cellular Antioxidant Methods. J. Dairy Sci. 101, 10792–10806. doi: 10.3168/jds.2018-14989
Mujeeb Rahiman K. M., Jesmi Y., Thomas A. P., Mohamed Hatha A. A. (2010). Probiotic Effect of Bacillus NL110 and Vibrio NE17 on the Survival, Growth Performance and Immune Response of Macrobrachium Rosenbergii (De Man). Aquaculture Res. 41, e120–e134. doi: 10.1111/j.1365-2109.2009.02473.x
Murashita K., Hashimoto H., Takashi T., Eba T., Kumon K., Matsunari H., et al. (2021). Characterization of Digestive Physiology in Pacific Bluefin Tuna Thunnus Orientalis Juveniles Fed a Raw Fish Feed and a Commercial Diet. Aquaculture 538, 736562. doi: 10.1016/j.aquaculture.2021.736562
Ninawe A. S., Selvin J. (2009). Probiotics in Shrimp Aquaculture: Avenues and Challenges. Crit. Rev. Microbiol. 35, 43–66. doi: 10.1080/10408410802667202
Pandiyan P., Balaraman D., Thirunavukkarasu R., George E. G. J., Subaramaniyan K., Manikkam S., et al. (2013). Probiotics in Aquaculture. Drug invention Today 5, 55–59. doi: 10.1016/j.dit.2013.03.003
Persichetti E., De Michele A., Codini M., Traina G. (2014). Antioxidative Capacity of Lactobacillus Fermentum LF31 Evaluated In Vitro by Oxygen Radical Absorbance Capacity Assay. Nutrition 30, 936–938. doi: 10.1016/j.nut.2013.12.009
Ramalho J. B., Soares M. B., Spiazzi C. C., Bicca D. F., Soares V. M., Pereira J. G., et al. (2019). In Vitro Probiotic and Antioxidant Potential of Lactococcus Lactis Subsp. Cremoris LL95 and its Effect in Mice Behaviour. Nutrients 11, 901. doi: 10.3390/nu11040901
Raza M. H., Siraj S., Arshad A., Waheed U., Aldakheel F., Alduraywish S., et al. (2017). ROS-Modulated Therapeutic Approaches in Cancer Treatment. J. Cancer Res. Clin. Oncol. 143, 1789–1809. doi: 10.1007/s00432-017-2464-9
Ringø E., Doan H. V., Lee S., Song S. K. (2020). Lactic Acid Bacteria in Shellfish: Possibilities and Challenges. Rev. Fisheries Sci. Aquaculture 28, 139–169. doi: 10.1080/23308249.2019.1683151
Rueda-Jasso R., Conceiçao L. E., Dias J., De Coen W., Gomes E., Rees J. F., et al. (2004). Effect of Dietary non-Protein Energy Levels on Condition and Oxidative Status of Senegalese Sole (Solea Senegalensis) Juveniles. Aquaculture 231 (1-4), 417–433. doi: 10.1016/S0044-8486(03)00537-4
Schaich K. M., Tian X., Xie J. (2015). Hurdles and Pitfalls in Measuring Antioxidant Efficacy: A Critical Evaluation of ABTS, DPPH, and ORAC Assays. J. Funct. foods 14, 111–125. doi: 10.1016/j.jff.2015.01.043
Schieber M., Chandel N. S. (2014). ROS Function in Redox Signaling and Oxidative Stress. Curr. Biol. 24, 453–462. doi: 10.1016/j.cub.2014.03.034
Shen Q., Shang N., Li P. (2011). In Vitro and In Vivo Antioxidant Activity of Bifidobacterium Animalis 01 Isolated From Centenarians. Curr. Microbiol. 62, 1097–1103. doi: 10.1007/s00284-010-9827-7
Song S. G., Chi S. Y., Tan B. P., Liang G. L., Lu B. Q., Dong X. H., et al. (2018). Effects of Fishmeal Replacement by Tenebrio Molitor Meal on Growth Performance, Antioxidant Enzyme Activities and Disease Resistance of the Juvenile Pearl Gentian Grouper (Epinephelus Lanceolatus ♂ × Epinephelus Fuscoguttatus♂). Aquaculture Res. 49, 2210–2217. doi: 10.1111/are.13677
Son S. H., Yang S. J., Jeon H. L., Yu H. S., Lee N. K., Park Y. S., et al. (2018). Antioxidant and Immunostimulatory Effect of Potential Probiotic Lactobacillus Paraplantarum SC61 Isolated From Korean Traditional Fermented Food, Jangajji. Microbial Pathogenesis 125, 486–492. doi: 10.1016/j.micpath.2018.10.018
Sopková D., Hertelyová Z., Andrejčáková Z., Vlčková R., Gancarčíková S., Petrilla V., et al. (2017). The Application of Probiotics and Flaxseed Promotes Metabolism of N-3 Polyunsaturated Fatty Acids in Pigs. J. Appl. Anim. Res. 45, 93–98. doi: 10.1080/09712119.2015.1124333
Sui L., Zhu X., Wu D., Ma T., Tuo Y., Jiang S., et al. (2020). In Vitro Assessment of Probiotic and Functional Properties of Bacillus Coagulans T242. Food Bioscience 36, 100675. doi: 10.1016/j.fbio.2020.100675
Sun B., Lei Y., Cao Z., Zhou Y., Sun Y., Wu Y., et al. (2019). TroCCL4, a CC Chemokine of Trachinotus Ovatus, is Involved in the Antimicrobial Immune Response. Fish Shellfish Immunol. 86, 525–535. doi: 10.1016/j.fsi.2018.11.080
Tovar-Ramirez D., Mazurais D, Gatesoupe J.F, Quazuguel P, Cahu C.L, Zambonino, □, Infante J.L (2010). Dietary Probiotic Live Yeast Modulates Antioxidant Enzyme Activities and Gene Expression of Sea Bass (Dicentrarchus Labrax) Larvae. Aquaculture 300, 142–147. doi: 10.1016/j.aquaculture.2009.12.015
Vine N. G., Leukes W. D., Kaiser H. (2006). Probiotics in Marine Larviculture. FEMS Microbiol. Rev. 30, 404–427. doi: 10.1111/j.15746976.2006.00017.x
Wang Y., Wu Y., Wang Y., Fu A., Gong L., Li W., et al. (2017a). Bacillus Amyloliquefaciens SC06 Alleviates the Oxidative Stress of IPEC-1 via Modulating Nrf2/Keap1 Signaling Pathway and Decreasing ROS Production. Appl. Microbiol. Biotechnol. 101, 3015–3026. doi: 10.1007/s00253-016-8032-4
Wang Y., Wu Y., Wang Y., Xu H., Mei X., Yu D., et al. (2017b). Antioxidant Properties of Probiotic Bacteria. Nutrients 9, 521. doi: 10.3390/nu9050521
Wei G., Tan H., Ma S., Sun G., Zhang Y., Wu Y., et al. (2020). Protective Effects of β-Glucan as Adjuvant Combined Inactivated Vibrio Harveyi Vaccine in Pearl Gentian Grouper. Fish Shellfish Immunol. 106, 1025–1030. doi: 10.1016/j.fsi.2020.09.027
Xu X., Liu K., Wang S., Guo W., Xie Z., Zhou Y. (2017). Identification of Pathogenicity, Investigation of Virulent Gene Distribution and Development of a Virulent Strain-Specific Detection PCR Method for Vibrio Harveyi Isolated From Hainan Province and Guangdong Province, China. Aquaculture 468, 226–234. doi: 10.1016/j.aquaculture.2016.10.015
Yang C., Lim W., Song G. (2020). Mediation of Oxidative Stress Toxicity Induced by Pyrethroid Pesticides in Fish. Comp. Biochem. Physiol. Part C: Toxicol. Pharmacol. 234, 108758. doi: 10.1016/j.cbpc.2020.108758
Yan Y. Y., Xia H. Q., Yang H. L., Hoseinifar S. H., Sun Y. Z. (2016). Effects of Dietary Live or Heat-Inactivated Autochthonous Bacillus Pumilus SE 5 on Growth Performance, Immune Responses and Immune Gene Expression in Grouper Epinephelus Coioides. Aquaculture Nutr. 22, 698–707. doi: 10.1111/anu.12297
Keywords: antioxidant probiotic, in vitro screening, hybrid grouper, safety, gut
Citation: Zhang Q, Cai Y, Chen Z, Shi H, Zhou Y, Yang Y, Tu R, Chen G and Wang S (2022) Screening and Characterization of Potential Antioxidant Probiotics Isolated from the Gut of Hybrid Grouper (Epinephelus fuscoguttatus ♀ × Epinephelus lanceolatus ♂). Front. Mar. Sci. 9:933304. doi: 10.3389/fmars.2022.933304
Received: 30 April 2022; Accepted: 09 June 2022;
Published: 19 July 2022.
Edited by:
Einar Ringo, UiT The Arctic University of Norway, NorwayCopyright © 2022 Zhang, Cai, Chen, Shi, Zhou, Yang, Tu, Chen and Wang. This is an open-access article distributed under the terms of the Creative Commons Attribution License (CC BY). The use, distribution or reproduction in other forums is permitted, provided the original author(s) and the copyright owner(s) are credited and that the original publication in this journal is cited, in accordance with accepted academic practice. No use, distribution or reproduction is permitted which does not comply with these terms.
*Correspondence: Shifeng Wang, U2hpZmVuZ18xNUAxNjMuY29t
†These authors have contributed equally to this work