- 1Key Laboratory of South China Sea Fishery Resources Exploitation and Utilization, Ministry of Agriculture and Rural Affairs, Guangzhou, China
- 2Tropical Aquaculture Research and Development Center, South China Sea Fisheries Research Institute, Chinese Academy of Fishery Sciences, Sanya, China
- 3Sanya Tropical Fisheries Research Institute, Sanya, China
- 4Guangxi Key Laboratory Cultivation Base for Polysaccharide Materials and Modifications, Guangxi University for Nationalities, Nanning, China
- 5Key Laboratory of Protection and Utilization of Marine Resources, Guangxi University for Nationalities, Nanning, China
This study sought to characterize the effect of density stress on greater amberjack (Seriola dumerili) survival to determine an optimal transport density. To achieve this, this experiment simulated the transport conditions of fish (body length: 4.09 ± 1.00 cm; weight: 0.9 ± 0.05 g) using closed oxygen transport at 5 different densities (D1 = 1.125 kg/m3, D2 = 2.25 kg/m3, D3 = 3.375 kg/m3, D4 = 4.5 kg/m3, and D5 = 6.75 kg/m3) for 8 hours, after which biochemical indicators, stress, metabolism, and antioxidant capacity were evaluated. After 8h, only the D1 and D3 groups exhibited survival rates above 90%. The pH of the water decreased with density, whereas the ammonia nitrogen and nitrite increased with density. Cortisol (COR) levels were not significantly different among all the groups, but tended to increase with increasing density. In this study, COR is a relatively stable index with the increase in density, but in D3 group, the change of COR will not cause the change of immune-related genes, so COR can inhibit the expression of immune genes within a certain density. The D3 density group exhibited the highest catalase (CAT) and glutathione peroxidase glutathione peroxidase (GSH‐PX) levels. In addition, the expression levels of immune related factor interleukin-1β (IL-1β), tumor necrosis factor (TNF-α), major histocompatibility complex (MHC-1) and nuclear factor kappa-B (NF-KB1) were the lowest in D3 density group. The density of fish during transportation significantly affects water quality, metabolism, and immunity. During transportation, assuming that the plastic bags are airtight to ensure aerobic conditions and that the transportation time is within 8 h, transport density should be maintained at approximately 3.375 kg/m3.
Introduction
In recent years, fish seed transportation has become increasingly common due to the gradual development of aquaculture. The impact of external forces, water quality, and extended transport time can increase the chances of bacterial infections, thus increasing fish mortality (Wang et al., 2017). How to effectively transport has become one of the complex problems in aquatic development (Yi-Kuan et al., 2020). In the process of transportation, the density is large and the environment is poor, which is easy to cause a stress reaction. Acute stress changes the behavioral state of fish and the concentration of hormones and affects metabolism, antioxidant function and nonspecific immunity (Ghisleni et al., 2012; Sahin et al., 2013; Song et al., 2019; Long et al., 2021).
Stress response may be caused by the deterioration of water quality (Singh et al., 2004). The deterioration of water quality may be caused by the increase of CO2 and ammonia nitrogen concentration and the decrease of pH due to the high density (Hong et al., 2021). Stress caused by increased density adversely affects fish growth, antioxidation, immune system, and health (Adineh et al., 2020). The stress response is closely regulated by cortisol (COR). COR can induce the synthesis of heat shock protein 70 (HSP70) (Deane et al., 2006). The stress response can cause the activity of Carnitine palmitoyltransferase-1 (CPT1) decreased, increasing the activity of fatty acid synthase (FAS) (Yu et al., 2020). COR secretion can promote glycogenolysis and gluconeogenesis (Hur et al., 2007). The increase in glycogen concentration would cause the increase of pyruvate kinase (PK). After fish are stressed, the contents of superoxide dismutase (SOD), catalase (CAT), glutathione peroxidase (GSH-PX), malondialdehyde (MDA) and heme oxygenase-1(HO-1) in antioxidant indexes will change significantly (Sahin et al., 2013; Song et al., 2019; Long et al., 2021). HO-1 protects the body from oxidant induced damage during inflammation (Yuan et al., 2012). According to relevant studies, the stress can cause the expression level of tumor necrosis factor (TNF-α) and interleukin-1β (IL-1β) were increased (Hoseini et al., 2019; Zhang et al., 2021). Overcrowding can also cause the expression level of transforming growth factor-β (TGF-β1) anti-inflammatory factors increased (Hou et al., 2020). The increase in COR concentration will limit the release of complement 3 (C3) concentration (Padgett and Glaser, 2003). Major histocompatibility complex (MHC-1) and immune globulin T (IgT) are both immune-related genes. MHC-1 is an essential immune indicator marking virus invasion (Long et al., 2019). In case of bacterial invasion, the relative expression level of IgT will increase (Buchmann, 2020). When fish have inflammation, the expression level of nuclear factor kappa-B (NF-KB1) will increase.
The greater amberjack (Seriola dumerili) is a large long-range fish that belongs to the Perciformes and is distributed from the Indian Ocean to the Western Pacific (Lozano-Bilbao et al., 2021). It lives in temperate and warm seawater. This species has attracted more and more attention from the global aquaculture industry and is also considered to be the main candidate for the diversification of mariculture in the Mediterranean region (Gamberoni et al., 2021). Greater amberjack is a fast-growing species that have made outstanding contributions to the diversification of global aquaculture (Peng et al., 2022). The greater amberjack is a migratory fish, often in shallow water. During its larval stage, the diet of this fish is primarily dominated by zooplankton, whereas larger fish are carnivorous. The greater amberjack is sought after by consumers due to its tender meat, which is rich in protein and fat, and therefore its market price is now higher than that of most marine fish (Rigos et al., 2020). At present, there are many studies on the transport density of fish, including golden pompano(Trachinotus ovatus), grouper(Epinephelus spp.), and siberian sturgeon(Acipenser baerii) (Pereira Cardona et al., 2017; Hong et al., 2019; Kurtoglu et al., 2021). However, there are few studies on greater amberjack transport density.
However, due to the importance of live transport for the development of the aquaculture industry, the effects of stress can be reduced by optimizing transport density. In this study, fish were placed in oxygen-filled bags to simulate their transportation on roads. The optimal conditions for live fish transportation were determined based on stress markers, metabolism, antioxidant, and immune indices. Our findings provide a theoretical basis for determining the optimal transport density of the greater amberjack in the context of aquaculture development.
Materials and Methods
Animals
Greater amberjack specimens were collected from the Tropical Fisheries Research and Development Center of the South China Sea Institute of Fishery Sciences and reared in an indoor recirculating aquaculture system. The experimental animals were transferred to a 5000 L cement tank and domesticated for one week. During acclimation, the environmental parameters were maintained at 33‰, 29 ± 1°C, pH 7.8 ± 0.1, DO > 6.5 mg/L, light intensity < 500 LX and natural photoperiod. Compound feed was fed at regular intervals (09: 00-09: 30) in the morning, and 50% of the seawater was replaced daily. The feeding was stopped at the day before the experiment started.
Simulated Transportation
The greater amberjack cultured in the circulatory system was selected as the experiment objects (weight: 0.9 ± 0.05 g; total length: 4.09 ± 1.00 cm). The trial was run at five fish densities [1.125 kg/m3 (D1), 2.25 kg/m3 (D2), 3.375 kg/m3 (D3), 4.5 kg/m3 (D4), and 6.75 kg/m3 (D5)] in triplicate. The fish were transferred to 40-liter polyethylene bags (48 cm × 37 cm × 23 cm) at the five aforementioned densities. Each bag contained 4 liters of fresh seawater. And adjust the salinity of seawater to 33.0‰. To calm the fish, the transport water was treated with 7 mg/L of eugenol (Shang Chi Dental Material Co., Ltd., Changshu, China) (Cupp et al., 2016). These bags are filled with oxygen, sealed with rubber bands, and then put into a box containing two 500ml ice bags. Put the bags in a unified position and seal them with tape. The simulated transport experiment lasted 8 h (Hong et al., 2019). The boxes were shaken up at 1-h intervals during the experiment to simulate water movement during transportation, as well as to increase dissolved oxygen levels.
Sampling and Analysis
After simulated transportation, a water quality meter (HACH HQ40d, Shanghai, China) was used to record the temperature, pH, and dissolved oxygen (DO) of each experimental group, and mortality rates were also calculated. A total of 500 ml water sample per bag was taken to determine ammonia nitrogen concentration with a multi-parameter water quality meter based on Nessler’s reagent spectrophotometry method (Octadem, W-11, Wuxi, China). Next, three fish were taken from each bag as whole fish samples, killed with overdosed MS222, and then put into 2 mL centrifuge tube separately, and 0.86% normal saline was added to the centrifuge tube in a ratio of 1:9. The mixture was then homogenized with a hand-held homogenizer (Gloucester, Prima PB100, England) on ice. The homogenate was centrifuged at 3500×g for 10 min, and the supernatant was collected and stored at -80°C for biochemical index analysis. MDA(Thiobarbituric Acid test method), glycogen (Anthrone method), COR, ACTH and CRH(Double antibody sandwich method) in whole fish were determined according to the manufacturer’s instructions using a commercial assay kit (Nanjing Jiancheng Bioengineering Institute, Nanjing, China).
Total RNA Extraction and Reverse Transcription
Total RNA was extracted from whole fish using the Trizol reagent (Invitrogen, Thermo Fisher Scientific Co., Ltd., Shanghai, China) according to the manufacturer’s instructions. RNA concentration and purity were assessed using a micro ultraviolet spectrophotometer (ND5000, Bioteke Corporation, Beijing, China) and agarose gel electrophoresis. RNA samples with an OD260/280 (optical density) ratio of 1.8–2.0 were considered pure. Next, to obtain first-strand cDNA, reverse transcription was conducted with 1µg of total RNA using the One-Step gDNA Removal and cDNA Synthesis SuperMix (EasyScript, Beijing TransGen Biotech Co., Ltd., Beijing, China) according to the manufacturer’s instructions.
Immune Gene Fluorescence Quantitation Analysis
Quantitative real-time PCR analysis was conducted using a PCR system (Q1000, Hangzhou Long Gene Scientific Instruments Co., Ltd., Hangzhou, China) using the following thermal cycling program: initial denaturation at 95°C for 15 min, 40 cycles of denaturation at 95°C for 10 s, annealing at 60°C for 20 s, extension at 72°C for 30 s. All PCR amplifications were performed in triplicate in 20 μL reaction volumes containing 10 μL of 2x RealUniversal PreMix, 0.6 μL (10 µmol/L) of each primer, 2 µL of template cDNA, and 6.8 µL of RNase-free ddH2O. β-action was used as the reference gene. A dissociation analysis was conducted to determine the absence of nonspecific products at the end of each PCR reaction. A single peak was seen on the melt curve analysis, thus confirming that only one PCR product was amplified. A standard curve was established based on 10-fold serial dilutions of cDNA for each primer pair, and all PCR reactions achieved efficiencies that ranged between 90% and 110%. Table 1 details the primers used for gene expression analysis.
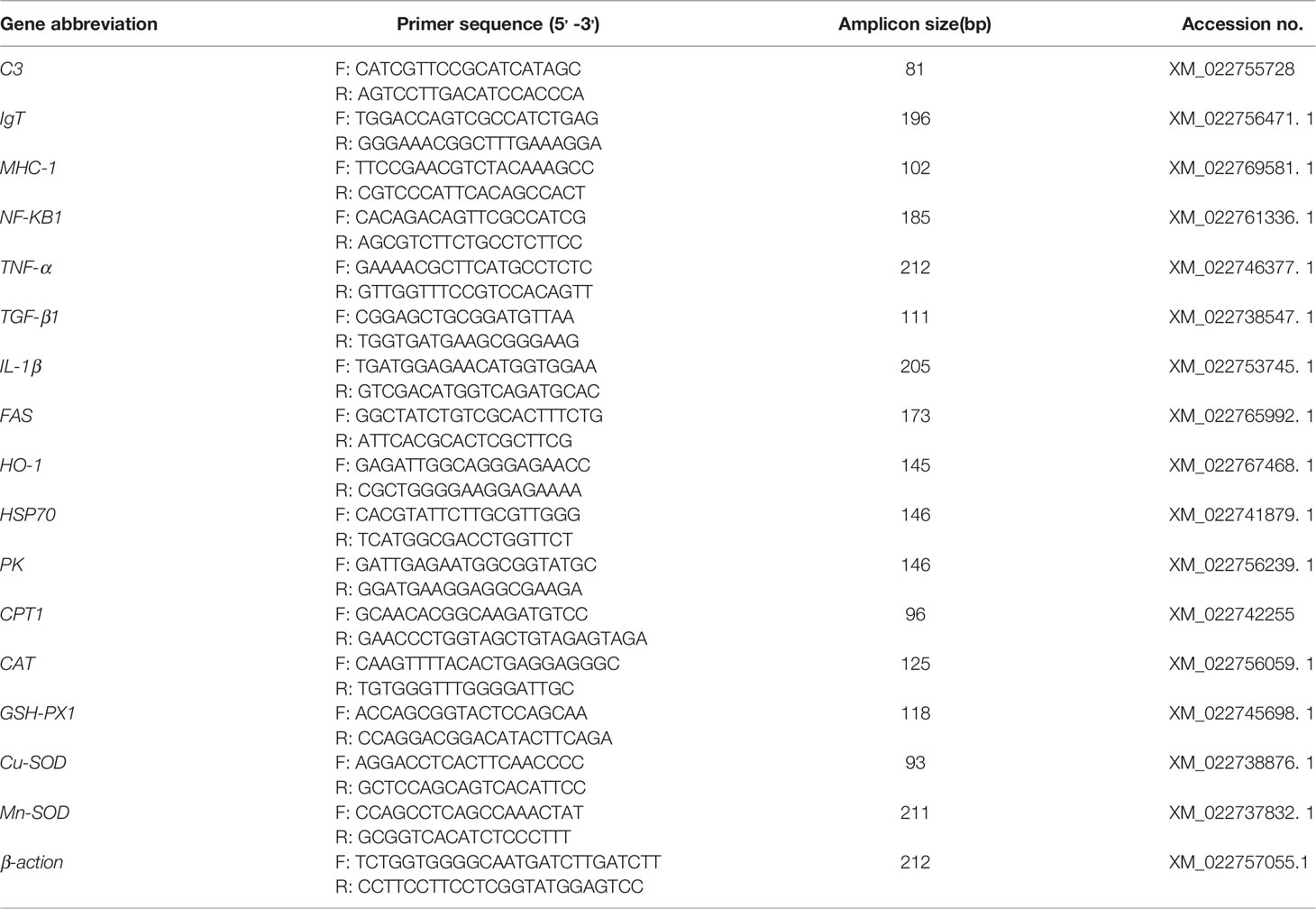
Table 1 Set of forward and reverse primers used for the immune gene expression analysis in greater amberjack.
Statistical Analysis
Excel software was used for data collation, and Origin 2021 was used for mapping. All data were analyzed using SPSS 26.0, and the results were presented as means ± standard deviation (mean ± SD). Comparisons between groups were performed via one-way ANOVA coupled with the LSD test. Significant difference was considered at P < 0.05.
Results
Water Quality and Survival Rate After Transportation
The temperature, dissolved oxygen, pH, and ammonia nitrogen of water at different transport densities are shown in Table 2. The temperature of D2 and D3 groups was significantly higher than in the other three groups. There were significant differences in dissolved oxygen between adjacent groups (P < 0.05), and group D1 was significantly higher than in the other four groups. In addition, ammonia nitrogen increased with the increase of density, and there were significant differences among the five density groups (P < 0.05). With the increase in density, the pH values gradually decreased, and there was no significant difference between D3 and D4 groups (P > 0.05). Figure 1 shows the survival rate after transportation. Only D1 and D3 had a survival rate of more than 90%, and the survival rate of group D1 was 100%, which was significantly higher than group D3.
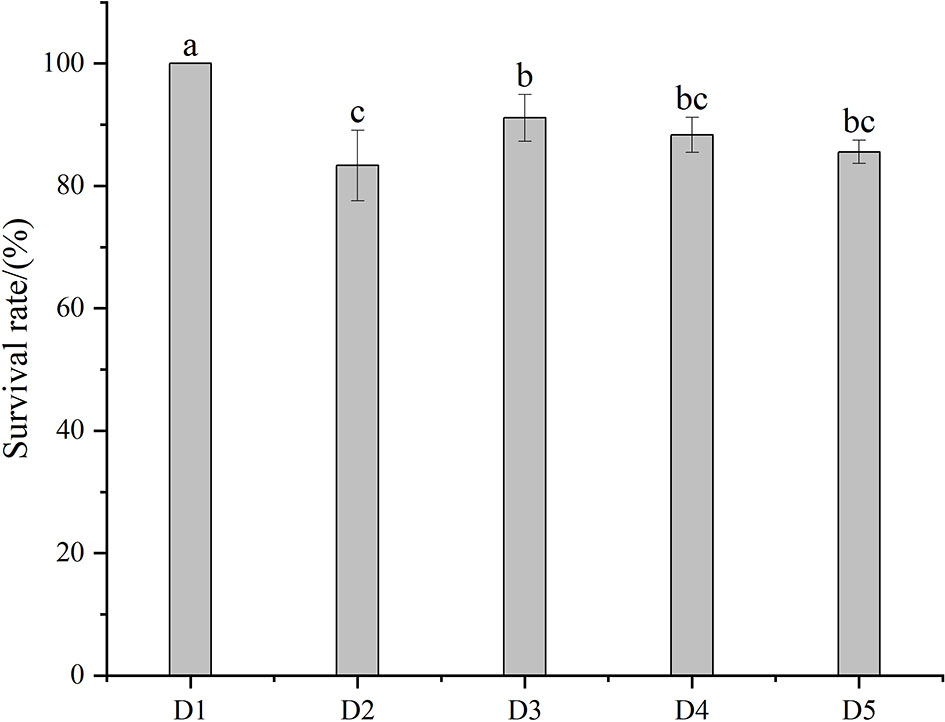
Figure 1 The survival rate after transportation at five different densities. Different letters above bars indicate significant differences at the 0.05 level.
Stress Indexes After Transportation
Figure 2 shows the concentration of ACTH, CRH, and COR and the related expression level of HSP70 after simulated transportation for 8 hours at different densities. The concentration of ACTH in D3 group was higher than in the other four groups. Except for D3 group, there was no significant difference among the other groups (P > 0.05). There was no significant difference in COR concentration among the five groups (P > 0.05). The concentration of CRH was the highest in group D5. ACTH, CRH and COR decreased first and then increased with the increase of density. In the same density group, ACTH concentration was the highest, followed by COR, and CRH concentration was the lowest. HSP70 changed stably, but there were significant differences between adjacent groups and fluctuated within the normal range.
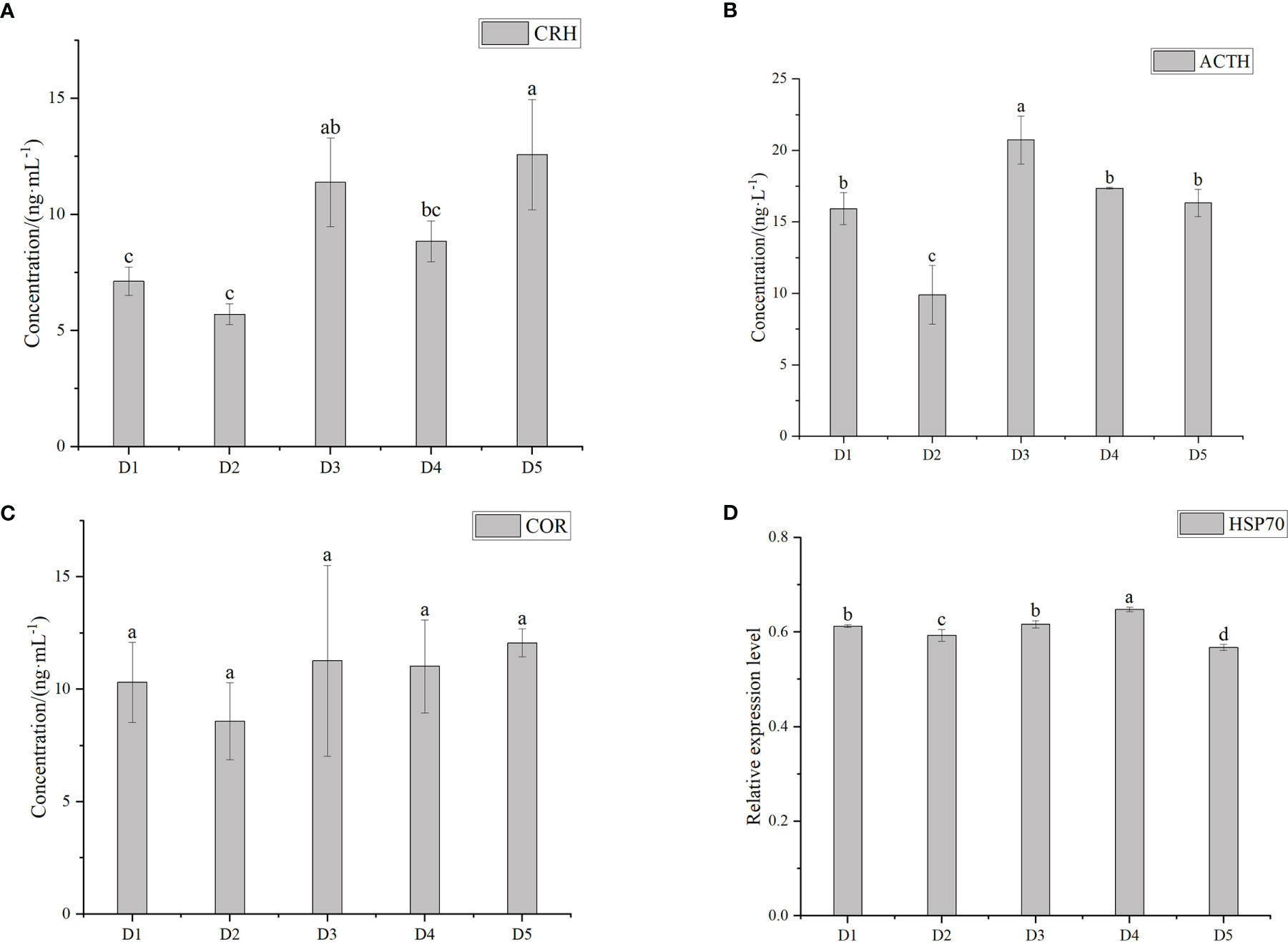
Figure 2 The concentration of corticotropin releasing hormone (CRH) (A), adrenocorticotrophic hormone (ACTH) (B), cortisol (COR) (C) and the relative expression level of heat shock protein 70 (HSP70) (D) after transported at five different densities. Different letters above bars indicate significant differences at the 0.05 level.
Metabolic Indicators After Transportation
Figure 3 shows the related expression levels of FAS, PK and CPT1, glycogen concentrations at different transport densities. After sealed transportation of plastic bags, PK and CPT1 of group D3 reached the lowest and highest values, respectively. It can be seen that the changing trend of CPT1 and PK was opposite and closely related. The relative expression level of FAS in D2 group was the highest and significantly higher than the other four groups (P < 0.05). The glycogen concentration in group D1 was the highest, which was significantly higher than the other four groups (P < 0.05). There was no significant difference between adjacent groups in D2, D3 and D4 groups (P > 0.05).
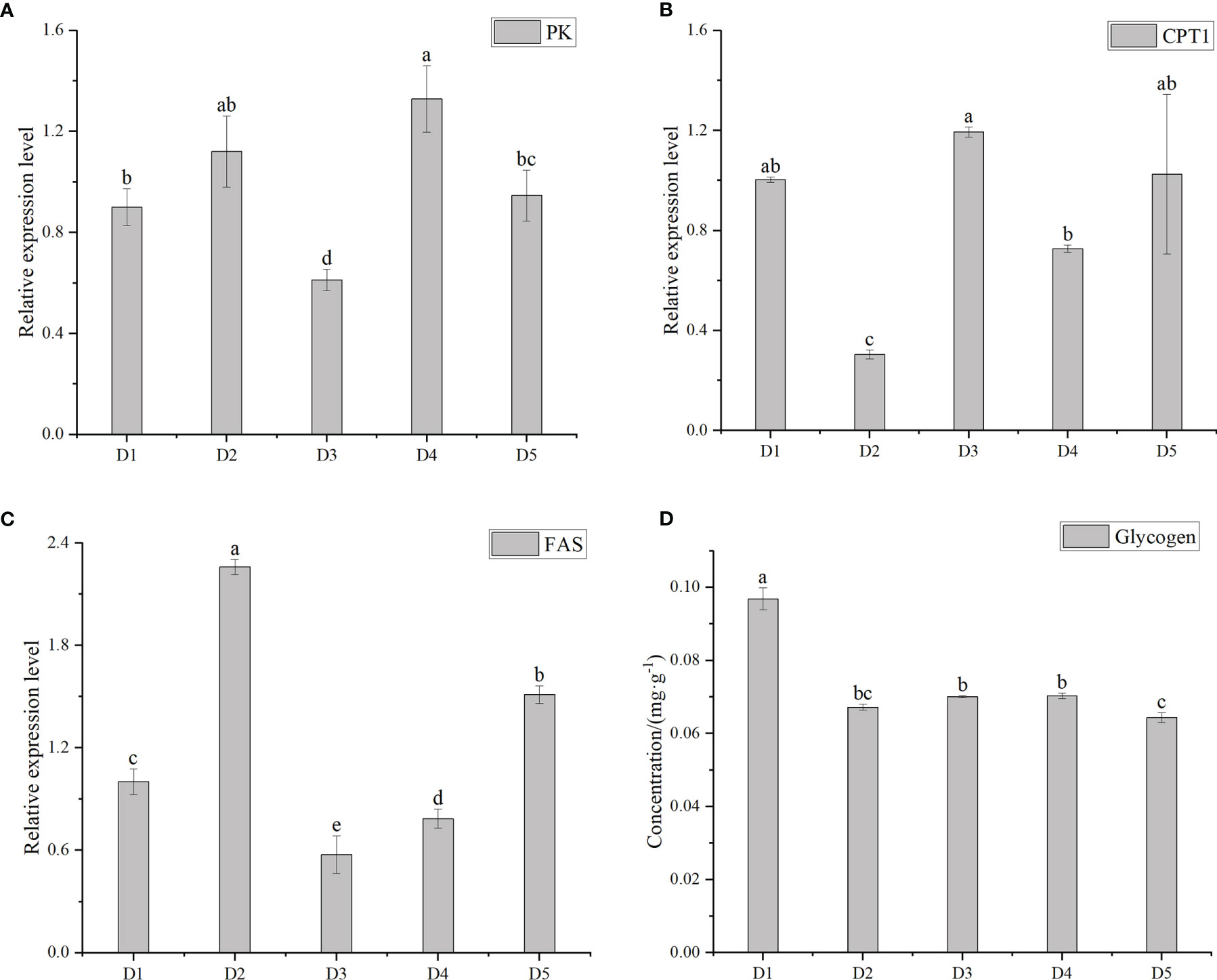
Figure 3 The relative expression level of pyruvate kinase (PK) (A), carnitine palmitoyltransterase-1(CPT1) (B) and fatty acid synthase (FAS) (C) and the concentration of glycogen (D) of greater amberjack after transportation under different durations. Different letters above bars indicate significant differences at the 0.05 level.
Antioxidant Indicators After Transportation
Figure 4 shows the relative expression levels of MDA, CAT, GSH-PX, HO-1, Cu-SOD and Mn-SOD under different transport densities. There were significant differences in CAT among D1, D2, D3 and D4 groups (P < 0.05), but there was no significant difference between D4 and D5 groups (P > 0.05). There were significant differences in GSH-PX between D2, D3, D4 and D5 groups (P < 0.05), but there was no significant difference in GSH-PX between D1 and D2 groups (P > 0.05). There were significant differences in Cu-SOD among the five groups (P < 0.05). In general, CAT, GSH-PX, and Cu-SOD increased first and then decreased with the increase of density. There were significant differences in Mn-SOD between adjacent groups (P < 0.05). The relative expression of HO-1 in D5 density group was significantly higher than in the other four groups, and there was no significant difference between the adjacent groups of the other four groups (P > 0.05). With the increase in density, the relative expression level of HO-1 increased first and then decreased. The concentration of MDA in D2 group was the lowest and D1 group reached the highest, which was significantly higher than in the other four groups. There was no significant difference among the other four groups (P > 0.05).
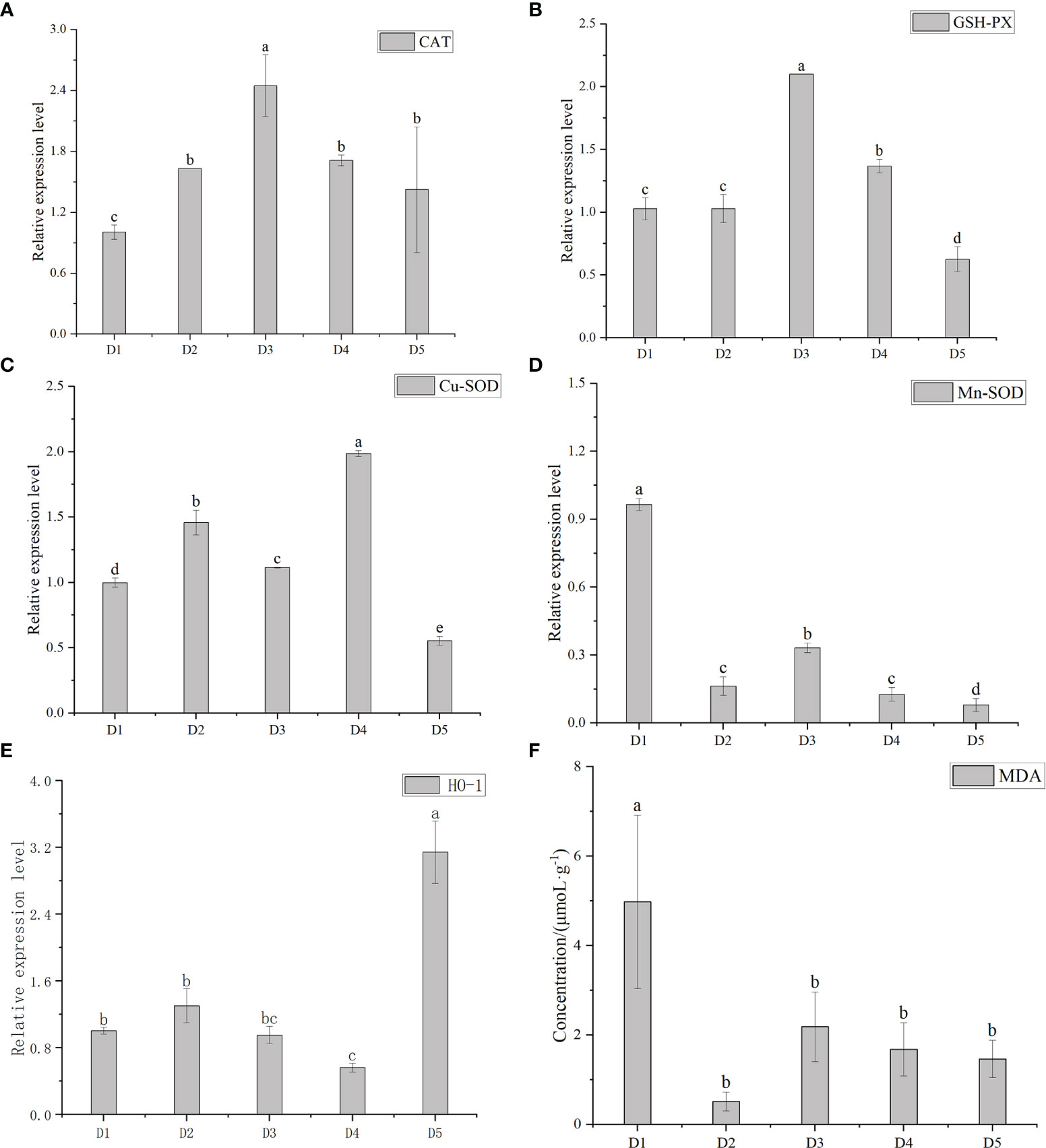
Figure 4 The relative expression of catalase (CAT) (A), glutathione peroxidase (GSH-PX) (B), Cu-Superoxide Dismutase (Cu-SOD) (C), Mn-Superoxide Dismutase (Mn-SOD) (D) and heme oxygenase- 1 (HO- 1) (E) and concentration of malondialdehyde (MDA) (F) of greater amberjack after transportation under different durations. Different letters above bars indicate significant differences at the 0.05 level.
Immune Indicators After Transportation
Figure 5 shows the relative expression of immune-related genes at different transport densities. In terms of cytokines, IL-1βand TNF-α of D3 density group were the lowest. Overall, IL-1β increased with the increase of density, and TNF-α decreased first and then increased. There was no significant difference in the expression level of TNF-α in groups D1, D2, D3, and D4 (P > 0.05). The relative expression of TGF- β1 was no significant difference between the D3 and D4 groups (P > 0.05), and it was significantly lower than in the other three groups. The complement 3(C3) in group D4 was the lowest and significantly lower than in the other four groups (P < 0.05). It reached the highest value in group D5, which was significantly higher than in the other four groups. In terms of immune function, the relative expression of MHC-1 was significantly higher in group D4 than in the other four groups (P < 0.05), and the expression level in D3 density group was significantly lower than in the other four groups. There were significant differences between the two adjacent groups (P < 0.05). The expression level of IgT in D4 density group was the lowest, and there was no significant difference between D4 density group and D1 and D3 groups (P > 0.05). In terms of nuclear transcription factors, the relative expression of NF-KB1 in D3 density group was significantly lower than in the other groups (P < 0.05), and there were significant differences between the two adjacent groups (P < 0.05).
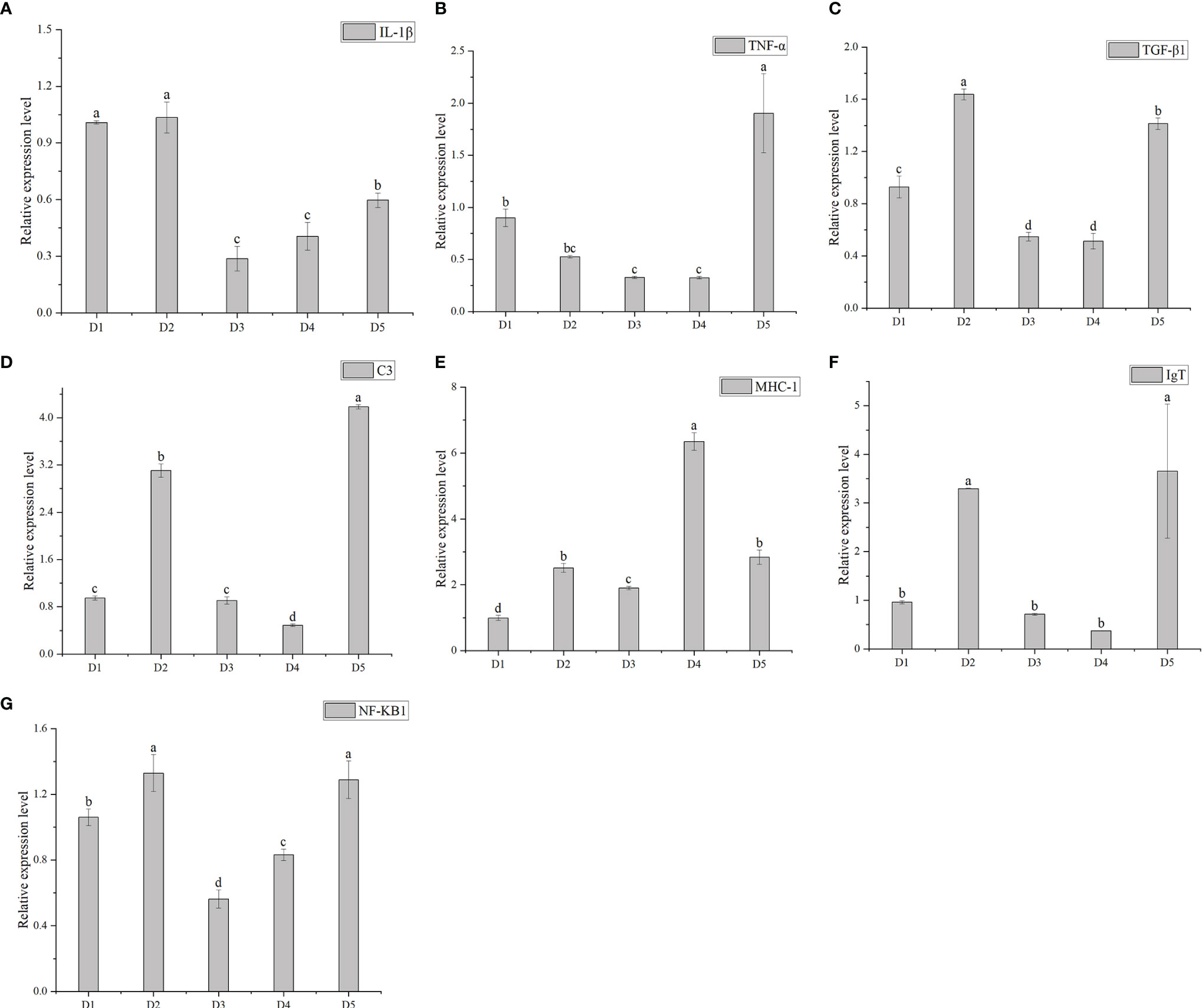
Figure 5 The relative expression of interleukin- 1β (IL- 1β) (A), tumor necrosis factor (TNF-α) (B) and transforming growth factor-β (TGF- β1) (C), complements (C3) (D), major histocompatibility complex (MHC- 1) (E) and immune globulin T (IgT) (F) and nuclearfactor kappa-B (NF-KB1) (G) genes of greater amberjack after transportation under different densities. Different letters above bars indicate significant differences at the 0.05 level.
Discussion
Effects of Density Stress on Water Quality and Survival Rate
With increased density, pH decreases and the concentration of ammonia nitrogen (NH4+-N) increases (Hong et al., 2019). This density-dependent decrease in pH is attributed to the production of carbon dioxide by the fish, which becomes carbonic acid upon contact with water, thereby making the water more acidic. From the results of this study, the concentration of ammonia nitrogen increased with the increase of density. Except D2, the overall trend is that the survival rate decreases with the increase of density.D2 and D3 are the increase of metabolism and respiration caused by the increase of density, and the increase of CO2 leads to the increase of temperature. Studies have shown that excessive density can lead to stress responses, which cause secondary metabolism, including elevated glucose and lactic acid, leading to tertiary reactions, such as decreased metabolism and reduced swimming performance (Thorarensen and Farrell, 2011). This shows that too high density will reduce fish metabolism, resulting in lower temperature. The dissolved oxygen in D4 and D5 density groups was significantly higher than that in D3 group, indicating that the metabolism decreased and the temperature decreased.
Effects of Transport Density on Stress Indexes of Whole Fish
When the fish is under pressure, the hypothalamus-pituitary-interrenal axis (HPI) senses and produces CRH to promote the secretion of ACTH and then promotes the release of COR (Toa et al., 2004; Hong et al., 2019; Lai et al., 2021; Klug et al., 2021; Upadhyay et al., 2021). COR is an important indicator of stress, which increases with the increase of stress. (Oyoo-Okoth et al., 2011). The main functions of this hormone are to raise blood sugar, act as an anti-inflammatory, inhibit the immune system’s response to foreign attacks, and increase blood pressure, among others. In our study, there was no significant difference in COR content between groups. This was different from the research result of Oyoo-Okoth. It showed that the stress produced by the five densities on the greater amberjack is similar. However, the five groups of ACTH and CRH were not as same as COR. This may be due to the different types of fish, and also depends on the length of time and the type of stress source (Braun et al., 2010).
HSP70 is a heat shock protein. It is responsible for correct protein folding, protecting cells from stressors, and presenting immune and inflammatory cytokines (Chen et al., 2019; Lubkowska et al., 2021). After 8 hours of simulated transportation, the expression level of HSP70 gene in the whole fish tissue of greater amberjack was significantly different between adjacent groups, but in a stable state. Previous studies have shown that the expression level of HSP70 in rainbow trout(Oncorhynchus mykiss) and zebrafish (Danio rerio) is gradually up-regulated under density pressure (Ramsay et al., 2006; Yarahmadi et al., 2016). In our study, the changes of HSP70 and COR in the five density groups were relatively stable, which was different from previous studies. The difference in HSP70 expression levels in different studies may be caused by species difference, stress source type or stress time. The different results under stress conditions may be related to the species, physiological response, transportation duration, stress degree and transportation conditions of fish (Barton et al., 2003; Refaey and Li, 2018), which can explain the experimental results. In this study, stress indicators were stable.
Effects of Transport Density on Metabolism Indexes of Whole Fish
After the simulated transport, the concentration of glycogen in D2 density group decreased suddenly, in each group, the glycogen reduction in D2 group was the most significant. This means that the D2 density group has begun to consume energy. The energy consumption of D5 group was the largest, and D3 and D4 groups were significantly higher than D5 group. In general, D5 group had the lowest glycolysis and the highest energy consumption. The results of this study show that the transport density exceeding 1.125kg/m3 will significantly reduce the glycogen concentration of greater amberjack and accelerate metabolism. Other authors have previously reported similar results, with increased energy consumption and decreased systemic lipid and glycogen content in fish at high density (Ibarz et al., 2005; Ibarz et al., 2007; Diogenes et al., 2018).
CPT1, FAS and PK are enzymes that regulate metabolism. CPT1 is the rate- limiting enzyme of fatty acid oxidation, which can maintain the balance of blood sugar and energy supply when the body or tissues are energy-deficient (Liu et al., 2020). FAS is a key enzyme in fat synthesis. It promotes de novo synthesis of fatty acids by catalyzing the synthesis of fatty acids by acetyl-CoA and malonyl-CoA (Liu et al., 2021). PK is a key enzyme in the glycolysis pathway that catalyzes the conversion of phosphoenolpyruvate to enol pyruvic acid and the production of ATP. The stress response can cause the formation of fatty liver (Long et al., 2021). After fish were stressed, the expression of CPT1 decreased, leading to lipid deposition and increasing the expression of FAS (Liu et al., 2020; Yu et al., 2020). In our study, the D3 group had the highest level of CPT1 expression and the lowest FAS, and the opposite was true in the D2 group. It may be due to the increased temperature of the D2 group and the rapid metabolism, so it leads to lipid deposition. The D4 and D5 groups were affected by density and the metabolism was slower than in the D2 group, so the FAS was higher than the D3 group and lower than the D2 group. From this, it can be concluded that the probability of lipid deposition in the D3 group is the smallest.
Studies have shown that PK activity increases with the increase of density (Wang et al., 2022). After simulating transportation at different densities, the PK of D4 group reached the highest level, which indicates that organisms in D4 density group need a lot of energy to resist environmental changes and more ATP was needed for metabolism. The D5 density group was significantly lower than the D4 density group, which was inconsistent with previous studies, possibly due to lower metabolism and less ATP required in the D5 group. The lowest PK expression level in the D3 group may be due to the highest CPT1 expression level, the ability to maintain energy balance is better, and more ATP is not needed to maintain metabolism. In summary, D3 is the most suitable shipping density.
Effects of Transport Density on Antioxidant Indexes of Whole Fish
Excessive production of reactive oxygen species will cause environmental pressure (Lesser, 2006). CAT, SOD and GSH-PX can prevent free radicals from damaging fish (Ribeiro et al., 2015; Piao et al., 2019; Chu et al., 2020; Chu et al., 2020), after fish are stressed, these antioxidant indexes will change significantly (Sahin et al., 2013; Song et al., 2019; Long et al., 2021). Firstly, SOD catalyzing the superoxide (O2-) radical into oxygen (O2) or hydrogen peroxide (H2O2), and then CAT converts the generated hydrogen peroxide to water and oxygen (Hong et al., 2019). In this study, the expression levels of CAT and GSH-PX reached the highest level in D3 group. Due to the weak metabolism in D4 and D5 groups, the production of reactive oxygen species and antioxidant index decreased, so it showed a downward trend. The expression level of Cu-SOD reached the highest in D4 group. Mn-SOD decreased with the increase of density, and was lower than Cu-SOD. The results of SOD showed that the change of density caused a significant response of the first defense against oxidative toxicity. However, Mn-SOD did not increase. This may be because Cu-SOD is sufficient to treat superoxide free radicals, and Mn-SOD plays a complementary role with the increase of density. Then CAT carried out H2O2 transformation and reached the highest value in D3 group. Meanwhile, the expression level of GSH-PX was the highest in D3. MDA is a marker of oxidative stress and a product of lipid peroxidation. High levels of MDA can damage body functions and destroy cell structures (Zhang et al., 2009). In this study, the relative expression levels of most antioxidant enzymes in D3 density group were the highest, while MDA was stable in the high density group. The results show that D3 density was the most suitable density for transportation.
HO-1 is the rate limiting enzyme of heme catabolism. HO-1 and its metabolites have anti-inflammatory, anti-proliferative, antioxidant and anti-apoptotic effects in the process of maintaining intracellular homeostasis (Lu et al., 2022). Studies have shown that a small amount of H2O2 can promote the slight or strong increase of HO-1, while higher H2O2 concentration can significantly reduce the expression of HO-1 (Jia et al., 2019). In this study, among the first four groups, the expression level in D2 group was the highest, then gradually decreased, and suddenly increased in D5 density group. It may be that H2O2 was largely decomposed by antioxidant enzymes in D3 and D4 density groups, and HO-1 only plays an auxiliary role. In D5 density group, due to decreased metabolism and H2O2 concentration, HO-1 was strongly increased. Through the result analysis, the relative expression level of most antioxidant enzymes in D3 density group was higher, indicating that D3 density group had stronger antioxidant capacity.
Effects of Transport Density on Immune Indexes of Whole Fish
Excessive inflammation can lead to cell damage, liver and kidney failure, and eventually death. IL-1β, TNF-α and TGF-β1 are important cytokines and a key mediator of inflammatory response. IL-1β and TNF-α are key pro-inflammatory cytokines, the anti-inflammatory cytokines such as TGF-β1 are responsible for inhibiting the inflammatory response and restoring the body to a normal state (Bemeur et al., 2010). Studies have shown that oxidative stress can promote nonspecific immunity and cause the upregulation of pro-inflammatory cytokine gene expression. When the stressor is short-term, the innate immune response of fish is stimulated (Lin et al., 2018; Hoseini et al., 2019). In this study, in D3 group, the expression level of IL-1β and TNF-α reached the lowest. It indicated that D3 density group had less possibility of inflammation. TGF-β1 the anti-inflammatory effect was the lowest in D4 density group, and there was no significant difference between D3 and D4, indicated that the nonspecific immune function of D3 density group was the weakest in D4 density group. All three cytokines evaluated in this study were lower in D3 group. GSH-PX and CAT in D3 group reached the peak. In contrast, IL-1β and TNF- α both reached the minimum value in D3 group. These results suggested that GSH-PX, CAT and other factors protect cells from reactive oxygen species can reduce inflammatory response, and metabolic changes can affect a series of nonspecific immune-related responses. D3 density was the most appropriate transport density.
C3 is the core component of complement activation, immune defense and immune regulation, which contributes to sterilization, conditioning and immunoadsorption (Wu et al., 2022). According to previous studies, COR produced during stress can inhibit immune function (Padgett and Glaser, 2003). In this study, COR inhibited the expression of these immune related genes due to mild stress in the first few groups, but in D5 group, the concentration of C3 was the highest and could no longer be inhibited. Because the COR concentration of D2 density group is the lowest and the inhibition ability was weak, the expression level of C3 was higher in D2 group. This was consistent with previous study. Its expression level was the lowest in D4 group, indicating that there were fewer bacteria in D4 density group.
IgT plays a key role in protecting the mucosal part of fish from pathogens (Han et al., 2021). In the case of bacterial invasion, the relative expression level of IgT will increase (Buchmann, 2020). MHC-1 participates in antigen recognition, binds with antigens, forms antigen-antibody conjugates, and is eventually phagocytized by phagocytes. MHC-1 belongs to specific immunity and belongs to the second line of defense (Long et al., 2019; Kitiyodom et al., 2021). The expression level of IgT in D4 group was the lowest, and there was no significant difference between D3 and D4 group, indicated that D3 group had the lowest degree of cell damage. In D3 density group, MHC-1 was significantly lower than that in the adjacent two groups, indicated that the antigen content in D3 density group was less. Group D4 was the highest, it is likely that the low expression level of immune factors and complement in D4 group and the increase of pathogens, bacteria and other microorganisms triggered specific immunity. NF-KB1 is a nuclear transcription factor that produces inflammatory cytokines after activation. When fish are inflamed, the expression level of NF-KB1 will increase. The relative expression level of NF-KB1 in D3 group was significantly lower than that in other groups, while other groups showed up-regulation of NF-KB1 gene. Our results showed that D3 group had the lowest cytokine release rate and the weakest inflammatory response. In conclusion, D3 density was the most suitable transportation density for greater amberjack.
Conclusion
Our study evaluated the effects of transport density on great amberjack stress, metabolism, antioxidant capacity, and immunity. Improper transportation density will destroy the water quality, increase ammonia nitrogen concentration, and reduce the survival rate. Fish are prone to stress response, affect metabolism, change antioxidant indexes and produce nonspecific immunity. Upon simulating transportation for 8 h at different densities, our findings indicated that transport density should not exceed 3.375 kg/m3.
Data Availability Statement
The original contributions presented in the study are included in the article/supplementary material. Further inquiries can be directed to the corresponding authors.
Ethics Statement
The animal study was reviewed and approved by Ethical Committee for Animal Experiments of South China Sea Fisheries Research Institute, Chinese Academy of Fishery Sciences, China.
Author Contributions
GY and ZLF: conceptualization. HL and ZYF: experimental operation. HL and ZYF: field sampling. HL and ZYF: sample determination. HL: writing – original draft preparation. ZM and ZYF: writing – review and editing. All authors read and approved the final manuscript.
Funding
This study was financially supported by Central Public-interest Scientific Institution Basal Research Fund, CAFS (2020TD55); Central Public-Interest Scientific Institution Basal Research Fund South China Sea Fisheries Research Institute, CAFS (2021SD09).
Conflict of Interest
The authors declare that the research was conducted in the absence of any commercial or financial relationships that could be construed as a potential conflict of interest.
Publisher’s Note
All claims expressed in this article are solely those of the authors and do not necessarily represent those of their affiliated organizations, or those of the publisher, the editors and the reviewers. Any product that may be evaluated in this article, or claim that may be made by its manufacturer, is not guaranteed or endorsed by the publisher.
References
Adineh H., Naderi M., Hamidi M. K., Harsij M. (2020). Biofloc Technology Improves Growth, Innate Immune Responses, Oxidative Status, and Resistance to Acute Stress in Common Carp (Cyprinus Carpio) Under High Stocking Density. Fish. Shellfish Immunol. 95, 440–448. doi: 10.1016/j.fsi.2019.10.057
Barton B. A., Haukenes A. H., Parsons B. G., Reed J. R. (2003). Plasma Cortisol and Chloride Stress Responses in Juvenile Walleyes During Capture, Transport, and Stocking Procedures. North Am. J. Aquaculture 65 (3), 210–219. doi: 10.1577/C02-030
Bemeur C., Qu H., Desjardins P., Butterworth R. F. (2010). IL-1 or TNF Receptor Gene Deletion Delays Onset of Encephalopathy and Attenuates Brain Edema in Experimental Acute Liver Failure. Neurochem. Int. 56 (2), 213–215. doi: 10.1016/j.neuint.2009.11.010
Braun N., de Lima R. L., Baldisserotto B., Dafre A. L., Nuner A. P. D. O. (2010). Growth, Biochemical and Physiological Responses of Salminus Brasiliensis With Different Stocking Densities and Handling. Aquaculture 301 (1-4), 22–30. doi: 10.1016/j.aquaculture.2010.01.022
Buchmann K. (2020). Immune Response To Ichthyophthirius Multifiliis and Role Of IgT. Parasite Immunol. 42 (8), e12675. doi: 10.1111/pim.12675
Chen W., Feng P. M., Liu T., Jin D. C. (2019). Recent Advances in Machine Learning Methods for Predicting Heat Shock Proteins. Curr. Drug Metab. 20 (3), 224–228. doi: 10.2174/1389200219666181031105916
Chu Y., Lan R. S., Huang R., Feng H., Kumar R., Dayal S., et al. (2020). Glutathione Peroxidase-1 Overexpression Reduces Oxidative Stress, and Improves Pathology and Proteome Remodeling in the Kidneys of Old Mice. Aging Cell 19 (6), e13154. doi: 10.1111/acel.13154
Cupp A. R., Hartleb C. F., Fredricks K. T., Gaikowski M. P. (2016). Effectiveness of Eugenol Sedation to Reduce the Metabolic Rates of Cool and Warm Water Fish at High Loading Densities. Aquaculture Res. 47 (1), 234–242. doi: 10.1111/are.12485
Deane E. E., Zhou L. R., Woo N. Y. S. (2006). Cortisol can be Pro- or Anti-Apoptotic in Sea Bream Cells: Potential Role Of HSP70 Induction for Cytoprotection. Mol. And Cell. Endocrinol. 259 (1-2), 57–64. doi: 10.1016/j.mce.2006.08.006
Diogenes A. F., Teixeira C., Almeida E., Skrzynska A., Costas B., Oliva-Teles A., et al. (2018). Effects of Dietary Tryptophan and Chronic Stress in Gilthead Seabream (Sparus Aurata) Juveniles Fed Corn Distillers Dried Grains With Solubles (DDGS) Based Diets. Aquaculture 498, 396–404. doi: 10.1016/j.aquaculture.2018.08.079
Gamberoni P., Yufera M., de las Heras V., Siguero I., Gilannejad N., Martinez-Rodriguez G., et al. (2021). Ontogeny and Diurnal Patterns of Molecular Gene Expression and Activity of Digestive Enzymes in Developing Greater Amberjack. Aquaculture 534, 736330. doi: 10.1016/j.aquaculture.2020.736330
Ghisleni G., Capiotti K. M., Bonan C. D. (2012). The Role of CRH in Behavioral Responses to Acute Restraint Stress in Zebrafish. Prog. Neuro-Psychopharmacology&Biological Psychiatry 36 (1), 176–182. doi: 10.1016/j.pnpbp.2011.08.016
Han Q., Hu Y. T., Lu Z. J., Wang J. L., Chen H. P., Mo Z. Q., et al. (2021). Study on the Characterization of Grouper (Epinephelus Coioides) Immunoglobulin T and its Positive Cells. Fish Shellfish Immunol. 118, 102–110. doi: 10.1016/j.fsi.2021.08.031
Hong J. W., Chen X., Liu S. X., Fu Z. Y., Han M. Y., Wang Y. F. (2019). Impact of Fish Density on Water Quality and Physiological Response of Golden Pompano (Trachinotus Ovatus) Flingerlings During Transportation. Aquaculture 507, 260–265. doi: 10.1016/j.aquaculture.2019.04.040
Hong J. W., Zhou S. J., Yu G., Qin C. X., Zuo T., Ma Z. H. (2021). Effects of Transporting Stress on the Immune Responses of Asian Seabass Lates Calcarifer Fry. Aquaculture Res. 52 (5), 2182–2193. doi: 10.1111/are.15071
Hoseini S. M., Yousefi M., Hoseinifar S. H., Van Doan H. (2019). Cytokines' Gene Expression, Humoral Immune and Biochemical Responses of Common Carp (Cyprinus Carpio, Linnaeus 1758) to Transportation Density and Recovery in Brackish Water. Aquaculture 504, 13–21. doi: 10.1016/j.aquaculture.2019.01.049
Hou Z. S., Wen H. S., Li J. F., He F., Li Y., Qi X. (2020). Effects of Long-Term Crowding Stress on Neuro-Endocrine-Immune Network Of Rainbow Trout (Oncorhynchus Mykiss). Fish &Shellfish Immunol. 95, 180–189. doi: 10.1016/j.fsi.2019.10.011
Hur J. W., Park I. S., Chang Y. J. (2007). Physiological Responses of the Olive Flounder, Paralichthys Olivaceus, to a Series Stress During the Transportation Process. Ichthyological Res. 54 (1), 32–37. doi: 10.1007/s10228-006-0370-2
Ibarz A., Blasco J., Beltran M., Gallardo M. A., Sanchez J., Sala R., et al. (2005). Cold-Induced Alterations on Proximate Composition and Fatty Acid Profiles of Several Tissues in Gilthead Sea Bream (Sparus Aurata). Aquaculture 249 (1-4), 477–486. doi: 10.1016/j.aquaculture.2005.02.056
Ibarz A., Blasco J., Sala-Rabanal M., Gallardo A., Redondo A. (2007). Metabolic Rate and Tissue Reserves in Gilthead Sea Bream (Sparus Aurata) Under Thermal Fluctuations and Fasting and Their Capacity for Recovery. Can. J. Fish. Aquat. Sci. 64 (7), 1034–1042. doi: 10.1139/f07-079
Jia R., Du J. L., Cao L. P., Li Y., Johnson O., Gu Z. Y., et al. (2019). Antioxidative, Inflammatory and Immune Responses in Hydrogen Peroxide-Induced Liver Injury of Tilapia (GIFT, Oreochromis Niloticus). Fish Shellfish Immunol. 84, 894–905. doi: 10.1016/j.fsi.2018.10.084
Kitiyodom S., Trullas C., Rodkhum C., Thompson K. D., Katagiri T., Temisak S., et al. (2021). Modulation of the Mucosal Immune Response of Red Tilapia (Oreochromis Sp.) Against Columnaris Disease Using a Biomimetic-Mucoadhesive Nanovaccine. Fish Shellfish Immunol. 112, 81–91. doi: 10.1016/j.fsi.2021.02.017
Klug J. J., Treuting P. M., Sanders G. E., Winton J. R., Kurath G. (2021). Effects of Stocking Density on Stress Response and Susceptibility to Infectious Hematopoietic Necrosis Virus in Rainbow Trout. J. Am. Assoc. Lab. Anim. Sci. 60 (6), 637–645. doi: 10.30802/AALAS-JAALAS-21-000003
Kurtoglu I. Z., Ak K., Genc S. (2021). Effects of Stocking Density During Live Transportation on Haematological Parameters of Siberian Sturgeon (Acipenser Baerii, Brandt 1869). J. Appl. Ichthyol 37 (6), 809–815. doi: 10.1111/jai.14266
Lai F., Royan M. R., Gomes A. S., Espe M., Aksnes A., Norberg B., et al. (2021). The Stress Response in Atlantic Salmon (Salmo Salar L.): Identification and Functional Characterization of the Corticotropin-Releasing Factor (Crf) Paralogs. Gen. Comp. Endocrinol. 313, 113894. doi: 10.1016/j.ygcen.2021.113894
Lesser M. P. (2006). Oxidative Stress in Marine Environments: Biochemistry and Physiological Ecology. Annu. Rev. Physiol. 68, 253–278. doi: 10.1146/annurev.physiol.68.040104.110001
Lin W., Li L., Chen J., Li D. P., Hou J., Guo H. H., et al. (2018). Long-Term Crowding Stress Causes Compromised Nonspecific Immunity and Increases Apoptosis of Spleen in Grass Carp (Ctenopharyngodon Idella). Fish Shellfish Immunol. 80, 540–545. doi: 10.1016/j.fsi.2018.06.050
Liu Y. F., Wu Z. X., Zhang J., Liu Y. X., Liu Z. Y., Xie H. K., et al. (2020). Seasonal Variation of Lipid Profile of Oyster Crassostrea Talienwhanensis From the Yellow Sea Area. J. Aquat. Food Prod. Technol. 29 (4), 360–72. doi: 10.1080/10498850.2020.1737998
Liu Z. L., Zhao W., Hu W. S., Zhu B., Xie J. J., Liu Y. J., et al. (2021). Lipid Metabolism,Growth Performance,Antioxidant Ability and Intestinal Morphology of Rainbow Trout (Oncorhynchus Mykiss) Under Cage Culture With Flowing Water Were Affected by Dietary Lipid Levels. Aquaculture Rep. 19, 100593. doi: 10.1016/j.aqrep.2021.100593
Long S. S., Dong X. H., Tan B. P., Zhang S., Xie S. W., Yang Q. H., et al. (2021). Growth Performance, Antioxidant Ability, Biochemical Index in Serum, Liver Histology and Hepatic Metabolomics Analysis of Juvenile Hybrid Grouper (Female Epinephelus Fuscoguttatus X Male Epinephelus Lanceolatus) Fed With Oxidized Fish Oil. Aquaculture 545, 737261. doi: 10.1016/j.aquaculture.2021.737261
Long A., Garver K. A., Jones S. R. M. (2019). Synergistic Osmoregulatory Dysfunction During Salmon Lice (Lepeophtheirus Salmonis) and Infectious Hematopoietic Necrosis Virus Co-Infection In Sockeye Salmon (Oncorhynchus Nerka) Smolts. J. Fish Dis. 42 (6), 869–882. doi: 10.1111/jfd.12989
Lozano-Bilbao E., Adern N., Hardisson A., Gonzalez-Weller D., Rubio C., Paz S., et al. (2021). Differences in Macroelements, Trace Elements and Toxic Metals Between Wild and Captive-Reared Greater Amberjack (Seriola Dumerili) From the Mediterranean Sea. Mar. Pollut. Bull. 170, 112637. doi: 10.1016/j.marpolbul.2021.112637
Lu Z. J., Zhang M. L., Tang M. Z., Li Y. A., Shi F., Zhan F. B., et al. (2022). Heme Oxygenase-1 Protects Against Inflammatory and Apoptosis Induced by Hemeproteins in Ctenopharyngodon Idellus Kidney Cells. Aquaculture 546, 737266. doi: 10.1016/j.aquaculture.2021.737266
Lubkowska A., Pluta W., Stronska A., Lalko A. (2021). Role of Heat Shock Proteins (HSP70 and HSP90) in Viral Infection. Int. J. Mol. Sci. 22 (17), 9366. doi: 10.3390/ijms22179366
Oyoo-Okoth E., Cherop L., Ngugi C. C., Chepkirui-Boit V., Manguya-Lusega D., Ani-Sabwa J., et al. (2011). Survival and Physiological Response of Labeo Victorianus (Pisces: Cyprinidae, Boulenger 1901) Juveniles to Transport Stress Under a Salinity Gradient. Aquaculture 319 (1-2), 225–231. doi: 10.1016/j.aquaculture.2011.06.052
Padgett D. A., Glaser R. (2003). How Stress Influences the Immune Response. Trends Immunol. 24 (8), 444–448. doi: 10.1016/S1471-4906(03)00173-X
Peng Y. H., Shi H. J., Liu Y. Q., Huang Y., Zheng R. C., Jiang D. N., et al. (2022). RNA Sequencing Analysis Reveals Divergent Adaptive Response to Hypo- and Hyper-Salinity in Greater Amberjack (Seriola Dumerili) Juveniles. Animals 12 (3), 327–344. doi: 10.3390/ani12030327
Pereira-Cardona P. M., Lisboa V., Barbas L. A. L., Robaldo R. B. (2017). Transport of Juvenile Dusky Grouper Epinephelus Marginatus Under Different Packing Densities: Metabolic and Haematological Responses. Aquaculture Res. 48 (10), 5356–5362. doi: 10.1111/are.13349
Piao X. M., Liu Z. R., Li Y. Y., Yao D. H., Sun L. W., Wang B. H., et al (2019). Investigation of the Effect for Bisphenol a on Oxidative Stress in Human Hepatocytes and Its Interaction With Catalase. Spectrochim Acta A Mol. Biomol. Spectrosc. 221, 117149. doi: 10.1016/j.saa.2019.117149
Ramsay J. M., Feist G. W., Varga Z. M., Westerfield M., Kent M. L., Schreck C. B. (2006). Whole-Body Cortisol is an Indicator of Crowding Stress in Adult Zebrafish, Danio Rerio. Aquaculture 258 (1-4), 565–574. doi: 10.1016/j.aquaculture.2006.04.020
Refaey M. M., Li D. P. (2018). Transport Stress Changes Blood Biochemistry, Antioxidant Defense System, and Hepatic HSPs mRNA Expressions of Channel Catfish Ictalurus Punctatus. Front. Physiol. 9, 1628. doi: 10.3389/fphys.2018.01628
Ribeiro T. P., Fernandes C., Melo K. V., Ferreira S. S., Lessa J. A., Franco R. W. A., et al. (2015). Iron, Copper, and Manganese Complexes With In Vitro Superoxide Dismutase and/or Catalase Activities That Keep Saccharomyces Cerevisiae Cells Alive Under Severe Oxidative Stress. Free Radical Biol. Med. 80, 67–76. doi: 10.1016/j.freeradbiomed.2014.12.005
Rigos G., Katharios P., Kogiannou D., Cascarano C. M. (2020). Infectious Diseases and Treatment Solutions of Farmed Greater Amberjack Seriola Dumerili With Particular Emphasis in Mediterranean Region. Rev. Aquaculture 13 (1), 201–323. doi: 10.1111/raq.12476
Sahin K., Yazlak H., Orhan C., Tuzcu M., Akdemir F., Sahin N. (2013). The Effect of Lycopene on Antioxidant Status in Rainbow Trout (Oncorhynchus Mykiss) Reared Under High Stocking Density. Aquaculture 418, 132–138. doi: 10.1016/j.aquaculture.2013.10.009
Singh R. K., Vartak V. R., Balange A. K., Ghughuskar M. M. (2004). Water Quality Management During Transportation of Fry of Indian Major Carps, Catla Catla (Hamilton), Labeo Rohita (Hamilton) and Cirrhinus Mrigala (Hamilton). Aquaculture 235 (1-4), 297–302. doi: 10.1016/j.aquaculture.2003.12.011
Song C. Y., Liu B., Xu P., Ge X. P., Zhang H. M. (2019). Emodin Ameliorates Metabolic and Antioxidant Capacity Inhibited by Dietary Oxidized Fish Oil Through PPARs and Nrf2-Keap1 Signaling in Wuchang Bream (Megalobrama Amblycephala). Fish Shellfish Immunol. 94, 842–851. doi: 10.1016/j.fsi.2019.10.001
Thorarensen H., Farrell A. P. (2011). The Biological Requirements for Post-Smolt Atlantic Salmon in Closed-Containment Systems. Aquaculture 312 (1-4), 1–14. doi: 10.1016/j.aquaculture.2010.11.043
Toa D. G., Afonso L. O. B., Iwama G. K. (2004). Stress Response Ofjuvenile Rainbow Trout (Oncorhynchus Mykiss) to Chemical Cues Released From Stressed Conspecifics. Fish Physiol. Biochem. 30 (2), 103–108. doi: 10.1007/s10695-005-0266-5
Upadhyay A., Swain H. S., Das B. K., Ramteke M. H., Kumar V., Krishna G., et al. (2021). Stocking Density Matters in Open Water Cage Culture: Influence on Growth, Digestive Enzymes, Haemato-Immuno and Stress Responses of Puntius Sarana (Ham,1822). Aquaculture 547, 737445. doi: 10.1016/j.aquaculture.2021.737445
Wang Q. J., Ju X., Chen Y. K., Dong X. Q., Luo S., Liu H. J., et al. (2017). L-Carnitine Exerts a Cytoprotective Effect Against H2O2-Induced Oxidative Stress in the Fathead Minnow Muscle Cell Line. Aquaculture Res. 48 (3), 941–954. doi: 10.1111/are.12937
Wang Q., Mei J., Cao J., Xie J. (2022). Effects of Melissa Officinalis L. Essential Oil in Comparison With Anaesthetics on Gill Tissue Damage, Liver Metabolism and Immune Parameters in Sea Bass (Lateolabrax Maculatus) During Simulated Live Transport. Biology-Basel 11 (1), 11–27. doi: 10.3390/biology11010011
Wu M., Jia B. B., Li M. F. (2022). Complement C3 and Activated Fragment C3a Are Involved in Complement Activation and Anti-Bacterial Immunity. Front. Immunol. 13, 813173. doi: 10.3389/fimmu.2022.813173
Yarahmadi P., Miandare H.K., Fayaz S., Caipang C.M.A. (2016). Increased Stocking Density Causes Changes in Expression of Selected Stress- and Immune-Related Genes, Humoral Innate Immune Parameters and Stress Responses of Rainbow Trout (Oncorhynchus Mykiss). Fish Shellfish Immunol. 48, 43–53. doi: 10.1016/j.fsi.2015.11.007
Yi-Kuan L. U., Ying L. U., Jing X. I. E. (2020). Research Progress on Detection Methods of Fishing Anesthetics. J. Food Saf. Qual. 11 (11), 3415–3421. doi: 10.20431/2454-7670.0504004
Yuan J. F., Su N. A., Wang M., Xie P., Shi Z. L., Li L. J. (2012). Down-Regulation of Heme Oxygenase-1 by SVCV Infection. Fish &Shellfish Immunol. 32 (2), 301–306. doi: 10.1016/j.fsi.2011.11.019
Yu T., Chen Y. K., Chen X. M., Ghonimy A., Guo Z. X., Zhao Y. L., et al. (2020). The Effect Of Oxidized Fish Oil On Lipid Metabolism In Rhynchocypris Lagowski Dybowski. Aquaculture Rep. 17, 100388. doi: 10.1016/j.aqrep.2020.100388
Zhang H., Mu Z. B., Xu L. M., Xu G. F., Liu M., Shan A. S. (2009). Dietary Lipid Level Induced Antioxidant Response in Manchurian Trout, Brachymystax Lenok (Pallas) Larvae. Lipids 44 (7), 643–654. doi: 10.1007/s11745-009-3313-7
Keywords: metabolism, antioxidant, immune, gene expression, greater amberjack
Citation: Liu H, Fu Z, Yu G, Ma Z and Fu Z (2022) Effect of Transport Density on Greater Amberjack (Seriola dumerili) Stress, Metabolism, Antioxidant Capacity and Immunity. Front. Mar. Sci. 9:931816. doi: 10.3389/fmars.2022.931816
Received: 29 April 2022; Accepted: 01 June 2022;
Published: 28 June 2022.
Edited by:
Neeraj Kumar, National Institute of Abiotic Stress Management (ICAR), IndiaReviewed by:
Soibam Khogen Singh, Central Agricultural University, IndiaMohamed Moaaz Refaey, Mansoura University, Egypt
Vikash Kumar, Central Inland Fisheries Research Institute (ICAR), India
Copyright © 2022 Liu, Fu, Yu, Ma and Fu. This is an open-access article distributed under the terms of the Creative Commons Attribution License (CC BY). The use, distribution or reproduction in other forums is permitted, provided the original author(s) and the copyright owner(s) are credited and that the original publication in this journal is cited, in accordance with accepted academic practice. No use, distribution or reproduction is permitted which does not comply with these terms.
*Correspondence: Zhenhua Ma, emhlbmh1YS5tYUBzY3NmcmkuYWMuY24=; Zhilu Fu, ZnpsMTlAMTYzLmNvbQ==
†These authors have contributed equally to this work